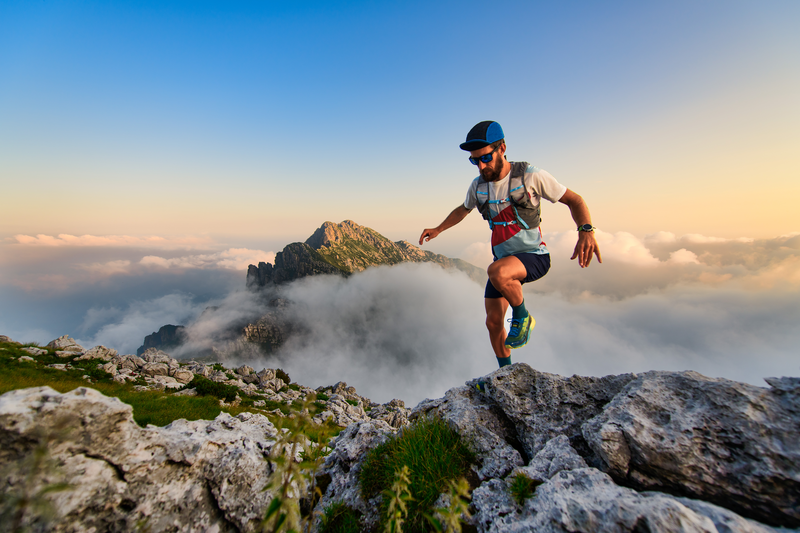
95% of researchers rate our articles as excellent or good
Learn more about the work of our research integrity team to safeguard the quality of each article we publish.
Find out more
ORIGINAL RESEARCH article
Front. Environ. Sci. , 27 June 2022
Sec. Soil Processes
Volume 10 - 2022 | https://doi.org/10.3389/fenvs.2022.903039
This article is part of the Research Topic Vermiremediation in Contaminated Soils: An Approach for Soil Stabilization View all 9 articles
Litterfall, directly and indirectly, affects the soil physicochemical properties, microbial activity, and diversity of soil fauna and flora by adding organic matter and nutrients to the soil. This study explores litterfall dynamics such as litterfall production, litter decomposition rate, and associated nutrient return in three forest types, that is, camphor tree forest (CTF), Masson pine forest (MPF), and camphor tree and Masson pine mixed forest (CMF), in subtropical China. Results showed that CMF had the highest mean annual litterfall production (4.30 ± 0.22 t ha−1), which was significantly higher than that of MPF (3.41 ± 0.25 t ha−1) and CTF (3.26 ± 0.17 t ha−1). Leaf represented the major fraction of litterfall, constituting over 71% of the total litterfall mass in the three forest types. The contribution of branch litter was 16.3, 8.9, and 16.9%, and miscellaneous litter was 12.6, 18.9, and 11.1% in CTF, MPF, and CMF, respectively. The concentration of macronutrients ranked as N > Ca > K > Mg > P in all litter fractions. The total annual macronutrient return to the soil from the litterfall was in order as CTF (74.2 kg ha−1‧yr−1) > CMF (70.7 kg ha−1‧yr−1) > MPF (33.6 kg ha−1‧yr−1). The decomposition rate was higher in leaf litter than in branch litter throughout the three forests. Among the forest types, the leaf and branch decomposition rates were in a pattern: CTF > CMF > MPF. The ratio of C/N in both leaf and branch litters was significantly higher in MPF than in CTF and CMF, while no significant differences in N/P ratio were found in these litters among the three forests. The high N:P ratios in leaf litter (23/30) and the branch (24/32) litter indicated the high N returning and low nutrient returning to the soil. Our results suggested that the broadleaved forests have faster litter decomposition and higher macronutrient returns than conifer forests. Moreover, the litter decomposition rate was mainly associated with litterfall quality and chemical composition. The introduction of broadleaved trees into monoculture coniferous stands could increase litter production nutrients return, and thus, it had advantages in soil nutrients restoration and sustainable forest management.
Masson pine (Pinus massoniana Lamb.) is a typical evergreen conifer tree species widely distributed in central and southern China (Farooq et al., 2021d). This specie is a common tree used in plantation forestry for replacing or compensating for the loss of the natural forest in this region. Camphor tree (Cinnamomum camphora (L.) J. Presl.) is a common evergreen broadleaved tree species and is often planted for timber production, camphor-related products, and shade trees. Both these tree species provide critical economic benefits to the country and also provide ecosystem services by periodically shedding parts of their biomass (litterfall), which is a key biogeochemical process within the ecosystems of the subtropical region (Chave et al., 2010; Gilani et al., 2021).
Litterfall and its sequential decomposition are important processes for transferring carbon (C) and other nutrients from the aboveground system to the below-ground system in forests (Sayer, 2006; Hansen et al., 2009; Cakir and Makineci, 2020; Farooq et al., 2020). Such processes constitute significant steps in nutrient cycling by converting organic materials to inorganic elements, which the plants can re-uptake. Litter quality in terms of nitrogen (N), phosphorus (P), and potassium (K) concentration has also been reported to be influenced by climate conditions such as temperature and precipitation in addition to various forest types (Liu et al., 2006). Different tree species have different litterfall production and nutrient release patterns (Khiewtam and Ramakrishnan, 1993). In addition, litterfall has direct and indirect effects on the soil’s physical and chemical properties, microbial activity, and diversity of soil fauna and flora by adding the organic matter and nutrients to the soil (Sayer, 2006; Wang et al., 2008). Therefore, with its associated flux of nutrients, litterfall provides long-term nutrient maintenance in forest ecosystems and has been a key research target for a better understanding of soil fertility, site productivity, and forest services (Liski et al., 2005; Sayer, 2006).
It is reported that mixed forests are more stand productive than pure forests (Staelens et al., 2003). Their productivity is due to the nutrients and elements from the litterfall of varying quality (Pretzsch et al., 2010). Moreover, litterfall production was positively related to net primary productivity (NPP) in forests, and overall annual litterfall production accounted for about one-third of NPP (Huang et al., 2017). Large variability of nutrients in forest floor accumulation should primarily be attributed to differences in litter decomposition (Hansen et al., 2009), litter quality (lignin content and lignin/N ratio), nutrient return capacity (Rashid et al., 2020; Farooq et al., 2021a,b), soil enzymatic activity (Farooq et al., 2021c) and climatic and soil conditions (monthly rainfall and soil moisture); those factors to influence the rate of decomposition (Dhanya et al., 2013). Celentano et al. (2010) evaluated litterfall and nutrient dynamics under different forest types and found litter production was highest in secondary forests and lowest in natural generation forests. Nakane (1995) suggested that the lower rate of decomposition of the leaf litter in Japanese cedar (Cryptomeria japonica D. Don) plantations and red pine (Pinusresinosa Ait.) plantations contrasted to a higher rate in an oak forest due to their chemical composition in China (Wu et al., 2000).
The litterfall quantity and dynamics have been recognized as an integrated response in forests, depending on tree species composition, forest structure, and environmental factors (Khanna et al., 2009). For sustainable management of a healthy forest ecosystem, knowledge about litter quality and quantity (Hansen et al., 2009), decomposition rate, and nutrient return (Wu et al., 2017; Farooq et al., 2018) is important for understanding energy flow and nutrient cycling in forest ecosystems. Even though different studies have been conducted on litterfall production and litter decomposition process in tropical and temperate forests (Martius et al., 2004; Yang et al., 2004; Bigelow and Canham 2015; Neumann et al., 2018), however, the combined effects of litterfall production, decomposition rates, and macronutrient elements and the characteristics of litter CNP stoichiometry in different forest types in subtropical region are still unknown. This study explores the amount of litterfall production, litter decomposition rate, and nutrient chemistry in three major forest types, that is, camphor tree forest (CTF), Masson pine forest (MPF), and camphor tree and Masson pine mixed forest (CMF) in subtropical China. The specific objectives of the study were: 1) to examine the seasonal patterns and quantify the components of litterfall production (litter decomposition rates, nutrient contents, and nutrient returns) in the studied forests 2) and to investigate the characteristics of C:N:P stoichiometry of litterfall in the studied forests.
The study site is located in Hunan Forest Botanical Garden, Changsha City, Hunan Province, China (280 06′-07′N, 1130 02′-03′ E) (Figure 1). The garden has a total of 200 ha of forested land. The elevation of the study area is around 46–114 m, with a slope between 5–15o. The mean annual temperature is 17.2°C, with the lowest and the highest mean monthly temperatures of 4.7 and 29.4°C in January and July, respectively. The mean annual precipitation is 1,422 mm, mainly from April to August. The mean annual sunshine is 1,677 h, with a mean annual relative humidity greater than 80% (Supplementary Table S1). The annual frost-free period is 270–310 days. The soil is a typical yellow soil type derived from the quaternary alluvial deposited grit with a soil pH of 5.0. The dominant tree species in this site are Masson pine (Pinus massoniana), Chinese fir (Cunninghamia lanceolata (Lamb.) Hook.), Chinese sweetgum (Liquidambar acalycina), camphor tree (Cinnamomum camphora), and Slash pine (Pinus elliottii).
FIGURE 1. Location of the study area, Hunan Botanical Garden in Changsha city, Hunan Province, China.
Three dominant forest types in the garden were selected for the research project. The study site’s Masson pine and camphor tree forests were planted during 1980–1990 with an initial tree spacing of 2 × 3 m. The Masson pine forests and camphor tree mixed forests (after that as the mixed forest) were planted in 1987 with an initial spacing of 2 × 3 m using a proportion of 50%:50% for the two tree species. Understory vegetation consisted of Sassafeastsumu Hemsl.; Cinnamomumcamphora; Symplocoscaudata Wall. ex A. DC.; Clerodendroncyrtophyllum Turcz; Nephrolepisauriculata Trimen; Lophantherumgracile Brengn.; Miscanthusfloridulus Warband, and Phytolaccaacinosa Roxb. The characteristics of forest structure in three types of forest stands are presented in Table 1.
The current experiment was set up as a completely randomized design (CRD), with the main factor being selected forest types (CTF, MPF, and CMF). A 20 × 20 m plot with three replicates was established in each forest type. A 2 × 3 m litter trap was designed using a nylon netting screen (mesh size 2 mm) and was mounted on wooden poles approximately 80 cm above ground using ropes. Three litter traps were randomly set up in each plot to collect the litterfall. Therefore, a total of 27 litter traps were set up in the studied forests.
Litterfall was collected from the litter traps biweekly from October 2014 to December 2015. The fresh weight of litterfall in each trap was measured using a balance. Subsamples were taken and placed in paper bags. These litterfall subsamples were brought to the laboratory, where the litterfall was sorted out into three fractions: 1) leaf letter, 2) branch letter, and 3) miscellaneous litter (bark, flowers, fruits, and unrecognizable remains of fine particles). All litterfall fractions were then dried at 70°C for approximately 48 h (until a constant weight was obtained). The dry weight of each litterfall fraction was recorded.
The oven-dried litterfall subsamples were ground using a Wiley mill and then passed through a 40 mesh steel sieve before chemical analysis. Litterfall samples were chemically analyzed for C, N, P, K, Ca (calcium), Mg (magnesium), and S (sulfur) elements. The total C concentration was analyzed using the sulfuric acid-potassium dichromate method, and the total N concentration was analyzed using the Kjeldahl method. P and K were extracted by wet ashing of 0.5 g plant material in an acid mixture consisting of 10 ml H2SO4, 3 ml HNO3, and 1 ml HClO4. The P concentration was determined by the molybdenum blue method. The K concentration was determined using the flame photometric method (Farooq et al., 2021b). The atomic absorption spectrophotometer method was used to determine the concentrations of Ca and Mg.
The litterbag technique was used to measure the litter decomposition process (Melillo et al., 1984). About 20 g of litterfall samples separately with dried leaf and branch litter and miscellaneous litter samples were placed into fiber mesh bags (20 × 20 cm). The bags were placed in the litter layer on the forest floor at the study site. Then three bags in each plot were retrieved to measure the dry weights at 2, 4, 6, 8, 10, 12, and 14 months intervals. The bimonthly weight loss from the decomposing litter was determined based on the difference between the mass remaining in the litterbags in the retrieved time and the initial weight.
The decomposition rate was calculated using a single exponential decay equation according to the work of Olson (1963):
where Wt was the dry mass of litter fraction remaining at time t, W0 was the initial dry mass of litter fraction at time t0, and k was decomposition constant.
The time for 50% litter mass loss (t0.5) and 95% of litter mass loss (t0.95) can be calculated as follows:
Two-way analysis of variance (ANOVA) was used to statistically assess the net effects of different forest types and plot temporal (month-to-month) variation and their interactions with litter production and nutrient concentrations. The original litter content and decomposition rate data were log-transformed to satisfy the normality and homoscedasticity assumptions of ANOVA. Statistical analyses were conducted using the SAS statistical package (SAS Institute, Inc., Cary, NC, United States). Multiple regression analysis examined relationships between litter amounts and decomposition rates at the surrounding environmental factors (soil temperature and moisture). The correlation relationship analysis was performed between the litter amounts and nutrient concentrations.
The annual litterfall production in the three examined forest types ranged from 3.26 to 4.30 t ha−1 yr−1 (Table 2). The CMF has a significantly higher total litterfall than CTF and MPF (p = 0.036), but no significant difference in total litterfall was found between CTF and MRF (p = 0.065). Leaf was the major component of the total litterfall, accounting for over 71% in the examined forests. The branch fraction was the second-largest litterfall component (>16% of the total litterfall) for all forests, except in MPF, where the branches contributed about 9% of the total litterfall. The MPF stands had a significantly higher content of miscellaneous components than in CTF and CMF stands (p = 0.027). The miscellaneous fraction in MPF accounted for about 19% of the total litterfall mass compared to about 11%–13% in CMF and CTF (Table 2).
TABLE 2. Annual biomass production and distribution percentage of litterfall components in the three subtropical forest types.
Monthly litterfall production ranged from 0.03–0.58, 0.10–0.77, and 0.13–1.23 t hm2 in the CTF, MPF, and CMF stand, respectively (Figure 2). The minimum litterfall production occurred in spring and summer (March-July), while the maximum litterfall occurred in fall and winter (Aug–Feb) (Figure 2).
FIGURE 2. Seasonal changes in total litterfall biomass in the three examined forest types. CTF, camphor tree forest; MPF, Masson pine forest; CMF, camphor tree and Masson pine mixed forest.
The percentage of litter mass remaining decreased throughout the study period of decomposition in the examined forests (Figure 2). The decomposition rate of leaf and branch litters was the highest in CTF, followed by CMF, and the lowest in MPF (Figures 3A,B). The leaf remained about 8, 45, and 71% of the initial weight in CTF, CMF, and MPF stands, respectively, 1-year post-experiment. A similar pattern of decomposition rates was found for branch litter in all forests. Still, the decomposition rate was considerably slower in branches than in leaf components during the study period (Figures 3A,B). After 1-year post-experiment, about 40, 46, and 48% of total branch litter mass remained in CTF, CMF, and MPF, respectively (Figure 3B).
FIGURE 3. The decomposition process of leaf litter (A) and branch litter (B) in the three examined forest types. CTF, camphor tree forest; MPF, Masson pine forest; CMF, camphor tree and Masson pine mixed forest.
No significant differences were found in C concentration for all components of litterfall in the examined forests (Table 3). The concentrations of macronutrients in various litterfall components ranked as leaf > miscellaneous > branch in the examined forests, except for P and K, which ranked as miscellaneous > leaf > branch (Table 3). The macronutrient concentrations in all litterfall components were significantly higher in CTF and CMF than in MPF (p = 0.016) (Table 3). The differences in macronutrient concentrations in leaf litter were not significantly different between CTF and CMF except for the Ca element (p = 0.029) (Table 3). However, the macronutrients in branch and miscellaneous components were significantly lower in CMF than in CTF, except for N in the branch litter (Table 3).
TABLE 3. The concentration of carbon and other macronutrients in litterfall components in the three studied forest types (g‧kg−1).
The annual return of macronutrients from litterfall to soil was about 74.2 and 33.6 kg ha−1 yr−1 in CTF and MPF, two times higher than in MPF (Table 4). Total macronutrients returned through litterfall were dominated by the portion of the leaf, contributing about 83, 78, and 83% in CTF, MPF, and CMF stands. Specifically, leaf litter contributed 80, 77, and 85% of total litter N return, 80, 75, and 80% of total litter P return, 75, 72, and 85% of the total litter K return, 83, 82, and 76% of total litter Ca return, and 86, 79, and 83% of total litter Mg return, respectively in CTF, MPF, and CMF. N and Ca elements represented the most returned nutrients from litterfall to soil in the examined forests among the macronutrients. The N and Ca elements accounted for about 44 and 36%, 50 and 29%, and 58 and 22% of the total returned macronutrients in CTF, MPF, and CMF stands, respectively (Table 4). P was the least returned element, and it accounted for only about 2% of the total returned macronutrients in the three forest types. Moreover, a highly significant correlation was observed among all macronutrient turnover rates (Figure 4).
TABLE 4. The annual return of macronutrients from litterfall components in the three examined subtropical forest types (kg‧ha−1‧yr−1).
FIGURE 4. Relationship between the annual return of macronutrients from litterfall among all the forest types and litterfall components. Forests: CTF, camphor tree forest; MPF, Masson pine forest; CMF, camphor tree and Masson pine mixed forest. Litter components: leaf litter, branch litter, and Miscellaneous litter. Pearson correlation is significant at p < 0.05; all the non-significant values are shown with a cross symbol.
In stoichiometry, the C:N, C:P, and N:P ratios were significantly higher in MPF than in CTF and CMF (p < 0.05, Table 5). There were no significant C, N, and P stoichiometry differences between CTF and CMF (p = 0.056). The C:N ratio ranged 38.2–41.5, the C:P ratio ranged 896–1979, and the N:P ratio ranged 23.5–30.5, respectively, in the studied forests. The C:N, C:P, and N:P ratios were higher in the branch litter than in the leaf litter throughout the three forest types (Table 5). Furthermore, C:N and C:P were significantly correlated; however, both were not significantly related to N:P (Figure 5). The time required for 50 and 95% of the litter mass loss in the three examined subtropical forest types has been shown in (Table 6), and it followed the order MPF > CMF > CTF.
TABLE 5. Characteristics of litter CNP stoichiometry in the three examined subtropical forest types.
FIGURE 5. Pearson correlation between the characteristics of litter CNP stoichiometry among all the forest types and litterfall components. Forests: CTF, camphor tree forest; MPF, Masson pine forest; CMF, camphor tree and Masson pine mixed forest. Litter components: leaf litter, branch litter, and miscellaneous litter. Pearson correlation is significant at p < 0.05; all the non-significant values are shown with a cross symbol.
TABLE 6. Time required for 50 and 95% of litter mass loss in the three examined subtropical forest types.
Litterfall content and pattern of litter production vary with tree species, their growth patterns, age, stand density, canopy characteristics, and the environmental factors, including temperature, water, and mineral nutrient availability to limit litter production (Singh et al., 1999; Nakagawa et al., 2019). Our study found that the annual litter production was higher in mixed forest stands than in pure forest stands and ranked as CMF > MPF > CTF. The high litter production in mixed forest stands was likely attributed to species composition. Species in the mixed stand may use site resources more efficiently in producing materials, resulting in greater biomass than monocultures. In addition, forest structure in mixed forest stand created different microclimate in forests (Hansen et al., 2009), and forest structure combined with microclimate resulted in seasonal changes in the amount of total litter, foliage, and twigs (Wang et al., 2008). The competitive production principle may also explain an increase in litter production (Kelty, 2006). These results are within the range of litterfall production reported worldwide in the different forest stands. For example, Augusto et al. (2002) analyzed the litterfall production of several European temperate forests and found that total litterfall production ranged between 3.5–4.0 t ha−1 yr−1. Other studies included various forest types in different regions, such as 3.4 t ha−1 yr−1 in a 30-year-old Norway spruce in South Sweden (Nilsson and Wiklund, 1992), 3.85 t ha−1 yr−1 in an Irish Sitka spruce stand (Carey and Farrell, 1978), and 3.0–3.35 t ha−1 yr−1 in a southern Swedish beech stand (Norde’n, 1994). Wang et al. (2008) reported that the mean annual litterfall in a mixed stand of Cunninghamia lanceolata and Michelia macclurei (699 g m2 yr−1) was 24% higher than the pure stand of C. lanceolata (565 g m2 yr−1) in southern China. Sundarapandian and Swamy (1999) reported that tree species composition was important for litter production within the same climate range. Moreover, in terms of both pure forest comparisons, litter production was higher in MPF than in CTF, which is attributed to forest stand characteristics such as physical and biochemical characteristics of the tree species. The variations in the litterfall production among the plantations were due to the differential litter production capacity for different tree species and their growth habits (Liu et al., 2004). The physiological and morphological differences in species and forest structure combined with microclimate in forests result in seasonal changes in the total litter, foliage, and twigs (Hansen et al., 2009), which has an empirical relationship with litterfall production (Matala et al., 2008).
The seasonal pattern of litterfall production presented that the litterfall in the present study was concentrated during the cool and dry period (December–February) of the year. The monthly minimum litterfall occurred in growing seasons, and maximum litterfall occurred in non-growing seasons in the studied forests. The tendency of litterfall to be concentrated in the cool and dry season is related to declining temperature and lowered soil moisture. The major litterfall coincided with the months between October and December, the low rainy season, and low-temperature months (Yadav et al., 2015). This pattern can be explained by annual cycles of moisture and temperature (Wang et al., 2008) and was comparable with other results in subtropical forest ecosystems (Sundarapandian and Swamy, 1999; Pandey et al., 2007). Pascal (1988) also reported that a heavy leaf litterfall occurred during the dry season in the evergreen forests of Attappadi, India. Research findings demonstrate that the variations of seasonal litterfall coincide with the rhythm of leaf senescence of the forest tree species and the changes in annual environmental parameters (i.e., temperature and moisture) at a regional scale (Sundarapandian and Swamy, 1999). Other climate factors, such as wind and rain, can also increase the amount of branch litter. The foliar litter had more than 70% of the total litterfall production in the three forests of our study. It is consistent with all research findings that most of the litterfall is composed of leaves (Çakır and Akburak, 2017), such as the average leaf litter was 86% in the oak site, 62% in the beech site, and 75% in the oak-beech mixed site (Çakır and Akburak, 2017). The pattern of significant proportion in foliage litter would occur to avoid seasonal moisture and temperature stress during the dry period. Water stress could cause to synthesis of abscisic acid in plants’ foliage, which could stimulate the senescence of leaves and other parts (Moore, 1980). In forests, nutrient concentrations of newly fallen leaf litter often correlate positively with nutrient concentrations of fresh leaves. Leaf litter is the primary and quick source of organic matter and nutrients to the soil compared to other parts of litter available through decomposition.
Litter quality has been considered an important factor in controlling the decomposition rate (Ribeiro et al., 2002; Tateno et al., 2007). The decomposition of tree litter is based on its physical and chemical composition and environmental factors in forest ecosystems. The litter mass loss was more rapid in CTF than in MPF stands due to the favorable conditions in the board-leaved canopy of CTF for fast decomposing litter and soil moisture contents and high biological activity (Isaac et al., 2018). It is similar to the results of Singh et al. (1999) which showed lowering soil moisture and temperature can decrease the activity of decomposer organisms.
The variation in nutrients concentration in the leaf litter of the studied species also contributed to the rate of nutrient uptake and re-translocation; types of leaf shading characteristics (i.e., evergreen, board-leaved, and conifer needle), growth and life form, and characteristics of individual nutrients (Niinemets and Tamm, 2005). In addition, the low leaf C:N and C:P ratios in CTF is a good indicator of the decomposition rate of litterfall (Tripathi et al., 2006). Many studies have suggested that initial N and P contents in the leaf litter are good indicators of the decomposition rate (Sundarapandian and Swamy, 1999; Yang et al., 2004; Tateno et al., 2007). Liao et al. (2000) found that the C. lanceolata leaf litter had a slower decomposition rate than M. macclurei did in the same stand because the initial N and P contents of C. lanceolata leaf litter were significantly lower than that of M. macclurei leaf litter. The decomposition rate was positively correlated with N and P content and negatively correlated with the C/N ratio (Wang et al., 2008). C was generally returned to the soil in the highest amount, followed by N and Ca. No significant differences existed in the returns of C among the three types of forest stands. Our results had a similar nutrient returning pattern to the finding documented by Wang et al. (2008). P return through leaf litter was the lowest (2%) throughout the three forest stand types. Significantly high N and Ca content in leaf litter accounted for over 80% of the total nutrients returning to the soil. The total concentrations of significant nutrient elements N, P, K, Ca, and Mg in foliage and other components of litterfall and the annual nutrients from the litterfall returning into the soil were all higher in CTF than in MPF and CMF. This pattern of nutrient content returning to soil is similar to the pattern of nutrients input N > Ca > K > Mg > P in the rainforest of southwestern Cameroon (Ngaiwi et al., 2018). The macronutrient concentrations in leaf litter showed that N and Ca were the primary elements with higher concentrations in litterfall. It has been indicated that Ca is immobile in the plant vascular system and is believed to be recycled through litter decomposition. Moreover, the high N and low P content in three forest stands resulted in a low C:P ratio, and the high N:P ratio indicated that soil is rich in N content and P deficient (Hobbie et al., 2006; Hansen et al., 2007).
This study suggests that mixed forest significantly increase litter production, the seasonal litterfall accumulation, decomposition rate, and nutrients returning to soil because of the chemical composition and morphological characteristic of evergreen broadleaved camphor tree species. In general, leaf N:P ratios indicate N-limitation or P-limitation in forest ecosystems; the N:P ratio was 24–32 in our study site, indicating that the P is the seriously limited nutrient element in the soil of the forest stands. Our results suggest that the mixed stand of camphor tree with conifer Masson pine stand increased nutrient litter production and nutrient returns and are helping to restore soil fertility.
The raw data supporting the conclusions of this article will be made available by the authors, without undue reservation.
This collaboration work was carried out among all the authors. THF, YP, and XC proposed the original idea. THF and ZL carried out the experiment. THF, UK, and EG wrote the original draft. WY supervised the research work. AS, RS, and XC reviewed the manuscript. SSA, JW, and HMK reviewed the manuscript and provided the funding. All authors read and approved the final submitted version of the manuscript.
This research was financially supported by the National Science and Technology Supporting Project (2015BAD07B00), Forestry Science and Technology Promotion Project of China [no. 12 (2017)], and Taif University Researchers Supporting Project number (TURSP-2020/38), Taif University, Taif, Saudi Arabia.
The authors declare that the research was conducted in the absence of any commercial or financial relationships that could be construed as a potential conflict of interest.
All claims expressed in this article are solely those of the authors and do not necessarily represent those of their affiliated organizations, or those of the publisher, the editors, and the reviewers. Any product that may be evaluated in this article, or claim that may be made by its manufacturer, is not guaranteed or endorsed by the publisher.
The authors thank their friend Chris Ijeoma for the manuscript’s English language and grammar checking. The authors extend their sincere appreciation to Taif University Researchers Supporting Project number (TURSP-2020/38), Taif University, Taif, Saudi Arabia for supporting the current research.
The Supplementary Material for this article can be found online at: https://www.frontiersin.org/articles/10.3389/fenvs.2022.903039/full#supplementary-material
Augusto, L., Ranger, J., Binkley, D., and Rothe, A. (2002). Impact of Several Common Tree Species of European Temperate Forests on Soil Fertility. Ann. For. Sci. 59, 233–253. doi:10.1051/forest:2002020
Bigelow, S. W., and Canham, C. D. (2015). Litterfall as a Niche Construction Process in a Northern Hardwood Forest. Ecosphere 6 (7), 1–14. doi:10.1890/es14-00442.1
Çakır, M., and Akburak, S. (2017). Litterfall and Nutrients Return to Soil in Pure and Mixed Stands of Oak and Beech. J. Fac. For. Istanbul U. 67 (2), 185–200. doi:10.17099/jffiu.301602
Cakır, M., and Makineci, E. (2020). Litter Decomposition in Pure and Mixed Quercus and Fagus Stands as Influenced by Arthropods in Belgrad Forest, Turkey. J. For. Res. 31 (4), 1123–1137. doi:10.1007/s11676-019-00915-y
Carey, M. L., and Farrell, E. P. (1978). Production, Accumulation and Nutrient Content of Sitka Spruce Litterfall. Ir. 35, 35–44. doi:10.1007/bf00344732
Celentano, D., Zahawi, R. A., Finegan, B., Ostertag, R., Cole, R. J., and Holl, K. D. (2010). Litterfall Dynamics under Different Tropical Forest Restoration Strategies in Costa Rica. Biotropica 43, 279–287. doi:10.1111/j.1744-7429.2010.00688.x
Chave, J., Navarrete, D., Almeida, S., Álvarez, E., Aragão, L. E. O. C., Bonal, D., et al. (2010). Regional and Seasonal Patterns of Litterfall in Tropical South America. Biogeosciences 7 (1), 43–55. doi:10.5194/bg-7-43-2010
Dhanya, B., Viswanath, S., and Purushothaman, S. (2013). Decomposition and Nutrient Release Dynamics of Ficus Benghalensis L. Litter in Traditional Agroforestry Systems of Karnataka, Southern India. ISRN For. 2013, 152–173. doi:10.1155/2013/524679
Farooq, T. H., Kumar, U., Mo, J., Shakoor, A., Wang, J., Rashid, M. H. U., et al. (2021c). Intercropping of Peanut-Tea Enhances Soil Enzymatic Activity and Soil Nutrient Status at Different Soil Profiles in Subtropical Southern China. Plants 10 (5), 881. doi:10.3390/plants10050881
Farooq, T. H., Shakoor, A., Rashid, M. H. U., Zhang, S., Wu, P., and Yan, W. (2021b). Annual Growth Progression, Nutrient Transformation, and Carbon Storage in Tissues of Cunninghamia Lanceolata Monoculture in Relation to Soil Quality Indicators Influenced by Intraspecific Competition Intensity. J. Soil Sci. Plant Nutr. 21 (4), 3146–3158. doi:10.1007/s42729-021-00595-w
Farooq, T. H., Xincheng, X., Shakoor, A., Rashid, M. H. U., Bashir, M. F., Nawaz, M. F., et al. (2021d). Spatial Distribution of Carbon Dynamics and Nutrient Enrichment Capacity in Different Layers and Tree Tissues of Castanopsis Eyeri Natural Forest Ecosystem. Environ. Sci. Pollut. Res. 29 (7), 10250–10262. doi:10.1007/s11356-021-16400-1
Farooq, T. H., Yan, W., Chen, X., Shakoor, A., Rashid, M. H. U., Gilani, M. M., et al. (2020). Dynamics of Canopy Development of Cunninghamia Lanceolata Mid-age Plantation in Relation to Foliar Nitrogen and Soil Quality Influenced by Stand Density. Glob. Ecol. Conservation 24, e01209. doi:10.1016/j.gecco.2020.e01209
Farooq, T., Shakoor, A., Wu, X., Li, Y., Rashid, M., Zhang, X., et al. (2021a). Perspectives of Plantation Forests in the Sustainable Forest Development of China. iForest 14 (2), 166–174. doi:10.3832/ifor3551-014
Farooq, T., Tigabu, M., Ma, X., Zou, X., Liu, A., Odén, P., et al. (2018). Nutrient Uptake, Allocation and Biochemical Changes in Two Chinese Fir Cuttings under Heterogeneous Phosphorus Supply. iForest 11 (3), 411–417. doi:10.3832/ifor2657-011
Gilani, M. M., Tigabu, M., Liu, B., Farooq, T. H., Rashid, M. H. U., Ramzan, M., et al. (2021). Seed Germination and Seedling Emergence of Four Tree Species of Southern China in Response to Acid Rain. J. For. Res. 32 (2), 471–481. doi:10.1007/s11676-020-01102-0
Hansen, K., Vesterdal, L., Bastrup-Birk, A., and Bille-Hansen, J. (2007). Are Indicators for Critical Load Exceedance Related to Forest Condition? Water Air Soil Pollut. 183, 293–308. doi:10.1007/s11270-007-9377-1
Hansen, K., Vesterdal, L., Schmidt, I. K., Gundersen, P., Sevel, L., Bastrup-Birk, A., et al. (2009). Litterfall and Nutrient Return in Five Tree Species in a Common Garden Experiment. For. Ecol. Manag. 257, 2133–2144. doi:10.1016/j.foreco.2009.02.021
Hobbie, S. E., Reich, P. B., Oleksyn, J., Ogdahl, M., Zytkowiak, R., Hale, C., et al. (2006). Tree Species Effects on Decomposition and Forest Floor Dynamics in a Common Garden. Ecology 87, 2288–2297. doi:10.1890/0012-9658(2006)87[2288:tseoda]2.0.co;2
Huang, Y., Ma, Y., Zhao, K., Niklaus, P. A., Schmid, B., and He, J.-S. (2017). Positive Effects of Tree Species Diversity on Litterfall Quantity and Quality along a Secondary Successional Chronosequence in a Subtropical Forest. J. Plant Ecol. 10, 28–35. doi:10.1093/jpe/rtw115
Isaac, S. R., Pushpakumari, R., and Varghese, J. (2018). Structural Diversity, Leaf Litter Recycling and Allelopathic Influences of Leaf Litter in an Agroforestry Homegarden of Southern Kerala. Internatio. Journ. Far. Scien. 8 (4), 81–87. doi:10.5958/2250-0499.2018.00111.8
Kelty, M. J. (2006). The Role of Species Mixtures in Plantation Forestry. For. Ecol. Manag. 233, 195–204. doi:10.1016/j.foreco.2006.05.011
Khanna, P. K., Fortmann, H., Meesenburg, H., Eichhorn, J., and Meiwes, K. J. (2009). “Biomass and Element Content of Foliage and Aboveground Litterfall on the Three Long-Term Experimental Beech Sites: Dynamics and Significance,” in Functioning and Management of European Beech Ecosystems. Berlin, Heidelberg: Springer, 183–205. doi:10.1007/b82392_12
Khiewtam, R. S., and Ramakrishnan, P. S. (1993). Litter and Fine Root Dynamics of a Relict Sacred Grove Forest at Cherrapunji in North-Eastern India. For. Ecol. Manag. 60, 327–344. doi:10.1016/0378-1127(93)90087-4
Liao, L. P., Wang, S. L., and Chen, C. Y. (2000). Dynamics of Litterfall in the Mixed Plantation of Cunninghamia Lanceolata and Michelia Macclurei: a Ten Year's Observation. Chin. J. Appl. Ecol. 11, 131–136. (In Chinese).
Liski, J., Palosuo, T., Peltoniemi, M., and Sievänen, R. (2005). Carbon and Decomposition Model Yasso for Forest Soils. Ecol. Modell. 189 (1-2), 168–182. doi:10.1016/j.ecolmodel.2005.03.005
Liu, C., Berg, B., Kutsch, W., Westman, C. J., Ilvesniemi, H., Shen, X., et al. (2006). Leaf Litter Nitrogen Concentration as Related to Climatic Factors in Eurasian Forests. Glob. Ecol. Biogeogr. 15, 438–444. doi:10.1111/j.1466-822x.2006.00251.x
Liu, C., Westman, C. J., Berg, B., Kutsch, W., Wang, G. Z., Man, R., et al. (2004). Variation in Litterfall-Climate Relationships between Coniferous and Broadleaf Forests in Eurasia. Glob. Ecol. Biogeogr. 13, 105–114. doi:10.1111/j.1466-882x.2004.00072.x
Martius, C., Höfer, H., Garcia, M. V. B., Römbke, J., and Hanagarth, W. (2004). Litter Fall, Litter Stocks and Decomposition Rates in Rainforest and Agroforestry Sites in Central Amazonia. Nutrient Cycl. Agroecosyst. 68 (2), 137–154. doi:10.1023/b:fres.0000017468.76807.50
Matala, J., Kellomäki, S., and Nuutinen, T. (2008). Litterfall in Relation to Volume Growth of Trees: Analysis Based on Literature. Scand. J. For. Res. 23, 194–202. doi:10.1080/02827580802036176
Melillo, J. M., Naiman, R. J., Aber, J. D., and Linkins, A. E. (1984). Factors Controlling Mass Loss and Nitrogen Dynamic of Plant Litter Decaying in Northern Streams. Bull. Mar. Sci. 35, 341–356.
Nakagawa, M., Ushio, M., Kume, T., and Nakashizuka, T. (2019). Seasonal and Long‐term Patterns in Litterfall in a Bornean Tropical Rainforest. Ecol. Res. 34 (1), 31–39. doi:10.1111/1440-1703.1003
Nakane, K. (1995). Soil Carbon Cycling in a Japanese Cedar (Cryptomeria Japonica) Plantation. For. Ecol. Manag. 72, 185–197. doi:10.1016/0378-1127(94)03465-9
Neumann, M., Ukonmaanaho, L., Johnson, J., Benham, S., Vesterdal, L., Novotný, R., et al. (2018). Quantifying Carbon and Nutrient Input from Litterfall in European Forests Using Field Observations and Modeling. Glob. Biogeochem. Cycles 32 (5), 784–798. doi:10.1029/2017gb005825
Ngaiwi, M. E., Molua, E. L., and Egbe, A. E. (2018). Litterfall and Nutrient Returns in the Rainforest of Southwestern Cameroon: Some Implications for Tropical Forest Productivity. Enrr 8, 25–32. doi:10.5539/enrr.v8n3p25
Niinemets, U., and Tamm, U. (2005). Species Differences in Timing of Leaf Fall and Foliage Chemistry Modify Nutrient Resorption Efficiency in Deciduous Temperate Forest Stands. Tree Physiol. 25, 1001–1014. doi:10.1093/treephys/25.8.1001
Nilsson, L.-O., and Wiklund, K. (1992). Influence of Nutrient and Water Stress on Norway Spruce Production in South Sweden ? the Role of Air Pollutants. Plant Soil 147, 251–265. doi:10.1007/bf00029077
Norde' n, U. (1994). Leaf Litterfall Concentrations and Fluxes of Elements in Deciduous Tree Species. Scand. J. For. Res. 9, 9–16.
Olson, J. S. (1963). Energy Storage and the Balance of Producers and Decomposers in Ecological Systems. Ecology 44, 322–331. doi:10.2307/1932179
Pandey, R. R., Sharma, G., Tripathi, S. K., and Singh, A. K. (2007). Litterfall, Litter Decomposition and Nutrient Dynamics in a Subtropical Natural Oak Forest and Managed Plantation in Northeastern India. For. Ecol. Manag. 240, 96–104. doi:10.1016/j.foreco.2006.12.013
Pascal, J. P. (1988). Wet Evergreen Forests of the Western Ghats of India. Inst. Fr. Pondicherry. Trav. Sec. Sci. Tech. Tome 20, 281–292.
Pretzsch, H., Block, J., Dieler, J., Dong, P. H., Kohnle, U., Nagel, J., et al. (2010). Comparison between the Productivity of Pure and Mixed Stands of Norway Spruce and European Beech along an Ecological Gradient. Ann. For. Sci. 67 (7), 712. doi:10.1051/forest/2010037
Rashid, M. H. U., Tigabu, M., Chen, H., Farooq, T. H., Ma, X., and Wu, P. (2020). Calcium-mediated Adaptive Responses to Low Phosphorus Stress in Chinese Fir. Trees 34 (3), 825–834. doi:10.1007/s00468-020-01961-4
Ribeiro, C., Madeira, M., and Araújo, M. C. (2002). Decomposition and Nutrient Release from Leaf Litter of Eucalyptus Globulus Grown under Different Water and Nutrient Regimes. For. Ecol. Manag. 171, 31–41. doi:10.1016/s0378-1127(02)00459-0
Sayer, E. J. (2006). Using Experimental Manipulation to Assess the Roles of Leaf Litter in the Functioning of Forest Ecosystems. Biol. Rev. Camb Philos. Soc. 81, 1–31. doi:10.1017/S1464793105006846
Singh, K. P., Singh, P. K., and Tripathi, S. K. (1999). Litterfall, Litter Decomposition and Nutrient Release Patterns in Four Native Tree Species Raised on Coal Mine Spoil at Singrauli, India. Biol. Fertil. Soils 29, 371–378. doi:10.1007/s003740050567
Staelens, J., Nachtergale, L., Luyssaert, S., and Lust, N. (2003). A Model of Wind-Influenced Leaf Litterfall in a Mixed Hardwood Forest. Can. J. For. Res. 33 (2), 201–209. doi:10.1139/x02-174
Sundarapandian, S. M., and Swamy, P. S. (1999). Litter Production and Leaf-Litter Decomposition of Selected Tree Species in Tropical Forests at Kodayar in the Western Ghats, India. For. Ecol. Manag. 123, 231–244. doi:10.1016/s0378-1127(99)00062-6
Tateno, R., Tokuchi, N., Yamanaka, N., Du, S., Otsuki, K., Shimamura, T., et al. (2007). Comparison of Litterfall Production and Leaf Litter Decomposition between an Exotic Black Locust Plantation and an Indigenous Oak Forest Near Yan'an on the Loess Plateau, China. For. Ecol. Manag. 241, 84–90. doi:10.1016/j.foreco.2006.12.026
Tripathi, S. K., Sumida, A., Shibata, H., Ono, K., Uemura, S., Kodama, Y., et al. (2006). Leaf litterfall and decomposition of different above- and belowground parts of birch (Betula ermanii) trees and dwarf bamboo (Sasa kurilensis) shrubs in a young secondary forest in Northern Japan. Biol. Fertil. Soils 43, 237–246. doi:10.1007/s00374-006-0100-y
Wang, Q., Wang, S., and Huang, Y. (2008). Comparisons of Litterfall, Litter Decomposition and Nutrient Return in a Monoculture Cunninghamia Lanceolata and a Mixed Stand in Southern China. For. Ecol. Manag. 255, 1210–1218. doi:10.1016/j.foreco.2007.10.026
Wu, C., Hong, W., and Jiang, Z. (2000). Advances in Research of Forest Litter-Fall in China. Acta Agri 90, 405–410. (In Chinese).
Wu, P., Wang, G., Farooq, T. H., Li, Q., Zou, X., and Ma, X. (2017). Low Phosphorus and Competition Affect Chinese Fir Cutting Growth and Root Organic Acid Content: Does Neighboring Root Activity Aggravate P Nutrient Deficiency? J. Soils Sediments 17 (12), 2775–2785. doi:10.1007/s11368-017-1852-8
Yadav, R. P., Bisht, J. K., and Pandey, B. M. (2015). Above Ground Biomass and Carbon Stock of Fruit Tree Based Land Use Systems in Indian Himalaya. Ecoscan 9 (3&4), 779–783.
Keywords: Litterfall, Decomposition, Macronutrients, Decay rate, Stoichiometry, Subtropical forest
Citation: Farooq TH, Li Z, Yan W, Shakoor A, Kumar U, Shabbir R, Peng Y, Gayathiri E, Alotaibi SS, Wróbel J, kalaji HM and Chen X (2022) Variations in Litterfall Dynamics, C:N:P Stoichiometry and Associated Nutrient Return in Pure and Mixed Stands of Camphor Tree and Masson Pine Forests. Front. Environ. Sci. 10:903039. doi: 10.3389/fenvs.2022.903039
Received: 23 March 2022; Accepted: 11 May 2022;
Published: 27 June 2022.
Edited by:
Balasubramani Ravindran, Kyonggi University, South KoreaReviewed by:
Junaid Ali Siddiqui, South China Agricultural University, ChinaCopyright © 2022 Farooq, Li, Yan, Shakoor, Kumar, Shabbir, Peng, Gayathiri, Alotaibi, Wróbel, kalaji and Chen. This is an open-access article distributed under the terms of the Creative Commons Attribution License (CC BY). The use, distribution or reproduction in other forums is permitted, provided the original author(s) and the copyright owner(s) are credited and that the original publication in this journal is cited, in accordance with accepted academic practice. No use, distribution or reproduction is permitted which does not comply with these terms.
*Correspondence: Wende Yan, Y3NmdXl3ZEBob3RtYWlsLmNvbQ==; Xiaoyong Chen, eGNoZW5AZ292c3QuZWR1
Disclaimer: All claims expressed in this article are solely those of the authors and do not necessarily represent those of their affiliated organizations, or those of the publisher, the editors and the reviewers. Any product that may be evaluated in this article or claim that may be made by its manufacturer is not guaranteed or endorsed by the publisher.
Research integrity at Frontiers
Learn more about the work of our research integrity team to safeguard the quality of each article we publish.