- 1Department of Animal Ecology and Systematics, Justus Liebig University Giessen, Giessen, Germany
- 2Naturalis Biodiversity Center, Leiden, Netherlands
- 3Département de Biologie-Chimie, Unité d’Enseignement et de Recherche en Hydrobiologie Appliquée (UERHA), Section des Sciences Exactes, Institut Supérieur Pédagogique de Bukavu, Bukavu, Democratic Republic of Congo
- 4Centre de Recherche en Environnement et Géo-Ressources, Université Catholique de Bukavu (UCB), Bukavo, Democratic Republic of Congo
- 5Department of Biology, Mbarara University of Science and Technology (MUST), Mbarara, Uganda
The Ruzizi River, the outlet of Lake Kivu in the Albertine Rift, flows into Lake Tanganyika and is important for hydropower generation and irrigation. The impacts of 2 dams in the Ruzizi River on macroinvertebrate community composition and diversity were surveyed every 3 months from December 2015 to October 2017. Macroinvertebrate samples were collected at sites upstream and downstream and additionally at two sites further downstream of the dams, in both comparatively pristine and highly disturbed areas. Several indices (Shannon-Wiener index, Simpson index, Pielou’s evenness, Rare Family Prevalence, and Average Score Per Taxa) were used to determine the alpha diversity and evenness of macroinvertebrates at the family level. Our results showed little to no immediate effect of the dams on macroinvertebrate diversity. Macroinvertebrate composition differed slightly below the dams compared to upstream. Communities near Dam II had slightly higher diversity compared to Dam I, probably because the vicinity to Lake Kivu has an immediate effect on diversity upstream of the first dam and likely because Dam II is 30 years younger than Dam I. This study suggests the importance of using species-level indices to better understand the ecological impacts of dams on macroinvertebrate diversity of tropical rivers with low species diversity.
Introduction
Increasing demand for electricity creates an urgent need to build hydropower plants for renewable energy and as a valuable source of revenue (IEA: International Energy Agency, 2019), especially in tropical regions where the world’s largest rivers are located (Latrubesse et al., 2005; Sinha et al., 2012). The demand for hydropower in such regions has even led to a global “megadam mania” (Gross, 2016). In addition to hydropower, dams provide many benefits to societies such as flood control, irrigation, and water level regulation (Altinbilek, 2002). Despite the great importance of dams, they alter rivers and their ecosystems significantly (Poff and Matthews, 2013; Zarfl et al., 2015; Couto and Olden, 2018; Grill et al., 2019) by changing the flow regime, e.g., reducing river connectivity, altering temperature and nutrient status (Bunn and Arthington, 2002; Renöfält et al., 2010; Reid et al., 2019; Kuriqi et al., 2019, 2020). These hydrological changes and habitat fragmentation are mainly related to loss of sediment connectivity, resulting in significant changes in downstream sections and affecting macroinvertebrate communities (Mueller et al., 2011; Martínez et al., 2013). In addition to the mentioned threats, dams can facilitate the establishment of invasive species that could further drive the loss of other aquatic organisms (Dick et al., 2002; Johnson et al., 2008).
Aquatic macroinvertebrates are the most popular organisms used to assess freshwater biological quality (Wright, 2010; Kaaya et al., 2015; Wronski et al., 2015; Dusabe et al., 2019), especially in the stream and river assessments (Dallas, 2021). Macroinvertebrates are recognized as good indicators for monitoring habitat quality (Greenwood et al., 1999; Carter et al., 2017) as well as many different anthropogenic stressors, including changes in flow regime, pollution, eutrophication, and biological invasions (Bonada et al., 2006; Fornaroli et al., 2018; Guareschi and Wood, 2019; Mellado-Díaz et al., 2019).
The effects of dams on downstream macroinvertebrate diversity and abundance have been documented repeatedly (Cortes et al., 1998; Santucci et al., 2005; Sharma et al., 2005; Xiaocheng et al., 2008; Bredenhand and Samways, 2009; Vaikasas et al., 2013; Serrana et al., 2018; Wang et al., 2019). Species richness and abundance of sensitive and tolerant taxa have been the most commonly used metrics to determine the ecological impacts of macroinvertebrates below dams (Martínez et al., 2013). Using biotic indicators to detect the effects of dams on the macroinvertebrate community requires a profound knowledge of the species identities because even closely related species may have different tolerances to environmental stressors (Macher et al., 2016; Mezgebu, 2022). However, accurate identification of freshwater macroinvertebrates to the species level can be difficult, especially for larval or subadult specimens, and often results in low taxonomic resolution or misidentification (Haase et al., 2010; Sweeney et al., 2011). This in turn reduces the accuracy of the approach and can lead to inaccurate biological assessments and eventually even misguided management (Stein et al., 2014).
A promising alternative to morphological identification is DNA-based identification (Elbrecht et al., 2017), which has been shown to be reliable in non-tropical regions (Stein et al., 2013; Elbrecht and Leese, 2015). Nevertheless, most available bio-indication systems are nowadays based on the family taxonomic level (Dallas, 2021; Mezgebu, 2022). The objective of this research is to evaluate whether a family-level bio-indication can determine the impact of dams on macroinvertebrates in a low-diversity tropical river.
Materials and Methods
Study Area
The Ruzizi River, also known as Rusizi, flows from Lake Kivu into Lake Tanganyika (Figure 1). It is the only outlet of Lake Kivu and one of the most important tributaries of Lake Tanganyika in the Congo Basin and lies between the Democratic Republic of Congo (DRC) and Rwanda on the one hand and DRC and Burundi on the other (Descy et al., 2012). The Ruzizi River has an average long-term annual flow of about 86 m³/s (Muvundja et al., 2014; ABAKIR, 2020). For the first 50 km from Lake Kivu to the village of Kamanyola (headwaters), the river lies between the steep, heavily deforested, and barren watersheds (upper Ruzizi) of South Kivu in the D.R. Congo and Rusizi District in Rwanda. After crossing the escarpment, the river drops from an elevation of 1,450 m to 962 m (ABAKIR, 2020). Numerous waterfalls make it a potential hydropower source. After the escarpments (Ngomo in DRC and Nzahaha in Rwanda), the Ruzizi River extends into a vast plain and gradually drops from an elevation of 962–770 m with a low average gradient before entering Lake Tanganyika. The river provides important habitat for a variety of aquatic species (Hughes and Hughes, 1992). The Ruzizi water at the first dam has a high salt concentration (∼1.1 g/L or 1,200 μS/cm electrical conductivity), being as is as salty as the surface waters of Lake Kivu due to dissolution of volcanic ashes in most rivers of North Kivu and subaquatic discharge of underground hydrothermal springs. Towards the Lower Ruzizi in Kiliba, the river water freshens considerably (∼0.5 g/L or 650 μS/cm) due to further freshwater inputs from the watershed (Muvundja et al., 2022). The Ruzizi River is of outstanding importance to the African Great Lakes Region (DRC, Rwanda, and Burundi) because of hydroelectric power generation. There are two active hydropower dams on the river: the first dam built in 1959 is located 3 km downstream of the outlet of Lake Kivu at Mururu at an altitude of 1,460 m a.s.l. It has an installed capacity of 28 MW (TRACTIONEL and RRI, 1980; Fichtner Gmbh und co, 2008). The second dam was built in 1989 and is located about 16 km from Bukavu in Mumosho at an altitude of 1,393 m a.s.l, with a capacity of 44 MW (Figure 1) (TRACTIONEL and RRI, 1980; Fichtner Gmbh und co, 2008; ABAKIR, 2020). Two more dams are planned: Dam III (147 MW) and Dam IV, to be built downstream of Dam II and between dams II and III, respectively (Fichtner Gmbh und co, 2008; Dombrowsky et al., 2014; ONEC-BAD, 2015; ABAKIR, 2020).
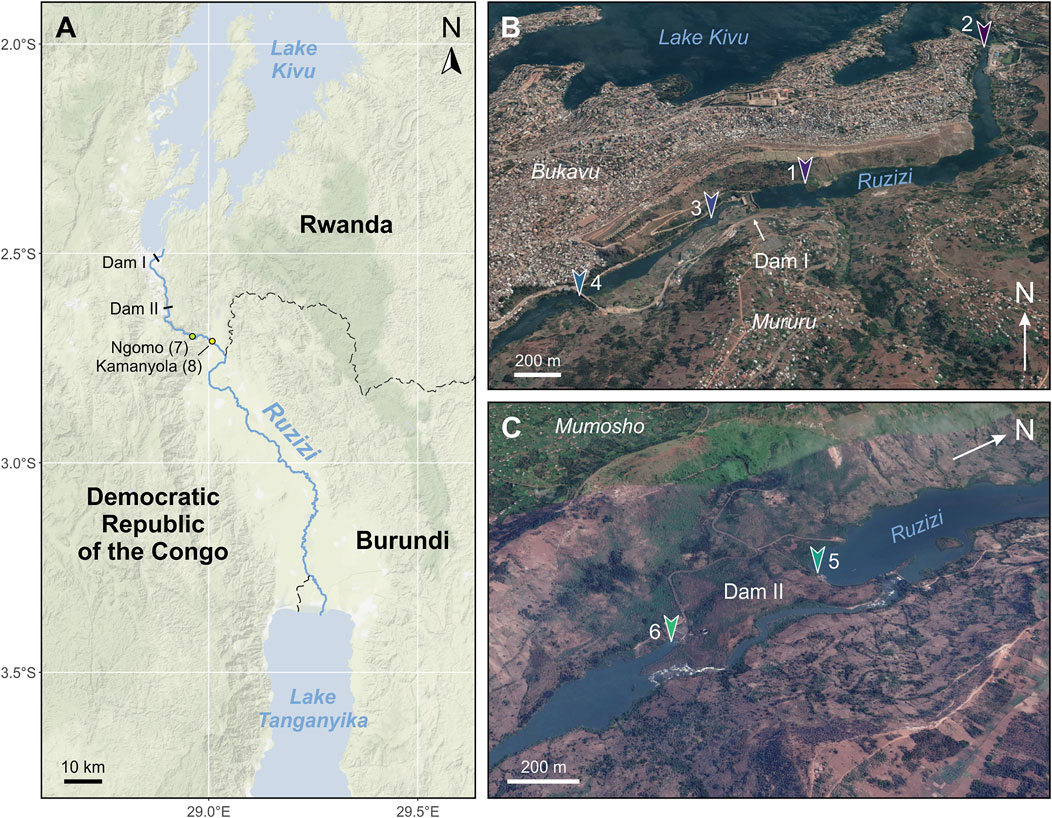
FIGURE 1. Location of the Ruzizi River, the dams, and collecting sites. See Table 1 for detailed information on the localities. (A) Ruzizi River with Dam I and Dam II and further downstream sites. (B, C) Sampling site locations downstream and upstream of Dam I (between Mururu and Bukavu) and Dam II (in Mumosho). The map in (A) was created with the R package ggmap 3.0.0 (Kahle et al., 2019). (B, C) were created in Google Earth Pro 7.3.4.8248, map data © 2022 Google/Maxar Technologies.
Macroinvertebrates Sampling
We sampled the macroinvertebrate community at eight stations from December 2015 to August 2017 (Supplementary Table S1). Samples were collected every 3 months to cover both wet and dry seasons. Each collection site was sampled eight times to account for a potential variation in community composition over time. Samples were collected at sites upstream and downstream of Dam I and Dam II. Additional samples were collected further downstream of Dam II at Manda/Ngomo and Kamanyola as reference sites that are unlikely to be impacted by the dams. We collected macroinvertebrates in various habitats by kicking, hand picking, or hand scooping samples for leaf litter and sapropel (Dusabe et al., 2019). We collected the samples on the banks of the Ruzizi River in different substrates (sand, stones, rocks, and macrophytes) by using hand tweezers on stones and rocks and scoop nets (diameter: 20 cm, mesh size: 1 mm) in sands and macrophytes and on the water surface as well as in the water column. Organisms were separated by taxonomic groups, sorted using featherweight tweezers.
At each sampling site, the samples were collected within 50 m along the river shoreline. Some sites were accessible by foot, others by boat. Latitude, longitude, and elevation were recorded at each sampling site using a Garmin GPS IV (Table 1). Sampling lasted 60 min and was conducted by three individuals. Samples were preserved with 70% ethanol. All macroinvertebrates were identified to family level using predominantly keys developed for the southern African sub-region (Cape Province to northern Zambia; Day et al., 1999, 2001a, 2001b, 2002; Day and de Moor, 2002; de Moor and Day, 2002; Stals and de Moor, 2007; de Moor et al., 2003). Oligochaeta and Polychaeta were determined to order level due to the lack of identification keys for the region.

TABLE 1. Locality information with the villages where Dam I and Dam II are located and coordinates of sampling sites upstream and downstream of the dams.
Data Analyses
We calculated five different diversity indices: Shannon-Wiener index, Simpson index, Pielou’s Evenness, Rare Family Prevalence (RFP) index, which indicates the proportion of families at each station represented by single individuals (Emberton et al., 1997), and Average Score Per Taxa (ASPT) based on TARISS (Tanzania Rivers Scoring System; Kaaya et al., 2015) to categorize the sensitive and tolerant taxa (Kaaya et al., 2015). The ASPT scores of macroinvertebrate groups were categorized as follows: Low sensitivity (1–5), moderate sensitivity (6–10), and high sensitivity (11–15) (Gerber and Gabriel, 2002).
In order to test for an effect of the sampling position with respect to dams (upstream vs. downstream) on each of the five diversity indices we ran linear mixed effects models. We divided the analyses into two batches, one including only the samples upstream and downstream of Dams I and II, respectively, the second one including the samples taken further downstream at Ngomo and Kamanyola (Figure 1) as a separate category. The rationale behind the second approach was to compare upstream/downstream samples to communities less impacted by dam building. The communities at Ngomo and Kamanyola are located several kilometers downstream of Dam II and are distinct from the communities downstream and upstream of Dam I and Dam II. This could be due to the streams that join the Ruzizi River upstream of the Ngomo site and therefore bring new macroinvertebrate communities. Kamanyola is disturbed by several anthropogenic stressors because the site is located on the Congo-Rwanda border in an area with a high population density, causing the river to be used heavily for domestic purposes and agriculture.
We tested for the influence of two different types of random effect structures, one including the sampling period (month/year), the other including sampling period and sampling area (Dam I, Dam II, and Ngomo/Kamanyola). In all cases we applied random intercept models. Preliminary analyses with more complex random effect designs yielded distinctly less supported or singular models.
We used Akaike Information Criterion (AIC) to select the best model, in each case comparing a candidate model with the corresponding null model lacking fixed effects but having the same random structure. Following Harrison et al. (2018) we used maximum likelihood estimation to compare models with the same random structure, but restricted maximum likelihood to estimate variance components of random effects and model parameters as well as to compare models with different random structure. We applied the classical ΔAIC cutoff of 2 after Burnham and Anderson (2004) to evaluate significant difference among models, but we are aware of the problems associated with such an assumption (compare Harrison et al., 2018) and critically discuss this issue below.
Models that were found significantly better than the corresponding null models were further examined for model adequacy and model fit. We assessed model residuals through simulations that transform them to a standardized scale, tested for potential dispersion issues, and inspected autocorrelation function plots to detect a possible influence of temporal autocorrelation. Marginal R2 and adjusted intraclass correlation coefficient (ICC) were calculated to assess the variances explained by the fixed and random effects, respectively (Nakagawa et al., 2017; Harrison et al., 2018).
Finally, to compare communities among sampling sites and periods, we ran a nonmetric multidimensional scaling based on a Bray-Curtis dissimilarity matrix for each sampling period. To account for strong variation in abundances, both Wisconsin double standardization and square-root transformation were applied.
All analyses were carried out in R vs. 4.0.3 (R Core Team, 2020) using the packages DHARMa 0.3.3.0 (Hartig and Lohse, 2020), ggeffects 1.0.1 (Lüdecke et al., 2020a), lme4 1.1–26 (Bates et al., 2020), performance 0.6.1 (Lüdecke et al., 2020b), and vegan 2.5–7 (Oksanen et al., 2020).
Results
A total of 4,439 individuals from 13 macroinvertebrate orders were detected across all sampling sites and periods (Supplementary Table S1). Mollusca formed the dominant group (40.9%), followed by Odonata (17.8%) and Diptera (13.4%). Other groups found in this study were Trichoptera (7.7%), Ephemeroptera (5.9%), Heteroptera (5.5%), Decapoda (3.9%), Coleoptera (2.2%), Hirudinea (1.2%), Plecoptera (0.72%), and Oligochaeta (0.2%). The composition of the macroinvertebrate community slightly varied across sites. Almost all groups were represented at all sites, but Trichoptera, Ephemeroptera, and Plecoptera dominated at the control site (Ngomo) of the Ruzizi River.
In general, the Shannon-Wiener index was very low, ranging from 0.63 to 2.39. Most families at the sites were represented by more than one individual. The Rare Family Prevalence (RFP) score was low at most sites before and after the dams. At one site from Dam I downstream (loc. 4), a very low RFP value of 0.8 was recorded in December 2015. The highest RFP value (60) was recorded in October 2016 at Kamanyola, where most of the taxa collected were represented by single individuals. The evenness was high when RFP increased, meaning that the number of individuals in a community was fairly constant when many families were represented by single individuals. The ASPT ranged from 2.5 to 6.8, and almost all sites were represented by tolerant and moderately tolerant taxa, except for the Ngomo site, which had more sensitive taxa.
The nonmetric multidimensional scaling analyses for the eight sampling periods yielded stress values between 0.016 and 0.097, suggesting a good fit for each of the ordinations. The plots indicate variation among communities as well as through time (Supplementary Figure S1). The plots showed that the least sensitive taxa were represented both upstream and downstream of the 2 dams. Belostomatidae, Libellulidae, Lymnaeidae, and Bithyniidae dominated upstream of Dam I, while Planorbidae, Lymnaeidae, and Atyidae dominated downstream (Supplementary Figure S1). Sites at Dam II were more represented with taxa moderately sensitive to pollution and disturbance. Corduliidae and Chlorocyphidae dominated upstream of Dam II, while Hydraenidae and Belostomatidae dominated downstream of Dam II (Supplementary Figure S1). Community composition differed at sites further downstream. The Ngomo site was dominated by sensitive taxa of Trichoptera (Hydropsychidae), Plecoptera (Perlidae), and Ephemeroptera (Baetidae). The Kamanyola site, further downstream, was dominated by taxa that are both tolerant and moderately tolerant to pollution and disturbance, such as Nepidae, Libellulidae, and Gomphidae.
For most of the linear mixed effects models between each of the five diversity indices and sampling position (upstream vs. downstream), a null model yielded a better or equally good (ΔAIC <2) fit (Supplementary Table S2). Moreover, despite the generally simple structures of the models, several of them had a singular fit (Supplementary Table S2), suggesting an overfitting of the model or insufficient data. Since the structure of the models cannot be further reduced reasonably, these models will not be further considered. Only five models were found better than their corresponding null models (Table 2). However, ΔAIC values for four out of the five model are below 5, suggesting only a weakly better fit than a null model. Upstream/downstream position had significant but weak effects on Pielou’s Evenness and Rare Family Prevalence (RFP) when including only Dams I and II. However, most of the variation detected by the models is due to the random effects structure, particularly the sampling area. Conversely, a moderate effect of upstream/downstream position on ASPT was found when including the communities further downstream of Dam II (Table 2; Figure 2). As in the other models, sampling area contributed more to the variation of the models than sampling period. Residual and dispersion checks found no violation of model assumptions in any of the five models (Supplementary Results S1). Only minor signs of temporal autocorrelation were detected for the two models with ASPT (Supplementary Results S1).

TABLE 2. Linear mixed effects models that are better than their corresponding null models, with indication of random effects structure (random intercept) and model fit. See Supplementary Table S2 for the complete list and Supplementary Results S1 for model diagnostics. AIC, Akaike Information Criterion; ASPT, average score per taxon; ICC, intraclass correlation coefficient; RFP, rare family prevalence.
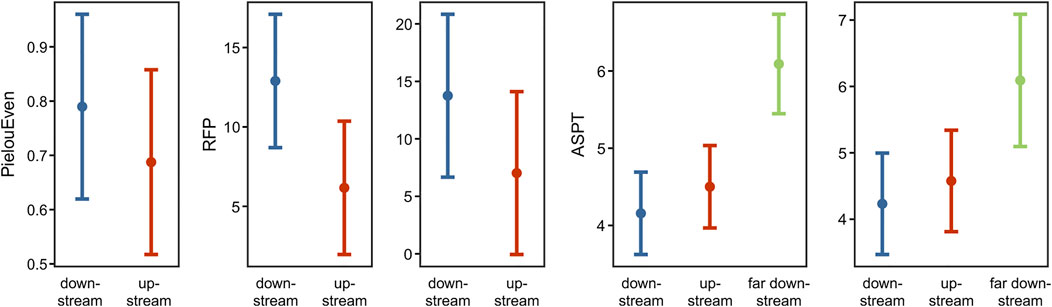
FIGURE 2. Predicted values of the fixed effects of the five significant linear mixed effects models. The fixed effects of the ASPT models show that the variance in both models is mostly contributed by the communities sampled further downstream of Dam II. ASPT, average score per taxon; RFP, rare family prevalence.
Discussion
The studied river system provided an opportunity to examine whether family-level biotic indices should be used to assess the ecological impacts of dams, particularly in a low-diversity river. We found low-diverse macroinvertebrate communities both upstream and downstream of the dams. Our analyses indicated general differences in the community compositions through space and time (Supplementary Figure S1), but upstream/downstream communities were found significantly different only for few selected indices (Table 2; Figure 2).
There are several potential causes for the low macroinvertebrate diversity in the Ruzizi River. Generally, factors contributing to low taxon richness can be low habitat diversity, unstable water levels, altered thermal regime, and altered food supply (Munn and Brusven, 1991). Moreover, macroinvertebrate assemblages below dams often have lower taxon richness and are typically dominated by certain species (Bona et al., 2008; Takao et al., 2008). In the specific case of the Ruzizi River, the hydrological and geographical setting of the dams is also relevant. The water predominantly comes from Lake Kivu, which is a species-poor lake because of its geological history and catastrophic volcanic events that affect also its current limnology (Jones, 2021). The lake’s environmental condition affects the Ruzizi River, especially at Dam I close to the outlet. We found that the habitat is disturbed and degraded by high water release and retention downstream of dams, leading to hydropeaking events (Tonolla et al., 2017; Muvundja et al., 2022). Sometimes water is retained upstream, dramatically altering the river’s habitat to the point of complete dry-up downstream. This kills many small organisms such as macroinvertebrates due to their low mobility (Bruder et al., 2016). Conversely, habitat alteration due to flooding is the proposed cause of low macroinvertebrate diversity in the Ruzizi River just upstream of the dams (Hyangya et al., 2014).
The slight difference in macroinvertebrate diversity between sites near dams I and II could be due to the proximity of Dam I to Lake Kivu, which carries the same saline water and therefore has lower diversity than communities around Dam II, whose water is diluted by tributaries (Muvundja et al., 2022). The age difference in their existence could be another factor contributing to the lower diversity at Dam I compared to Dam II, which was constructed 30 years later. For the benthic macroinvertebrates, the impacts may be more noticeable in the short and medium-term due to their reduced capacity for movement and their affinity for the bottom substrates that constitute their living environment (Bhandari et al., 2018; Min and kong, 2020). Our result, however, is consistent with a study that showed that dams of different ages can affect downstream organisms differently, with older dams having a greater impact than those recently built along the same river (Wang et al., 2020).
Additionally to richness differences, we found differences in the community compositions. This especially concerns the relative abundance of sensitive and tolerant taxa. In general, at the sites near Dam I and Dam II, between December 2015 and October 2017, we observed an increase of families with low TARISS scores (representing species that tolerate pollution and disturbance), particularly Mollusca, Diptera (strongly represented by Chironomidae), and Heteroptera (compare Kaaya et al., 2015), while species that are highly sensitive to pollution (such as Trichoptera, Ephemeroptera, and Plecoptera; Kaaya et al., 2015) were rare both upstream and downstream of the dams. We observed considerable variation in flow rate and water level over the studied time interval. Such hydropeaking and drying events usually cause changes in macroinvertebrate communities and decrease the number of sensitive taxa in impacted areas (Wang et al., 2013).
Another factor causing the rarity of highly sensitive taxa could be river bank disturbance and domestic pollution observed upstream and downstream of the dams. Macroinvertebrates are known to be differentially sensitive to water quality degradation (Bonada et al., 2006; Arimoro and Muller, 2010; Fouche and Vlok, 2010). Their presence is therefore considered an indicator of the state of water quality and aquatic health of the environment in which they live. The disturbance and domestic utilization of water at the shores of sites of Dam II were minimal compared to the sites of Dam I. Dam II sites contained more taxa moderately sensitive to pollution and disturbance. Taxa tolerant or moderately tolerant to disturbance dominated at sites further downstream in Kamanyola on the Rwanda-Congo border. The low abundance of sensitive taxa at this site, which is not directly impacted by dams and related flow fluctuations, may have been caused by further anthropogenic activities (such as swimming, washing), agriculture, nearby irrigation, and pollution of the on-site bank. In contrast, the Ngomo site is isolated, and we witnessed little anthropogenic activities and no disturbance, making it a suitable habitat for taxa that do not tolerate pollution.
The surprisingly little effect of the dams on upstream vs. downstream community composition contrasts several previous studies (Cortes et al., 1998; Santucci et al., 2005; Xiaocheng et al., 2008; Bredenhand and Samways, 2009; Serrana et al., 2018; Wang et al., 2019). Recently, a global analysis of the ecological impacts of small hydropower dams generally showed negative ecological effects (Kuriqi et al., 2021) affecting macroinvertebrate communities downstream due to changes in flow velocity (Mcintosh et al., 2002; Sharma et al., 2005; Martínez et al., 2013). However, there are a number of studies that found similarly weak or no immediate impact of dams on macroinvertebrate communities (Ambers, 2007; Xiaocheng et al., 2008; Vaikasas et al., 2013). A global review (Mbaka and Wanjiru, 2015) reports that more than 70% of small dams have either a positive or negative impact on macroinvertebrates by causing either a decrease or an increase in macroinvertebrate abundance and richness downstream of the dams (Mueller et al., 2011; Martínez et al., 2013; Wang et al., 2013).
The reason for this contrast could be the different methods used (Wang et al., 2020). Some studies look at specific taxonomic groups of macroinvertebrates, while others include the entire community. Other factors include differences in the climatic and geomorphological conditions at dam sites (Carr et al., 2019; Turgeon et al., 2019), downstream distance from the dam (Ruhi et al., 2018), and dam size (Poff and Hart, 2002). Additionally, using family-level identifications could potentially obscure relevant information. Most rapid assessment systems for impacts on rivers are based on family-level data (Dallas, 2021; Mezgebu, 2022). However, different macroinvertebrate species within the same family may have very different pollution tolerances (Arimoro and Ikomi, 2008) or ecosystem functions (Baulechner et al., 2020). Lumping them in a single unit can severely bias assessments and eventually be the result of the apparently lacking impact of dams on biodiversity in the Ruzizi River.
Africa has a very high demand for energy supply and hydropower facilities will play an important role in the near future, especially in the Congo River system (Winemiller et al., 2016). At the same time, there is still very little knowledge about the impact of dams on environmental flows and biodiversity in these drainage systems. The outlook for freshwater biodiversity near dams requires a more organized assessment for predicting, restoring, and managing the resulting changes in river ecosystems (Rolls et al., 2018; Turgeon et al., 2019). Changes in the management of environmental flow regimes can assist the protection and restoration of the aquatic fauna and maintaining river ecosystems downstream of dams in order to maintain ecosystem functioning (Poff and Schmidt, 2016; Kuriqi et al., 2019). This could also help increase the poor macroinvertebrate fauna of the Ruzizi River, and suggestions for altering flow practices have already been made (Muvundja et al., 2022; see also Bruder et al., 2016). Our study examining the macroinvertebrate communities in the low-diversity tropical Ruzizi River shows a weak impact of dams on downstream macroinvertebrates when using family-level bio-indications. We recommend that future studies focus on species level identifications to deliver more precise and ecologically relevant assessment. For such an approach to work, we urgently require more profound baseline studies on the species compositions of freshwater macroinvertebrate communities in Africa.
Data Availability Statement
The original contributions presented in the study are included in the article/Supplementary Material; further inquiries can be directed to the corresponding author.
Author Contributions
MD collected data, performed laboratory identification, and wrote the draft article; TN performed data analyses and contributed to writing the manuscript; FM contributed to the conceptualization of the topic, data collection and writing the manuscript; BH contributed to data collection; CA contributed to the conceptualization of the study and writing the manuscript. All authors read and approved the final version of the manuscript.
Funding
This study was financially supported by Volkswagen Foundation to FAM (Grant no. 89358 in the framework of “Knowledge for Tomorrow-Cooperative Research Projects in Sub-Saharan Africa”). MCD received a PhD scholarship from the German Academic Exchange service (DAAD).
Conflict of Interest
The authors declare that the research was conducted in the absence of any commercial or financial relationships that could be construed as a potential conflict of interest.
Publisher’s Note
All claims expressed in this article are solely those of the authors and do not necessarily represent those of their affiliated organizations, or those of the publisher, the editors and the reviewers. Any product that may be evaluated in this article, or claim that may be made by its manufacturer, is not guaranteed or endorsed by the publisher.
Acknowledgments
We thank Riziki Walumona and Georges Alunga for their contributions during data collection. The Unité d’Enseignement et de Recherche en Hydrobiologie Appliquée (UERHA) ISP-Bukavu is gratefully acknowledged for providing laboratory space.
Supplementary Material
The Supplementary Material for this article can be found online at: https://www.frontiersin.org/articles/10.3389/fenvs.2022.902246/full#supplementary-material
References
ABAKIR, (2020). Baseline Study for the Basin of Lake Kivu and the Ruzizi River (Report No: 20.214.5-022.00). Available at: https://uploads.water-energy food.org/resources/1.ABAKIR_SCR16_WP1_WP2_A4_EN_20210312.pdf
Altinbilek, D. (2002). The Role of Dams in Development. Water Sci. Technol. 45 (8), 169–180. doi:10.2166/wst.2002.0172
Ambers, R. K. R. (2007). Effects of a Small, Century-old Dam on a Secondorder Stream in the Virginia Piedmont. Southeast. Geogr. 47 (2), 181–201. doi:10.1353/sgo.2007.0018
Arimoro, F. O., and Ikomi, R. B. (2008). Response of Macroinvertebrate Communities to Abattoir Wastes and Other Anthropogenic Activities in a Municipal Stream in the Niger Delta, Nigeria. Environmentalist 28 (2), 85–98. doi:10.1007/s10669-007-9033-8
Arimoro, F. O., and Muller, W. J. (2010). Mayfly (Insecta: Ephemeroptera) Community Structure as an Indicator of the Ecological Status of a Stream in the Niger Delta Area of Nigeria. Environ. Monit. Assess. 166 (1), 581–594. doi:10.1007/s10661-009-1025-3
Bates, D., Maechler, M., Bolker, B., Walker, S., Christensen, R. H. B., Singmann, H., et al. (2020). lme4: Linear Mixed-Effects Models Using 'Eigen' and S4. R Package Version 1.1-26. Available from http://CRAN.R-project.org/package=lme4.
Baulechner, D., Jauker, F., Neubauer, T. A., and Wolters, V. (2020). Convergent Evolution of Specialized Generalists: Implications for Phylogenetic and Functional Diversity of Carabid Feeding Groups. Ecol. Evol. 10 (20), 11100–11110. doi:10.1002/ece3.6746
Bhandari, B., Shah, R. D. T., and Sharma, S. (2018). Status, Distribution and Habitat Specificity of Benthic Macro-Invertebrates: a Case Study in Five Tributaries of Buddhiganga River in Western Nepal. J. Inst. Sci. Tech. 23 (1), 69–75. doi:10.3126/jist.v23i1.22198
Bona, F., Falasco, E., Fenoglio, S., Iorio, L., and Badino, G. (2008). Response of Macroinvertebrate and Diatom Communities to Human-Induced Physical Alteration in Mountain Streams. River Res. Applic. 24 (8), 1068–1081. doi:10.1002/rra.1110
Bonada, N., Prat, N., Resh, V. H., and Statzner, B. (2006). Developments in Aquatic Insect Biomonitoring: a Comparative Analysis of Recent Approaches. Annu. Rev. Entomol. 51, 495–523. doi:10.1146/annurev.ento.51.110104.151124
Bredenhand, E., and Samways, M. J. (2009). Impact of a Dam on Benthic Macroinvertebrates in a Small River in a Biodiversity Hotspot: Cape Floristic Region, South Africa. J. Insect Conserv. 13 (3), 297–307. doi:10.1007/s10841-008-9173-2
Bruder, A., Tonolla, D., Schweizer, S. P., Vollenweider, S., Langhans, S. D., and Wüest, A. (2016). A Conceptual Framework for Hydropeaking Mitigation. Sci. Total Environ. 568, 1204–1212. doi:10.1016/j.scitotenv.2016.05.032
Bunn, S. E., and Arthington, A. H. (2002). Basic Principles and Ecological Consequences of Altered Flow Regimes for Aquatic Biodiversity. Environ. Manag. 30, 492–507. doi:10.1007/s00267-002-2737-0
Burnham, K. P., and Anderson, D. R. (2004). Multimodel Inference. Sociol. Methods Res. 33 (2), 261–304. doi:10.1177/0049124104268644
Carr, M., Li, L., Sadeghian, A., Phillips, I. D., and Lindenschmidt, K. E. (2019). Modelling the Possible Impacts of Climate Change on the Thermal Regime and Macroinvertebrate Species of a Regulated Prairie River. Ecohydrology 12 (6), pe2102. doi:10.1002/eco.2102
Carter, J. L., Resh, V. H., and Hannaford, M. J. (2017). “Macroinvertebrates as Biotic Indicators of Environmental Quality,” in Methods in Stream Ecology (Academic Press), 293–318. doi:10.1016/B978-0-12-813047-6.00016-4
Cortes, R. M. V., Ferreira, M. T., Oliveira, S. V., and Godinho, F. (1998). Contrasting Impact of Small Dams on the Macroinvertebrates of Two Iberian Mountain Rivers. Hydrobiologia 389 (1), 51–61. doi:10.1023/A:1003599010415
Couto, T. B., and Olden, J. D. (2018). Global Proliferation of Small Hydropower Plants - Science and Policy. Front. Ecol. Environ. 16 (2), 91–100. doi:10.1002/fee.1746
Dallas, H. F. (2021). Rapid Bioassessment Protocols Using Aquatic Macroinvertebrates in Africa-Considerations for Regional Adaptation of Existing Biotic Indices. Front. Water 3, 628227. doi:10.3389/frwa.2021.628227
Day, J. A., and De Moor, I. J. (2002). Guides to the Freshwater Invertebrates of Southern Africa. Non-arthropods (the protozoans, Porifera, Cnidaria, Platyhelminthes, Nemertea, Rotifera, Nematoda, Nematomorpha, Gastrotrichia, Bryozoa, Tardigrada, Polychaeta, Oligochaeta and Hirudinea). WRC Report No. TT 167/02, 5. Pretoria: Water Research Commission, 304.
Day, J. A., de Moor, I. J., Stewart, B. A., and Louw, A. E. (2001a). Guides to the Freshwater Invertebrates of Southern Africa, Volume 3. Crustacea 2: Ostracoda, Copepoda and Brachiura. WRC Report No. TT140/08. Pretoria: Water Research Commission, 177.
Day, J. A., Harrison, A. D., and de Moor, I. J. (2002). Guides to the Freshwater Invertebrates of Southern Africa, Volume 3. Diptera. WRC Report TT201/02. Pretoria: Water Research Commission, 200.
Day, J. A., Stewart, B. A., De Moor, I. J., and Louw, A. E. (1999). Guides to the Freshwater Invertebrates of Southern Africa: Volume 2, Crustacea I – Notostraca, Anostraca, Conchostraca and Cladocera. WRC Report No. TT 121/00. Pretoria: Water Research Commission, 126.
Day, J. A., Stewart, B. A., de Moor, I. J., and Louw, A. E. (2001b). Guides to the Freshwater Invertebrates of Southern Africa, Volume 4. Crustacea 3: Bathynellacea, Amphipoda, Isopoda, Spelaeogripacea, Tanaidacea and Decapoda. WRC Report TT 141/00. Pretoria: Water Research Commission, 141.
De Moor, I. J., Day, J. A., and De Moor, F. C. (2003). Guides to the Freshwater Invertebrates of Southern Africa: Volume 7 Insecta I—Ephemeroptera, Odonata and Plecoptera. WRC Report No. TT 207/03. Pretoria: Water Research Commission, 288.
de Moor, I. J., and Day, J. A. (2002). Guides to the Freshwater Invertebrates of Southern Africa. Arachnida and Mollusca. WRC Report No. TT182/02, 6. Pretoria: Water Research Commission, 141.
Descy, J.-P., Darchambeau, F., and Schmid, M. (2012). “Lake Kivu Research: Conclusions and Perspectives,” in Lake Kivu. Aquatic Ecology Series. Editors J. P. Descy, F. Darchambeau, and M. Schmid (Dordrecht: Springer), 5, 181–190. doi:10.1007/978-94-007-4243-7_11
Dick, J. T. A., Platvoet, D., and Kelly, D. W. (2002). Predatory Impact of the Freshwater Invader Dikerogammarus villosus (Crustacea: Amphipoda). Can. J. Fish. Aquat. Sci. 59 (6), 1078–1084. doi:10.1139/F02-074
Dombrowsky, I., Bastian, J., Däschle, D., Heisig, S., Peters, J., and Vosseler, C. (2014). International and Local Benefit Sharing in Hydropower Projects on Shared Rivers: the Ruzizi III and Rusumo Falls Cases. Water Policy 16 (6), 1087–1103. doi:10.2166/wp.2014.104
Dusabe, M.-C., Wronski, T., Gomes-Silva, G., Plath, M., Albrecht, C., and Apio, A. (2019). Biological Water Quality Assessment in the Degraded Mutara Rangelands, Northeastern Rwanda. Environ. Monit. Assess. 191 (3), 1–13. doi:10.1007/s10661-019-7226-5
Elbrecht, V., and Leese, F. (2015). Can DNA-Based Ecosystem Assessments Quantify Species Abundance? Testing Primer Bias and Biomass-Sequence Relationships with an Innovative Metabarcoding Protocol. PloS One 10 (7), e0130324. doi:10.1371/journal.pone.0130324
Elbrecht, V., Vamos, E. E., Meissner, K., Aroviita, J., and Leese, F. (2017). Assessing Strengths and Weaknesses of DNA Metabarcoding‐based Macroinvertebrate Identification for Routine Stream Monitoring. Methods Ecol. Evol. 8 (10), 1265–1275. doi:10.1111/2041-210X.12789
Emberton, K. C., Pearce, T. A., Kasigwa, P. F., Tattersfield, P., and Habibu, Z. (1997). High Diversity and Regional Endemism in Land Snails of Eastern Tanzania. Biodivers. Conserv. 6 (8), 1123–1136. doi:10.1023/A:1018384101789
Fichtner Gmbh und Co, K. G. (2008). Etudes préalables pour l’aménagement d’un site hydroélectrique communautaire Ruzizi III sur la Rivière Ruzizi et le Développement du volet Energie des activités de la Communauté Economique des Paysdes Grands lacs (CEPGL). Lot 1 et Lot 2: Etude de faisabilité pour l’aménagement hydroélectrique de Ruzizi III, Etude de préfaisabilité du site SISI 5 sur la Ruzizi et du réaménagement de l’exutoire du lac Kivu à Cyangugu (Topographie), Rapport de Topographie 5761P21. Energie des Grands lacs (EGL)-Union Européenne(UE). Décembre.
Fornaroli, R., Ippolito, A., Tolkkinen, M. J., Mykrä, H., Muotka, T., Balistrieri, L. S., et al. (2018). Disentangling the Effects of Low pH and Metal Mixture Toxicity on Macroinvertebrate Diversity. Environ. Pollut. 235, 889–898. doi:10.1016/j.envpol.2017.12.097
Fouche, P., and Vlok, W. (2010). Water Quality Impacts on Instream Biota of the Shingwedzi River, South Africa. Afr. J. Aquat. Sci. 35 (1), 1–11. doi:10.2989/16085914.2010.466644
Gerber, A., and Gabriel, M. J. M. (2002). Aquatic Invertebrates of South African Rivers Field Guide. Pretoria: Institute of water quality studies, department of water affairs and forestry, 149.
Greenwood, M. T., Bickerton, M. A., Gurnell, A. M., and Petts, G. E. (1999). Channel Changes and Invertebrate Faunas below Nant‐y‐Môch Dam, River Rheidol, Wales, UK: 35 Years on. Riv. Res. Appl. 15 (1‐3), 99–112. doi:10.1002/(SICI)1099-1646(199901/06)15:1/3<99::AID-RRR530>3.0.CO;2-I
Grill, G., Lehner, B., Thieme, M., Geenen, B., Tickner, D., Antonelli, F., et al. (2019). Mapping the World's Free-Flowing Rivers. Nature 569 (7755), 215–221. doi:10.1038/s41586-019-1111-9
Gross, M. (2016). A Global Megadam Mania. Curr. Biol. 26 (17), R779–R782. doi:10.1016/j.cub.2016.08.050
Guareschi, S., and Wood, P. J. (2019). Taxonomic Changes and Non-native Species: An Overview of Constraints and New Challenges for Macroinvertebrate-Based Indices Calculation in River Ecosystems. Sci. Total Environ. 660, 40–46. doi:10.1016/j.scitotenv.2019.01.008
Haase, P., Pauls, S. U., Schindehütte, K., and Sundermann, A. (2010). First Audit of Macroinvertebrate Samples from an EU Water Framework Directive Monitoring Program: Human Error Greatly Lowers Precision of Assessment Results. J. North Am. Benthol. Soc. 29 (4), 1279–1291. doi:10.1899/09-183.1
Harrison, X. A., Donaldson, L., Correa-Cano, M. E., Evans, J., Fisher, D. N., Goodwin, C. E. D., et al. (2018). A Brief Introduction to Mixed Effects Modelling and Multi-Model Inference in Ecology. PeerJ 6, e4794. doi:10.7717/peerj.4794
Hartig, F., and Lohse, L. (2020). DHARMa: Residual Diagnostics for Hierarchical (Multi-Level/Mixed) Regression Models. R package version 0.3.3.0. Available from http://CRAN.R-project.org/package=DHARMa
Hughes, R. H., and Hughes, J. S. (1992). A Directory of African Wetlands. IUCN, UNEP, and WCMC, Gland, Nairobi and Cambridge
Hyangya, L., Munini, M., Mushagalusa, M., Kisekelwa, T., Masilya, M. P., Isumbisho, M. P., et al. (2014). Inventaire systématique des macroinvertébrés du lac de barrage de Mumosho sur La rivière Ruzizi (Bukavu, RDC). Bukavu: Cahiers du CERUKI, Nouvelle Série, Numéro spécial Professeur Alphonse Byamungu, 51–62.
IEA: International Energy Agency (2019). Southeast Asia Outlook 2019. Available at: https://webstore.iea.org/download/direct/2887?filename=southeast_asia_energy outlook_2019.pdf (Accessed Mar 31, 2019).
Jellyman, P., and Harding, J. (2012). The Role of Dams in Altering Freshwater Fish Communities in New Zealand. N. Z. J. Mar. Freshwater Res. 46 (4), 475–489. doi:10.1080/00288330.2012.708664
Johnson, P. T., Olden, J. D., and Vander Zanden, M. J. (2008). Dam Invaders: Impoundments Facilitate Biological Invasions into Freshwaters. Front. Ecol. Environ. 6 (7), 357–363. doi:10.1890/070156
Jones, N. (2021). How Dangerous Is Africa's Explosive Lake Kivu? Nature 597 (5877), 466–469. doi:10.1038/d41586-021-02523-5
Kaaya, L., Day, J., and Dallas, H. (2015). Tanzania River Scoring System (TARISS): a Macroinvertebrate-Based Biotic Index for Rapid Bioassessment of Rivers. Afr. J. Aquat. Sci. 40 (2), 109–117. doi:10.2989/16085914.2015.1051941
Kahle, D., Wickham, H., Jackson, S., and Korpela, M. (2019). Ggmap: Spatial Visualization with ggplot2. R Package Version 3. Available from http://CRAN.R-project.org/package=ggmap.
Kuriqi, A., Pinheiro, A. N., Sordo-Ward, A., Bejarano, M. D., and Garrote, L. (2021). Ecological Impacts of Run-Of-River Hydropower Plants-Current Status and Future Prospects on the Brink of Energy Transition. Renew. Sust. Energ. Rev. 142, 110833. doi:10.1016/j.rser.2021.110833
Kuriqi, A., Pinheiro, A. N., Sordo-Ward, A., and Garrote, L. (2019). Flow Regime Aspects in Determining Environmental Flows and Maximising Energy Production at Run-Of-River Hydropower Plants. Appl. Energy 256, 113980. doi:10.1016/j.apenergy.2019.113980
Kuriqi, A., Pinheiro, A. N., Sordo-Ward, A., and Garrote, L. (2020). Water-energy-ecosystem Nexus: Balancing Competing Interests at a Run-Of-River Hydropower Plant Coupling a Hydrologic-Ecohydraulic Approach. Energy Convers. Manag. 223, 113267. doi:10.1016/j.enconman.2020.113267
Latrubesse, E. M., Stevaux, J. C., and Sinha, R. (2005). Tropical Rivers. Geomorphology 70 (3-4), 187–206. doi:10.1016/j.geomorph.2005.02.005
Lessard, J. L., and Hayes, D. B. (2003). Effects of Elevated Water Temperature on Fish and Macroinvertebrate Communities below Small Dams. River Res. Applic. 19 (7), 721–732. doi:10.1002/rra.713
Lüdecke, D., Aust, F., Crawley, S., and Ben-Shachar, M. S. (2020a). Ggeffects: Create Tidy Data Frames of Marginal Effects for ‘ggplot’ from Model Outputs. R package version 1.0.1. Available from http://CRAN.R-project.org/package=ggeffects.
Lüdecke, D., Makowski, D., Waggoner, P., Patil, I., and Ben-Shachar, M. S. (2020b). Performance: Assessment of Regression Models Performance. R package version 0.6.1. Available from http://CRAN.R-project.org/package=performance.
Macher, J. N., Salis, R. K., Blakemore, K. S., Tollrian, R., Matthaei, C. D., and Leese, F. (2016). Multiple-stressor Effects on Stream Invertebrates: DNA Barcoding Reveals Contrasting Responses of Cryptic Mayfly Species. Ecological Indicators 61, 159–169. doi:10.1016/j.ecolind.2015.08.024
Malmqvist, B. r., and Englund, G. r. (1996). Effects of Hydropower-Induced Flow Perturbations on Mayfly (Ephemeroptera) Richness and Abundance in North Swedish River Rapids. Hydrobiologia 341 (2), 145–158. doi:10.1007/BF00018118
Martínez, A., Larrañaga, A., Basaguren, A., Pérez, J., Mendoza-Lera, C., and Pozo, J. (2013). Stream Regulation by Small Dams Affects Benthic Macroinvertebrate Communities: from Structural Changes to Functional Implications. Hydrobiologia 711 (1), 31–42. doi:10.1007/s10750-013-1459-z
Mbaka, J. G., and Wanjiru Mwaniki, M. (2015). A Global Review of the Downstream Effects of Small Impoundments on Stream Habitat Conditions and Macroinvertebrates. Environ. Rev. 23 (3), 257–262. doi:10.1139/er-2014-0080
McIntosh, M. D., Benbow, M. E., and Burky, A. J. (2002). Effects of Stream Diversion on Riffle Macroinvertebrate Communities in a Maui, Hawaii, Stream. River Res. Applic. 18 (6), 569–581. doi:10.1002/rra.69410.1002/rra.694
Mellado-Díaz, A., Sánchez-González, J. R., Guareschi, S., Magdaleno, F., and Toro Velasco, M. (2019). Exploring Longitudinal Trends and Recovery Gradients in Macroinvertebrate Communities and Biomonitoring Tools along Regulated Rivers. Sci. Total Environ. 695, 133774. doi:10.1016/j.scitotenv.2019.133774
Mezgebu, A. (2022). A Review on Freshwater Biomonitoring with Benthic Invertebrates in Ethiopia. Environ. Sustain. Ind. 14, 100174. doi:10.1016/j.indic.2022.100174
Min, J.-K., and Kong, D.-S. (2020). Distribution Patterns of Benthic Macroinvertebrate Communities Based on Multispatial-Scale Environmental Variables in the River Systems of Republic of Korea. J. Freshw. Ecol. 35 (1), 323–347. doi:10.1080/02705060.2020.1815599
Mueller, M., Pander, J., and Geist, J. (2011). The Effects of Weirs on Structural Stream Habitat and Biological Communities. J. Appl. Ecol. 48 (6), 1450–1461. doi:10.1111/j.1365-2664.2011.02035.x
Munn, M. D., and Brusven, M. A. (1991). Benthic Macroinvertebrate Communities in Nonregulated and Regulated Waters of the Clearwater River, Idaho, U.S.A. Regul. Rivers Res. Mgmt. 6 (1), 1–11. doi:10.1002/rrr.3450060102
Muvundja, F. A., Walumona, R. J., Dusabe, M. C., Alunga, L. A., Kankonda, A. B., Albrecht, C., et al. (2022). The Land-Water-Energy Nexus at the Ruzizi River Dams (Lake Kivu Outflow, African Great Lakes Region): Status, Challenges, and Perspectives. Front. Environ. Sci. (Accepted). doi:10.3389/fenvs.2022.892591
Muvundja, F. A., Wüest, A., Isumbisho, M., Kaningini, M. B., Pasche, N., Rinta, P., et al. (2014). Modelling Lake Kivu Water Level Variations over the Last Seven Decades. Limnologica 47, 21–33. doi:10.1016/j.limno.2014.02.003
Nakagawa, S., Johnson, P. C. D., and Schielzeth, H. (2017). The Coefficient of Determination R2 and Intra-class Correlation Coefficient from Generalized Linear Mixed-Effects Models Revisited and Expanded. J. R. Soc. Interface. 14 (134), 20170213. doi:10.1098/rsif.2017.0213
Oksanen, J., Blanchet, F. G., Friendly, M., Kindt, R., Legendre, P., McGlinn, D., et al. (2020). vegan: Community Ecology Package. R package version 2.5-6. 2019. Available from http://CRAN.R-project.org/package=vegan.
Onec-Bad, (2015). Groupe de la Banque Africaine de Développement Projet : centrale hydroélectrique de Ruzizi III (147 MW) Pays : Multinational (Burundi-Rd Congo-Rwanda). ONEC-2/ONE. Abidjan: Banque Africaine de Développement.
Poff, N. L., and Hart, D. D. (2002). How Dams Vary and Why it Matters for the Emerging Science of Dam Removal. BioScience 52 (8), 659–668. doi:10.1641/0006-3568(2002)052[0659:hdvawi]2.0.co;2
Poff, N. L., and Matthews, J. H. (2013). Environmental Flows in the Anthropocence: Past Progress and Future Prospects. Curr. Opin. Environ. Sustain. 5 (6), 667–675. doi:10.1016/j.cosust.2013.11.006
Poff, N. L., and Schmidt, J. C. (2016). How Dams Can Go with the Flow. Science 353 (6304), 1099–1100. doi:10.1126/science.aah4926
R Core Team (2020). R: A Language and Environment for Statistical Computing. Vienna: R Foundation for Statistical Computing. Available from https://www.r-project.org/.
Reid, A. J., Carlson, A. K., Creed, I. F., Eliason, E. J., Gell, P. A., Johnson, P. T. J., et al. (2019). Emerging Threats and Persistent Conservation Challenges for Freshwater Biodiversity. Biol. Rev. 94 (3), 849–873. doi:10.1111/brv.12480
Renã–Fã„Lt, B. M., Jansson, R., and Nilsson, C. (2010). Effects of Hydropower Generation and Opportunities for Environmental Flow Management in Swedish Riverine Ecosystems. Freshw. Biol. 55 (1), 49–67. doi:10.1111/j.1365-2427.2009.02241.x
Rolls, R. J., Heino, J., Ryder, D. S., Chessman, B. C., Growns, I. O., Thompson, R. M., et al. (2018). Scaling Biodiversity Responses to Hydrological Regimes. Biol. Rev. 93 (2), 971–995. doi:10.1111/brv.12381
Ruhi, A., Dong, X., McDaniel, C. H., Batzer, D. P., and Sabo, J. L. (2018). Detrimental Effects of a Novel Flow Regime on the Functional Trajectory of an Aquatic Invertebrate Metacommunity. Glob. Change Biol. 24 (8), 3749–3765. doi:10.1111/gcb.14133
Santucci, V. J., Gephard, S. R., and Pescitelli, S. M. (2005). Effects of Multiple Low-Head Dams on Fish, Macroinvertebrates, Habitat, and Water Quality in the Fox River, Illinois. N. Am. J. Fish. Manag. 25 (3), 975–992. doi:10.1577/M03-216.1
Serrana, J. M., Yaegashi, S., Kondoh, S., Li, B., Robinson, C. T., and Watanabe, K. (2018). Ecological Influence of Sediment Bypass Tunnels on Macroinvertebrates in Dam-Fragmented Rivers by DNA Metabarcoding. Sci. Rep. 8 (1), 1–10. doi:10.1038/s41598-018-28624-2
Sharma, C. M., Sharma, S., Borgstrom, R., and Bryceson, I. (2005). Impacts of a Small Dam on Macroinvertebrates: A Case Study in the Tinau River, Nepal. Aquat. Ecosys. Health Managem. 8 (3), 267–275. doi:10.1080/14634980500218332
Sinha, R., Latrubesse, E. M., and Nanson, G. C. (2012). Quaternary Fluvial Systems of Tropics: Major Issues and Status of Research. Palaeogeogr. Palaeoclimatol. Palaeoecol. 356-357, 1–15. doi:10.1016/j.palaeo.2012.07.024
Stals, R., and de Moor, I. J. (2007). Guides to the Freshwater Invertebrates of Southern AfricaColeoptera, WRC Report No. TT 320/07, 10.
Stein, E. D., White, B. P., Mazor, R. D., Jackson, J. K., Battle, J. M., Miller, P. E., et al. (2014). Does DNA Barcoding Improve Performance of Traditional Stream Bioassessment Metrics? Freshw. Sci. 33 (1), 302–311. doi:10.1086/674782
Stein, E. D., White, B. P., Mazor, R. D., Miller, P. E., and Pilgrim, E. M. (2013). Evaluating Ethanol-Based Sample Preservation to Facilitate Use of DNA Barcoding in Routine Freshwater Biomonitoring Programs Using Benthic Macroinvertebrates. PloS One 8 (1), e51273. doi:10.1371/journal.pone.0051273
Sweeney, B. W., Battle, J. M., Jackson, J. K., and Dapkey, T. (2011). Can DNA Barcodes of Stream Macroinvertebrates Improve Descriptions of Community Structure and Water Quality? J. North Am. Benthol. Soc. 30 (1), 195–216. doi:10.1899/10-016.1
Takao, A., Kawaguchi, Y., Minagawa, T., Kayaba, Y., and Morimoto, Y. (2008). The Relationships between Benthic Macroinvertebrates and Biotic and Abiotic Environmental Characteristics Downstream of the Yahagi Dam, Central Japan, and the State Change Caused by Inflow from a Tributary. River Res. Applic. 24 (5), 580–597. doi:10.1002/rra.1135
Tonolla, D., Bruder, A., and Schweizer, S. (2017). Evaluation of Mitigation Measures to Reduce Hydropeaking Impacts on River Ecosystems - a Case Study from the Swiss Alps. Sci. Total Environ. 574, 594–604. doi:10.1016/j.scitotenv.2016.09.101
TRACTIONELRRI (1980). Projet Définitif D’une Deuxième Centrale Hydroélectrique sur la Ruzizi, Hydrologie. Pièce Contractuelle No. 2A. A.S.B.L. pour l’Electrification de la Région des Grands Lacs “EGLBujumbura”. Bruxelles-Dortmund.
Turgeon, K., Turpin, C., and Gregory‐Eaves, I. (2019). Dams Have Varying Impacts on Fish Communities across Latitudes: a Quantitative Synthesis. Ecol. Lett. 22 (9), 1501–1516. doi:10.1111/ele.13283
Vaikasas, S., Palaima, K., and Pliūraitė, V. (2013). Influence of Hydropower Dams on the State of Macroinvertebrates Assemblages in the Virvyte River, Lithuania. J. Environ. Eng. Landsc. Manag. 21 (4), 305–315. doi:10.3846/16486897.2013.796956
Wang, J., Ding, C., Heino, J., Jiang, X., Tao, J., Ding, L., et al. (2020). What Explains the Variation in Dam Impacts on Riverine Macroinvertebrates? A Global Quantitative Synthesis. Environ. Res. Lett. 15 (12), 124028. doi:10.1088/1748-9326/abc4fc
Wang, J., Ding, L., Tao, J., Ding, C., and He, D. (2019). The Effects of Dams on Macroinvertebrates: G Lobal Trends and Insights. River Res. Applic. 35 (6), 702–713. doi:10.1002/rra.3431
Wang, X., Cai, Q., Jiang, W., and Qu, X. (2013). Assessing Impacts of a Dam Construction on Benthic Macroinvertebrate Communities in a Mountain Stream. Fresenius Environ. Bull. 22 (1), 103–110.
Winemiller, K. O., McIntyre, P. B., Castello, L., Fluet-Chouinard, E., Giarrizzo, T., Nam, S., et al. (2016). Balancing Hydropower and Biodiversity in the Amazon, Congo, and Mekong. Science 351 (6269), 128–129. doi:10.1126/science.aac7082
Wright, J. (2010). Biomonitoring with Aquatic Benthic Macroinvertebrates in Southern Costa Rica in Support of Community Based Watershed Monitoring. Ontario, Canada: MSc Thesis, York University.
Wronski, T., Dusabe, M. C., Apio, A., Hausdorf, B., and Albrecht, C. (2015). Biological Assessment of Water Quality and Biodiversity in Rwandan Rivers Draining into Lake Kivu. Aquat. Ecol. 49 (3), 309–320. doi:10.1007/s10452-015-9525-4
Xiaocheng, F., Tao, T., Wanxiang, J., Fengqing, L., Naicheng, W., Shuchan, Z., et al. (2008). Impacts of Small Hydropower Plants on Macroinvertebrate Communities. Acta Ecol. Sin. 28 (1), 45–52. doi:10.1016/s1872-2032(08)60019-0
Keywords: Ruzizi River dams, macroinvertebrates, biodiversity indices, pollution, environmental flows, hydropower
Citation: Dusabe MC, Neubauer TA, Muvundja FA, Hyangya BL and Albrecht C (2022) Family-Level Bio-Indication Does not Detect the Impacts of Dams on Macroinvertebrate Communities in a Low-Diversity Tropical River. Front. Environ. Sci. 10:902246. doi: 10.3389/fenvs.2022.902246
Received: 22 March 2022; Accepted: 06 May 2022;
Published: 01 June 2022.
Edited by:
Frank Onderi Masese, University of Eldoret, KenyaReviewed by:
Alban Kuriqi, Universidade de Lisboa, PortugalSilvia Quadroni, University of Insubria, Italy
Copyright © 2022 Dusabe, Neubauer, Muvundja, Hyangya and Albrecht. This is an open-access article distributed under the terms of the Creative Commons Attribution License (CC BY). The use, distribution or reproduction in other forums is permitted, provided the original author(s) and the copyright owner(s) are credited and that the original publication in this journal is cited, in accordance with accepted academic practice. No use, distribution or reproduction is permitted which does not comply with these terms.
*Correspondence: Marie Claire Dusabe, dusclaire25@gmail.com