- 1School of Chemical and Materials Engineering, National University of Science and Technology, Islamabad, Pakistan
- 2Department of Agronomy, PMAS-Arid Agriculture University Murree Road Rawalpindi, Rawalpindi, Pakistan
- 3Department of Chemical Engineering, The University of Faisalabad, Engineering Wing, Faisalabad, Pakistan
- 4Institute of Soil and Environmental Sciences, PMAS Arid Agriculture University, Rawalpindi, Pakistan
- 5Department of Environmental Sciences & Engineering, Government College University Faisalabad, Faisalabad, Pakistan
The rise in global population, urbanization, and desertification pushes the farming community toward intensive cropping to meet the augmented food demands with consequent exhaustion of soil’s nutritional status and fertility. In recent times, environmental pollution and cost of crop production have been enhanced due to excessive and inefficient use of nitrogenous fertilizers. As an abatement strategy, seven different coated urea fertilizers, namely, gelatin-coated (G), zinc oxide with gelatin-coated (ZnOG), zinc oxide-coated (ZnO), sonicated zinc oxide-coated (ZnO-Son), zinc oxide with molasses-coated (ZnOM), zinc sulfate with molasses-coated (ZnSM), and zinc sulfate-coated (ZnS) were prepared in a fluidized bed coater. The coated samples were characterized through XRD, SEM, and FTIR techniques, while a crushing strength test was carried out to assess the impact of inventory operations on physical integrity of coated prills. Pot tests with ryegrass (Lolium perenne) as a test crop were carried out to evaluate the effect of coated urea fertilizers on yield and nitrogen (N) and zinc uptake in order to enhance nitrogen use efficiency and reduce pollution. Our results suggest that the affinity between urea surface and coating materials was of physical nature. All zinc oxide- or gelatin-coated treatments significantly increased the dry matter yield, nitrogen uptake, apparent nitrogen recovery (ANR), zinc uptake, and apparent zinc recovery (AZnR). ZnOG was proved to be the best sample in terms of balancing soil chemical properties with improved soil nutrition and producing best with plant yield (94% higher than UC), N uptake (75% higher than UC), Zn uptake (450% higher than UC), and nitrogen use efficiency (48 vs. 23% for UC). Our results suggest that the use of such coated fertilizers can lead to improve yields, nutritional values of crops, and overall agro-ecological scenario.
1 Introduction
Nitrogen plays a major role in plant growth and its critical processes but due to nitrogen losses of applied urea due to a number of factors result in lower crop production and net economic loss (Trinh et al., 2014; Azeem et al., 2018). These losses occur through ammonia volatilization, leaching, denitrification, and runoff, resulting in very low effectiveness of urea and crop up-taking very less amount of applied urea (30–50%). Nutrient use efficiency and the exhaustive depletion of soil micronutrients are major barriers to a productive and sustainable agricultural system with a forecast of 30% of global agricultural soil turning into degraded land in a few decades (Rashid et al., 2016). Urea prill coating by a wide range of materials such as sulfur, synthetic polymers, biomaterials, and geopolymers has been employed to offset these issues (Azeem et al., 2017).
About 49% of cultivable soils globally are zinc-deficient with low levels of plant-available zinc (Milani et al., 2012). In a study of 250,000 soils and 25,000 plant samples in India, 48% of soils and 44% of plant samples were found deficient in zinc and other micronutrients such as Fe, Cu, and Mn standing at 11, 7, and 5%, respectively (Alloway, 2008). This deficiency is reflected in grains and straws from these crops that subsequently causes malnutrition in human beings when these grains are used as food intake. Nearly three billion people suffer from zinc deficiency globally (Hafeez et al., 2013). Zinc is essential for plant growth (via enzymatic reactions and metabolic processes) and capacity for uptake of water and nutrients. Deficiency of zinc can reduce the yields of crops by up to 40% without showing symptoms of any plant diseases (Das and Green, 2013).
Several studies on the direct application or coating of zinc sources (ZnO/ZnSO4) have revealed a positive impact of zinc supplementation on crop yield. Zinc is also reported to inhibit urease activity effectively while reducing ammonia volatilization losses and enhancing nutrition quality and yield of crops (Bremner and Douglas, 1971). Different crops respond differently to the deficiency of zinc with considerably higher response in case of higher deficiency of Zn in the soil. Pooniya et al. (2017) synthesized 2.5% zinc-coated urea using zinc sulfate heptahydrate and gum acacia as a binder. On the basis of results of 2 years with maize as a test crop, yield increased by 9.9%, total nitrogen uptake by 17.1%, and zinc uptake by 32.4% over conventional urea. Junejo (2012) used zinc and copper sulfate micronutrients as urease inhibitors for coating urea with agar, gelatin, and palm stearin as adhesive agents in a field test with guinea grass as the test crop. All the treatments demonstrated significant advantages with respect to yield and nutrient uptake over uncoated urea.
Most of the coated controlled release urea reported in various studies has not been applicable in large fields due to limitations. Most of the urease inhibitors are expensive with some of them banned due to phytotoxicity in most parts of the world (Junejo, 2012). Polymer-coated urea has limitations of high costs, non-degradability of coating materials, and complexity of the manufacturing process. The use of biodegradable polymers alone has not produced promising results with respect to longevity of nutrients’ release (Naz and Sulaiman, 2016). Separate application of zinc sources has multiple limitations such as 1) farmers’ lack of awareness, 2) low quality and high costs of zinc sources, and 3) extra labor required for field applications.
In the light of the aforementioned facts, we designed a research experiment with the aim to develop single urea-based fertilizer that was supposed to be inexpensive, more efficient in terms of nitrogen use efficiency, and has micronutrient zinc as an essential component. This coating was designed to get added benefits and avoid any extra cost. It was hypothesized that coating materials will not significantly influence the physical and chemical properties of urea and properties of soil in negative terms. It was anticipated that fertilizer treatments will enhance the nutrient (N, P, K, and Zn) availability in soil and may result in increased dry matter (DM) yield and N and Zn uptake in plants as compared to pristine urea (control). The current research work was conducted with the following research objectives: 1) to check the efficacy of zinc-coated urea fertilizers in improving nitrogen use efficiency and nutritional status of experimental soil; 2) to validate the zinc coating of urea prills using advanced analytical techniques like FTIR, XRD, and SEM; and 3) to assess the role of novel zinc-coated urea fertilizers in nutrient use efficiency of ryegrass as the test crop.
2 Materials and methods
2.1 Materials
All chemicals used in this study were of analytical grade and not subjected to any further purification. Urea prills were provided by Fauji Fertilizer Co., Ltd., Pakistan, and were sieved to obtain prills of uniform size (∼2 mm). Zinc oxide, zinc sulfate heptahydrate, paraffin oil, and gelatin were procured from Daejung Korea®. Molasses was obtained from Al-Moiz Sugar Mills, Dera Ismail Khan, Pakistan.
2.2 Synthesis of coating formulations and production of coated urea
Seven different coating formulations were prepared using various components as presented in Table 1. All solutions were prepared in de-ionized water, and constant stirring was employed without any heating. ZnO-Son solution was prepared by sonication of ZnO to ensure good dispersion and uniform suspension.
Urea coating was accomplished in the YX-1000 mini-fluidized spray granulator developed by Shanghai Pilotech Instrument & Equipment Co., Ltd., China. Urea prills of 500 g were introduced at the middle of the bed, and a spray nozzle located at the bottom was used to spray the coating solution. A peristaltic pump rotating at 30 rpm was used to pump the coating solution to the nozzle head. A coating solution was atomized with pressurized air from a compressor. Hot air was blown from the bottom that kept the bed fluidized at all times with a blower frequency of 45 Hz at a constant 0.2 MPa air pressure. To prevent prill agglomeration and the ultimate bed collapse, the intermittent coating was conducted rather than continuous. The coating process was started once a steady temperature (80°C) was achieved, and a 15-min air-drying spell after the spray session concluded a coating run to receive the dried final product. The coated product thus received was subjected to different characterization techniques and pot tests using ryegrass (Lolium perenne) as a test crop.
2.3 Characterization of the coated product
The coated product was analyzed for its morphological (SEM), structural (FTIR and XRD), nutrient release (UV-vis spectrophotometer), and surface (universal testing machine) properties.
The microstructure and surface morphology of samples were examined using a Hitachi S-4700 scanning electron microscope operated at an accelerating voltage of 20 kV. Gold sputtering up to 250 Å was carried out on all samples prior to SEM analysis. The magnification ranged from ×25 to ×2,000. FTIR spectra of samples were achieved using a Perkin Elmer Spectrum 100 spectrometer to study the interaction and bonding nature between urea and coating materials. Pellets were prepared using potassium bromide, and the wavelength for analysis ranged from 400 to 4,000 cm−1.
XRD characterization of samples was carried out on X-Ray JSX 3201, JEOL, Japan (40 kV and 40 mA using Cu Kα-1 radiation), to study crystallinity and confirm the presence of zinc. The diffraction patterns were collected at scan angles varying from 10 to 70 with a step size of 0.04 and one counting time per step (Rashidzadeh and Olad, 2014).
Coated urea fertilizers should have ample mechanical strength to endure physical handling throughout the supply chain and storage without leading to fracture. Therefore, crushing strength was measured by applying increasing pressure to individual prills using a universal testing machine (AGX Plus) that recorded compressive force when prills were crushed (EghbaliBabadi et al., 2015). The nitrogen release behavior of coated samples was investigated using a water dissolution test by the P-methyl amino benzaldehyde method (EghbaliBabadi et al., 2015) Supplementary Figure S2.
2.4 Fertilizer application to the experimental pots
Standard pot tests were conducted at a research farm of Pir Mehr Ali Shah (PMAS) University of Arid Agriculture, Rawalpindi, Pakistan (33.6492°N, 73.0815°E, and 508 m above the mean sea level). The study was conducted starting from December 2020 till June 2021. The temperature during winter remained between 2 and 25°C and 40°C in summer. The pot tests were carried out in clay loam soil and with ryegrass that was selected because it is a perennial crop with a fast germination rate and quick establishment as shown in Figure 2. The experiment consisted of nine treatments labelled as: 1) untreated control (C), 2) uncoated urea (UC), 3) gelatin-coated (G), 4) zinc oxide with gelatin-coated (ZnOG), 5) zinc oxide-coated (ZnO), 6) sonicated zinc oxide-coated (ZnO-Son), 7) zinc oxide with molasses-coated (ZnOM), 8) zinc sulfate with molasses-coated (ZnSM), and 9) zinc sulfate-coated (ZnS). Three similar samples were prepared for all the batches to get better and more accurate results. A sampling of soil and harvesting of the ryegrass was carried out twice during the growing season Supplementary Figure S3.
2.5 Soil analyses
The soil samples from all treatment units were collected thrice. The first sample was taken before applying any fertilizer, the second sample at the time of the first harvest after 109 days, and the third sample at the time of final harvest after 177 days. Samples were taken from three different locations from each pot with the help of a hand auger. These samples from the same pot were then mixed to form a single composite sample and tested for pH, electrical conductivity (EC), dissolved organic carbon, mineral nitrogen (Nmin), plant-available potassium (PAK), plant-available phosphorus (PAP), zinc, and microbial biomass carbon and nitrogen.
The pH and EC of soil were recorded from the suspension of soil and water (1:2.5) equilibrated for 30 min using a multimeter (Ino-Lab® Multi 9430 IDS, WTW, GmbH and Co. KG, Germany) standardized with 0.01 N KCl at 25°C. The soil mineral N, i.e., NH4+-N and NO3−-N, was determined by following two separate methods: the nitrate content in soil solution (NO3-N) was determined using the salicylic acid nitration method (Cataldo et al., 1975), while the ammonium content in soil solution (NH4+-N) was determined by using the indophenol blue method (Keeney et al., 1982).
The plant-available potassium (PAK) and plant-available phosphorus (PAP) were determined by using a flame photometer (Jenway, PFP7) following the methods as reported by Jackson (1962). The zinc content of the soil was extracted using the di-acid method and analyzed using atomic absorption spectroscopy (Jones, 2001).
The dissolved organic carbon content was evaluated by using the method described by Altaf and Rashid (2021). The carbon and nitrogen contents in microbial biomass were determined using the fumigation–extraction method (Brookes et al., 1985; Vance et al., 1987). Microbial biomass carbon (MBC) and microbial biomass nitrogen (MBN) were calculated by the following equation:
where TNf and TNnf are total nitrogen in fumigated and nonfumigated samples, respectively, and kEC and kEN are 0.45 and 0.54 used for the calculation of MBC (Jenkinson et al., 2004) and MBN (Brookes et al., 1985; Joergensen and Mueller, 1996), respectively.
2.6 Plant analyses
The grass was harvested two times during the experiment period after 109 and 177 days of sowing. Plant height was recorded only once at the maturity level from three different locations in the pot before the first harvest using a meter rod. Roots were extracted at the final harvest by taking out the whole soil clump and placing it in cold water. After keeping it soaked for 2 h, root parts were placed in a 0.5-mm mesh and put under high-speed tap water to separate soil from it. They were further dried in an oven at 70°C for 48 h to determine dry matter (DM) yield (Ali et al., 2019). The nitrogen content in the plant was determined using the Kjeldahl digestion method. For Zn analysis, 1.0 g of ground dry plant powder was added to the digestion tube. A volume of 10 ml of concentrated sulfuric acid (H2SO4) was added to the digestion tube and heated for 1.0 h at 145°C. Additionally, 5 ml of the tri-acid mixture was added, and the temperature was increased to 240°C for another 1.0 h. The samples were allowed to cool to room temperature and filtered with Whatman filter paper no. 42. The samples were analyzed using a atomic absorption spectrophotometer (Hitachi Polarized Zeeman, ZA3000 Series). Using the N and Zn uptake values, apparent nitrogen recovery (ANR) and apparent zinc recovery (AZnR) for coated samples were calculated as follows:
where Ns or Zns, respectively, are nitrogen or zinc contents in the ryegrass sample, DMs represents the dry matter yield of the treatment, No or Zno indicates nitrogen or zinc content in the control treatment, respectively, DMo represents the dry matter yield of control treatment, and TNa is the total nitrogen applied in the pots.
2.7 Statistical analysis
The pot test experimental data were statistically analyzed using analysis of variance (ANOVA) to determine treatment effects on soil and plants. When a significant treatment difference was detected by the F-test, mean comparisons were made using Tukey’s test at 5% probability. The tests were performed using the SPSS Statistics 17.0 (IBM, New York, United States) statistical software package.
3 Results
3.1 Physical and chemical properties of fertilizers
3.1.1 Surface morphology
The coating surface was analyzed for uniformity, shape, and structure of the coating layer deposited on the urea prills’ surface. The SEM images showed significant differences between morphologies for different coated samples as illustrated in Figure 1. Samples with additives, i.e., molasses and gelatin produced more uniform surface morphology as compared to samples with only a waterborne coating. Samples with a coating of waterborne zinc solution (Figures 1B–E) appeared to have an irregular and fissured surface that may potentially lead to an unstable fracture of the coating film, especially in zinc oxide samples (Figures 1D,E), numerous gaps and pores were visible. Coating particles were more randomly spread over the surface. Sonication of waterborne zinc oxide solution was found to have no significant advantages apart from uniformity of the coating layer to a little extent (Figure 1E). Molasses and paraffin oil improved the uniformity for both zinc sulfate and zinc oxide samples and adhesion between zinc and urea (Figures 1C,F). Agglomeration was observed over the prills’ surface for zinc sulfate-coated samples (Figures 1B,C). Gelatin with its film formation properties played a positive role by forming a membrane-like structure and making a compact film over the surface (Figure 1H). The combination of gelatin with zinc oxide showed a nearly homogenous distribution of coating on the surface of urea prills (Figure 1G).
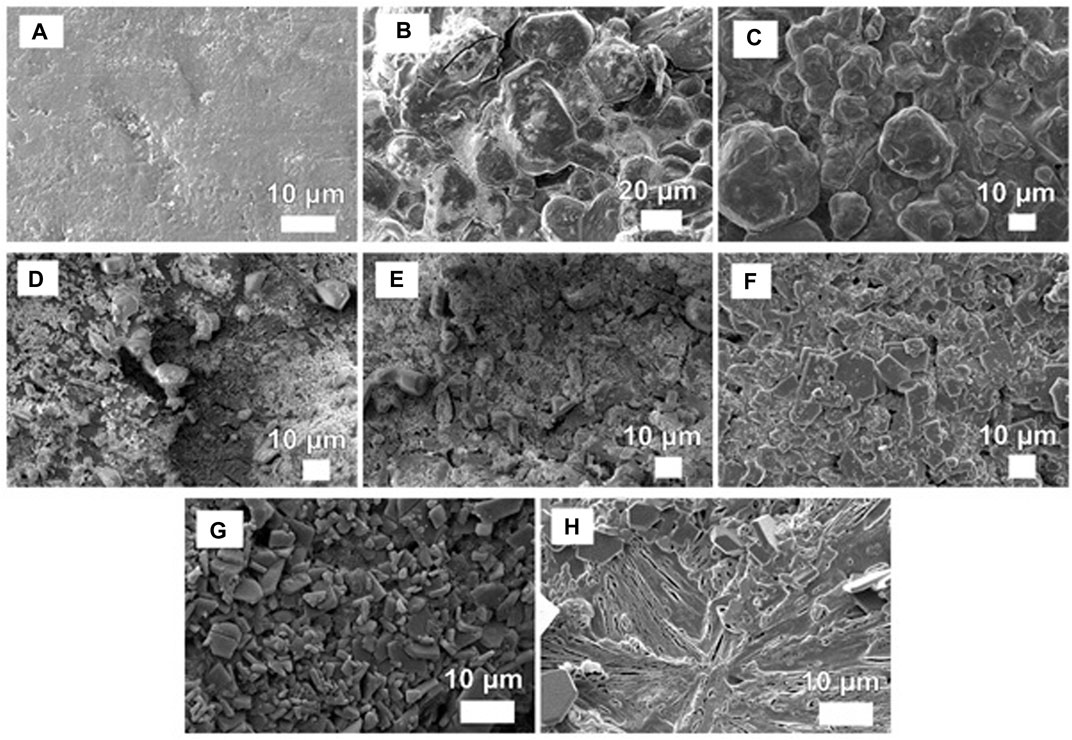
FIGURE 1. SEM images of fertilizer samples. (A) UC, (B) ZnS, (C) ZnSM, (D) ZnO, (E) ZnO-Son, (F) ZnOM, (G) ZnOG, and (H) G.
3.1.2 Fourier transform infrared spectroscopy (FTIR)
The FTIR spectrum showed the presence of different functional groups with IR spectra of coated urea samples almost exactly similar to that of uncoated urea as shown in Figure 2. Corresponding peaks were assigned as: 3,447 cm−1 and 3,343 cm−1 to asymmetric and symmetric stretching vibrations of N-H, respectively, 1,688 cm−1 to the carbonyl functional group (C=O), and 1,613 cm−1 to binding vibration of N-H and stretching vibration of C-H. A medium intensity band at 1,425 cm−1 and 1,154 cm−1 was attributed to vibrational stretching of C-N and C-C-C bonds, respectively. No significant variations in FTIR spectra suggested no chemical bond formation; thus, component coating materials were attached to urea particles mainly through physical mechanisms, that is, hydrogen bonds, Van der Waals forces, and electrostatic attraction.
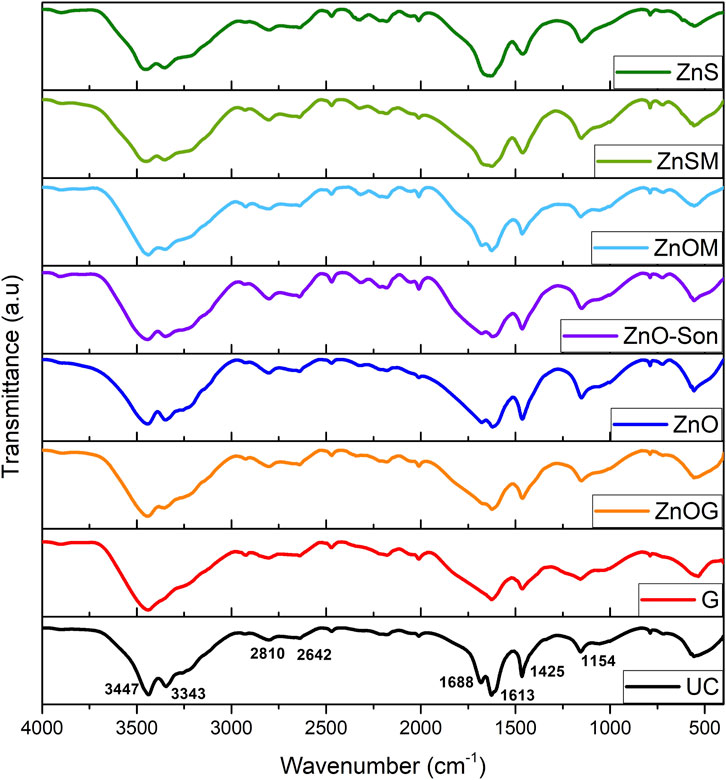
FIGURE 2. FTIR images of fertilizer samples, namely, UC, G, ZnOG, ZnO, ZnO-Son, ZnOM, ZnSM, and ZnS.
3.1.3 XRD
The XRD spectra of fertilizer samples (Figure 3) indicated no shift in the position of peaks after surface modification of urea prills with different coating materials. The uncoated urea prills showed dominant peaks at 2θ = 22o, 2θ = 24.5o, 2θ = 29.5o, and 2θ = 36o. The spectra of all coated samples showed sharp peaks similar to uncoated urea. The sharp peaks represented high crystallinity indicating that a clear coating was formed on the urea surface. The XRD patterns indicated the presence of Zn (in form of ZnO) on the surface with major peaks at 2θ = 36o and 2θ = 42o, and minor peaks approximately at 2θ = 55o and 2θ = 68o for zinc oxide-coated samples (Figure 3: ZnO, ZnO-Son, ZnOM, and ZnOG). The XRD spectra also suggested that zinc sulfate and gelatin were present in poor crystalline forms and were, therefore, not detected by XRD. All the spectra were almost alike, and all the peaks observed could be assigned to base urea material or the coating materials. With no substantial differences in spectra, it can be stated that the interaction between urea and coating materials was of physical nature, and the overall structure was not significantly modified.
3.1.4 Crushing strength
The crushing strength of pure and coated urea prills randomly picked from samples has been represented in Figure 4. Coating the samples did not improve the crushing strength apart from gelatin-coated urea (G) that showed a slight increase in crushing strength. Rest of the coated samples had either similar or lower crushing strength than pristine urea prills. Maximum crushing strength was recorded for the G sample (6.62 N) that was closely followed by uncoated urea (6.45 N). The minimum crushing strength of 4.31 N was recorded for the ZnSM sample. The results were satisfactory with no significant reduction in crushing strength (P
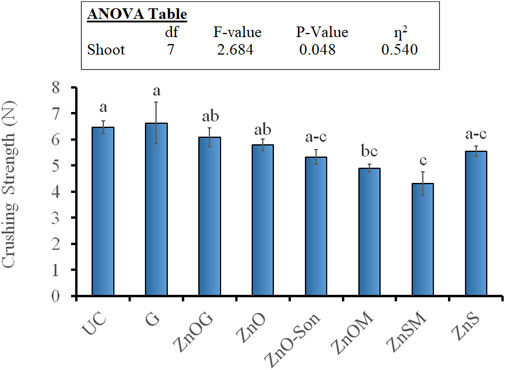
FIGURE 4. Crushing strength of fertilizer samples. Small letters over each bar indicate the difference among treatments at a 5% probability level. Error bars represent the standard error of the mean (n = 3). Inset in the figure represent the result of the analysis of variance (ANOVA).
3.1.5 Effect of coatings on the dissolution rate of urea
All samples were tested for the release rate to compare the effect of coatings on the release rate of urea. Figure 5 shows the release profile (percentage of nitrogen vs. time) of all coated samples. Uncoated urea prills released their nutrients quickly, and a complete release was observed between 6 and 9 min with 90% of nutrients released in 6 min. With no coating over its surface and high solubility of urea in water, the release of nutrients occurs at a very quick pace following the burst release mechanism. A similar profile was obtained for samples coated with zinc-only sources, that is, ZnO, ZnO-Son, ZnS, and zinc sources along with molasses (ZnOM and ZnSM). A relatively sluggish release of nutrients was observed for gelatin-coated samples (G and ZnOG) with 38% release in 6 min and 95% release in 15 min while following a comparatively less steep path. The hydrophilic nature of gelatin made a swollen hydrogel layer on the surface of granules. Water penetrates through it, dissolves solute urea, and a gradual release of nutrient through liquid bridges in the swollen coating layer is achieved.
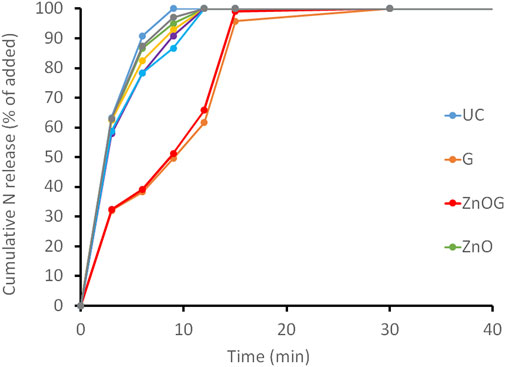
FIGURE 5. Release profile of coated samples, namely, UC, G, ZnOG, ZnO, ZnO-Son, ZnOM, ZnSM, and ZnS.
3.2 Soil chemical properties and nutrient availability
The pH of the soil was found to be reduced for all coated treatments in the first 109 days (Figure 6A). The lowest pH (6.96) was recorded for ZnOG in comparison to 8.23 for UC after 109 days. However, this reduction in pH disappeared after 177 days, and no significant differences among the treatments were observed in the entirety of this agronomic study (p > 0.05). Soil EC was not affected by coating, and all the coated treatments were statistically the same as uncoated urea (p > 0.05) (Figure 6B). Soil mineral N was significantly influenced during the first 109 days (p < 0.001) (Figure 6C). However, multiple comparisons showed that all the treatments were statistically the same and not significantly different after 109 days. Overall, the mineral N decreased for all treatments with time. All the zinc-coated samples showed significant improvement over uncoated urea in soil mineral for first 109 days with an increase of 108% for ZnOG (25 vs. 12 kg/ha), 100% for ZnO (24 vs. 12 kg/ha), 58% for ZnO-Son and ZnOM (19 vs. 12 kg/ha), 42% for ZnSM (17 vs. 12 kg/ha), and 33% for ZnS (16 vs. 12 kg/ha). Gelatin-coated urea (G) showed similar results as uncoated urea (UC).
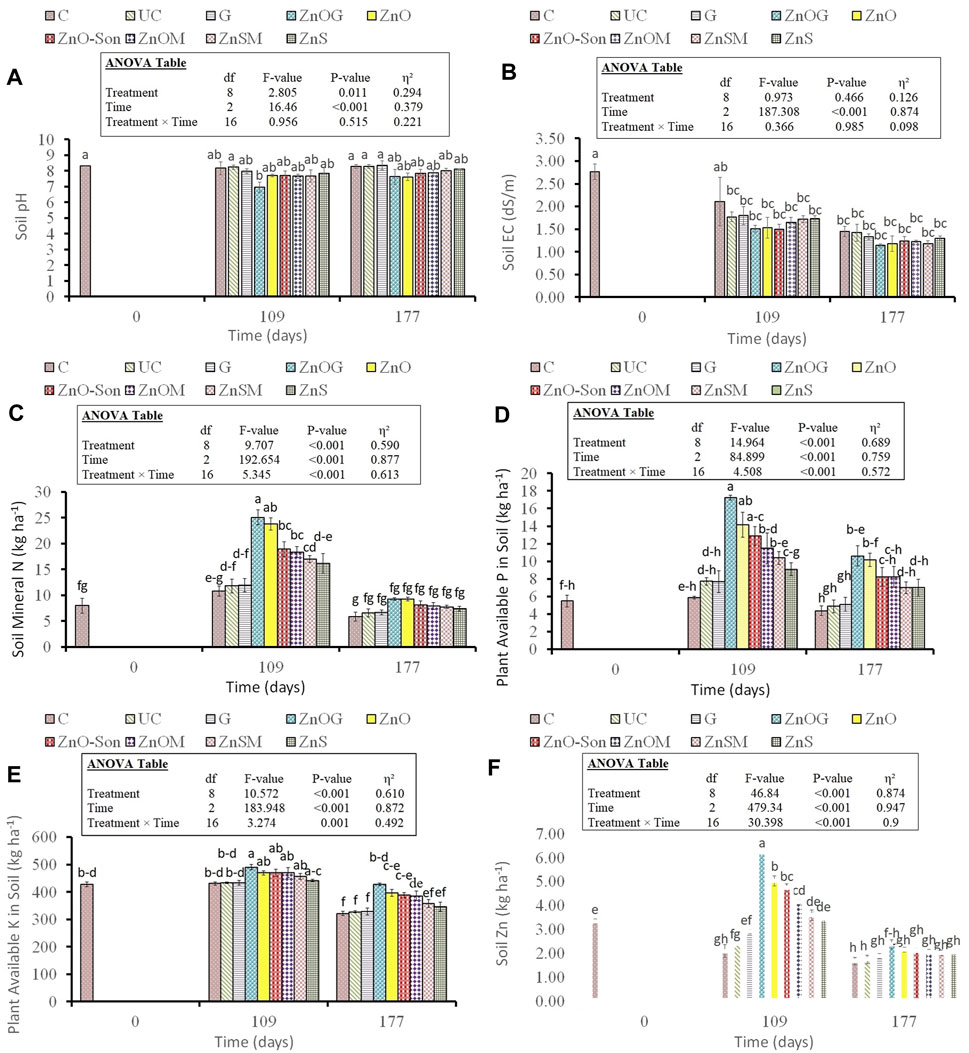
FIGURE 6. Soil pH (A), electrical conductivity (EC) (B), mineral nitrogen (Nmin) (C), plant-available phosphorus (D), plant-available potassium (E), and soil zinc content (F) in different treatments at various time intervals during the growth season of ryegrass. Small letters over each bar indicate the difference among treatments at a 5% probability level. Error bars represent the standard error of the mean (n = 3). Inset in the figure represent the result of the analysis of variance (ANOVA).
Plant-available P was significantly affected by treatments, time, and their interaction (p-value < 0.001) (Figure 6D). Apart from G, the plant-available P was found to increase significantly as compared to uncoated urea (UC) for all coated samples. The highest amount of P was recorded after 109 days for ZnOG (17.23 vs. 7.72 kg/ha) followed by ZnO (14.14 vs. 7.72 kg/ha), ZnO-Son (12.89 vs. 7.72 kg/ha), ZnOM (11.47 vs. 7.72 kg/ha), ZnSM (10.39 vs. 7.72 kg/ha), and ZnS (9.1 vs. 7.72 kg/ha). Overall, the trend of plant-available P decreased with time.
Similarly, plant-available K (PAK) also increased for all coated samples apart from G in comparison to uncoated urea (UC) (Figure 6E). The highest amount of PAK is recorded after 109 days for ZnOG (490 vs. 433 kg/ha) followed by ZnO (470 vs. 433 kg/ha), ZnO-Son (469 vs. 433 kg/ha), ZnOM (469 vs. 433 kg/ha), ZnSM (456 vs. 433 kg/ha), and ZnS (442 vs. 433 kg/ha). Generally, a decreasing trend was observed with time, and all treatments were found significantly different (p < 0.05). Soil Zn was significantly influenced during the first 109 days (p-value < 0.001) (Figure 6F). The largest increment in soil Zn over UC was recorded after 109 days as 159% for ZnOG (6.22 vs. 2.4 kg/ha), 109% for ZnO (5.02 vs. 2.4 kg/ha), 97% for ZnO-Son (4.73 vs. 2.4 kg/ha), 72.5% for ZnOM (4.14 vs. 2.4 kg/ha), 49.6% for ZnSM (3.59 vs. 2.4 kg/ha), and 44% for ZnS (3.45 vs. 2.4 kg/ha). However, differences among the treatments were not found statistically significant after 109 days. As a whole, soil Zn decreased for all treatments with time.
3.3 Dissolved organic carbon and microbial biomass
Dissolved organic C was significantly influenced during the first 109 days (p-value < 0.001) (Figure 7A). However, comparative analysis revealed that all the treatments are statistically the same after 109 days. Overall, dissolved organic C decreased for all treatments with time until 109 days. All the zinc-coated samples showed significant improvement over uncoated urea in dissolved organic C for the first 109 days with a percentage increment of 72% for ZnOG (9.08 vs. 5.27 mg/kg), 49% for ZnO (7.86 vs. 5.27 mg/kg), 46% for ZnO-Son (7.72 vs. 5.27 mg/kg), 45% for ZnOM (7.62 vs. 5.27 mg/kg), 21% for ZnSM (6.39 vs. 5.27 mg/kg), and 8% for ZnS (5.68 vs. 5.27 mg/kg). Gelatin-coated urea (G) showed similar results as uncoated urea (UC). Apart from gelatin-coated urea (G), both microbial biomass carbon (MBC) and microbial nitrogen (MBN) increased in soil for all the coated samples (p < 0.05; Figure 7B). The highest MBC was recorded for ZnOG with a % increase over uncoated urea by 153% (233 vs. 92 mg/kg), 133% for ZnO (214 vs. 92 mg/kg), 116% for ZnO-Son (199 vs. 92 mg/kg), 99% for ZnOM (183 vs. 92 mg/kg), 66% for ZnSM (153 vs. 92 mg/kg), and 33% for ZnS (122 vs. 92 mg/kg). Similar increments were observed for MBN with the highest increment of 130% over uncoated urea for ZNOG (69 vs. 30 mg/kg) followed by 103% for ZnO (61 vs. 30 mg/kg), 93% for ZnO-Son (58 vs. 30 mg/kg), 87% for ZnOM (56 vs. 30 mg/kg), and 73% for ZnSM and ZnS (52 vs. 30 mg/kg).
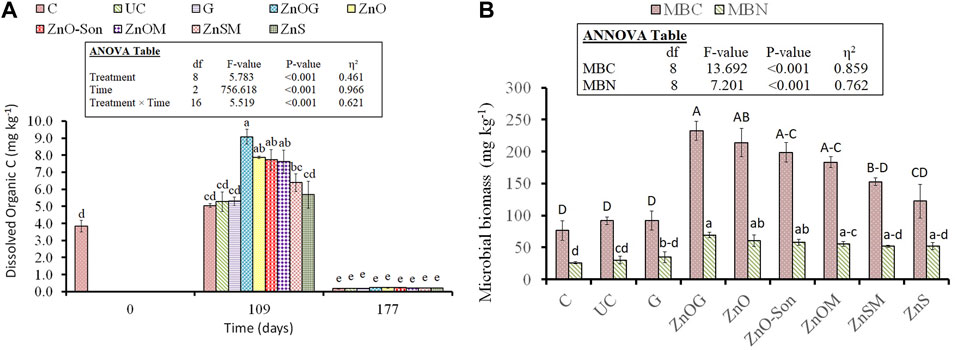
FIGURE 7. Soil-available carbon (A) and soil microbial biomass carbon (MBC) and nitrogen (MBN) (B). Small letters over each bar indicate the difference among treatments at a 5% probability level. Error bars represent the standard error of the mean (n = 3). Inset in the figure represent result of the analysis of variance (ANOVA).
3.4 Plant height, yield, and N and Zn uptake
Plant height was significantly increased with ZnOG and G treatments. All the ZnO-coated treatments showed a difference between uncoated urea and the control. However, ZnSO4 appeared almost similar in results to the conventional urea. The maximum plant height (70 cm) was recorded for ZnOG followed by 68 and 59 cm in the case of G and UC treatments, respectively (Figure 8A). The shoot dry matter yields were higher for ZnO and gelatin-coated treatments. The highest dry matter yields were recorded for ZnOG with an increase in yield of 94% (8,746 vs. 4,502 kg/ha) over uncoated urea (UC), followed by an increase of 45% for G (6,530 vs. 4,502 kg/ha), 31% for ZnO (5,884 vs. 4,502 kg/ha), 10% for ZnO-Son (4,948 vs. 4,502 kg/ha), and 6% for ZnOM (4,771 vs. 4,502 kg/ha) (Figure 8B). The multiple mean comparisons indicated that the difference among treatments was significant (p < 0.05) over control. Zinc sulfate-coated samples did not result in any increase in yields and were not statistically different (p > 0.05) from uncoated urea. For root dry matter yield, a significant increase was observed for the ZnOG sample over UC (1,580 vs. 576 kg/ha), while the rest of the treatments were statistically at par with uncoated urea.
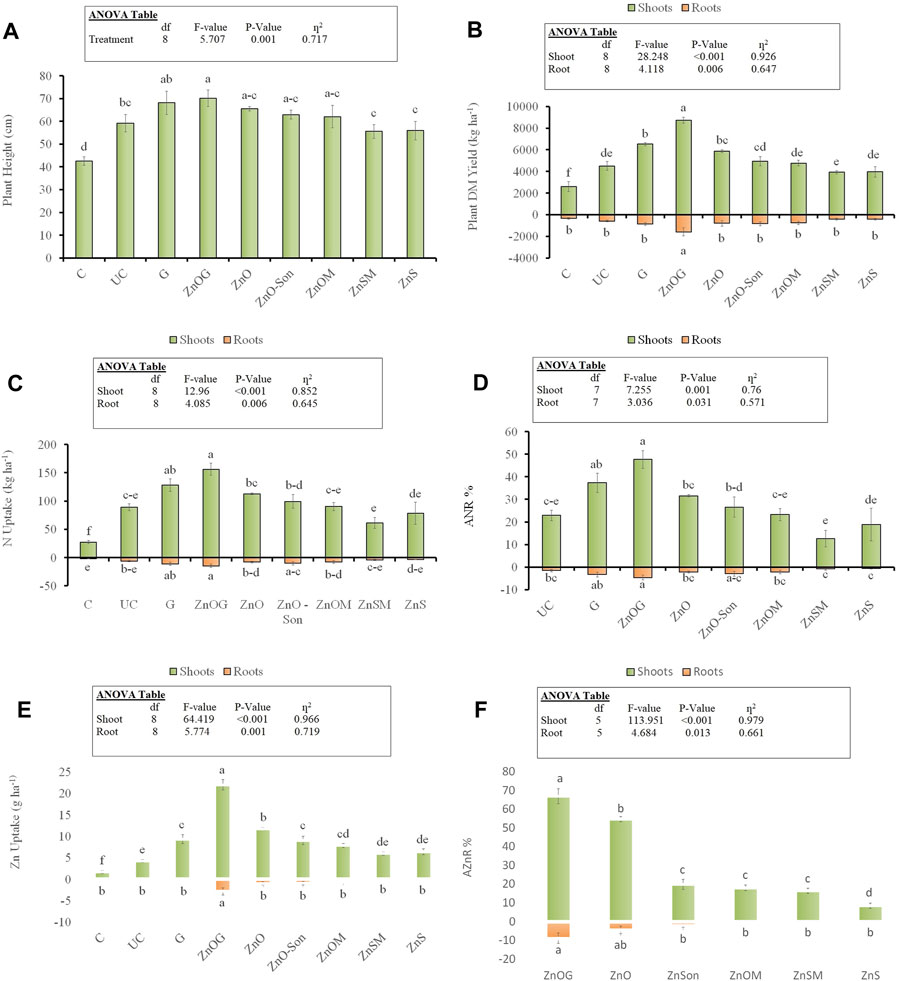
FIGURE 8. Ryegrass plant height (A), shoot and root DM yield (B), shoot and root N uptake (C), shoot and root apparent N recovery (ANR) (D), shoot and root Zn uptake (E), and shoot and root apparent Zn recovery (AZnR) (F). Small letters over each bar indicate the difference among treatments at a 5% probability level. Error bars represent the standard error of the mean (n = 3). Inset in the figure represent the result of the analysis of variance (ANOVA).
Similar to the dry matter, shoot N uptake was significantly increased with N uptake for ZnOG being 75% (156 vs. 89 kg/ha) higher than uncoated urea (UC), followed by an increase of 44% for G (128 vs. 89 kg/ha), 27% for ZnO (113 vs. 89 kg/ha), and 11% for ZnO-Son (99 vs. 89 kg/ha) (Figure 8C). The rest of the samples did not show promising effects with ZnSO4-coated samples having a negative impact on nitrogen uptake. Likewise, the shoot ANR was significantly improved with ANR for ZnOG (48%), G (37%), ZnO (32%), ZnO-Son (27%), ZnOM (23%), UC (23%), ZnS (19%), and ZnSM (13%) treatments (Figure 8D). The increase in nitrogen use efficiency was in agreement with the dissolution results of gelatin-coated urea. ANR for roots also improved for all ZnO and gelatin-coated samples as compared to UC, that is, ZnOG (4.6% vs. 1.5%), G (3.4% vs. 1.5%), ZnO-Son (2.9% vs. 1.5%), ZnO (2.2% vs. 1.5%), and ZnOM (2.2% vs. 1.5%). All the treatments were significantly different for the aforementioned parameters (p < 0.05).
We found that shoot Zn uptake was significantly higher in all coated treatments (p < 0.05). The shoot Zn uptake was improved by 450% (22 vs. 4 g/ha) over uncoated urea for ZnOG followed by an increase of 200% for ZnO (12 vs. 4 g/ha), 125% for ZnO-Son (9 vs. 4 g/ha), 125% for G (9 vs. 4 g/ha), 100% for ZnOM (8 vs. 4 g/ha), and 50% for ZnSM and ZnS (6 vs. 4 g/ha) (Figure 8E). The order for the resulting shoot apparent Zn recovery (AZnR) was ZnOG (67%), ZnO (55%), ZnO-Son (20%), ZnOM (18%), ZnSM (17%), and ZnS (9%) for the treatments (Figure 8F). For roots, the increase in Zn uptake over UC was significant for ZnOG (2.84 vs. 0.34 g/ha) whereas rest of the treatments were at par with UC.
4 Discussion
Clear coating is formed over the prills’ surface for all coated treatments. Gelatin with film-forming properties formed a smoother and compact coating layer over the surface, and its compatibility with ZnO was very good. Molasses along with paraffin oil that was used as a binder for ZnO and ZnSO4 improved coating uniformity over the surface to some extent (Zhang et al., 2021). ZnSO4-coated treatments produced agglomerates and lumps, while coating with ZnO was composed of fine particles. ZnO combined with gelatin produced the best coating in terms of uniformity and zinc distribution over the prills’ surface. The results of XRD and FTIR vindicate our hypothesis that the coating particles did not alter the physical and chemical properties of urea significantly. The XRD peaks were as sharp as for uncoated urea which showed a higher crystallinity. No significant shift in peaks’ position was observed which showed that the affinity between the coating formulation and urea particles was merely of physical nature, and new phases were not formed. All the peaks and functional groups were associated with the base or coating materials.
From an inventory and transportation perspective, the samples with better strength are preferred. However, in the current experiment crushing strength of coated samples was found to be comparable with the control (pristine urea prills). Similar results were also reported by Mohd Ibrahim et al. (2014), and they showed that none of the coated samples showed superior strength in comparison to uncoated urea. The release test for gelatin-coated (G) and conventional urea (UC) supported our hypothesis that gelatin retards the release rate of urea. Uncoated urea and coated samples without gelatin followed the burst release mechanism with a complete nutrient release in 6–9 min. Gelatin-coated urea (G and ZnOG) delayed the release till 15 min due to its film-forming properties and acted as a barrier between nutrient core and water. The swollen coating film allowed water penetration with the subsequent diffusion release mechanism of dissolved nutrients (Imran et al., 2018).
According to our hypothesis, coated treatments do not influence pH or electrical conductivity of soil adversely. Soil EC was not impacted by coated treatments as compared to uncoated urea. However, pH of the soil was slightly decreased for all zinc-coated treatments. The decrease in pH for zinc sulfate-coated treatments may be attributed to the acidic effect of zinc sulfate dissolution in water. For zinc oxide, it resulted in an alkaline reaction consuming H+ ions in coated treatments, but the soil provided a pull for zinc and retarded the pH increase around the oxide particle (or provided H+ ions) resulting in the dissolution of zinc oxide (Ali et al., 2019). The optimal pH for most of the plants ranges from 5.5 to 7. Above this range, the soil was at greater risk of nitrogen losses. Conversion of ammonia from ammonium is 0.06% at pH ≤ 6, 0.6% at pH ≤ 7, 5.4% at pH ≤ 8, and 36.5% at pH ≤ 9. The pH of the soil for the ZnOG sample after 109 days was noted to be 6.96, lying in the optimal range for plant growth as compared to 8.23 for UC. Similarly, a decreasing trend of Zn availability was reported for the increasing pH (Hafeez et al., 2013). As per the reported literature, in the case of soils with high pH and OH− concentration, it is difficult to get a positive response from the crop even with Zn application (Frame, 2017).
The soil mineral N content and nutrient availability, that is, PAP and PAK were significantly increased (p < 0.05) for all zinc-coated treatments. This increment in soil mineral N and plant-available nutrients was due to the mobilization of nutrients triggered by various enzymatic activities such as urease or phosphatase activity in the soil and microbial population of the soil when we used zinc-coated products. The enzymes played a vital role in the regularization of available nitrogen and phosphorus in the soil (Frame, 2017). The present study showed that in the first 109 days of the experiment dissolved organic carbon (DOC) was significantly improved in coated urea treatments in comparison to the control treatment which might be due to readily available organic compounds like plant root exudates, decomposable organic matter, and sloughed off root cap cells (Ghosh et al., 2012). DOC results of the current experiment are also in line with the findings of Zhu et al. (2020) who reported significant improvements in DOC as a result of coated urea fertilizers. Several other studies also support the fact that the application of N fertilizers improves DOC and subsequent crop growth and production (Mehmood et al., 2018; Liyuan et al., 2020).
It was observed that microbial biomass carbon and nitrogen were significantly higher in the case of all zinc-coated treatments as compared to UC and C treatments which indicates that zinc coating improved enzymatic activities leading to increased soil mineral N, PAP, and PAK in the soil. The nutrient availability in soil significantly reduced after 109 days and first harvest, but it was still higher than control and uncoated urea for the coated treatments Supplementary Figure S1.
Our results also supported our hypothesis in terms of dry matter yield, nitrogen uptake, and apparent nitrogen recovery in zinc oxide or gelatin-coated treatments. Similar results were reported by Pooniya et al. (2017) where the results of 2 years with maize as a test crop revealed that yield increased by 9.9%, total nitrogen uptake by 17.1%, and zinc uptake by 32.4% over conventional urea with the application of 2.5% zinc-coated urea. The findings for gelatin along with zinc-coated urea (ZnOG) were also in line with the results by Junejo (2012) who used micronutrients (zinc and copper sulfate) as urease inhibitors for coating of urea and reported an augmented crop yield with fertilizer nutrients released in synchrony with metabolic needs of the plants. This fact supported the higher crop yield, nitrogen uptake, and ANR than uncoated urea in our study (Olander and Vitousek, 2000). Our results are also in line with many other studies where coated treatments successfully delayed hydrolysis of urea as compared to conventional urea and achieved better nitrogen use efficiencies (Yaseen et al., 2021). Contrary to our expectation, we found that ZnSO4-coated treatments did not increase the dry matter yield, nitrogen uptake, and ANR, although the soil mineral N and plant-available nutrients were increased. This emphasized that other factors might also play a role like the increase in microbial biomass N and Zn could lead to immobilization of nutrients in the soil that leads to a reduction in their uptake by the plant. Thus, more studies are required to fully understand the mechanism of nitrogen and other nutrient uptake by plants as influenced by zinc sulfate-coated treatments (Azeem et al., 2017). However, the application of Zn coating significantly improved the available Zn contents in soil and Zn uptake by the plant for all Zn-coated samples as per our hypothesis (Shivay and Prasad, 2012).
5 Conclusion
In the aforementioned results and discussion, it was concluded that ZnOG was the best treatment among all, and it significantly improved the plant height and yield, nitrogen uptake, and nitrogen use efficiency followed by G and ZnO treatments. ZnOG treatment showed promising results in increasing nitrogen use efficiency and provided zinc micronutrients efficiently to soil and plant along with the improvement in the availability of other soil nutrients, that is, Nmin, PAP, and PAK. Gelatin alone may bring adequate benefits where soils are not severely Zn-deficient. The use of zinc oxide alone (ZnO) is a promising and economically feasible option to meet zinc nutrition requirement and improve plant productivity. In comparison, uncoated urea showed low efficiency and yield due to higher nitrogen losses. Our study clearly demonstrated the positive outcomes and benefits of use of zinc-alone and zinc with gelatin coatings keeping economic and manufacturing feasibility in consideration. These are important findings for environmental sustainability with urea being prone to hazardous losses and low efficiencies.
Data availability statement
The original contributions presented in the study are included in the article/Supplementary Material; further inquiries can be directed to the corresponding author.
Author contributions
BA and MN conceived the idea of the experiments. ZJ, GS, ZI, and BA carried out the experiments and analyzed the data. MZ and GS provided support during the pot test. BA, MN, and OK wrote the manuscript with support from all co-authors. The authors declare no competing financial interests.
Funding
This work was supported by the Pakistan Science Foundation (PSF) under the PSF/CRP/C-NUST/T-Helix (186) research project titled “Development of value added zincated urea with slow-release nitrogen feature.” PI is thankful to the Pakistan Science Foundation (PSF) for funding this project.
Conflict of interest
The authors declare that the research was conducted in the absence of any commercial or financial relationships that could be construed as a potential conflict of interest.
Publisher’s note
All claims expressed in this article are solely those of the authors and do not necessarily represent those of their affiliated organizations, or those of the publisher, the editors, and the reviewers. Any product that may be evaluated in this article, or claim that may be made by its manufacturer, is not guaranteed or endorsed by the publisher.
Supplementary material
The Supplementary Material for this article can be found online at: https://www.frontiersin.org/articles/10.3389/fenvs.2022.888865/full#supplementary-material
Supplementary Figure S1 | Plants before first harvest.
Supplementary Figure S2 | Calibration curve between concentration and absorbance of known samples of urea.
Supplementary Figure S3 | Pots preparation of all treatments.
References
Ali, B., Shah, G. A., Traore, B., Shah, S. A. A., Shah, S. U., Al-Solaimani, S. G. M., et al. (2019). Manure storage operations mitigate nutrient losses and their products can sustain soil fertility and enhance wheat productivity. J. Environ. Manag. 241, 468–478. doi:10.1016/j.jenvman.2019.02.081
Altaf, B. A. S., and Rashid, M. I. (2021). Home field advantage describes the carbon and nitrogen dynamics of Cynodon dactylon and Phoenix dactylifera leaf litters in arid sandy soil. Appl. Soil Ecol. 157, 103728. doi:10.1016/j.apsoil.2020.103728
Azeem, B., KuShaari, K., Man, Z., and Irfan, S. A. (2018). Parametric study of tumbling fluidized bed to evaluate nitrogen release characteristics of biopolymer-coated controlled release urea. Chem. Eng. Commun. 205 (10), 1397–1414. doi:10.1080/00986445.2018.1451993
Azeem, B., KuShaari, K., Man, Z., and Trinh, T. H. (2017). Nutrient release characteristics and coating homogeneity of biopolymer coated urea as a function of fluidized bed process variables. Can. J. Chem. Eng. 95 (5), 849–862. doi:10.1002/cjce.22741
Bremner, J. M., and Douglas, L. A. (1971). Inhibition of urease activity in soils. Soil Biol. Biochem. 3 (4), 297–307. doi:10.1016/0038-0717(71)90039-3
Brookes, P. C., Landman, A., Pruden, G., and Jenkinson, D. S. (1985). Chloroform fumigation and the release of soil nitrogen: A rapid direct extraction method to measure microbial biomass nitrogen in soil. Soil Biol. Biochem. 17 (6), 837–842. doi:10.1016/0038-0717(85)90144-0
Cataldo, D. A., Maroon, M., Schrader, L. E., and Youngs, V. L. (1975). Rapid colorimetric determination of nitrate in plant tissue by nitration of salicylic acid. Commun. Soil Sci. Plant Anal. 6, 71–80. doi:10.1080/00103627509366547
Eghbali Babadi, F., Yunus, R., Abdul Rashid, S., Mohd Salleh, M. A., and Ali, S. (2015). New coating formulation for the slow release of urea using a mixture of gypsum and dolomitic limestone. Particuology 23, 62–67. doi:10.1016/j.partic.2014.12.011
Frame, W. (2017). Ammonia volatilization from urea treated with NBPT and two nitrification inhibitors. Agron. J. 109 (1), 378–387. doi:10.2134/agronj2016.08.0464
Ghosh, S., Wilson, B., Ghoshal, S., Senapati, N., and Mandale, B. (2012). Organic amendments influence soil quality and carbon sequestration in the Indo-Gangetic plains of India. Agric. Ecosyst. Environ. 156, 134–141. doi:10.1016/j.agee.2012.05.009
Hafeez, B., Khanif, Y., and Saleem, M. (2013). Role of zinc in plant nutrition—a review. Am. J. Exp. Agric. 3, 374–391. doi:10.9734/ajea/2013/2746
Imran, M., Irfan, M., Yaseen, M., and Rasheed, N. (2018). Application of glycerin and polymer coated diammonium phosphate in alkaline calcareous soil for improving wheat growth, grain yield and phosphorus use efficiency. J. Crop Sci. Biotechnol. 21 (5), 425–434. doi:10.1007/s12892-018-0126-0
Jenkinson, D. S., Brookes, P. C., and Powlson, D. S. (2004). Measuring soil microbial biomass. Soil Biol. Biochem. 36 (1), 5–7. doi:10.1016/j.soilbio.2003.10.002
Joergensen, R. G., and Mueller, T. (1996). The fumigation-extraction method to estimate soil microbial biomass: Calibration of the kEN value. Soil Biol. Biochem. 28 (1), 33–37. doi:10.1016/0038-0717(95)00101-8
Junejo, N. (2012). Evaluation of coated urea for the effects of coating on the physical and chemical properties of urea fertilizer. Int. J. Phys. Sci. 7 (13), 1250. doi:10.5897/ijps11.1250
Keeney, D. R., and Nelson, D. W. (1982). “Nitrogen - inorganic forms,” in Methods of soil analysis, Part 2. Editors A. L. Page, and R. H. Miller (United kingdom: Routledge publisher, Taylor & Francis Group).
Liyuan, L., Chuanzong, L., Shuhao, Z., Yan, X., Houyu, L., Xiangqun, Z., et al. (2020). Combined application of organic and inorganic nitrogen fertilizers affects soil prokaryotic communities compositions. Agronomy 10 (1), 132. doi:10.3390/agronomy10010132
Mehmood, K., Baquy, M. A-A., and Xu, R-K. (2018). Influence of nitrogen fertilizer forms and crop straw biochars on soil exchange properties and maize growth on an acidic Ultisol. Archives Agron. Soil Sci. 64 (6), 834–849. doi:10.1080/03650340.2017.1385062
Milani, N., McLaughlin, M. J., Stacey, S. P., Kirby, J. K., Hettiarachchi, G. M., Beak, D. G., et al. (2012). Dissolution kinetics of macronutrient fertilizers coated with manufactured zinc oxide nanoparticles. J. Agric. Food Chem. 60 (16), 3991–3998. doi:10.1021/jf205191y
Mohd Ibrahim, K. R., Eghbali Babadi, F., and Yunus, R. (2014). Comparative performance of different urea coating materials for slow release. Particuology 17, 165–172. doi:10.1016/j.partic.2014.03.009
Naz, M. Y., and Sulaiman, S. A. (2016). Slow release coating remedy for nitrogen loss from conventional urea: A review. J. Control. Release 225, 109–120. doi:10.1016/j.jconrel.2016.01.037
Olander, L. P., and Vitousek, P. M. (2000). Regulation of soil phosphatase and chitinase activityby N and P availability. Biogeochemistry 49 (2), 175–191. doi:10.1023/a:1006316117817
Pooniya, V., Choudhary, A. K., Swarnalakshmi, K., and India Section, B.Biological Sciences (2017). High-value crops’ imbedded intensive cropping systems for enhanced productivity, resource-use-efficiency, energetics and soil-health in indo-gangetic plains. Proc. Natl. Acad. Sci. India Sect. B. Biol. Sci. 87 (4), 1073–1090. doi:10.1007/s40011-015-0679-6
Rashid, M. I., Mujawar, L. H., Shahzad, T., Almeelbi, T., Ismail, I. M. I., and Oves, M. (2016). Bacteria and fungi can contribute to nutrients bioavailability and aggregate formation in degraded soils. Microbiol. Res. 183, 26–41. doi:10.1016/j.micres.2015.11.007
Rashidzadeh, A., and Olad, A. (2014). Slow-released NPK fertilizer encapsulated by NaAlg-g-poly(AA-co-AAm)/MMT superabsorbent nanocomposite. Carbohydr. Polym. 114, 269–278. doi:10.1016/j.carbpol.2014.08.010
Shivay, Y. S., and Prasad, R. (2012). Zinc-coated urea improves productivity and quality of basmati rice (oryza sativa l.) under zinc stress condition. J. Plant Nutr. 35 (6), 928–951. doi:10.1080/01904167.2012.663444
Trinh, T. H., Shaari, K. Z. K., Basit, A., and Azeem, B. (2014). Effect of particle size and coating thickness on the release of urea using multi-diffusion model. Int. J. Chem. Eng. Appl. 5 (1), 58–63. doi:10.7763/ijcea.2014.v5.351
Vance, E. D., Brookes, P. C., and Jenkinson, D. S. (1987). An extraction method for measuring soil microbial biomass C. Soil Biol. Biochem. 19 (6), 703–707. doi:10.1016/0038-0717(87)90052-6
Yaseen, M., Ahmad, A., Naveed, M., Ali, M. A., Shah, S. S. H., Hasnain, M., et al. (2021). Subsurface-applied coated nitrogen fertilizer enhanced wheat production by improving nutrient-use efficiency with less ammonia volatilization. Agron. (Basel). 11 (12), 2396. doi:10.3390/agronomy11122396
Zhang, L., Liang, Z., Hu, Y., Schmidhalter, U., Zhang, W., Ruan, S., et al. (2021). Integrated assessment of agronomic, environmental and ecosystem economic benefits of blending use of controlled-release and common urea in wheat production. J. Clean. Prod. 287, 125572. doi:10.1016/j.jclepro.2020.125572
Keywords: coated urea fertilizers, nitrogen use efficiency, ryegrass, zinc, apparent zinc recovery, apparent nitrogen recovery
Citation: Abdullah B, Niazi MBK, Jahan Z, Khan O, Shahid A, Shah GA, Azeem B, Iqbal Z and Mahmood A (2022) Role of zinc-coated urea fertilizers in improving nitrogen use efficiency, soil nutritional status, and nutrient use efficiency of test crops. Front. Environ. Sci. 10:888865. doi: 10.3389/fenvs.2022.888865
Received: 03 March 2022; Accepted: 18 August 2022;
Published: 19 September 2022.
Edited by:
Dibyendu Chatterjee, National Rice Research Institute (ICAR), IndiaReviewed by:
Tapan Kumar Adhya, KIIT University, IndiaHayssam M. Ali, King Saud University, Saudi Arabia
Copyright © 2022 Abdullah, Niazi, Jahan, Khan, Shahid, Shah, Azeem, Iqbal and Mahmood. This is an open-access article distributed under the terms of the Creative Commons Attribution License (CC BY). The use, distribution or reproduction in other forums is permitted, provided the original author(s) and the copyright owner(s) are credited and that the original publication in this journal is cited, in accordance with accepted academic practice. No use, distribution or reproduction is permitted which does not comply with these terms.
*Correspondence: Muhammad Bilal Khan Niazi, m.b.k.niazi@scme.nust.edu.pk