- College of Economics & Management, Northwest A&F University, Yangling, China
Based on 432 sets of data from three resource-based provinces in China, Anhui, Shanxi, and Sichuan, a fixed-effects model was established using panel data for empirical analysis to investigate the relationship between industrial structure, environmental pressure, and ecological resilience of resource-based cities, using entropy value method and principal component analysis to measure the industrial structure, environmental pressure and ecological resilience of resource-based cities. It is found that for resource-based cities, the economic growth mainly relies on the development of the secondary industry, which is primarily dependent on local natural resources. The empirical results show that the regional industrial structure has a significant negative impact on the cities’ ecological resilience, and the regional industrial structure has a significant positive effect on their environmental pressure. The article enriches the study of urban resilience theory and clarifies the relationship between industrial structure and environmental pressure on urban ecological resilience, which is of guiding significance to further promote the green development of resource-based cities.
Introduction
Since the Industrial Revolution, natural resources have gradually become an important material basis for the production and manufacture of various industrial and agricultural products and play an important role in the economic and social development of a country. Countries are also paying more and more attention to the exploration and development of natural resources, and there are more and more resource-based cities with natural resource development and processing as their leading industries. In the past period of time, resource-based cities have provided strong material guarantee for regional and national energy security and industrialization level enhancement, and made outstanding contributions to China’s economic development. However, with the constant adjustment of the structure of production factors in the process of economic growth, resource-based cities are faced with dramatically increasing extraction costs and external competitiveness due to resource depletion, and resource-based cities with gradually insufficient economic development momentum (Zeng, 2013; Zhang and Wang, 2014). The early resource-based industries were developed crudely, and the development model of high input, high energy consumption, and increased pollution caused significant damage to the regional ecological environment. The environmental pollution level of some resource-based cities has approached or exceeded the “threshold” of the ecosystem (Zhao and Liu, 2013). The urban ecosystem suffers from unavoidable disturbances and impacts, not only from the ecological disorder brought about by human’s rough development patterns (Lu, 2013), but also from various natural disasters caused by natural laws, and resource-based cities are particularly affected. The impact on resource-based cities is undeniable. In the face of increasing human pressure on natural resources in economic development, urban ecosystems need to improve their resilience, which means the stability of urban ecosystems needs to be improved, i.e., the ability of cities to resist shocks, adapt, and recover aftershocks to achieve the dissipation and absorption of these disturbances (Wang et al., 2021). Most resource-based cities are facing severe pressure and challenges, on the one hand, the challenge of industrial structure transformation, in order to cope with resource depletion, resource-based cities must switch to new leading industries and seek new growth points for urban economic development; on the other hand, ecological and environmental pressure, due to the lack of environmental awareness, many resource-based cities have caused greater pollution and damage to the ecological environment during resource extraction and processing, and urban and regional ecosystems are under greater pressure (Wang, 2022). Chinese resource-based cities have difficulties in the process of economic development and transformation, among which the industrial structure and serious industrial environmental pollution are important factors limiting the transformation and development of resource-based cities (Zheng et al., 2020). In this context, it is crucial to study the relationship between industrial structure, environmental stress, and ecological resilience in resource-based cities.
The word “resilience” is derived from the Latin word “resilio”, which initially meant “to return to the original state”. As time evolved, the concept of resilience was applied to different disciplines. It was first applied to system ecology to describe the steady state of ecological systems and then gradually extended from natural ecology to human ecology. In general, the research has gone through “engineering resilience, ecological resilience, and socio-ecological resilience” (Holling, 1973). In terms of resilience theory, Canadian ecologist Holling proposed the theory of “hierarchy, chaos, and adaptive cycles,” which revealed the meaning of sustainable development. Breaking the current thinking, building the idea of steady-state equilibrium and achieving the innovative breakthrough of cross-scale dynamic interaction cycle and system. That laid the ideological foundation for the formation of urban resilience theory (Berks and Folke, 1998; Safa and Jorge, 2016). In general, the academic community has reached a certain consensus on the definition of urban resilience, which includes the ability of the urban system to coordinate and organize itself and cope with uncertain external risks. It is a combination of urban material and immaterial (Wang et al., 2021).
On this basis, existing studies have quantitatively evaluated the level of urban resilience using different perspectives and methods (Du et al., 2019), providing basic ideas for planning and constructing resilient cities (Zhang and Feng, 2019). However, few studies have combined urban industrial structure and urban environmental stress levels to investigate their effects on urban ecological resilience. Therefore, based on 432 sets of data from three major resource-based provinces, Anhui, Shanxi and Sichuan, this paper will use panel data to establish a fixed effect model for empirical analysis based on the entropy value method and principal component analysis to measure industrial structure, environmental pressure and ecological resilience of cities, and explore the role of the relationship between industrial structure, environmental pressure and ecological resilience of resource-based cities. The marginal contribution of this paper is to quantitatively measure the environmental pressure and its ecological resilience in 24 resource-based cities in China using various indicators, and to further explore the role of human economic production and social activities on the ecological resilience of resource-based cities from the perspectives of industrial structure and environmental pressure. This article theoretically enriches the theoretical study of urban ecological resilience and realistically provides theoretical and policy references for promoting the industrial transformation of resource-based cities, improving their ecological resilience, and thus promoting the construction of ecological civilization in resource-based cities.
Theoretical Analysis and Research Hypothesis
The Impact of Industrial Structure on the Ecological Resilience of Cities
Ecological resilience focuses on depicting the coordinated development of human and environmental systems. It refers to the extent to which urban ecosystems are able to defuse change between reorganization and the formation of new structures (Zheng et al., 2013). Socio-ecological resilience further focuses on the extent to which social systems avoid collapse or unsuitability for human survival due to disasters, which focuses on how to foster and maintain the resilience of socio-environmental systems in human-nature interactions (Zhou, 2015). Research focuses on cultivating and strengthening the resilience of socio-environmental systems in the interaction between humans and nature (Zhou, 2015). The industrial structure, also known as the industrial system, is essential to socio-economic system and an expression of human economic activities. The influence between regional industrial structure and urban ecological environment is mutual. The effect of the ecological environment on industrial development is realized through environmental regulation. The promotion of environmental protection policies will inhibit the growth of high pollution and high energy-consuming enterprises. The promotion of environmental protection policies will impede the development of high pollution and high energy-consuming enterprises and promote industrial restructuring and upgrading.
On the other hand, industrial restructuring will promote technological progress and support new industries and reduce the proportion of highly polluting and energy-consuming industries and control the generation and emission of pollution at source. (Yuan and Xie, 2014). (Li, 2018) studied the Yangtze River Economic Belt and found that the regional industrial structure could aggravate the deterioration of the ecological environment. The development of the primary industry has the least pollution on the ecological environment; the secondary industry has the most significant impact on industrial solid waste emissions. The tertiary industry is conducive to improving the quality of the ecological environment (Yang and Xu, 2015). However, the data show that the proportion of secondary industry in resource-based cities is mostly as high as 60–70% or even over 80% (Li and Zou, 2018), and the development model of cities dominated by secondary industry shows the typical “path dependence” and “lock-in effect”. The development pattern of cities with mainly secondary industries shows typical “path dependence” and “lock-in effect” characteristics. The large amount of capital gathered in the highly profitable secondary industry not only hinders the pace of industrial optimization and transformation, but also aggravates carbon emissions and deteriorates the urban ecological environment (Hou et al., 2018). And a single industrial structure can cause regional locking. A single industrial structure can cause the phenomenon of regional locking, which adversely affects the anti-risk ability of cities (Martin and Sunley, 2015). Based on this, this paper proposes the following hypothesis.
The Impact of Environmental Stress on Urban Ecological Resilience
A social-ecological system is one in which human beings are at the core. In this system, human activities play a vital role in the system’s stability (Wang, 2011). The components of the social-ecological system include people and their living environment. The productive activities of humans and their living environment interact with each other and constrain each other (Zhao and Wen, 2013). In other words, the socio-ecological system includes economic production, social life, and natural system. The main body of the economic output is human material life, which changes the spatial diversity and biodiversity of the ecosystem through industrial intensification and specialization, and the economic production system provides products and services for the social life system. The main body of the social life system is the various spatial activities of human beings, and the process of the activities must be accompanied by the consumption of natural resources and the encroachment on the ecological space of the natural ecosystem (Wang and Ouyang, 2012). Especially in developing countries with rapid economic development, accelerating urbanization, and expanding urban scale, the environment is under tremendous pressure in the development process. The emissions of haze, automobile exhaust, and industrial waste gas constantly threaten the natural environment of cities and made them constrained and hindered in the development process (Cheng et al., 2019). Urban development requires sustainable natural environmental conditions, such as sound and diverse ecosystems and sufficient and available natural resources. Meanwhile, infrastructure in a suitable environment can meet the basic needs of urban development and crisis response. Good land planning and utilization policies can help reduce environmental stress in cities, all of which contribute positively to the resilience of cities (OECD 2016). However, for resource-based cities, economic development depends on the consumption of natural resources and ecological damage. While maintaining rapid economic growth and increasing economic development, it often causes severe environmental damage, and environmental pressure exacerbates the impact of the level of economic development on urban resilience (Feng et al., 2020). Based on this, this paper proposes the following hypothesis.
The Interaction Between Industrial Structure and Environmental Pressure
The relationship between industrial structure and environmental pressure is also reciprocal. In recent years, China’s economy has continued to develop rapidly, and the process of industrialization and urbanization has accelerated significantly while the environmental problems brought about by the rough development have become increasingly serious. Cai and Li (2009) analyzed environmental pollution and economic development in China since 1991 based on a model of three wastes, per capita income, and industrial structure. They found that industrial structure had a significant effect on environmental quality, and the higher the share of industry in the national economy, the worse the environmental quality. However, other scholars found that industrial structure significantly affected ecological stress, state, response, and overall environmental quality in Hunan Province. The positive impact of industrial structure on ecology is more significant than environmental stress (Han and Li, 2010). Wan and Dong (2012) analyzed the mechanism and evolution of the coupling between industrial restructuring and environmental quality in Gansu from the perspective of industrial restructuring. They concluded that although industrial restructuring had promoted economic development, it had brought enormous environmental pressure and different industries had different pressures on the environment due to their different nature. Bai et al. (2017) studied the spatial and temporal characteristics of cities and environmental stress using the middle reaches of the Yangtze River urban agglomeration as a sample, and the results of the study showed that the scale of the urban secondary industry had a significant positive effect on regional environmental pressure. In conclusion, the industrial structure and ecological environment are mutually constrained and influenced by each other. Only an advanced and rational industrial structure can make efficient use of resources, and an unreasonable industrial structure will lead to waste of resources, environmental pollution and ecological damage (Nie, 2012). In the three industrial structures, when the secondary industry dominates, its production uses a large amount of fossil fuels, and the more energy it consumes and the more pollution it emits, the more environmental pressure will increase with the increase in the proportion of the secondary industry (Zou., 2015). Compared with other cities, the industrial development of resource-based cities is more dependent on resources, and the industrial structure of most cities is an industry-led “two-three-one-one” model, which does not have a high enough level of resource utilization and excessive pressure on resources and environment in the urbanization process (Feng and Dong, 2018). Based on the above analysis, the following hypotheses are proposed in this paper. Figure 1 shows the theoretical analysis framework of this paper.
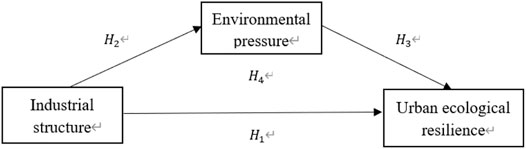
FIGURE 1. Relationship between environmental pressure, industrial structure and urban ecological resilience.
Study Design
Study Object
According to the relevant provisions of the National Sustainable Development Plan for Resource-based Cities (2013–2020) promulgated by the State Council, China has 262 resource-based cities, including 126 prefecture-level cities, 62 county-level cities, 58 counties (autonomous counties and forest areas), and 16 municipal districts (development zones and management areas). Based on this Plan, and considering the level and influence of resource-based cities, as well as the urgent factors of transformation and development, three resource-based provinces in the east, middle and west are selected for the study, namely Anhui, Shanxi and Sichuan provinces. These three provinces are rich and complete in mineral resources compared with other provinces in China, among which Anhui Province has 25 billion tons of coal reserves, 2.99 billion tons of iron ore reserves, 3.849 million tons of copper ore reserves and 564 million tons of sulphide iron ore reserves, ranking 7th, 5th, 5th, and 2nd respectively in China; Shanxi Province has the first coal-bed methane, bauxite, refractory clay, magnesium ore and Metallurgical dolomite and other 5 in China; Sichuan Province has 32 kinds of mineral reserves in the top 5 in the country, including natural gas, titanium ore, vanadium ore, sulfur iron ore and other seven kinds of mineral reserves in the first place in the country. At the same time, considering the availability, objectivity and scientificity of the relevant data indicators, the 24 prefecture-level municipal districts finally selected for the study, including Datong, Changzhi, Yangquan, Shuozhou, Jincheng, Jinzhong, Yuncheng; Anhui province, including Huainan, Maanshan, Huaibei, Tongling, Chuzhou, Suizhou, Chizhou, Xuancheng; Sichuan province, including Zigong, Panzhihua, Luzhou, Guangyuan, Guang’an, Nanchong, Dazhou, and Ya’an.
Data Sources and Construction of Indicator System
Data Source
This paper selects panel data indicators related to the socio-economic development of 24 resource-based cities from 2001 to 2018. The original data were obtained from the China City Statistical Yearbook (2002–2019), the statistical yearbooks of each city, the statistical yearbooks of the corresponding provinces, and the statistical database of the China Economic Network, starting and ending in 2001–2018, with 432 data sets.
Standardization of Data
Since the obtained data have different dimensions, orders of magnitude, and attributes, in order to show the accurate empirical results as much as possible, it is necessary to exclude the influence caused by the different units, meanings, and orders of magnitude of indicators, and this paper adopts the extreme difference method to standardize the indicators dimensionless treatment. The calculation equation are as follows.
Where
Determination of Index Weights
In this paper, the entropy weight coefficient method and the principal component analysis method are mainly used to determine the weights of each index.
(1) Entropy weighting method. First, according to the n evaluation indicators and m evaluated objects, the entropy value of the I indicator is defined.
Where
In this Eq. 4
(2) Principal component analysis method.
The principal component analysis is a method of dividing the original multiple variables into a few composite indicators. It is a technique of dimensionality reduction. The study conducted the principal component analysis with the help of SPSS software. Firstly, the data were standardized and then the correlation coefficient matrix was calculated, and the correlation coefficient matrix is used to calculate the eigenvalues, contribution rate, and cumulative contribution rate of each principal component to determine each index’s composite score and weight.
Construction of Indicator System
Regarding previous studies and considering the measurability of index data, this paper selects the proportion of primary industry to GDP, the proportion of secondary industry to GDP and the proportion of tertiary industry to GDP to measure the industrial structure of each city, and obtains the industrial structure index through the entropy weight method to downscale the index. (Chen and Bai, 2020) Urban ecological resilience is mainly expressed as the ability of cities to resist ecological risks, and urban ecological risks are manifested as a sharp decrease in the area of public green space in urban construction areas, defects in sewage and waste treatment systems, interruption of energy flow and ecosystem overload. Therefore, this paper selects five indicators to measure urban ecological resilience from three aspects: green space coverage, pollutant discharge efficiency and resource reuse capacity: comprehensive utilization rate of general industrial solid waste, centralized treatment rate of sewage treatment plants, harmless treatment rate of domestic waste, park green space area and green coverage rate of built-up areas (Zhu and Sun, 2020). Among them, the comprehensive utilization rate of general industrial solid waste reflects the ability of urban ecological resources reuse; the centralized treatment rate of sewage treatment plants and the harmless treatment rate of domestic waste reflect the ability of urban resources recycling and reuse; the area of park green space and the green coverage rate of built-up areas reflect the ability of urban self-purification. The weights of each indicator are shown in the following Table 1.
Environmental stress is a concept with many factors and complex connotations, which is currently defined by academics as the disturbance force on the state of the environment caused by human activities that can cause degradation of environmental service functions (Gu et al., 2005). Urban environmental pressure is mainly manifested in the impact and pressure exerted by human economic and social activities on urban ecological environment, which is mainly reflected in the occupation of urban space and the consumption of natural resources. In this paper, with reference to previous studies and the actual situation, the measurement of environmental pressure includes four aspects including population pressure, water resource pressure, energy pressure, and land resource pressure, and six indicator measures are selected accordingly (Cheng et al., 2019). The environmental pressure index system was constructed by standardizing the data through the polar difference method and applying the principal component analysis to determine the weights.
Specifically, Bartlett’s sphericity test and KMO test were performed on the indicator data from 2001 to 2018. The KMO value was 0.624 > 0.5 and passed Bartlett’s sphericity test, indicating that the sample was suitable for factor analysis, as shown in Table 2.
Secondly, in order to make the factor analysis results have more reasonable economic meanings, this paper selects the maximum variance method for factor rotation. It uses the principal component method to extract the two common factors with characteristic roots more significant than 1, whose cumulative variance contribution rate is 63.55%. The variance contribution rate of common factor 1 is 41.723%, which includes the water consumption per resident, electricity consumption per resident, paved road area per resident, and total population at the end of the year in the cause factor. Factor 2, on the other hand, includes per capita household gas usage, total population at the end of the year, and natural growth rate. Finally, the weights of each indicator were calculated by weighting and summing the scores of each indicator of environmental stress with the variance contribution of each common factor as the weight, as shown in the following Table 3.
Model Setting and Variable Selection
Selection of Variables
Based on the above analysis, the dependent variable of this paper is the ecological resilience of resource-based cities, which is a continuous variable. The independent variables are industrial structure and environmental pressure in resource-based cities, the measurement methods of which are shown in the above analysis. The control variables are selected as total investment in fixed assets, per capita gross regional product, and gross regional product of the city. The specific meaning symbols and descriptive statistics of each variable are shown in the following Table 4.
Model Selection
Before using panel data for regression analysis, it is necessary to consider the choice of fixed-effects, random-effects, and mixed estimation models. First, the mixed estimation or fixed-effects model is determined by the F test, which shows that F (23, 403) = 9.41, F = 0.000 < Prob, and the original hypothesis is rejected, and the fixed-effects model should be chosen. Second, the Hausman test was used to determine further whether it was a fixed-effects model or a random-effects model. Since the random effects model sets the individual effect as part of the disturbance term, it requires that the explanatory variables are not correlated with the individual effect. In contrast, the fixed-effects model does not require this assumption. The Hausman test results show a significance chi (5) = 458.72 at the 5% level, p = 0.000 < 0.05, implying that the fixed effects model is superior to the random-effects model. This paper selected the fixed effects model for analysis using stata15 software with the following equation.
Where i represents the prefecture-level city, t represents the year,
This paper draws on the intermediary effect test proposed by Baron and Kenny (1986) and Wen et al. (2004) to construct the following model to test whether environmental pressure plays an intermediary role in the process of an industrial structure affecting urban ecological resilience. The formula are as follows.
In Eq. 6,
Regression Analysis
This paper uses hierarchical regression analysis of mediating variables to test the hypotheses. First, model one is constructed by putting in the control variables (GI, D, G) and the dependent variable (ER). Second, model two is constructed by putting in the independent variable (I), the control variables (GI, D, G), and the dependent variable (ER). Third, model three is constructed by putting in the independent variable (I), the control variables (GI, D, G), and the dependent variable (ET). Fourth, model four is constructed by putting in the mediating variable (ET) as the independent variable, the control variables (GI, D, G), and the dependent variable (ER). Fifth, model 5 is constructed by placing independent variables (I), control variables (GI, D, G), mediating variables (ET), and dependent variables (ER), where model two is used to test hypothesis
As can be seen from the above table, the regression coefficients of the control variables in model one all pass the significance test of p < 0.05, indicating that total investment in fixed assets, per capita gross regional product, and gross regional product have significant effects on the dependent variable, and all of them positively improve the ecological resilience of the city. The regression coefficient of the independent variable environmental stress in model two is −0.910 (p < 0.01), indicating that environmental stress has a significant negative effect on the ecological resilience of resource-based cities, i.e., environmental stress significantly reduces the ecological resilience of resource-based cities, and hypothesis
According to the results of the above regression analysis, it is found that the industrial structure of resource-based cities has a significant positive effect on environmental pressure, and both industrial structure and environmental pressure have a significant negative effect on urban ecological resilience. This is because the economic development level of resource-based cities is lower than other cities and they mainly rely on the development of the secondary industry to drive economic development. Economic growth largely depends on the input of energy factors. At the same time, it is inevitable to cause a large consumption of natural resources and continuous destruction of the ecological environment, i.e., economic growth has increased the environmental pressure. The results are also consistent with the findings of previous studies, which show that the advanced industrial structure of economic growth has not yet revealed the feedback to the ecological environment, so the environmental pressure and industrial structure will reduce the ecological resilience of resource-based cities.
Heterogeneity Test
In order to further explore the influence of industrial structure and environmental pressure on the ecological resilience of cities in different spaces, the panel data are subjected to panel regression analysis in other provinces, and the regression results are shown in the table below. From the regression results, the industrial structure of all three provinces has a significant effect on the ecological resilience of cities, among which the absolute value of the regression coefficient of Shanxi province is the largest at 2.474, indicating that the industrial structure of each resource-based city in Shanxi province has a significant negative effect on the ecological resilience of its cities at the significance level of 0.01. This is followed by the absolute value of the regression coefficient of 1.693 for Anhui Province and 0.675 for Sichuan Province. The reason for this is that the economic development of Shanxi Province is more dependent on natural resources than the other two provinces, and its secondary industry has a greater proportion, so the industrial structure has a stronger effect on the ecological resilience of the city, which again verifies hypothesis
Robustness Test
In order to test the robustness of the above results, the proportion of employees in the three industries to total employees in resource-based cities is substituted for the industrial structure measure, and this variable is brought into the regression model to observe whether the results obtained are consistent with the estimated results. The results of the test are shown in the following Table 7.
According to the regression results in, it is found that both industrial structure and environmental pressure have significant adverse effects on urban ecological resilience, with the negative effect of environmental pressure being more significant. In contrast, industrial structure has a significant positive effect on environmental stress, i.e., the unreasonable industrial structure of resource-based cities will aggravate the environmental stress of cities. The results of model 5 indicate that environmental stress does not play a mediating role in reducing urban ecological resilience by industrial structure. The overall robustness test results are basically consistent with the regression analysis results above, and the results of each control variable do not change significantly, indicating that the regression model is basically robust.
Conclusions and Policy Recommendations
Industrial structure and environmental pressure are the key factors affecting the ecological resilience of resource-based cities. Based on 432 sets of data from three resource-based provinces, Anhui, Shanxi, and Sichuan, this paper explores the relationship between industrial structure, environmental pressure, and ecological resilience of resource-based cities by establishing a fixed effect model using panel data for empirical analysis on the basis of measuring industrial structure, environmental pressure and ecological resilience of cities using entropy value method and principal component analysis. The results of the study indicate that:
First, both regional industrial structure and environmental pressure significantly negatively affect urban ecological resilience. The economic development of resource-based cities mainly relies on the development of secondary industry, primarily dependent on natural resources. Still, natural resources are limited, and the rough economic growth will accelerate the depletion of natural resources. As a result, these cities have to break through the dilemma caused by internal resource depletion and cope with the disturbance of the external environment at the same time. Therefore, to enhance the resilience level of resource-based cities’ ecosystems, the transformation and development of resource-based cities are imminent.
Second, regional industrial structure has a significant positive effect on urban environmental pressure. The economic development of resource-based cities relies on the consumption of natural resources, which leads to a gradual increase in environmental stresses, such as air pollution, soil degradation, natural disasters, etc. With the implementation of energy revolution strategies around the world, such as the information platform of green, safe and efficient coal mining in Shanxi Province, the recycling of coal washing, coal motor and coal chemical waste, as well as the coordinated development of new energy sources such as wind power and photoelectricity and the multi-level energy supply system of coal-bed methane power generation, the dependence of economic development on natural resources will certainly be slowed down, while reducing environmental pressure and promoting the continuous improvement of urban resilience.
Third, the separate studies of the impact of industrial structure on urban resilience in each province found that the industrial structure of resource-based cities in Shanxi province has a greater negative effect on the ecological resilience of its cities, followed by Anhui and Sichuan. The reason for that is Shanxi Province, as a major coal resource province, is dependent on coal resource consumption for its economic growth, while other provinces are relatively less resource-dependent. At the same time, it is found that the per capita gross regional product has a positive effect on the ecological resilience of cities, which means that a certain degree of economic development level is conducive to strengthening the ecological resilience of cities.
This paper combines the above research findings and puts forward the following policy recommendations.
First, accelerate the transformation and upgrading of industrial structure and improve the ecological resilience of resource-based cities. The government departments of each region should formulate reasonable industrial policies taking into account their own ecological and environmental conditions and industrial development needs. According to local conditions, select high-tech industries as the leading industries and accelerate strategic emerging industries such as new energy, artificial intelligence, energy conservation, environmental protection, green agriculture, etc. At the same time, each region should also make reasonable use of the local natural environment and vigorously develop tourism and cultural and creative industries as the representative of clean service industries, gradually optimize and improve the transformation and upgrading of industrial structure and promote the green and healthy development of the city.
Second, strengthen the implementation of environmental policies, actively guide the public to use clean energy and green consumption. At the same time bring into play the social power of social third-party public welfare organizations and non-profit environmental protection organizations to fully mobilize the public to protect the environment in order to reduce urban environmental pressure and improve urban ecological resilience.
Data Availability Statement
Publicly available datasets were analyzed in this study. This data can be found here: China City Statistical Yearbook. And the specific websites of the original data are as follows: https://kns.cnki.net/kns8/DefaultResult/Index?dbcode=CYFD&kw=Chinacitystatisticalyearbook&korder=SU
Author Contributions
HW: Conceptualization, Methodology, Supervision; YC: Data curation, Writing-Reviewing and Editing.
Funding
This study is supported by Shaanxi Provincial Soft Science Project, “Research on the mode and effect of rural ecological environment governance in Shaanxi in the post-poverty alleviation era” (Project No. 2021KRM051); Shaanxi Provincial Social Sciences Major Theoretical and Practical Issues Research Major Project Subproject, Shaanxi Agricultural Industry High Quality Development Level Measurement and Target Framework Construction. (Project No. 2021ZD1055).
Conflict of Interest
The authors declare that the research was conducted in the absence of any commercial or financial relationships that could be construed as a potential conflict of interest.
Publisher’s Note
All claims expressed in this article are solely those of the authors and do not necessarily represent those of their affiliated organizations, or those of the publisher, the editors and the reviewers. Any product that may be evaluated in this article, or claim that may be made by its manufacturer, is not guaranteed or endorsed by the publisher.
References
Bai, L., Jiang, L., and Liu, Y. B. (2017). Spatial and Temporal Characteristics of Environmental Pressure in the Middle Reaches of Yangtze River Urban Agglomeration--Taking Industrial SO2 Emissions as an Example. Econ. Geogr. 37 (03), 174–181. doi:10.15957/j.cnki.jjdl.2017.03.023
Baron, R. M., and Kenny, D. A. (1986). The Moderator-Mediator Variable Distinction in Social Psychological Research: Conceptual, Strategic, and Statistical Considerations. J. Pers. Soc. Psychol. 51 (6), 1173–1182.
Berkes, F., and Folke, C. (1998). “Linking Social and Ecological Systems for Resilience and Sustainability,” in Linking Social and Ecological Systems: Management Practices and Social Mechanisms for Building Resilience. Editors F. Berkes, and C. Folke (Cambridge, UK: Cambridge University Press), 13–20.
Cai, H. G., and Li, H. Z. (2009). Per Capita income, Industrial Structure and Environmental Quality-Aan Analysis Based on EKC Curve. Econ. Manage. 23 (01), 15–17+34.
Chen, Y., and Bai, Z. (2020). Analysis of the Influence Mechanism of Technological Progress Bias, Industrial Structure and the Change of Labor Income Share in China: a Test Based on Provincial Data. Shanghai Econ. Res. 1-14. doi:10.19626/j.cnki.cn31-1163/f.20200526.001
Cheng, H., Yang, G. L., and Ji, X. J. (2019). Study on the Relationship between Urban Resilience and Environmental Pressure Decoupling in Ten Major Urban Clusters in China. Stat. Decis. Making 35 (07), 79–83. doi:10.13546/j.cnki.tjyjc.2019.07.020
Du, Z. W., Zhao, H. O., Ye, Y. Y., Jin, L. X., and Xu, Q. (2019). Urban shrinkage and growth: Measurement and determinants of economic resilience in the Pearl River Delta. J. Geogr. Sci. 29 (08), 1331–1345.
Feng, J. Y., Liu, Y. L., Wang, J., and Zhang, H. M. (2020). The Impact of Economic Development Level and Environmental Pressure on Urban Resilience - Based on Panel Data of 11 Prefecture-Level Cities in Shanxi Province. Ecol. Econ. 36 (09), 101–106+163.
Feng, X., and Dong, M. (2018). Research on the Resource and Environmental Impact of Economic Development in Northwest China. Journal of Northwest University for Nationalities, 67–72. doi:10.14084/j.cnki.cn62-1185/c.2018.01.011
Gu, X. W., Wang, Q., Liu, J. Z., Ding, Y., and Liu, J. X. (2005). Environmental Stress Indicators and Applications. China Environ. Sci. 03, 315–319.
Han, F., and Li, H. (2010). Analysis of the Impact of Industrial Structure on Ecological Environment in Hunan Province. Reg. Res. Develop. 29 (05), 89–93+98.
Holling, C. S. (1973). Resilience and Stability of Ecological Systems. Annu. Rev. Ecol. Syst. 4. doi:10.1146/annurev.es.04.110173.000245
Hou, Y. L., Long, R. Y., and Chen, H. (2018). Research on the Sustainable Development of China’s Coal Cities Based on Lock-in Effect. Resour. Pol. 59, 479–486.
Li, H., and Zou, Q. (2018). Research on Environmental Regulation, Resource Endowment and Urban Industrial Transformation - a Comparative Analysis Based on Resource-Based Cities and Non-resource-based Cities. Econ. Res. 53 (11), 182–198.
Li, Q. (2018). Does Industrial Upgrading Promote Ecological Environment Optimization-an Analysis Based on Panel Data of 108 Cities in the Yangtze River Economic Belt. Finance Trade Res. 29 (12), 39–47. doi:10.19337/j.cnki.34-1093/f.2018.12.004
Lu, D. D. (2013). Comprehensive and Comprehensive Deployment of Resource-Based Cities into a New Stage of Sustainable Development. China Reform News 09. doi:10.28074/n.cnki.ncggb.2013.001116
Martin, R., and Sunley, P. (2015). On the Notion of Regional Economic Resilience: Conceptualization and Explanation. J. Econ. Geogr. 15 (1), 1–42. doi:10.1093/jeg/lbu015
Nie, X. T. (2012). Study on the Relationship between Industrial Structure Evolution and Environmental Quality in Guangzhou. Jinan University.
Safa, M., and Jorge, R. (2016). Modeling Sustainability: Population, Inequality, Consumption , and Bidirectional Coupling of the Earth and Human Systems. Natl. Sci. Rev. 3 (04), 470–494.
Tang, M. F., and Wu, Y. F. (2021). Research on the Construction and Governance of Resilient Communities Based on the Perspective of Innovation Ecosystem. Hunan Soc. Sci. (01), 96–103.
Wan, Y. K., and Dong, S. C. (2012). Research on the Interactive Coupling Mechanism of Industrial Structure and Environmental Quality--Gansu Province as an Example. Reg. Res. Develop. 31 (05), 117–121.
Wang, Q. Y. (2011). A Review of Research on the Conceptual Framework of Social-Ecological Systems, China Population. Resour. Environ. 21 (S1), 440–443.
Wang, R. S., and Ouyang, Z. Y. (2012). Social-economic-natural Composite Ecosystem and Sustainable Development. Proc. Chin. Acad. Sci. 27 (03), 337–345+403404+254.
Wang, S. J., Cui, Z. T., Lin, J. J., Xie, J. Y., and Su, K. (2021). A Study on the Coupling and Coordination of Urbanization and Ecological Resilience in the Pearl River Delta Region. J. Geogr. 76 (04), 973–991.
Wang, Y. X. (2022). Research on the Construction of Ecological Civilization in Resource-Based Cities in China--a Review of "Theoretical Construction and Practical Exploration of Ecological Civilization. Ecol. Economy 38 (03), 230–231.
Wen, Z. L., Zhang, L., and Hou, J. T. (2004). Mediating Effect Test Procedure and its Application. J. Psychol. (05), 614–620.
Yang, J. L., and Xu, J. (2015). Analysis of Dynamic Effects of Industrial Structure Changes on Ecological Environment in Economic Zones: Hu Bao-Ying Yu Economic Zone as an Example. Econ. Geogr. 35 (10), 179–186. doi:10.15957/j.cnki.jjdl.2015.10.025
Yuan, Y. J., and Xie, R. H. (2014). Research on the Effect of Industrial Restructuring of Environmental Regulation - an Empirical Test Based on Inter-provincial Panel Data in China. China Ind. Economy (08), 57–69. doi:10.19581/j.cnki.ciejournal.2014.08.005
Zeng, W. P. (2013). Research on the Transformation Policy of Resource-Based Cities in China. Ministry of Finance: Institute of Finance Science.
Zhang, M. D., and Feng, X. Q. (2019). A Comparative Study on the Coordination of Urban Resilience and Economic Development Level of Cities within the Yangtze River Delta City Cluster. Urban Develop. Res. 26 (01), 82–91.
Zhang, W. Z., and Wang, D. (2014). Characteristics of Urbanization and Development Path Selection of Resource-Based Cities in China. China Land Resour. Economy 27 (06), 12–17.
Zhao, H., and Liu, X. M. (2013). Analysis of the Transformation Path of Resource-Based Economy" [J]. Urban Issues (07), 31–35. doi:10.13239/j.bjsshkxy.cswt.2013.07.009
Zhao, Q. J., and Wen, Z. M. (2013). Research on social-ecological systems and their resilience--a perspective based on complexity theory. J. Nanjing For. Univ. 13 (04), 82–89. doi:10.16397/j.cnki.1671-1165.2013.04.004
Zheng, Y., Wang, W. J., and Pan, J. H. (2013). Low Carbon Resilient Cities: Concepts, Approaches and Policy Options. Urban Develop. Res. 20 (03), 10–14.
Zheng, Z. Y., Qiu, F. D., Zhang, C. L., Li, X., Li, Cai., and Yin, P. X. (2020). Analysis of Functional Transformation Heterogeneity of Regenerative Resource-Based Cities and Their Industrial Structure. Resource Sci. 42 (03), 570–582. doi:10.18402/resci.2020.03.15
Zhou, L. M. (2015). From Social Vulnerability to Socio-Ecological Resilience: Paradigm Transformation of Disaster Social Science Research. Thought Line 41 (06), 50–57.
Keywords: resource-based cities, ecological resilience, industrial structure, environmental pressure, the secondary industry
Citation: Chen Y and Wang H (2022) Industrial Structure, Environmental Pressure and Ecological Resilience of Resource-Based Cities-Based on Panel Data of 24 Prefecture-Level Cities in China. Front. Environ. Sci. 10:885976. doi: 10.3389/fenvs.2022.885976
Received: 28 February 2022; Accepted: 14 March 2022;
Published: 26 April 2022.
Edited by:
Huaping Sun, Jiangsu University, ChinaReviewed by:
Zhen Liu, Nanjing Normal University, ChinaFeng Wang, Chongqing University, China
Yueqing Ji, Nanjing Agricultural University, China
Copyright © 2022 Chen and Wang. This is an open-access article distributed under the terms of the Creative Commons Attribution License (CC BY). The use, distribution or reproduction in other forums is permitted, provided the original author(s) and the copyright owner(s) are credited and that the original publication in this journal is cited, in accordance with accepted academic practice. No use, distribution or reproduction is permitted which does not comply with these terms.
*Correspondence: Hongmei Wang, d2htZWNvQG53c3VhZi5lZHUuY24=