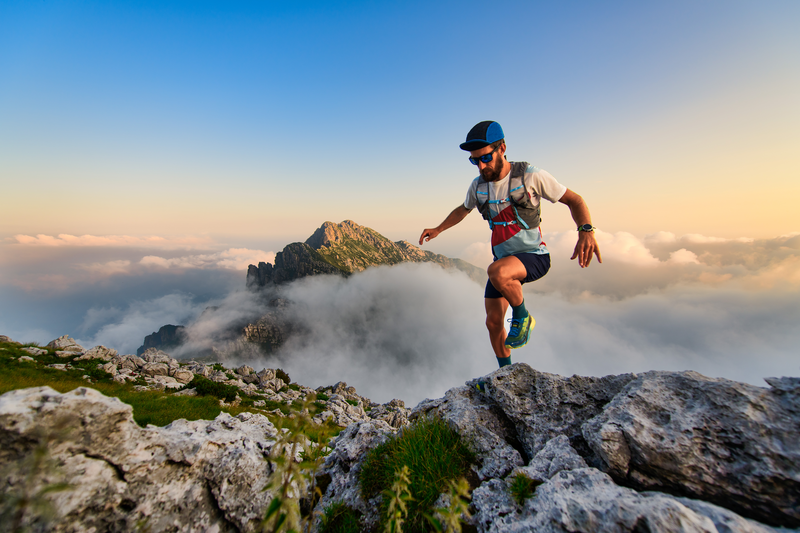
95% of researchers rate our articles as excellent or good
Learn more about the work of our research integrity team to safeguard the quality of each article we publish.
Find out more
ORIGINAL RESEARCH article
Front. Environ. Sci. , 12 May 2022
Sec. Toxicology, Pollution and the Environment
Volume 10 - 2022 | https://doi.org/10.3389/fenvs.2022.895075
This article is part of the Research Topic Novel Insights into Mercury Sources and Behavior in the Surface Earth Environment View all 5 articles
Municipal sewage has been identified as an important source of mercury (Hg) to the environment, and sewage sludge is the major sink of sewage-borne Hg. Knowledge of Hg species in sludge and potential Hg emissions during sludge treatment/disposal is still limited. A survey on Hg concentrations and species in sewage sludge of 16 provinces and municipalities in China was conducted. Hg was detected in all sewage sludge samples, with total Hg (THg) concentrations ranging from 0.3 to 7.7 mg/kg. Results from sequential chemical extractions (SCE) indicated that sludge-borne Hg mainly occurred in the form of Hg sulfide, with a small amount of organo-chelated Hg (Hg-OM) and HgO, and a negligible amount of soluble Hg. Thermal decomposition results indicated that Hg is generally released from sludge at a temperature range of 200–400°C, with the highest release at 250–350°C (38%–86%), consistent with the thermal decomposition of HgS, Hg-OM, and HgO. THg in sewage samples under ventilation at room temperature remained constant over a month period, indicating negligible Hg emission under such conditions. The mass loading of sludge-borne THg in China for year 2019 was estimated to be 30 tons, about 3.6% of the total anthropogenic THg released (including direct and secondary anthropogenic releases) in China. At the temperature range for sludge incineration, sludge carbonization, and sludge/brick/cement production, most of the Hg in sludge will be released to air due to thermal decomposition of Hg compounds. As such, Hg-capture systems are essential in sludge treatment processes involving high temperatures.
Mercury (Hg), a naturally occurring element found in air, water and soil, is considered as a hypertoxic and harmful metal to the health of humans and ecosystems (Gustin et al., 2020). The toxicity and bio-accumulative properties of Hg are well known, and different Hg species (elemental, inorganic compounds, or organic compounds) exhibit different behavior, solubility, bioavailability, routes of exposure, and toxicological effects (Reis et al., 2016). Hg emissions around the globe mainly come from two sources: natural (e.g., volcanic eruptions, erosion, etc.,) and anthropogenic (e.g., metal smelting and industrial development) (Driscoll et al., 2013; Liu et al., 2021). Primary anthropogenic Hg emissions greatly exceed the natural geogenic content, resulting in increased Hg concentrations in environmental reservoirs and significant secondary Hg emissions that contribute to its global distribution. Among various global regions, Asia has become the largest contributor of anthropogenic atmospheric Hg, responsible for over half of the global Hg emissions (Streets et al., 2019), and China alone contributes to about 840 tons (Habuer et al., 2021).
Hg ingested by humans through consumption of Hg-containing food (Wang and Mao, 2019) and exposures to daily necessities like batteries (Yang et al., 2019) would be partially excreted and enter municipal sewage. Hg from hospitals and dental clinics and some other service facilities is also a significant contributor to Hg in sewage (Singh et al., 2014). Atmospheric deposition of Hg (Gratz et al., 2013; Fu et al., 2016; Siudek et al., 2016; Chen et al., 2020) may also contribute to Hg content in sewage in combined sewer systems. Municipal sewage has become the second most important source (after industrial wastes) for the direct release of anthropogenic Hg into aquatic environments (Gbondo-Tugbawa et al., 2010; Liu et al., 2016b; Kocman et al., 2017). Considering the vast amount of global municipal sewage production, sewage-borne Hg would be an important component of the global inventory of Hg emissions. It is estimated that Hg released through sewage discharge accounted for one quarter of the global Hg release inventory (Cheng et al., 2019). However, many previous studies have shown that most Hg (∼92%) in sewage would be transferred into sewage sludge in municipal sewage treatment plants (Gbondo-Tugbawa et al., 2010; Li et al., 2022). In 2019, the annual municipal sewage treatment capacity of China reached 52.5 billion m3, with 11 million tons of dry sludge produced (MOHURD, 2020). About 29.3% of the sludge was disposed via land application, followed by incineration (26.7%) and sanitary landfills (20.1%) (Wei et al., 2020).
Most sludge-related Hg studies focused on THg in sludge and changes of THg at various stages of sewage treatment (e.g., Balogh and Liang, 1995; Gbondo-Tugbawa et al., 2010), and Hg speciation in terms of inorganic/organic Hg and dissolved/particulate-bound Hg (e.g., Bodaly et al., 1998; Stoichev et al., 2009; Liu et al., 2018a; Perusini and Hammerschmidt, 2020; Suess et al., 2020; Li et al., 2022). However, very few have examined Hg species in sludge using sequential chemical extractions (SCE) or thermal decomposition for the purpose of assessing different sludge treatment/disposal methods on Hg emissions. SCE uses appropriate reagents to determine the speciation of Hg in sediments and soils (Fernandez and Rucandio, 2013; Su et al., 2021), and has played an important role in fraction analysis of heavy metals in such media (Bacon and Davidson, 2008). Thermal decomposition, or pyrolysis, is used to distinguish different Hg species in various solid samples by their thermally induced Hg compound decomposition and release characteristics (Reis et al., 2015; Zhu et al., 2016). Because the latter method is based on the volatility of Hg compounds and the properties related to the atomic structure, results are less likely to be affected by the substrate matrix than those obtained by the SCE method, which is based on chemical interactions in the complex substrate (Rumayor et al., 2015).
The overarching goal of this study is to assess potential emissions of Hg from sewage sludge to the environment during various sewage sludge treatment/disposal processes in China, thereby providing scientific support in policy making towards the implementation of the Minamata Convention on mercury (UNEP, 2013). We collected municipal sewage sludge samples from 59 wastewater treatment plants (WWTPs) in various regions of China and performed SCE as well as thermal decomposition experiments under temperatures from 50 to 600°C (covering the temperatures of composting, drying, carbonization, and incineration of sewage sludge). A 1-month ventilation test under room temperature was also conducted to examine potential Hg emissions at temperatures similar to land applications of sewage sludge. The latest Chinese dry sludge yield and sludge Hg concentrations (from this study as well as from published literature) were then used to estimate the total potential Hg emissions from sewage sludge treatment/disposal in China.
Sewage sludge samples were collected from 59 WWTPs in 16 provinces and municipalities in China (see Supplementary Table S1 for detailed information on the WWTPs) from December 2017 to December 2019. Sampling sites were concentrated in the eastern and southern regions of China, the most developed regions with the largest population densities. Three sewage sludge samples (∼60–80% water content) were collected at each WWTP (2–3 kg per day for 3 consecutive days), composited, sealed in plastic bottles, put into insulated foam boxes with ice bags and then express-delivered to our laboratory (typically within 24 h). Once in the laboratory, samples were freeze-dried, ground and passed through a 200-mesh screen, and stored in a freezer for further analysis.
Total Hg (THg) in the sludge samples was analyzed using a Hydra II-C Hg analyzer (Leeman, United States) according to EPA Method 7473 (USEPA, 1998). Prior to testing, a multi-point calibration curve was obtained with soil mercury standards. A blank and a standard reference material (limestone soil GBW07404, GSS-4) were analyzed with every 10 sludge samples. The THg detected in GSS-4 (0.60 ± 0.03 mg/kg, n = 37) was consistent with the certified value (0.59 ± 0.05 mg/kg). Each municipal sludge sample had 5 analytical replicates and the relative standard deviations (RSDs) of the five assays were generally less than 15%. The absolute detection limit for THg was 0.01 ng.
Total nitrogen (TN) in sewage sludge was determined by ultraviolet spectrophotometry with alkaline potassium persulfate digestion, and total phosphorus (TP) was determined by molybdenum-antimony spectrophotometry with potassium persulfate digestion (Feng and Zhang, 2012; Yang et al., 2020). A total organic carbon analyzer (Shimadzu TOC-VCPH, Japan) was used to determine organic carbon (TOC) in municipal sludge.
Fractionation of Hg in the sludge samples was assessed using a seven-step SCE method proposed by Sanchez et al. (2005). This method was applied to a case study of the Almadén Hg mine area (Spain) and showed a high recovery of each extracted sample. The SCE also has been employed to study Hg species in soil samples and mine environments (Alberto et al., 2004; Sanchez et al., 2005; Bernaus et al., 2006). This method differentiates Hg compounds into water-soluble fraction (F1), exchangeable fraction (F2), carbonates fraction (F3), easily reducible fraction (F4), HCl soluble fraction (F5), oxidizable fraction (F6), and residual fraction (F7) of THg (Supplementary Table S2). The supernatant in each step was stored in a refrigerator until analysis by atomic fluorescence spectrophotometry. The calibration curve was obtained with liquid mercury standards (GSB 04-1729–2004), and the method detection limit was 0.1 μg/L. Five to six blank samples were run prior to real samples, and a blank and a standard were analyzed every 10 samples. For each sludge sample three replicates were extracted and analyzed.
A total of 26 sludge samples (2-3 per city) were analyzed using the SCE. There is a good 1:1 correlation between the sum of Hg in F1-F7 and THg concentration measured by Hydra-C (Supplementary Figure S1), indicating the robustness of the analytical methods.
Eight sludge samples (from different regions with different Hg levels) were selected to study the thermal desorption of Hg for the purpose of determining Hg species as well as potential emissions under various sludge treatment at elevated temperatures. A subsample was placed in a tube furnace (Kejin STF-1200, Hefei, China) and heated at a specified temperature (50, 100, 150, 200, 250, 300, 350, 400, 500, or 600°C) for 30 min. Preliminary tests indicated that longer treatment time would not further change the Hg content. After cooling down to room temperature, the total Hg remaining in the sample (THgT) was measured by the Hydra IIC analyzer as described previously. Triplicates were analyzed for each sample at each temperature. A subsample without heat treatment was analyzed 5 times to establish the original total Hg content (THgo). Percentage Hg release at a particular temperature was calculated as follows:
Two sludge samples were selected to conduct a 1-month ventilation test in a fume hood. During the experiment, constant room temperature and humidity were maintained. Samples were laid on petri dishes, and a subsample was analyzed every week for THg remaining in the sludge using the Hg analyzer.
The mass loadings of sludge-borne Hg were estimated based on the concentrations of THg in sludge samples and the sewage sludge production data. According to the China Construction Yearbook (MOHURD, 2020), there were 11.2 million tons of dry sewage sludge produced in 2019, and the production varied widely among provinces. The annual mass loading of THg carried by dry sewage sludge of China was calculated by the formula below:
where R is the total annual mass of THg (t) released from municipal sewage sludge of China, Ci is the concentration of THg in the dry sewage sludge of province/municipality i (mg/kg), Mi is the total annual mass (t/yr) of municipal sewage sludge produced from province/municipality i, and K is the unit conversion factor (10–6).
Total Hg in the sludge samples varied from 0.3 to 7.7 mg/kg (dry weight, Supplementary Table S1), with a median value of 0.99 mg/kg and an arithmetic mean value of 1.38 ± 1.46 mg/kg. The mean value is 66 times higher than the mean THg concentration in urban soils of China (0.021 mg/kg) (Cheng et al., 2014). Sewage sludge in Hohhot and Beijing, both in the northern region of China, showed the highest THg concentrations (4.2 ± 1.0 and 6.7 ± 0.9 mg/kg, respectively, Figure 1; Supplementary Table S1). The lowest THg concentrations were found in the sewage sludge of Ningbo (0.5 ± 0.3 mg/kg), Nanchang (0.8 ± 0.2 mg/kg), and Wuhan (0.8 ± 0.3 mg/kg). The observation of high sludge THg levels in the northern part of China and low THg in the eastern and southern regions (Figure 1) is consistent with previous studies (Liu et al., 2016a; Cheng et al., 2019). It is interesting to note that the THg concentrations in most of the providences/municipalities examined here (except for Beijing and Inner Mongolia, Supplementary Table S1) are similar to those observed in United Kingdom (median value of 0.7 mg/kg, Jones et al., 2014) and Switzerland (median value of 0.6 mg/kg, Suess et al., 2020), suggesting similar intensities of Hg sources for domestic wastewater.
FIGURE 1. Total Hg concentrations in sewage sludge of 16 Chinese cities. The number on top of each bar represents the mean THg (mg/kg, dry weight) of that city. Mean THg values for individual WWTPs can be found in Supplementary Table S1.
Most of the WWTPs in China receive domestic and industrial wastewater as well as stormwater during precipitation. As such, sources of Hg in sewage sludge include household contributions, industries, and road-deposited sediment associated with rainfall (Ma et al., 2011; Liu et al., 2021). Coal burning and metal smelting are the largest sources of anthropogenic mercury emissions in China, accounting for up to 83% of the total release (Ying et al., 2017), and most metal smelting industries and heavy coal uses occur in the northern part of China. For instance, coal-fired power plants in Inner Mongolia contributed more than 25 tons of Hg to the environment (Hui et al., 2015), higher than the contributions from many other regions. Mean total gaseous mercury concentrations were found to be 8.30 and 3.22 ng/m3 at urban and rural sites in Beijing, respectively, two orders of magnitude higher than the background concentration of the Northern Hemisphere (Zhang et al., 2013). Therefore, the high THg levels in the sludge in Inner Mongolia and Beijing are likely influenced by atmospheric deposition of mercury from coal burning and metal smelting.
Previous studies indicated that dental offices to be the largest source of Hg entering WWTPs in the United States, followed by hospitals and residential wastes (Wang and Mao, 2019). In addition, traditional medicines with a high Hg content (e.g., cinnabar and gold amalgam) are also important sources of Hg in wastewater. This has been shown to be the cause of high Hg levels in sewage in Tibet (Liu et al., 2018b). Some medical measuring devices (such as thermometers and blood pressure monitors) use a notable amount of Hg (e.g., an annual demand of more than 200 tons in China), and mercury spills from such broken devices may end up in WWTPs (Lin et al., 2016). The eastern part of China is the most developed region in the whole country, and the level of medical treatment is far more developed than that in other regions. As such, mercury associated with medical treatment is an important source of mercury in sewage sludge in eastern part of China (MEE, 2018).
In addition to the above-mentioned geographical and economic factors that may influence mercury levels in sewage sludge, we also attempted to correlate the sludge THg levels to different sewage treatment processes as well as some chemical parameters (TOC, TN, and TP). Many of the 59 WWTPs sampled in this study use the activated sludge process (ASP), with Anaerobic-Anoxic-Oxic (AAO) being the most widely used process (n = 24), followed by Oxidation Ditch (n = 7) and Anaerobic-Anoxic-Oxic in combination with the membrane bio-reactor process (AAO + MBR, n = 5). The rest of the WWTPs use the so-called Sequential Batch Reactor process (SBR, n = 4), cyclic activated sludge technology (CAST, n = 1), biological aerated filters (BAF, n = 2), Anaerobic- Oxic (AO, n = 1). A box-plot of the mercury content under various sewage treatment processes is presented in Figure 2. There are no statistical differences (one-way ANOVA, p = 0.914) of the THg levels among the different treatment processes. Previous studies showed that 92%–95% of the THg entering WWTPs was removed as sludge (55% during primary treatment), regardless of the overall treatment processes (Balogh and Nollet, 2008; Gbondo-Tugbawa et al., 2010; Li et al., 2022).
FIGURE 2. Box-plot of total Hg concentrations (mg/kg, dry weight) of different sewage treatment methods (A/A/O - Anaerobic-Anoxic-Oxic process; MBR - Membrane Bio-Reactor process; SBR - Sequential Batch Reactor process). The bottom and top of the box represent the first and third quartiles (25th and 75th percentile values), the line inside the box represents the second quartile (median), and the open square inside the box represents the average value. The whiskers extend 1.5 interquartile ranges (75th percentile value–25th percentile value) from each end of the box, and markers (solid diamond) plotted outside each whisker are considered as outliers.
There is little correlation between THg and TOC, TN, or TP (R2 = 0.031, 0.109, and 0.089, respectively, Supplementary Figures S2–S4), consistent with the general consensus that THg in sewage is controlled by the intensity of sources in each catchment rather than by the biogeochemistry of the sewage (Liu et al., 2018b; Cheng et al., 2019; Wang and Mao, 2019).
SCE results (Figure 3) show that most of the Hg (70–85%) could be extracted only by concentrated HCl (F5) or aqua regia (F7), indicating that Hg is associated with crystalline oxy-hydroxides and/or acid-soluble Hg compounds (such as HgS, Hg2Cl2, Hg-HA (Hg bound to humic acid) and Hg0 (Alberto and Marta, 2004; Sanchez et al., 2005; Terzano et al., 2010; Rumayor et al., 2013). Only in sludge samples from Beijing and Hohhot a small percentage of Hg (7% and 3%, respectively) was observed in the oxidizable fraction (F6) (Figure 3). Since these sludge samples contain the highest Hg concentrations, it is possible that reduced sulfur groups are saturated with Hg (or other metals), resulting in the presence of Hg in the oxidizable fraction. Essentially, no Hg was recovered in the labile SCE fractions (F1-F4).
FIGURE 3. Percent of Hg extracted in different fractions during sequential chemical extraction of sewage sludge samples.
Using a similar SCE procedure, Terzano et al. (2010) concluded that most of the Hg compounds in their soil samples (in F5 and F7) were meta-cinnabar (β-HgS), cinnabar (α-HgS), and corderoite (Hg3S2Cl2). Usually, HgS is associated with the residual fraction (Sanchez et al., 2005). However, Mikac et al. (2002) established that 6 M HCl can also extract both metacinnabar and cinnabar, with metacinnabar showing a higher tendency to be dissolved in this step. The same authors also found that poorly crystalline metacinnabar synthesized in the laboratory seemed to be re-dissolved more readily under high acidic conditions than the corresponding crystalline forms. Therefore, metacinnabar, especially the poorly crystalline one, may have been preferentially dissolved in F5 fraction rather than remaining in the residual (F7). This is very consistent with the poor crystallinity of sewage sludge samples. Therefore, HgS is the most probable Hg species in the sludge samples.
Although the THg concentrations in sludge from different regions of China are very different, the thermal decomposition patterns (Hg release vs. temperature) are all similar (Figure 4). Most Hg was released from sludge samples in the temperature range of 200–400°C, corresponding to the thermal decomposition temperatures of HgCl2, Hg-HA, HgS, organo-chelated Hg (Hg-OM), and HgO (Feng et al., 2004; Reis et al., 2012). However, SCE results excluded the soluble Hg species (e.g., HgCl2 and Hg-HA) as nothing was leached out in F1-F4. Therefore, the only remaining sludge Hg compounds are HgS, HgO, and Hg-OM based on the thermal decomposition experiments (Feng et al., 2004; Rumayor et al., 2015). Cheng et al. (2019) reached similar conclusions in their analysis of sewage sludge speciation in China.
FIGURE 4. Thermal release of Hg from selected sewage sludge samples. (A) from Northern China; (B) from Eastern China; (C) from Western China; (D) from Central China.
The THg concentrations of the two sludge samples remained constant in the fume hood for a month (Figure 5). One-way ANOVA tests show that there is no statistical difference between mercury concentrations at the four sampling dates (p = 0.81 and 0.54, respectively). Thermal decomposition experiments show that no Hg is released at 50°C. Together with the Hg species inferred from SCE and thermal decomposition it is safe to state that there would be minimal, if any, Hg emission from sewage sludge under well-ventilated ambient conditions.
FIGURE 5. Total Hg concentrations (mg/kg, dry weight) in selected sewage sludge samples during the ventilation test.
The total estimated amount of sludge-borne THg in China in 2019 is about 30 tons (see Supplementary Table S2 for details), twice the estimate made by Cheng et al. (2019) 3 years ago. While the increased sludge production in recent years partially explains the discrepancy, the main reason behind the discrepancy is the method of calculation. Cheng et al. (2019) used the national average of THg in sludge and national sludge production rate, whereas we used average THg in sludge and sludge production rate for each province/municipality. Geographically, the northern region accounts for 53% of the total sewage-borne Hg (Figure 6, see Supplementary Table S4 for the geographical division of China). In particular, Beijing has the largest share of sewage-borne Hg (16.3 tons in 2019), accounting for 40% of the national total. As an important anthropogenic source that has previously been ignored, THg released from municipal sewage sludge constituted 3.6% of the total anthropogenic THg released (including direct and secondary anthropogenic releases) in China (Habuer et al., 2021).
FIGURE 6. Contributions of sludge-borne total Hg from different regions of China in 2019. The total estimated amount is 30 tons for the entire country.
According to the latest statistics, 29.3% of the sludge in China was disposed via land applications, followed by incineration (26.7%), sanitary landfills (20.1%) in 2019 (Wei et al., 2020). A separate analysis by Li (2020) indicated that sludge used for construction materials accounted for 15.9% of the total sludge disposal volume in China. Due to the concerns of heavy metal pollution of soils and water resulted from land applications of sewage sludge, sludge incineration and sludge for construction materials will likely increase in the near future in China.
According to this study, Hg starts to release from sludges to air at 150–200°C. As such, Hg emissions during sludge land applications or landfills (ambient conditions) and composting (mostly 40–60°C) (Wei and Liu, 2005; Guerrini et al., 2017; Li et al., 2021; Wang et al., 2021; Xue et al., 2021) are negligible. However, if anoxic conditions develop during sludge storage, transportation, or land applications then oxidized Hg species could be reduced to Hg0, leading to volatilization of Hg0 (Selvendiran et al., 2008). Further, the risks of soil and aquatic Hg contamination, especially by the cumulative toxicity of methyl Hg, during sludge land applications are well-documented (Zhang et al., 2010; Meng et al., 2011; Strickman and Mitchell, 2017).
The temperature range for thermal hydrolysis and anerobic digestion is from 60 to 200°C (Feng et al., 2015; Barber, 2016; Cao et al., 2020), and the typical temperature range for sludge drying is around 100–200°C. A small amount of mercury in the sludge will be released to air during these sludge treatment processes (a few percent in most cases, up to ∼30%, at 200°C).
Sludge carbonization (Park and Jang, 2011; Wang et al., 2013) is popular in developed countries and is gaining attraction in China as well. Low temperature carbonization is carried out at 140–300°C (Wang et al., 2020a; Wang et al., 2020b) but higher temperatures (300–500°C) are common (Park and Jang, 2011). According to our thermal decomposition results, ∼50% of Hg in sludge will be released at 300°C and almost all the Hg will be released at 400°C.
The temperature range for sludge incineration (Marani et al., 2003; Murakami et al., 2009) or co-incineration (O’connor et al., 2019; Sun et al., 2020) is typically >850°C. At this temperature range all Hg in sludge will be released to air.
Sludge used for construction materials is often treated at 500–800°C (Weng et al., 2003; Chang et al., 2020). The temperature can reach 1,000°C in cement production (Chiou et al., 2006; Latosinska et al., 2021). Like incineration, all Hg in sludge will be released to air in brick/cement production.
While coal-fired power plants (e.g., used for sludge co-incineration) in China are regulated for gas emissions (including Hg emissions), sludge incineration, carbonization, and brick/cement production plants often do not have clear regulations for Hg emissions. As such, the latter treatment processes pose the biggest risk of atmospheric Hg pollution among all treatment methods. Thus, regulations for Hg emissions in sludge treatment under high temperatures should be set in place, and Hg capture systems used for coal-fired power plants (e.g., activated carbon injection) should be considered for such sludge treatment processes.
We measured the THg concentrations in sewage sludge samples collected from 59 WWTPs in 16 provinces and municipalities in China. Together with other sludge THg data from published literature and sludge production rate for each province/municipality, we estimated the mass loading of sludge-borne THg in China in 2019 to be 30 tons, about 3.6% of the total anthropogenic THg released (including direct and secondary anthropogenic releases) in China. We conducted sequential chemical extraction (SCE) experiments for 26 sludge samples, and found that sludge-borne Hg mainly occurred in the form of Hg sulfide, with a small amount of organo-chelated Hg (Hg-OM) and HgO, and a negligible amount of soluble Hg. We performed thermal decomposition experiments on eight sludge samples, and found that Hg generally released from sludge at the temperature range of 200–400°C, with the highest release at 250–350°C (38%–86%), consistent with the thermo-decomposition of HgS, Hg-OM, and HgO. THg in sewage samples under ventilation at room temperature remained constant over a month period, indicating negligible Hg emission under such conditions. Of the various sludge treatment/disposal methods used in China, land applications have the least potential of Hg emissions to air but pose the biggest risk for soil/aquatic contamination. Due to thermal decomposition of Hg in sludge and the lack of regulations on Hg emissions in sludge incineration, sludge carbonization, and sludge/brick/cement production facilities, these sludge treatment processes pose the highest risk of atmospheric Hg pollution.
The original contributions presented in the study are included in the article/Supplementary Material, further inquiries can be directed to the corresponding authors.
PZ and ZX conceived the work. JL, LL, KW, and RD carried out sampling, sample analysis, and data analysis. All contributed to manuscript writing.
This work was financially supported by the Ministry of Education of China and National Natural Science Foundation of China (41930532).
The authors declare that the research was conducted in the absence of any commercial or financial relationships that could be construed as a potential conflict of interest.
All claims expressed in this article are solely those of the authors and do not necessarily represent those of their affiliated organizations, or those of the publisher, the editors and the reviewers. Any product that may be evaluated in this article, or claim that may be made by its manufacturer, is not guaranteed or endorsed by the publisher.
We thank J.G. Bao (China University of Geosciences—Wuhan), Profs. X.Q. Li (Peking University) and D.S Wang (Zhejiang University) for their assistance in accessing WWTPs for sample collection, and Dr. Hui Kang for her assistance in Hg analysis.
The Supplementary Material for this article can be found online at: https://www.frontiersin.org/articles/10.3389/fenvs.2022.895075/full#supplementary-material
Alberto, J. Q., and Marta, F. (2004). “Distribution of Mercury and Trace Elements in a Soil Profile from Almadén Area,” in Proceeding of the 7th International Conference on Mercury as a Global Pollutant.
Bacon, J. R., and Davidson, C. M. (2008). Is There a Future for Sequential Chemical Extraction? Analyst 133 (1), 25–46. doi:10.1039/b711896a
Balogh, S. J., and Nollet, Y. H. (2008). Mercury Mass Balance at a Wastewater Treatment Plant Employing Sludge Incineration with Offgas Mercury Control. Sci. Total Environ. 389 (1), 125–131. doi:10.1016/j.scitotenv.2007.08.021
Balogh, S., and Liang, L. (1995). Mercury Pathways in Municipal Wastewater Treatment Plants. Water Air Soil Pollut. 80, 1181–1190. doi:10.1007/BF01189780
Barber, W. P. F. (2016). Thermal Hydrolysis for Sewage Treatment: A Critical Review. Water Res. 104, 53–71. doi:10.1016/j.watres.2016.07.069
Bernaus, A., Gaona, X., Esbrí, J. M., Higueras, P., Falkenberg, G., and Valiente, M. (2006). Microprobe Techniques for Speciation Analysis and Geochemical Characterization of Mine Environments: The Mercury District of Almadén in Spain. Environ. Sci. Technol. 40 (13), 4090–4095. doi:10.1021/es052392l
Bodaly, R. A., Rudd, W. M., and Flett, R. J. (1998). Effect of Urban Sewage Treatment on Total and Methylmercury Concentrations in Effluents. Biogeochemistry 40, 279–291. doi:10.1023/a:1005922202681
Cao, X., Tian, Y., Jiang, K., Qiu, F., and Fu, K. (2020). Evaluation of Thermal Hydrolysis Efficiency of Sewage Sludge via Rheological Measurement. J. Environ. Eng. 146 (12), 1816. doi:10.1061/(ASCE)EE.1943-7870.0001816
Chang, Z., Long, G., Zhou, J. L., and Ma, C. (2020). Valorization of Sewage Sludge in the Fabrication of Construction and Building Materials: A Review. Resour. Conservation Recycl. 154, 104606. doi:10.1016/j.resconrec.2019.104606
Chen, L., Liang, S., Zhang, H., Cai, X., Chen, Y., Liu, M., et al. (2020). Rapid Increase in Cement-Related Mercury Emissions and Deposition in China during 2005-2015. Environ. Sci. Technol. 54 (22), 14204–14214. doi:10.1021/acs.est.0c03512
Cheng, H. X., Li, K., Li, M., Tang, K., Liu, F., and Cheng, X. M. (2014). Geochemical Background and Baseline Value of Chemical Element in Urban Soil in China. Earth Sci. Front. 2014, 265–306. doi:10.13745/j.esf.2014.03.028
Cheng, L., Wang, L., Geng, Y., Wang, N., Mao, Y., and Cai, Y. (2019). Occurrence, Speciation and Fate of Mercury in the Sewage Sludge of China. Ecotoxicol. Environ. Saf. 186, 109787. doi:10.1016/j.ecoenv.2019.109787
Chiou, I.-J., Wang, K.-S., Chen, C.-H., and Lin, Y.-T. (2006). Lightweight Aggregate Made from Sewage Sludge and Incinerated Ash. Waste Manag. 26 (12), 1453–1461. doi:10.1016/j.wasman.2005.11.024
Driscoll, C. T., Mason, R. P., Chan, H. M., Jacob, D. J., and Pirrone, N. (2013). Mercury as a Global Pollutant: Sources, Pathways, and Effects. Environ. Sci. Technol. 47 (10), 4967–4983. doi:10.1021/es305071v
Feng, X., Lu, J. Y., Gregoire, D. C., Hao, Y., Banic, C. M., and Schroeder, W. H. (2004). Analysis of Inorganic Mercury Species Associated with Airborne Particulate Matter/aerosols: Method Development. Anal. Bioanal. Chem. 380 (4), 683–689. doi:10.1007/s00216-004-2803-y
Feng, G., Guo, Y., and Tan, W. (2015). Effects of Thermal Hydrolysis Temperature on Physical Characteristics of Municipal Sludge. Water Sci. Technol. 72 (11), 2018–2026. doi:10.2166/wst.2015.425
Feng, X. Z., and Zhang, L. L. (2012). Determination of Total Phosphorus in Surplus Sludge of Sewage Treatment Plant. Environ. Sci. Guide 31 (3), CN53-1205/X. doi:10.13623/j.cnki.hkdk.2012.03.028
Fernández-Martínez, R., and Rucandio, I. (2013). Assessment of a Sequential Extraction Method to Evaluate Mercury Mobility and Geochemistry in Solid Environmental Samples. Ecotoxicol. Environ. Saf. 97, 196–203. doi:10.1016/j.ecoenv.2013.07.013
Fu, X., Yang, X., Lang, X., Zhou, J., Zhang, H., Yu, B., et al. (2016). Atmospheric Wet and Litterfall Mercury Deposition at Urban and Rural Sites in China. Atmos. Chem. Phys. 16 (18), 11547–11562. doi:10.5194/acp-16-11547-2016
Gbondo-Tugbawa, S. S., Mcalear, J. A., Driscoll, C. T., and Sharpe, C. W. (2010). Total and Methyl Mercury Transformations and Mass Loadings within a Wastewater Treatment Plant and the Impact of the Effluent Discharge to an Alkaline Hypereutrophic Lake. Water Res. 44 (9), 2863–2875. doi:10.1016/j.watres.2010.01.028
Gratz, L. E., Keeler, G. J., Morishita, M., Barres, J. A., and Dvonch, J. T. (2013). Assessing the Emission Sources of Atmospheric Mercury in Wet Deposition across Illinois. Sci. Total Environ. 448, 120–131. doi:10.1016/j.scitotenv.2012.11.011
Guerrini, I. A., Croce, C. G. G., Bueno, O. d. C., Jacon, C. P. R. P., Nogueira, T. A. R., Fernandes, D. M., et al. (2017). Composted Sewage Sludge and Steel Mill Slag as Potential Amendments for Urban Soils Involved in Afforestation Programs. Urban For. Urban Green. 22, 93–104. doi:10.1016/j.ufug.2017.01.015
Gustin, M. S., Bank, M. S., Bishop, K., Bowman, K., Branfireun, B., Chételat, J., et al. (2020). Mercury Biogeochemical Cycling: A Synthesis of Recent Scientific Advances. Sci. Total Environ. 737, 139619. doi:10.1016/j.scitotenv.2020.139619
Habuer,, , Fujiwara, T., and Takaoka, M. (2021). The Response of Anthropogenic Mercury Release in China to the Minamata Convention on Mercury: A Hypothetical Expectation. J. Clean. Prod. 323, 129089. doi:10.1016/j.jclepro.2021.129089
Hui, M., Wang, Z., and Wang, S. (2015). The Mercury Mass Flow and Emissions of Coal Fired Power Plants in china. China Environ. Sci. 35 (8), 2241–2250.
Jones, V., Gardner, M., and Ellor, B. (2014). Concentrations of Trace Substances in Sewage Sludge from 28 Wastewater Treatment Works in the UK. Chemosphere 111, 478–484. doi:10.1016/j.chemosphere.2014.04.025
Kocman, D., Wilson, S., Amos, H., Telmer, K., Steenhuisen, F., Sunderland, E., et al. (2017). Toward an Assessment of the Global Inventory of Present-Day Mercury Releases to Freshwater Environments. Int. J. Environ. Res. Public Health 14, 138. doi:10.3390/ijerph14020138
Latosińska, J., Żygadło, M., and Czapik, P. (2021). The Influence of Sewage Sludge Content and Sintering Temperature on Selected Properties of Lightweight Expanded Clay Aggregate. Materials 14 (12), 3363. doi:10.3390/ma14123363
Li, Q. Y. (2020). Curren Situation and Development Trend of Urban Sludge Disposal in china Based on Carbon Emission Reduction Analysis. Harbin: MA Thesis of Harbin Institute of Technology. doi:10.27061/d.cnki.ghgdu.2020.002785
Li, Y., Sun, B., Deng, T., Lian, P., Chen, J., and Peng, X. (2021). Safety and Efficiency of Sewage Sludge and Garden Waste Compost as a Soil Amendment Based on the Field Application in Woodland. Ecotoxicol. Environ. Saf. 222, 112497. doi:10.1016/j.ecoenv.2021.112497
Li, Y., Wang, Y., Liu, R., Shao, L., Liu, X., Han, K., et al. (2022). Variation of Mercury Fractionation and Speciation in Municipal Sewage Treatment Plant: Effects of Mercury on the Atmosphere. Environ. Sci. Pollut. Res. online ahead of print. doi:10.1007/s11356-021-18103-z
Lin, Y., Wang, S., Wu, Q., and Larssen, T. (2016). Material Flow for the Intentional Use of Mercury in China. Environ. Sci. Technol. 50 (5), 2337–2344. doi:10.1021/acs.est.5b04998
Liu, M., Chen, L., Wang, X., Zhang, W., Tong, Y., Ou, L., et al. (2016a). Mercury Export from Mainland China to Adjacent Seas and its Influence on the Marine Mercury Balance. Environ. Sci. Technol. 50 (12), 6224–6232. doi:10.1021/acs.est.5b04999
Liu, M., Zhang, W., Wang, X., Chen, L., Wang, H., Luo, Y., et al. (2016b). Mercury Release to Aquatic Environments from Anthropogenic Sources in China from 2001 to 2012. Environ. Sci. Technol. 50 (15), 8169–8177. doi:10.1021/acs.est.6b01386
Liu, H., Cui, Y., Li, H., and Mao, Y. (2018a). A Case Study on the Occurrence, Transport, and Fate of Mercury Species in a Sewage Treatment Plant in Jiaozuo, China. Environ. Sci. Pollut. Res. 25, 21616–21622. doi:10.1007/s11356-018-2312-8
Liu, M., Du, P., Yu, C., He, Y., Zhang, H., Sun, X., et al. (2018b). Increases of Total Mercury and Methylmercury Releases from Municipal Sewage into Environment in China and Implications. Environ. Sci. Technol. 52 (1), 124–134. doi:10.1021/acs.est.7b05217
Liu, K., Wu, Q., Wang, S., Ouyang, D., Li, Z., Ding, D., et al. (2021). Highly Resolved Inventory of Mercury Release to Water from Anthropogenic Sources in China. Environ. Sci. Technol. 55 (20), 13860–13868. doi:10.1021/acs.est.1c03759
Ma, M. X., Weng, H. X., and Zhang, J. J. (2011). Regional Characteristics and Trend of Heavy Metals and Nutrients of Sewage Sludge in China. China Environ. Sci. 31 (8), 1306–1313. (in Chinese)
Marani, D., Braguglia, C. M., Mininni, G., and Maccioni, F. (2003). Behaviour of Cd, Cr, Mn, Ni, Pb, and Zn in Sewage Sludge Incineration by Fluidised Bed Furnace. Waste Manag. 23 (2), 117–124. doi:10.1016/s0956-053x(02)00044-2
MEE (2018). Ministry of Ecology and Environment of the People's Republic of China. Beijing: China Environmental Annual Report. Available from: https://www.mee.gov.cn/hjzl/sthjzk/sthjtjnb/ (Accessed Feb 20, 2022).
Meng, B., Feng, X., Qiu, G., Liang, P., Li, P., Chen, C., et al. (2011). The Process of Methylmercury Accumulation in Rice (Oryza Sativa L.). Environ. Sci. Technol. 45 (7), 2711–2717. doi:10.1021/es103384v
Mikac, N., Foucher, D., Niessen, S., and Fischer, J.-C. (2002). Extractability of HgS (Cinnabar and Metacinnabar) by Hydrochloric Acid. Anal. Bioanal. Chem. 374 (6), 1028–1033. doi:10.1007/s00216-002-1522-5
MOHURD (2020). Ministry of Housing and Urban-Rural Development of the People's Republic of China. Available from: https://www.mohurd.gov.cn/gongkai/index.html (Accessed Feb 20, 2022).
Murakami, T., Suzuki, Y., Nagasawa, H., Yamamoto, T., Koseki, T., Hirose, H., et al. (2009). Combustion Characteristics of Sewage Sludge in an Incineration Plant for Energy Recovery. Fuel Process. Technol. 90 (6), 778–783. doi:10.1016/j.fuproc.2009.03.003
O'connor, D., Hou, D., Ok, Y. S., Mulder, J., Duan, L., Wu, Q., et al. (2019). Mercury Speciation, Transformation, and Transportation in Soils, Atmospheric Flux, and Implications for Risk Management: A Critical Review. Environ. Int. 126, 747–761. doi:10.1016/j.envint.2019.03.019
Park, S.-W., and Jang, C.-H. (2011). Characteristics of Carbonized Sludge for Co-combustion in Pulverized Coal Power Plants. Waste Manag. 31 (3), 523–529. doi:10.1016/j.wasman.2010.10.009
Perusini, H. B., and Hammerschmidt, C. R. (2020). Temporal Variation of Mercury in Effluent from Two Municipal Wastewater‐Treatment Plants in Southwest Ohio. Environ. Toxicol. Chem. 39 (5), 1027–1031. doi:10.1002/etc.4692
Reis, A. T., Coelho, J. P., Rodrigues, S. M., Rocha, R., Davidson, C. M., Duarte, A. C., et al. (2012). Development and Validation of a Simple Thermo-Desorption Technique for Mercury Speciation in Soils and Sediments. Talanta 99, 363–368. doi:10.1016/j.talanta.2012.05.065
Reis, A. T., Coelho, J. P., Rucandio, I., Davidson, C. M., Duarte, A. C., and Pereira, E. (2015). Thermo-desorption: A Valid Tool for Mercury Speciation in Soils and Sediments? Geoderma 237-238, 98–104. doi:10.1016/j.geoderma.2014.08.019
Reis, A. T., Davidson, C. M., Vale, C., and Pereira, E. (2016). Overview and Challenges of Mercury Fractionation and Speciation in Soils. TrAC Trends Anal. Chem. 82, 109–117. doi:10.1016/j.trac.2016.05.008
Rumayor, M., Diaz-Somoano, M., Lopez-Anton, M. A., and Martinez-Tarazona, M. R. (2013). Mercury Compounds Characterization by Thermal Desorption. Talanta 114, 318–322. doi:10.1016/j.talanta.2013.05.059
Rumayor, M., Diaz-Somoano, M., Lopez-Anton, M. A., and Martinez-Tarazona, M. R. (2015). Application of Thermal Desorption for the Identification of Mercury Species in Solids Derived from Coal Utilization. Chemosphere 119, 459–465. doi:10.1016/j.chemosphere.2014.07.010
Sánchez, D. M., Quejido, A. J., Fernández, M., Hernández, C., Schmid, T., Millán, R., et al. (2005). Mercury and Trace Element Fractionation in Almaden Soils by Application of Different Sequential Extraction Procedures. Anal. Bioanal. Chem. 381 (8), 1507–1513. doi:10.1007/s00216-005-3058-y
Selvendiran, P., Driscoll, C. T., Montesdeoca, M. R., and Bushey, J. T. (2008). Inputs, Storage, and Transport of Total and Methyl Mercury in Two Temperate Forest Wetlands. J. Geophys. Res. 113, a–n. doi:10.1029/2008JG000739
Singh, R. D., Jurel, S. K., Tripathi, S., Agrawal, K. K., and Kumari, R. (2014). Mercury and Other Biomedical Waste Management Practices Among Dental Practitioners in India. BioMed Res. Int. 2014, 1–6. doi:10.1155/2014/272750
Siudek, P., Kurzyca, I., and Siepak, J. (2016). Atmospheric Deposition of Mercury in Central Poland: Sources and Seasonal Trends. Atmos. Res. 170, 14–22. doi:10.1016/j.atmosres.2015.11.004
Stoichev, T., Tessier, E., Garraud, H., Amouroux, D., Donard, O. F. X., and Tsalev, D. L. (2009). Mercury Speciation and Partitioning along a Municipal Sewage Treatment Plant. J. Balkan Ecol. 12, 135–145.
Streets, D. G., Horowitz, H. M., Lu, Z., Levin, L., Thackray, C. P., and Sunderland, E. M. (2019). Global and Regional Trends in Mercury Emissions and Concentrations, 2010-2015. Atmos. Environ. 201, 417–427. doi:10.1016/j.atmosenv.2018.12.031
Strickman, R. J., and Mitchell, C. P. J. (2017). Accumulation and Translocation of Methylmercury and Inorganic Mercury in Oryza Sativa: An Enriched Isotope Tracer Study. Sci. Total Environ. 574, 1415–1423.doi:10.1016/j.scitotenv.2016.08.068
Su, Y., Liu, X., Teng, Y., and Zhang, K. (2021). Mercury Speciation in Various Coals Based on Sequential Chemical Extraction and Thermal Analysis Methods. Energies 14 (9), 2361. doi:10.3390/en14092361
Suess, E., Berg, M., Bouchet, S., Cayo, L., Hug, S. J., Kaegi, R., et al. (2020). Mercury Loads and Fluxes from Wastewater: A Nationwide Survey in Switzerland. Water Res. 175, 115708. doi:10.1016/j.watres.2020.115708
Sun, Y., Chen, G., Yan, B., Cheng, Z., and Ma, W. (2020). Behaviour of Mercury during Co-incineration of Sewage Sludge and Municipal Solid Waste. J. Clean. Prod. 253, 119969. doi:10.1016/j.jclepro.2020.119969
Terzano, R., Santoro, A., Spagnuolo, M., Vekemans, B., Medici, L., Janssens, K., et al. (2010). Solving Mercury (Hg) Speciation in Soil Samples by Synchrotron X-Ray Microspectroscopic Techniques. Environ. Pollut. 158 (8), 2702–2709. doi:10.1016/j.envpol.2010.04.016
UNEP (2013). Global Mercury Assessment: Sources, Emissions, Releases and Environmental. Available from: https://www.unep.org/resources/report/minamata-convention-mercury (Accessed March 10, 2022).
USEPA (1998). Method 7473 (SW-846): Mercury in Solids and Solutions by Thermal Decomposition. Washington, DC: Amalgamation, and Atomic Absorption Spectrophotometry.
Wang, X., and Mao, Y. (2019). Mercury in Municipal Sewage and Sewage Sludge. Bull. Environ. Contam. Toxicol. 102 (5), 643–649. doi:10.1007/s00128-018-02536-3
Wang, N.-Y., Shih, C.-H., Chiueh, P.-T., and Huang, Y.-F. (2013). Environmental Effects of Sewage Sludge Carbonization and Other Treatment Alternatives. Energies 6 (2), 871–883. doi:10.3390/en6020871
Wang, W., Chen, W. H., and Jang, M. F. (2020a). Characterization of Hydrochar Produced by Hydrothermal Carbonization of Organic Sludge. Future Cities Environ. 6 (1), 102. doi:10.5334/fce.102
Wang, Z., Zhai, Y., Wang, T., Peng, C., Li, S., Wang, B., et al. (2020b). Effect of Temperature on the Sulfur Fate during Hydrothermal Carbonization of Sewage Sludge. Environ. Pollut. 260, 114067. doi:10.1016/j.envpol.2020.114067
Wang, Z., Wu, D., and Lin, Y. (2021). Role of Temperature in Sludge Composting and Hyperthermophilic Systems:a Review. Bioenerg. Res. doi:10.1007/s12155-021-10281-5
Wei, L., Zhu, F., Li, Q., Xue, C., Xia, X., Yu, H., et al. (2020). Development, Current State and Future Trends of Sludge Management in China: Based on Exploratory Data and CO2-equivaient Emissions Analysis. Environ. Int. 144, 106093. doi:10.1016/j.envint.2020.106093
Wei, Y., and Liu, Y. (2005). Effects of Sewage Sludge Compost Application on Crops and Cropland in a 3-year Field Study. Chemosphere 59 (9), 1257–1265. doi:10.1016/j.chemosphere.2004.11.052
Weng, C.-H., Lin, D.-F., and Chiang, P.-C. (2003). Utilization of Sludge as Brick Materials. Adv. Environ. Res. 7 (3), 679–685. doi:10.1016/s1093-0191(02)00037-0
Xue, S., Zhou, L., Zhong, M., Kumar Awasthi, M., and Mao, H. (2021). Bacterial Agents Affected Bacterial Community Structure to Mitigate Greenhouse Gas Emissions during Sewage Sludge Composting. Bioresour. Technol. 337, 125397. doi:10.1016/j.biortech.2021.125397
Yang, L., Li, K., Cui, S., Kang, Y., An, L., and Lei, K. (2019). Removal of Microplastics in Municipal Sewage from China's Largest Water Reclamation Plant. Water Res. 155, 175–181. doi:10.1016/j.watres.2019.02.046
Yang, T., Yin, C., Wan, Q. L., and Bai, X. (2020). Determination of Total Nitrogen in Municipal Sludge by Ultraviolet Spectrophotometry with Alkaline Potassium Persulfate Digestion. Yunnan Chem. Technol. 47 (1), 29. doi:10.3969/j.issn.1004-275X.2020.001.029
Ying, H., Deng, M. H., Li, T. Q ., Jan, J. P. G., Chen, Q. Q., Yang, X. E ., et al. (2017). Anthropogenic Mercury Emissions from 1980 to 2012 in China. Environ. Pollut. 226, 230–239. doi:10.1016/j.envpol.2017.03.059
Zhang, H., Feng, X., Larssen, T., Qiu, G., and Vogt, R. D. (2010). In Inland China, Rice, rather Than Fish, Is the Major Pathway for Methylmercury Exposure. Environ. Health Perspect. 118 (9), 1183–1188. doi:10.1289/ehp.1001915
Zhang, L., Wang, S. X., Wang, L., and Hao, J. M. (2013). Atmospheric Mercury Concentration and Chemical Speciation at a Rural Site in Beijing, China: Implications of Mercury Emission Sources. Atmos. Chem. Phys. 13 (20), 10505–10516. doi:10.5194/acp-13-10505-2013
Keywords: mercury, sewage sludge, speciation, sequential chemical extractions, thermal decomposition
Citation: Liu J, Lin L, Wang K, Ding R, Xie Z and Zhang P (2022) Concentrations and Species of Mercury in Municipal Sludge of Selected Chinese Cities and Potential Mercury Emissions From Sludge Treatment and Disposal. Front. Environ. Sci. 10:895075. doi: 10.3389/fenvs.2022.895075
Received: 12 March 2022; Accepted: 25 April 2022;
Published: 12 May 2022.
Edited by:
Yi Liu, Tianjin University, ChinaReviewed by:
Nicholas Kiprotich Cheruiyot, Cheng Shiu University, TaiwanCopyright © 2022 Liu, Lin, Wang, Ding, Xie and Zhang. This is an open-access article distributed under the terms of the Creative Commons Attribution License (CC BY). The use, distribution or reproduction in other forums is permitted, provided the original author(s) and the copyright owner(s) are credited and that the original publication in this journal is cited, in accordance with accepted academic practice. No use, distribution or reproduction is permitted which does not comply with these terms.
*Correspondence: Zhouqing Xie, enF4aWVAdXN0Yy5lZHUuY24=; Pengfei Zhang, cHpoYW5nQGNjbnkuY3VueS5lZHU=
Disclaimer: All claims expressed in this article are solely those of the authors and do not necessarily represent those of their affiliated organizations, or those of the publisher, the editors and the reviewers. Any product that may be evaluated in this article or claim that may be made by its manufacturer is not guaranteed or endorsed by the publisher.
Research integrity at Frontiers
Learn more about the work of our research integrity team to safeguard the quality of each article we publish.