- 1Marine Biology Branch, Zoology Department, Faculty of Science, Al-Azhar University, Cairo, Egypt
- 2Department of Biology, College of Science, Taif University, Taif, Saudi Arabia
Despite wide studies of biomonitoring aquatic environment through dynamics of host–parasite interaction, bio-indicators to track the influence and accumulation of heavy metals on fish are still few. The present study sheds light on the relation between fish parasites and heavy metals as it threatens fish’s health and, as a consequence, that of humans after fish consumption. Samples of Nile tilapia (Oreochromis niloticus) were collected in Burullus Lake, a wild fish source, and from a private fish farm in Kafr El-Sheikh Governorate, in Egypt. They were exposed to various pollutants associated with anthropogenic activities to determine the levels of accumulation of Fe, Zn, and Cu, along with the top three most toxic metals (As, Cd, and Pb) in water and fish tissues of gills, intestine, liver, and muscles in both wild and farmed O. niloticus. The results showed the order of abundance: Fe < Zn < Pb < Cu < As < Cd. In waters of both farmed and wild fish, there was a significant negative relation between parasite prevalence and heavy metals, including Zn, Pb, and As. Also, there was a significant positive relation between parasite prevalence with Cu while no significant relation was found with Fe and Cd. Heavy metal content was significantly higher (p > 0.05) in non-infected than infected farmed and wild O. niloticus. In addition, a significantly decreased concentration (p > 0.05) of essential heavy metal was recorded in wild fish compared to farmed specimens, while non-essential heavy metal was significantly higher (p > 0.05) in wild compared to farmed fish. Bioaccumulation factors (BAF) of different organs of O. niloticus were ranked in ascending order: Liver > Gills > Intestine > Muscles. In general, the risk assessment showed safe human consumption of farmed and wild fish under the reported environmental conditions in this study. Moreover, the parasite's presence can be adopted as a surrogate indicator to estimate the potential impact of heavy metal pollution and accumulation.
Introduction
Aquaculture has grown to become a major source of low-cost protein in many countries globally, and production has doubled in the last 10 years, especially in Egypt, where aquaculture accounts for more than 80% of Egypt’s total fish production (GAFRD, 2019; David et al., 2021). In poor countries, fish provides up to approximately 30% of the total animal protein consumed per capita (Wang et al., 2015). Nile tilapia, Oreochromis niloticus, is considered the most important and well-known farmed fish in Egypt and Africa (Authman et al., 2012; Abdel-hakim et al., 2016). It has grown more than twenty-fold in the last 20 years (Henriksson et al., 2017).
Due to their environmental persistence and incapacity to dissolve, metals are considered a particular cause of concern across the world (Abah et al., 2016; Jiang et al., 2018). Contaminants are accumulated in aquatic species, such as fish, either directly from contaminated water or indirectly through the food chain (Abah et al., 2016; Ahmed et al., 2020). Some heavy metals are important for fish metabolism within accepted ranges, but when their quantities exceed these ranges, they become extremely hazardous (Keke et al., 2015; Padrilah et al., 2018). Fish uptake the heavy metals directly from the water via their skin and gills, as well as through the consumption of contaminated food (Hassan et al., 2018). As such, the concentration of metal in a fish tissue depends on its amount in the water and its prey (Mousa et al., 2015). With the capability of metals to bioaccumulate within the fish organs, these may constitute a health risk for consumers (Tytła, 2019). It is vital to estimate the risks of metals on human health as a result of targeted fish intake (Salam et al., 2019).
Concentrations of heavy metals do not have to reach high levels in the organism's body to be dangerous, since even if accumulated in low quantities in the body tissues, they can exceed the allowed limits (Sardar et al., 2013). As a result, the consequences of heavy metals’ negative effects on fish can be exploited in biomonitoring freshwater environments. In parallel, the quality of fish living in environments subject to agricultural drainage and the fresh water of the Nile should be examined to assess their acceptability and safety for human consumption.
Metals, such as Cu, Mn, Zn, and Fe, are classified as essential for enzyme functioning and play vital roles in a variety of biological mechanisms. Nonetheless, when an organism assimilates them in high quantities over long periods, they can become poisonous. In parallel, Cr, Pb, and Cd are categorized as non-essential metals as they have no biological significance for living creatures. Furthermore, even in small amounts, they are hazardous (Ju et al., 2017; Mehana et al., 2020).
Fish consumption is a small part of the overall human diet, whereas the risk range provided by the US Environmental Protection Agency (EPA) is for the total dietary intake of metals. However, the metal accumulation risk through fish consumption should not be ignored. Furthermore, given harmful metals' non-biodegradability and probable accumulation in fish tissues, metal supplementation in fish feed should be reduced, and fish should be monitored regularly to help reduce the risk of non-essential metal poisoning for consumers (Resma et al., 2020).
Analyses of fish-parasite-metals interactions have been proposed as an efficient monitoring method for assessing the health of the environmental fish ecosystem, with parasites indicating the presence of various contaminants in aquatic habitats, including toxic metals and sewage pollutants (Mehana et al., 2020).
Indeed, fish parasites help their hosts survive in heavy metal-polluted environments by collecting larger quantities of heavy metals and therefore acting as metal sinks (Eissa et al., 2012).
This study intended to explore the following:
(1) The metal content in water, gills, intestines, livers, and edible tissues of both farmed and wild Oreochromis niloticus;
(2) The potential human health risks associated with the consumption of both farmed and wild fish with the use of combined indices;
(3) The role and relationships between heavy metal accumulation and fish parasite infestation in monitoring ecosystem pollutions.
Moreover, by examining the possible relationship between parasites and heavy metals as bio-monitors of pollution, the current study fills a significant gap in the ecotoxicology field.
Material and Methods
Collection of Fish and Water Samples
Water samples were taken from two different locations. The first location was Burullus Lake (lake origin), while the second was a private fish farm in the governorate of Kafr El-Sheikh in Egypt (Farmed origin) (Figure 1). A total of 538 Nile Tilapia specimens, O. niloticus, were taken, 324 males and 214 females, with a standard length of 17.48 ± 6.87 cm and an average weight of 236.26 ± 14.35 g. Fish were taken simultaneously with the water samples from the same location and transported to the Marine Biology Department of the Faculty of Science at Al-Azhar University in Egypt.
Physico-Chemical Parameters
Physico-chemical parameters (pH, turbidity, TDS, ammonia, NO2, NO3, total alkalinity, total hardness, total phosphate, H2S, and dissolved oxygen) were measured in the water of Burullus Lake and the private fish farm according to procedures outlined by the American Public Health Association (APHA, 2005). The pH levels were determined using a digital pH meter. Total dissolved solids were calculated using a digital TDS meter (TDS). Ammonia (NH3), nitrite (NO2), nitrate (NO3), and total phosphate levels were measured using colorimetric methods. Water alkalinity was precisely evaluated following sample collection using phenolphthalein and methyl orange as indicators. The Winkler method was used to analyze dissolved oxygen and titration methods were utilized to estimate H2S.
Length-Weight Relationship
After collection, the standard length of each fish was measured to the nearest millimeter. The body weight was also determined to the nearest 0.1 g. The Length-weight relationship was determined by using power equation or its logarithmic modification according to the following method:
(Lagler, 1956).where W is the weight of the fish in grams; L is the standard length in centimeter; and a and b are constants, whose values are estimated by the least square method.
By grouping the fish in 10 mm length groups, the empirical and calculated weights were determined.
Parasitological Assessments
Specimens were examined in the laboratory. The gills, intestines, muscles, and liver were isolated. After incisions on the ventral side, the excised organs were initially checked externally for parasites with a magnifying glass. The alimentary tracts were then isolated under a stereomicroscope in 0.09% sterile saline for parasite extraction (Marcogliese and Pietrock, 2011). According to Sohn’s (2009) procedures, 1 g of each tissue was placed between two glass slides and investigated for the detection of encysted metacercariae using a binocular dissecting microscope. Distinct parasites were carefully removed, and morphological features were utilized to differentiate them at the species level. Their proportions were documented, as well as whether they appeared alone or in clusters. They were not tarnished when they were photographed (Elsheikha and Elshazly, 2008; El-Shahawy et al., 2017). However, the approaches described in Yamaguti (1963), Cheng (1973), and Radwan (2022) were used to identify helminths. Cestodes, nematodes, and trematodes were relaxed in warm water for 6 h before being fixed in 5% formalin and transferred to 70% ethanol (Oros et al., 2010).
According to Radwan et al. (2021) and El-Shahawy et al. (2017), the specimens were stained with acetic acid alum carmine in 70% acid ethanol, dehydrated in ascending concentrations of ethanol, cleaned with clove oil, and permanently mounted in Canada balsam for microscopic examination. Parasites prevalence (PP) and intensity (IN) in fish were estimated according to the following formula:
where FPP (%) denotes fish PP; No. HI represents the number of individuals of a host species infected with a particular parasite species; and No. HE indicates the number of hosts investigated.
where IN is the intensity; TNPS is the total number of parasite individuals in a sample of host species; and NIHS is the number of infected hosts in the sample.
Heavy Metals Analysis
Sample Digestion
The studied organs (intestine, gills, liver, and muscles) of infected and non-infected fish were selected for heavy metals analysis after parasitological inspection. The organs (0.5 g each) were treated with 5 ml of concentrated nitric acid, warmed on the hot plate at 100°C for 10 h, cooled at room temperature, and thoroughly digested. The digested sample was transferred to a volumetric flask, and the volume was diluted to a final volume of 50 ml with deionized water. Heavy metals in water samples were determined using an acid digestion technique for total metals. The digested solution was poured into a volumetric flask and then diluted to a final volume of 100 ml with deionized water. After that, the diluted solution of organs and water samples were tested (AOAC, 2012).
ICP Analysis
Heavy metal (zinc Zn, cadmium Cd, copper Cu, iron Fe, lead Pb, and arsenic As) levels were measured in diluted solutions of tissues and water samples. The heavy metals levels in the serial dilutions (water and fish samples, n = 5) were determined using an inductively coupled plasma optical emission spectrophotometer (ICP-OES, Model 4300 DV, Perkin Elmer, Shelton, CT, United States). To estimate the ppm of each analysis in the digested solution, samples were treated with a multi-element standard solution containing 1 μg/L of each metal used for calibration curve calculation. The Quality Control sample was analyzed every ten samples to ensure that the calibration accuracy and instrument drift were both within acceptable limits (within 20% of the known QC values acceptable). In each analytical batch, blank samples were run in duplicate in random order and utilized to calculate the technique detection limit. The heavy metals levels in the water samples were measured in mg/L and the fish samples in mg/kg on a wet weight basis (Abbas et al., 2021).
Bioaccumulation Factor Calculation
The BAF is the ratio between heavy metals deposited in fish tissues and in the water. It was calculated using the following equation:
(Adolfsson-Erici et al., 2012).where BAF is the bioaccumulation factor, C-fish is the heavy metal levels in fish organs (ppm), and C-water is the heavy metal levels in water (ppm).
Human Risk Assessment Calculation
To assess the human risk of heavy metals absorption through the ingestion of the analyzed fish, the approach provided by the US Environmental Protection Agency was adopted (USEPA 2018). The average daily dosage (EDI; an average daily intake of a given chemical over a lifetime) determined the degree of exposure produced by oral human ingestion of certain heavy metals found in fish tissues. The average daily dose (ADD) was calculated using the following equation, which is given as mg−1 kg−1 day−1.
(Mwakalapa et al., 2019).where CF is the average heavy metals concentration in fish muscle—mg/kg wet wt.; IR refers to the daily intake (DI) of fish consumed (kg/day), which in this study was considered to be 7.9 g/day for children (age 6–11 years old) and 20.1 g/day for adults; ER is the exposure rate (365 days/year); EP is the exposure period over a lifetime (suspected to be 70 years old); BW is the body weight, which for adults was considered to be 70 kg (Mannzhi et al., 2021) and for children 6–11 years old 52.5 kg body weight which refers to the 95th percentile (USEPA, 2008); AT is the average lifetime (70 years × 365 days/year).
The target hazard quotient (THQ), a non-cancer indicator of unfavorable health consequences due to the ingestion of particular heavy metals contaminants in fish samples, was used to quantify human risk. THQ was computed using the equation below, which was derived from the proportion of ADD to ORD (oral reference dose of heavy metals).
(USEPA, 2018). where ORD refers to the oral reference doses of heavy metals (mg/kg/days) for an adult person weighing 70 kg, it’s recommended as an upper limit of heavy metals oral consumption. ORDs for Cu, Pb, Zn, Cd, As, and Fe are 0.04, 0.00357, 0.3, 0.001, 0.0003, and 0.7 mg/kg/day, respectively (USEPA, 2018). When the target hazard quotient (THQ) falls below 1.0, it indicates that unfavorable health effects on people are improbable. If the calculated THQ is greater than 1.0, people should expect unfavorable health consequences.
The hazard index (HI) is a scientific formula that measures the effect of non-carcinogenic hazards by the sum of THQ values of the metals under investigation as follows:
(USEPA, 2011). When the HI value is higher than 10, the exposed persons have a higher non-carcinogenic risk.
Data Analysis
The normal distribution and homogeneity of variance were demonstrated using Levene’s test. The statistical analysis was performed using IBM, SPSS Statistical Program, Version 22; SPSS Inc., IL, United States. The one-way ANOVA was applied and when significant differences were found, multivariate, post hoc Tukey evaluations were utilized to quantify the statistical difference between the heavy metals’ levels in various fish organs for each metal. The correlations between physico-chemical parameters and heavy metal levels in farmed and wild fish were investigated using Pearson’s correlation coefficient. The T-test was used to compare infected and non-infected fish, and the statistical significance was established at p < 0.05.
Results
Water Quality
Data indicated that fish farm waters had significantly higher levels of ammonia (mg/L), pH, turbidity (%), nitrogen dioxide (mg/L), TDS (mg/L), nitrate (mg/L), total alkalinity (mg/L), total hardness (mg/L), phosphate (mg/L), and hydrogen sulfide (mg/L) compared to the water of Burullus Lake. The only exception to this pattern was the dissolved oxygen (mg/L). On the contrary, Fe, Zn, Pb, Cu, and As levels in Burullus Lake waters were much lower than in fish farm waters. Concentrations of heavy metals in Burullus Lake and fish farm water were reported in this order: Fe, Zn, Pb, Cu, As, and Cd (Table 1, 2).
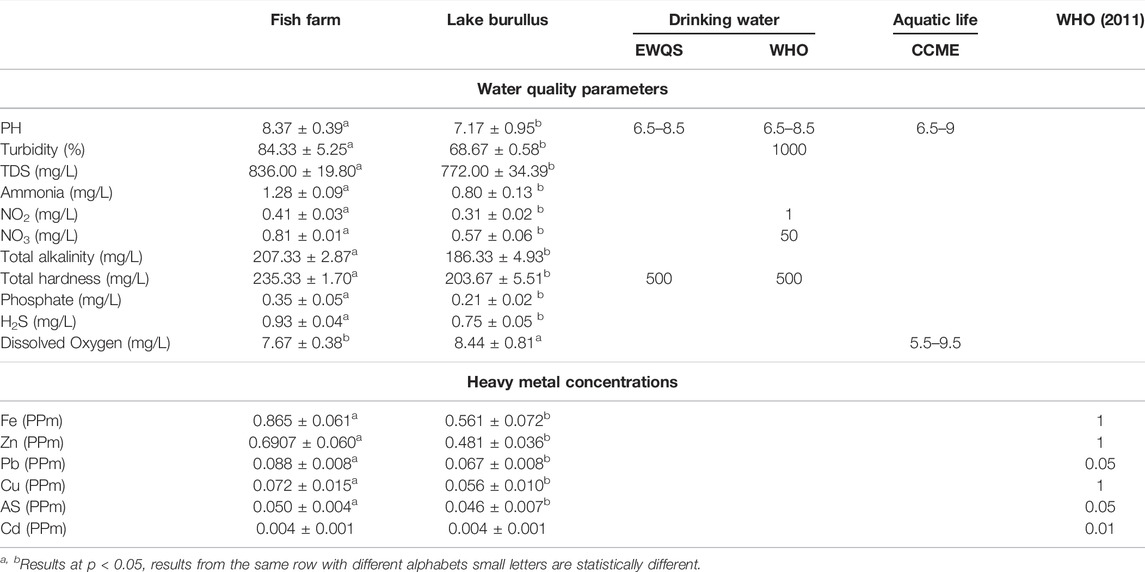
TABLE 1. Water quality parameters and heavy metal concentrations of the water collected from fish farm and Burullus Lake. At p < 0.05, results from the same row with different alphabetic letters are statistically different.
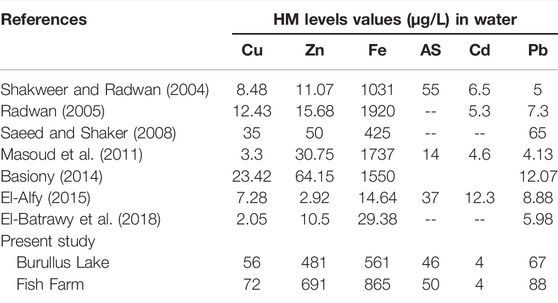
TABLE 2. Comparison between HMs levels of (μg/L = ppb) in Burullus Lake water of the present study with the previous studies.
Length-Weight Relationship of Uninfected and Infected Fish
Figure 2A–D shows the length-weight relationship of O. niloticus fish and its relationship with parasite infection. The wild and farmed infected and non-infected O. niloticus specimens exhibited b values ranging from 2.56 to 2.92. The value of “b” was less than 3 in the infected and non-infected fish of both wild and farmed fish, indicating a negative allometric growth pattern for fish regardless of their infection status. Nearly, all species’ length and weight had a strong and very significant correlation (r > 0.96).
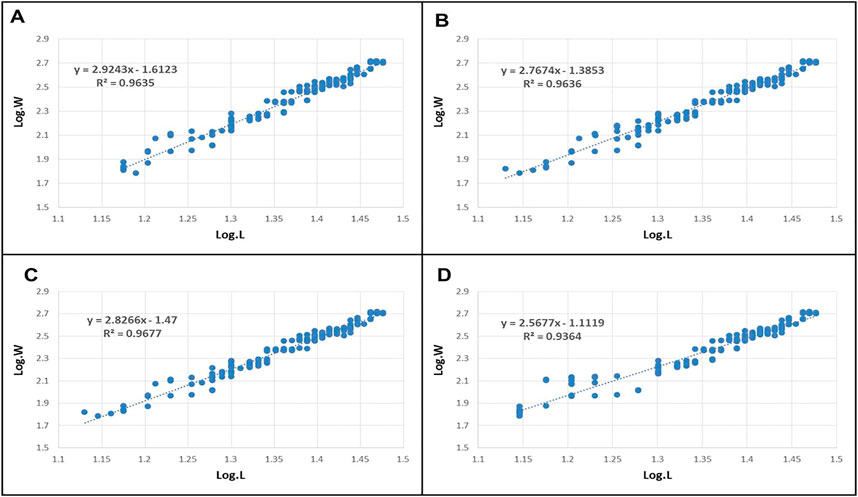
FIGURE 2. Length-weight relationship of lake and farmed Nile Tilapia, O. niloticus. (A): Non-infected farmed fish (B): Infected farmed fish; (C): Non-infected lake fish (D): Infected lake fish.
Parasitological Outcomes
The parasitic infection rate was 71.34% in farmed fish, while in wild fish it was 61.16%. Furthermore, farmed fish infection was lower in the liver (11.78%), increased gradually in the gills (14.65%), and intestine (16.88%), and reached a higher rate (28.03%) in muscle tissue. The parasitic infection in different organs in lake fish fluctuated between (9.82%) in the liver and (21.43%) in muscles. Farmed fish organs were more susceptible to infection than lake fish. Moreover, organs were ordered as muscles, gills, liver, and intestine according to the ratio of infection (Table 3).
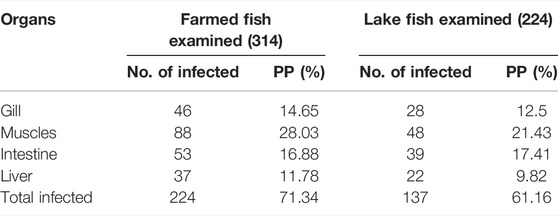
TABLE 3. Number of infected specimens of O. niloticus considering the different analyzed organs and their percentage on the total number of fish examined.
Table 4 showed that the prevalence of protozoa was higher in farmed O. niloticus fish (14.01%) in comparison to lake fish (7.59%). Trematodes have fluctuated between 31.53% in farmed fish and 31.70% in lake fish. The prevalence of cestodes was 4.14% in farmed fish and 4.91% in lake fish, respectively. Furthermore, the highest prevalence of Acanthocephala was found in lake fish, while the lowest rate was found in farmed fish, at 21.66% and 16.96%, respectively.
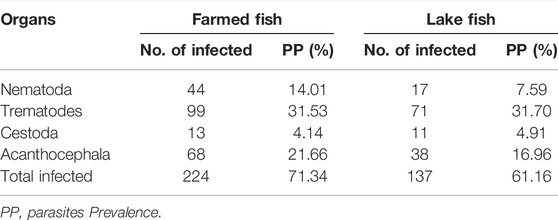
TABLE 4. Prevalence of different parasites species (PP) among the examined fish O. niloticus, collected from Fish Farm and Burullus Lake.
A total of 13 parasites, were isolated from the examined fish. Platyhelminthes, two trematodes (Cichlidogyrus tilapiae, Enterogyrus cichlidarum), one cestode (Procercoid and plerocercoid stage of Polyonchobothrium clarias), two nematodes (Procamallanus sp and Paracamallanus sp), one acanthocephala (Acanthosentis tilapiae), were found, as well as seven species of encysted metacercariae Centrocestusn formosanus, Heterphyes sp., Pygidiopsis genata, Diplostomum tilapiae, Cyanodiplostomum sp., Opisthorchis sp., Prohemistomum sp., (Table 5 and Figure 3A–O).
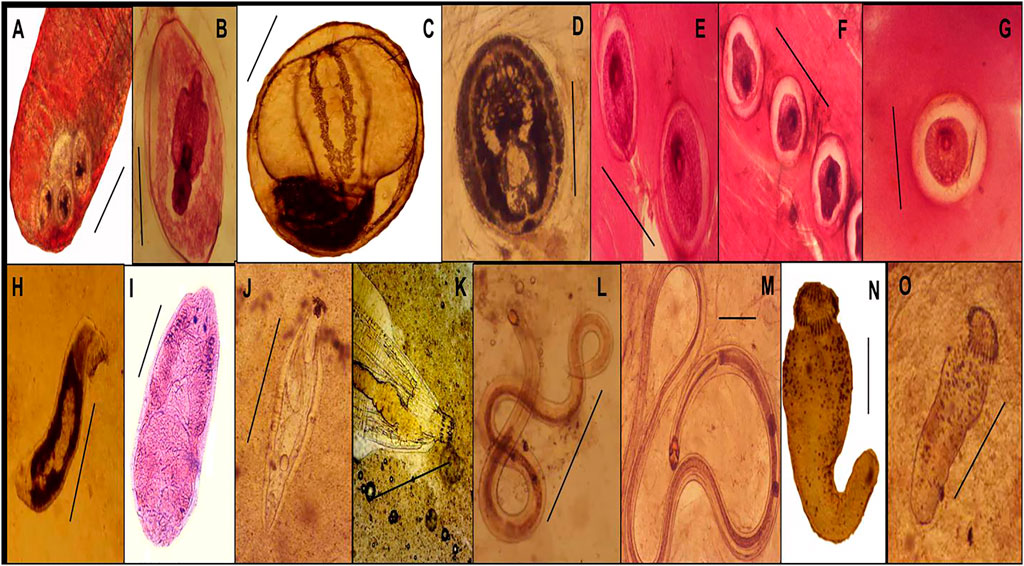
FIGURE 3. Encysted metacercariae (EMC) of identified infected fishes (Scale bar = 50 µm). (A): Centrocestus formosanus. (B): Diplostomum tilapiae stained with acetic acid alum carmine. (C): Opisthorchis sp. (D): Cyanodiplostomum sp. (E): Prohemistomum sp. stained with acetic acid alum carmine (F): Pygidiopsis genata stained with acetic acid alum carmine (G): Heterphyes sp. stained with acetic acid alum carmine (H–O). Helminth parasites identified in infected fish (Scale bar = 0.5 mm). (H): Cichlidogyrus tilapiae. (I): Enterogyrus cichlidarum, stained with acetic acid alum carmine (J): Acanthosentis tilapiae. (K): anterior part of A. tilapiae. (L): Procamallanus sp. (M): Paracamallanus sp. (N,O): Procercoid and pleurocercoid stage of Polyonchobothrium clarias.
Pearson Correlation Coefficients
Table 6 showed the Pearson correlation between parasite prevalence, physico-chemical parameters, and water heavy metals. There was a positive correlation between parasite prevalence and turbidity, nitrogen dioxide, nitrate, total alkalinity, and phosphate (r = 0.75, 0.69, 0.65, and 0.67; p < 0.05). The parasite prevalence of farmed and lake fish was correlated negatively with pH and dissolved oxygen (r = −0.51 and −49 previously and −0.66 and −0.58 later; p < 0.05). In both fish farm and Burullus Lake waters, there was a significant negative correlation between parasite prevalence (PP) and water heavy metals, including PP-Zn, PP-Pb, and PP-As. However, there was a significant positive correlation between PP and Cu. There is no significant link between PP-Fe and PP-Cd.
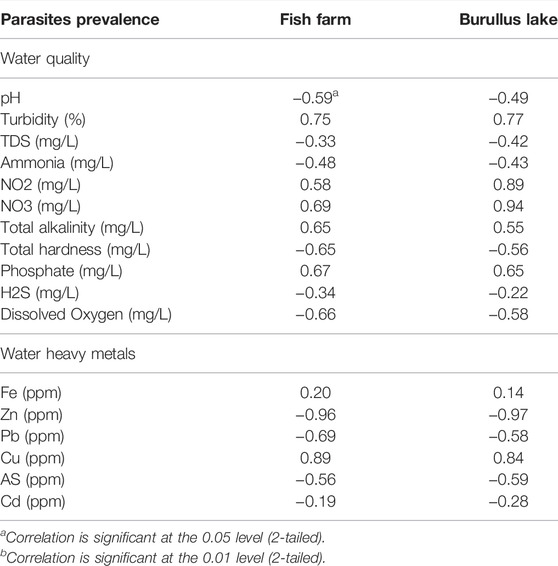
TABLE 6. Pearson correlation coefficients between water quality and water-heavy metals with parasites prevalence of O. niloticus, collected from Fish Farm and Burullus Lake.
Level of Heavy Metals in Fish Organs
Non-essential heavy metal concentrations, As, Cd, and Pb, were significantly higher in both non-infected lake and farmed fish compared to the infected specimens (p < 0.05), while the concentrations of the essential metals Fe, Zn, and Cu were significantly lower in non-infected fish than in infected specimens. However, for both infected and non-infected fish, there was a substantial (p < 0.05) increase in non-essential heavy metals in lake fish compared to farmed fish. Similarly, for both infected and non-infected groups, organs of lake and farmed Nile tilapia had the same sequence of essential and non-essential metals: Fe > Zn > Pb > Cu > As > Cd (Table 7).
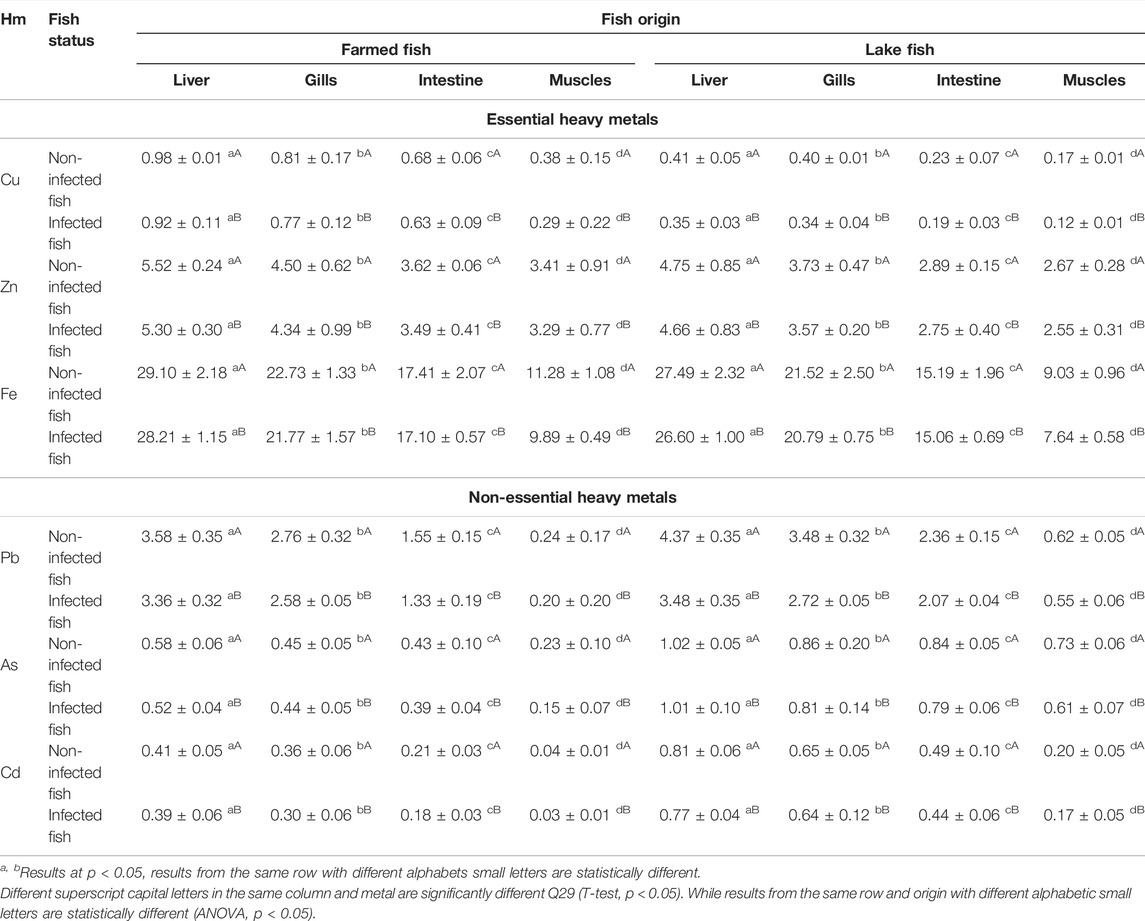
TABLE 7. Heavy metal concentrations in different organs of lake and farmed O. niloticus. Different superscript letters in the same column and metal are significantly different (T-test, p < 0.05). While results from the same row and origin with different alphabetic letters are statistically different (ANOVA, p < 0.05).
Table 8 revealed that the Pearson correlation had a strong positive correlation (p < 0.05) for Fe-Zn, Fe-Pb, Fe-Cu, Zn-Pb, Zn-Cu, and Pb-Cu. However, As and Cd showed no significant correlation with the remaining examined elements in infected and non-infected groups of farmed fish. In contrast, in lake fish, there was a substantial positive correlation (p < 0.05) between Fe-Zn, Fe-Pb, and Zn-Pb, as well as a non-significant correlation between the other analyzed metals.
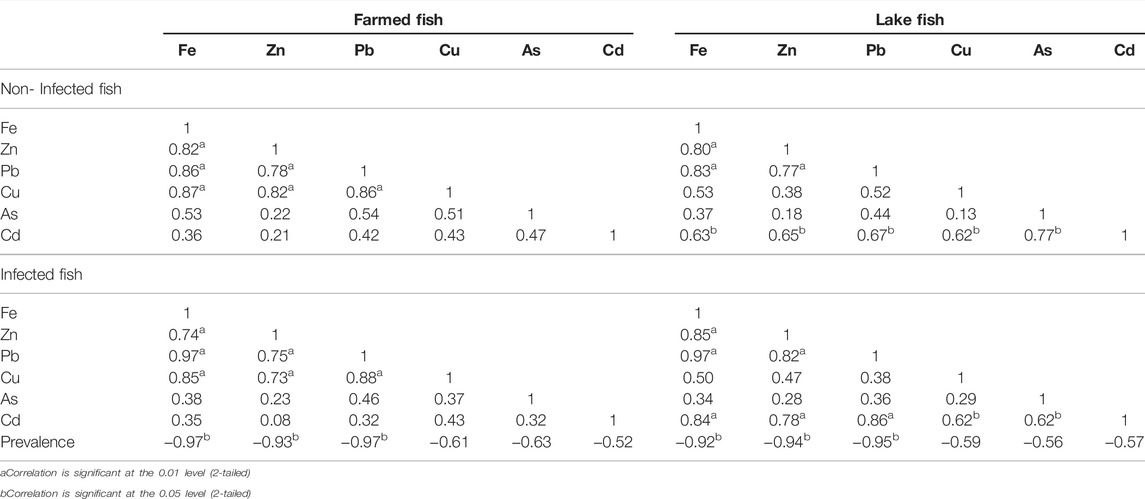
TABLE 8. Pearson correlations between heavy metal concentrations of lake and farmed in O. niloticus.
Bioaccumulation Factors of Heavy Metals in Fish Organs
BAF of heavy metals in organs of lake and farmed Nile Tilapia are shown in Figures 4, 5. BAF was evaluated from water to fish and in various organs of lake and farmed fish with increasing order of metal ions according to BAF Liver > BAF Gills > BAF Intestine > BAF Muscles. However, BAF in infected organs of lake and farmed fish was lower when compared to non-infected fish. In the infected and non-infected organs of lake fish, the highest accumulation factor was detected for Cd, while the lowest factor was observed for Cu. On the other hand, the intestine, liver, and gills of farmed fish organs accumulated the majority of Cd from the water, whereas Zn had the lowest accumulation factor. Fe was accumulated in the muscles to the maximum extent in farmed fish.
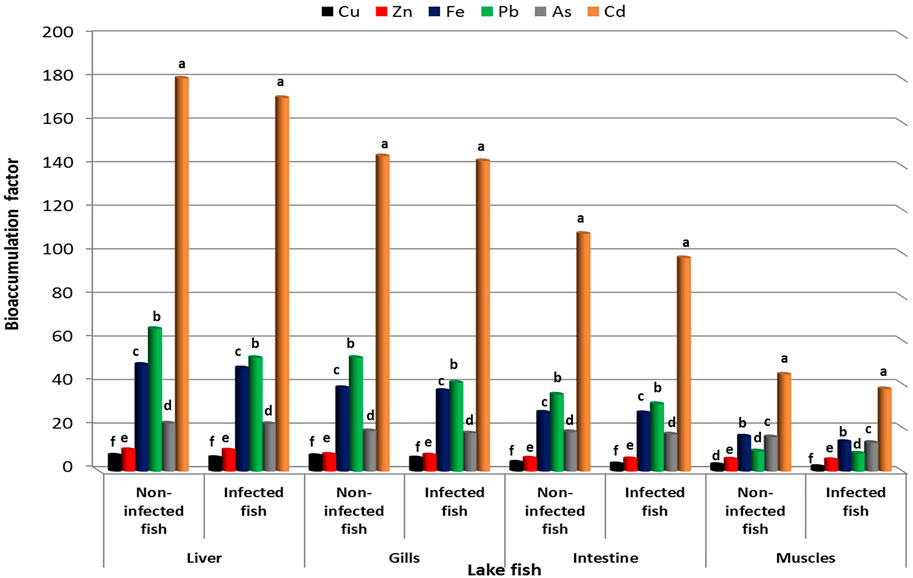
FIGURE 4. Bioaccumulation factor of heavy metals in different organs of lake Nile Tilapia, O. niloticus.
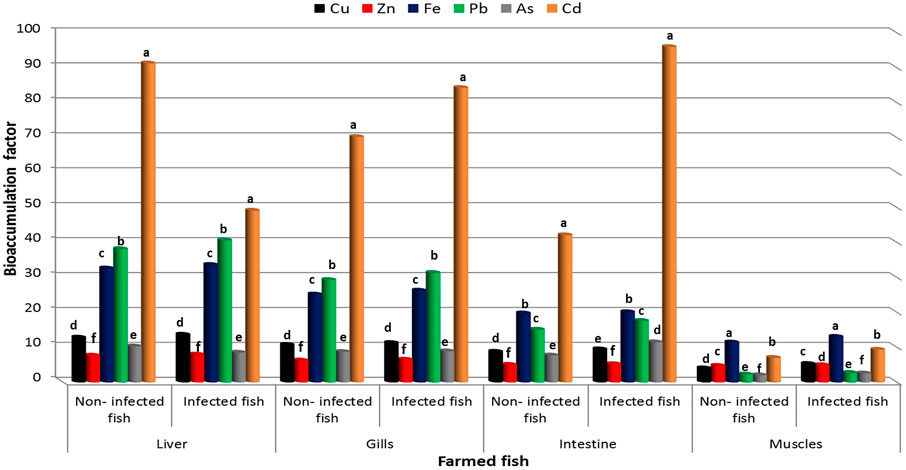
FIGURE 5. Bioaccumulation factor of heavy metals in different organs of farmed Nile Tilapia, O. niloticus.
Human Health Assessment
Table 9 reports the estimated daily intake (EDI, mg/kg/day) and target hazard quotients (THQ) for trace metals in the muscles of lake and farmed fish. Children's EDI in farmed O. niloticus muscles varied from 5 × 10−6 to 2 × 10−3 mg/kg/day, while adults’ EDI ranged from 9 × 10−6 to 3 × 10−3 mg/kg/day. In lake fish, however, EDI varied from 8 × 10−5 to 1 × 10−2 mg/kg/day for children, and from 4 × 10−5 to 3 × 10−2 mg/kg/day for adults. Furthermore, the THQ in this investigation was all lower than 1, indicating that eating lake and farmed fish had no negative health consequences for both age groups. Figure 6 shows the hazard index (HI) for heavy metals in the muscles of lake and farmed fish. The HI values determined for this study were all lower than 1, indicating that lake and farmed fish intake is safe. In both groups examined, lake fish had higher HI values than farmed fish. For both children and adults, it was greater in lake fish than in farmed fish. Furthermore, for both children and adults, it was greater in non-infected muscles than in infected muscles.
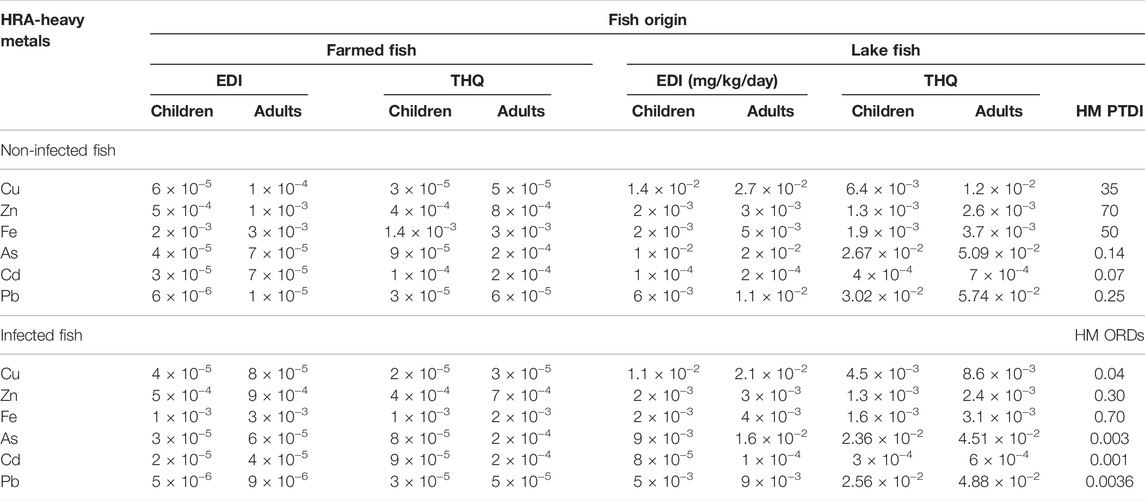
TABLE 9. Estimated daily intake (EDI, mg/kg/day) and target hazard quotients (THQ) for HMs in muscles of farmed and lake O. niloticus.
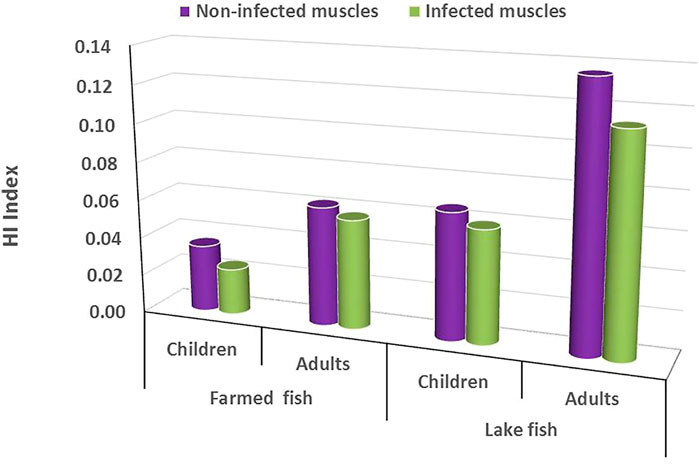
FIGURE 6. Hazard index (HI) for heavy metals in the muscles of lake and farmed Nile Tilapia, O. niloticus.
Discussion
Water Quality and Fish Parasites Relationship
Water quality is an essential factor in the growth of healthy fish. Therefore, the risk of infections is linked to the water’s sanitary conditions as well as to the overall fish health (Ojwala et al., 2018). The values of pH in this study were neutral, even though the optimal pH range for freshwater fish was between 6.5 and 7.0. Also, a pH range of 6.1–8.0 was deemed adequate for fish reproduction and survival (Makori et al., 2017). According to the results from Soltan et al. (2016), reduced water transparency can be linked to a higher abundance of phytoplankton, but in lakes, it is possible that it can be due to untreated wastewater (Negm et al., 2017). Total ammonia was also found to be higher in fish farms than in lakes, which might be due to large fish stocks, fish excretion, and the breakdown of extra un-consumed diet, all of which are ammonia sources in ponds. These findings corroborate previous research (Konsowa, 2007; Radwan et al., 2021; Radwan, 2022).
The elevated level of dissolved oxygen concentration (DO) detected in the farm water might be related to the abundance and distribution of phytoplankton, which promotes photosynthesis, resulting in the creation of a huge quantity of DO (El-Nemaki et al., 2008). This is consistent with other observations by Soltan et al. (2016). On the other hand, a depletion in DO concentrations might be owing to a higher usage rate caused by biological processes and high temperature (Radwan, 2005; Goheret al., 2015; El Sayed et al., 2020). Dissolved oxygen and pH, on the other hand, displayed a strong negative relation with the Parasites Prevalence (PP) in lake and farmed Nile Tilapia. This observation agrees with Zargar et al. (2012) and Radwan et al. (2021) who observed strong negative relationships between parasite presence frequency and pH and DO concentrations. Meanwhile, these findings contradict the findings of Sosanya (2002) who found a positive relationship between water contaminants and parasite prevalence rates. Furthermore, H2S is very hazardous to fish. Boyd (1985) indicated a safe H2S concentration for freshwater fish of 0.002 mg/L. The correlation coefficient of physico-chemical elements with parasite prevalence in this study implies that the measured factors have an influence on the PP (Wali et al., 2016; Qayoom and Shah, 2017; Radwan et al., 2021). Turbidity, total alkalinity, NO2, NO3, and phosphate were shown to be substantially related to PP (Abba et al., 2018; Ojwala et al., 2018).
Length-Weight Relationship
The present study showed that the values of “b” of infected fishes were significantly lower than “3” which indicates a negative allometric growth, i.e., the fish grows but it is slender (Bagenal and Tesch, 1978). Negative allometric growth is an indication of slow growth, which might be due to the non-availability of food or weakened immune status (Veeramani et al., 2010; Ndeda et al., 2013). On the other hand, the variation of growth patterns may be due to ecological parameters through periods of study, which include several biotic and abiotic interactions contingent on seasonality (Ngodhe and Owuor-JB, 2019). In contrast, parasites are also known to act as stressors on their hosts and then cause altered growth in their hosts (Froese, 2006; Kahraman et al., 2014).
Parasitological Outcomes
Parasite prevalence (PP) was found to be higher in farmed fish than in lake Nile Tilapia in the current investigation. This may be attributed to stoking density of fish at farms and feeding habits, which depend mainly on artificial feed and other natural aquatic pollutants (Enyidi and Eneje, 2015). Parasites prevalence may also be related to increased fish numbers in culture systems and variations in physico-chemical variables related to a larger density of fish in the cultivated site than in the lake (Violante-González et al., 2009; Ibrahim and Soliman, 2010). This research also revealed that trematodes have been the most common source of parasite infection in fish populations. This finding might be due to fish skin that may be more sensitive to infective parasite stages penetration and the detritus and benthic invertebrates consumed by this fish at different periods. Nile Tilapia is considered an intermediate host which is suitable for many parasite phases when favorable physico-chemical properties are present (El-Shahawy et al., 2017; Shehata et al., 2018; Radwan, 2022).
Assay of Heavy Meals in Water and Fish Tissues
Heavy metals concentrations are essential indicators of fish health and the aquatic environment (Padrilah et al., 2018). Heavy metals estimated levels (Fe, Zn, Pb, Cu, and As) in the water of Burullus Lake and fish farm were within the standard allowed values except for Pb, which exceeded the maximum permitted levels (WHO, 2011), as a result of contamination by industrial effluents and municipal sewage concentrations that might be blamed (Chen et al., 2010; Nafea and Zyada, 2015). Moreover, HM levels in the current study’s findings (Burullus Lake and Farmed fish waters) are still within the range of prior examinations, except for Zn, Cu, and Pb, which were greater in both analyzed locations than in past studies (Younes and Nafea, 2012; Ghabour et al., 2013; El-Batrawy et al., 2018; Melegy et al., 2019). The following is the order of the metals: Fe < Zn < Pb < Cu < As < Cd as reported in other studies (Darwish, 2016; El Morshedy, 2017; El-Batrawy et al., 2018).
Metal levels (Fe, Zn, Cu, As, Cd, and Pb) in the non-infected lake and farmed Nile Tilapia were significantly higher than in the infected fish. This may be due to parasites accumulating high levels of metal in their tissues, thereby acting as metal sinks for their fish host and helping in their survival in the presence of toxins. These observations agree with Sures (2007), Azmat et al. (2008), and Eissa et al. (2012). Furthermore, infected fish had decreased concentration of some heavy metals, which included Cu, Fe, Pb, and Zn, according to some studies (Oyoo-Okoth et al., 2010; Dural et al., 2011). This result is supported by Shahat et al. (2011) who observed that parasitic-infected organs had lower amounts of Cu, Cd, and Pb than non-infected fish. Similarly, gastrointestinal parasitic worms appeared capable to decrease levels of heavy metals in fish muscle by accumulating them (Al-Hasawi, 2019; Mehana et al., 2020).
Cu, Zn, and Pb concentrations were found to be higher in Burullus Lake compared to previous studies, while Cd and Fe were decreased (Shakweer and Radwan, 2004; Radwan 2005; Saeed and Shaker, 2008; Masoud et al., 2011; Basiony, 2014; El-Alfy, 2015; El-Batrawy et al., 2018). However, in the fish farm, Cu, Zn, As and Pb concentrations were higher compared to the previous studies (Radwan 2005; Masoud et al., 2011; Basiony, 2014; El-Alfy, 2015; El-Batrawy et al., 2018), while Cd and Fe concentrations were lower compared to previous studies (Shakweer and Radwan, 2004; Saeed and Shaker, 2008).
The level of heavy metals concentration in fish tissues is influenced by various factors such as water quality, fish species, and maturity stage (Łuczy´nska and Paszczyk, 2019). The non-essential heavy metals such as As, Cd, and Pb were much higher than the levels recommended by USEPA (2010) and FAO (2016), which may be caused by the increase in human activities in the lake or fish farms. On the other hand, Yildiz, (2008), Fallah et al. (2011), Kim et al. (2018), and Simukoko et al. (2022) reported that a diet that contains animal protein ingredients has a much higher level of heavy metals, especially of the essential heavy metals such as Zn, Fe, and Cu.
Non-essential heavy metal levels (As, Cd, and Pb) in cultivated Nile Tilapia were much lower than in lake fish, maybe due to the fact that lake fish live for several years while cultivated fish are caught within 6 months (Oumar et al., 2018). When compared to cultivated Nile Tilapia and lake fish, Nile Tilapia can absorb contaminants with long biological half-lives, such as, Cd, and Pb, throughout a long lifespan. These findings agree with Chatta et al. (2016), who reported that the farmed Cirrhinus mrigala and Labeo rohita accumulated less Cd and Pb than the lake ones. Lake fish also had higher levels of non-essential heavy metals due to a longer lifetime and exposure, but they also had reduced levels of essential metals because they were not fed a commercial diet (Simukoko et al., 2022).
Bioaccumulation Factor of HM Levels in Fish Tissues
The bioaccumulation (BAF) of heavy metal levels in fish tissues is the percentage of heavy metal levels in tissues to their levels in the aquatic environment (Hatem et al., 2015). In the current study, gill, muscles, liver, and intestine tissues presented a strong probability of heavy metal bioaccumulation in fish. All gill and liver samples had a high BAF value, whereas the muscle and intestine presented lower values. This revealed that the heavy metals were accumulating in fish tissues after being absorbed from the water. The BAF in the current study revealed that the heavy metal levels in the analyzed tissues were in the following order: liver > gills > intestines > muscles, as already reported in a previous study (Maurya et al., 2019). Active metabolic tissues, such as the kidneys, gills, and liver, accumulate more heavy metals than less metabolically active tissues, such as the muscles and skin (Ali et al., 2019). Generally, muscles store the lowest amount of heavy metals (Ronagh et al., 2009). These results are nearly similar to those reported by Badr et al. (2014), who concluded that the level of heavy metals in Nile Tilapia tissues were higher in the liver than in gills and muscle.
In general, the current study found that the tissues of lake and cultivated Nile Tilapia contained more iron (Fe) than every heavy metal tested, with cadmium (Cd) being the lowest, and concentrations of heavy metals were in the sequence Fe > Zn > Pb > Cu > As > Cd. The increase in Fe accumulation in fish tissue in all tested organs was greater than the increase of other metals. This might be related to an increase in the total dissolved Fe in water of fish farms and lakes, with a consequent increase in free metal Fe levels and hence metal absorption by various organs. These results are supported by Tayel et al. (2008) and Al-Halani et al. (2021), who determined that the heavy metal concentration in lake fish tissues, Dicentrarchus labrax, occurred in the following order: Fe > Zn > Mn > Cu > Pb > Ni > Cr > Cd.
Human Health Risk Assessment
Humans consume large amounts of fish, and fish muscles are a good source of protein. As a result, determining the human daily consumption of heavy metals from fish is important (Rajeshkumar and Li, 2018). The daily intake of metal through the consumption of commercially relevant fish species by people who eat a normal quantity of fish on a regular basis corresponds to a hazard index (HI) of 1.0, as suggested by USEPA (2018).
To explain the safe levels of heavy metals, the average daily intake was utilized (Keshavarzi et al., 2018). The oral reference dose (ORD) is an oral reference dosage established on the basis of worldwide and Egyptian fish consumption and daily exposure levels. This research focused on the estimated daily intake (EDI) (mg/kg/day). Target hazard quotient (THQ) and HI were used to determine the levels of heavy metals in the muscles of farmed and lake Nile Tilapia. EDI levels in cultivated Nile Tilapia muscles ranged from 1 × 10−5 to 0.193 mg/kg/day. On the other hand, the lake fish had a range of 2 × 10−4 to 0.024 mg/kg/day. The EDI distribution against the examined heavy metals was arranged in the following order: Cd > As > Cu > Pb > Zn > Fe. The measured EDI of both groups was compared to the FAO/WHO (2004). Similarly, for permissible tolerable daily intake (PTDI), which is the acceptable tolerable daily intake for 70 kg weight, it was determined that the metals’ mean EDI levels have to be lower than the PTDI values (FAO, 2016).
The THQ values estimate— for this study were all lower than one, indicating that eating cultivated and lake Nile Tilapia will not harm people. According to Lei et al. (2015), if the HI value is lower than one (HI1), the impacts on humans would be unfavorable, HI > 1 would most likely have a negative impact, and HI > 10 would have strong or chronic acute consequences.
Human health risk assessment due to exposure to heavy metals through farmed and lake Nile Tilapia consumption revealed no significant non-carcinogenic adverse health risk to humans, as all calculated values for THQ and HI were lower than 1, indicating that the analyzed fish would not cause any health problems in both children and adults.
Conclusion
Generally, all studied metals in the water were found to be lower than the permissible limits, except for Pb. HM levels were significantly lower in infected than in non-infected Nile tilapia. HM levels in lake and farmed Nile tilapia tissues in the area of study were mostly recognized by the legislation of national and international limits for not determining human risk upon their consumption. THQ and HI values calculated for HMs levels of lake and farmed Nile tilapia predicted for adults and children were below the threshold level of 1. Parasites are capable to decrease concentrations of heavy metals in the tissues of fish by accumulating them. Also, they are a possible application as early warning indicators of heavy metal pollution in fish. Fish farmers lacked knowledge of diseases that affect fish and the importance of water quality in disease transmission. Heavy metal levels in fish tissue and water must be continuously monitored not just for human health but also to inform aquaculture management. In addition, employing the EDI, THQ, and HI indices to estimate human health risk is a highly recommended method.
Data Availability Statement
The raw data supporting the conclusion of this article will be made available by the authors, without undue reservation.
Ethics Statement
All applicable international, national, and/or institutional guidelines for the care and use of fish were followed by the authors.
Author Contributions
MR. Conceptualization, formal analysis, investigation, methodology, validation, writing—original draft writing—review, and editing. MA. Conceptualization, formal analysis, investigation, methodology, validation, writing. MM. The investigation, methodology, validation, writing—original draft. Finally, all authors have read and approved the manuscript. AM: Formal analysis, Methodology, Conceptualization, Editing. JA: Formal analysis, Methodology, Visualization, Editing.
Funding
This research was funded by The Taif University researchers, supporting project number (TURSP-2020/299), Taif University, Taif, Saudi Arabia.
Conflict of Interest
The authors declare that the research was conducted in the absence of any commercial or financial relationships that could be construed as a potential conflict of interest.
Publisher’s Note
All claims expressed in this article are solely those of the authors and do not necessarily represent those of their affiliated organizations, or those of the publisher, the editors, and the reviewers. Any product that may be evaluated in this article, or claim that may be made by its manufacturer, is not guaranteed or endorsed by the publisher.
Acknowledgments
The authors would like to thank the Deanship of Scientific Research, Taif University for the financial support. The authors would also like to thank the research staff of the departments of Zoology, Al-Azhar University for the scientific guidance.
References
Abah, J., Mashebe, P., and Onjefu, S. (2016). Preliminary Assessment of Some Heavy Metals Pollution Status of Lisikili River Water in Zambezi Region, Namibia. Int. J. Environ. Pollut. Res. 4, 13–30.
Abba, A. M., Abdulkarim, B., Omenesa, R. L., Abdulhamid, Y., and Mudassir, I. (2018). Study on Physico-Chemical Parameters and Prevalence of Fish Parasites in Jibia Reservoir Katsina State Nigeria. UMYU J. Microbiol. Res. 3 (2), 1–6.
Abbas, M. M. M., Shehata, S. M., Talab, A. S., and Mohamed, M. H. (2021). Effect of Traditional Processing Methods on the Cultivated Fish Species, Egypt. Part I. Mineral and Heavy Metal Concentrations. Biol. Trace Elem. Res. 200, 2391–2405. doi:10.1007/s12011-021-02840-w
Abdel-hakim, N. F., Helal, A., Salem, M., Zaghloul, M., and Hanbal, M. (2016). Effect of Some Heavy Metals on Physiological and Chemical Parameters in Nile tilapia (Oreochromis niloticus L.). J. Egypt. Acad. Soc. Environ. Dev. D, Environ. Stud. 17, 81–95. doi:10.21608/jades.2016.63382
Adolfsson-Erici, M., Åkerman, G., and Mclachlan, M. S. (2012). Measuring Bioconcentration Factors in Fish Using Exposure to Multiple Chemicals and Internal Benchmarking to Correct for Growth Dilution. Environ. Toxicol. Chem. 31, 1853–1860. doi:10.1002/etc.1897
Ahmed, N. F., Sadek, K. M., Soliman, M. K., Khalil, R. H., Khafaga, A. F., Ajarem, J. S., et al. (2020). Moringa Oleifera Leaf Extract Repairs the Oxidative Misbalance Following Sub-chronic Exposure to Sodium Fluoride in Nile tilapia oreochromis Niloticus. Animals 10, 626. doi:10.3390/ani10040626
Al-Halani et al., A. A., Soliman, A., and Shady, S. H. H. (2021). The Seasonal Assessment of Heavy Metals Bioaccumulation in European Seabass (Dicentrarchus labrax). Egypt. J. Aqua. Biolo. Fish. 25 (5), 607–626. doi:10.21608/ejabf.2021.205025
Al-Hasawi, Z. M. (2019). Environmental Parasitology: Intestinal Helminth Parasites of the Siganid Fish Siganus Rivulatus as Bioindicators for Trace Metal Pollution in the Red Sea. Parasite 26, 12. doi:10.1051/parasite/2019014
Ali, H., Khan, E., and Ilahi, I. (2019). Environmental Chemistry and Ecotoxicology of Hazardous Heavy Metals: Environmental Persistence, Toxicity, and Bioaccumulation. J. Chem. 2019, 1–14. doi:10.1155/2019/6730305
AOAC [Association of Official Analytical Chemists] (2012). Official Methods of Analysis. 15th ed. Washington, DC USA: Association of official analytical chemists Inc, 478.
APHA (2005). Standard Methods for Examination of Water and Wastewater. 21st. Edn. Washington DC, USA: American Public Health Association APHA, the American Water Works Association AWWA, and the Water Environment Federation WEF.
Authman, M. M. N., Abbas, W. T., and Gaafar, A. Y. (2012). Metals Concentrations in Nile tilapia Oreochromis niloticus (Linnaeus, 1758) from Illegal Fish Farm in Al-Minufiya Province, Egypt, and Their Effects on Some Tissues Structures. Ecotoxicol. Environ. Saf. 84, 163–172. doi:10.1016/j.ecoenv.2012.07.005
Azmat, R., Fayyaz, S., Kazi, N., Mahmood, S. J., and Uddin, F. (2008). Natural Bioremediation of Heavy Metals through Nematode Parasite of Fish. Biotechnology 7 (1), 139–143. doi:10.3923/biotech.2008.139.143
Badr, A. M., Mahana, N. A., and Eissa, A. (2014). Assessment of Heavy Metal Levels in Water and Their Toxicity in Some Tissues of Nile Tilapia (Oreochromis niloticus) in River Nile Basin at Greater Cairo, Egypt. Glob. Veterinaria 13 (4), 432–443. doi:10.5829/idosi.gv.2014.13.04.8561
Bagenal, T. B., and Tesch, F. W. (1978). “Age and Growth,” in Methods of Assessment of Fish Production in Fresh Waters. Editor T. Bagenal (Oxford: Oxford Blackwell Scientific Publication), 101–136.
Basiony, A. I. (2014). Environmental Studies on Heavy Metals Pollution and Management of Lake Burullus, Egypt. M.Sc. Thesis. Port Said, Egypt: Faculty of science, Port Said University.
Boyd, C. E. (1985). Chemical Budgets for Channel Catfish Ponds. Trans. Am. Fish. Soc. 114, 291–298. doi:10.1577/1548-8659(1985)114<291:cbfccp>2.0.co;2
Chatta, A. M., Khan, M. N., Mirza, Z. S., Ali, A., and Ali, A. (2016). Heavy Metal (Cadmium, Lead, and Chromium) Contamination in Farmed Fish: a Potential Risk for Consumers’ Health. Turk. J. Zool. 40, 248–256. doi:10.3906/zoo-1506-1
Chen, Z., Salem, A., Xu, Z., and Zhang, W. (2010). Ecological Implications of Heavy Metal Concentrations in the Sediments of Burullus Lagoon of Nile Delta, Egypt. Estuarine. Estuar. Coast. Shelf Sci. 86 (3), 491–498. doi:10.1016/j.ecss.2009.09.018
Darwish, S. I. (2016). Water Quality and Heavy Metals Pollution in Soil, Water and Fish in Lake Burullus. Ph.D. Thesis. Alexandria: Faculty Of Agriculture Saba Basha- Alexandria University. Dep. of fish production.
David, L. H., Pinho, S. M., Agostinho, F., Kimpara, J. M., Keesman, K. J., and Garcia, F. (2021). Emergy Synthesis for Aquaculture: A Review on its Constraints and Potentials. Rev. Aqua. 13, 1119–1138. doi:10.1111/raq.12519
Dural, M., Genc, E., Sangun, M. K., and Güner, O. (2011). Accumulation of Some Heavy Metals in Hysterothylacium Aduncum (Nematoda) and its Host Sea Bream, Sparusaurata (Sparidae) from North-Eastern Mediterranean Sea (Iskenderun Bay). Environ. Monit. Assess. 174 (1–4), 147–155. doi:10.1007/s10661-010-1445-0
Eissa, I. A. M., Gehan, I. S., Wafeek, M., and Nashwa, A. S. (2012). Bioremediation for Heavy Metals in Some Red Sea Fishes in Suez, Egypt. SCVMJ, XVII 18 (2), 341–356.
El Morshedy, E. M. (2017). Effect of Pollution on Water Quality, Soil and Fish Performance in Lakes and Fish Farm Ponds. Ph.D. Thesis. Alexandria: Faculty Of Agriculture Saba Basha- Alexandria University. Dep. of fish production.
El Sayed, S. M., Hegab, M. H., Mola, H. R. A., Ahmed, N. M., and Goher, M. E. (2020). An Integrated Water Quality Assessment of Damietta and Rosetta Branches (Nile River, Egypt) Using Chemical and Biological Indices. Environ. Monit. Assess. 192, 228. doi:10.1007/s10661-020-8195-4
El-Alfy, M. A. (2015). Comparative Ecological Studies on the Northern Deltaic Lakes Using Geographic Information system-Egypt. Ph.D. Thesis. Mansoura: Faculty of science, Mansoura University.
El-Batrawy, O. A., El-Gammal, M. I., Mohamadein, L. I., Darwish, D. H., and El-Moselhy, K. M. (2018). Impact Assessment of Some Heavy Metals on tilapia Fish, Oreochromis niloticus, in Burullus Lake, Egypt. J. Basic Appl. Zool. 79, 13. doi:10.1186/s41936-018-0028-4
El-Nemaki, F. A., Ali, N. A., Zeinhom, M. M., and Radwan, O. A. (2008). “Impacts of Different Water Resources on the Ecological Parameters and Quality of tilapia Production at ElAbbassa Fish Farms in EGYPT,” in Proceedings of the 8th International Symposium on Tilapia in Aquaculture.
El-Shahawy, I. S., El-Seify, M. O., Metwally, A. M., and Fwazi, M. M. (2017). Survey on Endoparasitic Fauna of Some Commercially Important Fishes of the River Nile, Southern of Egypt (Egypt). Rev. Méd Vét 168 (4-6), 126–134.
ElSheikha, H. M., and Elshazly, A. M. (2008). Host-dependent Variations in the Seasonal Prevalence and Intensity of Heterophyid Encysted Metacercariae (Digenea: Heterophyidea) in Brackish Water Fish in Egypt. Vet. Parasitol. 153, 65–72. doi:10.1016/j.vetpar.2008.01.026
Enyidi, U. D., and Eneje, U. I. (2015). Parasites of African Catfish Clarias gariepinus Cultured in Homestead Ponds. Res. J. Agric. 2 (12), 1–10.
Fallah, A. A., Saei-Dehkordi, S. S., Nematollahi, A., and Jafari, T. (2011). Comparative Study of Heavy Metal and Trace Element Accumulation in Edible Tissues of Farmed and Wild Rainbow Trout (Oncorhynchus mykiss) Using ICP-OES Technique. Microchem. J. 98, 275–279. doi:10.1016/j.microc.2011.02.007
FAO (2016). Fishery Information Data and Statistics Unit. FISHSTAT, Databases and Statistics. Rome, Italy: Food and Agriculture Orga,nization of the United Nation.
FAO/WHO (2004). “FAO/WHO Summary of Evaluations Performed by the Joint FAO/WHO Expert Committee on Food Additives (JECFA 1956e2003),” in Evaluation of Certain Contaminants in Food: Sixty-Fourth Report of the Joint (2004).
Froese, R. (2006). Cube Law, Condition Factor and Weight-Length Relationships: History, Meta-Analysis and Recommendations. J. Appl. Ichthyol. 22 (4), 241–253. doi:10.1111/j.1439-0426.2006.00805.x
GAFRD (2019). “General Authority for Fish Resources Development,” in Fish Statistics Yearbook (Cairo, Arab Republic of Egypt: Agriculture Ministry Press).
Ghabour, T. K., Rahim, I. S., and Morgan, R. S. (2013). Heavy Metals Status of Some Wetlands of Egypt. J. Appl. Sci. Res. 9 (7), 4345–4350.
Goher, M. E., Abdo, M. H., Mangood, A. H., and Hussein, M. M. (2015). Water Quality and Potential Health Risk Assessment for Consumption of Oreochromis niloticus from El-Bahr El-Pharaony Drain, Egypt. Fresenius Environ. Bull. 24 (11), 3590–3602.
Hassan, A., Moharram, S., and El Helaly, H. (2018). Role of Parasitic Helminths in Bioremediating Some Heavy Metal Accumulation in the Tissues of Lethrinus Mahsena. Turk. J. Fish. Aquat. Sci. 18, 435–443. doi:10.4194/1303-2712-v18_3_09
Hatem, A. J., Azim, B., Nguyen, B., Sylvain, G., and Zoppoli, C. S. (2015). Genomic Aberrations in Young and Elderly Breast Cancer Patients. BMC Med. 13, 266. doi:10.1186/s12916-015-0504-3
Henriksson, P. J. G., Dickson, M., Allah, A. N., Al-Kenawy, D., and Phillips, M. (2017). Benchmarking the Environmental Performance of Best Management Practice and Genetic Improvements in Egyptian Aquaculture Using Life Cycle Assessment. Aquaculture 468, 53–59. doi:10.1016/j.aquaculture.2016.09.051
Ibrahim, M. M., and Soliman, M. F. M. (2010). Prevalence and Site Preferences of Heterophyid Metacercariae in Tilapia Zilli from Ismalia Freshwater Canal, Egypt. Parasite 17 (3), 233–239. doi:10.1051/parasite/2010173233
Jiang, Z., Xu, N., Liu, B., Zhou, L., Wang, J., Wang, C., et al. (2018). Metal Concentrations and Risk Assessment in Water, Sediment and Economic Fish Species with Various Habitat Preferences and Trophic Guilds from Lake Caizi, Southeast China. Ecotoxicol. Environ. Saf. 157, 1–8. doi:10.1016/j.ecoenv.2018.03.078
Ju, Y.-R., Chen, C.-W., Chen, C.-F., Chuang, X.-Y., and Dong, C.-D. (2017). Assessment of Heavy Metals in Aquaculture Fishes Collected from Southwest Coast of Taiwan and Human Consumption Risk. Int. Biodeterior. Biodegrad. 124, 314–325. doi:10.1016/j.ibiod.2017.04.003
Kahraman, A., Göktürk, D., and Aydin, E. (2014). Length-Weight Relationships of Five Fish Species from the Sakarya River, Turkey. Annu. Rev. Res. Biol. 4 (15), 2476–2483. doi:10.9734/arrb/2014/7513
Keke, U. N., Arimoro, F. O., Ayanwale, A. V., and Aliyu, S. M. (2015). Physicochemical Parameters and Heavy Metals Content of Surface Water in Downstream Kaduna River, Zungeru, Niger State, Nigeria. Appl. Sci. Res. J. 3, 46–57.
Keshavarzi, B., Hassanaghaei, M., Moore, F., Rastegari Mehr, M., Soltanian, S., Lahijanzadeh, A. R., et al. (2018). Heavy Metal Contamination and Health Risk Assessment in Three Commercial Fish Species in the Persian Gulf. Mar. Pollut. Bull. 129, 245–252. doi:10.1016/j.marpolbul.2018.02.032
Kim, H.-T., Loftus, J. P., Mann, S., and Wakshlag, J. J. (2018). Evaluation of Arsenic, Cadmium, Lead and Mercury Contamination in Over-the-counter Available Dry Dog Foods with Different Animal Ingredients (Red Meat, Poultry, and Fish). Front. Vet. Sci. 5, 264. doi:10.3389/fvets.2018.00264
Konsowa, A. H. (2007). Ecological Studies on Fish Farms of El-Fayoum Depression. E. J. Aquat. Res. 33 (1), 290–299.
Lei, M., Tie, B.-q., Song, Z.-g., Liao, B.-H., Lepo, J. E., and Huang, Y.-z. (2015). Heavy Metal Pollution and Potential Health Risk Assessment of White Rice Around Mine Areas in Hunan Province, China. Food Sec. 7 (1), 45–54. doi:10.1007/s12571-014-0414-9
Łuczy´nska, J., and Paszczyk, B. (2019). Health Risk Assessment of Heavy Metals and Lipid Quality Indexes in Freshwater Fish from Lakes of Warmia and Mazury Region, Poland. Int. J. Environ. Res. Public Health 16, 3780. doi:10.3390/ijerph16193780
Makori, A. J., Abuom, P. O., Kapiyo, R., Anyona, D. N., and Dida, G. O. (2017). Effects of Water Physico-Chemical Parameters on tilapia (Oreochromis niloticus) Growth in Earthen Ponds in Teso North Sub-County, Busia County. Fish. Aquat. Sci. 20, 30. doi:10.1186/s41240-017-0075-7
Mannzhi, M. P., Edokpayi, J. N., Durowoju, O. S., Gumbo, J., and Odiyo, J. O. (2021). Assessment of Selected Trace Metals in Fish Feeds, Pond Water and Edible Muscles of Oreochromis mossambicus and the Evaluation of Human Health Risk Associated with its Consumption in Vhembe District of Limpopo Province, South Africa. Toxicol. Rep. 8, 705–717. doi:10.1016/j.toxrep.2021.03.018
Marcogliese, D. J., and Pietrock, M. (2011). Combined Effects of Parasites and Contaminants on Animal Health: Parasites Do Matter. Trends Parasitol. 27 (27), 123–130. doi:10.1016/j.pt.2010.11.002
Masoud, M. S., Fahmy, M. A., Ali, A. E., and Mohamed, E. A. (2011). Heavy Metal Speciation and Their Accumulation in Sediments of Lake Burullus, Egypt. Afr. J. Environ. Sci. Technol. 5, 280–298.
Maurya, P. K., Malik, D. S., Yadav, K. K., Kumar, A., Kumar, S., and Kamyab, H. (2019). Bioaccumulation and Potential Sources of Heavy Metal Contamination in Fish Species in River Ganga Basin: Possible Human Health Risks Evaluation. Toxicol. Rep. 6 (6), 472–481. doi:10.1016/j.toxrep.2019.05.012
Mehana, E.-S. E., Khafaga, A. F., Elblehi, S. S., Abd El-Hack, M. E., Naiel, M. A. E., Bin-Jumah, M., et al. (2020). Biomonitoring of Heavy Metal Pollution Using Acanthocephalans Parasite in Ecosystem: an Updated Overview. Animals 10, 811. doi:10.3390/ani10050811
Melegy, A. A., El-Bady, M. S., and Metwally, H. I. (2019). Monitoring of the Changes in Potential Environmental Risk of Some Heavy Metals in Water and Sediments of Burullus Lake, Egypt. Bull. Natl. Res. Cent. 43, 143. doi:10.1186/s42269-019-0182-y
Mousa, M. A., Ibrahim, A. A. E., Hashem, A. M., and Khalil, N. A. (2015). The Effect of Water Quality on the Immunoreactivity of Stress-Response Cells and Gonadotropin-Secreting Cells in the Pituitary Gland of Nile tilapia, Oreochromis niloticus. J. Exp. Zool. 323, 146–159. doi:10.1002/jez.1901
Mwakalapa, E. B., Simukoko, C. K., Mmochi, A. J., Mdegela, R. H., Berg, V., Bjorge Müller, M. H., et al. (2019). HMs Levels in Cultivated and Wild Milkfish (Chanos Chanos) and Wild Mullet (Mugil cephalus) along the Coasts of Tanzania and Associated Health Risk for Humans and Fish. Chemosphere 224, 176–186. doi:10.1016/j.chemosphere.2019.02.063
Nafea, E. M. A., and Zyada, M. A. (2015). Biomonitoring of Heavy Metals Pollution in Lake Burrullas, Northern Delta, Egypt. Afr. J. Environ. Sci. Technol. 9 (1), 1–7. doi:10.5897/AJEST2014.1764
Ndeda, V. M., Aketch, B. O, and Onyango, D. M. (2013). Effect of Diplostomum Species on Length–Weight Relationship of Farmed Nile tilapia in Kibos Area, Kisumu City, Kenya. Fish. Aquac. J, 1–9.
Negm, A., Abdel-Aziz, T. M., Salem, M. N., and Yousef, W. (2017). “Morphology of the Nile River Due to a Flow Rate over the Maximum Current: Case Study Damietta Branch,” in The Nile River,. Editor A. M. Negm (Springer International Publishing AG), 239–257. Hdb Env Chem. doi:10.1007/698_2017_3
Ngodhe, S. O., and Owuor-Jb, O. (2019). Assessment of Length-Weight Relationship and Condition Factor of Nile Tilapia (Oreochromis Niloticus) in Cage and Open Waters in Winam Gulf of L. Victoria, Kenya. Ijesnr 22 (3), 556088. doi:10.19080/ijesnr.2019.22.556088
Ojwala, R. A., Otachi, E. O., and Kitaka, N. K. (2018). Effect of Water Quality on the Parasite Assemblages Infecting Nile tilapia in Selected Fish Farms in Nakuru County, Kenya. Parasitol. Res. 117 (11), 3459–3471. doi:10.1007/s00436-018-6042-0
Oros, M., Scholz, T., Hanzelová, V., and Mackiewicz, J. S. (2010). Scolex Morphology of Monozoic Cestodes (Caryophyllidea) from the Palaearctic Region: A Useful Tool for Species Identification. Folia Parasit. 57, 37–46. doi:10.14411/fp.2010.006
Oumar, D., Flibert, G., Tidjani, A., Rirabe, N., Patcha, M., Bakary, T., et al. (2018). Risks Assessments of Heavy Metals Bioaccumulation in Water and tilapia Nilotica Fish from Maguite Island of Fitri Lake. Curr. J. Appl. Sci. Technol. 26, 1–9. doi:10.9734/cjast/2018/39384
Oyoo-Okoth, E., Admiraal, W., Osano, O., Hoitinga, L., and Kraak, M. H. S. (2010). Metal Specific Partitioning in a Parasite-Host Assemblage of the Cestode Ligula Intestinalis and the Cyprinid Fish Rastrineobola Argentea. Sci. Total Environ. 408 (7), 1557–1562. doi:10.1016/j.scitotenv.2009.11.054
Padrilah, S. N., Shukor, M. Y. A., Yasid, N. A., Ahmad, S. A., Sabullah, M. K., and Shamaan, N. A. (2018). Toxicity Effects of Fish Histopathology on Copper Accumulation. Pertanika J. Trop. Agric. Sci. 2018, 519–540.
Qayoom, I., and Shah, F. A. (2017). Parasitic Bioload in Schizothoracine Fishes of Kashmir Valley with Respect to the Tropic Status of Anchar Lake. Res. J. Agric. Sci. 8 (2), 364–369.
Radwan, A. M. (2005). Some Factors Affecting the Primary Production of Phytoplankton in Burullus Lake. Egypt. J. Aquatic Res. 31 (2), 72–88.
Radwan, M., Shehata, S., Abdelhadi, Y., Mohammed, R., Mohamed, M., and Magdy, M. (2021). Histopathological, Haematological and Biochemical Indices of Clarias gariepinus (Burchell, 1822) Parasitized by Endoparasitic Fauna in Fish Farm of the Northeastern Egypt. Turkish J. Fish. Aquatic Sci. 21, 465–478. doi:10.4194/1303-2712-v21_9_05
Radwan, M. (2022). Vital Economic Threat of Predatory Birds and Parasites to Cultivated Fishes in Egypt. Aquaculture 548, 737666. doi:10.1016/j.aquaculture.2021.737666
Rajeshkumar, S., and Li, X. (2018). Bioaccumulation of Heavy Metals in Fish Species from the Meiliang Bay, Taihu Lake, China. Toxicol. Rep. 5, 288–295. doi:10.1016/j.toxrep.2018.01.007
Resma, N. S., Meaze, A. K. M. M. H., Hossain, S., Khandaker, M. U., Kamal, M., and Deb, N. (2020). The Presence of Toxic Metals in Popular Farmed Fish Species and Estimation of Health Risks through Their Consumption. Phys. Open 5, 100052. doi:10.1016/j.physo.2020.100052
Ronagh, M. T., Savari, A., Papahn, F., and Hesni, M. A. (2009). Bioaccumulation of Heavy Metals in Euryglossa Orientalis from the Hendijan Seaport (Coastal of Persian Gulf). J. Biol. Sci. 9, 272–275. doi:10.3923/jbs.2009.272.275
Saeed, S. M., and Shaker, I. M. (2008). “Assessment of Heavy Metals Pollution in Water and Sediments and Their Effect on Oreochromis niloticus in the Northern Delta Lakes, Egypt,” in Proceedings of the 8th International Symposium on Tilapia in Aquaculture, Cairo, 12-14 October 2008, 475–490.
Salam, M. A., Paul, S. C., Noor, S. N. B. M., Siddiqua, S. A., Aka, T. D., Wahab, R., et al. (2019). Contamination Profile of Heavy Metals in Marine Fish and Shellfish. Glob. J. Environ. Sci. Manag. 5, 225–236. doi:10.22034/gjesm.2019.02.08
Sardar, K., Shafaqat, A., Hameed, S., Afzal, S., Fatima, S., Shakoor, M. B., et al. (2013). Heavy Metals Contamination and what Are the Impacts on Living Organisms. Greener J. Environ. Manag. Public Saf. 2, 172–179. doi:10.15580/GJEMPS.2013.4.060413652
Shahat, M. A ., Amer, O. S. O., AbdAllah, A. T ., Abdelsater, N., and Moustafa, M. A. (2011). The Distribution of Certain Heavy Metals between Intestinal Parasites and Their Fish Hosts in the River Nile at Assuit Province, Egypt. Egypt. J. Hosp. Med. 43, 241–257. doi:10.21608/ejhm.2011.16783
Shakweer, L. M., and Radwan, A. M. (2004). Environmental and Fishery Investigations on Lake Burullus. 2, B. Rates of Trace Elements Accumulation in the Macrophytes and Fish at Lake Borollus. Egypt. J. Aquat. Res. 30, 99–120.
Shehata, S. M., Mohammed, R. A., Ghanem, M. H., Abdelhadi, Y. M., and Radwan, M. K. (2018). Impact of the Stresses Environmental Condition on the Prevalence of Parasite in Freshwater Aquaculture. J. Fish. Sci. 12 (2), 1–8. doi:10.21767/1307-234x.1000147
Simukoko, C. K., Mwakalapa, E. B., Bwalya, P., Muzandu, K., Berg, V., Mutoloki, S., et al. (2022). Assessment of HMs Levels in Wild and Cultivated tilapia (Oreochromis niloticus) on Lake Kariba, Zambia: Implications for Human and Fish Health. Food Addit. Contam. Part A 39 (1), 74–91. doi:10.1080/19440049.2021.1975830
Sohn, W.-M. (2009). Fish-borne Zoonotic Trematode Metacercariae in the Republic of Korea. Korean J. Parasitol. 47, S103–S113. doi:10.3347/kjp.2009.47.S.S103
Soltan, M., Hassaan, M., and Khattaby, A. (2016). Agricultural Drainage Water as a Source of Water for Fish Farming in Egypt. Ecol. Evol. Biol. 1 (3), 68–75. doi:10.11648/j.eeb.20160103.15
Sosanya, M. O. (2002). Fish Parasite as Indicators of Environmental Quality. M. Sc Thesis. Ibadan: University of Ibadan, 142.
Sures, B. (2007). Host-parasite Interactions from an Ecotoxicological Perspective. Parassitologia 49 (3), 173–176.
Tayel, S. I., Yacoub, A. M., and Mahmoud, S. A. (2008). Histopathological and Haematological Responses to Freshwater Pollution in the Nile Catfish Clarias gariepinus. J. Egypt. Acad. Soc. Environ. Dev. 9 (4), 43–60.
Tytła, M. (2019). Assessment of Heavy Metal Pollution and Potential Ecological Risk in Sewage Sludge from Municipal Wastewater Treatment Plant Located in the Most Industrialized Region in Poland - Case Study. Int. J. Environ. Res. Public Health 16, 2430–2516. doi:10.3390/ijerph16132430
USEPA (2010). Lists of Contaminants and Their Maximum Contaminant Levels (MCLs). Washington, DC: USEPA.
USEPA (2011). Risk Assessment Guidance for Superfund. Volume I: (Part A: Human Health Evaluation Manual; Part E, Supplemental Guidance for Dermal Risk Assessment; Part F, Supplemental Guidance for Inhalation Risk Assessment). Washington, DC: US Environmental Protection Agency.
USEPA (2018). US EPA Regional Screening Levels (RSLs) (November 2018). Dataset- California open data. Available at: https://data.ca.gov/dataset/us-epa-regional-screening-levels-rsls-november-2018/resource/b66c9172-a61a-4131-997f- 01f45a473a08 (Accessed Jul 28, 2021).
USEPA, United States Environmental Protection Agency (2008). Child-Specific Exposure Factors Handbook. EPA/600/R-06/096F. Washington. District of Columbia: National Center for Environmental Assessment Office of Research and Development, 1–687. Available at: http://www.epa.gov/ncea.
Veeramani, T., Ravi, V., and Kesavan, K. (2010). Length-weight Relationship of Parrot Fish, Scarus Ghobban, (Forsskal 1775) from Nagapattinam, Southeast Coast of India. Adv. Biol. Res. 4 (3), 182–184. doi:10.1186/1752-0509-4-95
Violante-González, J., García-Varela, M., Rojas-Herrera, A., and Guerrero, S. G. (2009). Diplostomiasis in Cultured and Wild tilapia Oreochromis niloticus in Guerrero State, Mexico. Parasitol. Res. 105 (3), 803–807. doi:10.1007/s00436-009-1458-1
Wali, A., Balkhi, M.-u. H., Maqbool, R., Darzi, M. M., Shah, F. A., Bhat, F. A., et al. (2016). Distribution of Helminth Parasites in Intestines and Their Seasonal Rate of Infestation in Three Freshwater Fishes of Kashmir. J. Parasitol. Res. 2016, 1–16. doi:10.1155/2016/8901518
Wang, Q., Cheng, L., Liu, J., Li, Z., Xie, S., and De Silva, S. S. (2015). Freshwater Aquaculture in PR China: Trends and Prospects. Rev. Aquacult. 7, 283–302. doi:10.1111/raq.12086
WHO (2011). Guidelines for Drinking-Water Quality. 4th edn. Geneva, Switzerland: World Health Organization.
Yildiz, M. (2008). Mineral Composition in Fillets of Sea Bass (Dicentrarchus labrax) and Sea Bream (Sparus aurata): A Comparison of Cultured and Wild Fish. J. Appl. Ichthyol. 24, 589–594. doi:10.1111/j.1439-0426.2008.01097.x
Younes, A. M., and Nafea, E. M. (2012). Impact of Environmental Conditions on the Biodiversity of Mediterranean Sea Lagoon, Burullus Protected Area, Egypt. World Appl. Sci. J. 19 (10), 1423–1430. doi:10.5829/idosi.wasj.2012.19.10.66110
Zargar, U. R., Yousuf, A. R., Chishti, M. Z., Ahmed, F., Bashir, H., and Ahmed, F. (2012). Effects of Water Quality and Trophic Status on Helminth Infections in the Cyprinid Fish, Schizothorax niger Heckel, 1838 from Three Lakes in the Kashmir Himalayas. J. Helminthol. 86 (01), 70–76. doi:10.1017/S0022149X11000071
Keywords: Nile tilapia, aquatic pollution, infection, bioaccumulation factor, hazard index
Citation: Radwan M, Abbas MMM, Afifi MAM, Mohammadein A and Al Malki JS (2022) Fish Parasites and Heavy Metals Relationship in Wild and Cultivated Fish as Potential Health Risk Assessment in Egypt. Front. Environ. Sci. 10:890039. doi: 10.3389/fenvs.2022.890039
Received: 05 March 2022; Accepted: 10 May 2022;
Published: 23 June 2022.
Edited by:
Elisabetta Manea, National Research Council (CNR), ItalyReviewed by:
Edison Barbieri, Agência de Agronegócio e Tecnologia de São Paulo (APTA), BrazilWynand Malherbe, North-West University, South Africa
Copyright © 2022 Radwan, Abbas, Afifi, Mohammadein and Al Malki. This is an open-access article distributed under the terms of the Creative Commons Attribution License (CC BY). The use, distribution or reproduction in other forums is permitted, provided the original author(s) and the copyright owner(s) are credited and that the original publication in this journal is cited, in accordance with accepted academic practice. No use, distribution or reproduction is permitted which does not comply with these terms.
*Correspondence: Mahmoud Radwan, TWFobW91ZEtocml5LjIwMUBhemhhci5lZHUuZWc=