- 1Laboratory of Microbial Biotechnologies, Agro-Sciences and Environment (BioMAgE), Labeled Research Unit-CNRST N◦4, Cadi Ayyad University, Marrakesh, Morocco
- 2Department of Food Science, Faculty of Agricultural and Food Sciences, Laval University, Quebec City, QC, Canada
- 3Botany and Microbiology Department, College of Science, King Saud University, Riyadh, Saudi Arabia
- 4Department of Biological Sciences, Faculty of Science, Kuwait University, Safat, Kuwait
The present study aims to evaluate the effects of the exotic shrub Acacia cyanophylla Lindl. on soil fertility by studying 1) its ability to modify the soil physicochemical composition, 2) its contribution to the soil mycorrhizal potential and its impact on the richness and diversity of the arbuscular mycorrhizal fungi (AMF) community in the rhizospheric soil (RS), and finally 3) its atmospheric nitrogen fixation potential. The physicochemical analysis of the RS has shown that soil invasion by A. cyanophylla has a beneficial effect on its fertility; this advantage is demonstrated by the increase of the organic matter and the nutrient contents (N, P, K, Na, Ca) in the RS. Furthermore, the roots of this shrub exhibited broad AMF colonization, which confirms its high mycotrophic aspect. Four differentiated morphotypes of mycorrhizal spores were isolated from the RS of A. cyanophylla by use of the wet sieving method. In addition, the most probable number method showed that A. cyanophylla was capable of dramatically increasing the mycorrhizal potential of the soil. Indeed, more than 1,213 infectious propagules per one hundred grams of soil were detected in the RS of A. cyanophylla. Moreover, A. cyanophylla roots showed a significant presence of nodules indicating an active atmospheric nitrogen fixation. Counting revealed the presence of at least 130 nodules in the root fragments contained in 1 kg of soil. In conclusion, the biological invasion of sand dunes by the exotic shrub A. cyanophylla exhibited beneficial effects on the soil’s chemical composition and functioning, the activity of rhizobacteria in fixing atmospheric nitrogen, and phosphate bioavailability under the action of the native AMF community.
Introduction
A plant species is said to be “invasive” when its proliferation in natural environments induces significant changes in the composition, structure, and functioning of ecosystems. These species are categorized by their ability to adapt to a wide range of environmental conditions and resist disturbances (Blackburn et al., 2011; Sfairi et al., 2012; Baumel et al., 2018). In Morocco, several phyllode plant species native to Australia have been introduced to enrich the local flora, stabilize soils, constitute green belts and produce fuelwood and fodder (Lahdachi et al., 2015). Acacia cyclops, Acacia mollissima and Acacia cyanophylla are among the most widely used species (Benbrahim et al., 2014). A. cyanophylla is used almost everywhere for reforestation, ornamentation, production of soil tannins and coastal dune fixation (Marzialetti et al., 2019). Its tolerance to cold, drought, salinity and fires has enabled it to be classified among the most forest species used in the reforestation of arid and semi-arid zones (Derkaoui et al., 2016; Kheloufi et al., 2019). In addition, this hardy competitive legume species is nodulating with a vast number of Rhizobium strains, fixing atmospheric nitrogen (Amrani et al., 2010; Pathak et al., 2017). Furthermore, the species can establish a symbiotic partnership with many soil arbuscular mycorrhizal fungi (AMF). These fungal symbionts are the most widespread on the surface of the globe and adapted to many environments and different host plants (Vaishnav et al., 2017; Jacobs et al., 2020); they can form mutualistic associations with the roots of about 80% of terrestrial plants (Smith and read 2008). Golden wreath wattle (A. cyanophylla Lindl or A. saligna Labill) is a species native to the temperate region of southwestern Australia, where it has a naturally widespread distribution (Millar and Byrne, 2012) in various habitats, especially on soils with a high proportion of sand, such as dunes, sandy plains, or rocky ridges where it forms open forests (Griffin et al., 2011). Regarding climatic requirements, A. cyanophylla grows under average temperatures close to 13 °C in winter and 30°C in summer (Thompson et al., 2015). This shrub prefers sandy soils receiving more than 250 mm annual rainfall (El-Euch, 2000; Derbel et al., 2009). Outside of its natural range, A. cyanophylla has become an invasive species due to several factors, including its ability to regenerate after cutting or burning, its rapid growth in low-nutrient soils, its ability to fix nitrogen and its early reproductive maturity, and finally, the seeds’ ability to survive in fire (St-Denis et al., 2017; Badalamenti et al., 2018). A. cyanophylla presents direct socio-economic interests by the production of wood and edible seeds (Le Maitre et al., 2011); this shrub is also a source of high-quality fodder and complementary food for livestock due to its richness in proteins (Ee and Yates, 2013; Gebreyohaness, 2016). Regarding the ecological interests of A. cyanophylla, this species contributes to the softening of the climate of arid and semi-arid zones and ensures the fixation of sandy and coastal dunes. The lateral root system favors better maintenance of the cohesion of the soil particles (Boukhatem et al., 2012; Martínez et al., 2013; Lozano et al., 2020).
In addition, this shrub exhibits an arbuscular mycorrhizal symbiotic association receptiveness, particularly in phosphorus-deficient soils (Belay et al., 2013; Ilahi et al., 2021). The mycorrhizal fungi symbiosis is a mutualistic collaboration between a soil fungus and the roots of a host plant. This symbiosis is established by the development of a network of hyphae that can reach up to 1 km per 1 m of the root system (Fortin et al., 2015). The fungus removes sugars from the plant while the plant receives minerals and water from the fungus (Jadrane et al., 2021). Moreover, without this association, the AMF cannot complete its life cycle (Barea et al., 2005; Ouahmane et al., 2007b; He and Nara, 2007). The AMF develop long extra-root hyphae that allow the plant to exploit a large soil area. They can therefore have access to additional resources of water and mineral elements, which are then transmitted to the host plant through the roots (Smith and read 2008; Bouskout et al., 2022). Mycorrhizal plants receive phosphate from AMF and this most often leads to an increase in biomass compared to non-colonized plants (Balzergue et al., 2013). This difference can be explained by several mechanisms, among others, the expansion of the plant’s root absorption surface thanks to the extra-root network (Duponnois et al., 2009) and consequently, better exploitation of the phosphate of the soil beyond the zone of exhaustion of the root (Tisdall, 1991; Wahid et al., 2020). Additionally, the AMF hyphae possess phosphatases that promote the release of immobile phosphorus in the soil and allow the mineralization of organic sources of phosphates (Wahid et al., 2020). The phosphorus thus mobilized becomes available in the soil. On the other hand, several studies have reported an improvement in nitrogen nutrition provided by AMF, which has the necessary enzymatic equipment for the use of ammonium and nitrates (Nakmee et al., 2016). So, mycorrhizae play an important role in the plant’s uptake of certain forms of nitrogen (Beltrano et al., 2013). According to better use of the soil by the extra-root network, AMF increases the absorption of other mineral elements such as K, Ca, Mg and certain trace elements such as Zn, S and Cu (Abbaspour et al., 2012; Lehmann et al., 2014). AMF also have direct effects on soil quality, the extra-root mycelium produced by mycorrhizal roots, which constitutes a three-dimensional network that connects the plant to the surrounding soil, contributes to the formation of stable aggregates in the soil. The stability of these soil aggregates can be explained by the production of glomalin, a glycoprotein that acts by its hydrophobic properties to stabilize the aggregates (Singh et al., 2013). The stabilization of soil aggregates is also a result of the fixation of soil particles by hyphae and roots and the exudation of polysaccharides (Kohler et al., 2017).
The current study examined the effects of the invasive plant Acacia cyanophylla on the soil’s physicochemical parameters, on the soil’s richness and diversity of mycorrhizal fungi morphotypes, on the rhizospheric soil’s mycorrhizal potential, and finally on atmospheric nitrogen fixation in the sand dune ecosystem of Essaouira, Morocco.
Material and Methods
Study Site and Soil Sampling
The Essaouira region in Morocco seems to be a suitable study area due to the importance of the living dune ecosystem (latitude of 31° 30′ 0.00″ N; longitude of −9° −45′ −36, 00″ W) and the heavy invasion process conducted by A. cyanophylla. Three sites were randomly chosen to find out how A. cyanophylla changes soil chemical and biological fertility. They were named site 1, site 2 and site 3. On 4 March 2019, five A. cyanophylla plants were randomly picked at each site. At a depth of 10–20 cm, soils directly influenced by the roots (rhizosphere) were collected. Additionally, the bare soil (served as a control) was gathered well away from any plant roots at each location. Fifteen rhizosphere soil samples and three bare soil samples were collected. A composite soil was produced for each of the three sites by mixing the five samples homogeneously. The bare soils were also combined to generate a homogeneous sample (control).
Soil pH and Electric Conductivity
The physicochemical properties of the examined soils are given in Table 1. Initially, all soil samples were air-dried and sieved at 2 mm. Afterward, an aliquot of soil was suspended in distilled water (1:2.5, w/v) before being stirred for 30 min using a mechanical agitator. The pH of each soil was measured by a calibrated digital pH meter (JP Selecta pH-2006). The electric conductivity of the soil was measured after magnetic stirring for 20 min of 10 g of soil in 50 ml of distilled water; the measurement was carried out using a conductivity meter calibrated with a KCl solution (0.001N).
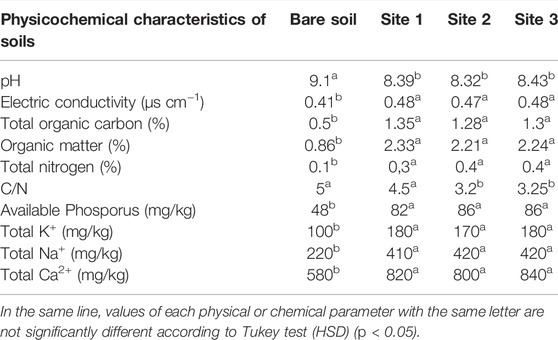
TABLE 1. pH, electrical conductivity and mineral contents of the rhizospheric soils of Acacia cyanophylla and the bare soil (control) in the sand dunes of Essaouira.
Total Organic Carbon and Soil Organic Matter
The organic matter was determined according to Anne’s method as described by Genin et al. (2017), which is based on the oxidation of soil organic carbon by potassium dichromate in an acidic medium and the back titration of the excess potassium dichromate by a solution of Mohr’s salt in the presence of diphenylamine sulfonate as an indicator. After titration, the percentage of total organic carbon (%TOC) was calculated according to the following formula:
where: Vc (cm3) = Control volume, Vs (cm3) = sample volume and m (g) = soil dry mass.
The soil organic matter content (%OM) was calculated as follows:
Soil Mineral Content
To assess the mineral content of A. cyanophylla soil, 2 g of rhizospheric and bare soil were digested in sulfuric acid solution at 600°C for 6 h. Ash was mixed with 2 ml of HCl (10N), agitated, evaporated on a hot plate, and collected in 100 ml of distilled water. The contents of K+, Na+ and Ca2+were determined according to the method of Brown and Lilleland, (1946) using a flame spectrophotometer. The total nitrogen was determined using the Kjeldahl method (Bremner, 1960). The available phosphorus was determined using the molybdenum blue colorimetric technique (Wei et al., 2009). The optical densities of the solutions were measured at 880 nm using an S-22 UV/Vis spectrophotometer (Boeco, Germany).
Enumeration of Arbuscular Mycorrhizal Fungi Spores in Soils
Isolation of arbuscular mycorrhizal fungi spores from rhizosphere soil was performed according to the method described by (Brundrett et al., 1996), based on the extraction of spores by wet sieving and decanting of soil followed by centrifugation in a sucrose solution. So, 100 g of rhizosphere soil was suspended in 1 L of tap water. The mixture was then stirred and then left to settle. After decantation, the supernatant was poured through a series of sieves ranging in size from 800 μm to 50 μm to remove the largest particles of organic matter from the soil while still retaining spores of different sizes. The operation was repeated several times to recover as many spores as possible. Spores suspension retained in sieves was suspended in distilled water and centrifuged for 5 min at 2000 rpm, the supernatant and floating debris were removed to add 20 ml of sucrose solution (65%) to the pellet for 1 min centrifugation at 2000 rpm to separate the spores of denser soil components. The supernatant was filtered through a filter paper under a vacuum, and the spores were collected on Petri dishes for enumeration using a binocular magnifying glass (Magnification:×40).
Morphological Description of Arbuscular Mycorrhizal Fungi Spores Associated With Acacia cyanophylla
Spore extraction was based on the wet sieving and decantation of the soil samples (Gerdemann and Nicolson, 1963) and the concentration of the spores by centrifugation on a sucrose solution (Brundrett et al., 1996). The isolated spores were sorted under the binocular (Magnification ×40) according to morphological characters (color, size, shape). Other morphological parameters were used under a microscope after mounting the spores between slide and coverslip, such as the diameter of the spores, the lamellar structure of the spore wall, and the form of attachment of the hyphae to the spore. Finally, recovered spores were stored in a polyvinyl-lacto-glycerine (PVLG) medium until use (Estaún et al., 1997). Spores with similar morphological traits were grouped in a homogeneous group called a morphotype. The relative abundance of each morphotype was calculated as the number of spores of that morphotype divided by the total number of spores from each soil (El kinany et al., 2018).
Determination of Mycorrhizal Traits in the Roots of Acacia cyanophylla
Thinning and coloring of roots were carried out following the modified method of Phillips and Hayman (1970). The roots were washed under a gentle stream of water to remove attached soil, organic matter, and foreign roots. A 10% KOH solution was used to bleach the roots for 60 min at a temperature of 90°C. The samples were then rinsed and acidified by adding a few drops of 5% lactic acid, which neutralized the remaining KOH. The thinned roots were stained with a 0.05% acidic trypan blue solution, diluted in lactoglycerol (1/3 water, 1/3 glycerol and 1/3 lactic acid) for 15 min at 90°C. The colored roots were then mounted between the slide and coverslip and observed using a Microscope. The mycorrhizal frequency represents the number of mycorrhizal roots in the total examined roots. While the mycorrhizal colonization provides information on the total volume of the root colonized by different fungal structures. It was estimated according to five classes of colonization 0 (non mycorrhized at all), 1 (trace of mycorrhization), 2 (less than 10% colonization), 3 (between 11 and 50% colonization), 4 (between 51 and 90%), 5 (more than 91% colonization) (McGonigle et al., 1990; Brundrett et al., 1996). The volume occupied by each different mycorrhizal structure (Mycelium, vesicles, arbuscules) was also estimated by microscopic observations.
where n5 is the number of fragments with index 5, n1 is the number of fragments with index 1, and I is the frequency assigned to the index 5.
The absolute mycorrhization intensity (Mycorrhization intensity among mycorrhized fragments) is calculated as follows:
where Tf = Total fragments and Mf = Mycorrhized fragments.
Determination of the Mycorrhizal Rhizospheric Soil Infectivity of A. cyanophylla by the Method of the MPN of Fungal Propagules
The Most Probable Number (MPN) method was performed to determine the mycorrhizal infectious potential of a soil which exhibits the ability of that soil to initiate the formation of mycorrhizal associations in a test plant from a quantity of inoculum present in rhizosphere soil in the form of spores, mycelium and root debris bearing vesicles (propagules) (Ouahmane et al., 2012). For this, the five samples of rhizospheric soils taken were mixed to have a homogeneous composite, and the latter was diluted in sterile soil (121°C, 2 h) in proportion (1, 1/4, 1/16, 1/64, 1/256, 1/1,024) with 5 repetitions for each dilution level. In parallel, the control soil was diluted in the same way. Maize was used as an endophytic plant to trap the native mycorrhizal complex associated with A. cyanophylla. Corn seeds were pre-germinated in Petri dishes at an ambient temperature of 25°C and then planted in plastic cups containing 100 g of dilution soil. After 4 weeks of cultivation, the plants were harvested and their root systems were carefully prepared for staining. During reading, roots representing at least one point of infection were classified as positive and negative ones were presented by lack of colonization (Ouahmane et al., 2007a; Ramos-Zapata et al., 2010). The estimation of the MPN was performed using the table of Fisher and Yates (1970) and the following formula:
where x is the average number of mycorrhized pots, a is the dilution factor and k is a constant from table VIIItbl of Fisher and Yates for fivefold dilutions.
The standard deviation of log MPN is estimated and given by the formula (Cochran, 1950).
where n is the number of samples per dilution and a is the dilution ratio.
Nodulation Intensity
The evaluation of modulation of A. cyanophylla shrubs was carried out by direct counting of the functional nodules formed on the root system encountered in 1-kg rhizosphere soil.
Statistical Analysis
The results were processed with XL Stat software and SPSS software using the analysis of variance test (ANOVA) at a threshold of statistical significance set at 5% and the Tukey’s HSD test for the comparison of the means.
Results
Soil pH and Electrical Conductivity
The soil pH and electric conductivity analysis showed significant differences between the rhizosphere soil and the control (bare soil) (Table 1). The introduction and establishment of golden wreath wattle (A. cyanophylla) in the sand dune of Essaouira resulted in widespread acidification of the soil in the study area. The reading of pH showed that the rhizospheric soil samples had values that were 7.4–8.6% lower than those of bare soil samples, which range from 9.10 to 8.3–8.4. Similarly, the presence of A. cyanophylla had shown a significant influence on electrical conductivity, which raised by 14.6–17.1% in rhizospheric soil (sites 1, 2 and 3) compared to bare soil (Table 1).
Total Organic Carbon and Mineral Content
A significant increase in the carbon and consequently in the organic matter contents of the sampled soils in the rhizosphere of A. cyanophylla was recorded. In comparison to adjacent bare soil, the amount of carbon in the rhizosphere rose approximately more than twice in the presence of trees at all sample locations. The pleading restitution of carbon to soil is certainly linked to the massive production of foliage by this legume (Table 1). The analysis of the rhizospheric soils under the crown of A. cyanophylla shrubs at the three sites studied showed organic matter contents two to three times greater than those in the bare soil. Mineral analysis of the soils showed a substantial accumulation of the main nutrients in the rhizosphere of A. cyanophylla. A significant difference was recorded for all the analyzed elements (N, P, K+, Ca2+, and Na+) compared with non-rhizosphere soil without the influence of the shrub. Enrichment of the soil by the golden wreath wattle was noticed for all the analyzed elements and which testified their contents doubling (P, K+, Ca2+, and Na+), particularly the nitrogen contents were three (site 1) to four (sites 2 and 3) times higher in rhizospheric soil than in control soil (Table 1). The quantity of available phosphorus and total potassium in the rhizosphere enhanced roughly 1.7–1.8 times in the presence of tree roots when compared to bare soil at all study sites as compared to bare soil.
Enumeration and Morphological Description of AMF Spores Associated With A. cyanophylla
Extraction and counting of spores from subjacent soils of A. cyanophylla showed at least 800 spores per 100 g of rhizosphere soil, while non-rhizosphere soil showed 160 spores per 100 g of soil only (Figure 1). A cyanophylla had shown a greater capacity to establish a symbiosis with arbuscular mycorrhizal fungi and higher production of fungal spores in the soil. The community of AMF associated with A. cyanophylla was described by the relative abundance of different morphotypes of encountered spores. Observation of the spores under the binocular microscope at high magnification revealed the presence of four different morphotypes depending on their color: black, dark brown, light brown, yellow and grey (Figure 2). As demonstrated in Figure 3, each AMF morphotype is represented by its relative abundance in Acacia cyanophylla rhizospheric soils as well as in control (bare soil).
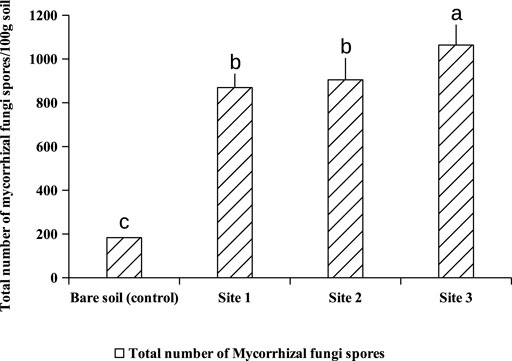
FIGURE 1. Total number of mycorrhizal spores per 100 g soil in different rhizospheric soils of Acacia cyanophylla and in the control (bare soil) in the sand dune ecosystem of Essaouira. Graphs indexed by the same letter are not significantly different at p < 0.05 according to Tukey’s test (HSD).
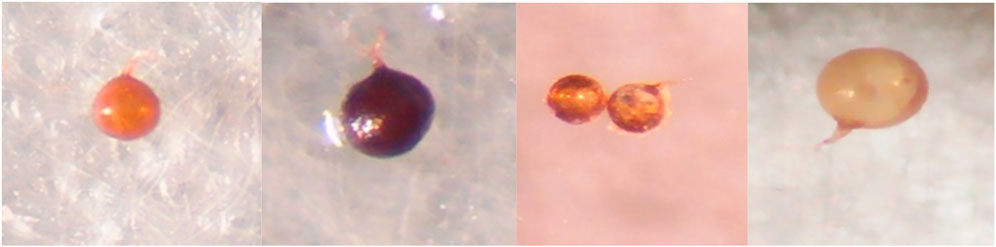
FIGURE 2. Photos of the main representative mycorrhizal morphotypes encountered in the rhizosphere soil of Acacia cyanophylla in the sand dune ecosystem of Essaouira, Morocco.
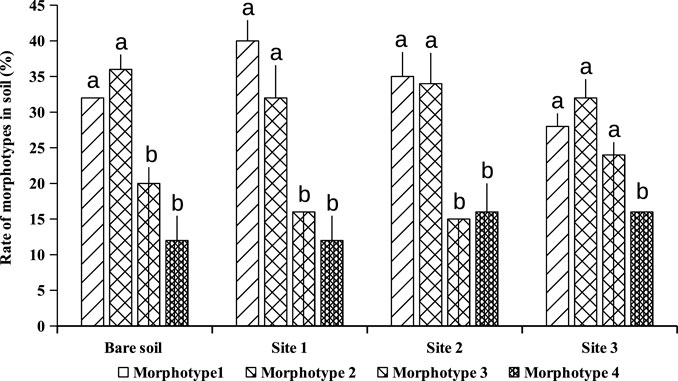
FIGURE 3. Relative abundance of each AMF morphotype in rhizospheric soils of Acacia cyanophylla and in the control (bare soil) in the sand dune of Essaouira. For each soil sampling site, the bar-graphs (rate of each morphotype) indexed by the same letter are not significantly different at p < 0.05 according to the Tukey’s test (HSD).
Morphotype1: dark brown spores; this is the most common group in different plants-sites with a relative abundance of 40–50%.
Morphotype 2: light brown spores which exhibit a relative abundance of 30–47%.
Morphotype 3: black spores with a relative abundance of 15–23%.
Morphotype 4: greyish transparent spores with a relative abundance of only 3–7%.
Determination of Mycorrhizal Traits in Acacia cyanophylla Plant Roots
Microscopic observation of the roots of A. cyanophylla in the various sites showed that all the plants presented an arbuscular mycorrhizal fungus infection, and at least the mycorrhizal frequency reached 90%. The mycorrhizal colonization assessment showed rates between 40 and 50% (Table 2). The intensity of mycorrhizal infection (Ma %) varied between 40, 44, and 50% of roots collected in rhizospheric soil at sites 3, 1, and 2, respectively. It was discovered that the levels of vesicles and hyphae varied according to the location of the plants and rhizospheric soil collected; for example, Acacia cyanophylla roots from site 1 had the highest proportion of vesicles (60%), while those from sites 2 and 3 had the highest percentage of hyphae (70%). The proportion of arbuscules, on the other hand, is almost identical (10%) throughout the three sampling sites. A. cyanophylla roots showed higher levels of colonization by arbuscular mycorrhizal fungi, indicating that A. cyanophylla is one of the symbiotic plants that weave a strong relationship with AMF in their natural habitats.
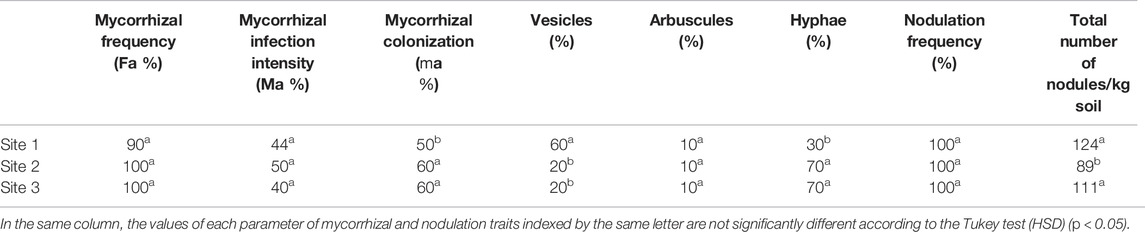
TABLE 2. Mycorrhizal and nodulation traits in roots of Acacia cyanophylla collected in different sites in the sand dune ecosystem of Essaouira.
Assessment of Mycorrhizogenic Infectious Potential of A. cyanophylla Rhizospheric Soil Using the MPN of Fungal Propagules Test
Maize plants have been used as an endophytic plant to trap the native mycorrhizal complex naturally associated with A. cyanophylla. Corn seedlings were grown on different dilutions of rhizosphere soils (1; 1/4; 1/16; 1/64; 1/256; 1/1,024) from different sites under A. cyanopylla. A seedling is considered to be mycorrhized when it has at least one point of infection. Only one among five corn seedlings was mycorrhizal in the control soil, which confirms the lower number of spores in this soil (Figure 1). However, all the roots of Maize seedlings grown in rhizospheric soils of A. cyanophylla exhibited a mycorrhizal infection with different colonization rates. The number of fungal propagules (viable spores, vesicles, root fragments) in each soil was determined using the table of Fisher and Yates. Based on the calculation, the MPN per 100 g was: MPN (IC 95%) = 1,240 for rhizosphere soil and was MPN (95% CI) = 48 for non-rhizospheric soil (Figure 4). The mycorrhizal fungal community of A. cyanophylla rhizosphere soil was assessed for its self-regeneration by testing the most probable number of fungal propagules in the soil without distinguishing between these propagules (viable spores, vesicles, root fragments).
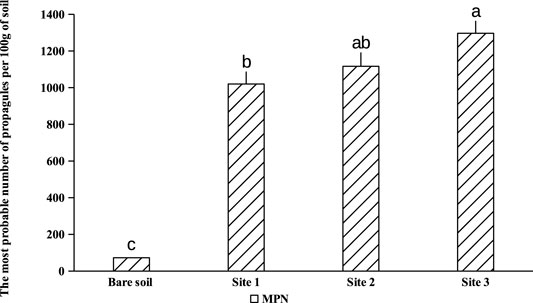
FIGURE 4. The most probable number of fungal propagules in different rhizospheric soils of Acacia cyanophylla in the sand dune ecosystem of Essaouira. Bar-graphs indexed by the same letter are not significantly different according to the Tukey test (HSD) (p < 0.05).
Nodulation Activity
The direct counting of the total number of nodules formed by symbiotic rhizobacteria on A. cyanophylla roots showed that all the rhizosphere soil samples contained nodulated root fragments. All the root fragments at the level of the rhizosphere soil samples were examined and approximately 130 nodules per kilogram of soil were noted (Table 2).
Discussion
In this study, golden wreath wattle (Acacia cyanophylla), an exotic shrub/tree species, was selected to examine the soil’s chemical and biological fertility in the sand dunes of Essaouira, Morocco. Generally, soils are the result of the activities of plants, which provide organic matter and play an important role in the weathering of rocks and mineral resources (Liu et al., 2022; Qin et al., 2019). In our study, the results of the physicochemical analysis suggest that A. cyanophylla has an advantage on chemical soil fertility, explained by the increase in the total organic matter and the content of mineral elements in the soil surrounding roots of A. cyanophylla. These results can be attributed to the fact that this shrub species is able to produce considerable amounts of biomass (Guarino and Sciarrillo, 2017). The roots and aerial biomass turn into the soil and undergo the degradation and mineralization process. This phenomenon leads to the soil enrichment in organic matter and mineral elements (C, N, P, K, Ca, Mg, N) (Yusoff et al., 2019). The environmental conditions of the study site (precipitations, temperature and sandy nature of the soil) allow the rapid transformation of organic matter and its incorporation into the soil, especially under the crown of shrubs. In addition, A. cyanophylla is a legume species capable of conducting dual symbiosis relationships with arbuscular mycorrhizal fungi and atmospheric nitrogen-fixing rhizobacteria (Birnbaum et al., 2017). These two types of symbiosis are traditionally known for their undeniable respective roles in phosphate and nitrogen nutrition. The levels of available phosphorus and total nitrogen in rhizospheric soils bear witness to these effects (Amira et al., 2012; Yusoff et al., 2019). In the current work, the phosphorus contents are twice as high and the total nitrogen contents are four times higher compared to a non-rhizosphere soil without the influence of the roots of A. cyanophylla. On the other hand, it is possible that soil type, climate, and plant species have an impact on the species composition of AMF fungal communities. Our findings suggest that A. cyanophylla is associated with a diversified fungal community, and at least we could distinguish four different morphotypes among the mycorrhizal community (Figure 3). The study of the morphological diversity of mycorrhizal fungi associated with the rhizospheric soil of A. cyanophylla has shown the existence of several morphotypes which show significant diversity (Afaf et al., 2015; Bouazza et al., 2015). This community of AMF adapted to these environments forms a mycelial network around the root system and allows the exploitation of a large surface at ground level, hence their capital role in the supply of water and phosphate for the plant. Otherwise, the self-regeneration capacity of the mycorrhizal fungal community in A. cyanophylla rhizosphere soil was determined by counting the most probable number of fungal propagules in the soil. We discovered that all the roots of Maize seedlings grown in A. cyanophylla rhizosphere soils had a mycorrhizal infection with varying colonization rates. These results confirmed the existence of an active community and a large number of viable propagules around the roots of A. cyanophylla, which provides information on the adaptation and functioning of these symbionts in the sand dune ecosystem of Essaouira (Guarino and Sciarrillo, 2017). This effect was also noticed in the rhizospheric soil of other invasive plants such as Retama monosperma, which presented a greater mycorrhizal potential, as well as Nicotiana glauca, which is a very mycotrophic shrub capable of increasing the stock of mycorrhizal propagules and thus serving as an effective source of mycorrhizal inoculum in severely disturbed and degraded soils characterized by very low mycorrhizal potential (Maier et al., 2000). Similar results were recently proved in mixed-species plantations of Eucalyptus and Acacia (Tchichelle et al., 2017). Moreover, A. cyanophylla was previously reported to exhibit a large number of nodules on the root system and to form symbioses with a higher number of Rhizobia strains (Amira et al., 2012; Bouazza et al., 2015; Birnbaum et al., 2017). The exotic shrub A. cyanophylla has shown its primordial role in maintaining and improving the chemical and biological fertility of the soil in the sand dunes ecosystem of Essaouira (Kavroulakis et al., 2020; Lozano et al., 2020; Tigka and Ipsilantis, 2020). These ecological and nutritional functions are ensured thanks to the rapid growth of the species, its enormous production of biomass, and its symbiotic status as a host plant for the rhizobacteria fixing atmospheric nitrogen and for arbuscular mycorrhizal fungi, which are known for their intervention in biogeochemical cycles of major nutrients such as phosphorus and nitrogen as well as their crucial role in the water nutrition of the host plants. According to our collected data, the total nitrogen level in the rhizosphere was three to four times greater than the nitrogen content in bare soil (Table 1). These interesting findings are in line with some previous studies and directly comparable to those published recently by Jian et al. (2022), who revealed the beneficial effects of Leymus secalinus and Carex praeclara on soil characteristics and microbial community composition in a Zoige desertified alpine grassland.
Indeed, all these potentialities and biological qualities highlighted and possessed by A. cyanophylla allow it to be advocated and recommended as an exotic plant in the programs aiming to combat soil degradation and to protect natural resources. Hence, the association of A. cyanophylla with fungal and rhizobial symbionts in reforestation programs would be of great importance to guarantee the success of Atlantic dunes fixation and to fight against the desertification phenomenon.
Conclusion
A. cyanophylla as an exotic plant has shown a vast ability to establish a dual symbiotic association with arbuscular mycorrhizal fungi and with atmospheric nitrogen-fixing rhizobacteria. A. cyanophylla showed beneficial effects on the quality and the fertility of the sand dune ecosystem soil of Essaouira. The rhizosphere microbial communities work in harmony with the plant to improve its nutrition and growth. Indeed, the fungal and bacterial partners provide the plant with sufficient amounts of water, phosphorus, nitrogen and other nutrients elements. On the other hand, A. cyanophylla is a fast-growing plant, and the large quantities of biomass produced by the plant are returned to the soil after their degradation and mineralization, which induces high levels of organic and mineral matter in the rhizosphere soil. A. cyanophylla can therefore modulate and maintain a sustainable balance between the different abiotic and biotic components involved in these interactions. The high potential of the rhizosphere soil to regenerate mycorrhizal and rhizobial associations testifies to the ecological balance established between the plant and its microbial symbionts. Hence, using golden wreath wattle in reforestation programs to rehabilitate and fix sand dunes is strongly recommended.
Data Availability Statement
The raw data supporting the conclusion of this article will be made available by the authors, without undue reservation.
Author Contributions
HD, MB, EO, and MB: methodology, writing—original draft, formal analysis, reviewing, and editing. H-AN, ME-S, GA-A: reviewing, visualization, and editing. LO: visualization, supervision and validation.
Conflict of Interest
The authors declare that the research was conducted in the absence of any commercial or financial relationships that could be construed as a potential conflict of interest.
Publisher’s Note
All claims expressed in this article are solely those of the authors and do not necessarily represent those of their affiliated organizations, or those of the publisher, the editors and the reviewers. Any product that may be evaluated in this article, or claim that may be made by its manufacturer, is not guaranteed or endorsed by the publisher.
Acknowledgments
The authors acknowledge the funding support from Researchers Supporting Project Number (RSP-2022/182), King Saud University, Riyadh, Saudi Arabia.
References
Abbaspour, H., Saeidi-Sar, S., Afshari, H., and Abdel-Wahhab, M. A. (2012). Tolerance of Mycorrhiza Infected Pistachio (Pistacia Vera L.) Seedling to Drought Stress under Glasshouse Conditions. J. Plant Physiology 169, 704–709. doi:10.1016/j.jplph.2012.01.014
Afaf, N., Zohra, I., Faiza, B. Z., and Abdelkader, B. (2015). Diversity of Arbuscular Mycorrhizal Fungi in Two Perturbed Ecosystems (Dune and Saline Soil) in West Algeria. Int. J. Agric. Crop Sci. 8, 380.
Amira, S., Amira, S., Nermeen, S., Osama, N. M., and Swelin, D. M. (2012). Improving Salinity Tolerance of Acacia Saligna (Labill.) Plant by Arbuscular Mycorrhizal Fungi and Rhizobium Inoculation. Afr. J. Biotechnol. 11, 1259–1266. doi:10.5897/ajb11.2287
Amrani, S., Noureddine, N. E., Bhatnagar, T., Argandoña, M., Nieto, J. J., and Vargas, C. (2010). Phenotypic and Genotypic Characterization of Rhizobia Associated with Acacia Saligna (Labill.) Wendl. In Nurseries from Algeria. Syst. Appl. Microbiol. 33, 44–51. doi:10.1016/j.syapm.2009.09.003
Badalamenti, E., Bueno, R. da. S., Campo, O., Gallo, M., La Mela Veca, D. S., Pasta, S., et al. (2018). Pine Stand Density Influences the Regeneration of Acacia Saligna Labill. H.L. Wendl. And Native Woody Species in a Mediterranean Coastal Pine Plantation. Forests 9, 1–16. doi:10.3390/f9060359
Balzergue, C., Chabaud, M., Barker, D. G., Bécard, G., and Rochange, S. F. (2013). High Phosphate Reduces Host Ability to Develop Arbuscular Mycorrhizal Symbiosis without Affecting Root Calcium Spiking Responses to the Fungus. Front. Plant Sci. 4, 426. doi:10.3389/fpls.2013.00426
Barea, J. M., Pozo, M. J., Azcón, R., and Azcón-Aguilar, C. (2005). Microbial Co-operation in the Rhizosphere. J. Exp. Bot. 56, 1761–1778. doi:10.1093/jxb/eri197
Baumel, A., Mirleau, P., Viruel, J., Bou Dagher Kharrat, M., La Malfa, S., Ouahmane, L., et al. (2018). Assessment of Plant Species Diversity Associated with the Carob Tree (Ceratonia Siliqua, Fabaceae) at the Mediterranean Scale. Plant Ecol. Evol. 151 (2), 185–193. doi:10.5091/plecevo.2018.1423
Belay, Z., Vestberg, M., and Assefa, F. (2013). Diversity and Abundance of Arbuscular Mycorrhizal Fungi Associated with acacia Trees from Different Land Use Systems in Ethiopia. Afr. J. Microbiol. Res. 7, 5503–5515. doi:10.5897/AJMR2013.6115
Beltrano, J., Ruscitti, M., Arango, M. C., and Ronco, M. (2013). Effects of Arbuscular Mycorrhiza Inoculation on Plant Growth, Biological and Physiological Parameters and Mineral Nutrition in Pepper Grown under Different Salinity and P Levels. J. Soil Sci. Plant Nutr. 13, 123–141. doi:10.4067/s0718-95162013005000012
Benbrahim, K. F., Berrada, H., El Ghachtouli, N., and Ismaili, M. (2014). Acacia: Promising Nitrogen Fixing Trees for Sustainable Development in Arid and Semi-arid Areas. Int. J. Innov. Appl. Stud. 8, 46–58.
Birnbaum, C., Bradshaw, L. E., Ruthrof, K. X., and Fontaine, J. B. (2017). Topsoil Stockpiling in Restoration: Impact of Storage Time on Plant Growth and Symbiotic Soil Biota. Ecol. Restor. 35, 237–245. doi:10.3368/er.35.3.237
Blackburn, T. M., Pyšek, P., Bacher, S., Carlton, J. T., Duncan, R. P., Jarošík, V., et al. (2011). A Proposed Unified Framework for Biological Invasions. Trends Ecol. Evol. 26, 333–339. doi:10.1016/j.tree.2011.03.023
Bouazza, M. K., Ighilharizn, Z., de Lajudie, P., Duponnois, R., and Bekki, A. (2015). Assessing the Native Arbuscular Mycorrhizal Symbioses to Rehabilitate a Degraded Coastal Sand Dune in Algeria. Int. J. Agric. Crop Sci. 8, 194–202.
Boukhatem, Z. F., Domergue, O., Bekki, A., Merabet, C., Sekkour, S., Bouazza, F., et al. (2012). Symbiotic Characterization and Diversity of Rhizobia Associated with Native and Introduced Acacias in Arid and Semi-arid Regions in Algeria. FEMS Microbiol. Ecol. 80, 534–547. doi:10.1111/j.1574-6941.2012.01315.x
Bouskout, M., Bourhia, M., Al Feddy, M. N., Dounas, H., Salamatullah, A. M., Soufan, W., et al. (2022). Mycorrhizal Fungi Inoculation Improves Capparis Spinosa’s Yield, Nutrient Uptake and Photosynthetic Efficiency under Water Deficit. Agronomy 12 (1), 149. doi:10.3390/agronomy12010149
Bremner, J. M. (1960). Determination of Nitrogen in Soil by the Kjeldahl Method. J. Agri. Sci. 55, 11–33. doi:10.1017/S0021859600021572
Brown, J. G., and Lilleland, O. (1946). “Rapid Determination of Potassium and Sodium in Plant Materials and Soil Extracts by Flame Photometry,” in Proceedings of the American Society for Horticultural Science (Alexandria, VA: North Saint Asaph Street), 341–346.
Brundrett, M., Bougher, N., Dell, B., Grove, T., and Malajczuk, N. (1996). Working with Mycorrhizas in Forestry and Agriculture (ACIAR Monograph 32). Canberra, Aust. Aust. Cent. Int. Agric. Res.
Cochran, W. G. (1950). Estimation of Bacterial Densities by Means of the" Most Probable Number. Biometrics 6, 105–116. doi:10.2307/3001491
Derbel, S., Cortina, J., and Chaieb, M. (2009). Acacia Saligna Plantation Impact on Soil Surface Properties and Vascular Plant Species Composition in Central Tunisia. Arid. L. Res. Manag. 23, 28–46. doi:10.1080/15324980802599209
Derkaoui, N., Bouchiba, Z., Boukhatem, Z. F., Yahia, N., Duponnois, R., Baudoin, E., et al. (2016). Study on Biotic and Abiotic Factors Explaining Acacia Saligna Growth Heterogeneity in Revegetated Terga Pit in Western Coast of Algeria. Int. J. Agric. Crop Sci., 41–49.
Duponnois, R., Hafidi, M., Thioulouse, J., Galiana, A., Ouahmane, L., Dreyfus, B., et al. (2009). “Monitoring the Development of Nurse Plant Species to Improve the Performances of Reforestation Programs in Mediterranean Areas,” in Microbial Strategies for Crop Improvement. Editors M. Khan, A. Zaidi, and J. Musarrat (Berlin, Heidelberg: Springer). doi:10.1007/978-3-642-01979-1_12
Ee, K. Y., and Yates, P. (2013). Nutritional and Antinutritional Evaluation of Raw and Processed Australian Wattle (Acacia Saligna) Seeds. Food Chem. 138, 762–769. doi:10.1016/j.foodchem.2012.10.085
El Kinany, , Achbani, E., Faggroud, M., Ouahmane, L., El Hilali, R., Haggoud, A., et al. (2018). Effect of Organic Fertilizer and Commercial Arbuscular Mycorrhizal Fungi on the Growth of Micropropagated Date Palm Cv. Feggouss. J. Saudi Soc. Agric. Sci. 18, 411. doi:10.1016/j.jssas.2018.01.004
El-Euch, F. (2000). Role of Acacia Cyanophylla in Livestock Feeding in Tunisia. Cah Options Méditerranéennes 45, 431–434.
Estaún, V., Savé, R., and Biel, C. (1997). AM Inoculation as a Biological Tool to Improve Plant Revegetation of a Disturbed Soil with Rosmarinus Officinalis under Semi-arid Conditions. Appl. Soil Ecol. 6, 223–229. doi:10.1016/S0929-1393(97)00014-0
Fisher, R. A., and Yates, F. (1970). Statistical Tables: For Biological, Agricultural and Medical Research. 6th ed. Edinburgh: Oliver & Boyd.
Fortin, J. A., Plenchette, C., and Piché, Y. (2015). Les mycorhizes: la nouvelle révolution verte. Montréal (Québec): Éditions MultiMondes.
Gebreyohaness, S. (2016). Effect of Feeding Graded Level of Dried Acacia Saligna Leaves on Milk Yield and Milk Composition of Crossbred Dairy Cows Fed Grass Hay as Basal Diet. J. Biol. Agric. Healthc. 6, 15–20.
Genin, M., Alifriqui, M., Fakhech, A., Hafdi, M., Ouahmane, L., and Genin, D. (2017). Back to Forests in Pre-Saharan Morocco? when Prickly Pear Cultivation and Traditional Agropastoralism Reduction Promote Argan Tree Regeneration. Silva Fenn. 51, 1618. doi:10.14214/sf.1618
Gerdemann, J. W., and Nicolson, T. H. (1963). Spores of Mycorrhizal Endogone Species Extracted from Soil by Wet Sieving and Decanting. Trans. Br. Mycol. Soc. 46, 235–244. doi:10.1016/s0007-1536(63)80079-0
Griffin, A. R., Midgley, S. J., Bush, D., Cunningham, P. J., and Rinaudo, A. T. (2011). Global Uses of Australian Acacias - Recent Trends and Future Prospects. Divers. Distrib. 17, 837–847. doi:10.1111/j.1472-4642.2011.00814.x
Guarino, C., and Sciarrillo, R. (2017). Effectiveness of in situ Application of an Integrated Phytoremediation System (IPS) by Adding a Selected Blend of Rhizosphere Microbes to Heavily Multi-Contaminated Soils. Ecol. Eng. 99, 70–82. doi:10.1016/j.ecoleng.2016.11.051
He, X., and Nara, K. (2007). Element Biofortification: Can Mycorrhizas Potentially Offer a More Effective and Sustainable Pathway to Curb Human Malnutrition? Trends Plant Sci. 12, 331–333. doi:10.1016/j.tplants.2007.06.008
Ilahi, H., Hsouna, J., Ellouze, W., Gritli, T., Chihaoui, S., Barhoumi, F., et al. (2021). Phylogenetic Study of Rhizobia Nodulating Pea (Pisum Sativum) Isolated from Different Geographic Locations in Tunisia. Syst. Appl. Microbiol. 44, 126221. doi:10.1016/j.syapm.2021.126221
Jacobs, K., Conradie, T., and Jacobs, S. (2020). Microbial Communities in the Fynbos Region of South Africa: what Happens during Woody Alien Plant Invasions. Diversity 12, 254. doi:10.3390/d12060254
Jadrane, I., Al feddy, M. N., Dounas, H., Kouisni, L., Aziz, F., and Ouahmane, L. (2021). Inoculation with Selected Indigenous Mycorrhizal Complex Improves Ceratonia Siliqua’s Growth and Response to Drought Stress. Saudi J. Biol. Sci. 28, 825–832. doi:10.1016/j.sjbs.2020.11.018
Jian, T., Xia, Y., He, R., and Zhang, J. (2022). The Influence of Planting Carex Praeclara and Leymus Secalinus on Soil Properties and Microbial Community in a Zoige Desertified Alpine Grassland. Glob. Ecol. Conservation 34, e02002. doi:10.1016/j.gecco.2022.e02002
Kavroulakis, N., Tsiknia, M., Ipsilantis, I., Kavadia, A., Stedel, C., Psarras, G., et al. (2020). Arbuscular Mycorrhizal Fungus Inocula from Coastal Sand Dunes Arrest Olive Cutting Growth under Salinity Stress. Mycorrhiza 30, 475–489. doi:10.1007/s00572-020-00963-x
Kheloufi, A., Mansouri, L. M., Mami, A., and Djelilate, M. (2019). Physio-biochemical Characterization of Two acacia Species (A. Karroo Hayn and A. Saligna Labill.) under Saline Conditions. Reforesta 7, 33–49. doi:10.21750/refor.7.04.66
Kohler, J., Roldán, A., Campoy, M., and Caravaca, F. (2017). Unraveling the Role of Hyphal Networks from Arbuscular Mycorrhizal Fungi in Aggregate Stabilization of Semiarid Soils with Different Textures and Carbonate Contents. Plant Soil 410, 273–281. doi:10.1007/s11104-016-3001-3
Lahdachi, F. Z., Nassiri, L., Ibijbijen, J., and Mokhtari, F. (2015). Aperçu sur les acacias spontanés et introduits au Maroc. Eur. Sci. J. 11 (23), 88–102.
Le Maitre, D. C., Gaertner, M., Marchante, E., Ens, E. J., Holmes, P. M., Pauchard, A., et al. (2011). Impacts of Invasive Australian Acacias: Implications for Management and Restoration. Divers. Distrib. 17, 1015–1029. doi:10.1111/j.1472-4642.2011.00816.x
Lehmann, A., Veresoglou, S. D., Leifheit, E. F., and Rillig, M. C. (2014). Arbuscular Mycorrhizal Influence on Zinc Nutrition in Crop Plants - A Meta-Analysis. Soil Biol. biochem. 69, 123–131. doi:10.1016/j.soilbio.2013.11.001
Liu, S., He, F., Kuzyakov, Y., Xiao, H., Hoang, D. T. T., Pu, S., et al. (2022). Nutrients in the Rhizosphere: A Meta-Analysis of Content, Availability, and Influencing Factors. Sci. Total Environ. 826, 153908. doi:10.1016/j.scitotenv.2022.153908
Lozano, V., Marzialetti, F., Carranza, M. L., Chapman, D., Branquart, E., Dološ, K., et al. (2020). Modelling Acacia Saligna Invasion in a Large Mediterranean Island Using PAB Factors: A Tool for Implementing the European Legislation on Invasive Species. Ecol. Indic. 116, 106516. doi:10.1016/j.ecolind.2020.106516
Maier, W., Schmidt, J., Nimtz, M., Wray, V., and Strack, D. (2000). Secondary Products in Mycorrhizal Roots of Tobacco and Tomato. Phytochemistry 54, 473–479. doi:10.1016/s0031-9422(00)00047-9
Martínez, M. L., Gallego-Fernández, J. B., and Hesp, P. A. (2013). Coastal Dune Restoration: Trends and Perspectives. In Restoration of Coastal Dunes (Springer: Berlin/Heidelberg, Germany), 323–339. doi:10.1007/978-3-642-33445-0
Marzialetti, F., Bazzichetto, M., Giulio, S., Acosta, A. T. R., Stanisci, A., Malavasi, M., et al. (2019). Modelling Acacia Saligna Invasion on the Adriatic Coastal landscape:An Integrative Approach Using LTER Data. Nat. Conserv. 34, 127–144. doi:10.3897/natureconservation.34.29575
McGonigle, T. P., Miller, M. H., Evans, D. G., Fairchild, G. L., and Swan, J. A. (1990). A New Method Which Gives an Objective Measure of Colonization of Roots by Vesicular—Arbuscular Mycorrhizal Fungi. New Phytol. 115, 495–501. doi:10.1111/j.1469-8137.1990.tb00476.x
Millar, M. A., and Byrne, M. (2012). Biogeographic Origins and Reproductive Mode of Naturalised Populations of Acacia Saligna. Aust. J. Bot. 60, 383–395. doi:10.1071/BT12028
Nakmee, P. S., Techapinyawat, S., and Ngamprasit, S. (2016). Comparative Potentials of Native Arbuscular Mycorrhizal Fungi to Improve Nutrient Uptake and Biomass of Sorghum Bicolor Linn. Agric. Nat. Resour. 50, 173–178. doi:10.1016/j.anres.2016.06.004
Ouahmane, L., Hafidi, M., Thioulouse, J., Ducousso, M., Kisa, M., Prin, Y., et al. (2007a). Improvement of Cupressus Atlantica Gaussen Growth by Inoculation with Native Arbuscular Mycorrhizal Fungi. J. Appl. Microbiol. 103, 683–690. doi:10.1111/j.1365-2672.2007.03296.x
Ouahmane, L., Ndoye, I., Morino, A., Ferradous, A., Sfairi, Y., Al Feddy, M., et al. (2012). Inoculation of Ceratonia Siliqua L. With Native Arbuscular Mycorrhizal Fungi Mixture Improves Seedling Establishment under Greenhouse Conditions. Afr. J. Biotechnol. 11, 16422–16426.
Ouahmane, L., Thioulouse, J., Hafidi, M., Prin, Y., Ducousso, M., Galiana, A., et al. (2007b). Soil Functional Diversity and P Solubilization from Rock Phosphate after Inoculation with Native or Allochtonous Arbuscular Mycorrhizal Fungi. For. Ecol. Manage. 241, 200–208. doi:10.1016/j.foreco.2007.01.015
Pathak, R., Singh, S. K., and Gehlot, P. (2017). Diversity, Nitrogen Fixation, and Biotechnology of Rhizobia from Arid Zone Plants, 61–81. doi:10.1007/978-3-319-64982-5_5
Phillips, J. M., and Hayman, D. S. (1970). Improved Procedures for Clearing Roots and Staining Parasitic and Vesicular-Arbuscular Mycorrhizal Fungi for Rapid Assessment of Infection. Trans. Br. Mycol. Soc. 55, 158–161. doi:10.1016/s0007-1536(70)80110-3
Qin, K., Dong, X., Jifon, J., and Leskovar, D. I. (2019). Rhizosphere Microbial Biomass Is Affected by Soil Type, Organic and Water Inputs in a Bell Pepper System. Appl. Soil Ecol. 138, 80–87. doi:10.1016/j.apsoil.2019.02.024
Ramos-Zapata, J. A., Guadarrama, P., Alberto, J. A. N., and Orellana, R. (2011). Arbuscular Mycorrhizal Propagules in Soils from a Tropical Forest and an Abandoned Cornfield in Quintana Roo, Mexico: Visual Comparison of Most-Probable-Number Estimates. Mycorrhiza 21 (2), 139–144. doi:10.1007/s00572-010-0336-0
Sfairi, Y., Ouahmane, L., and Abbad, A. (2012). Breaking Seed Dormancy in Cupressus Atlantica Gaussen, an Endemic and Threatened Coniferous Tree in Morocco. J. For. Res. 23 (3), 385–390. doi:10.1007/s11676-012-0274-0
Singh, P. K., Singh, M., and Tripathi, B. N. (2013). Glomalin: An Arbuscular Mycorrhizal Fungal Soil Protein. Protoplasma 250, 663–669. doi:10.1007/s00709-012-0453-z
Smith Sally, E., and Read, David. (2008). Mycorrhizal Symbiosis. Third Edition. Cambridge: Academic Press. doi:10.1097/00010694-198403000-00011
St-Denis, A., Kneeshaw, D., Bélanger, N., Simard, S., Laforest-Lapointe, I., and Messier, C. (2017). Species-specific Responses to Forest Soil Inoculum in Planted Trees in an Abandoned Agricultural Field. Appl. Soil Ecol. 112, 1–10. doi:10.1016/j.apsoil.2016.12.008
Tchichelle, S. V., Mareschal, L., Koutika, L.-S., and Epron, D. (2017). Biomass Production, Nitrogen Accumulation and Symbiotic Nitrogen Fixation in a Mixed-Species Plantation of Eucalypt and acacia on a Nutrient-Poor Tropical Soil. For. Ecol. Manage. 403, 103–111. doi:10.1016/j.foreco.2017.07.041
Thompson, G. D., Bellstedt, D. U., Richardson, D. M., Wilson, J. R. U., and Le Roux, J. J. (2015). A Tree Well-Travelled: Global Genetic Structure of the Invasive Tree Acacia Saligna. J. Biogeogr. 42, 305–314. doi:10.1111/jbi.12436
Tigka, T., and Ipsilantis, I. (2020). Effects of Sand Dune, Desert and Field Arbuscular Mycorrhizae on Lettuce (Lactuca sativa, L.) Growth in a Natural Saline Soil. Sci. Hortic. Amst. 264, 109191. doi:10.1016/j.scienta.2020.109191
Tisdall, J. M. (1991). Fungal Hyphae and Structural Stability O Soil. Aust. J. Soil Res. 29, 729–743. doi:10.1071/SR9910729
Vaishnav, A., Hansen, A. P., Agrawal, P. K., Varma, A., and Choudhary, D. K. (2017). Biotechnological Perspectives of Legume–Rhizobium Symbiosis, 247–256. doi:10.1007/978-3-319-64982-5_12
Wahid, F., Fahad, S., Danish, S., Adnan, M., Yue, Z., Saud, S., et al. (2020). Sustainable Management with Mycorrhizae and Phosphate Solubilizing Bacteria for Enhanced Phosphorus Uptake in Calcareous Soils. Agric 10, 1–14. doi:10.3390/agriculture10080334
Wei, L. L., Chen, C. R., and Xu, Z. H. (2009). The Effect of Low-Molecular-Weight Organic Acids and Inorganic Phosphorus Concentration on the Determination of Soil Phosphorus by the Molybdenum Blue Reaction. Biol. Fertil. Soils 45 (7), 775–779. doi:10.1007/s00374-009-0381-z
Keywords: Acacia cyanophylla Lindl, arbuscular mycorrhizal fungi, rhizobacteria, nitrogen fixation, sand-dune ecosystem
Citation: Dounas H, Bourhia M, Outamamat E, Bouskout M, Nafidi H-A, El-Sheikh MA, Al-Abbadi GA and Ouahmane L (2022) Effects of Dual Symbiotic Interactions Performed by the Exotic Tree Golden Wreath Wattle (Acacia cyanophylla Lindl.) on Soil Fertility in a Costal Sand Dune Ecosystem. Front. Environ. Sci. 10:895462. doi: 10.3389/fenvs.2022.895462
Received: 13 March 2022; Accepted: 16 May 2022;
Published: 28 June 2022.
Edited by:
Upendra Kumar, National Rice Research Institute (ICAR), IndiaReviewed by:
Rajeev Padbhushan, Bihar Agricultural University, IndiaKundan Kishore, Indian Institute of Horticultural Research (ICAR), India
Copyright © 2022 Dounas, Bourhia, Outamamat, Bouskout, Nafidi, El-Sheikh, Al-Abbadi and Ouahmane. This is an open-access article distributed under the terms of the Creative Commons Attribution License (CC BY). The use, distribution or reproduction in other forums is permitted, provided the original author(s) and the copyright owner(s) are credited and that the original publication in this journal is cited, in accordance with accepted academic practice. No use, distribution or reproduction is permitted which does not comply with these terms.
*Correspondence: Lahcen Ouahmane, bC5vdWFobWFuZUB1Y2EuYWMubWE=