- 1Henan Province Engineering Research Center of Environmental Laser Remote Sensing Technology and Application, Nanyang Normal University, Nanyang, China
- 2Collaborative Innovation Center of Water Security for Water Source Region of Mid-line of South-to-North Diversion Project of Henan Province, Nanyang Normal University, Nanyang, China
- 3Non-Major Foreign Language Teaching Department, Nanyang Normal University, Nanyang, China
Smelter-impacted soils often result in soil degradation and the destruction of the soil structure. Although soil aggregate typically plays a crucial role in soil structure, the influence of phytoremediation on soil aggregate structure stability and stoichiometric characteristics remains unclear. To study the influence of phytoremediation on soil aggregate structure, stability and stoichiometric characteristics, a 3-year in situ experiment was conducted. After hydroxyapatite was applied, Elsholtzia splendens, Sedum plumbizincicola, and Pennisetum sp. were planted in a smelter-impacted soil. After 3 years, the soil aggregate structure, stability, and stoichiometric of chemical elements were analyzed. The results showed that the three phytoremediation treatments increased the content of >0.25 mm mechanically-stable (DR0.25) and water-stable (WR0.25) aggregates by 6.6%–10.4% and 13.3%–17.5%, respectively. Aggregate mean weight diameter (MWD), geometric mean diameter, and aggregate stability rate (AR, %) were significantly increased, and the soil mechanically stable aggregate fractal dimension (D) was significantly reduced after the 3-year remediation. Soil total nitrogen and phosphorus in aggregates with different particle sizes were significantly increased by 11.4%–46.4% and 107%–236% after different plant treatments. For the stoichiometric characteristics of the aggregates, the combined remediation only significantly reduced the value of N:P and C:P in different particle size aggregates and had no significant effect on the C:N in all particle size aggregates. Meanwhile, the combined remediation of hydroxyapatite and Elsholtzia splendens, Sedum plumbizincicola, and Pennisetum sp. in heavy metal heavily contaminated soil could reduce the availability of Cu and Cd by 54.1%–72.3% and 20.3%–47.2% during the 3 years, respectively. In summary, this combined remediation method can be used for the remediation of farmland that is contaminated by heavy metals.
1 Introduction
Metal pollutants in the environment mainly come from anthropogenic industrial and agricultural activities, and they can enter soil system in many different ways, such as atmospheric sedimentation, waste water irrigation, and slag leaching. Excessive accumulation of metals in the soil not only reduces soil quality, microbial activity, and crop productivity but also threatens ecological security and human health (Taghipour et al., 2011; Dankoub et al., 2012; Azizi et al., 2022). During the past few decades, more cost-effective and environmentally friendly techniques (e.g., bioremediation and integrated remediation) have been developed to reduce the mobility and bioavailability of metals in agricultural soils (Rzab et al., 2021). In situ remediation technology using combined plant and chemical additives has been proven to be one of the cheapest and most effective methods for remediating soils that are contaminated with metals (Liu et al., 2020; Li et al., 2021).
The selection of plants is very important in the process of phytoremediation. Elsholtzia splendens is widely distributed in the middle and lower reaches of the Yangtze River in China and in copper mine areas such as Zhuji City, Zhejiang Province (Yang et al., 2015). In recent years, Elsholtzia splendens has been shown to have high tolerance and strong accumulation ability to Cu, and can be applied to remediate Cu contaminated soil (Zhang et al., 2019). Sedum plumbizincicola has a high tolerance to Cd and does not show toxic symptoms when the content of Cd in the aboveground is as high as 587 mg/kg (Wu et al., 2012a). Therefore, it has been widely used in the remediation of Cd contaminated farmland (Zou et al., 2021). Pennisetum sp. has the characteristics of fast growth, strong stress resistance, and large biomass and has the potential in the remediation of heavy metal-contaminated soil (Lei et al., 2019). However, the low soil pH and high metal toxicity may impede the growth of plants, which reduces the remediation effect (Pardo et al., 2011). Evidence has been found that some chemical materials such as biosorbents or limestone can adsorb and stabilize soil Cu and Cd (Huang et al., 2015; Chen et al., 2020; Pennanen et al., 2020). A large number of studies have shown that phosphorus containing materials (e.g., apatite, potassium dihydrogen phosphate, superphosphate, and hydroxyapatite) can effectively reduce the activities of metals, such as Pb, Cd, and Cu in soil and wastewater (Valipour et al., 2016). Among them, hydroxyapatite has been widely used to remediate metal-contaminated soil and sediments owing to its strong adsorption and fixation ability for metals (Guo et al., 2018; Xu et al., 2019). However, most of the studies about hydroxyapatite only focus on its effect on metal activity and bioavailability, and only a few studies have paid attention to its potential effect on the soil aggregate structure and the influence of on soil stoichiometric characteristics.
The purpose of phytoremediation is not only to remove metals from soil but also to improve soil quality including soil physical structure, chemical properties, and biological properties (Qin et al., 2021). Soil aggregate is an important indicator of soil structure. At the same time, aggregate stability plays an important role in maintaining soil structure. Moreover, soil aggregate is the storage and transformation place of soil nutrients, such as carbon, nitrogen, and phosphorus. In addition, soil microorganisms accumulated in aggregate play an important role in regulating plant growth, reducing hydraulic erosion, and improving soil fertility (Ayoubi et al., 2012; Ayoubi et al., 2020). Different particle size aggregates have different abilities to fix and transform nutrients , which finally affects the geochemical cycle of soil nutrients (Gelaw et al., 2013). Meanwhile, different plants have different effects on soil aggregates. However, in the process of phytoremediation, there are few reports on the impact of phytoremediation model on soil aggregates.
Soil stoichiometric characteristics are the theoretical basis for analyzing the balance relationship between multiple elements, especially structural elements (C), restrictive elements (N and P), and the interaction of ecosystem. At the same time, stoichiometric characteristics are important indicators in plants, litter, soil and microorganism carbon and nitrogen nutrient cycling, nutrient limitation, carbon fixation and farmland vegetation restoration (An, 2012; Quan et al., 2015; Jia et al., 2018). Although there are many studies on the formation mechanism, stability, and nutrient retention of aggregate under different vegetation conditions, there are relatively few studies on the eco-stoichiometric characteristics of carbon, nitrogen, and phosphorus in soil aggregate in the process of phytoremediation (Erktan et al., 2016).
The objectives of this study were to (1) evaluate the changes of soil aggregate composition and structure after combined remediation, (2) analyze soil aggregate stability after combined remediation, (3) measure the stoichiometric characteristics of soil aggregate, and (4) explore the relationship between the combined remediation and the stoichiometric characteristics of nutrients in soil aggregate.
2 Materials and methods
2.1 Study site
The study site is located in Chenjia village group, Baili village, Binjiang Township, Guixi City, Jiangxi Province, China. This area is close to a large Cu smelter in Guixi City. Due to long-term diversion irrigation discharged by the local Cu smelter and atmospheric sedimentation, the farmland in this area is seriously contaminated by metals. The main pollutants are Cu and Cd (Zhou et al., 2015). Because of the heavy degree of contamination, most of the farmland in this area has been abandoned for many years. In addition, this area is located in a typical acid rain area in south China, the pH of precipitation rainwater is 3.62~4.98. Moreover, the annual deposition fluxes of Cu, Cd, Pb, Zn, and Cr in this area are 638, 6.56, 70.0, 225, and 22.7 mg m−2·a−1, respectively, which exacerbates the degree of soil contamination (Tao et al., 2014). The soil in the experimental area is sandy loam. Soil Cu and Cd concentrations are 632 and 0.41 mg kg−1, respectively. Soil pH is 4.35 (acidic), and soil organic carbon (SOC) and cation exchange capacity (CEC) are 28.5 and 8.31 cmol kg−1, respectively. Total N and total P in the soil are 1.11 and 0.19 g kg−1, respectively.
2.2 Hydroxyapatite and plant species
Hydroxyapatite (purity >96.0%, pH 7.71, particle size 3 μm, specific surface area 45.7 m2 g−1, Ca:P 1.72, Cd and Cu concentrations 3.83 × 10–2 and 5.85 mg kg−1) was purchased from Emperor Nano Material Co. Ltd. (Nanjing, China). Three phytoextractors were selected: a Cu-tolerant plant (Elsholtzia splendens) (Wang et al., 2005), high biomass plant (Pennisetum sp.) (Xu et al., 2014), and a Cd hyperaccumulator (Sedum plumbizincicola) (Wu et al., 2012b). All of the plants that were used in the experiment were grown from seed in pots in a greenhouse.
2.3 Plot design
There were four treatments in the field experiment (CK: untreated soil; ME: hydroxyapatite + Elsholtzia splendens; MS: hydroxyapatite + Sedum plumbizincicola; MP: hydroxyapatite + Pennisetum sp.), each treatment had three repetitions, a total of 12 plots, and the plot area was 4 m2 (2 m × 2 m). To prevent interaction between adjacent plots, the plots were separated by cement plates. The buried depth of cement plates was 0.3 m underground and 0.2 m above the ground. Meanwhile, 1% hydroxyapatite (based on the 0- to 17-cm soil weight) and 834 kg ha−1 fertilizer (the content of N, 15%; P2O5, 15%; and K2O, 15%) were applied into every plot on 23 December 2012, and the hydroxyapatite and fertilizer fully mixed into the soil by plowing. Elsholtzia splendens and Sedum plumbizincicola (planting spacing 0.2 m × 0.2 m), Pennisetum sp. (planting spacing 0.5 m × 0.5 m) were planted on 26 April each year (2013, 2014, and 2015), while the hydroxyapatite was applied once in 2012 but fertilizer was added into the soil each year for the next 3 years.
2.4 Sample collection
Elsholtzia splendens and Pennisetum sp. were harvested in the middle of December each year, Sedum plumbizincicola was harvested in early August each year. Soil samples were collected from each plot from an area of 20 cm × 20 cm × 17 cm in 2015, three samples were taken from each plot and then mixed together to form a mixed sample. These samples were air dried and sieved using a 5 mm sieve. The resulting samples were used for the analysis of soil aggregates. Surface soil (0–17 cm) was collected every year and passed through a 10-mesh sieve to determine the basic properties, and to assess the Cu and Cd concentrations.
2.5 Soil and plant chemical and metal analysis
Soil pH was measured by a pH electrode in a suspension of distilled water at a liquid to solid ratio of 2.5 (E-201-C, Shanghai Truelab Instrument Company, China) (Xu et al., 2016). SOC was measured by digesting soil with K2Cr2O7 and concentrated H2SO4 at 170°C–180°C, and then titrating with FeSO4 (Mikhailova et al., 2003). Total soil N was determined by the semimicro Kjeldahl method on a sample predigested with perchloric acid (HClO4) and hydrofluoric acid (HF) (Zhang and Sheng-Xiu, 2005). Total soil P was determined by acidic molybdate-ascorbic acid blue color method after the soil digestion with nitric acid/perchloric acid mixture (4:1) (Olson et al., 1982). Total Cu and Cd were measured by a flame or graphite furnace atomic absorption spectrophotometer (Hitachi Model Z-2000, Japan) after digestion with mixed nitric acid (HNO3), hydrofluoric acid (HF), and perchloric acid (HClO4) (5:10:5) on a hot plate (120°C–240°C). Available Cu and Cd were measured by extracting soil samples with 0.01 M CaCl2 at a 1:5 ratio and then shaking for 2 h at room temperature (25°C) (Walker et al., 2003). The suspension was centrifuged for 10 min, decanted and filtered through a 0.45-μm filter, acidified (with concentrated HNO3), and stored in a polyethylene container at 4°C until analysis.
Plant samples (0.25 g) were digested using a mixture of 4 ml HClO4 and 6 ml HNO3 on an electric heating plate. The metal contents were measured by atomic absorption spectroscopy.
Blanks, replicate samples, and a certified reference material (GBW07401, provided by the Institute of Geophysical and Geochemical Exploration, Langfang, Hebei Province, China) were used to ensure the reliability of the experimental data.
2.6 Aggregate analysis
Mechanically stable aggregates: The mechanically stable aggregate was measured by the dry sieve method (Yan et al., 2020), the aggregate was divided into six size fractions: >5 mm, 2–5 mm, 1–2 mm, 0.5–1 mm, 0.25–0.5 mm, and <0.25 mm.
Water-stable aggregates: The water-stable aggregate was measured by Wang’s method (Wang et al., 2020)— 25 g soil samples of each particle size according to the proportion of dry screening method was placed on a 5-mm soil sieve, the soil samples were soaked in distilled water for 10 min, and then passed the soil samples through 2, 1, 0.5, 0.25, and 0.053 mm soil sieves in turn. The aggregate was separated by moving the sieve 3 cm up and down, repeating 50 times (within 2 min), washing the soil particles that were left on each sieve into the aluminum box, drying at 50°C, and weighing. Water-stable aggregates were divided into six size fractions: >5 mm, 2–5 mm, 1–2 mm, 0.5–1 mm, 0.25–0.5 mm, and <0.25 mm. The percentage of >0.25 mm mechanically stable aggregates (DR0.25) and >0.25 mm water-stable aggregates (WR0.25) were calculated based on the results. DR0. 25 was >0.25 mm mechanically stable aggregate percentage (%), and WR0.25 was >0.25 mm water-stable aggregate percentage (%). The mean weight diameter (MWD, mm), geometric mean diameter (GMD, mm), and fractal dimension (D) of aggregates were calculated by Zhao’s method (Zhao et al., 2012):
Xi is the average soil aggregate diameter of any grade, which is numerically equal to the average value of two adjacent sieve holes, for >5 mm aggregates, the upper limit of aggregate diameter is 10 mm; Wi is the percentage of aggregates corresponding to Xi.
Xi is the average soil aggregate diameter of any grade, which is numerically equal to the average value of two adjacent sieve holes, for >5 mm aggregates, the upper limit of aggregate diameter is 10 mm; Xmax is the average particle size of the maximum particle size, mm; M (r < Xi) is the weight of the aggregate with particle size less than Xi; and M is the total weight of the aggregates.
2.7 Statistical analysis
SPSS20.0 (IBM SPSS, Somers, NY, United States) was used for one-way ANOVA and correlation analysis (Pearson index was used for size), Duncan new repolarization difference method was used for difference significance analysis, and the significance level was p < 0.05. All of the graphics were plotted by Sigmaplot 12.5.
3 Results and discussion
3.1 Soil chemical characteristics and metal availability
The pH of CK slightly decreased from 4.24 in the first year to 4.20 in the third year (Table 1). With hydroxyapatite application, the pH was significantly improved by 21.5%–25.7%, 21.3%–23.9%, and 20.7%–25.2% in 2013, 2014, and 2015, respectively. The study area was an acid rain settlement region, where H+ from the rainwater reduced soil pH over time (Tao et al., 2014). Differences in plant species did not significantly change soil pH (Table 1). Meanwhile, the roots may have secreted weak organic acid ions, sugars, amino acids, vitamins, and inorganic ions (HCO3−, OH−, and H+), all of which might alter soil pH (Bedolla-rivera et al., 2020). Untreated soil had the highest CaCl2 extractability (Cu 100 and Cd 0.15 mg kg−1 in 2015) (Table 1). Hydroxyapatite significantly decreased extractable Cu and Cd by 54.1%–73.5% and 20.1%–51.2%. The results showed that bioavailability of Cu and Cd in the CK were higher than that in hydroxyapatite treated soils, which might be mainly due to the lower pH in the CK.
3.2 Plant biomass and metal concentrations in plants
Although the three phytoextractors could grow normally after the application of hydroxyapatite, there was no plant growth in CK treatment without micron hydroxyapatite. There were significant differences in the biomass of the three plants, the largest biomass was found in Pennisetum sp. The biomass of Elsholtzia splendens was smaller than that of Pennisetum sp. but significantly higher than that of Sedum plumbizincicola (Table 2). Sedum plumbizincicola showed the highest absorption capacity for Cu and Cd in the enrichment capacity for metals (Figure 1).
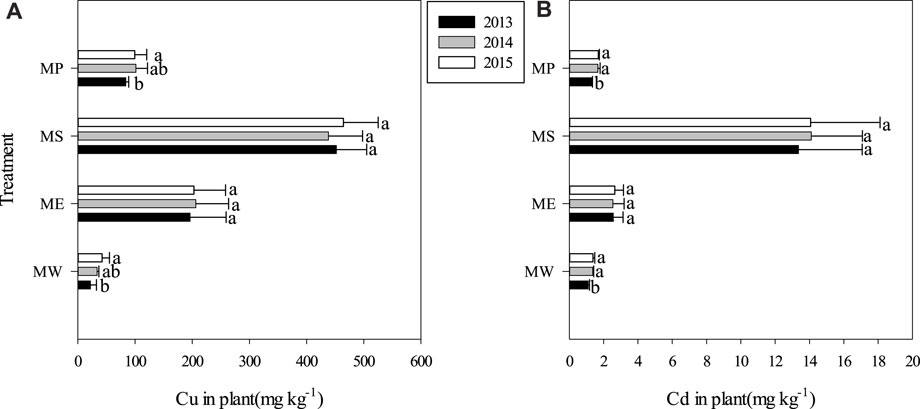
FIGURE 1. Concentrations of (A) Cu and (B) Cd in the shoots of each plant. MW = Setaria lutescens, ME = Elsholtzia splendens, MS = Sedum plumbizincicola, MP = Pennisetum sp. Different lowercase letters indicate significant differences in the same treatment during the 3 years (n = 3, p < 0.05).
As an effective and widely used remediation measure of soil heavy metal contamination, phytoremediation is considered to be an effective method to reduce the total amount of soil heavy metals over time (Ceschin et al., 2019). Thus, removal efficiency should always be used to evaluate the remediation capability during the phytoremediation, which can be calculated using tissue concentration and biomass produced (Mamirova et al., 2020). Therefore, the removal efficiency of different plants was not only related to the concentration of metals in plants but also depended on the biomass of plants. After considering biomass and Cu and Cd concentrations, Pennisetum sp. showed the greatest Cu and Cd removal efficiency, with 3-year cumulative amounts of 8.67 × 103 and 121 g hm−2 for Cu and Cd, respectively (Table 2). Although the concentrations of Cu and Cd in Elsholtzia splendens and Sedum plumbizincicola were quite different, they had similar removal efficiency of Cu and Cd after integrating the biomass. According to our study results and the living habits of different plants, we suggest that the intercropping of Sedum plumbizincicola and Pennisetum sp. or Elsholtzia splendens aided by amendments could be an efficient phytoremediation method. However, this assumption needs to be verified by further field experiments.
3.3 Changes in the soil aggregate structure
In 2015, the percentage of >0.25 mm mechanically-stable aggregates (DR0.25) in the soil was 69.4%–76.6% (Figure 2), which was lower than that in many reports in this area (84.6–90.2%) (Zheng et al., 2015a). This indicates that the soil physical structure in this area was poor, which might be related to the serious soil pollution in this area. After 3 years of phytoremediation, the soil DR0.25 was significantly increased. Moreover, the three phytoremediation modes mainly increased the percentage of >5 mm, 2–5 mm and 0.5–1 mm aggregates in mechanically stable aggregate, especially > 2 mm aggregates. Among the three plants, Pennisetum sp. had the greatest improvement on soil macroaggregates, especially for aggregates >5 mm and 2–5 mm, which were significantly increased by 29.0% and 10.1% compared with CK treatment, respectively. However, there was no significant difference among the three plant treatments in the impact on aggregates of 1–2 mm, 0.5–1 mm, and 0.25–0.5 mm, which indicates that Pennisetum sp. had the greatest improvement on macroaggregates in the process of phytoremediation during the past 3 years and it could best improve the physical structure of soil. This might be due to the large biomass of Pennisetum sp., the higher vegetation coverage could return more litter, the input of more exogenous organic matter increased the content of organic matter in the soil, and the small aggregates were bonded and aggregated into larger aggregates through organic matter cement (Zeng et al., 2018).
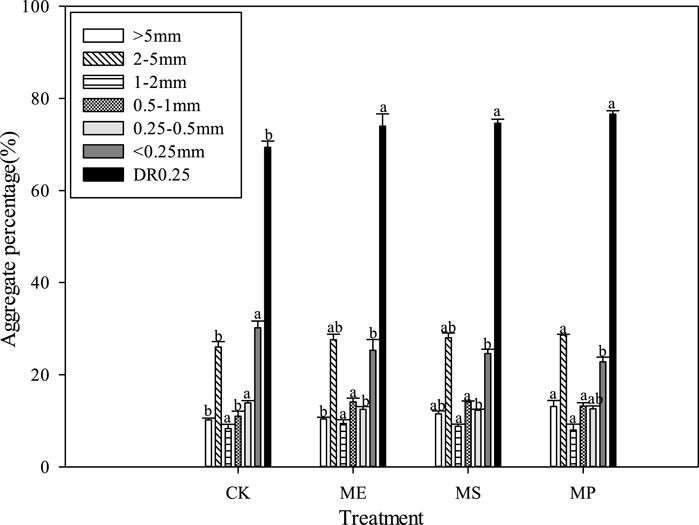
FIGURE 2. Effect of phytoremediation on mechanically stable aggregate composition of metal-contaminated soil. [CK: untreated soil; ME: hydroxyapatite + Elsholtzia splendens; MS: hydroxyapatite + Sedum plumbizincicola; MP: hydroxyapatite + Pennisetum sp; DR0.25: the percentage of >0.25 mm mechanically stable aggregates. Different lowercase letters indicate significant differences between treatments in the same year (n = 3, p < 0.05)].
In terms of the composition of soil water-stable aggregate, the percentage of >0.25 mm water-stable aggregate (WR0.25) in each treatment was 44.7%–52.6% (Figure 3). After 3 years of phytoremediation, the percentage of soil water-stable aggregate could be significantly increased. However, similar to the mechanically-stable aggregate, there was no significant difference among the three plant treatments. By analyzing the particle size composition of water-stable aggregate, it was found that the increased part was mainly concentrated in the > 5-mm aggregate. Meanwhile, the increasing range in the other particle size compositions was not significant. Even the 0.5 to 1-mm aggregate in Elsholtzia splendens and Sedum plumbizincicola treatments had a significant downward trend which was the same as the research results of Zheng et al. (2015a) who found that vegetation restoration could mainly increase the percentage of the > 5-mm aggregate but had little effect on the percentage of other particle size aggregates.
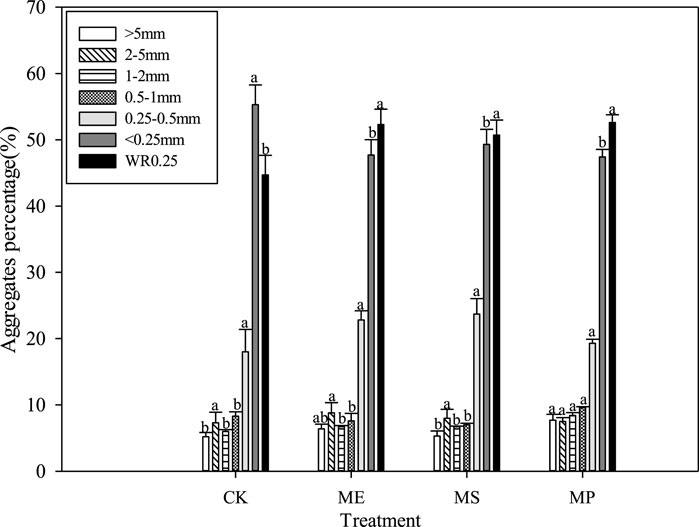
FIGURE 3. Effect of phytoremediation on water stability aggregates composition of metal-contaminated soil. [CK: untreated soil; ME: hydroxyapatite + Elsholtzia splendens; MS: hydroxyapatite + Sedum plumbizincicola; MP: hydroxyapatite + Pennisetum sp. Different lowercase letters indicate significant differences between treatments in the same year (n = 3, p < 0.05).].
The correlation between mechanical stability aggregates (DR0.25), water stability aggregates (WR0.25), and soil organic matter showed that DR0.25 and WR0.25 were significantly positively correlated with soil organic matter content, and the correlation coefficients R2 were 0.829** and 0.741** (p < 0.01), respectively. To obtain the relationship between soil organic matter and >0.25 mm mechanically stable aggregate and water-stable aggregate, we further fitted them linearly with the content of soil organic matter (Figure 4). The result shows that there was a linear relationship between DR0.25, WR0.25, and soil organic matter, which is consistent with the research results of Deng (Lei et al., 2018). Through the results of this correlation analysis, we infer that the main reason for the increase of the percentage of >0.25 mm mechanically stable aggregate and water-stable aggregate might be the increase in soil organic matter content. Increase of organic carbon concentration promoted the cementation process of aggregate and accelerated the transformation from small aggregates to large aggregates during the phytoremediation. This change played an important role in improving the soil structure and promoting the quality recovery of the metal-contaminated soil.
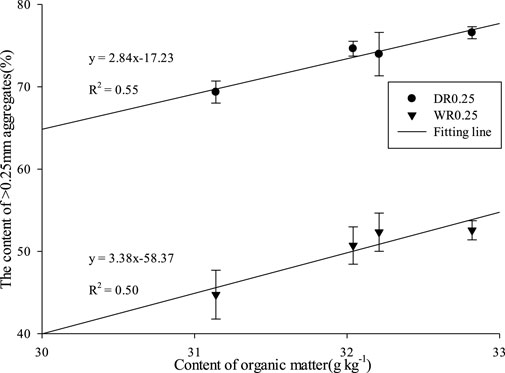
FIGURE 4. Fitting of >0.25 mm aggregates percentage (DR0.25, WR0.25) and organic matter content. DR0.25: the percentage of >0.25 mm mechanically stable aggregates, WR0.25: >0.25 mm water-stable aggregates.
3.4 Effect of phytoremediation on the stability of soil aggregates
3.4.1 Aggregate size
In this study, the MWD and GMD of mechanically stable aggregate and water-stable aggregate were significantly increased after 3 years of phytoremediation (Table 3). The MWD and GMD values of the two types of aggregate were the highest in the treatment of Pennisetum sp. In terms of MWD, the treatment of Pennisetum sp. improved the mechanical stability aggregate and water stability aggregate by 16.2% and 24.2% compared with CK treatment, while in terms of GMD, the mechanical stability aggregate and water stability aggregate were increased by 29.1% and 25.0%, respectively. This indicates that the phytoremediation by Pennisetum sp. could improve the stability of soil aggregates, which was conducive to the improvement of soil physical structure. At the same time, it was found that the MWD and GMD of mechanically stable aggregate in each treatment were greater than those of water-stable aggregates, which indicated that mechanically stable aggregate was the main aggregate type in this soil. This result is consistent with other regional studies on the stability of soil aggregates.
Correlation analysis showed that the percentage of large aggregate was significantly correlated with the index of aggregate stability, which indicates that the stability of soil aggregate was mainly determined by the percentage of large aggregates (Table 4). The percentage of soil aggregate with each particle size was significantly correlated with the index of aggregate stability, and the positive and negative correlation was bounded by a particle size of 2 mm. This result proves that phytoremediation promoted the formation of large aggregate from fine aggregate and micro-aggregate, resulting in the strengthening of the aggregate’s stability. This was different from the findings of Jiang et al. 2016), who found that the positive and negative correlation between MWD and GMD values and aggregate percentage of each particle size was bounded by 1 mm. This might be related to the high percentage of large aggregates and strong stability of aggregates in calcareous soil. In addition, compared with aggregate with particle size <0.25 mm, > 0.25 mm aggregate was more relevant to MWD and GMD (Table 5). Moreover, the percentage of > 0.25 mm particle size aggregate was significantly higher than that of <0.25 mm particle size aggregate. This shows that in the process of transformation from micro-aggregates to large aggregates, 0.25 mm is a critical value (Florio et al., 2019).

TABLE 4. Correlation between mechanical stability and water stability aggregates percentage and MWD, GMD, AR, and D.
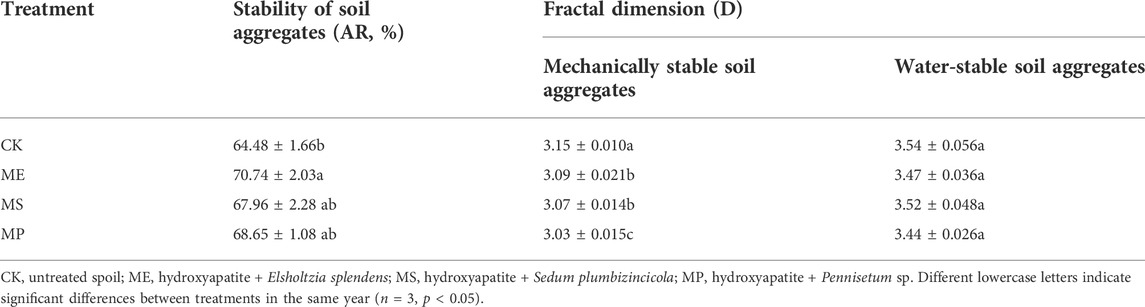
TABLE 5. Effects of phytoremediation on the stability rate and fractal dimension of soil aggregates polluted by metals.
3.4.2 Stability rate and fractal dimension of aggregates
This study found that the soil aggregate stability rates (AR) of phytoremediation treatments were significantly increased by 5.4%–9.7% compared with the CK treatment (Table 5). In terms of fractal dimension (D), 3 years of phytoremediation could significantly reduce the fractal dimension of mechanically-stable aggregate, with a reduction range of 1.9%–3.8% (Table 5). This result shows that the particle size composition of soil aggregate was more uniform and the soil physical structure had been improved after 3 years of phytoremediation.
It was found that DR0.25 and WR0.25 had a very significant negative correlation with fractal dimension (D), while WR0.25 had a significant positive correlation with aggregate stability rate (AR,%) (Table 4), which shows that WR0.25 made a greater contribution to improving the aggregate stability rate. In this study, we found that the increase of organic matter content under the condition of phytoremediation played an important role in the formation and stability of water-stable aggregate. This is also consistent with the conclusion that additional organic carbon could significantly improve the percentage, average mass diameter, and stability of soil water-stable aggregates (Dong et al., 2014). Micro-aggregates in the soil form water-stable large aggregate through the adhesion and cementation of organic matter (Rabbi et al., 2019; Hong et al.2020). Therefore, increasing the content of soil organic matter is an effective way to improve the stability of aggregates and prevent soil erosion.
A large number of studies have confirmed that with the increase of phytoremediation years, the vegetation coverage gradually increases, the aboveground biomass gradually increases, and the litter returns to the soil also increase (Catania et al., 2018; Lei et al., 2018). The decomposition of litter increases the content of soil organic matter, and the cementation of organic matter is strengthened, which enhances the agglomeration of the aggregates (Maietta et al., 2019). Vegetation restoration in the process of phytoremediation will increase the root biomass and root exudates, improve the soil structure, promote the formation and structural stability of soil aggregate, and improve the soil erosion resistance and soil erodibility decreased (Lxab et al., 2021).
3.5 Effect of phytoremediation on nutrient content of aggregates
In the process of phytoremediation, the law of organic carbon content in soil aggregates is as follows: when the particle size of soil aggregate was <1 mm, the content of organic carbon in aggregates increased with the increase of particle size; but when the particle size of aggregate was greater than 1 mm, the content of organic carbon in aggregates decreased with the increase of particle size (Figure 5). In all particle size aggregates, the organic carbon content of <0.25 mm aggregates was the lowest, which was 12.9–13.4 g kg−1; and the organic carbon content of 0.5–1 mm aggregate was the highest, which was 23.0–25.4 g kg−1. After 3 years of combined remediation with hydroxyapatite and different plants, the content of organic carbon in > 5 mm, 2–5 mm, and 1–2 mm aggregates were significantly increased by 6.3%–14.8%, 12.4%–25.5%, and 4.0%–12.2%, respectively. The pattern of the content of soil total nitrogen in soil aggregate was similar to the soil organic carbon. In all particle size aggregates, the soil total nitrogen content of <0.25 mm aggregate was the lowest, which was 0.85–1.02 g kg−1; and the soil total nitrogen content of 0.5–1 mm aggregates was the highest, which was 1.45–1.91 g kg−1. After 3 years of combined remediation with hydroxyapatite and different plants, the content of soil total nitrogen in > 5 mm, 2–5 mm aggregates were significantly increased by 16.9%–41.9% and 22.4%–46.4%, respectively. In contrast from soil organic carbon and total nitrogen, combined remediation could significantly increase the content of phosphorus in all particle size aggregates. At the same time, the change of phosphorus content in aggregates with different particle size had no obvious pattern. These results are similar to other studies, which showed that the organic carbon and total nitrogen of soil aggregates with various particle size would increase after phytoremediation. This indicating that phytoremediation made soil aggregates reduce nutrient loss and led to a stronger ability to collect organic matter (Xue et al., 2008; Nguyen et al., 2016).
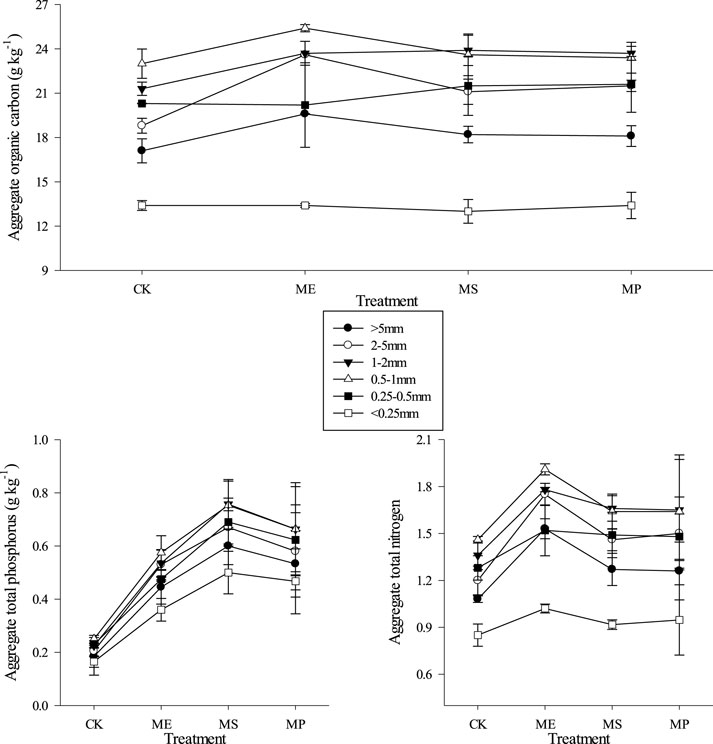
FIGURE 5. Distribution characteristics of soil organic carbon, total nitrogen, and total phosphorus concentration in soil aggregates relative to phytoremediation. (CK: untreated soil; ME: hydroxyapatite + Elsholtzia splendens; MS: hydroxyapatite + Sedum plumbizincicola; MP: hydroxyapatite + Pennisetum sp.).
Moreover, it was found that the organic carbon content of large aggregate was higher than that of the whole soil in this study, which showed that large aggregates had a certain enrichment effect on organic carbon (Xiao et al., 2017). Phytoremediation had an important impact on the distribution of organic carbon in soil aggregates. In the process of phytoremediation, the increase of organic carbon reserved in large aggregates was significantly faster than that in micro-aggregates. This shows that the newly added carbon might first appear in large aggregates, thus increasing the distribution proportion of organic carbon in large aggregates (GALE et al., 2000). The soil in this study was abandoned for a long time, the surface was bare, and the content of soil organic carbon was low. With the progress of phytoremediation, the content of soil organic carbon increased, which made the content of large aggregates increase faster, and improved the particle size and stability of aggregates (GALE et al., 2000).
In this study, it was found that the distribution of nitrogen in aggregates was similar to that of organic carbon. The results of this study were similar to the results of previous studies on the distribution of carbon and nitrogen in different forest vegetation types (Liu et al., 2019). In the process of vegetation change from grass–shrubwood–arbor forest, with the improvement of soil organic carbon content, other nutrients in the soil had also been improved, and there was a good positive correlation between soil organic carbon, total nitrogen, alkali-hydro nitrogen, and carbon nitrogen ratio (Hongkai et al., 2012).
Phosphorus in soil mainly comes from the weathering of geological rocks. The phosphorus content of southern red soil is mainly related to the soil forming process, weathering characteristics, and soil erosion related factors (Turner et al., 2007). At the same time, it is also affected by the decomposition of animal and plant residues (Hou et al., 2015). The strong weathering and leaching in low latitudes caused the low content of total phosphorus in the soil. In addition, the study area had suffered from serious soil erosion, the phosphorus had already been leached out, and the content of total phosphorus was very low. In this study, hydroxyapatite was added to the soil, hydroxyapatite could dissolve in the soil, which improves the content of phosphorus in the aggregate (Cui et al., 2017). At the same time, it was found that the decomposition of surface litter improved the accumulation of soil organic phosphorus, which improves the total storage of soil phosphorus. Therefore, the surface litter thickens and the total phosphorus content in mineralized soil aggregate increased through microbial decomposition over time (Tian et al., 2013).
3.6 Effect of phytoremediation on stoichiometric characteristics of soil aggregates
Figure 6 shows the effect of phytoremediation on stoichiometric characteristics of soil aggregate. The variation range of C:N of aggregates with each particle size was 12.9–15.9 after the phytoremediation. There was no significant difference in C:N of all different particle size aggregates during different plant treatments. Meanwhile, the change of C:N with different particle size was also not significant. The variation range of C:P and N:P of each particle size aggregates were 1.87–6.01 and 26.5–94.5, respectively. The same as the C:N, there was no significant difference in C:P and N:P among different particle sizes aggregates. However, in contrast to the C:N, the combined remediation of hydroxyapatite and phytoremediation significantly reduced the value of C:P and N:P in different particle size aggregates.
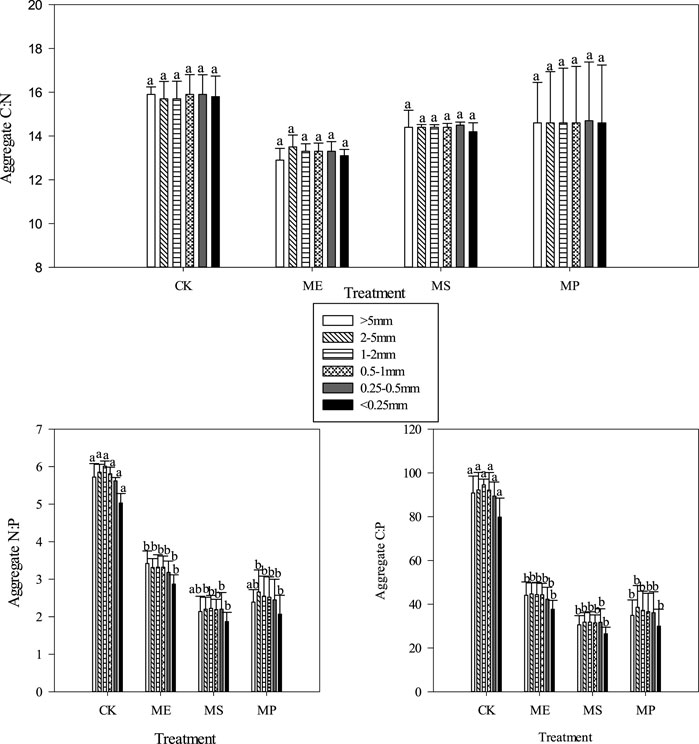
FIGURE 6. Stoichiometric characteristics of soil aggregates relative to phytoremediation [CK: untreated soil; ME: hydroxyapatite + Elsholtzia splendens; MS: hydroxyapatite + Sedum plumbizincicola; MP: hydroxyapatite + Pennisetum sp. Different lowercase letters indicate significant differences between treatments in the same year (n = 3, p < 0.05)].
Soil stoichiometry was the balance coupling mechanism between soil organic carbon, nitrogen, and phosphorus. The balance coupling between the input/output of soil carbon, nitrogen and phosphorus and its effectiveness determined the carbon cycle and carbon fixation efficiency of the ecosystem (Quanchao et al., 2016). In this study, the low value of C:N in aggregates indicated that the “sink” ability of carbon and nitrogen was poor, which was not conducive to the sequestration of carbon and nitrogen nutrients in soil aggregates. The value of C:N in different particle size aggregates increased significantly with phytoremediation. This result could improve the solid stock of soil carbon and nitrogen and increase the “sink” function of soil aggregates carbon and nitrogen, which is of great significance to improve soil fertility and even global climate change.
In this study, hydroxyapatite and phytoremediation significantly reduced the value of C:P in different particle size aggregates. This might be mainly related to the dissolution of added hydroxyapatite and the release of a large amount of phosphorus. The reduction of C:P could made microorganisms release phosphorus in mineralizing soil organic matter, which promotes the absorption and utilization of phosphorus by plants. This is of great significance because it can help to alleviate the widespread problem of phosphorus deficiency in southern China.
Hydroxyapatite and phytoremediation significantly reduced the value of N:P in different particle size aggregates. The reason for this phenomenon is similar to that of C: P. In CK treatment, the N:P level of the soil aggregates was higher than the average level of subtropical soil N:P (Tian et al., 2013). Previous studies showed that the subtropical area was generally limited by P element. In the process of phytoremediation, the value of N:P in eroded red soil aggregates remained high, and the soil aggregates were obviously limited by the P element (Fan et al., 2015). However, in this study, the addition of hydroxyapatite significantly changed this phenomenon, the value of N:P was reduced to a low level, which is of great significance to solve the problem of soil phosphorus deficiency.
4 Conclusion
Applying hydroxyapatite to metal heavily contaminated soil combined with phytoremediation for 3 years can increase the content of mechanically-stable aggregates (DR0.25) and water-stable aggregates (WR0.25) by 6.6%–10.4% and 13.3%–17.5%, respectively. After 3 years of combined restoration of vegetation and hydroxyapatite, the mechanical stability and water stability aggregates mean weight diameter (MWD) are significantly improved by 5.1%–16.2% and 5.3%–24.2%, and the geometric average diameter (GMD) is increased by 15.2%–29.1% and 9.4%–25.0%. At the same time, it can significantly reduce the fractal dimension of soil mechanical stability aggregates by 1.9%–3.8%. Soil organic carbon, total nitrogen, and total phosphorus in aggregates with different particle sizes were significantly increased by different plant treatments. However, for the stoichiometric characteristics of aggregates, the combined remediation only significantly reduces the value of N:P and C:P in different particle size aggregates, and has no significant effect on the C:N. By applying hydroxyapatite in metal heavily contaminated soil and reducing the toxicity of metals, planting Elsholtzia splendens, Sedum plumbizincicola, and Pennisetum sp. can improve soil structure, alleviate soil phosphorus deficiency, and improve soil quality. Therefore, it is suitable to be popularized in the field remediation practice of metal heavily contaminated soil.
Data availability statement
The raw data supporting the conclusions of this article will be made available by the authors, without undue reservation.
Author contributions
LX conducted all the experiments and wrote the manuscript. XX revise the language of the manuscript. JB conducted part of the experiments. DL conducted part of the experiments.
Funding
The research was funded by the Key Scientific Research Projects of Henan Province Colleges and Universities (22B180010), the Scientific and Technological Research Projects in Henan Province (222102320317 and 212102310844), and the PhD Special Project of Nanyang Normal University (2018ZX018).
Conflict of interest
The authors declare that the research was conducted in the absence of any commercial or financial relationships that could be construed as a potential conflict of interest.
Publisher’s note
All claims expressed in this article are solely those of the authors and do not necessarily represent those of their affiliated organizations, or those of the publisher, the editors, and the reviewers. Any product that may be evaluated in this article, or claim that may be made by its manufacturer, is not guaranteed or endorsed by the publisher.
References
An, S. (2012). Successional changes in soil stoichiometry after land abandonment inLoess Plateau, China [J]. Ecol. Eng. 58, 249–254. doi:10.1016/j.ecoleng.2013.06.036
Ayoubi, S., Karchegani, M., Mosaddeghi, P., and Honarjoo, N. (2012). Soil aggregation and organic carbon as affected by topography and land use change in Western Iran. Soil Tillage Res. 121, 18–26. doi:10.1016/j.still.2012.01.011
Ayoubi, S., Mirbagheri, Z., and Mohammad Reza, M. (2020). Soil organic carbon physical fractions and aggregate stability influenced by land use in humid region of northern Iran. Int. Agrophys. 34 (3), 343–353. doi:10.31545/intagr/125620
Azizi, K., Ayoubi, S., Nabiollahi, K., Garosi, Y., and Gislum, R. (2022). Predicting heavy metal contents by applying machine learning approaches and environmental covariates in west of Iran. J. Geochem. Explor. 233, 106921–106929. doi:10.1016/j.gexplo.2021.106921
Bedolla-rivera, H. I., Negrete-Rodr Guez, M. D. L. L. X., Medina-Herrera, M. D. R., Gamez-Vazquez, F. P., Alvarez-Bernal, D., Samaniego-Hernandez, M., et al. (2020). Development of a soil quality index for soils under different agricultural management conditions in the central lowlands of Mexico: Physicochemical, biological and ecophysiological indicators. Sustainability 12 (22), 9754–9778. doi:10.3390/su12229754
Catania, P., Badalucco, L., Laudicina, V. A., and Vallone, M. (2018). Effects of tilling methods on soil penetration resistance, organic carbon and water stable aggregates in a vineyard of semiarid Mediterranean environment. Environ. Earth Sci. 77 (9), 348–357. doi:10.1007/s12665-018-7520-5
Ceschin, S., Sgambato, V., Ellwood, N., and Zuccarello, V. (2019). Phytoremediation performance of Lemna communities in a constructed wetland system for wastewater treatment. Environ. Exp. Bot. 162, 67–71. doi:10.1016/j.envexpbot.2019.02.007
Chen, H., Yuan, X., Xiong, T., Jiang, L., Wang, H., and Wu, Z. (2020). Biochar facilitated hydroxyapatite/calcium silicate hydrate for remediation of heavy metals contaminated soils. Water Air Soil Pollut. 231 (2), 66–82. doi:10.1007/s11270-020-4425-1
Cui, H., Yi, Q., Yang, X., Wang, X., Wu, H., and Zhou, J. (2017). Effects of hydroxyapatite on leaching of cadmium and phosphorus and their availability under simulated acid rain. J. Environ. Chem. Eng. 5 (4), 3773–3779. doi:10.1016/j.jece.2017.07.001
Dankoub, Z., Ayoubi, S., Khademi, H., and Lu, S. G. (2012). Spatial distribution of magnetic properties and selected heavy metals in calcareous soils as affected by land use in the Isfahan region, Central Iran. Pedosphere 22 (1), 33–47. doi:10.1016/s1002-0160(11)60189-6
Dong, L., Chen, Y., and Li, X. (2014). Effects of the returning farmland to forests on content of water stable soil aggregates and the nutrients in wuqi county [J]. Sci. Silvae Sin. 50 (5), 140–146. doi:10.1016/j.catena.2019.104294
Erktan, A., Cillon, L., Graf, F., Roumet, C., Legout, C., and Rey, F. (2016). Increase in soil aggregate stability along a mediterranean successional gradient in severely eroded gully bed ecosystems: Combined effects of soil, root traits and plant community characteristics. Plant Soil 398 (1), 121–137. doi:10.1007/s11104-015-2647-6
Fan, H, J., Wu, J., Liu, W., Yuan, Y., Hu, L., and Cai, Q. (2015). Linkages of plant and soil C:N:P stoichiometry and their relationships to forest growth in subtropical plantations. Plant Soil 392 (1), 127–138. doi:10.1007/s11104-015-2444-2
Florio, B., Fawell, P. D., and Small, M. (2019). The use of the perimeter-area method to calculate the fractal dimension of aggregates. Powder Technol. 343, 551–559. doi:10.1016/j.powtec.2018.11.030
Gale, W. J., Cambardella, C. A., and Bailey, T. B. (2000). Root-derived carbon and the formation and stabilization of aggregates. Soil Sci. Soc. Am. J. 64 (1), 201–207. doi:10.2136/sssaj2000.641201x
Gelaw, A. M., Singh, B. R., and Lal, R. (2013). Organic carbon and nitrogen associated with soil aggregates and particle sizes under different land uses in tigray, northern Ethiopia. Land Degrad. Dev. 26, 690. doi:10.1002/ldr.2261
Guo, F., Ding, C., Zhou, Z., Huang, G., and Wang, X. (2018). Stability of immobilization remediation of several amendments on cadmium contaminated soils as affected by simulated soil acidification. Ecotoxicol. Environ. Saf. 161, 164–172. doi:10.1016/j.ecoenv.2018.05.088
Hong, Y., Zhao, D., Zhang, F., Shen, G., Yuan, Y., Gao, Y., et al. (2020). Soil water-stable aggregates and microbial community under long-term tillage in black soil of Northern China. Ecotoxicology 30 (8), 1754–1768. doi:10.1007/s10646-020-02317-x
Hongkai, L., Jian, L., and Juan, L. I. (2012). Distribution characteristics of soil carbon and nitrogen under different vegetation types in micro-habitats of karst dry-hot valley region of south Western China [J]. Soils 44 (3), 421–428. doi:10.13758/j.cnki.tr.2012.03.013
Hou, E., Chen, C., Wen, D., and Liu, X. (2015). Phosphatase activity in relation to key litter and soil properties in mature subtropical forests in China. Sci. Total Environ. 515-516, 83–91. doi:10.1016/j.scitotenv.2015.02.044
Huang, Y., Yang, C., Sun, Z., Zeng, G., and He, H. (2015). Removal of cadmium and lead from aqueous solutions using nitrilotriacetic acid anhydride modified ligno-cellulosic material. RSC Adv. 5 (15), 11475–11484. doi:10.1039/c4ra14859b
Jia, W., Nie, F., Yang, W., Xu, Z., Li, Z., Liyan, Z., et al. (2018). Ecological stoichiometry of soil carbon, nitrogen, and phosphorus within soil aggregates of four plantations in the Western edge of Sichuan Basin [J]. Chin. J. Appl. Environ. Biol. 24 (1), 112–118. doi:10.19675/j.cnki.1006-687x.2017.03049
Jiang, M., Liu, Y., Liu, C., Li, Z., Gu, C., Xie, J., et al. (2016). Study on the stability and fractal characteristics of soil aggregates under different land use patterns in the danjiangkou reservoir [J]. J. Soil Water Conservation 30 (6), 265–270.
Lei, D., Dong-Gill, K., Peng, C., and Zhouping, S. (2018). Controls of soil and aggregate-associated organic carbon variations following natural vegetation restoration on the Loess Plateau in China [J]. Land Degrad. Dev. 29 (11), 3974–3984. doi:10.1002/ldr.3142
Lei, X., Xing, X., Liang, J., Penge, J., and Zhou, J. (2019). In situ phytoremediation of copper and cadmium in a co-contaminated soil and its biological and physical effects [J]. RSC Adv. 9 (2), 993–1003. doi:10.1039/c8ra07645f
Li, Y., Deng, M., Wang, X., Wang, Y., Li, J., Xia, S., et al. (2021). In-situ remediation of oxytetracycline and Cr(VI) co-contaminated soil and groundwater by using blast furnace slag-supported nanosized Fe0/FeSx. Chem. Eng. J. 412 (5), 128706–128719. doi:10.1016/j.cej.2021.128706
Liu, M., Han, G. L., and Zhang, Q. (2019). Effects of soil aggregate stability on soil organic carbon and nitrogen under land use change in an erodible region in southwest China. Int. J. Environ. Res. Public Health 16 (20), 3809–3822. doi:10.3390/ijerph16203809
Liu, S., Yang, B., Liang, Y., Xiao, Y., and Fang, J. (2020). Prospect of phytoremediation combined with other approaches for remediation of heavy metal-polluted soils. Environ. Sci. Pollut. Res. 27 (14), 16069–16085. doi:10.1007/s11356-020-08282-6
Lxab, C., Wei, Z., Pha, B., Dan, X., Rong, Y., Ying, Y, et al. (2021). The formation of large macroaggregates induces soil organic carbon sequestration in short-term cropland restoration in a typical karst area [J]. Sci. Total Environ. 801, 149588–149600. doi:10.1016/j.scitotenv.2021.149588
Maietta, C. E., Bernstein, Z. A., Gaimaro, J. R., Buyer, J. S., Rabenhorst, M. C., Monsaint-Queeney, V. L., et al. (2019). Aggregation but not organo-metal complexes contributed to C storage in tidal freshwater wetland soils. Soil Sci. Soc. Am. J. 83 (1), 252–265. doi:10.2136/sssaj2018.05.0199
Mamirova, A., Pidlisnyuk, V., Amirbekov, A., Sevcu, A., and Nurzhanova, A. (2020). Phytoremediation potential of Miscanthus sinensis And. in organochlorine pesticides contaminated soil amended by Tween 20 and Activated carbon. Environ. Sci. Pollut. Res. 28 (1), 16092–16106. doi:10.1007/s11356-020-11609-y
Mikhailova, E. A., Noble, R. R. P., and Post, C. J. (2003). Comparison of soil organic carbon recovery by walkley-black and dry combustion methods in the Russian chernozem. Commun. Soil Sci. Plant Anal. 34 (13-14), 1853–1860. doi:10.1081/css-120023220
Nguyen, T. T., Cavagnaro, T. R., Ngo, H. T. T., and Marschner, P. (2016). Soil respiration, microbial biomass and nutrient availability in soil amended with high and low C/N residue: Influence of interval between residue additions[J]. Soil Biol. Biochem. 95, 189–197. doi:10.1016/j.soilbio.2015.12.020
Olson, R. V., Elhis, J. R., and Baker, R. H. (1982). Methods of soil analusis, Part 2: Chemical and microbiological properties[M]. Am. Soc. Agron. Acad. Press 9, 301–312. doi:10.2134/agronmonogr9.2.2ed
Pardo, T., Clemente, R., and Bernal, M. P. (2011). Effects of compost, pig slurry and lime on trace element solubility and toxicity in two soils differently affected by mining activities. Chemosphere 84 (5), 642–650. doi:10.1016/j.chemosphere.2011.03.037
Pennanen, T., Srivastava, V., Sillanp, M., and Sainio, T. (2020). Compost: Potent biosorbent for the removal of heavy metals from industrial and landfill stormwater. J. Clean. Prod. 273, 122736–122746. doi:10.1016/j.jclepro.2020.122736
Qin, X., Liu, Y., Wang, L., Li, B., Wang, H., Xu, Y., et al. (2021). Remediation of heavy metal–polluted alkaline vegetable soil using mercapto-grafted palygorskite: Effects of field-scale application and soil environmental quality [J]. Environ. Sci. Pollut. Res., 1–11. doi:10.1007/s11356-021-15034-7
Quan, Y., Wang, J. S., Wang, Y., and Hong-Wen, Y. (2015). Accumulations of copper in apple orchard soils: Distribution and availability in soil aggregate fractions [J]. J. Soils Sediments 15 (5), 1–8.
Quanchao, Z., Xin, L., Yanghong, D., Shaoshan, A., and Frederic, D (2016). Soil and plant components ecological stoichiometry in four steppe communities in the Loess Plateau of China [J]. Catena 147, 481–488. doi:10.1016/j.catena.2016.07.047
Rabbi, S., Minasny, B., Mcbratney, A. B., and Young, I. M. (2019). Microbial processing of organic matter drives stability and pore geometry of soil aggregates. Geoderma 360, 114033–114037. doi:10.1016/j.geoderma.2019.114033
Rzab, C., Xfa, C., Wza, C., Wangac, R., Yangac, D., Weia, W., et al. (2021). Converting loess into zeolite for heavy metal polluted soil remediation based on "soil for soil-remediation" strategy - ScienceDirect [J]. J. Hazard. Mater. 412, 125199–125208. doi:10.1016/j.jhazmat.2021.125199
Taghipour, M., Ayoubi, S., and Khademi, H. (2011). Contribution of lithologic and anthropogenic factors to surface soil heavy metals in western Iran using multivariate geostatistical analyses. Soil Sediment Contam. Int. J. 20 (8), 921–937. doi:10.1080/15320383.2011.620045
Tao, M. J., Zhou, J., and Liang, J. N. (2014). Atmospheric deposition of heavy metals in farmland area around a copper smelter[J]. J. Agro-Environment Sci. 33 (7), 1328–1334. doi:10.1023/A:1020853800937
Tian, H., Chen, G., Zhang, C., Melillo, J. M., and Hall, C. A. S. (2013). Pattern and variation of C:N:P ratios in China’s soils: A synthesis of observational data. Biogeochemistry 98 (3), 139–151. doi:10.1007/s10533-009-9382-0
Turner, B. L., Condron, L. M., Richardson, S. J., Peltzer, D. A., and Allison, V. J. (2007). Soil organic phosphorus transformations during pedogenesis. Ecosystems 10 (7), 1166–1181. doi:10.1007/s10021-007-9086-z
Valipour, M., Shahbazi, K., and Khanmirzaei, A. (2016). Chemical immobilization of lead, cadmium, copper, and nickel in contaminated soils by phosphate amendments. Clean. Soil Air Water 44 (5), 572–578. doi:10.1002/clen.201300827
Walker, D. J., Clemente, R., Roig, A., and Bernal, M. (2003). The effects of soil amendments on heavy metal bioavailability in two contaminated Mediterranean soils. Environ. Pollut. 122 (2), 303–312. doi:10.1016/s0269-7491(02)00287-7
Wang, F. Y., Lin, X. G., and Yin, R. (2005). Heavy metal uptake by arbuscular mycorrhizas of Elsholtzia splendens and the potential for phytoremediation of contaminated soil. Plant Soil 269, 225–232. doi:10.1007/s11104-004-0517-8
Wang, S., Zhang, Z., and Ye, S. (2020). Response of soil fertility characteristics in water-stable aggregates to tea cultivation age in hilly region of southern Guangxi, China. Catena 191, 104578–104589. doi:10.1016/j.catena.2020.104578
Wu, L. H., Li, Z., Akahane, L., Liu, L., Han, C., Makino, T., et al. (2012). Effects of organic amendments on Cd, Zn and Cu bioavailability in soil with repeated phytoremediation by Sedum plumbizincicola[J]. Int. J. Phytoremediation 14 (10), 1024–1038. doi:10.1080/15226514.2011.649436
Wu, L., Li, Z., Akahane, I., Liu, L., Han, C., Makino, T., et al. (2012). Effects of organic amendments on Cd, Zn and Cu bioavailability in soil with repeated phytoremediation bysedum plumbizincicola. Int. J. Phytoremediation 14 (10), 1024–1038. doi:10.1080/15226514.2011.649436
Xiao, S., Wei, Z., Ye, Y., Zhao, J., and Wang, K. (2017). Soil aggregate mediates the impacts of land uses on organic carbon, total nitrogen, and microbial activity in a Karst ecosystem. Sci. Rep. 7, 41402–41412. doi:10.1038/srep41402
Xu, L., Cui, H., Zheng, X., Zhu, Z., Liang, J., and Zhou, J. (2016). Immobilization of copper and cadmium by hydroxyapatite combined with phytoextraction and changes in microbial community structure in a smelter-impacted soil. RSC Adv. 6 (106), 103955–103964. doi:10.1039/c6ra23487a
Xu, L., Xing, X. Y., Liang, J. N., Peng, J., and Zhou, J. (2019). In situ phytoremediation of copper and cadmium in a co-contaminated soil and its biological and physical effects. RSC Adv. 9 (2), 993–1003. doi:10.1039/c8ra07645f
Xu, L., Zhou, J., and Liang, J. N. (2014). The Remediation potential of Pennisetum sp on Cu、Cd contaminated soil[J]. Acta Ecol. Sin. 34 (18), 5342–5348. doi:10.5846/stxb201405110960
Xue, S., Liu, G. B., Dai, Q. H., Li, X. L., and Wu, R. J. (2008). [Dynamic changes of soil microbial biomass in the restoration process of shrub plantations in loess hilly area]. Chin. J. Appl. Ecol. 19 (3), 517–523. doi:10.13287/j.1001-9332.2008.0141
Yan, L., Jiang, X., Ji, X., Zhou, L., Li, S., Chen, C., et al. (2020). Distribution of water-stable aggregates under soil tillage practices in a black soil hillslope cropland in Northeast China. J. Soils Sediments 20 (5), 24–31. doi:10.1007/s11368-019-02361-z
Yang, R., Guo, F., Zan, S., Zhou, G., Wille, W., Tang, J., et al. (2015). Copper tolerant Elsholtzia splendens facilitates Commelina communis on a copper mine spoil. Plant Soil 397 (1), 201–211. doi:10.1007/s11104-015-2616-0
Zeng, Q., Darboux, F., Man, C., Zhu, Z., and An, S. (2018). Soil aggregate stability under different rain conditions for three vegetation types on the Loess Plateau (China). Catena 167, 276–283. doi:10.1016/j.catena.2018.05.009
Zhang, C. Y., and Sheng-Xiu, L. I. (2005). Does the total soil N determined by Kjeldahl method include fixed NH4+? [j]. J. Agro-environment Sci. 4 (2), 134–141.
Zhang, W. H., Sun, R. B., Xu, L., Liang, J. n., Wu, T. y., and Zhou, J. (2019). Effects of micro-/nano-hydroxyapatite and phytoremediation on fungal community structure in copper contaminated soil. Ecotoxicol. Environ. Saf. 174, 100–109. doi:10.1016/j.ecoenv.2019.02.048
Zhao, Y. S., Han, C. H., Zhang, H. G., Chen, Q., Liu, M., and Han, X. C. (2012). Soil hydrologic functions of main forest types in ashi river's upstream watershed [J]. J. Soil Water Conservation 26 (2), 203–208.
Zheng, X. B., Fan, J. B., and Zhou, J. (2015). Effects of biogas slurry on soil organic matter and characteristics of soil aggregates in upland red Earth[J]. Sci. Agric. Sin. 48 (16), 3201–3210. doi:10.1371/journal.pone.0170491
Zhou, J., Cui, H. B., Liang, J. N., Xu, L., Zhu, Z. Q., Wang, X., et al. (2015). Remediation technologies and current problems of heavy metal contaminated sites with "demonstration Project of soil remediation on the periphery of Guixi smelter" as example. Soils 47 (2), 283–288. doi:10.13758/j.cnki.tr.2015.02.014
Keywords: vegetation restoration, aggregate structure, aggregate stability, stoichiometric characteristics, contamination
Citation: Xu L, Xing X, Bai J and Li D (2022) Soil aggregate structure, stability, and stoichiometric characteristics in a smelter-impacted soil under phytoremediation. Front. Environ. Sci. 10:900147. doi: 10.3389/fenvs.2022.900147
Received: 20 March 2022; Accepted: 04 July 2022;
Published: 29 August 2022.
Edited by:
Andrés Rodríguez-Seijo, University of Porto, PortugalReviewed by:
Shamsollah Ayoubi, Isfahan University of Technology, IranMasahiko Katoh, Meiji University, Japan
Copyright © 2022 Xu, Xing, Bai and Li. This is an open-access article distributed under the terms of the Creative Commons Attribution License (CC BY). The use, distribution or reproduction in other forums is permitted, provided the original author(s) and the copyright owner(s) are credited and that the original publication in this journal is cited, in accordance with accepted academic practice. No use, distribution or reproduction is permitted which does not comply with these terms.
*Correspondence: Lei Xu, aGVkYXhsQDE2My5jb20=