- 1Institute of Eco-Environmental Forensics, Qingdao Institute of Humanities and Social Sciences, Shandong University, Qingdao, China
- 2Ministry of Justice Hub for Research and Practice in Eco-Environmental Forensics, Qingdao, China
- 3Research Center for Eco-Environmental Sciences, Chinese Academy of Sciences, Beijing, China
- 4Center for Environmental Remediation, Institute of Geographical Sciences and Natural Resources, Chinese Academy of Sciences, Beijing, China
- 5College of Land and Environment, Shenyang Agricultural University, Shenyang, China
Plant metabolic regulation is an important strategy for dealing with abiotic stress, which can initiate dramatic changes in metabolite profiles. Metabolomic studies have been extensively used to reveal the responses of plants to abiotic stress, but research concerning how shade growth conditions impact the physiological responses of purple Perilla under Cd stress is still rare. Therefore, the research was designed to observe the important metabolites of purple Perilla under shaded conditions and Cd stress. In this study, we compared the metabolite profiles of purple Perilla exposed to shade and Cd stresses. The results showed that the metabolite profiles of purple Perilla under these stresses could be classified separately. Compared with the normal sunlight treatment, important energy metabolic pathways, including the citrate cycle, and glyoxylate and dicarboxylate metabolism, were inhibited under shade stress. Metabolite analysis revealed that pyruvate was involved in numerous metabolic pathways under Cd stress, and decreased pyruvate was regarded as a metabolite marker for physiological changes. The results show that increased thiamine is an important intermediate that is beneficial for enhancement of Cd stress tolerance.
Introduction
Heavy metal contamination in soil is a growing concern worldwide (Zhang et al., 2019), as it is not easily degraded and can be readily transferred from the soil to the human body via the food chain, thus endangering human health (Zhang et al., 2016). In China, about 20% of agricultural soil has been contaminated by heavy metals or metalloids. Therefore, it is essential to remediate heavy metal-contaminated soil, thereby preventing heavy metal from entering the human body via the consumption of contaminated crops.
Purple Perilla [Perilla frutescens (L.) Britt.] is an annual herb that has been commonly used as a traditional Chinese medicine for more than 1,000 years. Purple Perilla has significant anti-allergic, anti-inflammatory, and strong antitumor-promoting activities (You et al., 2014). In addition, purple Perilla has great potential for the phytoremediation of Cd-contaminated soil. However, research concerning how shade growth conditions impact the physiological responses of purple Perilla under Cd stress is still rare.
Metabolomics is a branch of science that focuses on endogenous metabolite varieties, quantities, and change rules in living organisms due to external stimuli, pathophysiological changes, and their own gene mutations. Metabolomics has been applied in studying plant growth and development and stress tolerance. Through the use of metabonomic technology to analyze the metabolic level differences between experimental and the control groups, differential metabolites can be identified in order to reveal the mechanisms of biological processes and life activities. Plants grown on metal-contaminated soils can regulate the synthesis of secondary metabolites depending on the plant species tested, the type of secondary metabolite, the period of exposure, and the level of heavy metal stress (Xiong et al., 2013). Elevated atmospheric CO2 enhances photosynthesis, thus altering flavonoid, total phenolic, and condensed tannin content in plants (Jia et al., 2014). The combination of heavy metal contamination in soils and elevated atmospheric CO2 has important effects on plant physiology and growth (Jia et al., 2016). Besides CO2, irradiance is one of the dominant factors influencing plant photosynthesis. The production of plant metabolites is affected by Cd under different levels of irradiance.
The main aims of this research were: 1) to study Cd enrichment in purple Perilla under shaded conditions; 2) to observe the physiological characteristics of purple Perilla under shaded conditions and Cd stress; and 3) to identify the differential metabolites under shade and Cd stress, and to reveal the important metabolic pathway of adaptation to the external environment.
Materials and Methods
Plant Materials and Cultivation
Purple Perilla seeds were sterilized with 1% (m/v) hydrogen peroxide for 60 min and rinsed thoroughly with distilled water. Then, purple Perilla seeds were initially grown on artificial, non-polluted soil for 2–3 weeks until the seedlings developed two healthy, tender leaves. These uniform seedlings were used as research subjects for hydroponic experiments in a greenhouse located at Shenyang Agricultural University, Shenyang, Liaoning, China. The hydroponic experiment was carried out in a greenhouse at temperatures of 25/15°C (day/night), a 16-h photoperiod of approximately 300 mE/m2/s intensity, and 65% average relative humidity.
Experimental Design
The uniform seedlings were transplanted in to 1 L pots containing 400 ml of Hoagland’s solution (half strength) of the following composition: 2.5 mmol/L Ca(NO3)2, 2.5 mmol/L KNO3, 0.5 mmol/L KH2PO4, 0.5 mmol/L MgSO4, 25 μmol/L H3BO3, 2.25 μmol/L MnCl2, 1.9 μmol/L ZnSO4, 0.15 μmol/L CuSO4, 0.05 mmol/L (NH4)6Mo7O24, and 5 μmol/L Fe-EDTA. A cadmium salt, CdCl2 2.5H2O (guaranteed reagent) was used as the pollutant source. The Cd salt was added to the hydroponic culture. The pH of the nutrient solution was maintained at 6.0 by the addition of 0.1 mol/L NaOH. Four treatments were used: control check (CK), 50% shade treatment (T1), Cd treatment (T2), and 50% shade + Cd treatment (T3). The hydroponic solution was renewed every 4 days. After 16 days, the plants were harvested. The dry tissues were used for Cd concentration analysis with three biological replicates for each treatment. Meanwhile, fresh leaves were frozen in liquid nitrogen and stored at −80°C for metabolite analysis, with six biological replicates for each stress treatment.
Plant Sample Preparation and Cd Concentration Analysis
Each harvested plant was divided into root and stem sections. Individual plant parts (roots and stems) were first washed with tap water and then rinsed three times with deionized water. The plant parts used for the determination of Cd concentrations were oven-dried at 110°C for 30 min, and then dried at 70°C for 96 h to constant weight.
Roots and stems were separately ground in a mill, and then digested in flasks at 80°C on an electric heating plate with concentrated HNO3. The temperature was then increased to 110°C, and the mixture was maintained at that temperature until the solution became clear (Zhang et al., 2016). The sample volume was adjusted to 25 ml with ultrapure water. The Cd concentrations in each sample were measured with an inductively coupled plasma mass spectrometer (ICP-MS) (Agilent 7500a, United States). Standard reference material for bush twigs and leaves (GBW07603: GSV-2) was used to monitor the heavy metal recovery from the plant samples (recovery: 90 ± 10%).
The chemical analysis was carried out at the Analytical and Testing Center, Shenyang Agricultural University, Shenyang, Liaoning, China.
Plant Tissues for Liquid Chromatography Mass Spectrometry Analysis
The plant tissues (80 mg of leaves) were quickly frozen in liquid nitrogen and ground into fine powder with a mortar and pestle. One thousand microliters of methanol/acetonitrile/H2O (2:2:1, v/v/v) were added to homogenized solution for metabolite extraction. The mixture was centrifuged for 15 min (14,000 g, 4°C) and the supernatant was dried in a vacuum centrifuge. For LC-MS analysis, the samples were re-dissolved in 100 μL acetonitrile/water (1:1, v/v) solvent.
Analyses were performed using ultra-high performance liquid chromatography (UHPLC) (1290 Infinity LC, Agilent Technologies, United States) coupled to a quadrupole time-of-flight (AB Sciex TripleTOF 6600, United States).
For HILIC separation, samples were analyzed using a 2.1 mm × 100 mm ACQUIY UPLC BEH 1.7-µm column (Waters, Ireland). In both electrospray ionization (ESI)-positive and ESI-negative modes, the mobile phase contained A = 25 mm ammonium acetate and 25 mm ammonium hydroxide in water and B = acetonitrile. The gradient was 85% B for 1 min and was linearly reduced to 65% in 11 min. It was then reduced to 40% in 0.1 min and maintained for 4 min, after which it increased to 85% in 0.1 min, with a 5-min re-equilibration period.
Statistical Analysis
The data of Cd concentrations detected by ICP-MS were analyzed using ANOVA testing and SPSS 18.0 software (SPSS, Chicago, IL, United States). Data, presented as mean values, were compared by Duncan’s test. A p-value less than 0.05 was considered to indicate a significant difference.
For the metabolite data, the processed data were analyzed using the R package (ropls) after being normalized to total peak intensity. The data were then subjected to multivariate data analysis, including Pareto-scaled principal component analysis (PCA) and orthogonal partial least-squares discriminant analysis (OPLS-DA). Seven-fold cross-validation and response permutation testing were used to evaluate the robustness of the model. The variable importance in the projection (VIP) value of each variable in the OPLS-DA model was calculated to indicate its contribution to the classification. Metabolites with VIP values > 1 were further applied to the Student’s t-test at the univariate level to measure the significance of each metabolite, and p-values less than 0.05 were considered to be statistically significant.
Results
Heavy Metal Accumulation
The Cd accumulation in the roots and stems of purple Perilla grown in each treatment is shown in Figure 1. Compared with the normal sunlight treatment (T2), the shade stress (T3) increased the Cd concentrations in the roots and reduced the Cd concentrations in the stem. In addition, shade stress caused the translocation rate from roots to shoots to improve relative to the normal sunlight treatment.
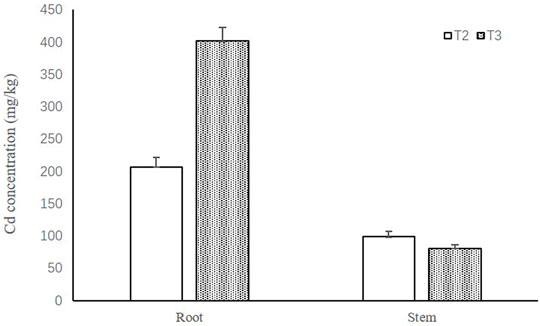
FIGURE 1. Cd concentrations in the roots and stems of purple Perilla. T2: Cd + normal sunlight; T3: Cd + shade condition.
Metabolite Profiling in Response to Shade and Cd Stresses
To investigate the purple Perilla metabolism in response to shade and Cd stresses, the metabolites in leaves were measured using UHPLC-MS. In total, 247 metabolites were detected in the leaves, including 134 metabolites detected by the positive mode, and 113 metabolites detected by the negative mode. These metabolites could be mainly classified into three groups, including organic acids and derivatives, organic oxygen compounds, and lipids and lipid-like molecules (Supplementary Figure S1).
With the OPLS-DA model, the samples from the control and all stress treatments could be clearly separated for the examined metabolites in leaves, indicating that the metabolite profiles were changed markedly by any stress treatment (Supplementary Figure S2). Variable importance for the projection (VIP) obtained from the OPLS-DA model can measure the explanatory ability of the expression patterns of metabolites for the classification and discrimination of each group of samples. Generally, metabolites with VIP values >1 were considered to have made a significant contribution to model interpretation. In this experiment, the metabolites with VIP values >1 and p-values < 0.05 were considered to show significant differences. These metabolites are displayed in Supplementary Table S1. Overall, we identified 18 increased metabolites and 55 decreased metabolites in the various stress treatments.
Metabolite Change in Purple Perilla Leaves Responding to Shade and Cd Stresses
To evaluate the effects of shade and Cd stresses on metabolite profiles, the ratios of metabolite levels under each stress and those in the control treatment were calculated (Supplementary Table S1). Notably, meso-tartaric acid was increased by 2.30-fold under shaded condition relative to the control. In the Cd treatment, 2-hydroxyadenine showed a 2.16-fold increase relative to the control. Additionally, DL-indole-3-lactic acid increased dramatically by 9.20-fold when exposed to shade and Cd stresses compared to shaded conditions alone.
Under shade and Cd stresses, the metabolites belonging to different classifications accumulated differently (Figure 2). In comparison with normal sunlight, the organic nitrogen compounds were significantly increased (Figure 2B), while the sharp decline of organic acids and derivatives in addition to lipids and lipid-like molecules occurred (Figures 2A,B) under the shade condition. With Cd stress, the organic acids and derivatives showed a marked increase (Figures 2C,D). However, the organic nitrogen compounds, organic oxygen compounds, lipids, and lipid-like molecules decreased markedly (Figure 2C). Relative to the shade treatment, the benzenoids and organic nitrogen compounds increased significantly, while the majority of the organic oxygen compounds showed a marked reduction when exposed to shade and Cd stresses (Figures 2E,F).
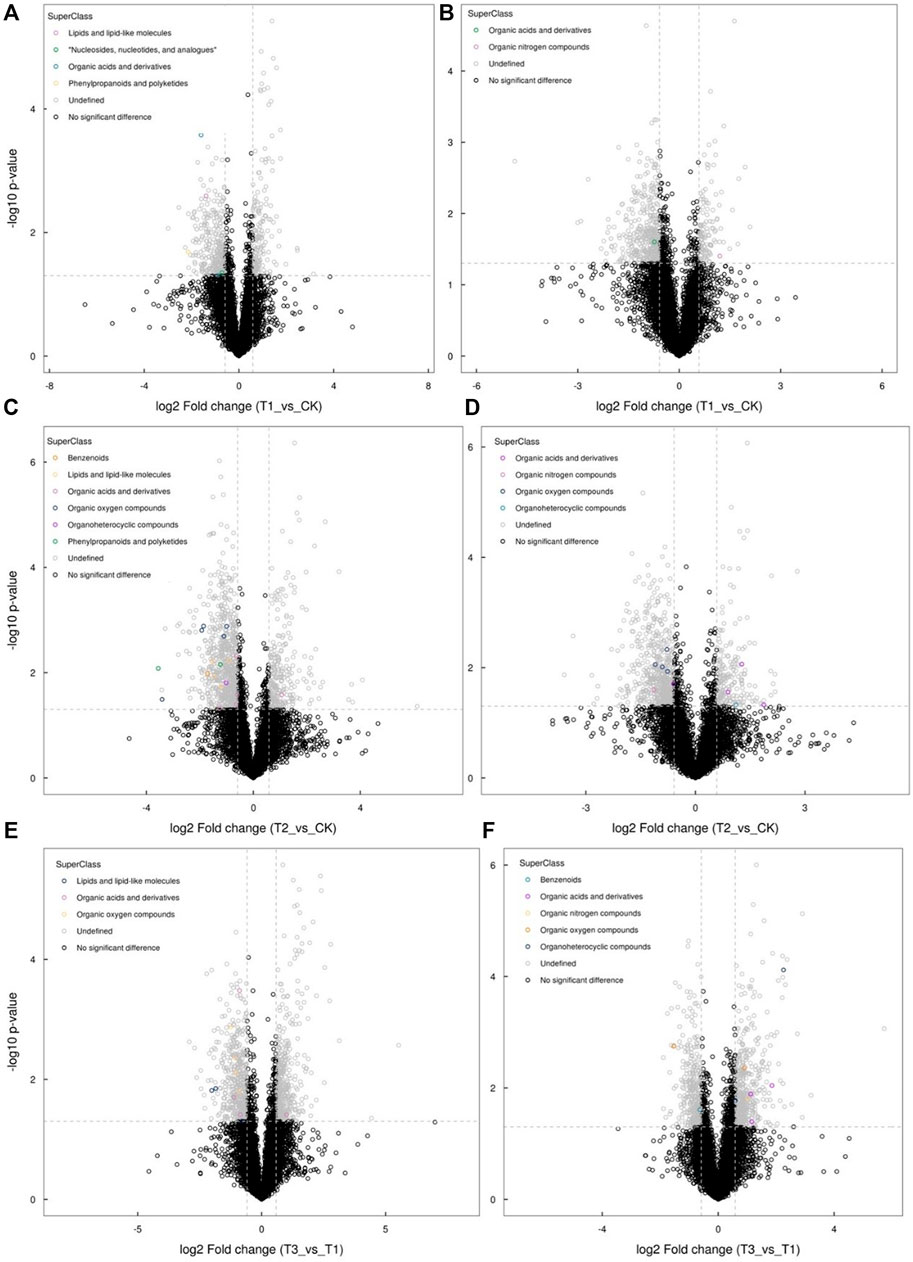
FIGURE 2. Differentially accumulated metabolites belonging to the different classifications, as shown using the negative ionization mode (A,C,E) and the positive ionization mode (B,D,F), between the control (CK) and shade treatment (T1), the control (CK) and Cd treatment (T2), and the shade treatment (T1) and shade + Cd treatment (T3).
Kyoto Encyclopedia of Genes and Genomes (KEGG) enrichment analysis was performed to identify metabolic pathways that were altered in response to abiotic stresses (Figure 3). Twenty pathways were the most significantly varied (p < 0.05, VIP >1). In comparison with the control, glyoxylate and dicarboxylate metabolism and the citrate cycle (TCA cycle) were highly changed (Figure 3A). Under exposure to Cd stress, we found that galactose metabolism and the TCA cycle were strongly influenced (Figure 3B). Moreover, shade and Cd stresses together generated the biosynthesis of amino acids and ascorbate and aldarate metabolism with striking differences relative to the shade treatment (Figure 3C).
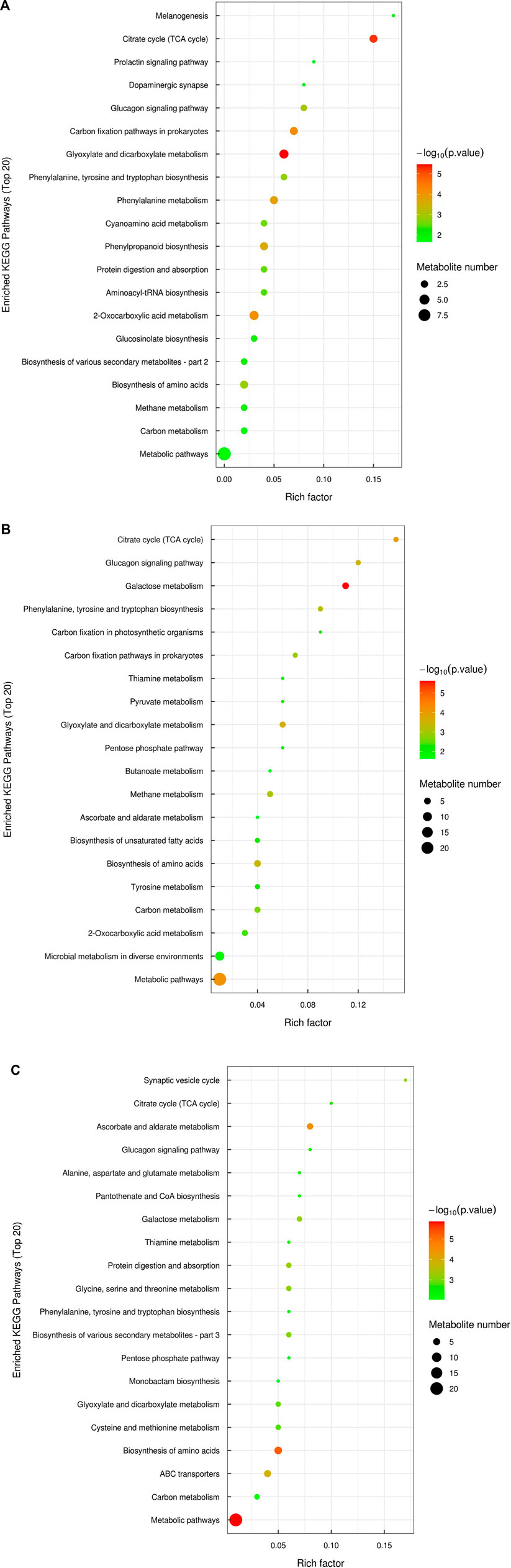
FIGURE 3. Kyoto Encyclopedia of Genes and Genomes (KEGG) enrichment analysis of differentially accumulated metabolites between the control and shade treatment (A), the control and Cd treatment (B), and the shade treatment and shade + Cd treatment (C). Each bubble represents a metabolic pathway. The larger the bubble, the greater the impact factor. The deeper the color, the more significant the enrichment degree.
Discussion
Cd Transportation Under Shaded Conditions
There are four major processes involved in Cd transport from roots to stems, including the symplast pathway and apoplast pathway in roots, xylem loading, the symplast pathway and apoplast pathway in leaves, and phloem loading (Mendoza-Cózatl et al., 2011; Wei et al., 2018). Our study found that shade stress inhibited the transport capability from the roots toward the stems relative to normal sunlight. Shade stress reduced photosynthesis and transpiration, which increased Cd accumulation in roots and decreased Cd accumulation in stems (Figure 1). Photosynthesis is an important physiological process for plant productivity that directly provides energy for plant growth (Chen et al., 2020). The reduction of photosynthesis decreased the energy supply for Cd transport in the symplast pathway and the phloem. Simultaneously, we found that the TCA cycle as well as glyoxylate and dicarboxylate metabolism, as the energy metabolic pathways, were importantly influenced by shade stress (Figure 4A). Even more importantly, the inhibition of transpiration reduced Cd transport in the xylem, which is the important pathway from roots toward stems.
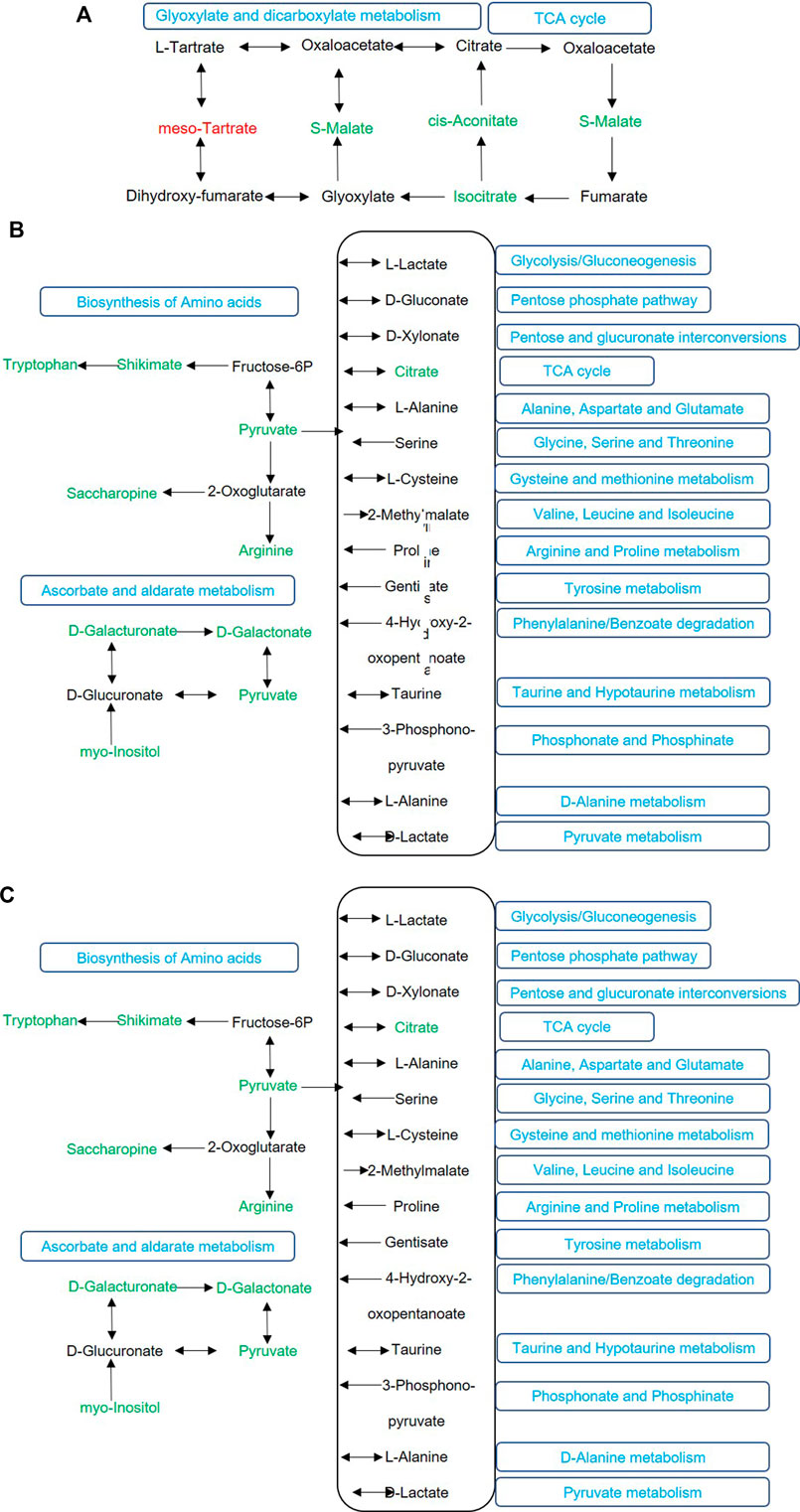
FIGURE 4. The important metabolic pathways of differentially accumulated metabolites between the control and shade treatment (A), the control and Cd treatment (B), and the shade treatment and shade + Cd treatment (C). The level of metabolites compared to that of control group is marked in red (upregulated) and green (downregulated). The black color represents the undetected metabolites. The blue words indicate the related metabolic pathways.
Differential Change of Metabolites Under Shade and Cd Stresses
When exposed to various abiotic stresses, plants can regulate their metabolisms in order to alleviate the stress effect (Sicher et al., 2012). Our study showed that all cis-(6,9,12)-linolenic acid accumulated significantly under both types of stress (Supplementary Table S1). Moreover, thiamine was upregulated importantly in Cd stress, whether under normal sunlight or shade stress (Supplementary Table S1). Similarly, Zhao et al. (2021) also found that some stresses (including osmotic stress and metal stress) have similar effects on changes in the metabolites in barley plants. It is thus deduced that more accumulation of all cis-(6,9,12)-linolenic acid or thiamine confers purple Perilla with higher tolerance to abiotic stresses. Previous studies have demonstrated the protective role of thiamine in plants grown under various stress conditions, such as salt (Kaya and Ashraf, 2015), copper (Al-Hakimi and Hamada 2011), and Cd stresses (Kaya and Aslan 2020). Our results also showed that the increase of thiamine improved the tolerance to Cd stress in plants (Supplementary Table S1). Previous research revealed that thiamine improved the antioxidant defense system, eliminated the reactive oxygen species (ROS), reduced the Cd content, and improved the Ca and K contents, inducing enhanced tolerance to Cd stress in plants (Kaya and Aslan 2020).
The majority of the metabolites with significant differences were downregulated under shade and Cd stresses (Supplementary Table S1). In the shade and Cd treatments, the organic acids and derivatives (l-malic acid and isocitrate), lipids and lipid-like molecules (3-hydroxy-3-methylglutaric acid), and phenylpropanoids and polyketides (hydroxyphenyllactic acid) decreased. Whether under normal sunlight or shaded conditions, under exposure to Cd, the organic acids and derivatives (pyruvate), the organic oxygen compounds (galactinol, d-galactarate, shikimate, glyceric acid, and d-lactose), and lipids and lipid-like molecules (oleanolic acid and palmitic acid) were downregulated. However, previous studies found that some of above-mentioned metabolites increased under Cd stress, suggesting that they were involved in a defense mechanism in response to abiotic stress. For instance, galactinol has been considered an ROS scavenger under abiotic stress, protecting cells from oxidative stress (Keunen et al., 2013). Thus, it may be assumed that different changes in these metabolites may be influenced by plant species and different forms of abiotic stress. This should be clarified in future work.
Metabolic Pathways in Response to Shade and Cd Stresses
Metabolic pathways were analyzed on the basis of the important metabolites listed in Supplementary Table S1. Compared with single shade stress, Cd stress inhibited the synthesis of pyruvate, whether under sunlight or shaded conditions (Figure 4). Therefore, it may be suggested that the decrease of pyruvate is a unique event for purple Perilla suffering from Cd stress. Moreover, the reduction of pyruvate, an important intermediate involved in various metabolic pathways, changed purple Perilla metabolism (Figures 4B,C). Thus, the decrease of pyruvate could be regarded as the metabolite marker for plants under Cd stress. Gil-Monreal et al. (2018) confirmed that pyruvate as a signal participated in the regulation of ethanol fermentation in plants treated with herbicides. To the authors’ knowledge, our study describes for the first time the significance of pyruvate involved in metabolic pathway under Cd stress.
Under exposure to different abiotic stresses (Figure 4), five common metabolic pathways were identified in the top 20 pathways, including the TCA cycle; glyoxylate and dicarboxylate metabolism; the glucagon signaling pathway; phenylalanine, tyrosine, and tryptophan biosynthesis; and carbon metabolism. Moreover, the TCA cycle, glyoxylate and dicarboxylate metabolism, and carbon metabolism are associated with energy metabolism. Zhao et al. (2021) found that cadmium treatment resulted in the upregulation of the TCA cycle, with some intermediates showing significant increases in roots, which may be attributed to enhanced respiration under abiotic stress. Therefore, the energy metabolism is changed, which may be a general phenomenon under different forms of abiotic stress.
Conclusion
In this study, we compared the metabolite profiles of purple Perilla exposed to two abiotic stresses and examined how the metabolite profiles were altered by shade and Cd stresses. Shade stress inhibited important energy metabolic pathways, reducing the Cd transportation capability from roots toward stems. Moreover, the results show that pyruvate may be used as a marker for diagnosing certain forms of abiotic stress in plants, and that thiamine is an important intermediate beneficial to the enhancement of Cd stress tolerance. In the future, we will focus on the pyruvate and the thiamine influencing the plant physiological responses under the abiotic stress.
Data Availability Statement
The original contributions presented in the study are included in the article/Supplementary Material, further inquiries can be directed to the corresponding author.
Author Contributions
YZ: Data analysis and Writing the manuscript. HZ: Experimental design, Sample measurement, Data analysis and Writing the manuscript.
Funding
This research was financially supported by the National Natural Science Foundation of China (No. 31901209), China Postdoctoral Science Foundation (No. 2018M641492), and Talent Introduction Program of Shenyang Agricultural University of China (No. 880418034).
Conflict of Interest
The authors declare that the research was conducted in the absence of any commercial or financial relationships that could be construed as a potential conflict of interest.
Publisher’s Note
All claims expressed in this article are solely those of the authors and do not necessarily represent those of their affiliated organizations, or those of the publisher, the editors and the reviewers. Any product that may be evaluated in this article, or claim that may be made by its manufacturer, is not guaranteed or endorsed by the publisher.
Acknowledgments
We thank LetPub (www.letpub.com) for its linguistic assistance during the preparation of this manuscript.
Supplementary Material
The Supplementary Material for this article can be found online at: https://www.frontiersin.org/articles/10.3389/fenvs.2022.896963/full#supplementary-material
Supplementary Figure S1 | The chemical classification of the identified metabolites.
Supplementary Figure S2 | The orthogonal partial least-squares discriminant analysis (OPLS-DA) under diverse stresses, with the negative ionization mode (A,C,E) and the positive ionization mode (B,D,F).
Supplementary Table S1 | Identification of significantly regulated metabolites between control and treatment groups, VIP: variable importance in the projection.
References
Al-Hakimi, A.-B. M., and Hamada, A. M. (2011). Ascorbic Acid, Thiamine or Salicylic Acid Induced Changes in Some Physiological Parameters in Wheat Grown under Copper Stress. Plant Protect. Sci. 47, 92–108. doi:10.17221/20/2010-pps
Chen, H.-C., Zhang, S.-L., Wu, K.-J., Li, R., He, X.-R., He, D.-N., et al. (2020). The Effects of Exogenous Organic Acids on the Growth, Photosynthesis and Cellular Ultrastructure of Salix Variegata Franch. Under Cd Stress. Ecotoxicology Environ. Saf. 187, 109790. doi:10.1016/j.ecoenv.2019.109790
Gil-Monreal, M., Fernandez-Escalada, M., Royuela, M., and Zabalza, A. (2018). An Aerated Axenic Hydroponic System for the Application of Root Treatments: Exogenous Pyruvate as a Practical Case. Plant Methods 14, 48. doi:10.1186/s13007-018-0310-y
Jia, X., Wang, W., Chen, Z., He, Y., and Liu, J. (2014). Concentrations of Secondary Metabolites in Tissues and Root Exudates of Wheat Seedlings Changed under Elevated Atmospheric CO2 and Cadmium-Contaminated Soils. Environ. Exp. Bot. 107, 134–143. doi:10.1016/j.envexpbot.2014.06.005
Jia, X., Zhao, Y., Liu, T., and Huang, S. (2016). Elevated CO2 Affects Secondary Metabolites in Robinia Pseudoacacia L. Seedlings in Cd- and Pb-Contaminated Soils. Chemosphere 160, 199–207. doi:10.1016/j.chemosphere.2016.06.089
Kaya, C., and Ashraf, M. (2015). Exogenous Application of Nitric Oxide Promotes Growth and Oxidative Defense System in Highly boron Stressed Tomato Plants Bearing Fruit. Scientia Horticulturae 185, 43–47. doi:10.1016/j.scienta.2015.01.009
Kaya, C., and Aslan, M. (2020). Hydrogen Sulphide Partly Involves in Thiamine-Induced Tolerance to Cadmium Toxicity in Strawberry (Fragaria X Ananassa Duch) Plants. Environ. Sci. Pollut. Res. 27, 941–953. doi:10.1007/s11356-019-07056-z
Keunen, E., Peshev, D., Vangronsveld, J., Van Den Ende, W., and Cuypers, A. (2013). Plant Sugars Are Crucial Players in the Oxidative challenge during Abiotic Stress: Extending the Traditional Concept. Plant Cel Environ 36, 1242–1255. doi:10.1111/pce.12061
Mendoza-Cózatl, D. G., Jobe, T. O., Hauser, F., and Schroeder, J. I. (2011). Long-distance Transport, Vacuolar Sequestration, Tolerance, and Transcriptional Responses Induced by Cadmium and Arsenic. Curr. Opin. Plant Biol. 14, 554–562. doi:10.1016/j.pbi.2011.07.004
Sicher, R. C., Timlin, D., and Bailey, B. (2012). Responses of Growth and Primary Metabolism of Water-Stressed Barley Roots to Rehydration. J. Plant Physiol. 169, 686–695. doi:10.1016/j.jplph.2012.01.002
Wei, R., Guo, Q., Yu, G., Kong, J., Li, S., Song, Z., et al. (2018). Stable Isotope Fractionation during Uptake and Translocation of Cadmium by Tolerant Ricinus communis and Hyperaccumulator Solanum nigrum as Influenced by EDTA. Environ. Pollut. 236, 634–644. doi:10.1016/j.envpol.2018.01.103
Xiong, H., Duan, C., A, X., and Chen, M. (2013). Response of Scutellarin Content to Heavy Metals in Erigeron Breviscapus. Ijesd 4, 277–281. doi:10.7763/ijesd.2013.v4.353
You, C.-x., Wang, Y., Zhang, W.-j., Yang, K., Wu, Y., Geng, Z.-f., et al. (2014). Chemical Constituents and Biological Activities of the Purple Perilla Essential Oil against Lasioderma Serricorne. Ind. Crops Prod. 61, 331–337. doi:10.1016/j.indcrop.2014.07.021
Zhang, D., Du, G., Chen, D., Shi, G., Rao, W., Li, X., et al. (2019). Effect of Elemental Sulfur and gypsum Application on the Bioavailability and Redistribution of Cadmium during rice Growth. Sci. Total Environ. 657, 1460–1467. doi:10.1016/j.scitotenv.2018.12.057
Zhang, H., Guo, Q., Yang, J., Ma, J., Chen, G., Chen, T., et al. (2016). Comparison of Chelates for Enhancing Ricinus communis L. Phytoremediation of Cd and Pb Contaminated Soil. Ecotoxicology Environ. Saf. 133, 57–62. doi:10.1016/j.ecoenv.2016.05.036
Keywords: purple perilla, Cd stress, shade environment, metabolite, physiological responses
Citation: Zhang Y and Zhang H (2022) Shade and Cd Conditions Strongly Impact the Physiological Responses of Purple Perilla. Front. Environ. Sci. 10:896963. doi: 10.3389/fenvs.2022.896963
Received: 15 March 2022; Accepted: 11 April 2022;
Published: 17 May 2022.
Edited by:
Chuan Wu, Central South University, ChinaReviewed by:
Hayssam M. Ali, King Saud University, Saudi ArabiaJuanjuan Fu, Northwest A&F University, China
Copyright © 2022 Zhang and Zhang. This is an open-access article distributed under the terms of the Creative Commons Attribution License (CC BY). The use, distribution or reproduction in other forums is permitted, provided the original author(s) and the copyright owner(s) are credited and that the original publication in this journal is cited, in accordance with accepted academic practice. No use, distribution or reproduction is permitted which does not comply with these terms.
*Correspondence: Hanzhi Zhang, emhhbmdoYW56aGloYW5AMTYzLmNvbQ==