- 1Federal University of Paraná, Curitiba, Brazil
- 2State University of Maringá, Maringá, Brazil
- 3Swedish University of Agricultural Sciences, Uppsala, Sweden
- 4Agroscope (Switzerland), Zürich, Switzerland
Single farming systems (SFS) such as monocultures may negatively affect soil structural quality. This study tested the hypothesis that integrated farming systems (IFS), i.e., the combination of cropping and forestry and/or livestock farming, improves soil structural quality, root development and soil organic carbon. An experimental area was set up in 2012 at the Canguiri experimental farm belonging to the Federal University of Paraná, Southern Brazil. The soils are predominantly Ferralsols. The experimental treatments representing different farming systems, organized in a random block design with three replicates, were: Forestry (F), Conventional Crop Production (C), Livestock (L), and integrated Crop-Forestry (CF), Crop-Livestock (CL), Livestock-Forestry (LF), and Crop-Livestock-Forestry (CLF). In situ measurements and sampling were carried out in the 0–0.3 m layer during summer 2019/20, and included soil penetration resistance (PR), soil structural quality based on visual evaluation of soil structure (SqVESS scores), root length (RL), root volume (RV) and soil organic carbon content (SOC). Soil structural quality, penetration resistance, root length and volume, and SOC varied between farming systems, but no significant differences were found between single (C, L, F) and integrated farming systems (CF, CL, LF, CLF). The single system Forestry (F) and the integrated systems including forestry (LF, CF, CLF) tended to have higher SqVESS scores, i.e. poorer soil structural quality, and higher PR, which we associate with the generally drier soil conditions that are due to higher soil water uptake and higher interception and reduce the frequency of wetting-drying cycles. Roots were concentrated in the shallow soil layer (0–0.1 m depth), and this was especially pronounced in the Crop (C) single farming system. Based on the measured values, our results suggest an acceptable soil structural quality in all farming systems. Our data revealed strong, significant relationships between soil structural quality, penetration resistance, root growth and SOC, demonstrating that improvements in soil structure results in lower soil penetration resistance, higher root volumes and higher SOC, and vice versa. Soil PR was positively correlated with SqVESS (R2 = 0.84), indicating that better soil structural quality resulted in lower soil mechanical resistance. This, in turn, increased root length and volume, which increases carbon input to soil and therefore increases SOC in the long run.
1 Introduction
The Sustainable Development Goals (SDGs) proposed by the United Nations (UN) aim to achieve a better quality of life in a sustainable way for all. To achieve the SDGs, it is necessary to adopt soil management to improve the health of agroecosystems (Keesstra et al., 2016). In Brazil, agriculture has been intensified through the use of a set of technologies since the green revolution in the 1950s. Considerable effort has been done to develop genuinely sustainable approaches to agriculture, including crop breeding for sustainability (Meena et al., 2020; Brooker et al., 2021). Agricultural practices with techniques that combine food production with little impact on other ecosystem functions ensure soil conservation and agricultural sustainability (Rahman et al., 2017).
No-tillage have been widely adopted in Brazil, and it is often combined with other conservation practices such as crop rotation (Derpsch, 2021; FEBRAPDP, 2021). No-tillage has become an important practice to achieve sustainable agricultural production systems, especially when it is practiced in combination with crop rotation and permanent crop residues on the soil surface (Derpsch et al., 2010; Bonetti et al., 2015; Bonetti et al., 2018). Currently, in Brazil, no-tillage is practiced on more than 33 million hectares (SIDRA/IBGE, 2021), which is about one sixth of the total global area under no-tillage (Kassam, et al., 2020). The “planting green technique” refers to no-till planting of primary crops into standing cover crops (Duiker et al., 2017). Few studies have reported on the use of no-tillage under the “planting green technique,” probably because this is a relatively new practice that is still under evaluation (Duiker et al., 2017), but has shown several benefits, e.g. greater amounts of mulch in the crop that reduce weed pressure.
Although single farming systems are most common in Brazilian farms, integrated farming systems are becoming more and more popular in Brazil. They are referred as agricultural systems that integrate livestock, forestry, and crop production (Soni et al., 2014), aiming at producing various products such as meat, milk, wool, grains, and biomass, and adopting mixed-farming, crop rotation and intercropping production systems (Moraes et al., 2014). These systems have been adopted both by small and large farms in Brazil (Bendahan et al., 2018). Despite the challenges for its (re-)integration in some regions (Schut et al., 2021), such systems have several benefits (Sharma et al., 2019). No-tillage is one of the pillars of integrated farming systems, being adopted in more than 65% of these systems (Valani et al., 2020), but few studies have analyzed soil and water conservation under integrated farming systems (Moraes et al., 2014).
Integrated farming systems have been adopted in the following configurations: integrated crop-livestock-forestry (CLF), integrated crop-forestry (CF), crop-livestock (CL), and livestock-forestry (LF). According to Zhang et al. (2019), the adoption of crop-livestock has a positive effect on soil quality, mainly due to the incorporation of organic matter. They indicated that moderate grazing stimulates the regrowth of forage plants and root growth leading to the formation of macro aggregates, thus improving soil structure, infiltration, and water availability. Soil compaction caused by animal trampling can be controlled or minimized through adequate animal stocking (Bonetti et al., 2018).
Soil structure affects root penetration, the amount of available water to plants, and other key soil properties and processes. According to Flávio Neto et al., 2015, degraded soils can be recovered using Brachiaria (syn. Urochloa spp) as forage grass in integrated farming systems. Soil structure can be evaluated through different approaches including visual methods. The visual evaluation of soil structure (VESS) method has been frequently used to assess soil structural quality (Pulido-Moncada et al., 2014; Tormena et al., 2016; Cherubin et al., 2017; Tuchtenhagen et al., 2018; Franco et al., 2019; Paiva et al., 2020; Çelik et al., 2020; Mutuku et al., 2021). The VESS method is applied globally, as it is an easy method that allows soil structure assessment directly on-farm. The VESS is a semi-quantitative method, and includes several aspects of structure and rooting to infer about the soil structural quality through assigned scores (Guimarães et al., 2017). Another important aspect of soil structure is the soil penetration resistance. Colombi et al. (2018) demonstrated that the interactive effects between soil penetration resistance, root architecture, and plant water uptake determine water accessibility by roots and ultimately affect crop yield. Popoya et al. (2016) noticed an increase in root tortuosity and reduced root elongation due to an increase in soil penetration resistance, which has been recently confirmed by Moraes and Gusmão (2021). The assessment of soil structural quality and root properties in integrated farming systems is needed to evaluate its adoption as a sustainability strategy for agricultural production.
In our study, integrated and single pesticide-free farming systems were evaluated in terms of soil structural quality and root growth. The aims of our study were: 1) to measure soil penetration resistance and VESS as indicators of soil structural quality; 2) to determine root properties and soil organic carbon down to 0.30 m depth, and; 3) to explore relationships between soil organic carbon, root growth properties and soil structural quality measured by VESS and penetrometer resistance.
2 Material and Methods
2.1 Experimental Area
The study was carried out at the Agricultural Technological Innovation Center (NITA) at the Canguiri experimental farm, belonging to the Federal University of Paraná (UFPR), Pinhais municipality, Paraná state, Southern Brazil (25°24′03″ S, 49°07′10″ W). The regional climate is humid-temperate (Cfb), with a mean annual rainfall and temperature of 1,602 mm and 17°C, respectively (Alvares et al., 2013). Rainfall and temperature data during the study period are presented in Supplementary Figure S1. The soils are identified as Ferralsols, with minor occurrence of Cambisols (WRB/FAO), differing mainly by the B horizon depth, or “Latossolo Vermelho and Cambissolo Háplico” according to Brazilian soil classification system (EMBRAPA, 2018). Soil texture is clayey with an average of 519 g kg−1 clay, 112 g kg−1 silt, and 369 g kg−1 sand.
The NITA is located in an environmental preservation area (Figure 1) according to Brasil (2000), on the banks of the river Iraí, which supplies water to the city of Curitiba and the metropolitan region. Therefore, the area must be managed pesticide free. Until 2011, the area was also used for training and testing of agricultural machinery traffic, which led to soil physical degradation (soil loss by erosion and compacted patches), as shown in Supplementary Figure S2. Before the implementation of the experimental area in 2012, maize (Zea mays) was grown under conventional tillage. The soil was chisel ploughed down to 0.40 m depth and subsequently harrowed (<0.20 m). After that, the experimental area received between 8 and 10 ton ha−1 sewage sludge treated by the N-VIRO® process for acidity correction as described by Kruchelski et al. (2021). Then, the area was cultivated with black oat (Avena strigosa) as a cover crop, which was fertilized with 100 kg ha−1 of P2O5. Soil chemical attributes evaluated in 2013 were: soil organic carbon (SOC) = 20 g kg−1, pH = 5.2, a CEC of 13 cmolc kg−1 and 60% for base saturation.
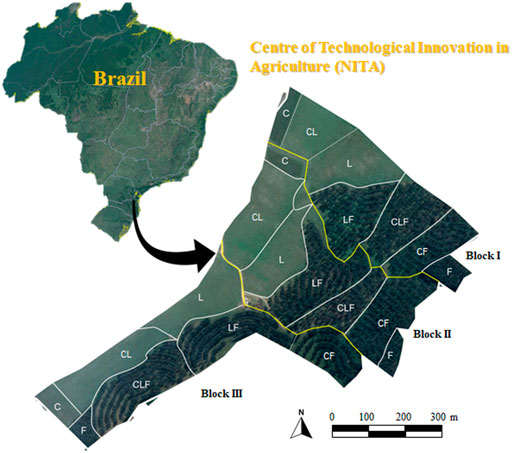
FIGURE 1. The experimental area at the Canguiri farm of the Federal University of Paraná. Treatments: C, conventional crop production; L, livestock; F, forestry; CF, integrated crop-forestry; CL, crop-livestock; LF, livestock-forestry; CLF, crop-livestock-forestry.
The experiment was established in 2012 in a randomized block design with three replicates, with three single farming systems (SFS), namely Forestry (F), Crop (C), Livestock (L), and four integrated farming systems (IFS) treatments including Crop-Forestry (CF), Crop-Livestock (CL), Livestock-Forestry (LF), and Crop-Livestock-Forestry (CLF). The plots varied in size between 0.2 and 1 ha−1 (areas without livestock) and >1 ha−1 for areas with livestock (Figure 1).
Soil fertilization has been done on the whole area by broadcasting 180 kg ha−1 N of urea; 45 kg ha−1 of P2O5 (natural phosphate) and 120 kg ha−1 of K2O (potassium chloride. Sowing has been done by a no-tillage seeder using the “planting green” technique without herbicides for desiccation, in all systems except in Forestry. In treatment C, crop succession has been carried out using black oat as a cover crop and maize as a cash crop. For L, black oat is cropped for the winter pasture and guinea grass cv. aries [Megathyrsus maximus (Jacq.) B. K. Simon and S. W. L. Jacobs cv. aries] as a summer pasture. Grazing is by animals, predominantly of Angus breed, since 2015, for around 10 months per year. There are three fixed test animals per plot and a variable number of regulatory animals, with an average of 1.6 animal unity (AU) per hectare (AU ha−1). In system F, eucalyptus (Eucalyptus benthamii) was planted in 2013, with seedlings of seminal origin, using fertilization of 16 g of N pit−1, 40 g of K2O pit−1, and 40 g of P2O5 pit−1, under a spatial arrangement of 3 m × 2 m, with a final density of 1,667 trees ha−1. The CLF, CF, and LF systems are in an alley-cropping spatial design with the seedlings planted at single rows, following the contour lines, at 14 m × 2 m spacing, obtaining 357 trees ha−1 and occupying about 14.3% of the area of the integrated systems. Details about forest component can be found in Kruchelski et al. (2021). In LF, the animal component followed the stocking rate adjustments described in system L, while the CF treatment had in the first 3 years black oats cultivated in winter, and sunflower (Helianthus annuus) varieties Aguará 4 and Aguará and maize hybrids 2B655HX, 30F53VYHR–early and P2866H–super early in summer. From 2015/2016 onwards, only maize is cultivated in the summer. The CL started with the pasture components, until the winter of 2015, but without grazing, and from then on, grazing with animals was started until the summer of 2016. Then, the first crop cycle was established, with black oats as a cover crop in winter, followed by maize in the summer of 2017, as described in treatment C, in a ley farming arrangement. The CLF integrated system followed the same arrangement as the LF.
In treatments with crops, between 2017 and 2019/2020, maize was harvested for the evaluation of yield. The machines used for the mechanized operations were: a New Holland tractor, model TL 75 E 4 × 4 (∼3,880 kg mass); a New Holland harvester, model TC 59 (∼10,300 kg mass) for the harvest in 2016/17 crop season. The implements used were a Marchesan Tatu seeder (∼2,595 kg mass), a Baldan mower (∼1,000 kg mass) used to control weeds, and a Marchesan Tatu fertilizer and limestone distributor (800 kg mass).
2.2 Soil and Roots Sampling, and in situ Measurements
Soil and root sampling were performed randomly at four locations within each plot, in summer 2019/20, a period with expected high amounts of plant roots for C–cash crop and L–pasture. Four undisturbed soil core samples were taken with a cup auger (Ratuchne et al., 2017) in the 0.0–0.10, 0.10–0.20, and 0.20–0.30 m layers depth, totaling 252 samples (4 samples × 3 soil layers × 7 farming systems × 3 experimental blocks). Disturbed soil samples were taken to determine soil water content, soil texture, and total soil organic carbon (SOC). Three soil penetration resistance (PR) measurements were taken near the sampling points at soil moisture close to field capacity, using a Falker® electronic penetrometer down to 0.30 m depth and a mean PR value was calculated for each studied layer.
The visual evaluation of the soil structure was carried out following the method proposed by Ball et al. (2007) and Guimarães et al. (2011). The scores identified in each soil layer were weighted and paired within the three depth layers (0–0.1, 0.1–0.2 and 0.2–0.3 m depth) to proceed with the correlation’s studies. Structural quality (SqVESS) reflect values between “good” structural quality (Sq1) and “poor” structural quality (Sq5).
2.3 Soil and Roots Laboratory Measurements
Undisturbed soil samples were manually disaggregated in a plastic tray, and washed by water into a set of sieves with 2.0, 1.0, and 0.5 mm of mesh size. This procedure was standardized in 10 replication and, after washing, the roots were placed in 50 ml pots containing 70% alcohol and stored at a temperature of 2°C. Subsequently, the roots were scanned using the WinRhizo® software, to obtain the following properties: root length (RL), and root volume (RV). After being scanned, the roots were weighed and taken to the oven between 45 and 65°C until reaching constant weight. Then, the dry mass of roots (RDM) was obtained. For soil texture, the Bouyoucos hydrometer method was used (Gee and Or, 2002), and for SOC the colorimetric method according to Quaggio and van Raij, 1979.
2.4 Statistical Analysis
All data were submitted to the test of homogeneity of variance (Bartlett) and normality (Shapiro-Wilk). Those data that did not reach the normality assumptions were submitted to the BoxCox transformation (Box and Cox, 1964). Then, the data were submitted to analysis of variance (ANOVA) and the means were compared by Tukey test (p < 0.05). To quantify the correlation between the studied properties, the root properties, SOC, PR, and VESS were submitted to Spearman correlation (p < 0.05). Regression analyses were performed to determine the relationships between properties. Statistical analyzes were performed in the R® software environment (Team R. Core, 2020).
3 Results
The SqVESS scores and PR varied between the studied farming systems, but most differences were not statistically significant (Table 1). For both PR and VESS, forestry (F) had the highest mean values, with PR = 2.2 MPa and Sq = 3.1, respectively. SqVESS of F was statistically different from Crop (C). The integrated farming systems showed no significant difference in structural quality (SqVESS = 2.7; 2.4; 2.5; and 2.8, for LF, CL, CF, and CLF, respectively) compared with SFS (SqVESS = 2.2; 2.5; and 3.1, for C, L, and F, respectively). SOC was around 40 g kg−1 at 0–0.30 m depth, with no statistical difference (p > 0.05) between farming systems.
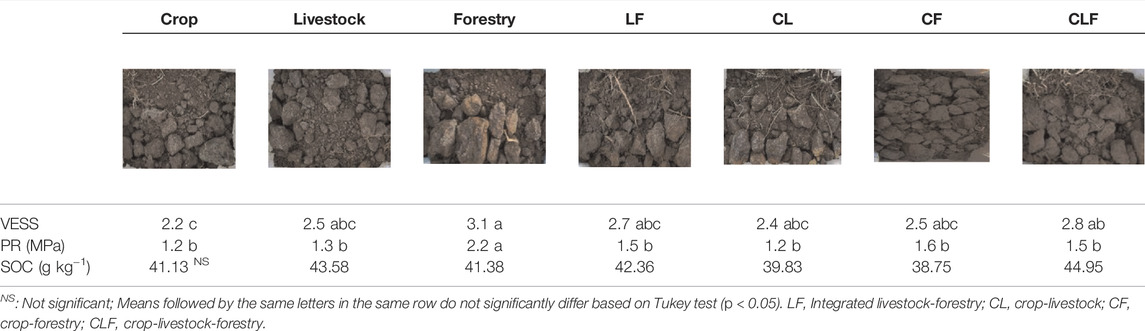
TABLE 1. Mean values of soil penetration resistance (PR), soil structural quality scores from visual evaluation of soil structure (VESS), and total organic carbon content (SOC) for different farming systems, at 0–0.30 m depth.
Average values of root length, root volume and root dry matter are shown in Table 2. Root length (but not root volume) was statistically different between farming systems, for 0–0.10 and 0.10–0.20 m layers. Root length was significantly lower in CF than in Crop at the 0–0.10 m of depth, while for the 0.10–0.20 m layer, root length in Forestry was lower than in L. No significant differences were found among the other treatments, in both soil layers. In the 0.20–0.30 m layer, no significant differences among farming systems were found for root properties. Roots were mostly concentrated at the 0–0.10 m depth, and root lengths and root volumes decreased with depth, as shown in Table 2.
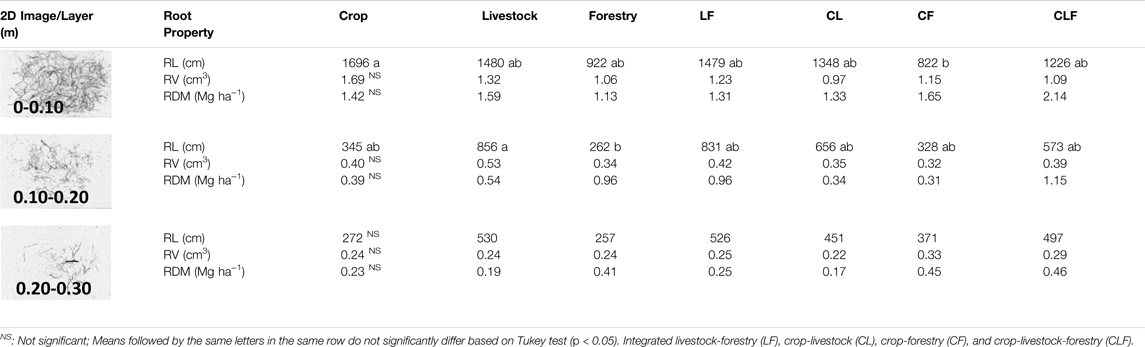
TABLE 2. Mean values of root length (RL), root volume (RV), and root dry matter (RDM) for different farming systems.
The root properties (root length, root volume) and SOC were negatively correlated (Spearman) with SqVESS (rs = −0.57, −0.62, and −0.62, respectively), and PR (rs = −0.64, −0.63, and −0.63, respectively). A positive relationship between SqVESS and PR (rs = 0.81) was found. Root properties and SOC was more strongly related to soil penetration resistance than SqVESS. Figure 2 shows a nonlinear relationship between root properties and SOC, and between SqVESS and PR. Our data show that for values of SqVESS between 3.0 and 4.0 (i.e., moderate to poor soil structural quality), root lengths and root volumes were low, with values of RL < 5 m and RV < 0.35 cm3, respectively (Figures 2A,C). Root length and volume were low for PR values between 1.7 and 2.2 MPa (Figures 2B,D). Based on the regression curves (Figures 2A–D), it was possible to estimate the influence of soil penetration resistance on root properties. PR values around 4 MPa were associated with a reduction in root length and root volume of around 75%. Similarly, at 75% reduction in root length and volume, respectively, was associated with values of SqVESS larger than 3. In addition, there is a negative relationship between SOC for both SqVESS and PR (Figures 2E,F).
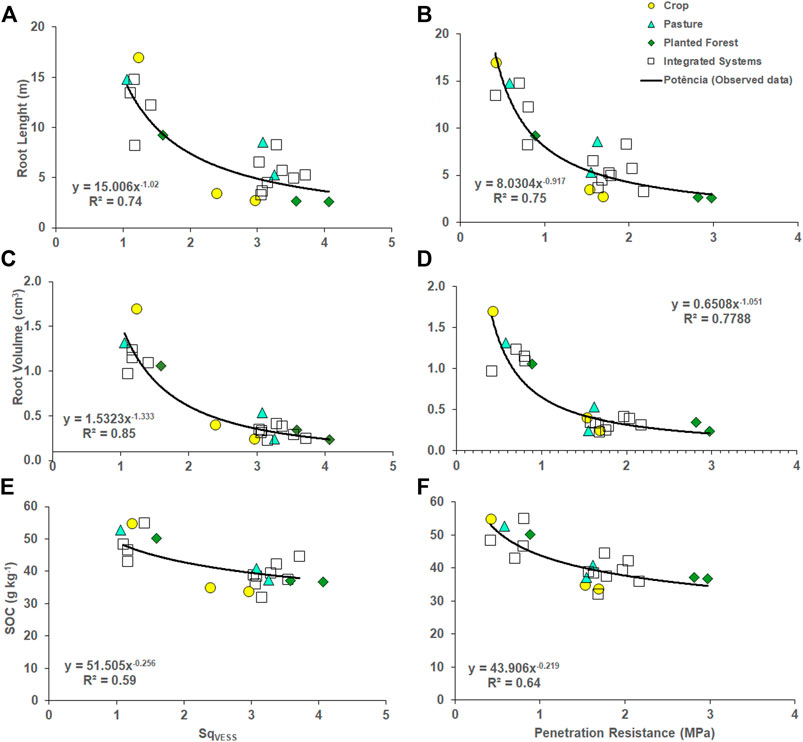
FIGURE 2. Relationship between root properties (root length and root volume), and total organic carbon (SOC), respectively, with soil quality (SqVESS) from visual evaluation of soil structure and soil penetration resistance (PR).
4 Discussion
4.1 Soil Structural Quality Based on Visual Evaluation of Soil Structure
Soil structural quality presented distinct SqVESS between farming systems. Farming system Forestry (F) had rounded and sub-angular aggregates with few visible pores and only a few fine roots compared to the other systems, which resulted in the highest SqVESS (Sq = 3.1). For the single farming systems, C and L had small and rounded aggregates, which resulted in lower SqVESS; however, with exception of F, the soil structural quality for single farming systems was not significantly different than for integrated farming systems. Despite the statistical differences found, all studied farming systems had acceptable soil structure, with SqVESS < 3 (Guimarães et al., 2011), except for the F system with SqVESS = 3.1 indicating moderate soil structural quality (Ball et al., 2017). The higher SqVESS and higher soil penetration resistance for forestry treatment might be explained by drier soil conditions at the 0–0.25 m of depth (∼0.27 kg kg−1) compared to the other treatments (∼0.35 kg kg−1). Acoording to Madani et al. (2018), evapotranspiration is higher in forests than grassland. In addition, rainfall data at the period of sampling (Supplementary Figure S1) indicates higher interception by trees compared with grassland, leading to a lower soil water content. Drier soil is characterized by higher soil cohesion, and this increases soil penetration resistance and decreases root development.
Several studies indicate that both systems, i.e., single and integrated farming systems, may provide suitable physical quality for plant development. Tuchtenhagen et al. (2018) found values for SqVESS of 2.5, 1.9, and 3.1, respectively, for cropping under no-tillage, native grassland, and crop-livestock, which is close to the scores found in our study. These authors mentioned that undisturbed soil surface, vegetation cover, and crop residues resulting from crop successions increased root biomass and SOC atin the surface layers, contributing to the improvement in soil structure. In our study, no-tillage, the management with low machinery traffic intensity, adequate animal trampling (<2 AU), proper fertilization, and large amounts of residues provided by winter and summer grasses had positive effects on soil structure. According to Carvalho et al. (2018) and Salton et al. (2014), proper pasture management encourages pasture regrowth and greater root growth, increasing inputs of organic matter into the soil. We highlight that root exudates stimulate the activity of soil microorganisms, leading to the formation of biopores that are important for air and water fluxes and preferentially used by new roots.
Single farming system F had the highest SqVESS, indicating moderate soil structural quality (Ball et al., 2017). Soil structural quality in this system could be improved by small changes in the management, e.g. by using plants with strong root systems (Guimarães et al., 2011). Introduction of intercropping species that can meet such conditions, with strong root systems, may be an option, such as pinto peanut (Arachis pintoi). However, eucalyptus must be at least 2 years old to avoid competition between species. When SqVESS approaches 4.0, it may be a warning sign that calls for a direct intervention with changes in management (Ball et al., 2007, 2017). In our case, high scores may be due to previous soil management in the studied area, which had a history of excessive machinery traffic and disturbance (Dominschek et al., 2018; Supplementary Figure S2). Our results suggest that soil structure is recovering more slowly in F, probably due to a lack of grasses in the F system, and because less water arrives on the soil surface due to interception from trees (Supplementary Figure S1). The SqVESS results obtained in C (Sq = 2.2), CL (Sq = 2.4), and CLF (Sq = 2.8) are close to the results found by Demétrio et al., 2022 that indicate that no-tillage system and cattle trampling increase SqVESS when compared to native forest (Sq = 1.53). For L, and LF, SqVESS were 2.5, and 2.7, respectively (Table 1), indicating that despite cattle trampling causing some negative alterations to soil structure, these IFS maintained adequate soil structural quality for plant development. According to Abdalla et al. (2018), the grass root system under low trampling pressure and rotational grazing is stimulated by compensatory growth due to herbivores, increasing new root growth and carbon input into the soil.
4.2 Soil Resistance to Soil Penetration Resistance in the Farming Systems
The highest mean value of PR was found in the F system (2.2 MPa), which corroborates with the SqVESS results. All integrated systems as well as C and L presented mean values of PR that are non-limiting for root plants. According to Morais et al. (2020) and Rosseti and Centurion (2017), PR values between 2.0 and 3.0 MPa do not cause a decrease in crop yield under no-tillage. Thus, our findings indicate that these farming systems preserve soil structural quality that is adequate for root development and yield. The efficiency of IFS in recovering soil structure has been reported by Polanía-Hincapié et al. (2021). Besides having found high PR values (3.8 MPa) in silvopasture farming system on a degraded Ferralsol, they observed soil structural improvements in comparison with its initial state. Similarly, Flávio Neto et al., 2015 reported that degraded soils can be recovered by pastures in IFS. We measured an increase in PR with soil depth. The increase was especially pronounced in the F system, which we attribute to the generally drier soil conditions (Supplementary Figure S1) and less heterogeneity of plant species cultivated. Consequently, roots are less exposed to ideal soil water conditions, affecting both plant growth and development as well as soil resilience.
4.3 Soil Organic Carbon
The SOC was ≥39 g kg−1 for all farming systems at the depth of 0–0.30 m. SOC >20 g kg−1 is considered very high for Paraná state, Brazil (Pauletti and Motta, 2019). According to Wiesmeier et al. (2019), climate is the major factor driving soil organic carbon storage at regional to global scales, while at regional or sub-regional scale, other factors such as vegetation, microorganisms/fauna, parent material, texture, and land-use and soil management play a role too. The experimental area is in a temperate summer climate (Cfb, second Köppen classification), the soil has a clayey texture, and management is pesticide free with minimum soil disturbance, and crop diversity is high in integrated farming systems. The combination of these factors likely results in enhanced soil organic carbon (Salton et al., 2014). Moreover, pesticide-free farming systems affect positively the biological functions of microorganisms and chemical processes (Meena et al., 2020). Data of SOC found in our study (Table 1) show that SOC has increased since the beginning of the experiment in 2013, where mean values did not surpass 30 g kg−1 at a depth of 0–0.20 m (Dominschek et al., 2018). Our data suggest that all farming systems studied, which include specific and conservative management practices, favored positively SOC content, with positive impacts on soil structural quality. No-tillage is considered a key practice for soil carbon sequestration, and for preventing structural and physical soil degradation (Calonego et al., 2017; Vizioli et al., 2021), and when adopted in IFS, no-tillage could minimize the risk of soil and environmental degradation (Ray et al., 2020).
4.4 Root Properties
Root length, root volume, and root dry matter are presented in Table 2, indicating that roots were concentrated in the surface layer (0–0.10 m). Although non-significant, root dry matter was higher in the CLF system, probably due to Eucalyptus root morphology, being thicker than grasses, and due to the combination of crop and livestock. The C and L systems had a greater volume of roots down to 0.20 m of depth, while F and the integrated systems had smaller amounts of roots at this depth. In the L farming system, there is an influence of cattle that stimulate regrowth and root growth as they feed (Bonetti et al., 2018), and deposit feces and urine, increasing SOC and keeping the soil more biologically active. For C, the specific management with reduced machinery traffic and fertilization provides a suitable environment for root development, which was detected by the highest root length mean value, which was also the only root parameter that indicated significant differences between farming systems. Our data indicate good management strategy of all farming systems, considering the fact that root length can be related with soil hydraulic properties (Shi et al., 2021), contributing to better soil water storage capacity and water and gas fluxes capacity. Vanhees et al. (2021), studying maize roots in compacted and non-compacted soils, showed that the ability of roots to grow to depth through compacted soil is not dependent on the amount of roots but on the favorable conditions provided by biopores. The production of roots in cropping systems stimulates biological soil activity, which positively affects soil structure and soil physical quality (Gamboa et al., 2020). Fine roots of the grasses have been shown to provide improvements to soil hydraulic properties, aggregate stability, and soil porosity (Hao et al., 2020; Chen et al., 2021; Shi et al., 2021).
4.5 Relationships Between Soil Structure, Root Properties and Soil Organic Carbon
Root length, root volume, and SOC are linked to soil structural quality, as shown in Figure 2, as well as by Spearman correlations (rs). The negative relationship between soil structural quality and SOC has been reported by several authors (Pulido-Moncada et al., 2014; Tuchtenhagen et al., 2018; Cherubin et al., 2019; Mutuku et la., 2021). Likewise, positive correlation between SqVESS and PR have been reported (Castioni et al., 2018; Cherubin et al., 2019). Here, we demonstrate how soil structure, root growth and soil organic carbon are linked. Based on the regression models found in this study, root length and root volume were reduced by about 75% for SqVESS = 4 and PR = 3 MPa, compared with soil that had SqVESS = 1 and PR = 0.5 MPa. These results corroborate with critical values for root development indicated in the literature (Taylor et al., 1966; Ehlers et al., 1983; Bengough et al., 2011; Colombi et al., 2018). Values of SqVESS > 3.0 indicate soil structural degradation, which require improvements of the current soil management practices.
Previous studies suggest that biopores are important as they can facilitate root growth (Cavalieri et al., 2009; Vanhees et al., 2021). A strong decrease in root length and volume under the highest PR values were found. This soils were associated with lower levels of soil organic carbon, evidencing the importance of carbon inputs from roots into the soil. Coblinski et al. (2019) studied the same experimental area, and showed that soil bulk density, porosity, soil water availability, as well SOC influence soil structural quality. According to Colombi et al. (2018), interactions between root architecture, plant water uptake, soil moisture, and soil penetration resistance are little studied. Our data of SqVESS and PR were obtained in moist soil, at a water content close to field capacity, but PR increases when soil dries, especially so in compacted soil, which reduces root development. Colombi et al. (2018) mention that water limiting crop yields may result from limiting water accessibility by roots (and not limited water availability), which is largely caused by high soil penetration resistance that decreases root growth and ultimately crop productivity. We observed that the pesticide-free management along with adequate fertilizer use have provided sufficient root development for the species grown at our site. This is supported by the satisfactory yields in the different systems as shown by Kruchelski et al. (2021). Moreover, Dominschek et al. (2021) indicated that the management applied in the experimental area is an economically viable and efficient non-chemical strategy to manage weeds and produce grains. Forage yield and beef production also presented good results (Campos, 2019; Cavalieri-Polizeli et al., 2021).
A positive relationship between PR and VESS (Figure 3) was also observed in other studies (Cherubin et al., 2017; Castioni et al., 2018; Tuchtenhagen et al., 2018; Çelik et al., 2020). Soil structural quality is classified as firm for values of 3.0 < SqVESS < 4.0, which corresponded to values of 1.76 < PR < 2.40 MPa (close to field capacity). However, PR is dependent on soil moisture, which can vary within days, weeks and seasons, and consequently, vary the conditions for root growth and development. However, critical PR values, such as more than 3.5 MPa, probably might be easily found, when soil remains dry for a considerable period. Ehlers et al. (1983) estimated that relative elongation rate of oats roots ceases at 4.9 MPa, which is very close to our finding (4.5 MPa) where root length was reduced by 75% The strong correlation between VESS and PR suggests that VESS can be used to detect possible harmful conditions related to critical PR values for root growth.
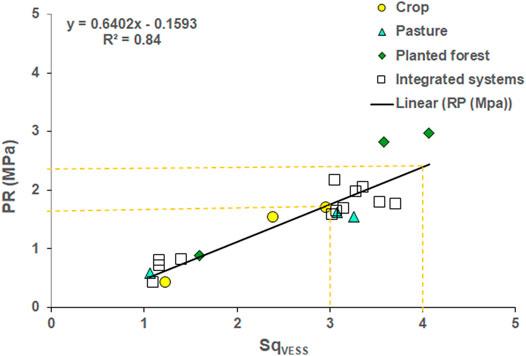
FIGURE 3. Relationship between soil penetration resistance (PR) and soil structural quality score (SqVESS) based on visual evaluation of soil structure.
The negative correlations between root properties and PR, SqVESS, and SOC, clearly show the importance of roots for soil structural quality. However, the results obtained in this study confirmed only partially the hypothesis that IFS promotes better soil structural quality compared to SFS. Management systems that favor improvement and conservation of soil physical properties are key for the environmental sustainability of agricultural production (Tuchtenhagen et al., 2018). Not all the studied integrated farming systems showed better performance for roots, for example, crop-forestry (CF) was less effective to stimulate root growth. Ray et al. (2020) pointed out that the adoption of IFS instead of SFS could enhance farm productivity, crop diversity, employment opportunity, and annual income for overall improvement of livelihood through efficient utilization of natural resources. However, the results for CF indicate that it is not enough just to integrate systems, but that the choice of crops and management have an influence on the success of such cropping strategies.
5 Conclusion
In general, both single farming systems (SFS) and integrated farming systems (IFS) presented adequate soil structural quality. The single Forestry system and integrated systems including forestry tended to have poorer soil structural quality and higher soil penetration resistance, but still adequate, which was associated with the generally drier soil conditions. Thus, our data could not support the hypothesis that integrated farming systems promote soil structural quality. However, the similarity between SFS (C and L) and IFS in providing good soil structural quality is probably due to the specific conditions of the study (pesticide-free in combination with mineral fertilizers, and no-till using the planting green technique). We found strong relationships between scores of soil structural quality (SqVESS), soil mechanical resistance (PR), SOC and root properties. Root length and volume were reduced by about 75% for SqVESS = 4 and PR = 4 MPa. These data demonstrate the positive feedbacks between soil structure, root growth and soil carbon input.
Data Availability Statement
The raw data supporting the conclusion of this article will be made available by the authors, without undue reservation.
Author Contributions
KC-P contributed to the conception, design of the study and wrote all sections of the manuscript. FM and KC-P organized the database, performed the statistical analysis, and wrote the first draft of the manuscript. CT wrote sections of the manuscript. AM contributed with experiment conception since the beginning, and read. KC-P, CT, and TK contributed to manuscript revision, read, and approved the submitted version.
Funding
This study was financially supported by Coordination for Higher Education in Brazil (CAPES) with a scholarship to FM, and by the National Council for Scientific and Technological Development (Processo: Chamada Pública: 420140/2016-6 Universal 01/2016) through funding research to AM.
Conflict of Interest
The authors declare that the research was conducted in the absence of any commercial or financial relationships that could be construed as a potential conflict of interest.
Publisher’s Note
All claims expressed in this article are solely those of the authors and do not necessarily represent those of their affiliated organizations, or those of the publisher, the editors and the reviewers. Any product that may be evaluated in this article, or claim that may be made by its manufacturer, is not guaranteed or endorsed by the publisher.
Acknowledgments
The authors thank all graduate and undergraduate students involved in the experiment during the years, taking care of animals, plants, and soils.
Supplementary Material
The Supplementary Material for this article can be found online at: https://www.frontiersin.org/articles/10.3389/fenvs.2022.901302/full#supplementary-material
Supplementary Figure S1 | Monthly mean rainfall and temperature for the study period.
Supplementary Figure S2 | Experimental area in 2011, one year before initiation of experiments, with signs of degradation. Arrows: red indicate soil loss by erosion, yellow indicate intensive traffic, and black indicate soil covering by plants, some signs of soil degradation. Image from Google Earth.
References
Abdalla, M., Hastings, A., Chadwick, D. R., Jones, D. L., Evans, C. D., Jones, M. B., et al. (2018). Critical Review of the Impacts of Grazing Intensity on Soil Organic Carbon Storage and Other Soil Quality Indicators in Extensively Managed Grasslands. Agric. Ecosyst. Environ. 253, 62–81. doi:10.1016/j.agee.2017.10.023
Alvares, C. A., Stape, J. L., sentelhas, P. C., de Moraes Gonçalves, j. L., and Sparovek, G. (2013). Köppen's Climate Classification Map for Brazil. Meteorol. Z. 22 (6), 711–728. doi:10.1127/0941-2948/2013/0507
Ball, B. C., Batey, T., and Munkholm, L. J. (2007). Field Assessment of Soil Structural Quality - a Development of the Peerlkamp Test. Soil Use Manag. 23 (4), 329–337. doi:10.1111/j.1475-2743.2007.00102.x
Ball, B. C., Guimarães, R. M. L., Cloy, J. M., Hargreaves, P. R., Shepherd, T. G., and Mckenzie, B. M. (2017). Visual Soil Evaluation: a Summary of Some Applications and Potential Developments for Agriculture. Soil Tillage Res. 173, 114–124. doi:10.1016/j.still.2016.07.006
Bendahan, A. B., Poccard-Chapuis, R., De Medeiros, R. D., Costa, N. L., and Tourrand, J. F. (2018). Management and Labour in an Integrated Crop-Livestock-Forestry System in Roraima, Brazilian Amazonia. Cah. Agric. 27. doi:10.1051/cagri/2018014
Bengough, A. G., McKenzie, B. M., Hallett, P. D., and Valentine, T. A. (2011). Root Elongation, Water Stress, and Mechanical Impedance: a Review of Limiting Stresses and Beneficial Root Tip Traits. J. Exp. Bot. 62, 59–68. doi:10.1093/jxb/erq350
Bonetti, J. d. A., Paulino, H. B., Souza, E. D. d., Carneiro, M. A. C., and Caetano, J. O. (2018). Soil Physical and Biological Properties in an Integrated Crop-Livestock System in the Brazilian Cerrado. Pesq. Agropec. Bras. 53 (1), 1239–1247. doi:10.1590/S0100-204X2018001100006
Bonetti, J. d. A., Paulino, H. B., Souza, E. D. d., Carneiro, M. A. C., and Silva, G. N. d. (2015). Influência Do sistema integrado de produção agropecuária no solo e na produtividade de soja e braquiária. Pesqui. Agropecu. Trop. 45, 104–112. doi:10.1590/1983-40632015v4529625
Box, G. E. P., and Cox, D. R. (1964). An Analysis of Transformations. J. R. Stat. Soc. Ser. B Methodol. 26, 211–252. doi:10.1111/j.2517-6161.1964.tb00544.x
Brasil (2000). Decreto estadual No 2200, 12DE junho de 2000. Regulamento ao zoneamento ecológico-econômico da área de proteção ambiental do iraí. Curitiba: PR.
Brooker, R. W., George, T. S., Homulle, Z., Karley, A. J., Newton, A. C., Pakeman, R. J., et al. (2021). Facilitation and Biodiversity-Ecosystem Function Relationships in Crop Production Systems and Their Role in Sustainable Farming. J. Ecol. 109, 2054–2067. doi:10.1111/1365-2745.13592
Calonego, J. C., Raphael, J. P. A., Rigon, J. P. G., Oliveira Neto, L. d., and Rosolem, C. A. (2017). Soil Compaction Management and Soybean Yields with Cover Crops under No-Till and Occasional Chiseling. Eur. J. Agron. 85, 31–37. doi:10.1016/j.eja.2017.02.001
Campos (2019). Produção de bovinos de corte, características de pasto e comportamento ingestivo em sistemas integrados de produção agropecuária. Brazil: Thesis, Curitiba, PR.
Carvalho, P. C. d. F., Peterson, C. A., Nunes, P. A. d. A., Martins, A. P., de Souza Filho, W., Bertolazi, V. T., et al. (2018). Animal Production and Soil Characteristics from Integrated Crop-Livestock Systems: toward Sustainable Intensification. J. animal Sci. 96 (8), 3513–3525. doi:10.1093/jas/sky085
Castioni, G. A., Cherubin, M. R., Menandro, l. M. S., Sanches, G. M., Bordonal, R. d. O., Barbosa, l. C., et al. (2018). Soil Physical Quality Response to Sugarcane Straw Removal in Brazil: a Multi-Approach Assessment. Soil Tillage Res. 184, 301–309. doi:10.1016/j.still.2018.08.007
Cavalieri, K. M. V., da Silva, a. P., Tormena, C. A., Leão, T. P., Dexter, A. R., and Håkansson, I. (2009). Long-term Effects of No-Tillage on Dynamic Soil Physical Properties in a Rhodic Ferrasol in Paraná, Brazil. Soil Tillage Res. 103 (1), 158–164. doi:10.1016/j.still.2008.10.014
Cavalieri-Polizeli, K. M. V., Calábria, Z. K. P., Keller, T., Domincheski, R. L., Kruchelski, S., Campos, B. M., et al. (2021). “Pesticide-free Farming Systems: Effects on Soil Quality and Yield,” in Proccedings in Eurosoil 2021.
Çelik, I., Günal, H., Acar, M., Acir, N., Bereket-Barut, Z., and Budak, M. (2020). Evaluating the Long‐term Effects of Tillage Systems on Soil Structural Quality Using Visual Assessment and Classical Methods. Soil Use Manag. 36 (2), 223–239. doi:10.1111/sum.12554
Chen, J., Wu, Z., Zhao, T., Yang, H., Long, Q., and He, Y. (2021). Rotation Crop Root Performance and its Effect on Soil Hydraulic Properties in a Clayey Utisol. Soil Tillage Res. 213, 105136. doi:10.1016/j.still.2021.105136
Cherubin, M. R., Chavarro-Bermeo, J. P., and Silva-Olaya, A. M. (2019). Agroforestry Systems Improve Soil Physical Quality in Northwestern Colombian Amazon. Agroforest Syst. 93, 1741–1753. doi:10.1007/s10457-018-0282-y
Cherubin, M. R., Franco, A. L. C., Guimarães, R. M. L., tormena, C. A., Cerri, C. E. P., karlen, d. L., et al. (2017). Assessing Soil Structural Quality under Brazilian Sugarcane Expansion Areas Using Visual Evaluation of Soil Structure (VESS). Soil Tillage Res. 173, 64–74. doi:10.1016/j.still.2016.05.004
Coblinski, J. A., Favaretto, N., Goularte, G. D., Dieckow, J., Moraes, A. d., and souza, L. C. d. P. (2019). Water, Soil and Nutrients Losses by Runoff at Hillslope Scale in Agricultural and Pasture Production in Southern Brazil. Jas 11 (6), 160. doi:10.5539/jas.v11n6p160
Colombi, T., Torres, L. C., Walter, A., and Keller, T. (2018). Feedbacks between Soil Penetration Resistance, Root Architecture and Water Uptake Limit Water Accessibility and Crop Growth - A Vicious Circle. Sci. Total Environ. 626, 1026–1035. doi:10.1016/j.scitotenv.2018.01.129
de Moraes, A., Carvalho, P. C. d. F., Anghinoni, I., Lustosa, S. B. C., Costa, S. E. V. G. d. A., and Kunrath, T. R. (2014). Integrated Crop-Livestock Systems in the Brazilian Subtropics. Eur. J. Agron. 57, 4–9. doi:10.1016/j.eja.2013.10.004
Demétrio, W., Cavalieri-Polizeli, K. M. V., Guimarães, R. M. L., Ferreira, S. A., Parron, L. M., and Brown, G. G. (2022). Macrofauna Communities and Their Relationship with Soil Structural Quality in Different Land Use Systems. Soil Res., SR21157. doi:10.1071/SR21157
Derpsch, R., Friedrich, T., Kassam, A., and Li, H. W. (2010). Current Status of Adoption of No-Till Farming in the World and Some of its Main Benefits. Int. J. Agric. Biol. Eng. 3, 1. doi:10.3965/j.issn.1934-6344.2010.01.001-025
Derpsch, R. 2021. No-Tillage, Sustainable Agriculture in the New Millennium. Available at: http://www.roCF-derpsch.com/en/no-till/(Acess november 22 2021).
Dominschek, R., Barroso, A. A. M., Lang, C. R., de Moraes, A., Sulc, R. M., and Schuster, M. Z. (2021). Crop Rotations with Temporary Grassland Shifts Weed Patterns and Allows Herbicide-free Management without Crop Yield Loss. J. Clean. Prod. 306, 127140. doi:10.1016/j.jclepro.2021.127140
Dominschek, R., Kruchelski, S., Deiss, L., Portugal, R., Denardin, L. G., Martins, A., et al. (2018). Sistemas integrados de produção agropecuária na promoção da intensificação sustentável. Available at: www.aliancasipa.org/wp-content/uploads/2018/12/Boletim-NITA.pdf.
Duiker, S. W., Myers, J. C., and Blasure, L. C. (2017). “Soil Health in Field and Forage Crop Production,” in Department of Agriculture, Natural Resources Conservation Service. Penn State Cooperative Extension (Washington, D.C., United States. Available at: https://extension.psu.edu/soil-health-in-field-and-forage-crop-production.
Ehlers, W., Kopke, U., Hesse, F., and Bohm, W. (1983). Penetration Resistance and Root Growth of Oats in Tilled and Untilled Loess Soil. Soil Tillage Res. 3, 261–275. doi:10.1016/0167-1987(83)90027-2
EMBRAPA (2018). Sistema brasileiro de classificação de solos. Brasília: Embrapa Solos. Available at: www.embrapa.br/busca-de-publicacoes/-/publicacao/1107206/sistema-brasileiro-de-classificacao-de-solos.
FEBRAPDP (2021). O Que É Sistema Plantio Direto? Available at: febrapdp.org.br/sistema-plantio-direto-o-que-e. (Acess novembro 22, 2021).
Flávio Neto, J., Severiano, E. D. C., Costa, K. A. D. P., Junnyor, W. S. G., Gonçalves, W. G., and Andrade, R. (2015). Biological Soil Loosening by Grasses from Genus Brachiaria in Crop-Livestock Integration. Acta Sci. Agron. 37 (3), 375–383. doi:10.4025/actasciagron.v37i3.19392
Franco, H. H. S., Guimarães, R. M. L., Tormena, C. A., Cherubin, M. R., and favilla, H. S. (2019). Global Applications of the Visual Evaluation of Soil Structure Method: A Systematic Review and Meta-Analysis. Soil Tillage Res. 190, 61–69. doi:10.1016/j.still.2019.01.002
Gamboa, C. H., Vezzani, F. M., Kaschuk, G., Favaretto, N., Cobos, J. Y. G., and Da Costa, G. A. (2020). Soil-root Dynamics in Maize-Beans-Eggplant Intercropping System under Organic Management in a Subtropical Region. J. Soil Sci. Plant Nutr. 20 (3), 1480–1490. doi:10.1007/s42729-020-00227-9
Gee, G. W., and Or, D. (2002). “Bulk Density and Linear Extensibility,” in Methods of Soil Analysis. Editors J. H. Dane, and G. C. Topp (Madison: Soil Science Society of America), 201–227.
Guimarães, R. M. L., Ball, B. C., and Tormena, C. A. (2011). Improvements in the Visual Evaluation of Soil Structure. Soil Use Manag. 27 (3), 395–403. doi:10.1111/j.1475-2743.2011.00354.x
Guimarães, R. M. L., Lamandé, M., Munkholm, L. J., Ball, B. C., and Keller, T. (2017). Opportunities and Future Directions for Visual Soil Evaluation Methods in Soil Structure Research. Soil Tillage Res. 173, 104–113. doi:10.1016/j.still.2017.01.016
Hao, H.-x., Wei, Y.-j., Cao, D.-n., Guo, Z.-l., and Shi, Z.-h. (2020). Vegetation Restoration and Fine Roots Promote Soil Infiltrability in Heavy-Textured Soils. Soil Tillage Res. 198, 104542. doi:10.1016/j.still.2019.104542
Kassam, A., Derpsh, R., Derpsch, R., and Friedrich, T. (2020). Development of Conservation Agriculture Systems Globally. Adv. Conservation Agric. 1, 31–86. doi:10.19103/AS.2019.0048.02
Keesstra, S. D., Bouma, J., Wallinga, J., Tittonell, P., Smith, P., Cerdà, A., et al. (2016). The Significance of Soils and Soil Science towards Realization of the United Nations Sustainable Development Goals. Soil 2, 111–128. doi:10.5194/soil-2-111-2016
Kruchelski, S., Trautenmüller, J. W., Deiss, L., Trevisan, R., Cubbage, F., Porfírio-da-Silva, V., et al. (2021). Eucalyptus benthamii Maiden et Cambage growth and wood density in integrated crop-livestock systems. Agroforest Syst. 95, 1577–1588. doi:10.1007/s10457-021-00672-0
Madani, E. M., Jansson, P. E., and Babelon, I. (2018). Differences in Water Balance between Grassland and Forest Watersheds Using Long-Term Data, Derived Using the CoupModel. Hydrol. Res. 49, 72–89. doi:10.2166/nh.2017.154
Meena, R., Kumar, S., Datta, R., Lal, R., Vijayakumar, V., Brtnicky, M., et al. (2020). Impact of Agrochemicals on Soil Microbiota and Management: A Review. Land 9, 34. doi:10.3390/land9020034
Moraes, M. T. d., and Gusmão, A. G. (2021). How Do Water, Compaction and Heat Stresses Affect Soybean Root Elongation? A Review. Rhizosphere 19, 100403. doi:10.1016/j.rhisph.2021.100403
Morais, M. C., Siqueira-Neto, M., Guerra, H. P., Satiro, L. S., Soltangheisi, A., Cerri, C. E. P., et al. (2020). Trade-Offs between Sugarcane Straw Removal and Soil Organic Matter in Brazil. Sustainability 12 (22), 9363. doi:10.3390/su12229363
Mutuku, E. A., Vanlauwe, B., Roobroeck, D., Boeckx, P., and Cornelis, W. M. (2021). Visual Soil Examination and Evaluation in the Sub-humid and Semi-arid Regions of Kenya. Soil Tillage Res. 213, 105135. doi:10.1016/j.still.2021.105135
Paiva, I. A. d., Rita, Y. L., and Cavalieri-Polizeli, K. M. (2020). Knowledge and Use of Visual Soil Structure Assessment Methods in Brazil - A Survey. Soil Tillage Res. 204, 104704. doi:10.1016/j.still.2020.104704
Pauletti, V., and Motta, A. C. V. (2019). Manual de Adubação e Calagem para o Estado do Paraná. 2.ed. Curitiba: NEPAR-SBSC.
Polanía-Hincapié, K. L., Olaya-Montes, A., Cherubin, M. R., Herrera-Valencia, W., Ortiz-Morea, F. A., and Silva-Olaya, A. M. (2021). Soil Physical Quality Responses to Silvopastoral Implementation in Colombian Amazon. Geoderma 386, 114900. doi:10.1016/j.geoderma.2020.114900
Popova, L., Van Dusschoten, D., nagel, K. A., Fiorani, F., and Mazzolai, B. (2016). Plant Root Tortuosity: an Indicator of Root Path Formation in Soil with Different Composition and Density. Ann. Bot. 118 (4), 685–698. doi:10.1093/aob/mcw057
Pulido Moncada, M., Gabriels, D., Lobo, D., Rey, J. C., and Cornelis, W. M. (2014). Visual Field Assessment of Soil Structural Quality in Tropical Soils. Soil Tillage Res. 139, 8–18. doi:10.1016/j.still.2014.01.002
Quaggio, J. A., and van Raij, B. (1979). Comparação de métodos rápidos para a determinação da matéria orgânica em solos. R. Bras. Ci. Solo 3, 184–187.
Rahman, S. A., Jacobsen, J. B., Healey, J. R., Roshetko, J. M., and Sunderland, T. (2017). Finding Alternatives to Swidden Agriculture: Does Agroforestry Improve Livelihood Options and Reduce Pressure on Existing Forest? Agroforest Syst. 91 (1), 185–199. doi:10.1007/s10457-016-9912-4
Ratuchne, L. C., Koehler, H. S., Koehler, H. S., Sanquetta, C. R., and Schamne, P. A. (2017). Comparison of Sampling Methods for the Evaluation of the Root System of Sugarcane. Rev. Ciencias Agrícolas 34 (1), 7–16. doi:10.22267/rcia.173401.59
Ray, S. K., Chatterjee, D., Rajkhowa, D. J., Baishya, S. K., Hazarika, S., and Paul, S. (2020). Effects of Integrated Farming System and Rainwater Harvesting on Livelihood Improvement in North-Eastern Region of India Compared to Traditional Shifting Cultivation: Evidence from an Action Research. Agroforest Syst. 94, 451–464. doi:10.1007/s10457-019-00406-3
Rosseti, K. V., and Centurion, J. F. (2017). Least Limiting Water Range in Oxisols under Different Levels of Machine Traffic. Comun. Sci. 8 (2), 337–346. doi:10.14295/cs.v8i2.2423
Salton, J. C., Mercante, F. M., Tomazi, M., Zanatta, J. A., Concenço, G., Silva, W. M., et al. (2014). Integrated Crop-Livestock System in Tropical Brazil: Toward a Sustainable Production System. Agric. Ecosyst. Environ. 190, 70–79. doi:10.1016/j.agee.2013.09.023
Schut, A. G. T., Cooledge, E. C., Moraine, M., van de Ven, G. W. J., Jones, D. L., and Chadwick, D. R. (2021). Reintegration of Crop-Livestock Systems in Europe: An Overview. Front. Agr. Sci. Eng. 8 (1), 111–129. doi:10.15302/j-fase-2020373
Sharma, P. K., Dwivedi, S., Arora, R. K., Bhagat, V., Kour, C., and Sharma, M. (2019). Efficiency under Integrated Farming Systems – A Review. Agro Econ. - Int. J. 6 (2), 47–52. doi:10.30954/2394-8159.02.2019.1
Shi, X., Qin, T., Yan, D., Tian, F., and Wang, H. (2021). A Meta-Analysis on Effects of Root Development on Soil Hydraulic Properties. Geoderma 403, 115363. doi:10.1016/j.geoderma.2021.115363
SIDRA/IBGE (2021). Instituto Brasileiro de Geografia e Estatística. Censo Agropecuário 2017 result. Defin. - Rio J. 8, 1–105. Available at: sidra.ibge.gov.br/Tabela/6856#resultado (Access November 22th, 2021).
Soni, R. P., Katoch, M., Katoch, M., and Ladohia, R. (2014). Integrated Farming Systems - A Review. Iosrjavs 7 (10), 36–42. doi:10.9790/2380-071013642
Taylor, H. M., Roberson, G. M., and Parker, J. J. (1966). Soil Strength-Root Penetration Relations for Medium- to Coarse-Textured Soil Materials. Soil Sci. 102, 18–22. doi:10.1097/00010694-196607000-00002
Tormena, C. A., Karlen, D. L., Logsdon, S., and Cherubin, M. R. (2016). Visual Soil Structure Effects of Tillage and Corn Stover Harvest in Iowa. Soil Sci. Soc. Am. J. 80 (3), 720–726. doi:10.2136/sssaj2015.12.0425
Tuchtenhagen, I. K., Lima, C. L. R. d., Bamberg, A. L., Guimarães, R. M. L., and Mansonia, P.-M. (2018). Visual Evaluation of the Soil Structure under Different Management Systems in Lowlands in Southern Brazil. Rev. Bras. Ciênc. Solo 42, e0170270. doi:10.1590/18069657rbcs20170270
Valani, G. P., Martíni, A. F., Silva, L. F. S., Bovi, R. C., and Cooper, M. (2020). Soil Quality Assessments in Integrated Crop-Livestock-Forest Systems: A Review. Soil Use Manage 37, 22–36. doi:10.1111/sum.12667
Vanhees, D. J., Loades, K. W., Bengough, A. G., Mooney, S. J., and Lynch, J. P. (2021). The Ability of Maize Roots to Grow through Compacted Soil Is Not Dependent on the Amount of Roots Formed. Field Crops Res. 264, 108013. doi:10.1016/j.fcr.2020.108013
Vizioli, B., Cavalieri-Polizeli, K. M. V., Tormena, C. A., and Barth, G. (2021). Effects of Long-Term Tillage Systems on Soil Physical Quality and Crop Yield in a Brazilian Ferralsol. Soil Tillage Res. 209, 104935. doi:10.1016/j.still.2021.104935
Keywords: soil conservation, root growth, soil structural quality, single farming systems, integrated farming systems
Citation: Cavalieri-Polizeli KMV, Marcolino FC, Tormena CA, Keller T and Moraes Ad (2022) Soil Structural Quality and Relationships With Root Properties in Single and Integrated Farming Systems. Front. Environ. Sci. 10:901302. doi: 10.3389/fenvs.2022.901302
Received: 21 March 2022; Accepted: 23 May 2022;
Published: 04 July 2022.
Edited by:
Miriam Muñoz-Rojas, University of New South Wales, AustraliaReviewed by:
Jagdish Chander Dagar, Indian Council of Agricultural Research (ICAR), IndiaMaria Martínez-Mena, Spanish National Research Council (CSIC), Spain
Copyright © 2022 Cavalieri-Polizeli, Marcolino, Tormena, Keller and Moraes. This is an open-access article distributed under the terms of the Creative Commons Attribution License (CC BY). The use, distribution or reproduction in other forums is permitted, provided the original author(s) and the copyright owner(s) are credited and that the original publication in this journal is cited, in accordance with accepted academic practice. No use, distribution or reproduction is permitted which does not comply with these terms.
*Correspondence: Karina Maria Vieira Cavalieri-Polizeli, a2FyaW5hLmNhdmFsaWVyaUB1ZnByLmJy