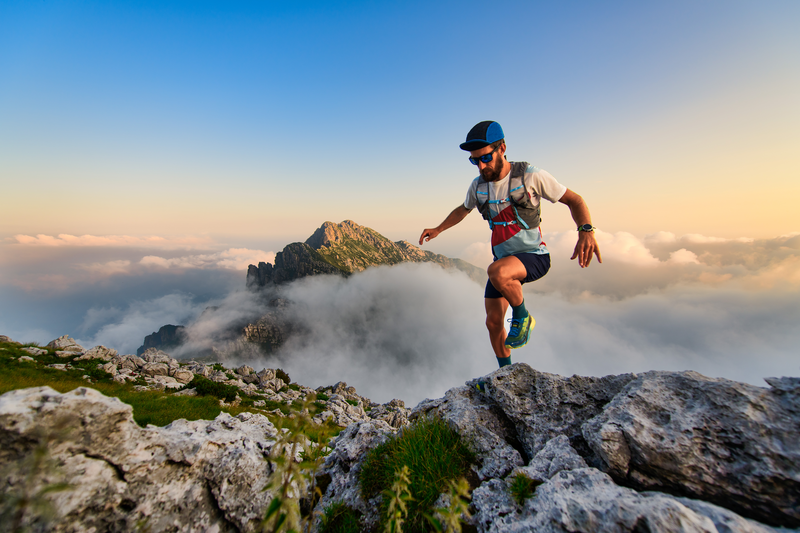
95% of researchers rate our articles as excellent or good
Learn more about the work of our research integrity team to safeguard the quality of each article we publish.
Find out more
ORIGINAL RESEARCH article
Front. Environ. Sci. , 01 June 2022
Sec. Soil Processes
Volume 10 - 2022 | https://doi.org/10.3389/fenvs.2022.886900
This article is part of the Research Topic Better Management of Phosphorus Fertilizer in Intensive Cropping Systems: An Approach Basing on Integrated Agronomic, Ecological and Environmental Compromises View all 11 articles
The use of organic materials has been increasing due to improving soil fertility by affecting phosphorus (P) adsorption and desorption behavior of soils. However, previous studies ignored the influences of increased P concentrations in equilibrium solutions caused by P released from applied organic materials in bath P adsorption experiments. To eliminate these influences, a modified Langmuir equation was applied to describe P adsorption properties in dairy manure composts and biosolids-incubated soils. The adsorption and desorption trends shifted around the initial P concentration of 70 mg/L. The fitted parameter of correlation coefficients showed that the modified Langmuir model fitted well for the adsorption data covering only the lower initial P concentrations (0–70 mg P/L) and covering the whole P concentrations applied in the present study (0–400 mg/L). For the fitted results covering the whole P concentrations, adding organic materials generally decreased both the Γmax (maximum adsorption capacity for P) and the MABC (maximum adsorption buffering capacity), except for the 20-g/kg biosolids incubation, indicating that the number of adsorption sites for P decreased as the organic materials applied, and the binding energy for the P adsorption to the soil also decreased to some extent with some fluctuation as reflected by K values which represent adsorption strength. Higher DOC content, lower molecular weight, and higher humic acid might result in the higher Γmax of 20-g/kg biosolids incubated soil. The linear equations are described well for P desorption. The constant a value of slope in a linear equation reflects the P desorption capacity of soils increased due to the application of organic materials, especially in high P concentrations with a value of 0.45 for the control soil increased to 1.02 for 10-g/kg biosolids treated soil. Thus, it would be important to control the P application amounts when the application of P fertilizers to the soils with organic materials applied, as the effects of organic materials on P adsorption and desorption characteristics were more efficient at high P concentrations.
Phosphorus (P) is the second most important macronutrient for plant growth and nutrition as a nonrenewable resource. Application of P fertilizers is vital for food production, especially for enhancing agricultural production in soils with low P availability, as all plants need an ample supply of P. When phosphate fertilizers are applied to the soils, their utilization efficiency for crops is only 10–25% and up to 75–90% of P would be accumulated in soils (Lu et al., 1995; Wang et al., 2011). Excessive phosphate fertilizers are annually applied to the soils and P input is often greater than its demand, and it will thus result in contamination of aquatic systems and underground water if P applied into soils is leached into surface water and groundwater (Heredia and Cirelli, 2007; Holman et al., 2010).
Adsorption and desorption of P in soils combined with mineralization and immobilization form the dynamic soil P cycling, and adsorption-desorption is an important internal cycle for determining the environmental fate of P and for understanding the soil fertility (Barros et al., 2005). Adsorption and desorption processes depend on many factors and soil conditions, such as soil types, soil texture and pH, contents of Al, Fe, soil organic matter, and clay minerals (Antelo et al., 2007; Weng et al., 2011; Wang and Liang, 2014; Fink et al., 2016; Kurnain, 2016). Soil organic matter plays an important role in P adsorption and desorption, as it could involve the competition between organic matter and P for mineral adsorption sites, removing adsorption sites by complexation of surface metals and release of these metals into solution, repulsion of phosphate anions by sorption of organic matter to positive sites, and formation of cation bridge leading to increase in P sorption sites (Hunt et al., 2007; Debicka et al., 2016).
The application of organic materials can change the content and constitute of soil organic matter, and thus will affect the adsorption and desorption characteristics of P in soil. Organic materials applied to acid sandy soils would commonly decrease P adsorption and increase P availability (Borggaard et al., 1990; Nziguheba et al., 1998; Hafiz et al., 2016). The application of manure in calcareous cinnamon soil also decreased the P adsorption and buffering capacity (Zhang et al., 2008). However, the exceptional results were also determined. Ma and Xu (2010) found that the application of rice straw decreased P adsorption to red soil and paddy soil, but there was nearly no effect on latosol soil, although these three soils all belong to the acid soils with low soil organic matter. Humic acids incubated in black soil enhanced the maximum adsorption capacity of P, soil available P, and P activation coefficient (Yang et al., 2019).
Furthermore, when calculating P sorption from solution to soils applied with organic materials, the release/desorbing of P from the organic materials have not been accounted for in these studies. Indeed, there is considerable evidence that increased P in soil solutions arises from the applied organic materials (Guppy et al., 2005). P equilibrium concentrations in batch adsorption experiments would be greater than expected due to the release of P, and finally, P adsorbed to soils would be underestimated. Therefore, the incubation of organic materials in soils has commonly been reported as reducing P sorption in soils. Whether the reported decreases in P sorption (as measured by the differences between P in the solutions before and after adsorption) are related to competition with organic matter (such as humic acid) decomposition products of organic materials breakdown or the results of P release from organic materials, need to be further determined.
For avoiding underestimating the effects of P release from organic materials applied into soils on P adsorption, the soil organic matter removal combated with batch adsorption experiments were applied to investigate the influence of organic matter on P adsorption and desorption. Hiradate and Uchida (2004) found that removing organic matter from the Andisol soil increased the P adsorbed amount, indicating that soils organic matter occupied sites that could adsorb P, and soil organic matter could inhibit P adsorption through competitive adsorption. But Debicka et al. (2016) found that the adsorption capacity for P in most of the topsoils that were tested decreased, and the desorption of P increased when organic matter was removed, indicating that soils organic matter was potentially P binding soil constituents and could adsorb P. These contradictory results may have been caused by the P adsorption capacity of soil depending on the type of organic matter present and the soil type. In addition, P adsorption experiments on matrix minerals (such as goethite, ferrihydrite, gibbsite, and kaolin) covered with organic matter (such as humic acid) were carried out to determine the effect of organic matter on P adsorption (Antelo et al., 2007; Hunt et al., 2007; Kurnain, 2016; Yan et al., 2016), and inhibition of P sorption to matrix minerals was commonly observed. Also, correlation analyses and path analyses were applied to find out the influences of organic matter on the adsorption and availability of P in soils (Kang et al., 2009; Yadav et al., 2017). But in these studies, the soil organic matter was not manipulated or controlled. To both control the soil organic matter (increasing soil organic matter only without changing the other soil components) and avoid the underestimating the effects of P release from organic materials applied to soils, Yang et al. (2019) applied humic acid-containing negligible P as the exogenous organic materials to identify the mechanism through which organic matter affects the adsorption of P in black soil, and found that P adsorption and availability were enhanced by increasing soil organic matter. Although humic acid was one of the most important decomposition products of organic materials breakdown applied to soils, it could not represent the organic materials applied to soils. So, the effects of organic materials applied to soils on the adsorption and availability of P in soils need to be further investigated by considering both the release of P from organic materials and the actual conditions of organic materials applied to soils.
Of all the obvious studies, the Langmuir equation has been used to describe P sorption reactions to soils. To account for the contributions of P releasing from organic materials-incubated soils, various Langmuir equation modifications have been developed (Villapando and Graetz, 2001; Brock et al., 2007; Zhang et al., 2010; Wang et al., 2014; Wang et al., 2016). The Langmuir equation is typically expressed as follows:
where ΓT (mg/kg) is the total amount of P adsorbed by the soil, which includes P that was already adsorbed to the soil before adsorption experiments (Γ0) and P adsorbed/desorbed from/into the equilibrating solution (Γs), C (mg/L) is the P concentration in the equilibrium solution, Γmax (mg/kg) is P adsorption maximum, and K (L/mg) represents P adsorption strength. When there is net P desorption into the equilibrating solutions, Γ0 represents the P that could be desorbed if the solution P concentration is maintained at or near zero (Barrow, 2008). For low-P soils, the amount of desorption/releasing is too small to be measured by standard techniques, and Γ0 is often ignored (Barrow, 2008; Zhang et al., 2010). But for P-enriched soils, Γ0 should be included in the Langmuir equation to describe P adsorption data because the amount of P releasing/desorbing should not be ignored. Based on comparisons of estimated adsorption parameters or goodness of fit for different Langmuir equation modifications, Wang et al. found that the estimate of Γ0 by fitting a linear equation to P adsorption data at low-P equilibrium concentration might be preferred (Wang et al., 2014). The Γ0 estimated in this way could cover the effect of P releasing from P-enriched soil and the effect of P releasing has been adjusted. The final fitting result is the result, that is, expected and P adsorbed to soil would not be underestimated or overestimated. So, in the present, a modified Langmuir equation was chosen and used to study the effects of organic materials applied in soils on the adsorption and availability of P in a Mollisol soil of northeast China (Wang et al., 2014; Wang et al., 2016). Dairy manure composts (MC) and biosolids (Bios) were chosen as the organic materials applied to the soil.
Furthermore, the previous research covers a different range of P concentrations applied to sorption experiments, and generally, it can be divided into two groups according to the upper initial P concentration limit, i.e., 10–60 mg P/L (McDowell and Condron, 2001; Guppy et al., 2005; Heredia and Cirelli, 2007; Wang et al., 2014; Debicka et al., 2016; Wang et al., 2016; Yang et al., 2019) with the Γmax of hundreds of in order of magnitude and 170–500 mg P/L (Bhatti et al., 1998; Hiradate and Uchida, 2004; Brock et al., 2007; Xue et al., 2014) with the Γmax of thousands of in order of magnitude. These also would affect the results of P sorption. To compare with the above results at a similar range of P concentrations, this study applied more P concentrations (up to 25 different concentrations of P) within a range of 0–400 mg P/L, with a smaller interval at lower initial P concentrations and with a larger interval at higher initial P concentrations. Then, the modified Langmuir equation has respectively been used to investigate P sorption behavior in the organic materials-amended soils at low P concentrations and high P concentrations.
A typical black soil (BS, a mollisol) was collected from an agricultural experimental base of Jilin University in Changchun City (latitude 43°56.603′-43°57.274′ N, 125°14.231′-125°14.914′ E). Surface soil samples (0–20 cm) were collected and then were air-dried, ground, homogenized, and sieved to pass through a < 2-mm sieve after removing stones and residual roots. Pre-decomposed dairy manure composts (MC) and biosolids (Bios) were chosen as exogenous organic materials. Organic materials were air-dried, ground to pass a < 2-mm sieve, and stored in a brown wide-mouth bottle prior to applying to the soils.
The soil of 3.0 kg was amended with 5, 10, and 20 g OM (organic matter)/kg soil from each of the organic material sources to increase the organic matter content and incubated for 30 days at 60% of the maximum field moisture capacity with adding deionized water (Hafiz et al., 2016; Yang et al., 2019). The soil without organic materials was also incubated for 30 days under the same condition and considered as the control. Three replicates have been applied for each treatment. Then the soils were air-dried, sieved to pass through a < 2-mm sieve, and stored in a brown bottle prior to use for adsorption and desorption experiments. Properties of soils and organic materials before and after incubation are listed in Table 1.
Solid samples were mixed with deionized water at the ratio of 1:1, shaken for 20 min, and then stood for 10 min to determine the pH and EC (Accumet AB 200, Fisher Scientific). The total organic carbon (TOC) of solids was measured by a Vario TOC cube (Elementar, German). The total P (TP) and available P contents were determined by an AutoAnalyzer 3 (Bran Luebbe Gmbh Company, German) after performing sulfuric acid—perchloric acid digestion and sodium bicarbonate extraction.
After incubation for 30 days, the release of P from soils by using 0.01 mol/L KCl at pH 7.0 was carried out (Guppy et al., 2005). Each soil sample (2.5 g) was placed into a 50-ml centrifuge tube and then was equilibrated with 25 ml of KCl (0.01 mol/L) at pH 7.0 for 24 h. After reaching equilibrium, the mixed suspensions were centrifuged at 4,000 r/min for 30 min followed by being filtered through 0.45-µM filters (Millipore, Billerica, MA, United States). P in supernatants was determined by an AutoAnalyzer 3 (Bran Luebbe Gmbh Company, German) and the release amounts of P were obtained (Table 1). Meanwhile, dissolved organic carbon (DOC) contents were also measured by a Vario TOC cube (Elementar, German), and the structural characteristics of DOC were determined by UV-Visible absorption spectroscopy (Kalbitz et al., 2000; Xie et al., 2018). UV/Vis spectra were recorded with a UV-2550 spectrophotometer (Shimadzu, Japan) in the wavelength range 200 ± 500 nm using 1 cm cuvettes. All spectra were normalized by the measured DOC contents and standardized at a concentration of 10 mg C/L by dilution.
Batch adsorption and desorption experiments were performed in this study. Each soil sample (1.0 g) was equilibrated with 10 ml of KCl (0.01 mol/L) at pH 7.0 supplied with P at different concentrations (0–400 mg/L) using KH2PO4. The mixed suspensions were added with three drops of chloroform to avoid microbial growth and horizontally shaken for 24 h, and then centrifuged at 4,000 r/min for 30 min followed by being filtered through 0.45-µM filters (Millipore, Billerica, MA, United States). P in the initial or equilibrium solutions were measured by an AutoAnalyzer 3 (Bran Luebbe Gmbh Company, German).
The solid samples were washed with deionized water for 30 min, centrifuged at 4,000 r/min for 30 min and the supernatants were discarded. Then the solid samples were shaken and re-equilibrated with 10 ml of KCl (0.01 mol/L) for 24 h. The suspensions were centrifuged at 4,000 r/min for 30 min followed by being filtered through 0.45-µM filters, and P in supernatants were determined by an AutoAnalyzer 3 (Bran Luebbe Gmbh Company, German) and the amounts of desorbed P were obtained.
A modified Langmuir model was used to analyze adsorption data in this study (Wang et al., 2014; Wang et al., 2016), and a sorption curve could be described as follows:
where C (mg/L) is the equilibrium P concentration of the solution, Γ (mg/kg) is the amount of P sorbed/desorbed from/into the solution during shaking, Γ0 (mg/kg) is the initial sorbed P that was already sorbed to the soil before analysis, Γmax (mg/kg) is the maximum amount of P, K (L/mg) is a constant related to the binding strength of P at the adsorption sites, and K × Γmax is the maximum adsorption buffering capacity (MABC, L/kg). The releasing experiment results indicated that P desorption reactions indeed occurred in the present study, especially for the soils incubated with more organic materials (Table 1).
If Γ = 0, C can be designated as Ce, which indicates the solution P concentration after shaking at zero netted sorption, and the following equation is obtained:
Combining Eq. 1, 2, the Langmuir equation could be modified as follows:
At low solution P concentrations, the relationship between Γ and C could be assumed to be linear (Wang et al., 2014; Wang et al., 2016):
where K1 (L/kg) is the partition coefficient. Accordingly, from Eq. 4, Ce can be calculated by the following equation:
In our study, the relatively lower initial P solutions (i.e., 0, 1, 3, 5 mg P/L) were used to determine the fitted values of the parameters of Eq. 4.
P desorption data were described by linear model D = a ×Γ + b, where D (mg/kg) is the amount of P desorbed from the soil after the adsorption experiment, Γ (mg/kg) is the amount of P adsorbed to the soil in the former P adsorption, slope a is the constant related to desorbing capacity.
The soil used in the present study is mollisol, a typical black soil with a TOC of 24.28 g/kg. The characteristics of organic materials used in this study were different from each other with a higher pH for MC and the lower pH for Bios (Table 1). On the contrary, the higher EC was found in Bios and the lower EC was present in MC. The organic matter contents of these two organic materials were similar to each other as described by TOC. Although the total P content of Bios was significantly higher than that of MC, the available P content of MC was close to that of Bios.
After adding the organic materials, the EC, TOC, TP, and available P of soil were all increased to some degree. The total P contents of the soils provided little information about the transformation of P in the soil and the availability of P to crops. The total P contents of the soils varied significantly with different organic materials being added, especially with the addition of bios and it increased the total P contents in the soils by 0.8–2.1 times. Also, available P contents were obviously increased due to the addition of Bios. The influences of MC on total P and available P in the soils were lower. The available P to total P ratio is called the P activation coefficient (PAC), which represents the degree of difficulty with which transformations between total P and available P. A higher coefficient indicates more P will be available to promote crops growth (Wu et al., 2017). The PAC increased after both MC and Bios were added to the soil.
Like the available P, organic materials applied in soils also enhanced the releasing/desorbing of P from the incubated soils (Table 1). The more organic materials added, the higher P released from the soils, and the highest P release was found from the soil incubated with 20-g/kg organic matter of Bios and almost 20 times more than that released from the control soil. So, it is important to account for the effects of P releasing/desorbing on the P adsorption experiments, and the modified Langmuir model rather than the original Langmuir model should be used in the present study.
Also dissolved organic carbon (DOC) released from the incubated soils increased with the application of the organic materials (Table 1). UV/Vis spectra of DOC extracted from the soils incubated with 20-g/kg OM from Bios show a higher absorbance over a wide range of wavelengths, with little change for 5- and 10 –g/kg OM incubated soils (Figure 1). A similar result was also obtained for the soils incubated with MC, but the absorbance change in the soil added with 20-g/kg OM from MC was smaller than that for the Bios added to the soil. MC and Bios application could increase UV spectra absorbance of DOM at 254, 260, and 280 nm, indicating that the fragrance, hydrophobicity, and molecular weight of DOM might increase to some extent (Xie et al., 2018) and the highest change was found in the 20-g/kg Bios-incubation soil. The UV spectra ratio of A250/A365 decreased for MC incubated soils as compared to control soil; it further proved that MC addition increased the molecular weight of organic matter in the soil. The ratio of A250/A365 also decreased for the soils incubated with 5- and 10 -g/kg OM from Bios (from 1.43 to 1.27 and 1.40), but increased to 1.48 for the soil incubated with 20-g/kg OM from Bios indicating that 20-g/kg Bios incubation decreased the molecular weight of soil organic matter. The ratio of A300/A400 were all below 3.5, which indicated that the soils with or without OM incubation were rich in humic acid, but humic acid in Bios was higher than that in MC (Xie et al., 2018).
FIGURE 1. UV spectra of dissolved organic matter extracted from incubated soils (absorbance was standardized to 10 mg C/L).
In the current study, we applied up to 25 initial P concentrations of adsorption experiments to cover both the lower and higher P concentrations. The more P added, the more P adsorbed to the soils. Supporting the general adsorption characteristics, the P adsorption increased rapidly as the initial P concentrations increased at lower P concentrations with the equilibrium P concentration increased to 40 mg/L, and then increased slowly to a lesser extent of higher P concentrations. When the equilibrium P concentration was lower than 40 mg/L, especially lower than 20 mg/L, the P adsorption amounts of the soils with MC and Bios were similar to the control soil which was also incubated under the same incubation condition but without organic materials added (Figure 2). The P adsorption by soils is regarded as being a multi-stage kinetic process (Wang and Liang, 2014), the present study results indicated that P adsorbed by soils went through an initial fast adsorption stage and then a slower adsorption stage. Previous studies have observed H-curve type isotherms (Azeez and Van Averbeke, 2011; Yu et al., 2013) or S-curve type isotherms (Hafiz et al., 2016) in P adsorption on soils incubated with organic materials. No matter H-curve type isotherms or S-curve type isotherms, the P adsorption trends both shifted at some specific P concentration. Yu et al. (2013) found that P adsorbed to soil parent materials with poultry manure compost and organic fertilizer application showed an H-shaped increasing trend, with P adsorption reaching a plateau when the initial P concentration was about 40–100 mg/L, but increased further beyond 100 mg/L. P adsorption to the dairy, poultry, and goat manure-incubated soils conformed to the S-curvature, with the lower P adsorption at low equilibrium concentration and become easier as the equilibrium concentration was rising to 50–100 mg/L (Hafiz et al., 2016). A careful observation of the adsorption isotherms in the present study indicated that the isotherms have more in common with the H-curve type isotherms, with P adsorption reaching a plateau when the equilibrium P concentration was 50–120 mg/L, and then increased further beyond 120 mg/L. High affinity with P for soils caused a high initial slope of the isotherms and likely appeared as the H-shaped isotherms. On the contrary, the low initial slopes of the isotherm curves were caused by the low affinity with P for soils and appeared as S-shaped curves (Hafiz et al., 2016). P adsorption isotherms forms depended on adsorption mechanisms and the P adsorption process could be divided into chemical and physical adsorption processes. When the initial P concentrations were relatively low, the chemical process could dominate the adsorption processes and would be completed rapidly, and ligand exchange and ion exchange were likely to be the dominant mechanisms contributing to the high adsorption rates (Lai and Lam, 2009). Correspondingly, the chemical adsorption process would slow down rapidly at high P concentrations due to the quickly being saturated of the available adsorption sites, and the P adsorption changed to the physicochemical and physical adsorption processes and presented slower rates (Lai and Lam, 2009; Wang and Liang, 2014).
FIGURE 2. Langmuir isotherms of P adsorbed onto soils incubated with different contents of organic matter derived from (A) (B) manure compost and (C) (D) biosolids.
Many models have been developed to describe adsorption isotherms and the Langmuir equation is one of the most used models to quantitatively fit P adsorption isotherms (Hussain et al., 2003; Lair et al., 2009; Rossi et al., 2012; Yang et al., 2019). The modified Langmuir model which is suitable for the P-enriched soils was applied to fit the adsorption data into this study (Wang et al., 2014; Wang et al., 2016). The adsorption data covering only the lower initial P concentrations (i.e., 0-70 mg P/L, Figures 2B,D) and the data covering the whole P concentrations applied in the present study (i.e., 0-400 mg/L, Figures 2A,C) were respectively fitted by the modified Langmuir model. Simulated Langmuir curves for the soils with or without organic materials incubation are illustrated in Figure 2 and the resulting fitted parameters are presented in Table 2. Results revealed that the modified Langmuir equation properly described the P adsorption properties of the soils, and the relationships indicated by the correlation coefficients were statistically significant.
The maximum adsorption capacity for P (Γmax) reflects the number of P adsorption sites per unit weight of the soil and is generally used to evaluate the adsorption capacity of soil for P (Hiradate and Uchida. 2004; Yan et al., 2016; Fink et al., 2016; Yang et al., 2019). Γmax obtained from the fitted curves covering only the lower initial P concentrations between 0–70 mg P/L were similar to each other for different organic materials-incubated soils at different levels, and all higher than that of the control soil with the increased rate of 14.6–29.9%, and the highest value was obtained in the soil with 20-g/kg bios incubation. Γmax changed slightly with MC added rates increased, but with Bios added rates increased, Γmax also increased significantly (Table 2). However, when we used the modified Langmuir model to fit the whole initial P concentrations, the higher Γmax values have been obtained, whether for the soils incubated with organic materials or not. Γmax for the control soil was 1,326.53 mg/kg and organic materials application clearly influenced the Γmax. The Γmax decreased as the spiked MC increased, indicating that the P storage capacity of the soil decreased as the MC incubation amounts increased. The Γmax also reduced along with the Bios addition when the Bios application was at lower rates of 5 g/kg and 10 g/kg, but when the application rate was up to 20 g/kg, the Γmax instead increased. Hafiz et al. (2016) found that the Γmax values of a silt loam agricultural soil also decreased after incubation with dairy, goat, and poultry manures for 1 month. Yu et al. (2013) found that poultry manure incubation reduced the Γmax of the soils derived from basalt, granite, and river alluvial deposits, no matter whether incubated for 30 days or 60 days; but organic fertilizer incubation increased the Γmax of the soils derived from granite and river alluvial deposits, although it also reduced the Γmax of the soils derived from basalt. The reduction in the Γmax due to the addition of MC and Bios in lower rates in the current study might be due to the affinity of organic ligands for sorption sites. Most ligands are capable of displacing phosphate ions in site-specific organic ligands such as oxalate, malate, citrate, tartrate, malonate, humic acid, and fulvic acid (Violante and Gianfreda, 1993; Bhatti et al., 1998; Guppy et al., 2005). Sharma et al. (2006) found that the possible blockade of P-fixing sites in the soil by soluble humic substances present in manure might reduce the adsorption capacity of the soil. The reduction in P adsorption capacity and consequently increase in P availability to plants or increase in P loss to water has commonly ascribed to competition between the decomposition products of organic matter and P for soil sorption sites, and the evidence for competition inhibition of P sorption by dissolved organic carbon compounds, derived from the breakdown of organic matter, includes the competition between P and low molecular weight organic acids, humic and fulvic acids, and organic matter leachates in soils with a high P sorption capacity (Guppy et al., 2005; Bayon et al., 2006; Zamuner et al., 2008). If the organic ligands decomposed from the incubated organic materials were previously adsorbed, the surface charge of the solids would be altered, and then might cause P to be electrostatically repelled and decrease P adsorption (Antelo et al., 2007). Meanwhile, Guppy et al. (2005) reported that decreases in P sorption as measured by P in the soil solution might also be the result of P release from the organic materials that were not considered when calculating the reduction in P sorption. In the present study, when calculating the P adsorption amounts, the P release from organic materials was accounted for by using the modified Langmuir model and MC incubation still reduced the P adsorption capacity of the soil. So, it could be assumed that the competition mechanism played a leading role in P adsorption processes in the current MC applied soils. The pH value changes in the incubated soils might also reflect the shifts in P adsorption capacity. Zhao et al. (2006) found that pH 6.0 was a turning point and increasing the organic matter content increased the P adsorption by soil at pH > 6.0, but decreased the amount of P adsorbed by soil at pH < 6.0. However, Guppy et al. (2005) considered that the magnitude of the inhibition of P sorption by the decomposition products of organic materials leachate is negligible at rates equivalent to those of organic materials applied field. Organic materials might also increase the P adsorption capacity through metal-chelate linkages provided by these organic compounds. The suddenly significant increase in the Γmax of the soil with 20 g/kg of Bios incubation resulted in part from the above reason. Besides, Bios was commonly characterized by containing more humic acid-like and fulvic acid-like compounds (Torrecillas et al., 2013; Campuzano and González-Martínez, 2016). Humic acid and fulvic acid do not simply compete with P for sorption sites in soils, but in many instances, act as P adsorbing surfaces also (Appelt et al., 1975; Perrott, 1978). In the present study, the DOM content and structure of the soil incubated with 20 g/kg of Bios were different from the other incubated soils, with higher DOC content, lower molecular weight, and higher humic acid (Figure 1), and this might result in higher Γmax. In some allophonic soils, reactions to metals with humic acid and fulvic acid greatly increase P sorption and are critical to P cycling processes (Borie and Zunino, 1983). In highly weathered soils, sorption of P to humic acid and fulvic acid might help alleviate problems associated with high P fixation through the slow mineralization of these complexes (Pushparajah et al., 1998). Yang et al. (2019) reported that the addition of humic acid to increase the soil organic matter content could efficiently increase the P adsorption capacity Γmax. This is possibly another reason to explain the increase of Γmax for the soil incubated with 20 g/kg of Bios.
The P adsorption energy (K) is another one of the most important parameters describing the P adsorption affinity with soils and related to binding energy to sorption sites. The higher value of K means a stronger trend of P adsorption, and the degree of spontaneous reaction is stronger, with weaker P supplying intensity (Wang and Liang, 2014; Hafiz et al., 2016). K obtained from the fitted curves of the lower initial P concentrations of 0–70 mg P/L were all significantly higher than those obtained from the fitted curves of the whole initial P concentrations. At lower initial P concentrations, organic materials incubation reduced K values as compared with control soil. But the K values changed significantly, and different shifting trends were found for the soils with different organic materials applied at whole initial P concentrations. With the OM incubation rates increasing, MC treatment first decreased K and then increased K, but Bios applied first enhanced K and finally reduced K, as compared with the control soil. These two organic materials had one thing in common was that incubation at the rate of 10-g/kg all increased K up to the highest. Without considering control soil, the K values approximately followed parabolic trends, which were consistent with the results previously reported by Xia and Wang (2009), first increasing, and then decreasing when the OM contents of the lime concretion black soil and the calcareous yellow fluvo-aquic soil increased. Hafiz et al. (2016) reported that manure treatments decreased K for dairy and goat manure incubated soil and increased for poultry manure incubated soil. K decreased by the lower MC incubation indicating that organic matter decomposed from MC competed with P for adsorption sites and decreased the amount of P adsorbed from the soil solution. When the soil was applied with higher OM, higher K values were obtained and this might be due to the high reactivity of the organic matter with phosphate anions (Yang et al., 2019). Then K decreased with OM further increasing might be possible as a result of P adsorption with little energy, such as cation bridges provided by organic materials (Kreller et al., 2003).
MABC is an integrated parameter of the Langmuir model Γmax and K. This is a useful index if evaluating the P supply and immobilization capacity and rates by soils (Holford and Patrick, 1979 ; Hafiz et al., 2016). The higher MABC means a higher P adsorption amount caused by changed unit equilibrium concentration when equilibrium liquid concentration tends to be very low (close to zero) (Wang and Liang, 2014). Organic materials treatments reduced the MABC of the soils for P. The similar results were also obtained by Hafiz et al. (2016), and this suggested that smaller P application rates would be better for maintaining a desired P concentration in the soil solution. Without considering control soil, the changing trend of MABC values was similar to that of K values and also likely followed parabolic trends, just like the results on the lime concretion black soil and the calcareous yellow fluvoaquic soil previously obtained by Xia and Wang (2009).
The Γmax and MABC values of the black soil obtained at the whole initial P concentrations decreased with the application of the organic materials, except the Γmax of the soil incubated with 20-g/kg OM from Bios, indicating that the number of adsorption sites for P decreased as the organic materials applied, and the binding energy for the P adsorption to the soil also decreased to some extent with some fluctuation as reflected by K values.
P desorption from soils is considered the reverse process of the adsorption process. It is more important than adsorption for reusing immobilized P in soils and possibly inducing the environmental problems caused by the release of P from soils (Wang and Liang, 2014). The amounts of P desorbed from the soils were less than the amounts of P adsorbed previously, indicating that the P adsorbed in soils was not fully reversible, although P that adsorbed to the soils could desorb to some extent and re-release to the soil solution (Figure 3). P desorption amounts from the soils tended to increase with increasing P adsorption amounts, and the desorption rates also approximately tended to increase and the obvious turning point appeared at the adsorption amounts of 350–450 mg/kg. When the adsorption amounts were lower than 400 mg/kg, the desorption rates were relatively low, and then increased higher. Considering the shift of P desorption rates, the desorption data before and after the turning points were described separately by the linear equation as shown in Figure 3, and the parameters for the linear equation were listed in Table 3.
FIGURE 3. P desorption from soils incubated with different contents of organic matter derived from (A) manure compost and (B) biosolids.
TABLE 3. Parameters of the linear model for P desorbed from soils with and without organic matter incubation.
The constant a of slope in the linear equation reflects the P desorption capacity from soils, and the higher a value, the stronger the P desorption ability. For the control soil, the a value is 0.22 when the adsorption amounts were low (corresponding to the low P concentration in the solution in the previous adsorption experiment), and increased to 0.45 with the P adsorption amounts increasing. P desorbed from the soils incubated with organic materials also presented similar trends with lower a values (0.23—0.39) in low P concentrations and higher a values (0.49–1.02) in high P concentrations. This indicated that P adsorption sites in soils were abundant when the P concentrations were relatively low and that the P-soil chemical adsorption binding capacity was high, resulting in a high degree of adsorption and a low degree of desorption. With the P concentrations of the solution increasing, the adsorption sites in soils gradually became saturated and finally decreased the P-soil adsorption binding capacity. The physical adsorption mechanism might be responsible for this adsorption stage and thus P bound by physical adsorption could readily be desorbed and re-released (Wang and Liang, 2014; Yang et al., 2019). So, it is important to control the amounts of P applied to soil, as a less level of P application will supply a less P concentration in the soil solution and more P will be absorbed by soils, and thus P fertilizer will not play its due role for plants; a higher P application will increase the amounts of P desorbed, then P concentration of the soil solution will increase and P fertilizer utilization will be improved. However, from the perspective of environmental risk, higher P concentration in the soil solution caused by higher P application to the soil will enhance the risk of P releasing and losing from soil to the surrounding environment, such as surface waters or underground waters.
The a values of the soils with organic materials incubation were generally greater than that of the control soil both in the low P concentrations and in the high P concentrations. In low P concentrations, a value increased with the Bios incubation rate increasing, and in high P concentrations, a value increased with MC added rate increasing. Also, it could be found that organic materials incubation promoted the desorption turning point ahead. The results suggested that incubation of organic materials can enhance the P desorption of the chemical adsorption phase in low P concentration to a lesser extent, and significantly promote the P desorption of the slow physical adsorption phase in high P concentration. This process could affect the activity and bioavailability of soil P, and then increase the amounts of bioavailable P; also, the risk of P loss may increase, especially in high P concentrations.
The effects of dairy manure compost and biosolids on adsorption and desorption of P were studied in a typical black soil (a mollisol) from Northeast China by using the batch equilibrium experiments. After being incubated by organic materials, P releasing/desorbing amounts of the soil significantly increased and should be accounted for when estimating P adsorption amounts. The modified Langmuir equation which has been proved to effectively account for the released P effect for P-enriched soil was chosen to fit P adsorption and the fitted results proved this modified model sufficiently to describe P adsorption. Except for the 20-g/kg biosolids incubation, adding organic materials generally decreased both the maximum adsorption capacity for P and the maximum adsorption buffering capacity at the whole P concentrations, indicating that the number of adsorption sites for P decreased as the organic materials were applied, and the binding energy for the P adsorption to the soil also decreased to some extent with some fluctuation as reflected by K values. The higher adsorption of 20-g/kg biosolids incubated soil might be due to the higher DOC content, lower molecular weight, and higher humic acid of 20-g/kg biosolids spiked soil. The linear equations described well for P desorption and organic materials incubation enhanced P desorbed from the soils. The constant a value of slope in a linear equation reflects the P desorption capacity from soils increased due to the application of organic materials, especially in high P concentrations with a value of 0.45 for the control soil increased to 1.02 for 10-g/kg biosolids treated soil.
The original contributions presented in the study are included in the article/supplementary material, further inquiries can be directed to the corresponding authors.
ZW conceived and designed the experiments, analyzed the data, wrote the original draft, and discussed the results. LH further analyzed the data, verified the analytical methods, and edited the manuscript. ZL reviewed and edited the manuscript. NC supervised the work. XW supervised the work and reviewed and edited the manuscript.
This work was supported by the State Key Research and Development Plan of China (2017YFD0200202-5).
The authors declare that the research was conducted in the absence of any commercial or financial relationships that could be construed as a potential conflict of interest.
All claims expressed in this article are solely those of the authors and do not necessarily represent those of their affiliated organizations, or those of the publisher, the editors and the reviewers. Any product that may be evaluated in this article, or claim that may be made by its manufacturer, is not guaranteed or endorsed by the publisher.
Antelo, J., Arce, F., Avena, M., Fiol, S., López, R., and Macías, F. (2007). Adsorption of a Soil Humic Acid at the Surface of Goethite and its Competitive Interaction with Phosphate. Geoderma 138, 12–19. doi:10.1016/j.geoderma.2006.10.011
Appelt, H., Coleman, N. T., and Pratt, P. F. (1975). Interactions between Organic Compounds, Minerals, and Ions in Volcanic-Ash-Derived Soils: II. Effects of Organic Compounds on the Adsorption of Phosphate. Soil Sci. Soc. Am. J. 39, 628–630. doi:10.2136/sssaj1975.03615995003900040018x
Azeez, J. O., and Van Averbeke, W. (2011). Effect of Manure Types and Period of Incubation on Phosphorus-Sorption Indices of a Weathered Tropical Soil. Commun. Soil Sci. Plant Analysis 42, 2200–2218. doi:10.1080/00103624.2011.602452
Barros, N. F., Comerford, F. N. B., and Barros, N. F. (2005). Phosphorus Sorption, Desorption and Resorption by Soils of the Brazilian Cerrado Supporting Eucalypt. Biomass Bioenergy 28, 229–236. doi:10.1016/j.biombioe.2004.08.005
Barrow, N. J. (2008). The Description of Sorption Curves. Eur. J. Soil Sci. 59, 900–910. doi:10.1111/j.1365-2389.2008.01041
Bayon, R. C. L., Weisskopf, L., Martinoia, E., Jansa, J., Frossard, E., Keller, F., et al. (2006). Soil Phosphorus Uptake by Continuously Cropped Lupinus Albus: A New Microcosm Design. Plant Soil 283, 309–321. doi:10.1007/s11104-006-0021-4
Bhatti, J. S., Comerford, N. B., and Johnston, C. T. (1998). Influence of Oxalate and Soil Organic Matter on Sorption and Desorption of Phosphate onto a Spodic Horizon. Soil Sci. Soc. Am. J. 62, 1089–1095. doi:10.2136/sssaj1998.03615995006200040033x
Borggaard, O. K., Jdrgensen, S. S., Moberg, J. P., and Raben-Lange, B. (1990). Influence of Organic Matter on Phosphate Adsorption by Aluminium and Iron Oxides in Sandy Soils. Eur. J. Soil Sci. 41, 443–449. doi:10.1111/j.1365-2389.1990.tb00078.x
Borie, F., and Zunino, H. (1983). Organic Matter-Phosphorus Associations as a Sink in P-Fixation Processes in Allophanic Soils of Chile. Soil Biol. Biochem. 15, 599–603. doi:10.1016/0038-0717(83)90056-1
Brock, E. H., Ketterings, Q. M., and Kleinman, P. J. A. (2007). Measuring and Predicting the Phosphorus Sorption Capacity of Manure-Amended Soils. Soil Sci. 172, 266–278. doi:10.1097/ss.0b013e318032ab2e
Campuzano, R., and González-Martínez, S. (2016). Characteristics of the Organic Fraction of Municipal Solid Waste and Methane Production: A Review. Waste Manag. 54, 3–12. doi:10.1016/j.wasman.2016.05.016
Debicka, M., Kocowicz, A., Weber, J., and Jamroz, E. (2016). Organic Matter Effects on Phosphorus Sorption in Sandy Soils. Archives Agron. Soil Sci. 62, 840–855. doi:10.1080/03650340.2015.1083981
Fink, J. R., Inda, A. V., Bavaresco, J., Barrón, V., Torrent, J., and Bayer, C. (2016). Adsorption and Desorption of Phosphorus in Subtropical Soils as Affected by Management System and Mineralogy. Soil Tillage Res. 155, 62–68. doi:10.1016/j.still.2015.07.017
Guppy, C. N., Menzies, N. W., Moody, P. W., and Blamey, F. P. C. (2005). Competitive Sorption Reactions between Phosphorus and Organic Matter in Soil: a Review. Soil Res. 43, 189–202. doi:10.1071/SR04049
Hafiz, N., Adity, S. M., Mitu, S. F., and Rahman, A. (2016). Effect of Manure Types on Phosphorus Sorption Characteristics of an Agricultural Soil in Bangladesh. Cogent Food & Agric. 2, 1270160. doi:10.1080/23311932.2016.1270160
Heredia, O. S., and Fernández Cirelli, A. (2007). Environmental Risks of Increasing Phosphorus Addition in Relation to Soil Sorption Capacity. Geoderma 137, 426–431. doi:10.1016/j.geoderma.2006.09.005
Hiradate, S., and Uchida, N. (2004). Effects of Soil Organic Matter on pH-dependent Phosphate Sorption by Soils. Soil Sci. Plant Nutr. 50, 665–675. doi:10.1080/00380768.2004.10408523
Holford, I. C. R., and Patrick, W. H. (1979). Effects of Reduction and pH Changes on Phosphate Sorption and Mobility in an Acid Soil. Soil Sci. Soc. Am. J. 43, 292–297. doi:10.2136/sssaj1979.03615995004300020010x
Holman, I. P., Howden, N. J. K., Bellamy, P., Willby, N., Whelan, M. J., and Rivas-Casado, M. (2010). An Assessment of the Risk to Surface Water Ecosystems of Groundwater P in the UK and Ireland. Sci. Total Environ. 408, 1847–1857. doi:10.1016/j.scitotenv.2009.11.026
Hunt, J. F., Ohno, T., He, Z., Honeycutt, C. W., and Dail, D. B. (2007). Inhibition of Phosphorus Sorption to Goethite, Gibbsite, and Kaolin by Fresh and Decomposed Organic Matter. Biol. Fertil. Soils 44, 277–288. doi:10.1007/s00374-007-0202-1
Hussain, A., Ghafoor, A., Anwar-Ul-Haq, M., and Nawaz, M. (2003). Application of the Langmuir and Freundlich Equations for P Adsorption Phenomenon in Saline-Sodic Soils. Int. J. Agric. Biol. 5, 349–356.
Kalbitz, k., Solinger, S., Park, J. H., Michalzik, B., and Matzner, E. (2000). Controls on the Dynamics of Dissolved Organic Matter in Soils: A review. Soil Sci. 165, 277–304. doi:10.1097/00010694-200004000-00001
Kang, J., Hesterberg, D., and Osmond, D. L. (2009). Soil Organic Matter Effects on Phosphorus Sorption: A Path Analysis. Soil Sci. Soc. Am. J. 73, 360–366. doi:10.2136/sssaj2008.0113
Kreller, D. I., Gibson, G., Novak, W., Van Loon, G. W., and Horton, J. H. (2003). Competitive Adsorption of Phosphate and Carboxylate with Natural Organic Matter on Hydrous Iron Oxides as Investigated by Chemical Force Microscopy. Colloids Surfaces A Physicochem. Eng. Aspects 212, 249–264. doi:10.1016/S0927-7757(02)00325-4
Kurnain, A. (2016). Inhibition of Phosphorus Adsorption to Goethite and Acid Soil by Organic Matter. Int. J. Soil Sci. 11, 87–93. doi:10.3923/ijss.2016.87.93
Lai, D. Y. F., and Lam, K. C. (2009). Phosphorus Sorption by Sediments in a Subtropical Constructed Wetland Receiving Stormwater Runoff. Ecol. Eng. 35, 735–743. doi:10.1016/j.ecoleng.2008.11.009
Lair, G. J., Zehetner, F., Khan, Z. H., and Gerzabek, M. H. (2009). Phosphorus Sorption-Desorption in Alluvial Soils of a Young Weathering Sequence at the Danube River. Geoderma 149, 39–44. doi:10.1016/j.geoderma.2008.11.011
Lu, R., Shi, Z., and Gu, Y. (1995). Study on Accumulated Soil Phosphorus II. Apparent Accumulation and Utilization Rate of Phosphate Fertilizers. Soils 27, 286–289. doi:10.13758/j.cnki.tr.1995.06.002
Ma, L., and Xu, R. (2010). Effects of Regulation of pH and Application of Organic Material on Adsorption and Desorption of Phosphorus in Three Types of Acid Soils. J. Ecol. Rural Environ. 26, 596–599. doi:10.1016/j.still.2018.11.016
McDowell, R., and Condron, L (2001). Influence of Soil Constituents on Soil Phosphorus Sorption and Desorption. Commun. Soil Sci. Plant Anal. 32, 2531–2547. doi:10.1081/CSS-120000389
Nziguheba, G., Palm, C. A., Buresh, R. J., and Smithson, P. C. (1998). Soil Phosphorus Fractions and Adsorption as Affected by Organic and Inorganic Sources. Plant Soil 198, 159–168. doi:10.1023/A:1004389704235
Perrott, K. (1978). The Influence of Organic Matter Extracted from Humified Clover on the Properties of Amorphous aluminosilicates.II. Phosphate Retention. Soil Res. 16, 341–346. doi:10.1071/SR9780341
Pushparajah, E., Sehgal, J., Blum, W. E., and Gajbhiye, K. S. (1998). Nutrient Management and Challenges in Managing Red and Lateritic Soils. Red Later. Soils, 293–304.
Rossi, C. G., Heil, D. M., Bonumà, N. B., and Williams, J. R. (2012). Evaluation of the Langmuir Model in the Soil and Water Assessment Tool for a High Soil Phosphorus Condition. Environ. Model. Softw. 38, 40–49. doi:10.1016/j.envsoft.2012.04.018
Sharma, R. K., Agrawal, M., and Marshall, F. (2006). Heavy Metal Contamination in Vegetables Grown in Wastewater Irrigated Areas of Varanasi, India. Bull. Environ. Contam. Toxicol. 77, 312–318. doi:10.1007/s00128-006-1065-0
Torrecillas, C., Martínez-Sabater, E., Gálvez-Sola, L., Agulló, E., Pérez-Espinosa, A., Morales, J., et al. (2013). Study of the Organic Fraction in Biosolids. Commun. Soil Sci. Plant Analysis 44, 492–501. doi:10.1080/00103624.2013.744150
Villapando, R. R., and Graetz, D. A. (2001). Phosphorus Sorption and Desorption Properties of the Spodic Horizon from Selected Florida Spodosols. Soil Sci. Soc. Am. J. 65, 331–339. doi:10.2136/sssaj2001.652331x
Violante, A., and Gianfreda, L. (1993). Competition in Adsorption between Phosphate and Oxalate on an Aluminum Hydroxide Montmorillonite Complex. Soil Sci. Soc. Am. J. 57, 1235–1241. doi:10.2136/sssaj1993.03615995005700050013x
Wang, L., and Liang, T. (2014). Effects of Exogenous Rare Earth Elements on Phosphorus Adsorption and Desorption in Different Types of Soils. Chemosphere 103, 148–155. doi:10.1016/j.chemosphere.2013.11.050
Wang, L., Liang, T., Kleinman, P. J. A., and Cao, H. (2011). An Experimental Study on Using Rare Earth Elements to Trace Phosphorous Losses from Nonpoint Sources. Chemosphere 85, 1075–1079. doi:10.1016/j.chemosphere.2011.07.038
Wang, Y. T., O’Halloran, I., Zhang, T. Q., Hu, Q. C., and Tan, C. S. (2014). Langmuir Equation Modifications to Describe Phosphorus Sorption in Soils of Ontario, Canada. Soil Sci. 179, 536–546. doi:10.1097/SS.0000000000000100
Wang, Y. T., Zhang, T. Q., O'Halloran, I. P., Tan, C. S., and Hu, Q. C. (2016). A Phosphorus Sorption Index and its Use to Estimate Leaching of Dissolved Phosphorus from Agricultural Soils in Ontario. Geoderma 274, 79–87. doi:10.1016/j.geoderma.2016.04.002
Weng, L., Vega, F. A., and Van Riemsdijk, W. H. (2011). Competitive and Synergistic Effects in pH Dependent Phosphate Adsorption in Soils: LCD Modeling. Environ. Sci. Technol. 45, 8420–8428. doi:10.1021/es201844d
Wu, Q., Zhang, S., Zhu, P., Huang, S., Wang, B., Zhao, L., et al. (2017). Characterizing Differences in the Phosphorus Activation Coefficient of Three Typical Cropland Soils and the Influencing Factors under Long-term Fertilization. PloS One 12, e0176437. doi:10.1371/journal.pone.0176437
Xia, H. Y., and Wang, K. R. (2009). Effects of Soil Organic Matter on Characteristics of Phosphorus Adsorption and Desorption in Calcareous Yellow Fluvo-aquic Soil and Lime Concretion Black Soil. Plant Nutr. Fert. Sci. 15, 1303–1310. doi:10.3321/j.issn:1008-505X.2009.06.009
Xie, J., Zhao, Y. N., Chen, X. J., Wang, K., Xu, C. L., Li, D. P., et al. (2018). Effect on Soil DOM Content and Structure Characteristics in Different Soil Layers by Long-Term Fertilizations. Spectrosc. Spectr. Analysis 38, 2250–2255. doi:10.3964/j.issn.1000-0593(2018)07-2250-06
Xue, Q., Lu, L., Zhou, Y., Qi, L., Dai, P., Liu, X., et al. (2014). Deriving Sorption Indices for the Prediction of Potential Phosphorus Loss from Calcareous Soils. Environ. Sci. Pollut. Res. 21, 1564–1571. doi:10.1007/s11356-013-2045-7
Yadav, L. K., Bhagat, R. K., and Jatav, G. K. (2017). A Study on Relationship between Soil and P Adsorption Parameters. Int. J. Curr. Microbiol. App. Sci. 6, 2822–2826. doi:10.20546/ijcmas.2017.608.336
Yan, J., Jiang, T., Yao, Y., Lu, S., Wang, Q., and Wei, S. (2016). Preliminary Investigation of Phosphorus Adsorption onto Two Types of Iron Oxide-Organic Matter Complexes. J. Environ. Sci. 42, 152–162. doi:10.1016/j.jes.2015.08.008
Yang, X., Chen, X., and Yang, X. (2019). Effect of Organic Matter on Phosphorus Adsorption and Desorption in a Black Soil from Northeast China. Soil Tillage Res. 187, 85–91. doi:10.1016/j.still.2018.11.016
Yu, W., Ding, X., Xue, S., Li, S., Liao, X., and Wang, R. (2013). Effects of Organic-Matter Application on Phosphorus Adsorption of Three Soil Parent Materials. J. Soil Sci. Plant Nutr. 13, 1003–1017. doi:10.4067/S0718-95162013005000079
Zamuner, E. C., Picone, L. I., and Echeverria, H. E. (2008). Organic and Inorganic Phosphorus in Mollisol Soil under Different Tillage Practices. Soil Tillage Res. 99, 131–138. doi:10.1016/j.still.2007.12.006
Zhang, H., Liu, J., Liao, W., Zhang, Z., and Hao, X. (2008). Effect of Phosphate Fertilizer and Manure on Properties of Phosphorus Sorption and Desorption in Soils with Different Phosphorus Levels. Plant Nutr. Fert. Sci. 14, 284–290.
Zhang, W., Faulkner, J. W., Giri, S. K., Geohring, L. D., and Steenhuis, T. S. (2010). Effect of Soil Reduction on Phosphorus Sorption of an Organic‐Rich Silt Loam. Soil Sci. Soc. Am. J. 74, 240–249. doi:10.2136/sssaj2009.0123
Zhao, X., Zhong, X., Li, G., Bao, H., Li, H., Xiong, G., et al. (2006). The Evaluation of Phosphorus Leaching Risk of 23 Chinese Soils II. The Relationships between Soils Properties, P Adsorption Characteristics and the Leaching Criterion. Acta Ecol. Sin. 26, 3011–3017. doi:10.1016/S1872-2032(06)60005-X
Keywords: black soil, organic materials, modified Langmuir equation, phosphorus adsorption, phosphorus desorption, UV spectroscopy
Citation: Wang Z, Hou L, Liu Z, Cao N and Wang X (2022) Using a Modified Langmuir Equation to Estimate the Influence of Organic Materials on Phosphorus Adsorption in a Mollisol From Northeast, China. Front. Environ. Sci. 10:886900. doi: 10.3389/fenvs.2022.886900
Received: 01 March 2022; Accepted: 19 April 2022;
Published: 01 June 2022.
Edited by:
Haigang Li, Inner Mongolia Agricultural University, ChinaReviewed by:
Chaochun Zhang, China Agricultural University, ChinaCopyright © 2022 Wang, Hou, Liu, Cao and Wang. This is an open-access article distributed under the terms of the Creative Commons Attribution License (CC BY). The use, distribution or reproduction in other forums is permitted, provided the original author(s) and the copyright owner(s) are credited and that the original publication in this journal is cited, in accordance with accepted academic practice. No use, distribution or reproduction is permitted which does not comply with these terms.
*Correspondence: Ning Cao, Y2FvX25pbmdAamx1LmVkdS5jbg==; Xiaoli Wang, d2FuZ194bEBqbHUuZWR1LmNu
†These authors have contributed equally to this work and share first authorship
Disclaimer: All claims expressed in this article are solely those of the authors and do not necessarily represent those of their affiliated organizations, or those of the publisher, the editors and the reviewers. Any product that may be evaluated in this article or claim that may be made by its manufacturer is not guaranteed or endorsed by the publisher.
Research integrity at Frontiers
Learn more about the work of our research integrity team to safeguard the quality of each article we publish.