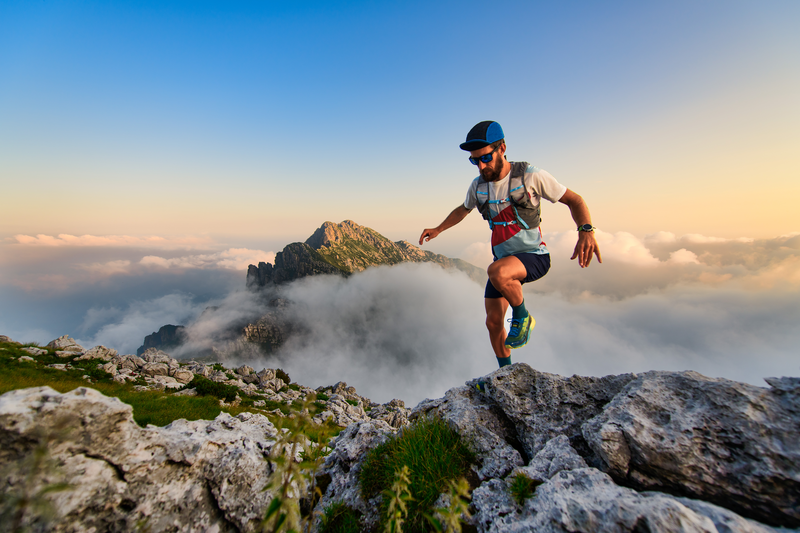
95% of researchers rate our articles as excellent or good
Learn more about the work of our research integrity team to safeguard the quality of each article we publish.
Find out more
ORIGINAL RESEARCH article
Front. Environ. Sci. , 01 June 2022
Sec. Water and Wastewater Management
Volume 10 - 2022 | https://doi.org/10.3389/fenvs.2022.895463
The present study comprises the synthesis of a novel composite polyaniline-wheat husk (PANI-WH) and the adsorption of heavy metals (Cu, Pb, and Ni) by using this composite. Characterizations such as SEM of the composite were used to check the chemical composition, state (XPS), crystallographic nature (XRD), and functional groups (FT-IR) of the novel composite before and after the adsorption of heavy metals. The results indicate that the adsorption performance of the PANI-WH novel composite (on heavy metals) is good with excellent binding capacity of 155 mg g−1 for Ni, 95 mg g−1 for Cu, and 110 mg g−1 for Pb. The PANI-WH novel composite shows maximum adsorption at optimum conditions. The remediation capacity of heavy metals was observed up to 99% by using the PANI-WH composite. The present study also involves kinetic, thermodynamic, and isotherm studies, showing that the adsorption of heavy metals is increased by using the PANI-WH composite. The composite material used in this work comes from industrial/agricultural by-products which provide a twofold benefit of not only providing a means of treating water but also an effective method of utilization of these by-products, which otherwise form an additional waste stream. The PANI-WH shows good stability and reusability. In future, the PANI-WH composite can be used for the detection of other heavy metals.
The discharge of industrial waste has polluted the environment due to increased industrialization worldwide. This wastewater is most of the time laden with significant amounts of heavy metals that are easily deposited into the natural ecosystem, specifically aquatic ecosystems (Upadhyay et al., 2021). In heavy metals, some of the most common pollutants are zinc, nickel, lead, arsenic, chromium, and copper, which are present in excess in industrial effluents (Dognani et al., 2019). These metals are toxic to organisms and humans as well, even at some low concentrations (Li et al., 2019). Among heavy metals, copper occurs in industrial wastewater effluents. Some potential sources are pulp, paper, metal cleaning, metal plating baths, fertilizers, and the wood pulp industry (Li et al., 2019). Excessive copper can accumulate in the liver and is harmful to aquatic life at all concentrations in the aquatic ecosystem (Dognani et al., 2019).
Among heavy metals, lead is the most significant toxin as it affects the brain. Lead in inorganic compounds affects the central nervous system, causing irreversible brain damage (Piri et al., 2016). It also has adverse effects on the renal, gastrointestinal, reproductive, and peripheral nervous systems (Dognani et al., 2019). Sources of lead pollution in industries are finishing industries, metal plating, and battery manufacturing industries (Abdullah et al., 2019). According to the EPA (Environmental Protection Agency), the standard for lead in inland surface water has a concentration of 0.1 mg/L [I.S.I. specification (IS) is 10,500 according to a 1991 report] and for human use such as drinking, it is 0.05 mg/L (I.S.I. specification IA is 2,490 according to a 1982 report).
Nickel and copper ions are present in industrial wastewater, especially in industries dealing with mineral processing, porcelain enameling, battery manufacturing, electroplating, and copper sulfate manufacturing (Arsalan et al., 2020; Bhaumik et al., 2020). Excess amounts of nickel from the human health standard set by the US-EPA (MCLG 0.1 mg/L) may be the reason for serious health problems (Hong-Tao et al., 2019).
Various techniques have been used until now for the removal of heavy metals from wastewater and industrial effluents, which include chitosan, zeolites, clay, and peat moss (Eletta et al., 2021). Some studies include different husks for the removal of heavy metals, that is, coconut husk, peanut husk, and sawdust (Ghorbani et al., 2011). Some composites of husks and polyaniline have been studied for this purpose, that is, polyaniline-saw dust and its composite (Ghorbani et al., 2012; Abu Taleb et al., 2020). Polyaniline-based materials are effective for the removal of heavy metals as they are available in adequate quantity and show good results for the adsorption of heavy metals. The other polymers are not as effective when compared with polyaniline. Polyaniline has some benefits over other polymers because of its low cost, simple monomer with easy binding capacity, conducting ability, and removal of heavy metals. Polyaniline has attracted much attention due to its unique optical and electrical properties and its different potential applications. Furthermore, modified PANI composites have multifunctional groups (active sites) and show high adsorption capacities for different materials (De Souza et al., 2021). The modification of polyaniline with wheat husk to form a polyaniline-WH composite as an adsorbent is significant in increasing the adsorption due to more active sites and consequently increases the removal efficiency of different metal ions from solutions or water samples. The schematic diagram (Scheme 1) shows the preparation of polyaniline, the attachment of the composite, and later the adsorption of any of the heavy metals such as Cu, Ni, or Pb. Furthermore, the modified composite provides more surface area which enhances the adsorption capacity of the composite. The success of adsorption mainly depends on the selection of a suitable adsorbent or material with high adsorption affinity toward the target contaminant (metals) along with a rapid sorption rate. The modification of wheat husk with polyaniline enhanced its adsorption capacity toward heavy metals.
SCHEME 1. Schematic diagram of the mechanism (reaction) for the preparation of polyaniline, polyaniline-WH, and adsorption of heavy metals (Cu, Ni, and Pb) by using the polyaniline-WH composite.
The present research focuses on finding an easy and effective way (obtaining optimum conditions) by using PANI-WH (polyaniline wheat husk) as the absorbing material for the remediation of heavy metals (Pb, Ni, and Cu) from wastewater. The PANI-WH composite is an effective and low-cost absorbing material for the adsorption of heavy metals with high adsorption capacity.
All analytical grade reagents were used throughout the experiment without any further purification, except aniline, which was further purified by distillation. Deionized distilled water was used for preparing solutions. Stock solutions (1,000 mg/L) of Cu, Ni, and Pb were prepared by dissolving the calculated amount of Cu(SO4), NiCl2, and Pb(CH3COOH)2, respectively, in deionized distilled water. By adding 0.1 N HNO3 and 0.1 N NaOH, the pH was adjusted when required. Procedures such as SEM (scanning electron microscopy) (JSM-6480), and XPS were conducted by using PHI-5000 Versa Probe-III (ULVAC-PHI), XRD was conducted by D/MAX-3C (Japan), and a filtration apparatus (MDA-015), furnace (MHA-11), weighing balance (Armfield), and AAS (atomic absorption spectrometry) (Perkin Elmer Analyst 800 Series) were used for analysis.
For the removal of heavy metals such as Cu, Ni, and Pb from the aqueous system, polyaniline-coated wheat husk is used as an adsorbent. For the preparation of polyaniline and its composite with wheat husk, the procedure followed was as reported by M. S Mansour for the synthesis of polyaniline composite with sawdust (Dinari and Neamati 2020). The procedure involves dissolving 5 g aniline in 250 ml HCl (1N) and then obtaining a temperature below 5°C by placing the mixture in an ice bath, followed by the slow addition of pre-cooled, 250 ml of 0.3 N (NH4)S2O8 in HCl (1N) solution under vigorous stirring. The material was placed in an ice bath because of the highly exothermic reaction having ΔH = 372 kJ/mol energy value. After oxidation, this mixture was stirred at 0–5°C for 2 h and then kept unstirred overnight at room temperature. Precipitates of polyaniline were then cleaned and purified with distilled water. It was then diluted with HCl until the colorless filtrate was obtained. To remove nonpolymeric impurities and oligomers, polyaniline was washed with methanol and distilled water. It was finally dried in the furnace keeping the temperature 50–60°C and stored in powdered form for usage.
The composite of polyaniline with wheat husk was preferred to be synthesized via the cast method as it gives a more homogeneous and uniform coating than the direct synthesis method (Zia et al., 2020; Arsalan et al., 2021a). PANI was treated for 2–3 h with 0.5 M sodium hydroxide to make it soluble in formic acid. Impurities were removed by repeated washing and drying in the oven at 65°C. 0.5 g dissolved polyaniline was base-treated in HCOOH (formic acid) (50 ml) followed by filtration to remove any undissolved impurities. Sieved wheat husk (5 g) was stirred with a 50-ml emeraldine base for 2 h at 25°C and left unstirred for the next 2 h. It was then kept in a furnace at 65°C until the evaporation of excess water. This powdered composite was stored for further experiments.
For the investigation of heavy metal (Ni, Cu, and Pb) removal, batch mode studies were carried out. Except for thermodynamic studies, all the experimental work was carried out at room temperature. A total of 50 ml of the metal solution of the required concentration is stirred with 0.5 g of the PANI-WH composite with appropriate agitation speed (except for studies of the influence of composite dosage on heavy metal removal for which different dosage compositions of the composite were used). The solution was filtered after a specified time. The concentration of the metals was obtained by atomic absorption spectroscopy (ASS).
The adsorption of heavy metals is affected by different factors. Factors affecting the percentage removal were studied, which are as follows: mesh size of the sorbent (50 μM, 80 μM, and 100 µM), pH (varying from 2 to 10), metal concentration ranging from 10 mg/L to 50 mg/L, time factor varying from 20 min to 60 min, rpm (ranging from 200 rpm to 400 rpm), and the dose of the sorbent ranging from 0.1 g to 1 g. The removal efficiency was calculated using the following formula:
where C = concentration of metals after adsorption and Ci = initial metal concentration.
The Langmuir isotherm model works with an assumption that metals adsorbed in monolayer adsorption form on the homogeneous surface of the adsorbent, having no contact among adsorbed ions (Agarwal and Singh, 2016). The results collected from isotherm studies were shown in the Langmuir isotherm.
This Eq. 2 corresponds to the linear line equation
The result is expressed by the graph between Ce/qe Versus Ce to evaluate the value of qmax and b, where Ce (mg/L) represents the equilibrium concentration of the heavy metal (Cu, Ni, and Pb) ions. qe represents the adsorption capacity (mg g−1) and qmax represents the maximum uptake of metals, which tells about site saturation (Awais et al., 2021).
The Freundlich isotherm neither assumes a homogeneous surface nor does it assume constant sorption as it is more general than the Langmuir isotherm. Its equation represented as
where the solid-phase metal ion concentration equilibrium is represented by q (mg/L), liquid-phase metal ion concentration equilibrium is represented by Ce (mg/L), and the Freundlich isotherm constant is represented by Kf and n. These factors affect adsorption processes such as adsorption intensity and adsorption capacity.
The linear line equation for Temkin is
Here, the amount of heavy metal adsorbed is represented as “q” per unit weight of composite (adsorbent) mg g−1, Ce represents the concentration of metal ions adsorbed (m g−1) at equilibrium, and “a” gives the equilibrium binding constant for the Temkin isotherm (L g−1) (Karthik and Meenakshi 2015; Arsalan et al., 2021b).
Thermodynamics of Cu, Ni, and Pb were studied by varying the temperature from 293 to 313 K (20–30°C).
Enthalpy of the reaction was found out by using the following formula:
The ΔS entropy of the reaction was determined by using the following formula:
The surface morphology of the novel PANI-WH composite was observed through scanning electron microscopic (SEM) images of the wheat husk and its composite before and after adsorption as shown in Figure 1. SEM images of the wheat husk and its composite (wheat husk and polyaniline) can be seen in Figures 1A,B. After the coating of polyaniline on the wheat husk, the surface has become coarse (Ghorbani et al., 2012).
FIGURE 1. (A)SEM images of wheat husk, (B) composite of the wheat husk and polyaniline, (C) composite after Cu adsorption, (D) composite after Pb adsorption, and (E) composite after Ni adsorption showing the size enhancement after heavy metal adsorption.
It was observed that the coating is visible in Figures 1C–E, and after the adsorption of heavy metals Pb, Ni, and Cu on the surface of the composite, the particle size has been increased, which clearly implies the adsorption of metals, and the surface has become rougher after adsorption. Scheme 2 shows the preparation, characterization, and adsorption of heavy metals by the PANI-WH composite.
SCHEME 2. Schematic diagram of the preparation, characterization, and adsorption of heavy metals by using the PANI-WH composite.
The Fourier transform infrared (FT-IR) spectra of polyaniline are shown in Supplementary Figure S1. The FT-IR peaks of the wheat husk and polyaniline are shown in Supplementary Tables S1, S2 (supplementary information tables 1 and 2), respectively, and compared with the existing literature (Arsalan et al., 2019), while the peak shifts of the composite before and after the adsorption of metals are shown in Table 1. All bands of the FT-IR spectra in a composite are seen to be shifted corresponding to all peaks. The interaction between the wheat husk and polyaniline was confirmed by literature (Ghorbani et al., 2012). The = NH stretching band peak corresponds with a peak at 3,170 cm−1 of the composite showing outstanding adsorption of the metals. It was observed that after adsorption, the metals have some interactions with the = NH bond on the polyaniline coating.
TABLE 1. FT-IR spectral peaks of the PANI-WH composite before and after the adsorption of Ni, Pb, and Cu.
The XRD spectra for wheat husk, PANI, and PANI-WH are compared and presented in Figure 2. The XRD spectra show the relevant peaks of the wheat husk at the 110 plane, which are mentioned in Figure 2 and its related literature (Patil et al., 2018), where the XRD pattern of PANI shows the peaks at (011), (020), and (200) planes, which are characteristics of the emeraldine salt phase of PANI (Patil et al., 2018). The XRD pattern of the PANI-WH shows the hydrocalcite structure of the material and shows peaks at (011), (020), (200), and (110) planes, comprising the good morphology of the composite.
The comparison of the PANI-WH before and after Pb, Ni, and Cu adsorption was examined by X-ray photoelectron spectroscopy (Patil et al., 2018) and is shown in Figure 3A. It was observed that the XPS scan of the composite shows the presence of carbon (C), nitrogen (N), and oxygen (O). The presence of the C peak at 312 eV, N peaks at 365 eV and 372 eV, and O peak at 559 eV shows the formation of the polyaniline composite (Patil et al., 2018). The results show the existence of both PANI and WH as components in the synthesized composite structure. The deconvoluted N 1s spectrum as expressed shows that two binding peaks at 365 eV and 372 eV are attributed to amine (─NH─) of benzenoid and imine (─NH+═) units. It can be seen from the figure that before metal adsorption, the PANI-WH does not show any peaks for Pb, Ni, and Cu, but after the adsorption of Pb, Ni, and Cu, their respective peaks appeared. The magnified spectra of the PANi-WH composite after Pb adsorption are shown in Figure 3B, having a pair of main peaks at 137.36 eV and 144.7 eV (Piri et al., 2016). The PANI-WH composite was also used for the adsorption of Ni metal as shown in Figure 3C, having a pair of main and satellite peaks. The main peaks of the Ni metal were observed at 855.6 eV (Ni 2p3/2) and 873.2 eV (Ni 2p1/2) (Bhaumik et al., 2020). The main peaks also have some satellite peaks at 861.3 and 880.2 eV (Bhaumik et al., 2020). Figure 3D shows the magnified spectra of the composite after Cu adsorption, having a pair of peaks at 932.2 and 947.8 eV and a pair of satellite peaks at 938.1 and 954.2 eV (Abu Taleb et al., 2020).
FIGURE 3. (A) Comparison of XPS spectra of PANI-WH: (a) after Pb adsorption, (b) after Ni adsorption, and (c) after Cu adsorption (d) (B) Magnified spectra of the PANI-WH after Pb adsorption, (C) magnified spectra of the PANI-WH after Ni adsorption, and (D) magnified spectra of the PANI-WH after Cu adsorption.
The PANI-WH composite particle size effect was demonstrated by passing the particles of the composite from different mesh sizes of the sieve. The mesh sizes used for this experiment were 50, 80, and 100 (particle size: 300 μM, 180 μM, and 150 µM, respectively) as shown in Figure 4. A total of 20 mg/L of the metal’s concentration was used with a composite dose of 0.5 g. At keeping pH 6, 7, and 5 of the aqueous solution for Cu, Ni, and Pb, respectively, the agitation speed was kept at 200 rpm for 30 min. The results obtained clearly depicted that 180 µM of the particle size gave maximum adsorption because the small size provides greater surface area for the adsorption; further decrease in the particle size did not have enough sites to adsorb the metal ions completely which lowers the percentage removal; hence, maximum removal efficiency was obtained by having a mesh size of 80 (Dinari and Neamati 2020). The surface area provides more active sites for metal particle adsorption (Ighalo and Eletta 2020). The particle size of 180 µM shows maximum adsorption because of maximum surface area at optimized conditions.
FIGURE 4. Effect of mesh size on percentage removal of Cu2+, Ni2+, and Pb2+ using the PANI-WH composite.
In heavy metals, adsorption pH plays a very important role in the sorbent surface. A change in pH affects the adsorbent surface charge and the ionization of functional groups present on the sorbent surface and sometimes replaces some of the positive ions. It also changes the chemical speciation of metal ions. On the other hand, H+ ions also act as strong competing ions (Dinari and Neamati 2020).
The effect of pH on the adsorption of heavy metals such as Pb, Cu, and Ni on the surface of the PANI-WH composite was investigated. The research was carried out at varying pH values, that is, 2, 3, 4, 5, 6, 7, 8, 9, and 10. The active sites on the polyaniline are N–H groups because of the lone pair present in nitrogen. At low pH, there exists a competition of H+ with metal cations for the active site, causing a reduction in adsorption. The adsorption of metals increases with the increase in pH. Metals get precipitated at higher basic pH. So, pH should be selected carefully.
It may seem apparent from the graph in Figure 5A that the optimized pH for the adsorption of copper from the PANI-WH composite is 9, but Cu gets precipitated at a pH above 6.3 (Pakdel Mojdehi et al., 2019). So, the removal of copper at pH greater than 9 is due to precipitation and not because of the sorbent, so the optimum pH for copper removal was observed at pH 6.
FIGURE 5. (A) Percentage removal efficiency of Cu, Pb, and Ni at different pH values. (B) Percentage removal efficiency of Cu, Pb, and Ni at different initial metal ion concentrations. (C) Percentage removal efficiency of Cu, Pb, and Ni at different time intervals. (D) Percentage removal efficiency of Cu, Pb, and Ni at varying agitation speeds.
The graph shows the maximum adsorption of Ni at pH 9, but above pH 7.4, the precipitates of Ni are more thermodynamically stable (Espinoza et al., 2012; Sun et al., 2020); hence, the best pH for the adsorption of Ni from the PANI-WH composite is considered to be 7. For Pb, maximum removal was obtained at pH 8, but Pb gets precipitated above pH 5 (Espinoza et al., 2012; Afshar et al., 2016), so pH 5 was taken as the optimum pH for other experiments. By repeating the experiments, maximum adsorption was obtained by using the PANI-WH composite, for Ni 155 mg g−1 and for Cu and Pb 95 mg g−1 and 110 mg g−1, respectively, under optimized conditions.
The stability and size of the composite are very important factors, which directly affect their applicability. These can be easily controlled by optimizing the amount, concentration, and pH of the composite solution as mentioned in the literature (Bhaumik et al., 2015). The stability of the composite was evaluated by zeta potential analysis. To analyze the initial pH of the PANI-WH composite, zeta potential analysis was conducted after regular intervals of time using the same sample. The effect of the initial solution pH on zeta potential values is given in Supplementary Figure S2, with a maximum zeta potential value of 38 mV. The increase in pH enhances the zeta potential which shows its stability and later after a pH 7, the zeta potential value decreases. The composite shows a constant zeta potential value, which shows good stability of the composite under optimized conditions.
As the contact time of metals with a sorbent plays a very significant role in adsorption, the time effect on the removal efficiency was observed for Cu, Pb, and Ni, keeping the optimum pH and optimum metal concentrations (30 mg/L, 40 mg/L, and 20 mg/L for Pb, Cu, and Ni, respectively). The composite was agitated at different time intervals from 20 to 60 min. It is evident from the graph in Figure 5C that equilibrium was achieved after 40, 50, and 40 min of agitation for Cu, Ni, and Pb, respectively. After equilibrium was attained, a further increase in removal efficiency was not observed because of the fixed amount of the metal concentration and composite, which implied that a certain time period was needed for the metals to be adsorbed on the sorbent surface (Ghorbani et al., 2012). From Figure 5B, it can be easily concluded that the metal ion concentration also plays an important role in removal efficiency. It can be seen from Figure 5B that at higher concentration, removal efficiency increases at certain levels by using the PANI-WH composite.
Metal solutions of optimized concentration at optimized pH were investigated to examine the agitation speed effect on the adsorption of metals. Keeping the time periods optimum, the metals were agitated in a magnetic stirrer ranging from 100 rpm to 400 rpm as shown in Figure 5D.
The optimum agitation speed for Cu and Ni was found to be 400 rpm, whereas for Pb, it was 200 rpm above which adsorption of metals would decrease. This over-agitation may cause the repulsive interactions to dominate the attractive ones on sorbent sites, resulting in the reduction of attractive forces between the sorbent and sorbate (Olad et al., 2019). Significant adsorption seems possible even at simple shaking.
Keeping all the aforementioned conditions fixed, including pH, metal concentration, time, and agitation speed optimum, the sorbent dose effect was observed by changing the sorbent dose from 0.1 g to 1 g. For Cu, it was found to be 0.3 g, for Ni 0.7 g, and for Pb, the maximum adsorption was observed at 0.5 g. The metal adsorption at a specific metal concentration (optimum) boosted with the increase in the dose of the sorbent. But after a specific dose, no significant increase in adsorption was found as shown in (Figure 6A).
FIGURE 6. (A) Removal efficiency of Cu, Pb, and Ni by taking a different amount of composite. (B) Langmuir isotherms for Cu and Ni. (C) Freundlich isotherm for Pb, Cu, and Ni. (D) Temkin isotherm for Cu, Ni, and Pb.
The adsorption isotherms for Cu, Ni, and Pb were carried out to observe the functional relationship between the amount of these metals present in bulk and the number of metals adsorbed. The concentrations of metals ranging from 10 mg/L to 50 mg/L were examined to study the Freundlich, Langmuir, Temkin, and Harkins–Jura isotherms. All three metals under investigation followed Freundlich and Temkin isotherms, while Cu and Ni followed the Langmuir isotherm. No metals followed the Harkins–Jura isotherm as their r2 values were between 0.3 and 0.6 for these metals.
These results may be anticipated with the fact that by increasing the sorbent dosage, there are increased active sites, providing more surface area for the metals to be adsorbed. For the specific metal concentration, at the optimum dose, the active sites complex all the metal atoms present in the solution, and no further enhanced adsorption is examined on further increasing the dose (Dognani et al., 2019; Sahu et al., 2019).
The Langmuir constant (Eq. 2) “b” shows an affinity between metal ions and the PANI-WH quantitatively. The data provide a linear plot if it conforms to the Langmuir isotherm (Elmi et al., 2017). Figure 6B shows that the adsorption of the aforementioned metals on the PANI-WH composite follows the Langmuir isotherm, whereas adsorption of Pb on the composite does not follow the Langmuir isotherm.
A graphical representation of the Freundlich isotherm is shown in Figure 6C (Dada et al., 2012). It is clear from Figure 6D that all three metals also follow the Temkin isotherm model.
Considering the regression data shown in Table 2, it is evident that for copper, the maximum value for R2 (0.98) is obtained in the Langmuir isotherm, which shows that for the adsorption of Cu on the PANI-WH composite, the best-fitted isotherm model is the Langmuir isotherm. But for Ni, the R2 has a maximum value (0.97) when the data were plotted for the Temkin isotherm model. The Langmuir isotherm for Pb adsorption was not observed, and the highest value of R2 (0.99) was obtained for the Temkin isotherm. For the Langmuir isotherm, along with R2, another important factor RL was also determined by the given formula
where b is the Langmuir constant, Co is the initial metal ion concentration (mg g−1), and RL is a dimensionless separation factor, a characteristic of the Langmuir isotherm used to define the affinity between the adsorbent and absorbate. The isotherm is irreversible if RL = 0, favorable if RL value is between 0 and 1, linear if RL = 1, and unfavorable if RL >1.
TABLE 2. Adsorption of Cu, Ni, and Pb on the PANI-WH composite by Langmuir, Freundlich, and Temkin isotherms.
The aforementioned data from Table 2 revealed that the values of RL are less than 1 and greater than 0, which means the isotherm is favorable for Cu and Ni metal ions.
Considering the Freundlich data, it is proven that the reaction/isotherm is favorable as the values of n are greater than 1 (Abu Taleb et al., 2020). The factor k of the Freundlich isotherm further confirms the favorability of metals ion concentration on the adsorbent.
Kinetics of all three metals under study was investigated, varying the time of agitation from 20 to 60 min, keeping all optimized factors constant.
The data were transferred into the pseudo-first and second-order kinetics. But, all three metals followed the pseudo-second-order kinetics for which the contact time was taken at the abscissa and t/qt was taken at the ordinate, as shown in Figure 7A. The following equation was used to transfer the data in pseudo-second-order kinetics.
where qt represents the amount of metals adsorbed (mg g−1) at time (t), the pseudo-second-order rate constant is represented by K2 (g mol−1 min−1), and qe represents the amount of metals adsorbed at equilibrium (mg g−1). Plotting the graph by taking time (t) on the x-axis and t/qt on the y-axis gives a straight line, with R2 values ranging from 0.92 to 0.99 for the three metals under study, which confirms that the adsorption of metals on this composite follows the pseudo-second-order kinetics. K2 and qe are the coefficient correlations, shown in Table 3.
FIGURE 7. (A) Adsorption kinetics of Cu, Ni, and Pb on the PANI-WH composite and (B) adsorption thermodynamics of Cu, Pb, and Ni on the PANI-WH composite.
The thermodynamics of Cu, Ni, and Pb were studied by varying the temperature from 293–313 K (20–30°C).
The value of dH and TdS (Eqs 8–10) can be observed by the intercept and slope of the graph, respectively, as shown in Figure 7B (Moazezi et al., 2018).
Table 4 shows that ΔG values are negative for all three metals. The negative value of ΔG shows the feasibility of the reaction, that is, the reaction is spontaneous in nature. As for the three metals, the values of ΔG decrease as the temperature increases, showing that the spontaneity of the process is decreased as the temperature increases.
The positive values of ΔH for the three metals clearly demonstrate that the adsorption process is endothermic in nature, which means an increase in the temperature causes enhanced adsorption of metals on the PANI-WH composite. The comparison of the prepared material (polyaniline-WH), wheat husk in pure form and previously reported materials is shown in Table 5. It can be seen from the table that wheat husk in pure form has some adsorption capacity under the same conditions, but with the addition of polyaniline, its adsorption capacity is enhanced.
Another important thermodynamic parameter studied was entropy. As the entropy of the system is a measure of the randomness of the system, from Table 4, it can be deduced that the entropy for this system increased after the adsorption, which is due to the increase in randomness at the interface of the adsorbent and aqueous solution. The increase in adsorption at higher temperatures causes enhancement in pore size. The adsorbate species displace the adsorbed water molecules, which in turn gain more energy (translational) when compared with the energy loss due to adsorbate ions, therefore allowing the enhanced randomness in the system.
A novel composite PANI-WH was prepared to investigate the adsorption properties of heavy metals, Cu, Ni, and Pb. SEM, XRD, XPS, and FT-IR techniques were performed to study the morphology and interactions of the composite with the metals. Optimum conditions for different parameters such as contact time, pH, adsorbent dose, metal concentration, and agitation speed were obtained to investigate the adsorption capacity of heavy metals. The adsorption of heavy metals mainly depends on the composite-optimized condition, where it shows the maximum adsorption. The results of all optimized conditions were explained in detail. Data were incorporated in kinetics studies and isotherm models. The data were best represented by pseudo-second-order kinetics. Experimental results show that the PANI-WH composite has an excellent absorbing capacity for all three metals having a binding capacity of 155 mg g−1 for Ni, 95 mg g−1 for Cu, and 110 mg g−1 for Pb. Percentage removal efficiency has proven to be increased by using the PANI-WH composite for heavy metals Ni (97%), Cu (92%), and Pb (99%) under optimum conditions. Thermodynamics studies showed the adsorption process to be endothermic in nature, which confirms its feasibility in nature, and it can be further used in the future for the adsorption of other heavy metals.
The raw data supporting the conclusions of this article will be made available by the authors, without undue reservation.
Conceptualization, MA, IS, and AA; methodology, IS, IK, MB, and AM; writing—original draft preparation, MA and AA; writing—review and editing, IS and MB; funding acquisition, IK, MB, and AM; software, AM, MB, and MB; All authors have read and agreed to the published version of the manuscript.
The authors declare that the research was conducted in the absence of any commercial or financial relationships that could be construed as a potential conflict of interest.
All claims expressed in this article are solely those of the authors and do not necessarily represent those of their affiliated organizations, or those of the publisher, the editors, and the reviewers. Any product that may be evaluated in this article, or claim that may be made by its manufacturer, is not guaranteed or endorsed by the publisher.
The Supplementary Material for this article can be found online at: https://www.frontiersin.org/articles/10.3389/fenvs.2022.895463/full#supplementary-material
Abdullah, N., Yusof, N., Lau, W. J., Jaafar, J., and Ismail, A. F. (2019). Recent Trends of Heavy Metal Removal from Water/wastewater by Membrane Technologies. J. Industrial Eng. Chem. 76, 17–38. doi:10.1016/j.jiec.2019.03.029
Abu Taleb, M., Kumar, R., Al-Rashdi, A. A., Seliem, M. K., and Barakat, M. A. (2020). Fabrication of SiO2/CuFe2O4/polyaniline Composite: A Highly Efficient Adsorbent for Heavy Metals Removal from Aquatic Environment. Arabian J. Chem. 13 (10), 7533–7543. doi:10.1016/j.arabjc.2020.08.028
Afshar, A., Sadjadi, S. A. S., Mollahosseini, A., and Eskandarian, M. R. (2016). Polypyrrole-polyaniline/Fe3O4 Magnetic Nanocomposite for the Removal of Pb(II) from Aqueous Solution. Korean J. Chem. Eng. 33 (2), 669–677. doi:10.1007/s11814-015-0156-1
Agarwal, M., and Singh, K. (2016). Heavy Metal Removal from Waste Water Using Various Adsorbents: A Review. J. Water Reuse Desalination 7 (4), 387–419. doi:10.2166/wrd.2016.104
Arsalan, M., Awais, A., Chen, T., Sheng, Q., and Zheng, J. (2019). Development of PANI/BN-based Absorbents for Water Remediation. Water Qual. Res. J. 54 (4), 290–298. doi:10.2166/wqrj.2019.048
Arsalan, M., Awais, A., Qiao, X., Sheng, Q., and Zheng, J. (2020). Preparation and Comparison of Colloid Based Ni50Co50(OH)2/BOX Electrocatalyst for Catalysis and High Performance Nonenzymatic Glucose Sensor. Microchem. J. 159, 105486. doi:10.1016/j.microc.2020.105486
Arsalan, M., Babar, N.-U. -A., Sadiqa, A., Mansha, S., Baig, N., Nisar, L., et al. (2021a). Surface-assembled Fe-Oxide Colloidal Nanoparticles for High Performance Electrocatalytic Water Oxidation. Int. J. Hydrogen Energy 46 (7), 5207–5222. doi:10.1016/j.ijhydene.2020.11.035
Arsalan, M., Qiao, X., Awais, A., Wang, Y., Yang, S., Sheng, Q., et al. (2021b). Enhanced Sensitive Electrochemical Sensor for Simultaneous Catechol and Hydroquinone Detection by Using Ultrasmall Ternary Pt‐based Nanomaterial. Electroanalysis 33, 1528–1538. doi:10.1002/elan.202100026
Awais, A., Arsalan, M., Qiao, X., Yahui, W., Sheng, Q., Yue, T., et al. (2021). Facial Synthesis of Highly Efficient Non-enzymatic Glucose Sensor Based on Vertically Aligned Au-ZnO NRs. J. Electroanal. Chem. 895, 115424. doi:10.1016/j.jelechem.2021.115424
Bhaumik, M., Noubactep, C., Gupta, V. K., McCrindle, R. I., and Maity, A. (2015). Polyaniline/Fe0 Composite Nanofibers: An Excellent Adsorbent for the Removal of Arsenic from Aqueous Solutions. Chem. Eng. J. 271, 135–146. doi:10.1016/j.cej.2015.02.079
Bhaumik, M., Maity, A., and Brink, H. G. (2020). Zero Valent Nickel Nanoparticles Decorated Polyaniline Nanotubes for the Efficient Removal of Pb(II) from Aqueous Solution: Synthesis, Characterization and Mechanism Investigation. Chem. Eng. J. 417, 127910. doi:10.1016/j.cej.2020.127910
Dada, A. O., Olalekan, A. P., Olatunya, A. M., and Dada, O. (2012). Langmuir, Freundlich, Temkin and Dubinin–Radushkevich Isotherms Studies of Equilibrium Sorption of Zn2+ unto Phosphoric Acid Modified Rice Husk. IOSR J. Appl. Chem. 3 (1), 38–45. doi:10.9790/5736-0313845
De Souza, E. C., Pimenta, A. S., da Silva, A. J. F., do Nascimento, P. F. P., and Ighalo, J. O. (2021). Oxidized eucalyptus Charcoal: A Renewable Biosorbent for Removing Heavy Metals from Aqueous Solutions. Biomass Convers. Biorefinery, 1–15. doi:10.1007/s13399-021-01431-y
Dinari, M., and Neamati, S. (2020). Surface Modified Layered Double Hydroxide/polyaniline Nanocomposites: Synthesis, Characterization and Pb2+ Removal. Colloids Surfaces A Physicochem. Eng. Aspects 589, 124438. doi:10.1016/j.colsurfa.2020.124438
Dognani, G., Hadi, P., Ma, H., Cabrera, F. C., Job, A. E., Agostini, D. L. S., et al. (2019). Effective Chromium Removal from Water by Polyaniline-Coated Electrospun Adsorbent Membrane. Chem. Eng. J. 372, 341–351. doi:10.1016/j.cej.2019.04.154
Eletta, O. A., Ayandele, F. O., and Ighalo, J. O. (2021). Adsorption of Pb (II) and Fe (II) by Mesoporous Composite Activated Carbon from Tithonia Diversifolia Stalk and Theobroma Cacao Pod. Biomass Convers. Biorefinery, 1–10. doi:10.1007/s13399-021-01699-0
Elmi, F., Hosseini, T., Taleshi, M. S., and Taleshi, F. (2017). Kinetic and Thermodynamic Investigation into the Lead Adsorption Process from Wastewater through Magnetic Nanocomposite Fe3O4/CNT. Nanotechnol. Environ. Eng. 2 (1), 13. doi:10.1007/s41204-017-0023-x
Espinoza, E., Escudero, R., and Tavera, F. J. (2012). Waste Water Treatment by Precipitating Copper, Lead and Nickel Species. Res. J. Recent Sci. 1, 1–6.
Ghorbani, M., Eisazadeh, H., and Ghoreyshi, A. A. (2012). Removal of Zinc Ions from Aqueous Solution Using Polyaniline Nanocomposite Coated on Rice Husk. Iran. J. Energy Environ. 3 (1), 83–88. doi:10.5829/idosi.ijee.2012.03.01.3343
Ghorbani, M., Lashkenari, M. S., and Eisazadeh, H. (2011). Application of Polyaniline Nanocomposite Coated on Rice Husk Ash for Removal of Hg (II) from Aqueous Media. Synth. Met. 161 (13-14), 1430–1433. doi:10.1016/j.synthmet.2011.05.016
Hong-Tao, Z. H. U., Jia-Qi, W., Xing, L. I., and Xiang-Xue, W. (2019). Recent Advances in Carbon Nitride-Based Nanomaterials for the Removal of Heavy Metal Ions from Aqueous Solution. J. Inorg. Mater. 35 (3), 260–270. doi:10.15541/jim20190436
Ighalo, J. O., and Eletta, O. A. (2020). Response Surface Modelling of the Biosorption of Zn (II) and Pb (II) onto Micropogonias undulatus Scales: Box–Behnken Experimental Approach. Appl. Water Sci. 10 (8), 1–12. doi:10.1007/s13201-020-01283-3
Karthik, R., and Meenakshi, S. (2015). Removal of Pb(II) and Cd(II) Ions from Aqueous Solution Using Polyaniline Grafted Chitosan. Chem. Eng. J. 263, 168–177. doi:10.1016/j.cej.2014.11.015
Li, T., Qin, Z., Shen, Y., Xu, X., Liu, N., and Zhang, Y. (2019). Polyaniline Nanofibers Formed by Seed Polymerization through Heat Treatment for Synergistic Enhancement of Cr(VI) Removal. Mater. Lett. 252, 130–133. doi:10.1016/j.matlet.2019.05.128
Moazezi, N., Baghdadi, M., Hickner, M. A., and Moosavian, M. A. (2018). Modeling and Experimental Evaluation of Ni(II) and Pb(II) Sorption from Aqueous Solutions Using a Polyaniline/CoFeC6N6 Nanocomposite. J. Chem. Eng. Data 63 (3), 741–750. doi:10.1021/acs.jced.7b00897
Olad, A., Bastanian, M., and Bakht Khosh Hagh, H. (2019). Thermodynamic and Kinetic Studies of Removal Process of Hexavalent Chromium Ions from Water by Using Bio-Conducting Starch-Montmorillonite/Polyaniline Nanocomposite. J. Inorg. Organomet. Polym. 29 (6), 1916–1926. doi:10.1007/s10904-019-01152-w
Pakdel Mojdehi, A., Pourafshari Chenar, M., Namvar-Mahboub, M., and Eftekhari, M. (2019). Development of PES/polyaniline-modified TiO2 Adsorptive Membrane for Copper Removal. Colloids Surfaces A Physicochem. Eng. Aspects 583, 123931. doi:10.1016/j.colsurfa.2019.123931
Patil, S. H., Gaikwad, A. P., Sathaye, S. D., and Patil, K. R. (2018). To Form Layer by Layer Composite Film in View of its Application as Supercapacitor Electrode by Exploiting the Techniques of Thin Films Formation Just Around the Corner. Electrochimica Acta 265, 556–568. doi:10.1016/j.electacta.2018.01.165
Piri, S., Zanjani, Z. A., Piri, F., Zamani, A., Yaftian, M., and Davari, M. (2016). Potential of Polyaniline Modified Clay Nanocomposite as a Selective Decontamination Adsorbent for Pb(II) Ions from Contaminated Waters; Kinetics and Thermodynamic Study. J. Environ. Health Sci. Eng. 14 (1), 20. doi:10.1186/s40201-016-0261-z
Sahu, S., Sahu, U. K., and Patel, R. K. (2019). Modified Thorium Oxide Polyaniline Core-Shell Nanocomposite and its Application for the Efficient Removal of Cr(VI). J. Chem. Eng. Data 64 (3), 1294–1304. doi:10.1021/acs.jced.8b01225
Sun, J., Wang, L., Yang, Q., Shen, Y., and Zhang, X. (2020). Preparation of Copper-Cobalt-Nickel Ferrite/graphene Oxide/polyaniline Composite and its Applications in Microwave Absorption Coating. Prog. Org. Coatings 141, 105552. doi:10.1016/j.porgcoat.2020.105552
Upadhyay, U., Sreedhar, I., Singh, S. A., Patel, C. M., and Anitha, K. L. (2021). Recent Advances in Heavy Metal Removal by Chitosan Based Adsorbents. Carbohydr. Polym. 251, 117000. doi:10.1016/j.carbpol.2020.117000
Keywords: PANI-WH composite, heavy metals, wastewater, thermodynamics, adsorption, remediation
Citation: Arsalan M, Siddique I, Awais A, Baoji M, Khan I, Badran M and Mousa AAA (2022) Highly Efficient PANI-WH Novel Composite for Remediation of Ni(II), Pb(II), and Cu(II) From Wastewater. Front. Environ. Sci. 10:895463. doi: 10.3389/fenvs.2022.895463
Received: 15 March 2022; Accepted: 22 April 2022;
Published: 01 June 2022.
Edited by:
Xiaofei Tan, Hunan University, ChinaReviewed by:
Yaocheng Deng, Hunan Agricultural University, ChinaCopyright © 2022 Arsalan, Siddique, Awais, Baoji, Khan, Badran and Mousa. This is an open-access article distributed under the terms of the Creative Commons Attribution License (CC BY). The use, distribution or reproduction in other forums is permitted, provided the original author(s) and the copyright owner(s) are credited and that the original publication in this journal is cited, in accordance with accepted academic practice. No use, distribution or reproduction is permitted which does not comply with these terms.
*Correspondence: Imran Siddique, aW1yYW5zbXNyemlAZ21haWwuY29t; Miao Baoji, bWlhb2Jhb2ppQGZveG1haWwuY29t
Disclaimer: All claims expressed in this article are solely those of the authors and do not necessarily represent those of their affiliated organizations, or those of the publisher, the editors and the reviewers. Any product that may be evaluated in this article or claim that may be made by its manufacturer is not guaranteed or endorsed by the publisher.
Research integrity at Frontiers
Learn more about the work of our research integrity team to safeguard the quality of each article we publish.