- 1Key Laboratory of Coastal Environmental Processes and Ecological Remediation, Yantai Institute of Coastal Zone Research, Chinese Academy of Sciences, Yantai, China
- 2Institute of Marine Science and Technology, Shandong University, Qingdao, China
- 3Qingdao Institute of Marine Geology, CGS, Qingdao, China
High-F groundwater has caused serious human health problems worldwide. In this paper, a typical saline-fresh water mixing zone (SFMZ) in a coastal plain (south of Laizhou Bay, China) was chosen as an example to analyze and summarize the distribution characteristics and enrichment mechanism human health risks of high-fluorine groundwater. Thirty-two groundwater samples have F- concentrations that exceed the drinking water guideline value of the World Health Organization (WHO; 1.5 mg/L), Thirty-two groundwater samples exceeded the drinking water guideline value of the WHO (1.5 mg/L) and 43 groundwater samples exceeded the National Sanitary Standard for drinking water of China (1.0 mg/L), accounting for 68.1 and 91.5% of the total groundwater samples. The groundwater quality is relatively poor in this study area, and the water is unsuitable for human consumption. High-F groundwater is mainly found in the central and northern parts of the study area, and the concentrations increase in the direction of water flow. High-F groundwater is mainly found in the central and northern parts of the study area, and the concentrations increase in the direction of water flow. According to the analysis, the groundwater environment, saline water intrusion (SWI), evaporation and cation exchange are the main factors influencing the enrichment of F in the SFMZ. The neutral and weakly alkaline environment is conducive to the enrichment of F-. Cation exchange and evaporation are the most important factors in the enrichment of F. Human activity is not the main source of groundwater F. Na+ and HCO3− are adequately abundant in the groundwater environment in the study area, creating conditions that are conducive to the dissolution of fluorite and the release of F into the groundwater. An increase in the Na+ concentration and a decrease in the Ca2+ concentration can promote further dissolution of fluorite and other F-containing minerals, thereby releasing F- into the groundwater. Fluorite dissolution is prevalent in the groundwater environment, which can lead to an increase in the F concentration. This study is helpful to the development of strategies for the integrated management of high-F groundwater in coastal plains. The health risk assessment shows that long-term exposure to high-F groundwater can pose a great threat to four age clusters, especially for children and infants. The HQ values for shallow groundwater range from 0.32 to 2.89, 0.39 to 3.61, 0.56 to 5.11, and 0.42 to 3.85 for adults, teenagers, children and infants, respectively. The groundwater in this study area is not for irrigation and animal husbandry, which may indirectly affect human health.
1 Introduction
Fluorine (F), a halogen element, has the potential to cause large-scale health effects through exposure via drinking water. Regional fluorosis, which can result in skeletal fluorosis, skeletal cancer and neurotoxicological effects, has been found in people living in environments with high levels of F, and chronic poisoning occurs as a result of long-term and excessive intake of F (Ghosh et al., 2015; Thapa et al., 2018; Jia et al., 2019). The World Health Organization (WHO), 2011 released that the drinking water guideline value of F is 1.5 mg/L. The safe concentration of fluorine in drinking water is less than 1.0 mg/L according to the National Sanitary Standard for drinking water in China (GB 5749-2022). Worldwide, some reports have noted that long-term ingestion of high-F groundwater can lead to water-derived fluorosis in some countries, such as Kenya, Pakistan, South Africa, South Korea and China (Kim et al., 2012; Lü et al., 2016; Yadav et al., 2019; Mcmahon et al., 2020; Rashid et al., 2020; Mwiathi et al., 2022; Table 1). Therefore, human health risk assessment of high-F groundwater is important.
The origin and enrichment mechanism for high-F groundwater and its environmental and health risks have been hot topics in recent years, and eExcessive F contents in groundwater have been found to depend on many factors, such as geological and hydrogeological conditions, the physical characteristics of aquifers, groundwater hydrochemistry, temperature, age, residence time and water-rock interaction (Chen et al., 2019; Chen et al., 2020a; Tarki et al., 2020). Because of adsorbed F in aquifers, which is a significant source of F in groundwater (Patel et al., 2014), dissolution and precipitation, adsorption and desorption, evaporation and concentration are the main hydrogeochemical processes that control F behavior in groundwater systems in arid and semiarid areas (Liu et al., 2015; Li et al., 2019). Multiple researchers have documented that high-F groundwater favors soft and alkaline conditions with high Na+ concentrations and low Ca2+ concentrations (Chen et al., 2014; Chen et al., 2020a). In coastal areas, groundwater F enrichment is related to the high pH values, high levels of Na+, HCO3−, total dissolved solids (TDS), and salinity and low levels of Ca2+ caused by seawater or brine intrusion. High-F groundwater and seawater intrusion have the same distribution pattern, evolution tendency, and associated processes, such as cation exchange (Chen et al., 2014; Wang et al., 2015; Jia et al., 2019; Chen et al., 2020b).
In this paper, Southern Laizhou Bay (SLB), a typical coastal plain, was chosen as the study area. Saline water with a wide range of TDS values in SLB hasve formed since the late Pleistocene due to the geological environment, paleoclimate and other unique hydrological conditions (Han et al., 2014). Because of excessive exploitation of fresh groundwater, saline water intrusion (SWI) has occurred, and a saline-fresh water mixing zone has formed (Yang et al., 2021). However, the research of high-F groundwater in the mixing zone has not been paid attention to. 47 Thus, forty-seven groundwater samples were collected from the SFMZ to obtain hydrochemical data, including F-. The distribution characteristics and origin of high-F groundwater in the study area are summarized. Furthermore, the groundwater quality index (WQI) and human health risks are calculated to discuss whether the groundwater is suitable for human consumption. Our findings are relevant to high-F groundwater investigation and assessment in the regions as well as other coastal plain around the world.
2 Materials and Methods
2.1 Background of Study Area
2.1.1 Location and Climatic Conditions of Study Area
Study area in the southern Bohai Sea and northern Weibei Plain, Shandong Province, China (Figure 1). The landform types consist of hilly areas, piedmont plain and inclined plain (coastal plains) from south to north (Figure 1A), with ground surface elevations ranging from 500 m to 1∼2 m (Figure 1B). The study area belongs to warm temperate subhumid monsoon climate. The average annual precipitation is approximately 731 mm, and 70–80% of the rainfall occurs between June and August September. The mean annual evaporation is 1,648 mm, with 50% of the total evaporation capacity occurring from March to June.
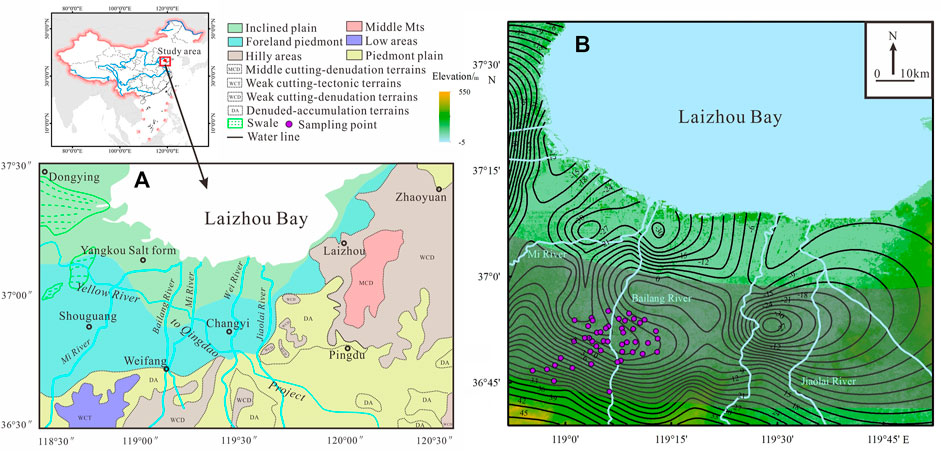
FIGURE 1. (A) Location map of the study area. Also shown are the landform types in the southern Laizhou Bay. (B) The locations of the sampling site and groundwater level in the study area. The shaded area refers to the saline-fresh water mixing zone (DEM and DEM data is derived from global bathymetric data, Yang et al., 2021).
The aquifer group in the study area is Quaternary aquifer group and belongs to Weibei Plain hydrogeologic subdivision, which is divided into two parts: freshwater hydrogeological subarea with low salinity of piedmont alluvial plain and saline water hydrogeology subarea of coastal alluvial and marine plain. The functional areas of groundwater are divided into the groundwater flow zone, intense groundwater mixing zone and groundwater-seawater interaction zone (Yang et al., 2021). In this study, groundwater quality types are divided into four classes based on total dissolved solids (TDS): fresh water (TDS < 1 g/L), brackish water (1 g/L ≤ TDS < 3 g/L), saline water (3 g/L ≤ TDS < 50 g/L), and brine (TDS ≥ 50 g/L) (Liu et al., 2017). The main hydrochemical types of fresh water are Cl·HCO3·SO4-Ca·Na, Cl-Ca·Na, HCO3-Ca·Na and HCO3·SO4·Cl-Na. The hydrochemical types of brackish water, saline water and brine are Cl-Na·Ca, Cl-Na·Mg, Cl·SO4-Na and Cl-Na.
The main land use types are artificial surface and cultivated land. Groundwater is often used for irrigation and animal husbandry. Generally, groundwater flows from north to south. Because of groundwater overexploitation, several groundwater depression zones have formed, which have changed the groundwater flow field in the study area. Under these conditions, saline water intrusion has occurred, which shows a wedge-shaped intrusion pattern and seriously affect groundwater quality and water safety. The process of saline water intrusion is not a single process by which saline water replaces fresh water but a mutual mixing process between saline water and fresh water (Liu et al., 2017).
2.2 Groundwater Samples and Chemical Analysis
Based on present studies on the groundwater environment in this study area, 47 groundwater samples were collected from May to June 2021 (Figure 1). The groundwater samples were collected and stored in polyethylene bottles (1,200 ml) in a low-temperature environment, and all samples were sent to the laboratory for testing and analysis soon after collection.
Hydrochemical data were obtained by using test instruments, such as an ultraviolet (UV)-visible spectrophotometer (T9CS), inductively coupled plasma optical emission spectroscopy instrument (iCAP 7,400), atomic fluorescence photometer (XGY-1011A), ion chromatograph (ICS-600), inductively coupled plasma mass spectrometer (Thermo iCAP RQ), and ion meter (PXSJ-216) (Supplementary Table S1).
2.3 Hydrogeochemical Characteristics Groundwater Quality Index
The WQI, which is the most widely reliable comprehensive analysis for evaluating drinking water quality, is used to evaluate the groundwater quality (Abbasnia et al., 2019; Nong et al., 2020; Singh et al., 2020; Alshehri et al., 2021; Iqbal et al., 2021). The determined WQI values were then classified into five categories: excellent (WQI < 50); good (WQI = 50–100); poor (WQI = 100.1–200); very poor (WQI = 200.1–300); and unsuitable for drinking water (WQI > 300) (Njuguna et al., 2020; Githaiga et al., 2021).
where Wi represents the relative weight, wi represents the weight, that is, often allocated to each parameter (Table 2), ∑wi represents the sum of the weights of all 11 parameters, Ci represents the detected concentration for each parameter in each sample, Si represents the WHO maximum allowable limits for each parameter, and SIi represents the water quality subindex of the ith parameter.
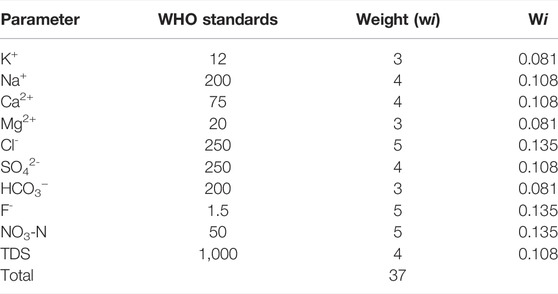
TABLE 2. The weight, relative weight and WHO standards of each of the parameters used for the WQI determination.
2.4 Human Health Risk
Because F is a noncarcinogenic risk factor, a noncarcinogenic evaluation risk model is chosen to discuss the human health risks (Rashid et al., 2019; Rashid et al., 2021).
where C is the average mass concentration of pollutants in water, mg/L;IR is the intake rate, L/d; EF is the exposure frequency, d/a; ED is the duration of exposure, a; BW is human body mass, kg; AT is the average time of exposure, d; and RfD is the reference dose of chemical pollutants in the drinking water exposure route, mg/(kg·d). The key parameters for calculating the health risks of fluoride are shown in Supplementary Table S2. The health risk level of HQ was divided into four levels: (1) HQ ≤ 1, no risk; (2) 1 < HQ ≤ 5, low risk; (3) 5 < HQ ≤ 10, medium risk; and (4) HQ > 10, high risk.
3 Results and Discussion
3.1 Groundwater Quality Types and Hydrochemical Types
The hydrochemical characteristics of the groundwater environment are presented in Supplementary Table S1. The groundwater environment shows a neutral and weakly alkaline environment (pH = 6.94 ∼ 8.72). The TDS values are 266.00 ∼ 5,082.00 mg/L and 1749.85 mg/L on average, which can indicate that the water quality is fresh water, brackish water and saline water. Na+ (39.37 ∼ 1,548.41 mg/L, 350.16 mg/L on average) and Ca2+ (27.33 ∼ 448.45 mg/L, 134.88 mg/L on average) are the main cations, while Cl- (32.29 ∼ 2,171.16 mg/L, 461.85 mg/L on average), HCO3− (148.01 ∼ 927.22 mg/L, 499.07 mg/L on average) and SO42- (39.13 ∼ 992.15 mg/L, 261.31 mg/L on average) are the dominant anions (Table 3). All ion concentrations seriously exceeded the WHO standard values. The distribution of the main ions shows an obvious enrichment characteristic in the downstream direction of groundwater flow. The high HCO3− groundwater samples are distributed on both sides of the river (Figure 2). The hydrochemical types are HCO3-Ca•Na, HCO3•Cl-Na•Mg•Ca and HCO3•Cl-Na in fresh water; HCO3•Cl-Ca•Mg, HCO3•Cl-Na, Cl•HCO3-Na and Cl-Na in brackish water; and Cl-Na and Cl-Na•Mg in saline water (Figure 3).

TABLE 3. Minimum, median, maximum and average values of chemical variables in 47 groundwater samples.
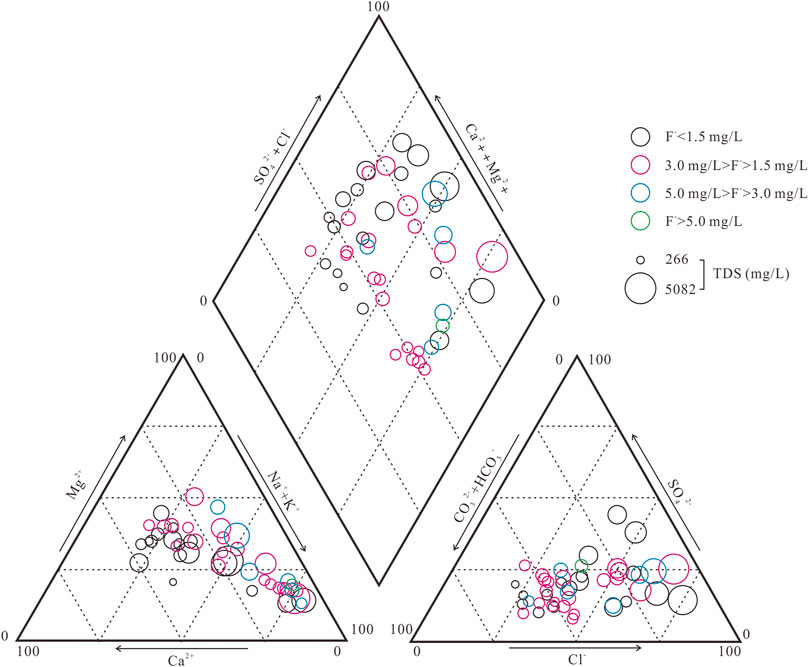
FIGURE 2. The spatial distribution of main ions in study area (Ca2+, Na+, Cl-, HCO3−, NO3-N and TDS).
3.2 Distribution Characteristics and Formation of High-F Groundwater
3.2.1 Distribution Characteristics of High-F Groundwater
The F- concentration is 0.59 ∼ 5.41 mg/L. The average value is 2.28 mg/L, and the median value is 2.31 mg/L (Table 2). Thirty-two groundwater samples exceed the drinking water guideline value of the WHO (1.5 mg/L), and 43 groundwater samples exceeded the National Sanitary Standard for drinking water of China (1.0 mg/L), accounting for 68.1 and 91.5% of the total groundwater samples. These results indicate that high-F groundwater is distributed widely in the study area, which is mainly found in the central and northern parts of the study area and that the F concentrations generally increase in the direction of groundwater flow (Figure 4). The conditions of geology, hydrogeology, groundwater hydrochemistry, water-rock interactions and human activities can lead to the formation of high-F groundwater (Chen et al., 2019; Chen et al., 2020a; Tarki et al., 2020).
3.2.2 Formation mechanism of high-F groundwater
Alkaline water can favor the dissolution of F-containing minerals (Lü et al., 2016). The Pearson correlation coefficient between F- and pH is 0.351, which indicates a positive correlation (Supplementary Table S2). The groundwater environment in the study area is neutral and weakly alkaline, which is conducive to the enrichment of F-. The reason is that the surface charge of minerals is neutral or biased negative in alkaline environments, which is not conducive to the adsorption of F-. The competitive adsorption of OH- and F- leads to the release of F- into groundwater (Ca(OH)2 → Ca2+ + 2OH-, CaF2 → Ca2+ + 2F-) (Guo et al., 2012). Neutral and weakly alkaline environments are conducive to the enrichment of F- but are not the main factor. In addition, HCO3− is a strong competitively adsorbed ion in alkaline environments (Su and Puls., 2001). The Pearson correlation coefficient between F- and HCO3− is 0.349. In this study area, HCO3− is at a high concentration (148.01 ∼ 927.22 mg/L, with an average of 499.07 mg/L), which is conducive to the release of F- in CaF2 attached to mineral surfaces into the groundwater (CaF2 + 2HCO3− = CaCO3 + 2F- + H2O+ CO2), resulting in an increase in the groundwater F concentration.
In most arid regions of the world, the F- concentrations in groundwater are generally increased by evaporation (Rashid et al., 2018a). Gibbs diagrams showing TDS values against the content ratios of Na+/(Na+ + Ca2+) have been widely employed to estimate the three significant natural factors controlling groundwater chemistry (Rashid et al., 2018b): rock weathering, precipitation and evaporation. Most of the groundwater samples fall into plot in the evaporation dominance area, indicating that evaporation and/or evaporite dissolution have a significant effect on the formation of dissolved solutes (Figure 5). The Gibbs diagram shows that most of the samples have Na+/(Na+ + Ca2+) ratios exceeding 0.5, indicating that cation exchange has significant effects on groundwater chemistry.
Cation exchange, which can drive the enrichment of F- in groundwater, is an important process in areas impacted by SWI (Rashid et al., 2018b). CAI 1 {[Cl-- (Na++K+)]/Cl-} and CAI 2 {[Cl-- (Na++K+)]/[SO42- + HCO3− + NO3− + CO32-]} can illustrate the possibility of cation exchange (Schoeller, 1967; Su et al., 2021). The CAI one and CAI two values of groundwater samples vary from -1.174 to 0.582 and from -0.001 to 0.0001, respectively. Only 14 groundwater samples (approximately 33.3% of the total samples) exhibit negative CAI one and CAI two values, indicating that the cation exchange of Ca2+ and Mg2+ relative to Na+ and K+ in groundwater in the aquifer may not be prevalent and that reverse cation exchange (2NaX + Ca2+ → CaX2 + 2Na+) is likely dominant in the study area (Figure 6). This process can lead to a decrease in the Ca2+ concentration (Chen et al., 2020b).
The role of calcite and dolomite dissolution in groundwater chemistry can be determined by the Ca2+/Mg2+ ratio (Li et al., 2018). As shown in Figure 7, almost all groundwater samples with high F- concentrations plot below the Ca2+/Mg2+ = 1:1 line, which suggests that dolomite dissolution (CaMg(CO3)2→Ca2++Mg2++2CO32-) is prevalent in high-F groundwater. The Pearson correlation coefficients between F- and Na+ and between F- and Ca2+ are 0.497 and -0.340, respectively. Moreover, the SI fluorite values are 0.08 ∼ −1.48 (only one sample is > 0.0) (Figure 7), which indicates that the potential for fluorite dissolution is high in the groundwater environment (CaF2→Ca2++2F-). The increase in Na+ concentration and decrease in Ca2+ concentration can promote further dissolution of fluorite and other F-containing minerals, thereby releasing F- into the groundwater (Su et al., 2013; Liu et al., 2015; Li et al., 2019). In high-F groundwater, dolomite dissolution and increasing F- concentrations can lead to decreasing SI fluorite values (Figure 7), hindering further fluorite dissolution.
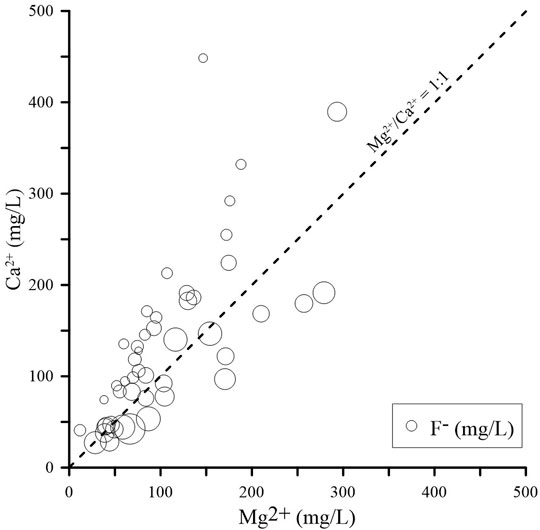
FIGURE 7. Ca2+ versus Mg2+ to evaluate the role of calcite and dolomite dissolution in groundwater chemistry, and SI fluorite vs. F-.
3.3 Effects of Human Activity on F
Human activity, such as irrigation and agricultural development, is an important factor that influences groundwater quality. Long-term agricultural activities in irrigation districts, including the unreasonable use of pesticides and fertilizers and the deterioration of irrigation water quality, have further worsened the groundwater quality, mainly reflected in the high NO3-N concentration (Zhang et al., 2021). According to investigations, the main human activity is agricultural irrigation, which can lead to an increase in NO3-N. The Pearson correlation coefficient between F- and NO3-N is -0.185. Hence, there is no obvious relationship between NO3-N and F- (Supplementary Table S2, Figure 5). It can be speculated that human activity is not the main source of groundwater F.
3.4 Groundwater Quality Index
The WQI results are given in Supplementary Table S1, and the values range from 41.64 in sample S2105097 to 489.34 in sample S2105095. Only one groundwater sample is classified as excellent-quality water. Two groundwater samples are classified as good-quality water. Twenty-seven groundwater samples (57.45%) are classified as poor-quality water. Eleven groundwater samples (23.40%) are classified as very poor-quality water. Moreover, 6 groundwater samples are classified as unsuitable for drinking water. The qualities of a total of 93.62% of the groundwater samples are classified as poor or worse. Figure 8 shows that poor-quality groundwater is widespread in this study area. Groundwater classified as very poor quality and unsuitable for drinking water is distributed in the downstream groundwater region.
3.5 Human Health Risks
To comprehensively analyze the impacts of high-F groundwater on human health comprehensively, four age groups were considered in this study: adults (≥16 years old), teenagers (6—16 years old), children (2—6 years old) and infants (<2 years old) (Su et al., 2021, Supplementary Table S2). The HQ values for adults, teenagers, children and infants were 0.32∼2.89 (1.22 on average, 1.23 in median), 0.39∼3.61 (1.52 on average, 1.54 in median), 0.56∼5.11 (2.15 on average, 2.18 in median) and 0.42∼3.85 (1.62 on average, 1.65 in median), respectively. The HQ results suggest that 28 samples (59.6) for adults, 32 samples (68.1%) for teenagers, 43 samples (91.5%) for children and 35 samples (74.5%) for infants are greater than 1. Only one sample’s HQ value (children) is greater than 5 (medium risk). Obviously, the noncarcinogenic risks caused by high-F groundwater become greater with decreasing age as a whole. It can be concluded that groundwater is not suitable for human drinking, especially for children. Moreover, the groundwater in this study area is not for irrigation and animal husbandry, which may indirectly affect human health.
4 Conclusion
This study aims to study the distribution characteristics and health risks of high-F groundwater in coastal plains. The main conclusions of the study are as follows:
1) All ion concentrations seriously exceeded the WHO standard values. High Ca2+, Na+ and Cl- concentrations are generally distributed on the west side of the river and are clearly higher in downstream areas. Based on the WQI values, 2.13% of the groundwater samples are of excellent quality, 4.26% are of good quality, 57.45% are of poor quality, 23.40% are of very poor quality, and 12.77% are unsuitable for drinking purposes. The groundwater quality is relatively poor in this study area, and the water is unsuitable for human consumption.
2) The F- concentration ranged from 0.59 to 5.41 mg/L. The average value was 2.28 mg/L, and the median value was 2.31 mg/L. High-F groundwater is mainly found in the central and northern parts of the study area, and the concentrations tend to increase in the direction of water flow. Evaporation and cation exchange are the main factors influencing the enrichment of F. Fluorite dissolution is prevalent in this groundwater environment, which can lead to an increase in the F concentration.
3) The health risk assessment shows that long-term exposure to high-F groundwater can pose a great threat to four age clusters, especially for children and infants. The HQ values for shallow groundwater range from 0.32 to 2.89, 0.39 to 3.61, 0.56 to 5.11, and 0.42 to 3.85 for adults, teenagers, children and infants, respectively. Given the freshwater resources are scarce in most coastal zones, this work’s findings will promote a better understanding that fresh or low salinity groundwater may not be suitable for irrigation and animal husbandry, which may indirectly affect human health in saline-fresh water mixing zone. In addition, it is important to recognize the potential for fluorine pollution along the groundwater flow due to the overextraction.
Data Availability Statement
The original contributions presented in the study are included in the article/Supplementary Material, further inquiries can be directed to the corresponding author.
Author Contributions
SL: Idea, Conceptualization, Formal analysis, Writing—original draft, Writing—review and; editing. YL: Formal analysis, Writing—original draft. CW: Investigation. XD: Writing—review and; editing.
Funding
This research is financially supported by the National Natural Science Foundation of China (No. U2106203, 41977173), Open Research Fund of State Key Laboratory of Estuarine and Coastal Research (Grant No. SKLEC-KF202010).
Conflict of Interest
The authors declare that the research was conducted in the absence of any commercial or financial relationships that could be construed as a potential conflict of interest.
Publisher’s Note
All claims expressed in this article are solely those of the authors and do not necessarily represent those of their affiliated organizations, or those of the publisher, the editors and the reviewers. Any product that may be evaluated in this article, or claim that may be made by its manufacturer, is not guaranteed or endorsed by the publisher.
Supplementary Material
The Supplementary Material for this article can be found online at: https://www.frontiersin.org/articles/10.3389/fenvs.2022.901637/full#supplementary-material
References
Abbasnia, A., Yousefi, N., Mahvi, A. H., Nabizadeh, R., Radfard, M., Yousefi, M., et al. (2019). Evaluation of Groundwater Quality Using Water Quality Index and its Suitability for Assessing Water for Drinking and Irrigation Purposes: Case Study of Sistan and Baluchistan Province (Iran). Hum. Ecol. Risk Assess. Int. J. 25 (4), 988–1005. doi:10.1080/10807039.2018.1458596
Alshehri, F., Almadani, S., El-Sorogy, A. S., Alwaqdani, E., Alfaifi, H. J., and Alharbi, T. (2021). Influence of Seawater Intrusion and Heavy Metals Contamination on Groundwater Quality, Red Sea Coast, Saudi Arabia. Mar. Pollut. Bull. 165, 112094. doi:10.1016/j.marpolbul.2021.112094
Berger, T., Mathurin, F. A., Drake, H., and Åström, M. E. (2016). Fluoride Abundance and Controls in Fresh Groundwater in Quaternary Deposits and Bedrock Fractures in an Area with Fluorine-Rich Granitoid Rocks. Sci. Total Environ. 569-570, 948–960. doi:10.1016/j.scitotenv.2016.06.002
Chae, G., Yun, S., Mayer, B., Kim, K., Kim, S., Kwon, J., et al. (2007). Fluorine Geochemistry in Bedrock Groundwater of South Korea. Sci. Total Environ. 385, 272–283. doi:10.1016/j.scitotenv.2007.06.038
Chen, Q., Dong, F., Jia, Z., Wei, J., Jia, C., An, M., et al. (2020a). The Experimental Study of Fluorine-Leaching Ability of Granite with Different Solutions: A New Insight into the Dynamic of Groundwater Fluorine Levels along Coastal Zones. J. Contam. Hydrol. 235, 103703. doi:10.1016/j.jconhyd.2020.103703
Chen, Q., Hao, D. C., Wei, J. C., Jia, C. P., Wang, H. M., Shi, L. Q., et al. (2019). Geo-chemical Processes during the Mixing of Seawater and Fresh Water in Estuarine Regions and Their Effect on Water Fluorine Levels. Mausam 70 (2), 329–338. doi:10.54302/mausam.v70i2.185
Chen, Q., Jia, C. P., Wei, J. C., Dong, F. Y., Yang, W. G., Hao, D. C., et al. (2020b). Geochemical Process of Groundwater Fluoride Evolution along Global Coastal Plains: Evidence from the Comparison in Seawater Intrusion Area and Soil Salinization Area. Chem. Geol. 552, 119779. doi:10.1016/j.chemgeo.2020.119779
Chen, Q., Lu, Q., Song, Z., Chen, P., Cui, Y., Zhang, R., et al. (2014). The Levels of Fluorine in the Sediments of the Aquifer and Their Significance for Fluorosis in Coastal Region of Laizhou Bay, China. Environ. Earth Sci. 71 (10), 4513–4522. doi:10.1007/s12665-013-2843-8
Choi, A. L., Sun, G., Zhang, Y., and Grandjean, P. (2012). Developmental Fluoride Neurotoxicity: a Systematic Review and Meta-Analysis. Environ. Health Perspect. 120 (10), 1362–1368. doi:10.1289/ehp.1104912
Currell, M., Cartwright, I., Raveggi, M., and Han, D. (2011). Controls on Elevated Fluoride and Arsenic Concentrations in Groundwater from the Yuncheng Basin, China. Appl. Geochem. 26, 540–552. doi:10.1016/j.apgeochem.2011.01.012
Ghosh, P., Roy, B. G., Mukhopadhyay, S. K., and Banerjee, P. (2015). Recognition of Fluoride Anions at Low Ppm Level inside Living Cells and from Fluorosis Affected Tooth and Saliva Samples. RSC Adv. 5, 27387–27392. doi:10.1039/C5RA01720C
Githaiga, K. B., Njuguna, S. M., Gituru, R. W., and Yan, X. (2021). Water Quality Assessment, Multivariate Analysis and Human Health Risks of Heavy Metals in Eight Major Lakes in Kenya. J. Environ. Manag. 297, 113410. doi:10.1016/j.jenvman.2021.113410
Guo, H., Zhang, Y., Xing, L., and Jia, Y. (2012). Spatial Variation in Arsenic and Fluoride Concentrations of Shallow Groundwater from the Town of Shahai in the Hetao Basin, Inner Mongolia. Appl. Geochem. 27, 2187–2196. doi:10.1016/j.apgeochem.2012.01.016
Han, D. M., Song, X. F., Currell, M. J., Yang, J. L., and Xiao, G. Q. (2014). Chemical and Isotopic Constraints on Evolution of Groundwater Salinization in the Coastal Plain Aquifer of Laizhou Bay, China. J. Hydrology 508, 12–27. doi:10.1016/j.jhydrol.2013.10.040
Huang, L., Sun, Z., Zhou, A., Bi, J., and Liu, Y. (2022). Source and Enrichment Mechanism of Fluoride in Groundwater of the Hotan Oasis within the Tarim Basin, Northwestern China. Environ. Pollut. 300, 118962. doi:10.1016/j.envpol.2022.118962
Iqbal, J., Su, C., Rashid, A., Yang, N., Baloch, M. Y. J., Talpur, S. A., et al. (2021). Hydrogeochemical Assessment of Groundwater and Suitability Analysis for Domestic and Agricultural Utility in Southern Punjab, Pakistan. Water 13 (24), 3589. doi:10.3390/w13243589
Jacks, G., Bhattacharya, P., Chaudhary, V., and Singh, K. P. (2005). Controls on the Genesis of Some High-Fluoride Groundwaters in India. Appl. Geochem. 20 (2), 221–228. doi:10.1016/j.apgeochem.2004.07.002
Jia, C. P., Chen, Q., Wei, J. C., Wang, H. M., Shi, L. Q., Ning, F. Z., et al. (2019). The Study on the Mechanism of Fluorine Transformation between Water and Rock (Soil) in Seawater Intrusion Areas Based on FTIR Spectrum. Spectrosc. Spect. Anal. 39 (4), 1036–1040.
Kim, K., and Jeong, G. Y. (2005). Factors Influencing Natural Occurrence of Fluoride-Rich Groundwaters: a Case Study in the Southeastern Part of the Korean Peninsula. Chemosphere 58, 1399–1408. doi:10.1016/j.chemosphere.2004.10.002
Kim, S.-H., Kim, K., Ko, K.-S., Kim, Y., and Lee, K.-S. (2012). Co-contamination of Arsenic and Fluoride in the Groundwater of Unconsolidated Aquifers under Reducing Environments. Chemosphere 87 (8), 851–856. doi:10.1016/j.chemosphere.2012.01.025
Li, D., Gao, X., Wang, Y., and Luo, W. (2018). Diverse Mechanisms Drive Fluoride Enrichment in Groundwater in Two Neighboring Sites in Northern China. Environ. Pollut. 237, 430–441. doi:10.1016/j.envpol.2018.02.072
Li, P., He, X., Li, Y., and Xiang, G. (2019). Occurrence and Health Implication of Fluoride in Groundwater of Loess Aquifer in the Chinese Loess Plateau: A Case Study of Tongchuan, Northwest China. Expo. Health 11, 95–107. doi:10.1007/s12403-018-0278-x
Liu, H., Guo, H., Yang, L., Wu, L., Li, F., Li, S., et al. (2015). Occurrence and Formation of High Fluoride Groundwater in the Hengshui Area of the North China Plain. Environ. Earth Sci. 74 (3), 2329–2340. doi:10.1007/s12665-015-4225-x
Liu, S., Tang, Z., Gao, M., and Hou, G. (2017). Evolutionary Process of Saline-Water Intrusion in Holocene and Late Pleistocene Groundwater in Southern Laizhou Bay. Sci. Total Environ. 607-608, 586–599. doi:10.1016/j.scitotenv.2017.06.262
Lü, J., Qiu, H., Lin, H., Yuan, Y., Chen, Z., and Zhao, R. (2016). Source Apportionment of Fluorine Pollution in Regional Shallow Groundwater at You'xi County Southeast China. Chemosphere 158, 50–55. doi:10.1016/j.chemosphere.2016.05.057
Mcmahon, P. B., Brown, C. J., Johnson, T. D., Belitz, K., and Lindsey, B. D. (2020). Fluoride Occurrence in United States Groundwater. Sci. Total Environ. 732, 139217. doi:10.1016/j.scitotenv.2020.139217
Mwiathi, N. F., Gao, X., Li, C., and Rashid, A. (2022). The Occurrence of Geogenic Fluoride in Shallow Aquifers of Kenya Rift Valley and its Implications in Groundwater Management. Ecotoxicol. Environ. Saf. 229, 113046. doi:10.1016/j.ecoenv.2021.113046
Njuguna, S. M., Onyango, J. A., Githaiga, K. B., Gituru, R. W., and Yan, X. (2020). Application of Multivariate Statistical Analysis and Water Quality Index in Health Risk Assessment by Domestic Use of River Water. Case Study of Tana River in Kenya. Process Saf. Environ. Prot. 133, 149–158. doi:10.1016/j.psep.2019.11.006
Nong, X., Shao, D., Zhong, H., and Liang, J. (2020). Evaluation of Water Quality in the South-To-North Water Diversion Project of China Using the Water Quality Index (WQI) Method. Water Res. 178, 115781. doi:10.1016/j.watres.2020.115781
Noor, S., Rashid, A., Javed, A., Khattak, J. A., and Farooqi, A. (2022). Hydrogeological Properties, Sources Provenance, and Health Risk Exposure of Fluoride in the Groundwater of Batkhela, Pakistan. Environ. Technol. Innovation 25, 102239. doi:10.1016/j.eti.2021.102239
Patel, S. C., Khalkho, R., Patel, S. K., Sheikh, J. M., Behera, D., Chaudhari, S., et al. (2014). Fluoride Contamination of Groundwater in Parts of Eastern India and a Preliminary Experimental Study of Fluoride Adsorption by Natural Haematite Iron Ore and Synthetic Magnetite. Environ. Earth Sci. 72, 2033–2049. doi:10.1007/s12665-014-3112-1
Rashid, A., Ayub, M., Javed, A., Khan, S., Gao, X., Li, C., et al. (2021). Potentially Harmful Metals, and Health Risk Evaluation in Groundwater of Mardan, Pakistan: Application of Geostatistical Approach and Geographic Information System. Geosci. Front. 12 (3), 101128. doi:10.1016/j.gsf.2020.12.009
Rashid, A., Farooqi, A., Gao, X., Zahir, S., Noor, S., and Khattak, J. A. (2020). Geochemical Modeling, Source Apportionment, Health Risk Exposure and Control of Higher Fluoride in Groundwater of Sub-district Dargai, Pakistan. Chemosphere 243, 125409. doi:10.1016/j.chemosphere.2019.125409
Rashid, A., Guan, D.-X., Farooqi, A., Khan, S., Zahir, S., Jehan, S., et al. (2018a). Fluoride Prevalence in Groundwater Around a Fluorite Mining Area in the Flood Plain of the River Swat, Pakistan. Sci. Total Environ. 635, 203–215. doi:10.1016/j.scitotenv.2018.04.064
Rashid, A., Khan, S., Ayub, M., Sardar, T., Jehan, S., Zahir, S., et al. (2019). Mapping Human Health Risk from Exposure to Potential Toxic Metal Contamination in Groundwater of Lower Dir, Pakistan: Application of Multivariate and Geographical Information System. Chemosphere 225, 785–795. doi:10.1016/j.chemosphere.2019.03.066
Rashid, A., Khattak, S. A., Ali, L., Zaib, M., Jehan, S., Ayub, M., et al. (2019b). Geochemical Profile and Source Identification of Surface and Groundwater Pollution of District Chitral, Northern Pakistan. Microchem. J. 145, 1058–1065. doi:10.1016/j.microc.2018.12.025
Schoeller, H. (1967). “Qualitative Evaluation of Groundwater Resources,”. Water Resources Series in Methods and Techniques of Groundwater Investigations and Development UNESCO, 33, 44–52.
Singh, D., Kumar, K., Singh, C., Ahmad, T., and Bhowmik, A. (2020). Assessment of Water Quality of Holy Kali Bein Rivulet (Punjab) India, Using Multivariate Statistical Analysis. Int. J. Environ. Anal. Chem. 192, 1–17. doi:10.1080/03067319.2020.1817425
Su, C., and Puls, R. W. (2001). Arsenate and Arsenite Removal by Zerovalent Iron: Effects of Phosphate, Silicate, Carbonate, Borate, Sulfate, Chromate, Molybdate, and Nitrate, Relative to Chloride. Environ. Sci. Technol. 35 (22), 4562–4568. doi:10.1021/es010768z
Su, C., Wang, Y., Xie, X., and Li, J. (2013). Aqueous Geochemistry of High-Fluoride Groundwater in Datong Basin, Northern China. J. Geochem. Explor. 135, 79–92. doi:10.1016/j.gexplo.2012.09.003
Su, H., Kang, W., Li, Y., and Li, Z. (2021). Fluoride and Nitrate Contamination of Groundwater in the Loess Plateau, China: Sources and Related Human Health Risks. Environ. Pollut. 286, 117287. doi:10.1016/j.envpol.2021.117287
Tarki, M., Enneili, A., and Dassi, L. (2020). An Appraisal of Natural Fluorine Contamination of Paleogroundwater in Tozeur Oases, Southern Tunisia, with Emphasis on the Anthropogenic Impact. Appl. Geochem. 120, 104661. doi:10.1016/j.apgeochem.2020.104661
Thapa, R., Gupta, S., Gupta, A., Reddy, D. V., and Kaur, H. (2018). Geochemical and Geostatistical Appraisal of Fluoride Contamination: an Insight into the Quaternary Aquifer. Sci. Total Environ. 640-641, 406–418. doi:10.1016/j.scitotenv.2018.05.360
Valenzuela-Vásquez, L., Ramírez-Hernández, J., Reyes-López, J., Sol-Uribe, A., and Lázaro-Mancilla, O. (2006). The Origin of Fluoride in Groundwater Supply to Hermosillo City, Sonora, México. Environ. Geol. 51, 17–27. doi:10.1007/s00254-006-0300-7
Wang, L. F., Chen, Q., Long, X. P., Wu, X. B., and Sun, L. (2015). Comparative Analysis of Groundwater Fluorine Levels and Other Characteristics in Two Areas of Laizhou Bay and its Explanation on Fluorine Enrichment. Water Supply 15 (2), 384–394. doi:10.2166/ws.2014.124
World Health Organization (WHO) (2011). Guidelines for Drinking-Water Quality. Genava: World Health Organization.
Xu, X., Xiong, G., Chen, G., Fu, T., Yu, H., Wu, J., et al. (2021). Characteristics of Coastal Aquifer Contamination by Seawater Intrusion and Anthropogenic Activities in the Coastal Areas of the Bohai Sea, Eastern China. J. Asian Earth Sci. 217, 104830. doi:10.1016/j.jseaes.2021.104830
Yadav, K. K., Kumar, S., Pham, Q. B., Gupta, N., Rezania, S., Kamyab, H., et al. (2019). Fluoride Contamination, Health Problems and Remediation Methods in Asian Groundwater: a Comprehensive Review. Ecotoxicol. Environ. Saf. 182, 109362. doi:10.1016/j.ecoenv.2019.06.045
Yang, F., Liu, S., Jia, C., Gao, M., Chang, W., and Wang, Y. (2021). Hydrochemical Characteristics and Functions of Groundwater in Southern Laizhou Bay Based on the Multivariate Statistical Analysis Approach. Estuar. Coast. Shelf Sci. 250, 107153. doi:10.1016/j.ecss.2020.107153
Zhang, Q., Xu, P., Chen, J., Qian, H., Qu, W., and Liu, R. (2021). Evaluation of Groundwater Quality Using an Integrated Approach of Set Pair Analysis and Variable Fuzzy Improved Model with Binary Semantic Analysis: A Case Study in Jiaokou Irrigation District, East of Guanzhong Basin, China. Sci. Total Environ. 767, 145247. doi:10.1016/j.scitotenv.2021.145247
Keywords: southern Laizhou Bay, cation exchange, high-F groundwater, health risk, WQI
Citation: Liu S, Liu Y, Wang C and Dang X (2022) The Distribution Characteristics and Human Health Risks of High- Fluorine Groundwater in Coastal Plain: A Case Study in Southern Laizhou Bay, China. Front. Environ. Sci. 10:901637. doi: 10.3389/fenvs.2022.901637
Received: 22 March 2022; Accepted: 19 April 2022;
Published: 16 May 2022.
Edited by:
Nsikak U. Benson, Covenant University, NigeriaReviewed by:
Mohd Yawar Ali Khan, King Abdulaziz University, Saudi ArabiaAbdur Rashid, Quaid-i-Azam University, Pakistan
Copyright © 2022 Liu, Liu, Wang and Dang. This is an open-access article distributed under the terms of the Creative Commons Attribution License (CC BY). The use, distribution or reproduction in other forums is permitted, provided the original author(s) and the copyright owner(s) are credited and that the original publication in this journal is cited, in accordance with accepted academic practice. No use, distribution or reproduction is permitted which does not comply with these terms.
*Correspondence: Xianzhang Dang, aHlkcm9keHpAMTYzLmNvbQ==