- Department of Range and Watershed Management, Faculty of Water and Soil, University of Zabol, Zabol, Iran
This research investigates the association between the succession and restoration of degraded land in the southeast of Iran with artificial Calligonum forests regarding vegetation changes, soil properties, carbon (C) and nitrogen (N) pools in vegetation and soil. Eight forestry sites were selected, aged 1, 4, 6, 9, 11, 16, 25, and 30 years. Observations indicated that vegetation percentage, density, frequency, richness, and diversity of species substantially increased (p < 0.01). The highest percentage of vegetation (80.30%), density (62.70 n ha−1), richness (14.15), and diversity (0.90) was observed in the 30-year site. At the end of the succession phase under study (the 25- and 30-year sites), the variation trend of vegetation was steady. As the age of the forests increased, the soil nutrient values increased significantly during succession (p < 0.01), even though acidity and electrical conductivity (EC) did not change significantly over time (p > 0.01). In the early stages of succession, the soil’s C and N pools (aerial biomass, root, and litter) did not increase significantly (p > 0.01). Over time, however, C and N pools of the soil and plants increased (the highest amounts were seen in the 30-year site). The results indicated a significant difference in the soil and vegetation properties in the forestry sites. In general, planting native species and the succession of vegetation can play an effective role in preserving the environment in degraded lands and increasing the C and N pools.
Introduction
The Iranian Plateau’s arid and semi-arid regions’ climatic characteristics have led to sensitive and fragile conditions. In these areas, soil erosion and desertification are among the processes directly or indirectly threatening water and soil resources strongly. The restoration of vegetation in degraded rangelands can have a considerable effect on reducing soil erosion and preventing further land degradation (Ebrahimi et al., 2019). Accordingly, forestry plans have been conducted throughout Iran to revive desert rangelands and degraded lands (Rigi Pardad et al., 2021).
Forestry practices in Iran involve covering the soil surface using plant species adaptable to arid climatic conditions. For instance, several species, such as Calligonum polygonoides, L., C. bungei Boiss, and C. comosum (L`Her), have been widely cultured for forestry and desertification control (Amiraslani and Dragovich, 2011; Rigi Pardad et al., 2021). However, cultivating native, non-native, and adaptable species in arid lands to revive vegetation requires a great deal of analysis and assessment (Ebrahimi et al., 2019). These programs should study the effect of cultivated species on the soil and vegetation where they are planted. Selecting the appropriate species for forestry to revive vegetation and conserve soil and water resources are the main components of forestry (Hashemi Rad et al., 2018). Plants affect soil by altering its physical and chemical properties. The soil properties and food reserves strongly depend on the type of vegetation (Rigi Pardad et al., 2021).
Programs for restoring vegetation in arid areas have multiple objectives: creating secondary succession (Ebrahimi et al., 2019; Udayana et al., 2019), curbing wind and water erosion, improving soil quality and fertility, increasing carbon (C) and nitrogen (N) storage in aerial and below-ground plant biomass, cleaning the air, reducing greenhouse gases, and ultimately increasing the plant production. Therefore, restoring vegetation can play a substantial role in sustainable development and ecosystem health (Cao et al., 2011; An et al., 2019; Udayana et al., 2019; Hu et al., 2020). Given the insufficient precipitation in arid areas and the subsequent lack of plant biomass, increasing biomass volume by cultivating adaptable plants can boost the ecosystem’s power to elevate C and N pools in the soil, reinforce vegetation and soil, and contribute to C and N sequestration (UNDP, 2000; Ritchie, 2020; Tessema et al., 2020).
CO2 and NO are among the most well-known greenhouse gases, whose atmospheric concentration increases due to increased industrial activities, leading to abnormal global warming and depletion of the Earth’s ozone layer (Conant et al., 2017; Hashemi Rad et al., 2018). The slightest change in the amount of C and N pools increases the amount of atmospheric C and N levels (Chapuis-Lardy et al., 2007; Hu et al., 2020; Tessema et al., 2020; Yang et al., 2020). Accordingly, the global community entered into the Kyoto Treaty in December 1997 in an extensive effort to reduce greenhouse gas emissions. One of the stressed ideas in this treaty is the attempt to reduce the atmospheric levels of greenhouse gases by preserving and creating vegetation and sequestration of C and N (Mehdipour et al., 2007; Rigi Pardad et al., 2021). C and N sequestration refers to a set of processes in which additional atmospheric concentrations of C and N are captured and stored in plant biomass and soil (Rigi Pardad, 2021). Semi-arid and arid rangelands are a great option for C and N sequestration. Moreover, increasing biomass in these areas has several advantages by reducing sequestration costs. With these advantages, international organizations such as the FAO and the UNDP have selected these areas as sites for implementing C sequestration programs (Veramesh et al., 2010). In preliminary studies in Iran, the UNDP reported that the C sequestration volume by forestry in arid and desert rangelands predominantly vegetated with Haloxylon spp equals 14 ton ha−1 after 20 years, reaching 21 ton ha−1 in 50 years by proper management (Abedi et al., 2008).
Arid rangelands have excellent potential for storing C and N (Conant et al., 2017; Tessema et al., 2020). Studies on the changes in C and N pools in arid rangelands cultivated with woody plants indicate that compared to other lands, arid rangelands display a significant increase in C and N pools due to biological reactions (Ritchie, 2020; Tessema et al., 2020). This increase can be attributed to the direct relationship between fluctuations in biomass generation with C and N pools in a region and the influential role of related management models (Tessema et al., 2020). Shams Abad is a desert region and one of the prominent locations known for wind erosion in Sistan and Baluchestan, Iran. Large parts of Shams Abad have been cultivated with perennial species, leading to considerable changes in the local ecosystem. Nonetheless, research on the effect of forestry in Iran’s degraded and desert regions on conserving biodiversity, soil properties, and C and N pools is rare.
This study was carried out to find out how afforestation changed the vegetation cover and the C and N pools of plant-soil in the vegetation communities in the arid desert steppe of Iran. We aimed to evaluate the plant variety and richness of afforestation succession in the arid area of southeastern Sistan and Baluchestan, Iran, and to investigate the influence of afforestation on native plant species, C. polygonoides L., C. bungei Boiss and C. comosum L`Her., on plant-soil C and N reservoirs. Our hypothesis is that 1) Calligonum planting aids in the development of plant species and soil fertility, and 2) soil fertility in the initial phase does not change significantly compared to the middle phase.
Material and methods
Study area
The artificial forests in the Shams Abad Desert (between latitude 27°9′31″–27°29′10″N and between longitude 60°10′32″–60°37′19″E), Sistan and Baluchestan, Iran, were selected as the study site, with an area of 112,350.30 ha (Figure 1). With less than 80 mm of annual rainfall, the area has a hot and dry environment. There have been drastic variations in rainfall over the years. Extreme rainfall volatility leads to severe instabilities of annual fodder production. A large portion of rainfall occurs during December, January, and February in the form of rainstorms, leading to torrents as water cannot penetrate the soil due to the region’s light soil texture and lack of vegetation. In other months, especially during spring and summer, the region suffers absolute aridity, a condition where only deep-rooted plants with extensive roots capable of maintaining their relationship with in-depth soil moisture can survive. It is only natural that under such conditions, the seedlings of native plants, which experience significant growth following the generous winter rainfalls, stop growing as they are deprived of access to the moisture below the surface when the rainfalls stop and cause the soil to lose its surface moisture. The seedlings thus shrivel and perish in hot weather and summer aridity. Thus, it is challenging to replace native plants perishing due to various factors. The average temperature of the area is 30°C. The minimum and maximum temperature the region experiences is −8°C and 57°C, respectively, recorded in December and July. On most summer days, the temperature exceeds 50°C, hampering any form of vital plant activities for a relatively long period. In addition to rainfall and temperature, winds and storms are factors that can restrict vegetation (Report of Rangeland Improvement, 2018).
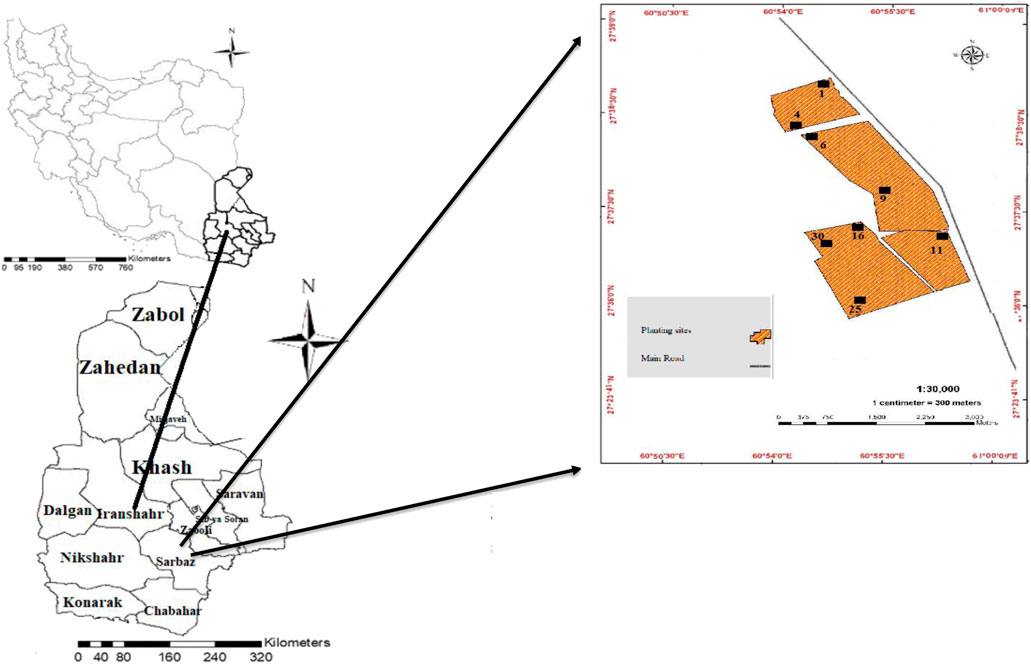
FIGURE 1. Sampling sites in the Shams Abad artificial forest, Iran. The black squares indicate the sampling sites, and the numbers below the squares indicate the ages of the sites.
The assessment of forestry area
In this study, the selected forestry sites were cultivated with the following species: C. polygonoides L., C. bungei Boiss, and C. comosum (L`Her). The species were aged 1, 4, 6, 9, 11, 16, 25, and 30 years. The vegetation was thoroughly analyzed in a specific 1-ha area in each site. In March 2019, data were gathered using a randomized complete block design (RCBD). The plant species were identified and named in the Botany Laboratory of the University of Zabol. The identified plants and assigned nomenclatures were matched with those in the studies by Rechinger et al. (1984), Rechinger et al. (1988). The chorotype of plants was matched with that in the study by Zohary (1963). Low-age sites (1, 4, 6, and 9 years) were protected against livestock grazing. However, livestock grazing was permissible in sites aged 11, 16, 25, and 30 years (sheep and goats). A total of 40 sample stands with 100 m × 100 m dimensions were selected to determine the changes in plant populations (five stands were assigned to each site). Quadrats (2 m × 3 m, 5 m × 5 m, 5 m × 5 m, 6 m × 6 m, 8 m × 8 m, 10 m × 10 m, 15 m × 15 m, 20 m × 20 m for 1, 4, 6, 9, 11, 16, 25, and 30 ages, respectively) were used to measure vegetation characteristics in each stand along 100-m transect lines by the systematic random sampling method (75 quadrats per stand). In general, 120 transects were sampled. The distance between the transect lines was 10 m.
The visual estimation method was used to estimate the percentages of vegetation and bare soil. The number of species in a sampled area was used to calculate the density of species (Ebrahimi et al., 2016). The relative density factors (RD), relative cover factors (RC), and relative frequency factors (RF) were used to compute the importance values (IV) of species. The characteristics of a dominant plant species may be derived from these characteristics. The relative density of a plant species is the ratio of its density to the overall density. An area or sample of vegetation may be defined as having a relative cover of a certain species in proportion to the cover of total plant species. Species frequency as a percentage of total plant cover is known as the relative frequency (Khosravi et al., 2017). Species richness was estimated by quantifying species per quadrate.
The formula H′ =
Plant C and N pools measurement
In each age class, inside the quadrate, the aerial parts (stems and leaves) related to the growth of the current year’s Calligonum, related species, and all the litters under the canopy cover were extracted. To ensure minimum degradation of vegetation, plant species were collected in three quadrats in each age class. At 45-cm soil depth in each quadrate, below-ground biomass was collected using the root auger (25 cm diameter). The samples were spread on a tray to remove the soil around the roots. In each age class, all aerial organs, roots, and litters were dried (70°C for 48 h) to determine dry mass. Total plant N was measured using the modified Kjeldahl method (Bremner, 1996), and organic plant C was measured using the method of Nelson and Sommers (1982).
Measuring soil properties
Soil samples at 45-cm depth were collected from under the shrubs’ canopy cover. At the same time, biomass samples were collected from roots and the lines between the shrubs (10–15 m distance) inside the quadrates. In case the quadrats had no shrubs, the plants nearest to the quadrats were selected. In each age class, a total of 15 soil samples were collected at random. A 2-mm sieve plate was used to separate soil samples that had been air-dried.
The laser diffractometry method (LDM) was used to determine the soil texture (Wang et al., 2012). The saturated soil-paste method (Thomas, 1996) was used to determine the acidity (pH) using a pH meter. The EC was measured using an EC meter (Rhoades, 1996). Total nitrogen (N) was measured using the Kjeldahl method (Bremner, 1996). The available phosphorus (P) was determined using the Olsen method via a spectrophotometer (Bray and Kurtz, 1954). The available potassium (K) was measured using a flame photometry device (Knudsen et al., 1982). Soil organic matter (OM) was measured based on the method of Lo et al. (2011), and the bulk density (BD) of soil was measured using the volumetric ring method (Wu et al., 2010). The soil organic C pool was determined using the equation: Cp = BD × SOC × D (Deng et al., 2013; Wang et al., 2014). In this equation, Cp is the soil organic C pool (kg m−2), BD (g cm−3) denotes the bulk density of the soil, SOC is an abbreviation for soil organic C content (g kg−1), and D (cm) denotes the thickness of the soil layer tested. The soil N pool was calculated using the following equation: Np = BD × TN × D (Deng et al., 2013; Wang et al., 2014). In this equation, Np (kg m−2) is the soil N pool, BD (g cm−3) is soil bulk density, and TN (g kg−1) is the total soil N content.
Data analysis
After studying the outlier values, verifying normal distribution, and testing homogeneity of variance, data analysis was conducted with a randomized complete block design (RCBD) in SPSS (Ver. 20.0) to investigate any significant difference among the age groups. To compare mean pairs, Duncan’s multiple range test (DMRT) was used. The values of indexes, including richness, evenness, and diversity, were calculated using the PAST data analyzer. The main trends in reclamation ages were described by fitting polynomial functions to vegetation indices using the curve fit feature in the SPSS (Ver. 20.0) software package.
Results
Vegetation changes
In the study sites, 15 plant species, including ten families and three genera, were listed (Table 1). The maximum number of plant species (15 species, Figure 2A), genera (13 genera, Figure 2B), and family (ten families, Figure 2C) was in the 25- and 30-year age classes. The lowest number of species (five species, Figure 2A), genus (three genera, Figure 2B), and family (three families, Figure 2C) was related to the age class of 1 year. The highest frequency was, respectively, seen in Polygonaceae (20%), Amaranthaceae (20%), and Fabaceae (13%) families. Most species were perennials (86.66%). Annuals had an insignificant share (13.33%) in the forestry sites. A chorological study (Table 1) showed that the largest proportion of the flora belongs to the Sahara-Sindian elements (60%), followed by Irano-Turanian (20%) and Saharo-Arabian (15%).
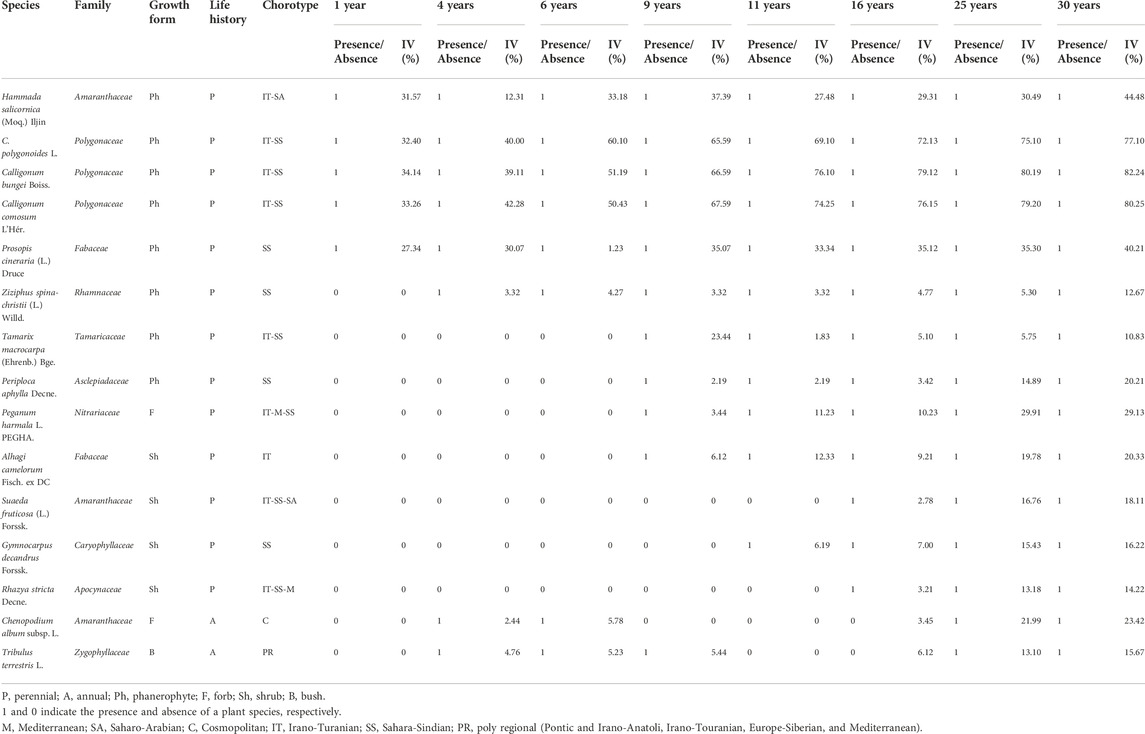
TABLE 1. Plant species composition in the eight study areas (1, 4, 6, 9, 11, 16, 25, and 30 years after afforestation).
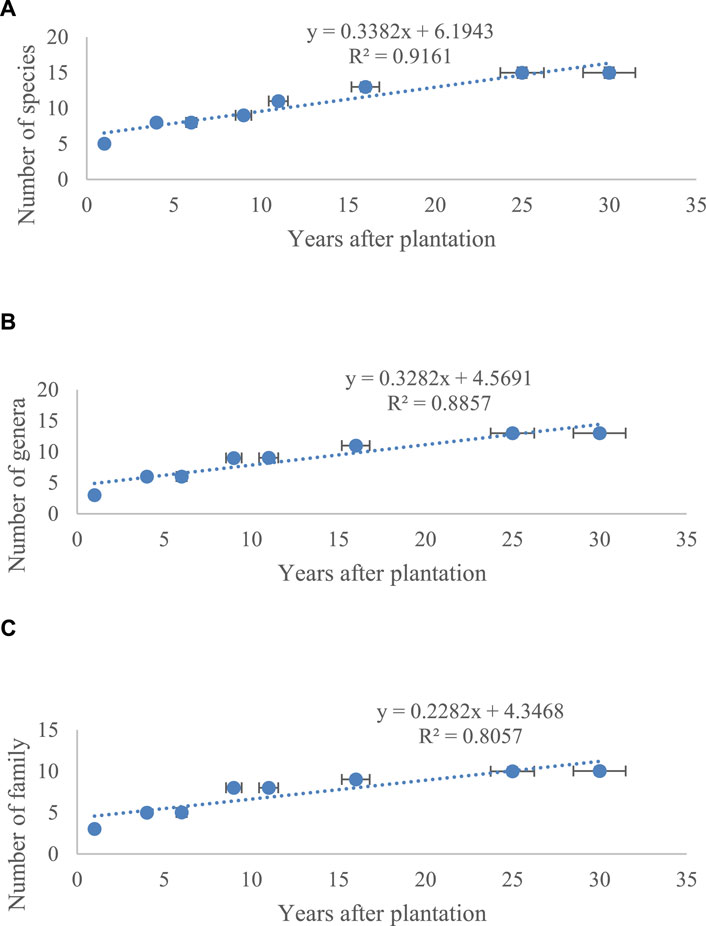
FIGURE 2. Variations of vegetation properties in the eight Calligonum planting sites. (A) Number of species, (B) genera, and (C) families. Vertical bars show standard errors.
According to importance value (IV), the following species were predominant in all sites: C. polygonaides, C. Bungei, and C. comosum, and with the increasing age from 1 year to 30 years, vegetation cover (Figure 3A) and density (Figure 3B) displayed an ascending trend.
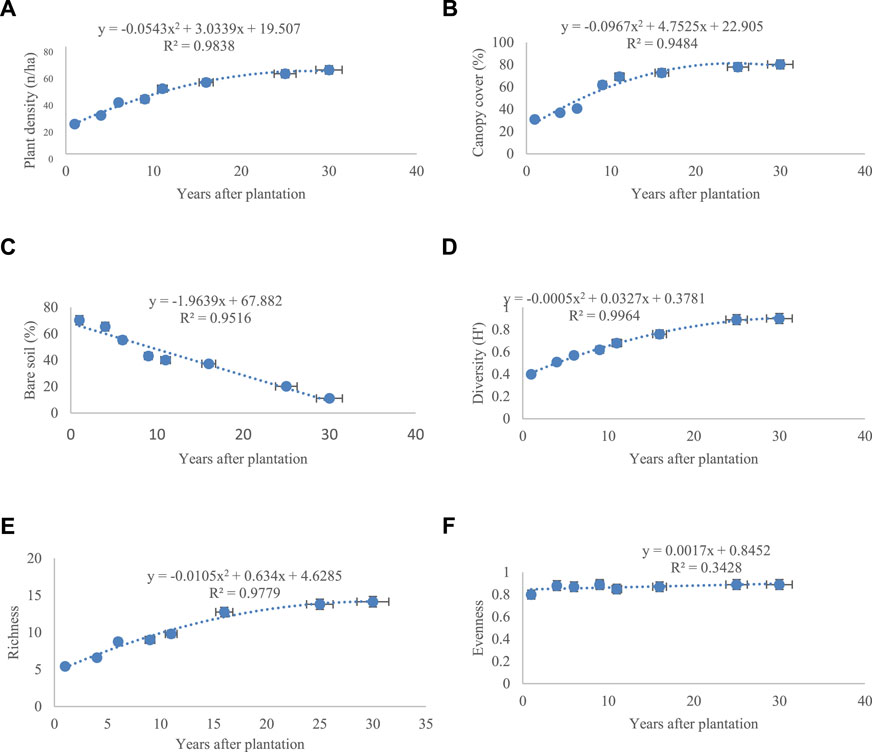
FIGURE 3. Vegetation characteristics in the eight Calligonum planting sites. (A) Plant density, (B) canopy cover, (C) bare soil, (D) diversity (H′), (E) richness, and (F) evenness. Vertical bars show standard error.
The maximum and minimum percentage of vegetation cover was seen in the 30-year site (80.30%) and the 1-year site (30.90%), respectively (Figure 3A). Vegetation percentage had an almost constant trend from the 16-year site to 30-year site.
The density of species increased from 22.30 n ha−1 in the 1-year site to 62.70 n ha−1 in the 30-year site (Figure 3A). The density of species in the 25- and 30-year sites displayed a steady trend. In lands cultivated with Calligonum, the bare soil (Figure 3C) decreased significantly over time (p < 0.01). The highest amount of litter was seen in the 30-year age class. The results indicated that cultivating Calligonum significantly elevated the diversity (Figure 3D) and richness (Figure 3E) of species in the region (p < 0.01).
In contrast, evenness (Figure 3F) did not change significantly in the age classes in the study (p > 0.01). The 30-year age group displayed the highest diversity (0.9) and richness (14.15). The 9- and 11-year age classes did not differ significantly (p > 0.01) in terms of richness and diversity, indicating that restoring vegetation in arid regions with harsh climatic conditions is time-consuming. Vegetation in disturbed land prone to wind erosion excellently responded to biological restoration with significant changes, thus confirming the primary hypothesis of the study.
Soil properties changes
Changes in soil properties and C and N pools in artificial forests re-vegetated with Calligonum in the period of 1–30 years have been shown in Table 2. Our findings showed that soil acidity changes were insignificant (p > 0.01). The results indicated that EC under and between the canopy cover did not change significantly over time (p > 0.01), despite the lower EC between the canopy cover of Calligonum plants compared to the inter-canopy cover of plants mass, indicating a significant difference (p < 0.01). Over time, as the re-vegetated forests aged, soil fertility factors including organic matter, N, P, and K increased significantly (p < 0.01). The lowest recorded amounts of organic matter, N, P, and K were observed at the initial phase (1-year) of restoration. The highest values of the parameters were recorded at the end of the period. The amount of soil organic matter in the 30-year site was approximately 3.09 times (under the canopy cover of Calligonum) greater than the beginning of the succession phase (1-year). At the end of the phase, the N, P, and K levels were 12, 6.44, and 6.84 times greater than those at the beginning of the succession phase. The level of the N pool followed the same pattern. N and C pool levels were the highest amounts underneath the Calligonum canopies in the area that underwent succession for 30 years than in the area that underwent treatment of 1-year. Moreover, the changes in the C and N pool levels were not significant among the 1- and 4-year sites. The soil surface layer in the 30 years’ Calligonum-planted area exhibited higher silt and clay than the other areas (Table 2).
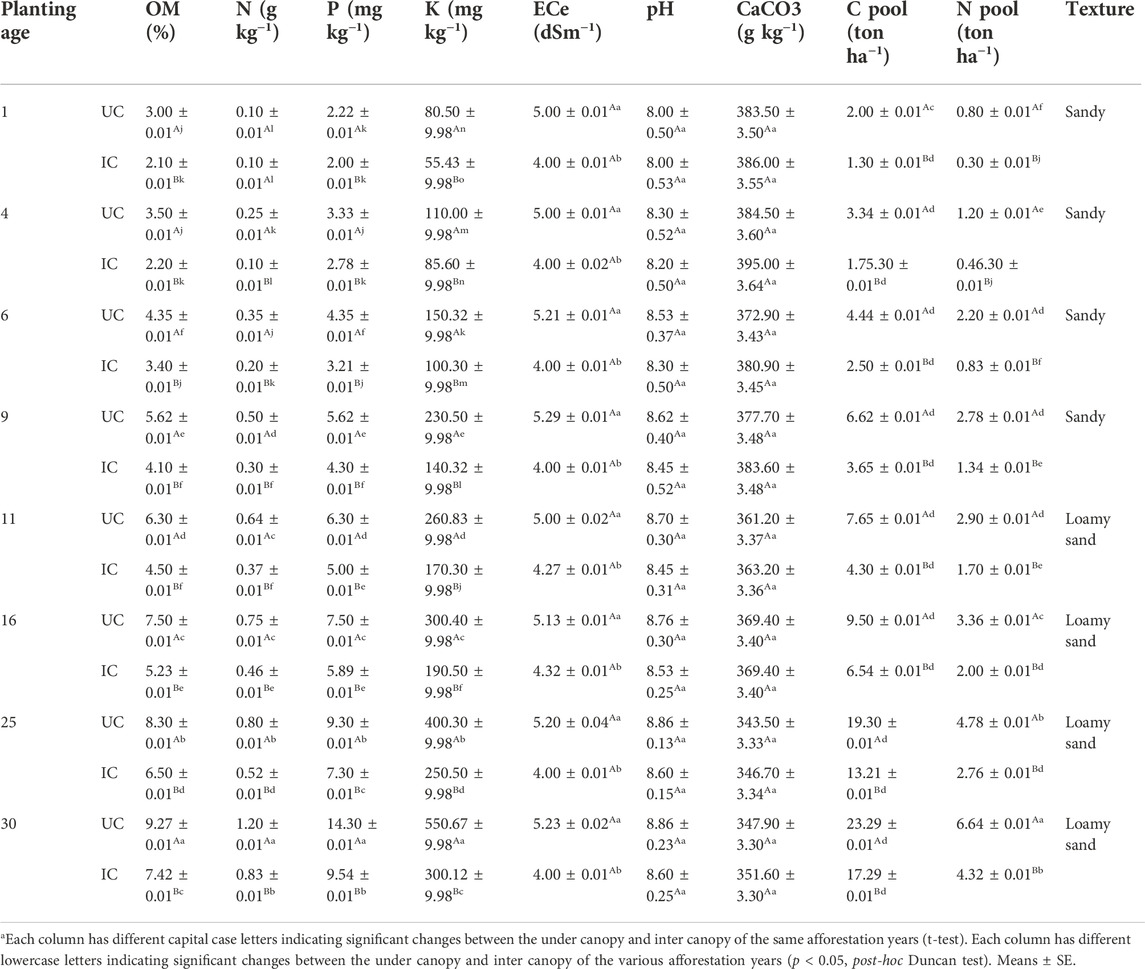
TABLE 2. Characteristics of soil under canopy (UC) and inter canopy (IC) in the eight Calligonum planting sites.
C and N pools variations
The amounts of C pool in aerial biomass, roots, and litter in artificial forests cultivated with Calligonum are shown in Table 3. The results showed that the C pool in aerial biomass, roots, and litters at the beginning of the phase was 1.30, 1.50, and 0.21 ton ha−1, respectively, an insignificant difference with the 4-year class (p > 0.01). Over time, the C pool increased substantially (p < 0.01). The maximum C pool was observed in the 30-year class. Notably, the C pool showed an ascending trend at the end of the study period. In contrast, soil C storage showed a steady trend. Over time, the N pool in aerial biomass, roots, and litters increased significantly as the re-vegetated forests aged (p < 0.01) (Table 4). The lowest N pool was observed at the beginning of the study period. Like C pool results, the N pool at the initial phase (1-year) did not change significantly compared to the 4-year age class (p > 0.01). Over time, the N pool in aerial biomass, roots, and litters increased significantly as the re-vegetated forests aged (p < 0.01) (Table 4). Maximum C and N pools in all age classes were observed in roots, aerial biomass, and litters, respectively, showing a significant difference (p < 0.01).
Discussion
The changes in plant communities and ecological processes extend the ecological niche and increase the capturing of seeds (Jules et al., 2008; Suganuma et al., 2014). These changes facilitate the distribution of new plant species and lead to the establishment of suitable species in later stages of succession (Liu et al., 2015). Forestry provides appropriate habitats where plants can grow in arid and desert regions (Amici et al., 2012). Established species can improve environmental conditions worldwide (Ebrahimi et al., 2019). The microclimate created in the understory of the cultivated species reduces the effect of extreme sunlight; the temperature under the canopy cover of these plants is low, thus reducing the evapotranspiration and, subsequently, water demand in these areas compared to vegetation-free areas (Ebrahimi et al., 2019; Moghbeli et al., 2021). In the study, shrub and forb cover are plant structures that increased due to the planting and establishment of Calligonum species in the afforested area. These species rapidly increase biomass and provide a diverse canopy and vertical structure. In addition, increasing the canopy helped to increase the substrate coverage (Moghbeli et al., 2021).
Furthermore, the established plant species in re-vegetated areas help extend plant species in arid and desert areas by capturing the seeds of plant species (Aghababaei et al., 2017). The bushes and shrubs function as seed distributors by conserving wind-scattered seeds, thus increasing the quantity and diversity of plants. The seedlings of other plants can be established under the shade of these bushes, allowing herbaceous species to create colonies and attain sustainability (Hamidi et al., 2020; Moghbeli et al., 2021). Improved conditions and reduced soil erosion due to developments in plant communities increase the soil fertility and permeability, creating a suitable context for seed germination, seedlings’ growth, and enhanced efficiency of plants in nutrient- and water-poor environments (Su et al., 2002; Zehtabian et al., 2006; Khosravi et al., 2017).
The 30-year site had the lowest bare soil percentage. It can be attributed to the C. comosum mass growing in large communities, covering the soil surface. Therefore, the percentage of canopy increases as the forestry sites age. Multiple research studies have indicated that increasing the quantity and diversity of plant species is among the most positive effects of biological measures in combating desertification (Zehtabian et al., 2006). The cultivation of Haloxlon in Shandan, Sistan and Baluchestan, Iran, confirmed that the quantity and diversity of plant species in the region increased significantly as the age of the re-vegetated forests increased (Ebrahimi et al., 2019). Pouyafar and Asgari Moghadam (2006) studied the environmental effects of oil mulch. They concluded that cultivating H. aphyllum significantly increases vegetation, species composition, and below-ground biomass. Teymuri Majnabadi et al. (2020) studied the effect of succession on the performance of desert rangelands in Iran. This study confirmed that succession has significantly improved the conditions of microhabitats for the growth of herbaceous plants in degraded desert lands.
Species composition and diversity are some of the essential characteristics of ecosystems; thus, vegetation diversity should be considered in the re-vegetation period (Brancalion et al., 2010). Johnston (2011) discussed coastal ecosystems in Sacramento, California, USA. He reported that species that can foster favorable conditions for other plants through succession are more critical than cultivating various species not resistant to harsh conditions. Our findings indicated that forestry helped enrich vegetation and provided better growth conditions for the plants to produce seeds. Over time, as the forestry sites aged, the emergence of other plant species was observed, increasing richness and diversity substantially. Strauss (2000) examined artificial forests in Australia and reported that forestry with native species substantially enriches native species in the re-vegetated forests. Ebrahimi et al. (2019) evaluated species composition of artificial forests in Sistan and Baluchestan and demonstrated that the diversity reaches a maximum amid succession. Ruiz-Jaen and Aide (2005) examined plant structure and diversity in Sabana Seca, Puerto Rico, in mid-successional re-vegetated forests. They concluded that plant diversity increases significantly over time.
In this study, the perennial species in the 30-year site displayed the highest growth in population. Increased plant density and diversity can be helpful for ecosystem composition (Teymuri Majnabadi et al., 2020; Moghbeli et al., 2021). The planted shrubs and trees can capture and store mineral and water nutrients in the soil and increase soil fertility (Mofidi et al., 2013; Ebrahimi et al., 2019). These plants can also protect bush species under the canopy cover against extreme sunlight and temperatures in arid regions (Khosravi et al., 2017). The fertile soil and microclimate under the shrubs function as fertile resources for low-height herbaceous plants (Moghbeli et al., 2021). These resources are vital for reviving desert lands since they establish natural substitutes by facilitating the growth of other plants (Ebrahimi et al., 2019). Research studies have shown that biodiversity consistently increases as the succession continues due to the increase in ecosystem complexity (Ruiz-Jaen and Aide, 2005; Jafarian et al., 2014). Aghababaei et al. (2017) examined the process of diversifying plant species from the beginning of Iran’s forest re-vegetation projects. They concluded that the introduction of additional species increases diversity after 25 years.
Livestock grazed on C. comosum sites aged 11, 16, 25, and 30 years. An average grazing rate increases the richness and diversity of species (Khosravi et al., 2017; Moghbeli et al., 2021). Omar (1991) discussed vegetation changes in Kuwait’s arid rangelands. He showed that fully protected rangelands, that is, long-term protection programs (10 years), display signs of rangeland degradation. Diversity and richness at the beginning of the succession phase can be attributed to the high dynamics of a limited number of annuals. High dynamics is an essential structural feature at the beginning of the succession phase (Liu et al., 2015). Moreover, the low quantity of species, diversity, and richness at the beginning of the succession phase in younger sites compared to older sites can be attributed to preventing grazing (El-Keblawy and Ksiksi, 2005; Ebrahimi et al., 2019). Therefore, it can be concluded that well-managed grazing is one of many tools for modifying rangelands and forest understory in modified areas.
The results indicated that re-vegetating desert land with Calligonum improved soil fertility in the region. The establishment of plant species in arid lands with harsh conditions significantly increased the soil N, P, and K under the crown of plants. The results of the researchers showed that the Calligonum species improves the soil structure, and the establishment of plant species increases the nutrients in the soil such as N, P, and K, and with the development of plants, the organic matter of the soil also increases significantly (Biabani, 2016). Zehtabian et al. (2006) reported that planting Calligonum in Semnan strengthened soil structure and increased soil N, P, and K. The increased diversity and frequency of perennials can be partially attributed to greater soil fertility. The increase in soil nutrient levels under the canopy cover of plants indicates the accumulation of litter, roots, and further root-related activities (Jozefowska et al., 2017). Two factors can activate this mechanism: First, improved vegetation in the region, resulting in decreased wind erosion. Vegetation can capture nutrients and particles carried by the wind. Second, higher nutrient levels are considerably associated with soil roots and adding root mass to the soil (Jozefowska et al., 2017; Yuuan et al., 2018).
We found that soil nutrient value gradually increased during succession; the maximum soil nutrient value was calculated in the 30-year site. This result confirms the second hypothesis presented in the Introduction section. The higher soil fertility in sites with higher ages can be partly explained by increased litter accumulation resulting in increased organic matter and nutrients in the soil due to increased vegetation canopy (Jozefowska et al., 2017; Yuuan et al., 2018; Teymuri Majnabadi et al., 2020).
In sites with higher ages, more extensive canopy covers will allow livestock to access more nutrients. Trees and shrubs with long lifespan increase soil fertility more than the annuals. This increase can be due to the immediate elimination of nutrients stored under canopy cover by the wind in sites with lower age (Cheng et al., 2004).
It should be noted that the biological restoration in arid areas where extreme sunlight and temperatures (high evapotranspiration) limit plant growth can increase vegetation and reduce exposure to sunlight in the space under plant species, hence lowering soil temperature and water loss. Moreover, higher amounts of plant litter and organic matter further increase water storage in the soil. Thus, more litter in low-age areas will result in higher soil fertility (Frouz and Novakova, 2005; Frouz et al., 2008; Ebrahimi et al., 2019).
The results showed that soil EC increased with the age of forests. This increase, however, was insignificant. This increase in EC, especially under the canopy cover, can be due to soluble salts generated by litter accumulation (Jafarian et al., 2014; Lalozaei et al., 2016). Biabani (2016) stated that Calligonum has increased soil EC in Iranshahr deserts, Iran.
The results indicated that organic C and N pools in the soil under the canopy were higher than between the canopy, which can be attributed to the higher percentage of the canopy of plant species, litter, and the higher root mass under the canopy, increasing organic C and N pools in surface soil by causing aerial organs and litter to decompose. Consequently, plant textures become an important source of organic soil C. These plant textures can gradually be transferred to the soil and undergo chemical and biological changes. The maximum C and N pools in the soil and plants were observed in the 25- and 30-year sites. In areas with more vegetation, the amounts of plant textures transferred to the soil will be higher; thus, there will be greater organic matter and, subsequently, N and C pools (Shirzai et al., 2020). Most of the C and N pools in the soil were elevated to the under canopy of sites. The high amount of C and N pools under canopy of Calligonum may be due to the fact that most of the organic matter in the soil, related to the decomposition of dead roots and the conversion of microbial biomass to organic matter, which is located at the surface layer soil under canopy of plants (Dianati Tilaki et al., 2009). Dong et al. (2014) reported that large amounts of plant litter in the top soil increase the C stored in this layer of soil compared to deep soil. Woomer et al. (2004) showed that approximately 60% of organic carbon is stored at a soil depth of 20 cm under canopy of plants. Soil C pool is positively correlated to organic matter (Garten and Charles, 2002). This result shows the positive effect of vegetation development in the studied sites.
The results showed that the amounts of C and N pools in the below-ground biomass were higher than those in the above-ground biomass. The reason for this can be attributed to the high amount of woody tissue in the roots compared to the above-ground parts of the plants (Capuana, 2020; Rigi Pardad et al., 2021). In arid rangelands, below-ground biomass of the plants has the largest proportion of total biomass, while above-ground biomass has a small proportion of the total plant biomass (Joneidi Jafari et al., 2013). Plant organs with woody tissue have a greater ability to store C and N (Motamedi et al., 2020). In this regard, other studies have acknowledged that the woodier the tissues in the plant, the greater the plant’s ability to uptake C and N (Motamedi et al., 2020; Tessema et al., 2020). Increasing the share of the root increases C and N entrance into the soil (Moghbeli et al., 2021). The type of plant species and even different organs of a plant have different potentials for C and N pools. In fact, the performance of plants to store carbon is a function of various factors such as morphological traits, including plant root height, canopy cover, plant density, plant distribution pattern, topographic characteristics, physical and chemical properties of the soil, and management factors (such as livestock grazing and rangeland exclusion) (Conti and Diaz, 2013; Mirlashkari, 2016). Expansion of vegetation cover will lead to reducing and modifying the amount of CO2 in the atmosphere by increasing photosynthetic levels and eventually increasing the level of C uptake (Souri et al., 2020). Mirlashkari (2016), in the investigation of the impacts of exclusion on the soil C and N pool in the Jonabad rangeland of Zahedan, Iran, reported that C and N pools were higher in the site with higher plant biomass than the grazed area.
Yang et al. (2020) also emphasized the significance of litter and roots in the increasing soil C pool. They reported that a high soil C pool originates from mycorrhiza, root exudates, and surface litter. Hu et al. (2020) examined the impact of decomposed litter on the amount of soil C pool in the Chinese Plateau. They reported that adding plant litter and root exudates significantly increases the soil C pool. Shirzai et al. (2020) examined the effect of species on C and N pools in the desert lands of Leuchonassi, Sistan and Baluchestan, Iran. They reported that C and N sequestration highly correlates with plant litter and organic matter amounts in the soil. With increasing age, the succession of soil organic matter increased substantially. Therefore, increased C and N pools due to increasing the succession age can be associated with improved vegetation, higher frequency of species and leaf accumulation, and, subsequently, more organic matter in these sites. Furthermore, by increasing the succession age, the mass of below-ground organs in the soil increases. Motamedi et al. (2020) concluded that total C sequestration has a positive and significant relationship with the height and mass of the plant, total aerial biomass, total below-ground biomass, and the amount of litter and soil organic C. The highest C and N pools of plant tissues in all the study sites were observed in roots, aerial organs, and litter, respectively. As the re-vegetated forests aged, tissue C and N increased in plants. This increase can be attributed to the wooden root texture of Calligonum compared to the aerial organ (Capuana, 2020). In desert rangelands, below-ground plant biomass has the highest portion of the total biomass (Tessema et al., 2020). Over time and by aging, the plant’s wooden textures thicken and increase their C and N sequestration ability. The roots’ increased effect in C and N sequestration stores more C and N in the soil (Souri et al., 2020).
In conclusion, in this paper, the Shams Abad Desert, known for its wind erosion in southeastern Iran, was selected as the study area to study the influence of biological restoration in a desert ecosystem. Was the biological restoration program in this region successful? (Resources Center, Mohammdi et al., 2018)
-The changes in vegetation, soil fertility, and C and N pools over time indicate the success of this forestry project.
-A greater value should be assigned to the leading species density at the beginning of the project to increase the restoration rate so that increased vegetation and soil fertility can more easily solve the land degradation problem for the locals.
-Agroforestry is a helpful tool to increase vegetation and improve soil fertility. However, biological restoration requires suitable species and precise management plans since any incorrect action wastes financial resources and causes irreparable damage to the environment.
Data availability statement
The raw data supporting the conclusion of this article will be made available by the authors, without undue reservation.
Author contributions
ME proposed the concept for the research and designed the research plan. ME carried out the experiments, evaluated the findings, and wrote the first version of the manuscript. MS worked on developing methodologies and overseeing the project on a local level. All authors participated in the editing of the work, reviewed, and approved the submitted version.
Acknowledgments
This study was conducted with the assistance of the Iranshahr Department of Natural Resources. Soil and plant tests were conducted using the facilities of the Soil Laboratory of the Faculty of Soil and Water at the University of Zabol.
Conflict of interest
The authors declare that the research was conducted in the absence of any commercial or financial relationships that could be construed as a potential conflict of interest.
Publisher’s note
All claims expressed in this article are solely those of the authors and do not necessarily represent those of their affiliated organizations, or those of the publisher, the editors, and the reviewers. Any product that may be evaluated in this article, or claim that may be made by its manufacturer, is not guaranteed or endorsed by the publisher.
References
Abdi, N., Maadah Arefi, H., and Zahedi Amiri, G. H. (2008). Estimation of carbon sequestration in Astragalus rangelands of markazi province (case study: Malmir rangeland in shzand region). Iran. J. Range Desert Res. 15 (2), 269–282. (In Persian).
Aghababaei, M., Asadi, E., Tahmasbi, P., and Shirmardi, H. A. (2017). Evaluation of diversity of soil seed bank for restoration of vegetation in succession patterns in semi steppe rang lands of Chahar Mahal and Bakhtiari province. Iran. J. Seed Sci. Res. 4 (1), 101–111. doi:10.22124/jms.2017.2251(In Persian)
Amici, V., Rocchini, D., Geri, F., Bacaro, G., Marcantonio, M., and Chiarucci, A. (2012). Effects of an afforestation process on plant species richness: A retrogressive analysis. Ecol. Complex. 9, 55–62. doi:10.1016/j.ecocom.2011.11.006
Amiraslani, F., and Dragovich, D. (2011). Combating desertification in Iran over the last 50 years: An overview of changing approaches. J. Environ. Manage. 92, 1–13. doi:10.1016/j.jenvman.2010.08.012
An, H., Tang, Z., Keesstra, S., and Zhouping, S. (2019). Impact of desertification on soil and plant nutrient stoichiometry in a desert grassland. Sci. Rep. 9, 9422. doi:10.1038/s41598-019-45927-0
Biabani, Z. (2016). The Relationship between succession and reclamation of vegetation cover and soil in afforestans with Calligonum spp. (Case study: Desert Land of Bampur). Iran: M.Sc Thesis, University of Zabol. (In Persian).
Brancalion, P. H. S., Rodrigues, R. R., Gandolfi, S., Kageyama, P. Y., Nave, A. G., Gandara, F. B., et al. (2010). Instrumentos legais podem contribuir para a restauracao de florestas tropicais biodiversas. Rev. Arvore 34, 455–470. doi:10.1590/s0100-67622010000300010
Bray, R. H., and Kurtz, L. T. (1954). Determination of total, organic and available forms of phosphorus in soils. Eur. J. Soil Sci. 39-46.50, 1,
Bremner, J. M. (1996). Nitrogen total, in Methods of soil analysis. Editor J. M. Bartels (Madison, WI: Soil Science Society of America), 1085–1122.
Cao, C. Y., Jiang, S. Y., Ying, Z., Zhang, F. X., and Han, X. S. (2011). Spatial variability of soil nutrients and microbiological properties after the establishment of leguminous shrub Caragana micro-phylla Lam. Plantation on sand dune in the Horqin sandy land of Northeast China. Ecol. Eng. 37, 1467–1475. doi:10.1016/j.ecoleng.2011.03.012
Capuana, M. (2020). A review of the performance of woody and herbaceous ornamental plants for phytoremediation in urban areas. Iforest 13 (2), 139–151. doi:10.3832/ifor3242-013
Chapuis-Lardy, L., Wrage, N., Metay, A., Chotte, J., and Bernoux, M. (2007). Soils, a sink for N2O? A review. Glob. Change Biol. Bioenergy 13, 1–17. doi:10.1111/j.1365-2486.2006.01280.x
Cheng, X., An, S., Liu, S., and Li, G. (2004). Micro-scale spatial heterogeneity and the loss of carbon, nitrogen and phosphorus in degraded grassland in Ordos Plateau, north Western China. Plant Soil 259, 29–37. doi:10.1023/b:plso.0000020948.66471.2b
Conant, R. T., Cerri, C. E. P., Osborne, B. B., and Paustian, K. (2017). Grassland management impacts on soil carbon stocks: A new synthesis. Ecol. Appl. 27 (2), 662–668. doi:10.1002/eap.1473
Conti, G., and Diaz, S. (2013). Plant functional diversity and carbon storage - an empirical test in semi-arid forest ecosystems. J. Ecol. 101 (1), 18–28. doi:10.1111/1365-2745.12012
Deng, L., Shangguan, Z. P., and Sweeney, S. (2013). Changes in soil carbon and nitrogen following land abandonment of farmland on the Loess plateau, China. PLoS One 8, e71923. doi:10.1371/journal.pone.0071923
Dianati Tilaki, G. H., Naghi pour borj, A. A., Tavakkoli, H., Haidarian Agha khani, M., and Saeed Afkhamoshoara, M. R. (2009). Effect of enclosure on soil and plant carbon sequestration in semi-arid rangeland of northern Khorasan. J. Iran. Range Manag. Soc. 3, 668–679.
Dong, W., Gao-Lin, W., Yuan-Jun, Z., and Zhi-Huam, S. (2014). Grazing exclusion effects on above- and below-ground C and N pools of typical grassland on the Loess Plateau (China). Catena 123, 120–113.
Ebrahimi, M., Khosravi, H., and Rigi, M. (2016). Short-term grazing exclusion from heavy livestock rangelands affects vegetation cover and soil properties in natural ecosystems of southeastern Iran. Ecol. Eng. 95, 10–18. doi:10.1016/j.ecoleng.2016.06.069
Ebrahimi, M., Mohammadi, F., Fakhireh, A., and Bameri, A. (2019). Effects of Haloxylon spp. of different age classes on vegetation cover and soil properties on an arid desert steppe in Iran. Pedosphere 29 (5), 619–631. doi:10.1016/s1002-0160(17)60378-3
El-Keblawy, A., and Ksiksi, T. (2005). Artificial forests as conservation sites for the native flora of the UAE. For. Ecol. Manag., 213, 288–296. doi:10.1016/j.foreco.2005.03.058
Frouz, J., and Novakova, A. (2005). Development of soil microbial properties in topsoil layer during spontaneous succession in heaps after Brown coal mining in relation to humus microstructure development. Geoderma 129, 54–64. doi:10.1016/j.geoderma.2004.12.033
Frouz, J., Prach, K., Pizl, V., Hanel, L., Stary, J., Tajovsky, K., et al. (2008). Interactions between soil development, vegetation and soil fauna during spontaneous succession in post mining sites. Eur. J. Soil Biol. 44, 109–121. doi:10.1016/j.ejsobi.2007.09.002
Garten, J., and Charles, T. (2002). Soil carbon storage beneath recently established tree plantations in Tennessee and South Carolina, USA. Biomass Bioenergy 23 (2), 93–102. doi:10.1016/s0961-9534(02)00033-8
Hamidi, N., and Attarroshan, S. (2020). Effect of Albizia lebbek (L.) Benth forestry on plant species diversity and physicochemical characteristics of soil in the warm and dry desert climate. J. Plant Ecosyst. Conservation 8 (16), 173–199.
Hashemi Rad, M., Ebrahimi, M., and Shirmohammadi, E. (2018). Land use change effects on plant and soil properties in a mountainous region of Iran. J. Environ. Sci. Manag. 21 (2), 47–56. doi:10.47125/jesam/2018_2/07
Hu, Y., Han, Y., and Zhang, Y. (2020). Land desertification and its influencing factors in Kazakhstan. J. Arid. Environ. 180, 104203. doi:10.1016/j.jaridenv.2020.104203
Jafarian, A., Jafari, M., and Tavili, M. (2014). Assessment of Haloxylon plantation effects for desert reclamation with emphasis on substratum’s soil and vegetation properties in Kale Shoor of Sabzevar region, Iran. Range Desert Res. 21, 51–61. (In Persian).
Johnston, D. B. (2011). Movement of weed seeds in reclamation areas. Restor. Ecol. 19, 446–449. doi:10.1111/j.1526-100x.2011.00785.x
Joneidi Jafari, H. (2013). Relationship between root biomass and soil organic carbon: Case Study of arid shrub lands of Semnan province. Desert 18, 173–176.
Jozefowska, A., Pietrzykowski, M., Wos, B., Cajthaml, T., and Frouz, J. (2017). The effects of tree species and substrate on carbon sequestration and chemical and biological properties in reforested post-mining soils. Geoderma 292, 9–16. doi:10.1016/j.geoderma.2017.01.008
Jules, M. J., Sawyer, J. O., and Jules, E. S. (2008). Assessing the relationships between stand development and understory vegetation using a 420-year chronosequence. For. Ecol. Manage. 255, 2384–2393. doi:10.1016/j.foreco.2007.12.042
Khosravi, H., Ebrahimi, M., and Rigi, M. (2017). Effects of rangeland exclusion on plant cover and soil properties in a steppe rangeland of Southeastern Iran. Arid Land Res. Manag. 31, 352–371. doi:10.1080/15324982.2017.1310147
Knudsen, D., Peterson, G. A., and Pratt, P. (1982). “Lithium, sodium and potassium,” in Methods of soil analysis. Editor A. L. Page (Madison, WI: American Society of Agronomy), 225–246.
Lalozaei, A., Dahmardeh Ghaleno, M. R., and Ebrahimi, M. (2016). Effect of the tree windbreakers of Tamarix and Eucalyptus on some physical and chemical properties of soil in Hamoon Plain. J. Watershed Eng. Manag. 7 (4), 536–542.
Liu, B., Zhao, W., Liu, Z., Yang, Y., Luo, W., Zhou, H., et al. (2015). Changes in species diversity, aboveground biomass, and vegetation cover along an afforestation successional gradient in a semiarid desert steppe of China. Ecol. Eng. 81, 301–311. doi:10.1016/j.ecoleng.2015.04.014
Lo, I., Tsang, D., Yip, T., Wang, F., and Zhang, W. H. (2011). Influence of injection conditions on EDDS-flushing of metal-contaminated soil. J. Hazard. Mat. 192, 667–675. doi:10.1016/j.jhazmat.2011.05.067
Mehdipour, l., Landi, A., and Amerikhah, H. (2007). Comparison the effect of land use on CO2 emissions in north of Khuzestan. Karaj: 10th conference on soil science of Iran.
Mirlashkari, F. (2016). The Impact of long-term grazing exclusion and different grazing intensity on some vegetation characteristics, soil carbon pool and nitrogen pool in Joonabad rangeland-Zahedan.., University of Zabol, Iran. (In Persian)
Mofidi, M., Jafari, M., Tavili, A., Rashtbari, M., and Alijanpour, A. (2013). Grazing exclusion effect on soil and vegetation properties in imam kandi rangelands, Iran. Arid Land Res. Manag. 27, 32–40. doi:10.1080/15324982.2012.719575
Moghbeli, Z., Ebrahimi, M., and Shirmohammadi, E. (2021). Effects of different livestock grazing intensities on plant cover, soil properties, and above and below ground C and N pools in arid ecosystems (Jiroft rangeland, Iran). Environ. Resour. Res. 9 (1), 13–30.
Mohammadi, T., Dastorani, M. T., Azimzadeh, H. R., and Jafarpoor, A. (2018). Study of watershed management biological practices on soil carbon sequestration (case study: Kelestan Watershed-Fars Province). Iran. J. Watershed Manag. Sci. Eng. 12 (41), 31–40. (In Persian).
Motamedi, J., Ebrahimi, Sh., and Sheidai Karkaj, E. (2020). Relationship between carbon storage of Astragalus brachyanus species with plant characteristics, habitat characteristics and rangeland management, Rajan, West Azerbaijan. J. Range Watershed Manag. 73 (2), 423 438. (In Persian).
Nelson, D. W., and Sommers, L. E. (1982). “Total carbon, organic carbon and organic matter,” in Methods of soil analysis. Editors A. L. Page, R. H. Miller, and D. R. Keeney (Madison, WI: American Society of Agronomy and Soil Science Society of American), 1–129.
Omar, S. A. (1991). Dynamics of range plants following 10 years of protection in arid rangelands of Kuwait. J. Arid. Environ. 21, 99–111. doi:10.1016/s0140-1963(18)30732-8
Pouyafar, A. M., and Asgari Moghadam, Z. (2006). Investigation of the environmental impacts of oil mulch application. Rangel. For. J. 70, 36–41. (In Persian).
Rechinger, K. H., Ali, S. I., Browicz, K., Chrtkova-Zertova, A., Heller, D., Heyn, C. C., et al. (1984). “Papilionaceae II,” in Flora iranica. Editor K. H. Rechinger (Graz: Akademische Druck U Verlagsanstalt), 65–77.
Rechinger, K. H., Melzheimer, V., Moschl, W., and Schiman-Czeika, H. (1988). “Caryophyllaceae II,” in Flora iranica. Editor K. H. Rechinger (Graz: Akademische Druck U Verlagsanstalt), 344–351.
Rhoades, J. D. (1996). “Salinity: Electrical conductivity and total dissolved solids,” in Methods of soil analysis. Editor A. L. Page (Madison, WI: American Society of Agronomy), 417–435.
Rigi Pardad, M., Ebrahimi, M., and Erfani, M. (2021). Carbon pool capacity of plant species Calligonum comosum L. and Haloxylon ammodendron (C.A.Mey.) bunge in Mirjaveh plain. Environmental Resources Research. 9(2), 267–276.
Ritchie, M. E. (2020). Grazing Management, forage production and soil carbon dynamics. Resources 9, 49. doi:10.3390/resources9040049
Ruiz-Jaen, M. C., and Aide, T. M. (2005). Vegetation structure, species diversity and ecosystem processes as measures of restoration success. For. Ecol. Manage. 218, 159–173. doi:10.1016/j.foreco.2005.07.008
Shirzai, M., Ebrahimi, M., and Saberi, M. (2020). The 7th scientific congress on the development and promotion of architecture and urbanism in Iran, Carbon sequestration in Artemisia sieberi, Leuchonassi rangeland of zahedan city
Souri, M., Fayyaz, M., Kamali, N., Ashuri, P., and Nateghi, S. (2020). The carbon storage capacity of the Artemisia sieberi under the enclosure (Kalat Sadat Abad, Sabzevar city). J. Plant Res. Iran. J. Biol. 32 (4), 736–748. (In Persian).
Strauss, S. Y. (2001). Benefits and risks of biotic exchange between Eucalyptus plantations and native Australian forests. Austral Ecol. 26, 447–457. doi:10.1046/j.1442-9993.2001.01136.x
Su, Y. Z., Zhao, H. L., and Zhang, T. H. (2002). Influencing mechanism of several shrubs and subshrubs on soil fertility in Keerqin sandy land. Chin. J. Appl. Ecol. 13, 802–806.
Suganuma, M. S., de Assis, G. B., and Durigan, G. (2014). Changes in plant species composition and functional traits along the successional trajectory of a restored patch of Atlantic Forest. Community Ecol. 15, 27–36. doi:10.1556/comec.15.2014.1.3
Tessema, B., Sommer, R., Piikki, K., Soderstrom, M., Namirembe, S., Notenbaert, A., et al. (2020). Potential for soil organic carbon sequestration in grasslands in east african countries: A review. Grassl. Sci. 66, 135–144. doi:10.1111/grs.12267
Teymuri Majnabadi, J., Ramak, P., and Karimian, V. (2020). Effect of temporal succession of biological restoration on desert rangeland (case study; khaf rangelands, khorasan razavi province). J. Range Watershed Manag. 27 (3), 657–664. (In Persian).
Thomas, G. W. (1996). “Soil pH and soil acidity,” in Methods of soil analysis. Editor J. M. Bartels (Madison, WI: Soil Science Society of America), 475–490.
Udayana, C., Skarpe, C., Solberg, S., Mathisen, K. M., and Andreassen, H. P. (2019). Soil properties after forest rehabilitation by planting teak and mahogany in Java, Indonesia. For. Sci. Technol. 15 (4), 230–237. doi:10.1080/21580103.2019.1673220
UNDP (2000). Carbon sequestration in the desertified rangelands of Hossein Abad, through community based Management, program coordination, 1–7.
Veramesh, S., Hoseini, M., Abdi, N., and Akbarnia, M. (2010). Increment of soil carbon sequestration due to forestation and its relation with some physical and chemical factors of soil. Iran. J. For. 2 (1), 91–102.
Wang, A., Luo, C., Yang, R., Chen, Y., Shen, Z., and Li, X. (2012). Metal leaching along soil profiles after the EDDS application-A field study. Environ. Pollut. 164, 204–210. doi:10.1016/j.envpol.2012.01.031
Wang, D., Wu, G. L., Zhu, Y. J., and Shi, Z. H. (2014). Grazing exclusion effects on above- and below ground C and N pools of typical grassland on the Loess Plateau (China). Catena 123, 113–120. doi:10.1016/j.catena.2014.07.018
Woomer, P. L., Tourc, A., and Sall, M. (2004). Carbon stocks in Senegal's sahel transition zone. J. Arid. Environ. 59 (3), 499–510. doi:10.1016/j.jaridenv.2004.03.027
Wu, G. L., Liu, Z. H., Zhang, L., Chen, J. M., and Hu, T. M. (2010). Long-term fencing improved soil properties and soil organic carbon storage in an alpine swamp meadow of Western China. Plant Soil 332, 331–337. doi:10.1007/s11104-010-0299-0
Yang, X., Xu, M., Zhao, Y., Bao, T., Ren, W., and Shi, Y. (2020). Trampling disturbance of biocrust enhances soil carbon emission. Rangel. Ecol. Manag. 73 (4), 501–510. doi:10.1016/j.rama.2020.02.005
Yuan, Y., Zhao, Z., Niu, S., Li, X., Wang, Y., and Bai, Z., (2006). The effects of Haloxylon aphyllum and Calligonum comosum species in stabilization and reclamation of sand dunes of Rezaabad, Semnan province. Desert, 165, 72167–79175.Reclamation promotes the succession of the soil and vegetation in opencast coal mine: A case study from Robinia pseudoacacia reclaimed forests, pingshuo mine, ChinaCatena
Keywords: arid rangelands, vegetation succession, species richness, carbon pool, soil degradation
Citation: Ebrahimi M and Saberi M (2022) The relationship between succession and reclamation of desertified areas in artificial forests of Calligonum spp. in an arid desert of southeastern Iran. Front. Environ. Sci. 10:901962. doi: 10.3389/fenvs.2022.901962
Received: 22 March 2022; Accepted: 01 November 2022;
Published: 21 November 2022.
Edited by:
Guang-Lei Gao, Beijing Forestry University, ChinaReviewed by:
Wei Shi, Xinjiang Institute of Ecology and Geography (CAS), ChinaZiyuan Zhou, Chinese Academy of Forestry, China
Copyright © 2022 Ebrahimi and Saberi. This is an open-access article distributed under the terms of the Creative Commons Attribution License (CC BY). The use, distribution or reproduction in other forums is permitted, provided the original author(s) and the copyright owner(s) are credited and that the original publication in this journal is cited, in accordance with accepted academic practice. No use, distribution or reproduction is permitted which does not comply with these terms.
*Correspondence: Mahdieh Ebrahimi, bWFlYnJhaGltaTIwMDdAdW96LmFjLmly