- 1Graduate Program in Ecology of Inland Water Ecosystems (PEA), Department of Biology (DBI), Centre of Biological Sciences (CCB), State University of Maringá (UEM), Maringá, Brazil
- 2Freshwater Centre, Finnish Environment Institute (SYKE), Oulu, Finland
- 3Department of Ecology, Institute of Biological Sciences, Federal University of Goiás, Goiânia, Brazil
- 4Research Centre in Limnology, Ichthyology and Aquaculture (NUPÉLIA), Centre of Biological Sciences (CCB), State University of Maringá (UEM), Maringá, Brazil
- 5Royal Belgian Institute of Natural Sciences (RBINS), Natural Environments, Brussels, Belgium
- 6Department of Biology, University of Ghent, Ghent, Belgium
- 7Graduate Course in Clean Technologies, Cesumar Institute of Science, Technology and Innovation (ICETI), Cesumar University Center - UniCesumar, Maringá, Brazil
An intensively debated issue in ecology is whether the variations in the biodiversity patterns of different biological groups are congruent in space and time. In addition, ecologists have recognized the necessity of accounting for both taxonomic and functional facets when analysing spatial and temporal congruence patterns. This study aimed to determine how the cross-taxon congruence of taxonomic and functional beta diversity varies across space and time, using data from four floodplains at a continental scale. Our general hypothesis was that the congruence between aquatic biological groups, either taxonomic or functional, would decrease with the “between-group” functional distance. Also, we examined how congruence patterns varied across spatial and temporal scales by focusing on how the cross-taxon relationships differ among Brazilian floodplains and between dry/wet periods. Our study comprised information on eight biological groups from the four largest Brazilian river-floodplain systems, and cross-taxon congruence was assessed using Procrustes analysis. Our results show how detailed analyses can reveal different patterns of cross-taxon congruence, and partially support the hypothesis that the strength of cross-taxon congruence is negatively related to between-group functional distance.
Introduction
Biodiversity decline has been attributed to several anthropogenic impacts, including habitat loss and fragmentation, introduction of alien species, and climate change (Cardinale et al., 2012). Thus, a key goal of ecologists is to evaluate how these impacts threaten biodiversity and, ultimately, ecosystem function and human well-being (Cardinale et al., 2012; Brose and Hillebrand, 2016). However, reaching this goal is often hampered by biodiversity knowledge shortfalls (e.g., Whittaker et al., 2005; Hortal et al., 2015). Given the accelerated pace of anthropogenic impacts and the paucity of financial and personnel resources (especially in areas of high biodiversity), ecologists often rely on biodiversity shortcuts by using proxies (Rosser, 2017).
Among the different biodiversity shortcuts, surrogates are often used in biomonitoring and conservation planning research (Rodrigues and Brooks, 2007). The use of surrogates is based on the assumption that diversity patterns of different taxa covary in space, a phenomenon called cross-taxon congruence or community concordance (Lovell et al., 2007; Westgate et al., 2014). Under budget shortage, the congruence-based approach may be useful to identify surrogates that portray fundamental aspects of biodiversity, providing a cost-effective biomonitoring program (Padial et al., 2012). However, the reliability of cross-taxon congruence strongly depends on the strength of the correlation among different groups of organisms (Heino, 2010).
The ecological processes affecting cross-taxon congruence are still poorly understood and, at least, three non-exclusive mechanisms operating at different spatial scales are thought to drive cross-taxon congruence (Lovell et al., 2007). The first one assumes the two biological groups may respond similarly to environmental gradients and temporal environmental variability (Padial et al., 2012). Secondly, congruence may emerge from common biogeographical history, whereby the resident biological groups underwent similar dispersal constraints and/or evolutionary trajectories (Burrascano et al., 2018). The third mechanism involves the role of biotic interactions independently of environmental and spatial factors. In this case, the diversity of a group of organisms can be positively correlated with the diversity of their resources (Vasconcelos et al., 2019) or negatively related with the diversity of predators and competitors (Banos et al., 2020).
The spatial covariation among taxa may also be mediated by trait similarity. For instance, as dispersal abilities are constrained by body size, similarity in this trait can be a predictor of cross-taxon congruence (Velghe and Gregory-Eaves, 2013; Hájek et al., 2014). Besides, dispersal abilities may decrease with body size affecting community composition and likely cross-taxon congruence (De Bie et al., 2012; Lansac-Tôha et al., 2021a). For example, organisms that are passively dispersed by wind, such as algae and ciliates, would drift further than fish, when considering a single hydrological basin (Incagnone et al., 2015). Body size can impose fundamental constraints on other life history traits, such as trophic level, reproductive rate, life span, and metabolic rate (Fenchel, 1974). Then, high congruence among taxa with similar body size may emerge because of the functional similarity among biological groups.
River-floodplains are environmentally heterogeneous systems, which typically harbor a high diversity of species (Diniz et al., 2021). Nevertheless, despite their importance for regulating flood regimes, biomass and nutrient cycling, floodplains as other freshwater ecosystems are understudied. In floodplains the annual hydrological regime is a key factor influencing their structure and functioning and flood pulses are of the utmost importance as they promote biotic and abiotic exchange between the river and its surrounding landscape (Junk et al., 1989). Floods also tend to produce the so-called “homogenization” phenomenon, reducing the spatial variability of biological (beta diversity) and environmental factors (Thomaz et al., 2007). In contrast, during droughts, many aquatic habitats are isolated from each other, and local communities are strongly and differently influenced by local factors such as resource fluctuation, competition and predation, which tend to increase beta diversity (Quirino et al., 2019).
Here we aimed to determine whether the cross-taxon congruence in taxonomic and functional beta diversity varies across space and time, using a comprehensive field dataset that encompasses a continental geographical extent. Our surveys combine information on eight biological groups from floodplain lakes that show high variation in species diversity and functional traits. We test the general hypothesis that the congruence between biological groups will decrease with increasing trait dissimilarity. Moreover, we anticipated that strengths in cross-taxon congruence will depend on temporal dynamics and spatial scale (Vieira et al., 2015; Lários et al., 2017). To accomplish this task, we used a sequential scale-out perspective focusing on the strength of cross-taxon congruence. First, the analyses were carried out for each floodplain and period (dry and wet) separately. Second, the analyses were repeated using spatio-temporal data from each floodplain separately. Third, for each period, we analysed the data from all floodplains. The data from the first strategy are similar (in structure) to those obtained in regional studies (e.g., within a specific floodplain) which were conducted once only. Similarly, the data from the second strategy are akin to those obtained in regional studies whose sampling designs considered the temporal variation. Finally, the last strategy emulates a study whose dataset was obtained once and at a broad spatial scale. We expect to highlight the importance of context dependence in using biological surrogates in ecological assessments.
Materials and Methods
Study Area
We surveyed four Neotropical floodplain systems (Amazônia, Araguaia, Pantanal, and Paraná; Figure 1) over a spatial extent of 2,300 km (3°–23° S). The Amazônia (3°02´ - 3°34′S and 59°38´ - 60°50′W) and the Araguaia (12°49′- 13°25′S and 50°36´ - 50°43′W) floodplains are located in northern and central Brazil, respectively, while the South Matogrossense Pantanal (18°46´ - 19° 34′S and 56°58′- 57°46′W) and the Upper Paraná (22°40´ - 22°54′S and 53º13’ - 53º38′W) floodplains are located south of the study area. In terms of human impacts, there is a gradient ranging from the Amazônia floodplain, which has relatively pristine forests and tributaries, through the Pantanal and Araguaia, with moderate to intense human activities, to the Paraná floodplain, which is located in the most economically developed region of Brazil (Rocha et al., 2017).
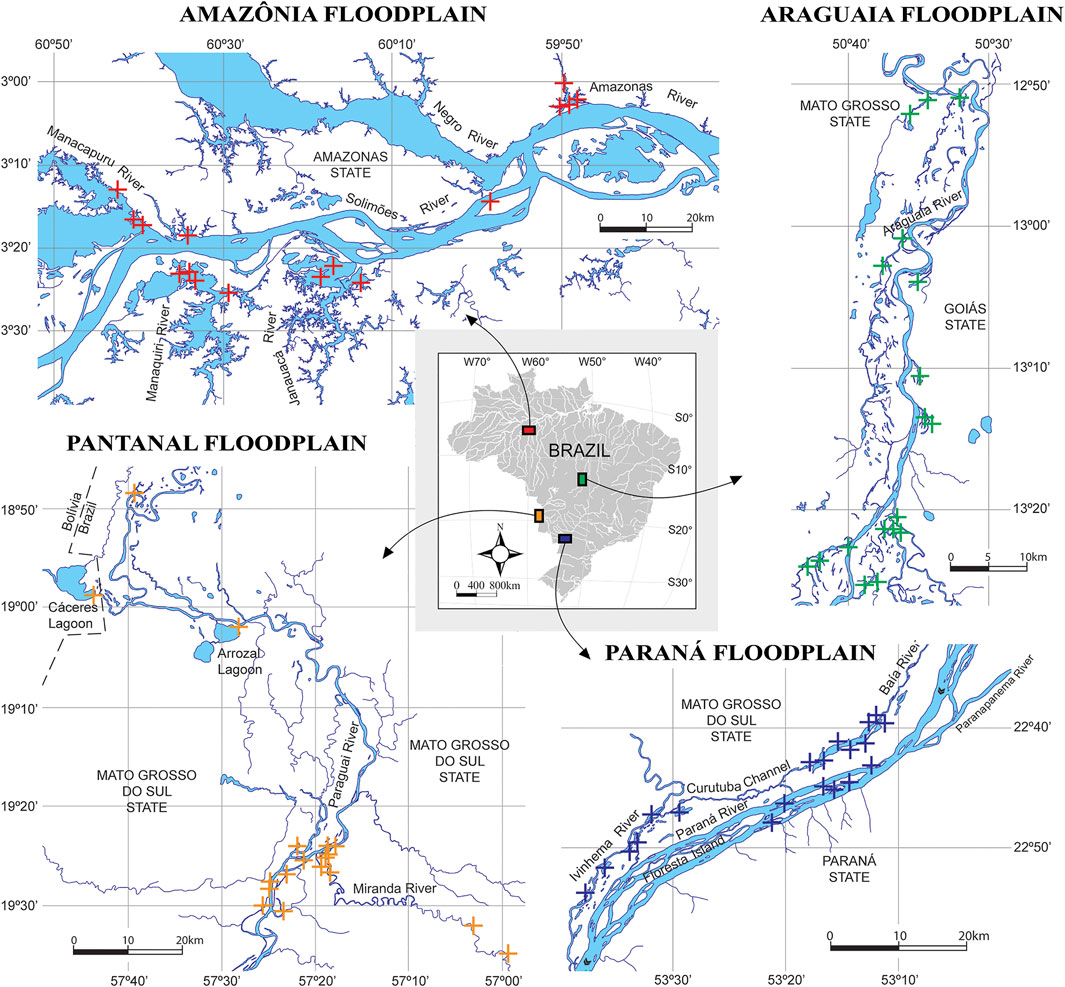
FIGURE 1. Map of the study area showing the surveyed freshwater lakes from the Amazônia (red symbols), Araguaia (green symbols), Pantanal (orange symbols), and Paraná (blue symbols) floodplains.
The Amazônia and Araguaia floodplains have an equatorial climate characterized by average temperatures ranging from 25 to 29°C and high average annual precipitation (1,300 to 2,000 mm) (Irion et al., 1997). Conversely, the Pantanal and Paraná floodplains have tropical and subtropical climates, respectively, with comparatively lower average temperatures (between 16 and 28°C) and annual precipitation rates (ca. 1,400 mm) (Stevaux, 1994). These floodplains have very marked and distinct hydrological periods (Stevaux, 1994; Irion et al., 1997; Agostinho et al., 2004), excepting the Paraná River floodplain, which is strongly influenced by an upstream cascade of reservoirs, thus reducing the amplitude and duration of the flood pulses (Agostinho et al., 2004). For more details on these four floodplains and their features, see Lansac-Tôha et al. (2021a, 2021b).
Sampling Periods
Two sampling campaigns were carried out during dry and wet water periods in each of the four floodplains. In the dry period, we sampled 66 lakes connected to the main rivers or their tributaries. Sampling was done in September 2011 in the Paraná floodplain (20 lakes), in October 2011 in Amazônia (16 lakes), in November 2011 in Araguaia (18 lakes), and in March 2012 in the Pantanal floodplain (12 lakes). During the wet period, samples were collected in August 2011 in the Pantanal (18 lakes), in February 2012 in Paraná (20 lakes), in March 2012 in Araguaia (18 lakes), and in May 2012 in Amazônia (15 lakes), totalling 71 lakes in this period (Figure 1).
Biological Communities
We analyzed data from eight biological groups, including phytoplankton, ciliates, testate amoebae, rotifers, planktonic microcrustaceans (cladocerans and copepods), ostracods, fish and macrophytes, in all four floodplains during dry and wet periods. All these groups are commonly used in studies of aquatic communities, distribution patterns, beta diversity and cross-taxon congruence. The sampling methods and laboratory analysis for each group can be found in detail in Lansac-Tôha et al. (2021a). Sampling, counting and identification of species of each biological group were performed by the same team. Sampling permit was issued by the Brazilian Environmental Ministry (Instituto Chico Mendes de Conservação da Biodiversidade - ICMBio, authorization from the System and Information on Biodiversity -SISBIO; protocol #29.652).
Functional Traits
We gathered information about the functional traits of the eight biological groups aforementioned. The literature sources used for functional classification and the relationship between each trait and its corresponding ecosystem function can be found in Supplementary Table S1. To investigate the effect of functional differences on the cross-taxon congruence, we estimated the functional distances among biological groups. For this purpose, we considered biological characteristics that are commonly known to interfere with the distribution patterns of organisms, such as body size (De Bie et al., 2012), cellular organization (uni or multicellular) (Hillebrand et al., 2001), reproductive type (binary fission, parthenogenesis, sexual or mixed) (Browne and MacDonald, 1982), dispersal mode (passive or active) (Lansac-Tôha et al., 2019) and nutrition (heterotrophic or autotrophic) (Barton et al., 2013) (Table 1).
Data Analysis
Before the analysis, we splitted our data in three different ways: the first dataset included separate data for each floodplain (Amazônia, Araguaia, Pantanal, and Paraná) and each period (dry and wet), generating eight subsets; the second dataset also considered each floodplain separately but included both periods, generating four subsets; and the third dataset included all floodplains, but separate periods (two subsets). All data handling and statistical analyses were performed in the R environment (R Core Team, 2021) and each procedure is described below.
First, we computed taxonomic site-by-site dissimilarity matrices for each biological group and subsets by applying the Sørensen index on presence-absence and the Bray-Curtis index on abundance data (except for aquatic macrophytes, for which we collected presence/absence data only) (Peres-Neto and Jackson, 2001; Legendre, 2014). Following Podani and Schmera (2011), the total taxonomic beta diversity
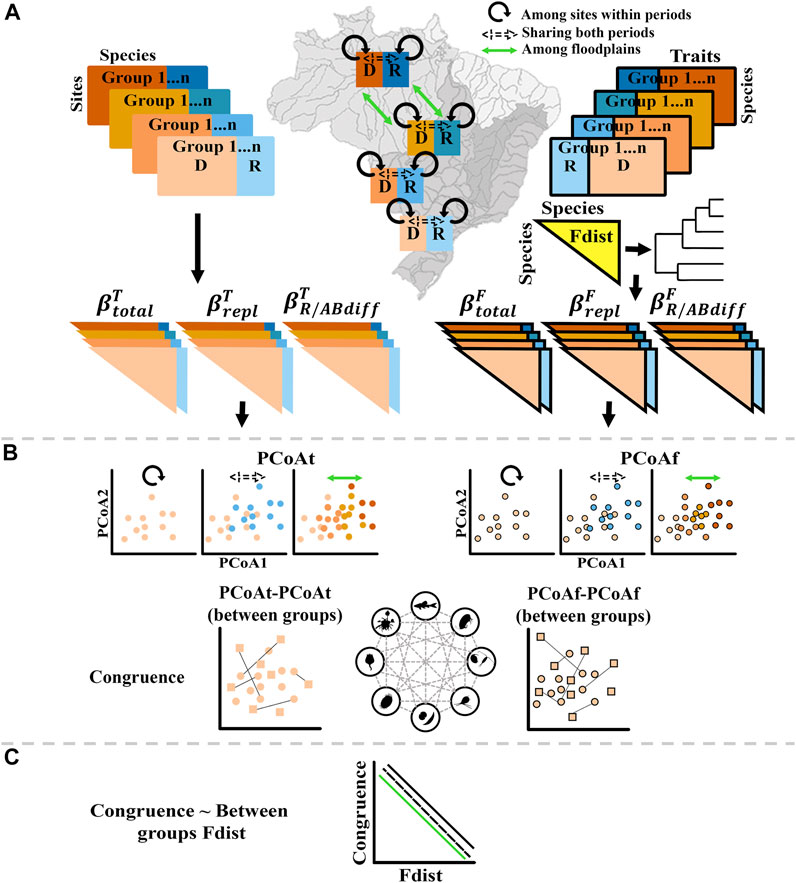
FIGURE 2. Summary of the analytical approach. (A) the combinations of the three nested subsets, how the taxonomic and functional datasets are organized, and the calculation of each beta diversity component. (B) the steps to obtain the cross-taxon congruence values. First, we performed a Principal Coordinates Analysis (PCoA) on each dissimilarity matrix and then the Procrustes correlations between each pair of ordinations, separately for taxonomic and functional data. (C) A Mantel test was carried out between pairs of congruence and functional dissimilarity between biological groups.
We first summarized each dissimilarity matrix using the two first axes of a Principal Coordinates Analysis (PCoA). This analysis was carried out using the function cmdscale from the “stats” R-package. Then, we assessed cross-taxon congruence using Procrustes analysis on the PCoA scores (Jackson, 1995; Peres-Neto and Jackson, 2001). We opted for Procrustes instead of other usual congruence-based methods (e.g., Mantel) because it is more robust in dealing with large environmental gradients (Peres-Neto and Jackson, 2001). In the Procrustes analysis, the mismatch between two ordination configurations (Jackson, 1995) is given by the badness-of-fit statistic (m2). We transformed the m2 into a goodness-of-fit Procrustes r statistic, where
Finally, to assess the relationship between the congruence strengths (as given by Procrustes r) and functional distances among biological groups, we used the Mantel test implemented in the function mantel from R-package “vegan”. Mantel tests considered the Gower-based functional dissimilarities among biological groups and the pairwise congruences among biological groups. Under our hypothesis, we expect a negative correlation between cross-taxon congruence and functional distance (Figure 2C).
Results
Cross-Taxon Congruence Patterns Across Spatial and Temporal Scales
All dissimilarities calculated for each biological group for taxonomic and functional facets, considering the three scale-out perspective, are given in the Supplementary Material S2. Overall, cross-taxon congruence was low (<0.65) for all beta diversity components regardless of the diversity facet. Congruence was higher for the βrepl component (presence/absence data) and the βABdiff component (abundance data), although variations were observed with spatial scale.
At floodplain scale, when hydrological periods were analysed individually, the cross-taxon congruence based on presence-absence data showed little variation between dry and wet periods (Figure 3). When the data of two hydrological periods were combined, the lowest values of congruence were, in general, found in the Upper Paraná River floodplain. At the continental scale, we found clear differences between taxonomic and functional facets, with higher congruence for the taxonomic facet, especially for
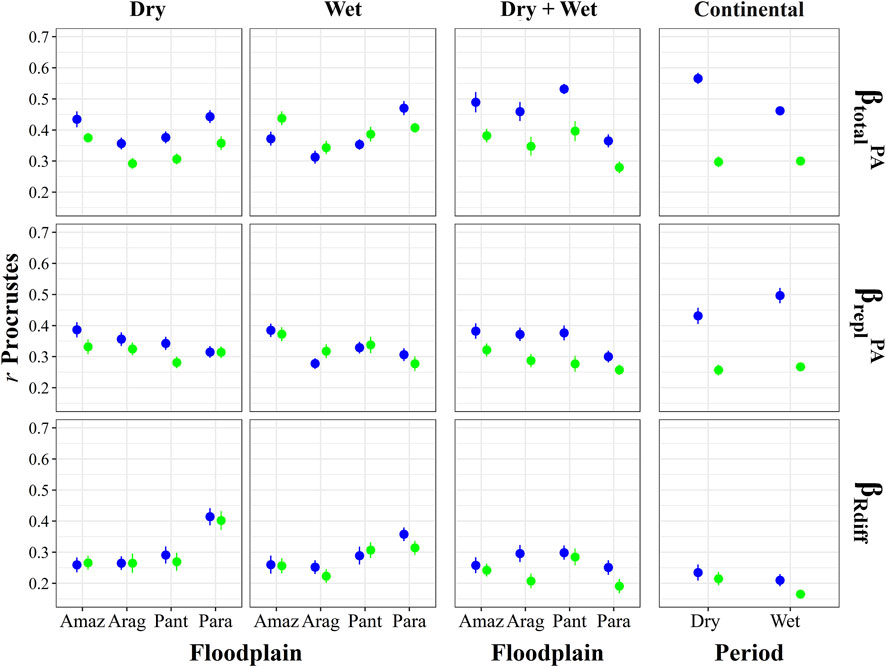
FIGURE 3. Mean and standard deviation of congruence calculated from all pairs of combinations between biological groups for presence-absence data. Blue, Taxonomic facet; Green, Functional facet; Amaz, Amazônia; Arag, Araguaia; Pant, Pantanal; Para, Paraná.
For abundance data, congruence decreased at the continental scale (Figure 4). The southern floodplains (Paraná and Pantanal) showed higher congruences within periods, but the pattern was reversed when periods were combined. That is, congruence values (based on
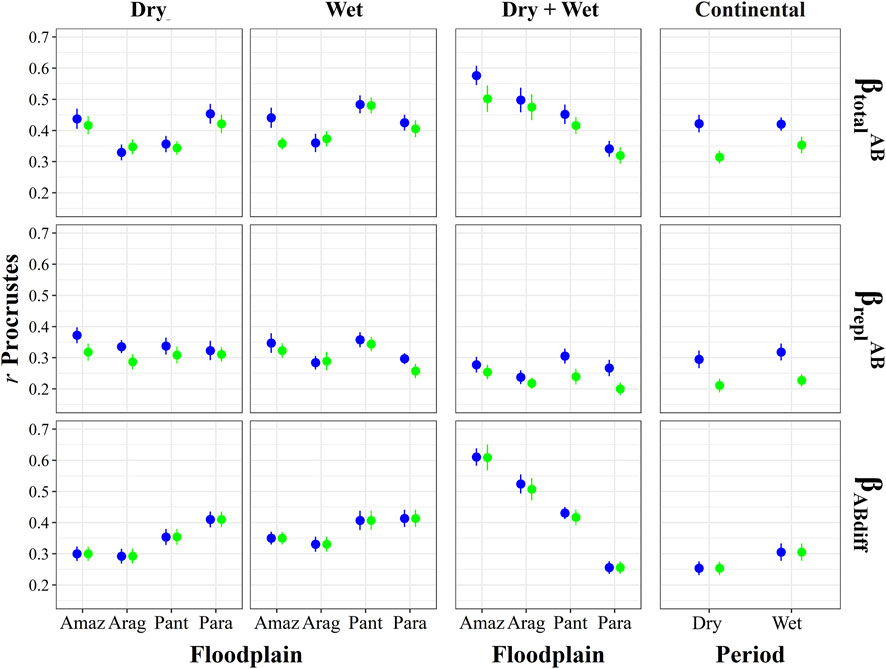
FIGURE 4. Mean and standard deviation of congruence calculated from all pairs of combinations between biological groups for abundance data. Blue, Taxonomic facet; Green, Functional facet; Amaz, Amazônia; Arag, Araguaia; Pant, Pantanal; Para, Paraná.
Relationships Between Congruence and Functional Resemblance Across Biological Groups
Higher functional distances were found between phytoplankton and fish, ciliates and macrophytes, and testate amoebae and macrophytes. In contrast, lower distances were found between ciliates and testate amoebae, microcrustaceans and ostracods, ciliates and phytoplankton, and rotifers and microcrustaceans (Figure 5).
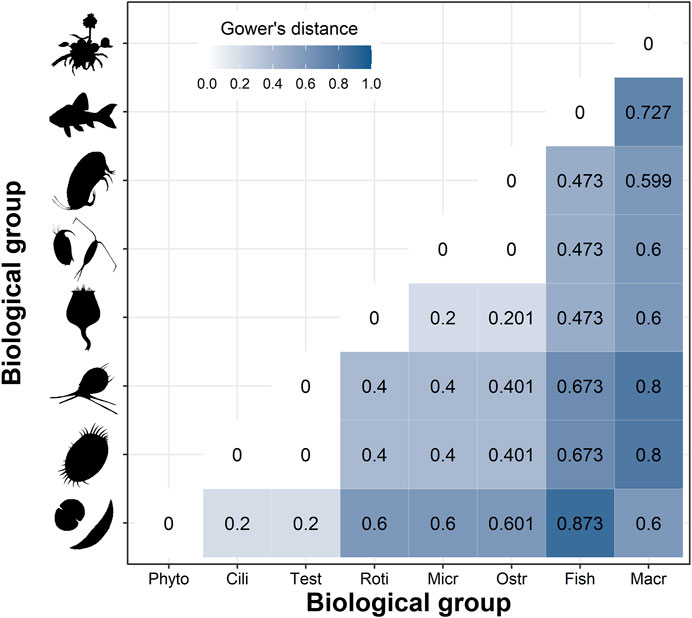
FIGURE 5. Cross-taxon functional dissimilarities among all eight biological groups based on Gower´s index. Group codes: phytoplankton (Phyt), ciliates (Cili), testate amoebae (Test), rotifers (Roti), microcrustaceans (Micr), ostracods (Ostr), macrophytes (Macr).
Mantel test showed that the expected negative relationship between functional dissimilarity and congruence appeared mainly at large spatial scale and mainly for the βtotal and βrepl components of beta diversity (Figures 6, 7). Although some positive relationships emerged at the floodplain scale, the average values of the Mantel test were negative, especially for abundance data (βtotal = -0.58 and βrepl = -0.70 for abundance and βtotal = −0.37 and βrepl. = −0.48, for presence-absence data).
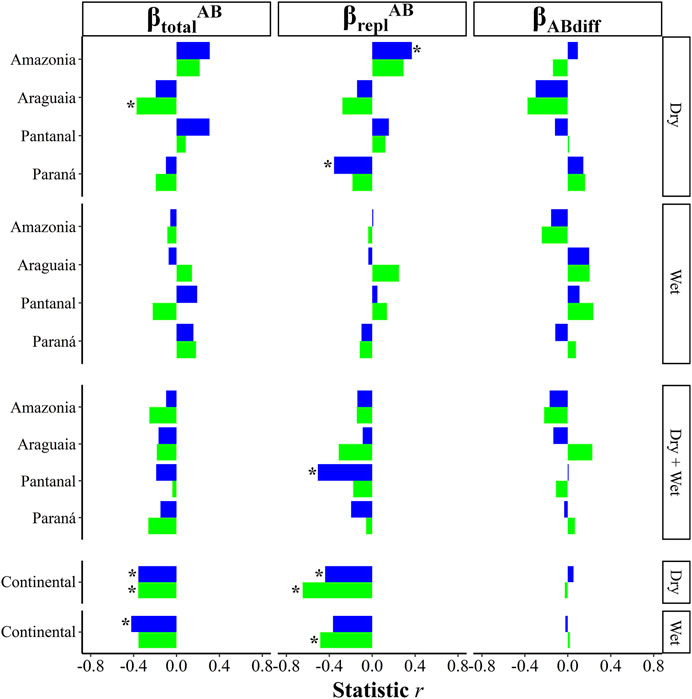
FIGURE 6. Mantel test results showing the r-statistic between the observed congruence and the functional distances between biological groups for presence-absence data. Blue = Taxonomic facet; Green = Functional facet. *p < 0.05.
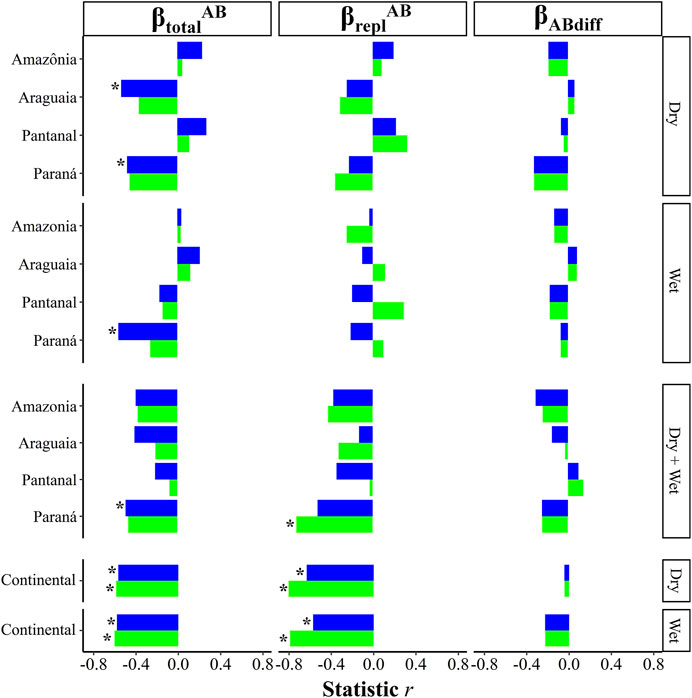
FIGURE 7. Mantel test results showing the r-statistic between the observed congruence and the functional distances between biological groups for abundance data. Blue = Taxonomic facet; Green = Functional facet. *p < 0.05.
Discussion
Cross-taxon congruence can indicate a common response to environmental variation and be caused by strong biotic interactions (Lansac-Tôha et al., 2021b). However, our results show that the spatial scale, the type of data, and the degree of anthropogenic impact can affect cross-taxon congruence. Besides, we found low levels of congruence independently of the biodiversity facet. Therefore, our results imply that using well-known taxonomic groups as surrogates of the changes in composition and diversity of other floodplain communities can have several limitations.
Cross-Taxon Congruence at Floodplain Scale
We found low levels (r < 0.55) of cross-taxon congruence at the floodplain scale. In river-floodplain systems, the low connectivity among habitats during the dry period produces high environmental heterogeneity and constraints dispersal, contributing to an increase in the relative importance of the environment (Dias et al., 2016). Under this rationale, one could expect a higher strength of cross-taxon congruence during the dry periods because the wide environmental gradients would cause large changes in species composition of different taxa (Padial et al., 2012). However, our results did not support this prediction as the strengths of cross-taxon congruence were similar between the hydrological periods. Thus, our results at the floodplain scale are in line with those obtained in other small-scale studies (Padial et al., 2012; Westgate et al., 2014; de Morais et al., 2018). In short, levels of cross-taxon congruence are unlikely to be higher in a specific hydrological period.
Seasonal variation in water level is known to result in large changes in composition and abundance (Bozelli et al., 2015). The magnitude of the temporal turnover in aquatic communities likely depends on the sampling interval relative to the length of the generation cycle of a given community (Van Allen et al., 2017). For instance, microbial and macroinvertebrate communities can show complete turnover in minutes (Fenchel, 1974) and days to months (Allan, 1976), respectively. Indeed, owing to the large environmental changes caused by the hydrological variation, we found increased cross-taxon congruence levels when both hydrological periods were analyzed together (for each floodplain). Such increase was evident when the analyses were based on abundance data, exhibiting a gradient from North to South. This pattern likely resulted from similar seasonal changes in the abundance of multiple groups (Thomaz et al., 2007). Interestingly, the low levels of cross-taxon congruence in the Upper Paraná River floodplain do not invalidate our inference about the importance of hydrological variation in promoting congruence. This is so because the hydrological regime in this floodplain is strongly regulated by upstream dams, reducing the duration and intensity of floods (Agostinho et al., 2004). Thus, cross-taxon congruence might tend to decline in the absence of a major force (i.e., hydrological variation) determining simultaneously the dynamics of different communities between seasons.
Cross-Taxon Congruence at Continental Scale
At large spatial extents, both biogeographic processes and higher environmental heterogeneity are thought to promote cross-taxon congruence (e.g., Rooney and Azeria, 2015; Burrascano et al., 2018). Indeed, studies have shown that cross-taxon congruence in species richness tends to increase with spatial scale (e.g., Wolters et al., 2006; Qian and Kissling, 2010; Burrascano et al., 2018). Thus, the low levels of cross-taxon congruence at the broader spatial scale in our study (i.e., when all floodplains were analyzed together), especially for
Relationship Between Cross-Taxon Congruence and Functional Distance
A negative relationship between functional dissimilarity and congruence is expected when ecologically dissimilar species have different niche requirements. For example, studies have shown that cross-taxon congruence is strongest between biological groups with similar body sizes (Velghe and Gregory-Eaves, 2013; Hájek et al., 2014). Our study demonstrated that the relationship between functional dissimilarity and congruence can vary with the spatial scale (i.e., positive at a small spatial scale and negative at a large spatial scale). At a small spatial scale, both direct and indirect biotic interactions might result in a positive relationship between functional dissimilarity and congruence (Lansac-Tôha et al., 2021b). For example, in the Amazonia floodplain, during the dry period, fish and phytoplankton had the highest congruence for βrepl (Figure 5; Supplementary Figure S1). Planktivorous fish are known to produce strong effects on the structure and dynamics of the zooplankton community through predation-driven control (Lazzaro et al., 2003). These changes can achieve the lowest trophic levels, through an effect known as “trophic cascade” (Carpenter et al., 1985). In this sense, reductions in zooplankton abundance, especially microcrustacean, may weaken the predation pressure on phytoplankton, triggering changes in their abundances (Attayde and Hansson, 2001).
Conclusion
Our findings highlight that the consistent low levels of cross-taxon congruence indicate a low prospect for the reliable use of surrogates in floodplain systems (Padial et al., 2012; Lários et al., 2017). Thus, inferences about the effects of anthropic activities in these systems (e.g., sand mining, water diversion, damming) should be restricted to the biological groups effectively monitored. Similarly, one should not expect that conservation actions planned for a specific biological group (e.g., fish, water birds) would necessarily be beneficial or optimal for other groups. Thus, our study adds to the growing body of evidence that surrogates should be used with caution (de Morais et al., 2018).
Enhancing the efficiency of monitoring programs can be more cost-effective if only one or a few biological groups can predict distribution patterns of other biological groups. However, the strength of community congruence in our study was weak and rarely exceeded 0.7, which is often recommended for a single biological group to predict the spatial patterns of others (Lovell et al., 2007; Heino, 2010). Furthermore, even when the degree of congruence between two biological groups was high at a certain scale, the same pattern was not always repeated at other spatial or temporal scales, making it difficult to unequivocally suggest any specific indicator taxon amongst the groups studied here.
Data Availability Statement
The original contributions presented in the study are included in the article/Supplementary Material, further inquiries can be directed to the corresponding author.
Ethics Statement
The animal study was reviewed and approved by the Sampling permit was issued by the Brazilian Environmental Ministry (Instituto Chico Mendes de Conservação da Biodiversidade - ICMBio, authorization from the System and Information on Biodiversity -SISBIO; protocol #29.652).
Author Contributions
FM-LT: Conceptualization, Methodology, Formal analysis, Data curation, Writing—original draft, Writing—review and editing, Visualization. JHe: Conceptualization, Methodology, Writing—review and editing, Supervision. LB: Conceptualization, Methodology, Writing—review and editing. MB: Conceptualization, Methodology, Formal analysis, Writing—review and editing. OP: Conceptualization, Methodology, Writing—review and editing, Visualization. BQ: Data Curation, Writing—review and editing, Visualization. AP: Data Curation, Writing—review and editing. BM: Data Curation, Writing—review and editing. FF: Writing—review and editing, Visualization. FO: Data Curation, Writing—review and editing. BM: Data Curation, Writing—review and editing. FB: Data Curation, Writing—review and editing. MS: Data Curation, Writing—review and editing. MD-F: Data Curation, Writing—review and editing. RC: Data Curation, Writing—review and editing. RD: Data Curation, Writing—review and editing. CB: Resources, Data Curation, Writing—review and editing. JHi: Investigation, Resources, Data Curation, Writing—review and editing, RM: Investigation, Resources, Data Curation, Writing—review and editing. EB: Resources, Writing—review and editing, CP: Resources, Data Curation, Writing—review and editing, LR: Investigation, Resources, Data Curation, Writing—review and editing. SJ: Investigation, Resources, Data Curation, Writing—review and editing. KM: Conceptualization, Investigation, Writing—review and editing. FA-LT: Conceptualization, Resources, Writing—review and editing, Project administration, Funding acquisition. LV: Conceptualization, Resources, Writing—review and editing, Supervision.
Funding
This research was financed by the Conselho Nacional de Desenvolvimento Científico e Tecnológico (CNPq) and Coordenação de Aperfeiçoamento de Pessoal de Nível Superior (CAPES) - Finance Code 001. We thank for the financial supports from SISBIOTA project (MCT/CNPqMEC/CAPES/FNDCT - no 503243/2010-4).
Conflict of Interest
The authors declare that the research was conducted in the absence of any commercial or financial relationships that could be construed as a potential conflict of interest.
Publisher’s Note
All claims expressed in this article are solely those of the authors and do not necessarily represent those of their affiliated organizations, or those of the publisher, the editors and the reviewers. Any product that may be evaluated in this article, or claim that may be made by its manufacturer, is not guaranteed or endorsed by the publisher.
Acknowledgments
We are grateful to the Conselho Nacional de Desenvolvimento Científico e Tecnológico (CNPq), Coordenação de Aperfeiçoamento de Pessoal de Nível Superior (CAPES) and to the Fundação Araucária for all the financial support to the SISBIOTA project (MCT/CNPqMEC/CAPES/FNDCT - no 503243/2010-4). To both the CNPq and the Coordenação de Aperfeiçoamento de Pessoal de Nível Superior (CAPES), for granting multiple doctoral, postdoctoral, and research productivity scholarships. We also thank the Núcleo de Pesquisas em Limnologia, Ictiologia e Aquicultura (Nupelia), the Instituto Nacional de Pesquisas da Amazônia (INPA), the Universidade de Brasília (UnB), and the Universidade Federal do Mato Grosso do Sul (UFMS) for providing infrastructure and staff during field surveys. FMLT is particularly grateful to the CAPES for the opportunity to carry out part of its doctoral research abroad (SYKE-Finland).
Supplementary Material
The Supplementary Material for this article can be found online at: https://www.frontiersin.org/articles/10.3389/fenvs.2022.903074/full#supplementary-material
References
Agostinho, A. A., Gomes, L. C., Veríssimo, S., and K. Okada, E. (2004). Flood Regime, Dam Regulation and Fish in the Upper Paraná River: Effects on Assemblage Attributes, Reproduction and Recruitment. Rev. Fish. Biol. Fish. 14, 11–19. doi:10.1007/s11160-004-3551-y
Allan, J. D. (1976). Life History Patterns in Zooplankton. Am. Nat. 110, 165–180. doi:10.1086/283056
Attayde, J. L., and Hansson, L.-A. (2001). The Relative Importance of Fish Predation and Excretion Effects on Planktonic Communities. Limnol. Oceanogr. 46, 1001–1012. doi:10.4319/lo.2001.46.5.1001
Banos, S., Gysi, D. M., Richter-Heitmann, T., Glöckner, F. O., Boersma, M., Wiltshire, K. H., et al. (2020). Seasonal Dynamics of Pelagic Mycoplanktonic Communities: Interplay of Taxon Abundance, Temporal Occurrence, and Biotic Interactions. Front. Microbiol. 11, 1305. doi:10.3389/fmicb.2020.01305
Barton, A. D., Pershing, A. J., Litchman, E., Record, N. R., Edwards, K. F., Finkel, Z. V., et al. (2013). The Biogeography of Marine Plankton Traits. Ecol. Lett. 16, 522–534. doi:10.1111/ele.12063
Bozelli, R. L., Thomaz, S. M., Padial, A. A., Lopes, P. M., and Bini, L. M. (2015). Floods Decrease Zooplankton Beta Diversity and Environmental Heterogeneity in an Amazonian Floodplain System. Hydrobiologia 753, 233–241. doi:10.1007/s10750-015-2209-1
Brose, U., and Hillebrand, H. (2016). Biodiversity and Ecosystem Functioning in Dynamic Landscapes. Phil. Trans. R. Soc. B 371, 20150267. doi:10.1098/rstb.2015.0267
Browne, R. A., and MacDonald, G. H. (1982). Biogeography of the Brine Shrimp, Artemia: Distribution of Parthenogenetic and Sexual Populations. J. Biogeogr. 9, 331. doi:10.2307/2844719
Burrascano, S., de Andrade, R. B., Paillet, Y., Ódor, P., Antonini, G., Bouget, C., et al. (2018). Congruence across Taxa and Spatial Scales: Are We Asking Too Much of Species Data? Glob. Ecol. Biogeogr. 27, 980–990. doi:10.1111/geb.12766
Cardinale, B. J., Duffy, J. E., Gonzalez, A., Hooper, D. U., Perrings, C., Venail, P., et al. (2012). Biodiversity Loss and its Impact on Humanity. Nature 486, 59–67. doi:10.1038/nature11148
Cardoso, P., Rigal, F., and Carvalho, J. C. (2015). BAT - Biodiversity Assessment Tools, an R Package for the Measurement and Estimation of Alpha and Beta Taxon, Phylogenetic and Functional Diversity. Methods Ecol. Evol. 6, 232–236. doi:10.1111/2041-210X.12310
Carpenter, S. R., Kitchell, J. F., and Hodgson, J. R. (1985). Cascading Trophic Interactions and Lake Productivity. Bioscience 35, 634–639. doi:10.2307/1309989
De Bie, T., Meester, L., Brendonck, L., Martens, K., Goddeeris, B., Ercken, D., et al. (2012). Body Size and Dispersal Mode as Key Traits Determining Metacommunity Structure of Aquatic Organisms. Ecol. Lett. 15, 740–747. doi:10.1111/j.1461-0248.2012.01794.x
de Morais, G. F., dos Santos Ribas, L. G., Ortega, J. C. G., Heino, J., and Bini, L. M. (2018). Biological Surrogates: A Word of Caution. Ecol. Indic. 88, 214–218. doi:10.1016/j.ecolind.2018.01.027
Dias, J. D., Simões, N. R., Meerhoff, M., Lansac-Tôha, F. A., Velho, L. F. M., and Bonecker, C. C. (2016). Hydrological Dynamics Drives Zooplankton Metacommunity Structure in a Neotropical Floodplain. Hydrobiologia 781, 109–125. doi:10.1007/s10750-016-2827-2
Diniz, L. P., Petsch, D. K., and Bonecker, C. C. (2021). Zooplankton β Diversity Dynamics and Metacommunity Structure Depend on Spatial and Temporal Scales in a Neotropical Floodplain. Freshw. Biol. 66, 1328–1342. doi:10.1111/fwb.13719
Fenchel, T. (1974). Intrinsic Rate of Natural Increase: The Relationship with Body Size. Oecologia 14, 317–326. doi:10.1007/BF00384576
Hájek, M., Poulíčková, A., Vašutová, M., Syrovátka, V., Jiroušek, M., Štěpánková, J., et al. (2014). Small Ones and Big Ones: Cross-Taxon Congruence Reflects Organism Body Size in Ombrotrophic Bogs. Hydrobiologia 726, 95–107. doi:10.1007/s10750-013-1754-8
Heino, J. (2010). Are Indicator Groups and Cross-Taxon Congruence Useful for Predicting Biodiversity in Aquatic Ecosystems? Ecol. Indic. 10, 112–117. doi:10.1016/j.ecolind.2009.04.013
Hillebrand, H., Watermann, F., Karez, R., and Berninger, U.-G. (2001). Differences in Species Richness Patterns between Unicellular and Multicellular Organisms. Oecologia 126, 114–124. doi:10.1007/s004420000492
Hortal, J., de Bello, F., Diniz-Filho, J. A. F., Lewinsohn, T. M., Lobo, J. M., and Ladle, R. J. (2015). Seven Shortfalls that Beset Large-Scale Knowledge of Biodiversity. Annu. Rev. Ecol. Evol. Syst. 46, 523–549. doi:10.1146/annurev-ecolsys-112414-054400
Incagnone, G., Marrone, F., Barone, R., Robba, L., and Naselli-Flores, L. (2015). How Do Freshwater Organisms Cross the “Dry Ocean”? A Review on Passive Dispersal and Colonization Processes with a Special Focus on Temporary Ponds. Hydrobiologia 750, 103–123. doi:10.1007/s10750-014-2110-3
Irion, G., Junk, W. J., and de Mello, J. A. S. N. (1997). “The Large Central Amazonian River Floodplains Near Manaus: Geological, Climatological, Hydrological and Geomorphological Aspects,” in The Central Amazon Floodplain: Ecology of a Pulsing System. Editor W. J. Junk (Heidelberg: Springer), 23–46. doi:10.1007/978-3-662-03416-3_2
Jackson, D. A. (1995). PROTEST: A PROcrustean Randomization TEST of Community Environment Concordance. Écoscience 2, 297–303. doi:10.1080/11956860.1995.11682297
Junk, W., Bayley, P. B., and Sparks, R. E. (1989). The Flood Pulse Concept in River-Floodplain Systems. Can. Spec. Publ. Fish. Aquat. Sci. 106, 110–127.
Laliberté, E., Legendre, P., Shipley, B., and Laliberté, M. E. (2014). Package ‘FD’. Measuring Functional Diversity from Multiple Traits, and Other Tools for Functional Ecology. R package version 1.0-12.1. Available at: https://cran.r-project.org/web/packages/FD/FD.pdf.
Lansac‐Tôha, F. M., Bini, L. M., Heino, J., Meira, B. R., Segovia, B. T., Pavanelli, C. S., et al. (2021a). Scale‐dependent Patterns of Metacommunity Structuring in Aquatic Organisms across Floodplain Systems. J. Biogeogr. 48, 872–885. doi:10.1111/jbi.14044
Lansac‐Tôha, F. M., Quirino, B. A., Souza, Y. R., Lansac‐Tôha, F. A., Velho, L. F. M., and Baumgartner, M. T. (2021b). The Commonality of Core Biological Groups across Freshwater Food Webs. Limnol. Oceanogr. 66, 1459–1474. doi:10.1002/lno.11697
Lansac-Tôha, F. M., Heino, J., Quirino, B. A., Moresco, G. A., Peláez, O., Meira, B. R., et al. (2019). Differently Dispersing Organism Groups Show Contrasting Beta Diversity Patterns in a Dammed Subtropical River Basin. Sci. Total Environ. 691, 1271–1281. doi:10.1016/j.scitotenv.2019.07.236
Lários, M. C., Nunes da Cunha, C., Penha, J., Landeiro, V. L., Pinho, J. B., Aragona, M., et al. (2017). Evidence of Cross-Taxon Congruence in Neotropical Wetlands: Importance of Environmental and Spatial Factors. Glob. Ecol. Conservation 12, 108–118. doi:10.1016/j.gecco.2017.09.003
Lazzaro, X., Bouvy, M., Ribeiro-Filho, R. A., Oliviera, V. S., Sales, L. T., Vasconcelos, A. R. M., et al. (2003). Do fish Regulate Phytoplankton in Shallow Eutrophic Northeast Brazilian Reservoirs? Freshw. Biol. 48, 649–668. doi:10.1046/j.1365-2427.2003.01037.x
Legendre, P. (2014). Interpreting the Replacement and Richness Difference Components of Beta Diversity. Glob. Ecol. Biogeogr. 23, 1324–1334. doi:10.1111/geb.12207
Lovell, S., Hamer, M., Slotow, R., and Herbert, D. (2007). Assessment of Congruency across Invertebrate Taxa and Taxonomic Levels to Identify Potential Surrogates. Biol. Conserv. 139, 113–125. doi:10.1016/j.biocon.2007.06.008
Oksanen, J., Blanchet, F. G., Friendly, M., Kindt, R., Legendre, P., McGlinn, D., et al. (2018). Vegan: Community Ecology Package. R package version 2.5-3. Available at: https://cran.r-project.org/web/packages/vegan/vegan.pdf.
Padial, A. A., Declerck, S. A. J., De Meester, L., Bonecker, C. C., Lansac-tôha, F. A., Rodrigues, L. C., et al. (2012). Evidence against the Use of Surrogates for Biomonitoring of Neotropical Floodplains. Freshw. Biol. 57, 2411–2423. doi:10.1111/fwb.12008
Peres-Neto, P. R., and Jackson, D. A. (2001). How Well Do Multivariate Data Sets Match? the Advantages of a Procrustean Superimposition Approach over the Mantel Test. Oecologia 129, 169–178. doi:10.1007/s004420100720
Podani, J., and Schmera, D. (2011). A New Conceptual and Methodological Framework for Exploring and Explaining Pattern in Presence - Absence Data. Oikos 120, 1625–1638. doi:10.1111/j.1600-0706.2011.19451.x
Qian, H., and Kissling, W. D. (2010). Spatial Scale and Cross-Taxon Congruence of Terrestrial Vertebrate and Vascular Plant Species Richness in China. Ecology 91, 1172–1183. doi:10.1890/09-0620.1
Quirino, B. A., Carniatto, N., Thomaz, S. M., and Fugi, R. (2019). Small Fish Diet in Connected and Isolated Lakes in a Neotropical Floodplain. Ecol. Freshw. Fish. 28, 97–109. doi:10.1111/eff.12434
R Core Team (2021). R: A Language and Environment for Statistical Computing. Vienna: R foundation for Statistical Computing.
Rocha, M. P., Heino, J., Machado-Velho, L. F., Lansac-Tôha, F. M., and Lansac-Tôha, F. A. (2017). Fine Spatial Grain, Large Spatial Extent and Biogeography of Macrophyte-Associated Cladoceran Communities across Neotropical Floodplains. Freshw. Biol. 62, 559–569. doi:10.1111/fwb.12885
Rodrigues, A. S. L., and Brooks, T. M. (2007). Shortcuts for Biodiversity Conservation Planning: The Effectiveness of Surrogates. Annu. Rev. Ecol. Evol. Syst. 38, 713–737. doi:10.1146/annurev.ecolsys.38.091206.095737
Rooney, R. C., and Azeria, E. T. (2015). The Strength of Cross‐taxon Congruence in Species Composition Varies with the Size of Regional Species Pools and the Intensity of Human Disturbance. J. Biogeogr. 42, 439–451. doi:10.1111/jbi.12400
Rosser, N. (2017). Shortcuts in Biodiversity Research: what Determines the Performance of Higher Taxa as Surrogates for Species? Ecol. Evol. 7, 2595–2603. doi:10.1002/ece3.2736
Stevaux, J. (1994). The Upper Paraná River (Brazil): Geomorphology, Sedimentology and Paleoclimatology. Quat. Int. 21, 143–161. doi:10.1016/1040-6182(94)90028-0
Thomaz, S. M., Bini, L. M., and Bozelli, R. L. (2007). Floods Increase Similarity Among Aquatic Habitats in River-Floodplain Systems. Hydrobiologia 579, 1–13. doi:10.1007/s10750-006-0285-y
Van Allen, B. G., Rasmussen, N. L., Dibble, C. J., Clay, P. A., and Rudolf, V. H. W. (2017). Top Predators Determine How Biodiversity Is Partitioned across Time and Space. Ecol. Lett. 20, 1004–1013. doi:10.1111/ele.12798
Vasconcelos, H. L., Maravalhas, J. B., Neves, K. C., Pacheco, R., Vieira, J., Camarota, F. C., et al. (2019). Congruent Spatial Patterns of Ant and Tree Diversity in Neotropical Savannas. Biodivers. Conserv. 28, 1075–1089. doi:10.1007/s10531-019-01708-9
Velghe, K., and Gregory-Eaves, I. (2013). Body Size Is a Significant Predictor of Congruency in Species Richness Patterns: A Meta-Analysis of Aquatic Studies. PLoS One 8, e57019–6. doi:10.1371/journal.pone.0057019
Vieira, L. C. G., Padial, A. A., Velho, L. F. M., Carvalho, P., and Bini, L. M. (2015). Concordance Among Zooplankton Groups in a Near-Pristine Floodplain System. Ecol. Indic. 58, 374–381. doi:10.1016/j.ecolind.2015.05.049
Westgate, M. J., Barton, P. S., Lane, P. W., and Lindenmayer, D. B. (2014). Global Meta-Analysis Reveals Low Consistency of Biodiversity Congruence Relationships. Nat. Commun. 5, 1–8. doi:10.1038/ncomms4899
Whittaker, R. J., Araújo, M. B., Jepson, P., Ladle, R. J., Watson, J. E. M., and Willis, K. J. (2005). Conservation Biogeography: Assessment and Prospect. Divers. Distrib. 11, 3–23. doi:10.1111/j.1366-9516.2005.00143.x
Keywords: turnover, richness difference, aquatic communities, freshwater, spatial concordance
Citation: Lansac-Tôha FM, Heino J, Bini LM, Peláez O, Baumgartner MT, Quirino BA, Pineda A, Meira BR, Florêncio FM, Oliveira FR, Bomfim FF, Silveira MJ, Dainez-Filho MS, Campos R, Dias RM, Bonecker CC, Higuti J, Mormul RP, Benedito E, Pavanelli CS, Rodrigues LC, Jati S, Martens K, Lansac-Tôha FA and Velho LFM (2022) Cross-Taxon Congruence of Taxonomic and Functional Beta-Diversity Facets Across Spatial and Temporal Scales. Front. Environ. Sci. 10:903074. doi: 10.3389/fenvs.2022.903074
Received: 23 March 2022; Accepted: 16 June 2022;
Published: 08 July 2022.
Edited by:
Roberto Júnio Pedroso Dias, Juiz de Fora Federal University, BrazilReviewed by:
Eduardo Périco, Universidade do Vale do Taquari - Univates, BrazilLifeng Zhu, Nanjing Normal University, China
Copyright © 2022 Lansac-Tôha, Heino, Bini, Peláez, Baumgartner, Quirino, Pineda, Meira, Florêncio, Oliveira, Bomfim, Silveira, Dainez-Filho, Campos, Dias, Bonecker, Higuti, Mormul, Benedito, Pavanelli, Rodrigues, Jati, Martens, Lansac-Tôha and Velho. This is an open-access article distributed under the terms of the Creative Commons Attribution License (CC BY). The use, distribution or reproduction in other forums is permitted, provided the original author(s) and the copyright owner(s) are credited and that the original publication in this journal is cited, in accordance with accepted academic practice. No use, distribution or reproduction is permitted which does not comply with these terms.
*Correspondence: Fernando Miranda Lansac-Tôha, ZmVybmFuZG9fdG9oYUBob3RtYWlsLmNvbQ==