- Laboratory of Ecology and Management of Fisheries Resources, Department of Botany and Ecology, Institute of Biosciences, Federal University of Mato Grosso, Cuiabá, Brazil
Aquatic organisms that consume resources from riparian vegetation or floodplain forests act as trophic links between aquatic and terrestrial ecosystems. For instance, the pacu Piaractus mesopotamicus (Holmberg 1887), which is a migratory fish species, can play an important role in this interaction on Neotropical floodplains. We compared the stomach content of individuals from this species between two different parts of a large river floodplain during contrasting hydrological seasons to quantify the spatiotemporal contribution of allochthonous and autochthonous resources in their diet. Moreover, we performed a bipartite network approach to evaluate the sensitivity of these populations to a simulated loss of food resources through the potential deforestation of riparian vegetation. We found that in the upper catchment, individuals fed mainly on allochthonous items, but their identity depended on the season: fruits were the most abundant resource during the wet season, whereas leaves were more consumed during the dry season. In the lower catchment, the contribution of allochthonous resources was slightly lower in the dry season than in the wet season, when the pacu was able to explore the floodplain. Finally, the results from our consumer-resource network model indicated that this system is vulnerable to riparian deforestation since P. mesopotamicus is highly dependent on input from the riparian vegetation items to its feed.
Introduction
The transfer of energy between ecosystems is performed by a few key species that move across these contrasting environments. For example, salmon are known to play an important role in transporting nutrients from marine ecosystems to aquatic-terrestrial ecosystems when they return from the ocean to spawn in their home stream (Hilderbrand et al., 1999; Helfield and Naiman 2006). Nevertheless, the contribution of terrestrial ecosystems to the aquatic food web has also been demonstrated, such as the passive transport of detritus from uplands into streams (Polis et al., 1997; Pusey and Arthington 2003; Baxter et al., 2005; Davis et al., 2010; Ferreira et al., 2012). Additionally, large herds of mammalian herbivores have been responsible for the active transfer of nutrients and energy subsidies from terrestrial to aquatic food webs in many African rivers (e.g., Masese et al., 2015; Pringle, 2017; Masese et al., 2018). In rivers with floodplains, large amounts of debris, nutrients and sediments that are rich in organic compounds are exchanged between the river channel and the terrestrial environment, especially during the wet season (Junk et al., 1989). The organic matter that is produced on land during the dry seasons increases the productivity of aquatic plants and is a rich food source for aquatic detritivores during the flooding period (Polis et al., 1997). Identifying the sources of organic matter that support aquatic food webs is therefore essential for understanding the ecology of riparian ecosystems and wetlands (Davis et al., 2010). To quantify energy sources in studies of trophic ecology, isotopic techniques are recognized as the best method (Boecklen et al., 2011). However, to reveal the diversity of trophic interactions between aquatic and terrestrial species, diet analysis could be an appropriate method, as many studies have demonstrated (e.g., Correa et al., 2015; Correa et al., 2016; Araujo et al., 2021). This critical knowledge is pressing to support wetland management and conservation actions, especially given the increasing human activity that is rapidly changing the spatial patterns of large-scale habitat interfaces (Nakano and Murakami 2001; Wantzen, 2006; Cristescu and Boyce, 2013).
The direct consumption of food items from allochthonous origin has been documented in various fish species in diverse ecosystems (Davis et al., 2010). For example, in tropical freshwater systems, there is a remarkable dependence of fishes on terrestrial invertebrates (Lowe-Mcconnell, 1999). Freshwater fish may also consume plant matter of terrestrial origin, including leaves and fruits (Reys et al., 2009; Horn et al., 2011; Correa et al., 2018; Araujo et al., 2020). Furthermore, mutualistic relationships between frugivore fish and terrestrial trees have been reported in many species of fish, especially because they can act as dispersal agents for plant seeds (e.g., Goulding 1980; Kibutzki and Ziburski 1994; Correa et al., 2007; Galetti et al., 2008; Anderson et al., 2009; Reys et al., 2009; Anderson et al., 2011; Horn et al., 2011; Correa et al., 2016). Plant-frugivore fish relationships imply that overfishing can cascade down to the dynamics of floodplain forests, as several studies have suggested (e.g., Anderson et al., 2011; Correa et al., 2015; Araujo et al., 2021).
Consumption by migratory fish of items that are derived from riparian or flooded forests establishes a link between different environments (aquatic and terrestrial). Some of these interactions involve species that cross ecosystem boundaries (Polis et al., 1997). These migratory animals transport nutrients, energy and genetic material between distinct food webs and thus may have a significant effect on their structure and dynamics (Helfield and Naiman 2006). For example, fish migrations connect food webs of nutrient-rich floodplains to oligotrophic rivers because they transport biomass between these systems and supply resources to resident consumers (Goulding, 1980; Araujo-Lima and Goulding, 1997; Polis et al., 1997; Polis et al., 2004).
To better understand the trophic links between aquatic and terrestrial environments in the Neotropical River floodplain, we studied the diet of the pacu Piaractus mesopotamicus (Holmberg 1887), a fish species that may play a key ecological role linking aquatic and terrestrial environments due to its feeding ecology and seasonal migrations (Makrakis et al., 2007). The pacu belongs to the family Serrassalmidae and has a wide geographical distribution in the Plata River basin (Romagosa et al., 1988; Reis et al., 2003). The pacu is a lotic species that can reach lengths of more than 70 cm and reach the first sexual maturation at approximately 35 cm (Costa and Mateus 2009). Although adult individuals can be found throughout the river, spawn are restricted to the upstream reach, at the onset of the rainy season (austral spring, between October and January) after upstream migration (Ferraz de Lima and Chabalin, 1981; Costa and Mateus 2009; Rauber et al., 2021). Pacu is an omnivorous with a tendency to herbivory, and its diet is mainly composed of leaves, fruits, seeds, and flowers, being able to feed occasionally on small invertebrates and fish (Britski et al., 2007; Urbinati et al., 2010; Sado et al., 2020). This fish is an important commercial species in the Paraguay River Basin because it is highly sought by both recreational and commercial fishermen (Mateus et al., 2004; Mateus et al., 2011; Massaroli et al., 2021).
In the present study, we analysed the spatiotemporal variation in the diet composition of P. mesopotamicus, comparing populations from the upper and lower catchments of the Cuiaba River in Brazil, during different hydrological seasons. The goal was to reveal the network of trophic interactions and quantify the relative importance of riparian vegetation resources in the diets of these two populations during both the dry and wet seasons. We used a bipartite network approach to describe this consumer-resource system, especially to identify the main links in these interactions and to simulate potential responses of P. mesopotamicus to the elimination of food items that the vegetation provides, a scenario that could happen through deforestation.
Materials and methods
Study area
The Cuiabá River originates in the south-central region of the state of Mato Grosso (MT), in the Brazilian midwest, and flows southwest toward the Paraguay River (Supplementary Figure S1). Its basin covers an area of approximately 28,732 km2 and extends up to the municipality of Barão de Melgaço, MT (Figueiredo and Salomão 2009). The regional climate is tropical, with dry winters and rainy summers (type Aw, according to the Köppen climate classification; Alvares et al., 2013). The hydrological regime is characterized by seasonal variation in water levels in the river due to the annual flooding cycle that starts in December and ends in June (Fantin-Cruz et al., 2011).
The Cuiabá River basin flows through the Cerrado region of central Brazil, which has become one of the world’s most intensive agricultural regions during the last 3 decades. However, the intensity of land use in the Cuiabá River basin varies spatially. The lower basin region, which is located in the Pantanal wetland, has a low human population density and mostly supports livestock ranching. In contrast, in the middle and upper regions of the basin, there is a much larger human population and a higher pressure from agricultural activity. Due to these differences in land use, deforestation is more prevalent in the middle and upper regions of the basin. Moreover, the lateral expansion of the river into the floodplain is greater in the lower basin (hereafter termed “lower catchment”) than in the upper region (hereafter termed “upper catchment”).
The fish survey was conducted in the river channel of the upper catchment (Cuiabazinho River, in the region of Rosário Oeste), and in the river channel of the lower catchment (SESC Pantanal, in the region known as Porto Cercado). The upper stream sampling site is located ∼190 km upstream from Cuiabá city, while the downstream site is situated in the northern part of the Pantanal in the municipality of Poconé, ∼140 km downstream from Cuiabá (State of Mato Grosso, Brazil) (Supplementary Figure S1). The upper catchment is an important breeding ground for the species, whereas the lower catchment is an important nursery and growth area.
Sampling
Individuals from P. mesopotamicus were collected monthly from August 2006 to July 2007. For our statistical analysis, we divided the data into two groups: dry season months (August to November 2006 and July 2007) and wet season months (December 2006 and January to June 2007). Fish were caught using cast nets and gillnets and were euthanized by thermal shock through immersion in ice. Individuals were measured (total length in cm) and their stomachs were removed through a ventral incision performed with a scalpel, fixed in 10% formaldehyde and transferred to 70% alcohol after five days for subsequent analysis. A total of 143 individuals were analysed (length range: 34–75 cm). We quantified the stomach contents of 139 individuals (four individuals had an empty stomach), 87 from the upper catchment (Ndry = 63; Nwet = 24) and 52 (Ndry = 24; Nwet = 28) from the lower catchment. The stomach contents were placed in a Petri dish and examined under a stereomicroscope to identify and quantify the food items. First, we aggregated the different food types found within stomachs into four broad functional categories [plants (excluding seeds and fruits), fruits, seeds and animals] and then identified them to the lowest possible taxonomic level. We also opted to analyse corn and soybeans as separated items because they are bait used by fishermen to attract fishes, and do not occur naturally in this river basin (Massaroli et al., 2021). Finally, the volumetric method was used to quantify the volume of the different food items (Hyslop 1980) and determine their proportions (item volume/total volume) in the diet of each individual fish.
Data analysis
First, although our goal was not to evaluate seasonal (or spatial) differences in individual sizes, we used factorial ANOVA to compare the total length among seasons and sites, because differences in the total length could affect the conclusions regarding spatiotemporal variation in the diet. We applied a Factorial Analysis of Variance (ANOVA) with permutation tests (Anderson and Ter Braak, 2003) to evaluate differences in the consumption of individual food categories (i.e. fruits/seeds, leaves/roots/stalks, animals) between sites and seasons. We used this procedure because our dataset had outliers. The response variable was the volumetric proportion of individual food categories, and the explanatory variables were the sites (i.e., lower and upper catchment) and seasons (i.e., wet and dry seasons). When significant differences were found, we applied the post-hoc Tukey’s test (Higgins 2004) for pairwise comparisons. Each test was performed with 10,000 permutations. Furthermore, Spearman correlation analyses were computed between the volumes of the different food categories to evaluate whether greater consumption of one functional category was accompanied by a lower consumption of others.
We grouped the food categories (volume) in according to their origin, i.e., whether they were derived from the aquatic environment (autochthonous) or the terrestrial environment (allochthonous). Allochthonous resources, including leaves, fruits, seeds, flowers, roots (Ruelia sp.) and insects from terrestrial environment and autochthonous resources, encompassing macrophyte stalks, decapods and molluscs. We used these two groups because we were not interested in the identity of any particular resource but rather in the two broader categories that could be used to identify whether the resource originated in aquatic or terrestrial ecosystems. To classify food items, we used the tradition in floodplain ecology in considering leaves, fruits, seeds, flowers, roots and adult insects from terrestrial origin in riparian and floodplain forests as allochthonous resources (Goulding 1980; Junk et al., 1997; Correa & Winemiller 2014). Although riparian and flooded forests house plant species of terrestrial origin (allochthonous) and aquatic macrophytes (autochthonous) during the flooding season, these two groups can be easily distinguished based on the morphology and anatomy of the vegetative and reproductive structures. We restricted the analysis to identified items because we were not interested in understanding the variation in nonidentified food items. Individuals (or stomachs) were the sampling units from which different items were classified into autochthonous and allochthonous. Therefore, we used an ANOVA mixed effect model (Zuur et al., 2009) with permutation tests to analyse differences in the proportion of volume of consumed food by origin, site, and season in which each fish was considered to be as random effect because the items were measured in the same individuals. When ANOVA tests were significant, we used the post-hoc Tukey’s test with pairwise permutations for a posteriori comparison.
To evaluate the degree of connectivity between individual fish and resources, we applied a bipartite network approach (i.e., interaction network with two trophic levels; Dormann et al., 2008). Networks are mathematical structures composed of nodes and links (i.e., edges) between the nodes. In an ecological context, nodes may represent individuals, populations or species, guilds or functional groups, entire communities, or even entire networks (Hagen et al., 2012). Links are defined in an interaction matrix wherein the coarsest measure of link strength is the presence or absence of the interaction (Hagen et al., 2012). These methods have been used to analyse pollinator-plant and predator-prey interactions (Bascompte et al., 2003; Dormann et al., 2008, 2009), and they may also be applied to evaluate the interactions between individuals and resources to understand resource partitioning among different populations of a particular species (e.g. Pires et al., 2011), which is the case in the present study. The presence and absence of links were assigned based on whether an individual consumed a particular resource. Finally, we generated a bipartite graph to visualize the consumer-resource network (Pires et al., 2011).
Moreover, we wanted to assess how the P. mesopotamicus populations respond to the loss of component resources. Thus, we first simulated the elimination of food items, starting with the most common (i.e., the one with the highest number of links in the bipartite network), to determine if removing items would have a noticeable effect on individuals. For instance, an individual who consumed only one resource type would be “eliminated” from the network if that resource was removed (i.e., strong effect), while another that consumed a broad range of resources would persist (i.e., weak, or no effect). We also repeated this procedure but started with the elimination of the least common item (i.e., the one with the lowest number of links in the bipartite network). Furthermore, we assumed that the proportion of living individuals (y) is an exponential function of the proportion of eliminated items (x), whose decay is estimated by the coefficient a (y = 1–xa; Dormann et al., 2009; Memmott et al., 2004). The bipartite-slope exponent (a) was fitted to the elimination curve under a null model where resources were eliminated at random (Memmott et al., 2004; Dormann et al., 2009). High estimates of slope a mean that the network is less affected by link removal (Dormann et al., 2009).
Additionally, we computed the robustness (R) of the network as an additional metric to assess the tolerance of the system to resource loss. This coefficient is based on the fact that the removal of a given fraction of members of one group (e.g., the resources) causes the elimination of a number of members from the other group (e.g., individuals) (Memmott et al., 2004). The R coefficient is defined as the area under the elimination curve (Burgos et al., 2007). Curves that decrease very slowly to a point in which almost all items are eliminated exhibit an R close to one (Burgos et al., 2007). This represents a robust system in which most of the individuals survive even if a large fraction of the items is removed. In contrast, R values near zero correspond to curves that decrease sharply with the loss of any one component. This represents a fragile system in which most individuals lose all of their interactions and are eliminated with the removal of small fractions of food items (Burgos et al., 2007; Dormann et al., 2009; Dormann et al., 2013). Finally, we compared the estimates of a and R to null models (1,000 simulations), which were generated by calculating the probability that each cell in the bipartite matrix is occupied (filled with 1) as the mean of the occupation (Bascompte et al., 2003). These procedures were carried out separately for the lower and upper catchment populations.
All analyses were performed in R (R Core Team, 2020) using the lmPerm (Wheeler 2010) and bipartite packages (Dormann et al., 2008).
Results
Individuals who were analysed had lengths close to or higher than the length of the first maturation (from 34 to 75 cm; mean = 43.78; sd = 6.59). There was no interaction between site and season factors in determining length variation (F1;135 = 0.246; p = 0.62). In addition, there was no significant difference in length between sites (F1;135 = 0.77; p = 0.38) and seasons (F3;135 = 0.108; p = 0.74). Therefore, the dietary differences that we found across individuals cannot be attributed to spatiotemporal differences in the size distribution of the species.
We were able to identify 74% of the overall food volume that was consumed by the Pacu (26% was unidentifiable digested material). The fish consumed mainly leaves (44.86% of the identified volume), fruits and seeds (24.44%). Other plant parts, including flowers, stalks and roots, represented 20.37% of the identified resource volume. Animal resources were in much smaller proportions (5.71%) and included aquatic (molluscs and decapods) and a few terrestrial insects. The remaining (4.89%) items consisted of incidental materials (including coal and stones) as well as corn and soybeans, which are generally used by fishermen to lure fish. We identified 21 plant species from the stomach content, in which 12 were consumed exclusively in the upper catchment, 11 in the lower catchment and only two species [Alchornea sp (leaves) and Ocotea sp. (fruits)] were consumed at both sites (Table 1).
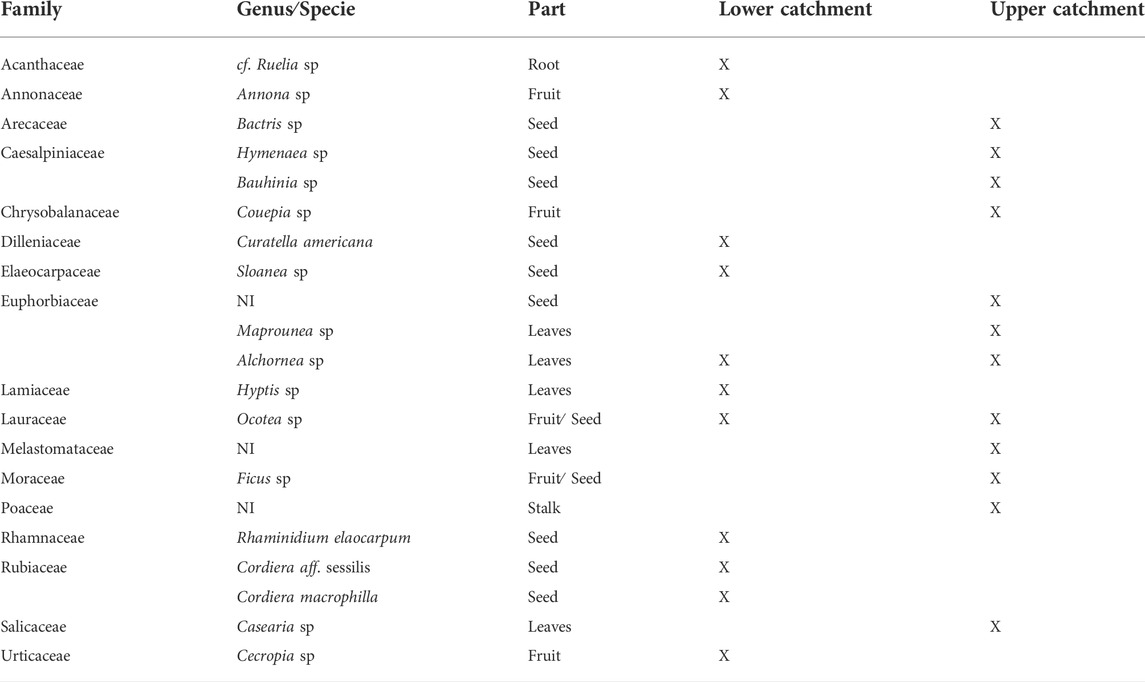
TABLE 1. Terrestrial plants identified in the stomach contents of Piaractus mesopotamicus at the sample sites. NI = not identified.
The seasonal difference in fruit consumption was site-dependent (site × season interaction: p = 0.004; Figure 1). While fruit consumption was greater during the flood season in the upper catchment (p < 0.001), no difference was found between seasons in the lower catchment (p = 0.487). The consumption of other plant parts also depended on the site (site × season interaction: p = 0.007; Figure 1) and was greater in the dry season than in the flood season in the upper catchment (p = 0.001) but not significantly different between seasons in the lower catchment (p = 0.704). The interaction between both factors was also significant for animal prey (site × season interaction: p = 0.012; Figure 1), although the pattern was different from what was observed for plant items. A greater number of animals were consumed during the dry season than in the wet season in the lower catchment (p = 0.018), but there was no significant difference between seasons in the upper catchment (p = 0.678).
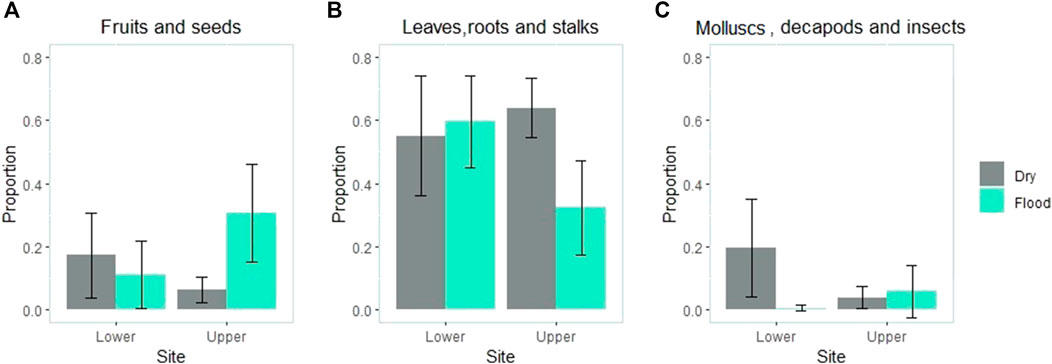
FIGURE 1. Volumetric proportion of items grouped into functional categories (A) fruit and seeds (B) roots, stalks and leaves; (C) molluscs, decapods and insects) consumed per site and season (blue = wet season; gray = dry season). Although we used a permutational ANOVA, mean values and 95% intervals of confidence bars are shown for clarity.
We examined the correlations between the consumption of items and found that the higher value of consumption of fruits and seeds in the upper catchment was associated with less consumption of other plant parts (rs = -0.334; p = 0.002). Conversely, the high value of consumption of plant parts in the lower catchment was associated with a lower consumption of animal items (rs = -0.311; p = 0.024).
The relative importance of resources from terrestrial and aquatic ecosystems in the P. mesopotamicus diet was also dependent on the site and season (site × item interaction: p < 0.001; season × item interaction: p = 0.038; Figure 2). The main food source in the upper catchment was allochthonous (p < 0.013), but there were similar volumes of allochthonous and autochthonous inputs in the lower catchment population (p = 0.897). In the upper catchment, resources came mainly from the riparian forest in both seasons (Pflood<0.001; Pdry<0.001; Figure 2). In the lower catchment, there was no significant difference between autochthonous and allochthonous inputs in dry season (p = 0.301; Figure 2), although the allochthonous input was higher than the autochthonous input (p = 0.024) during the rainy season.
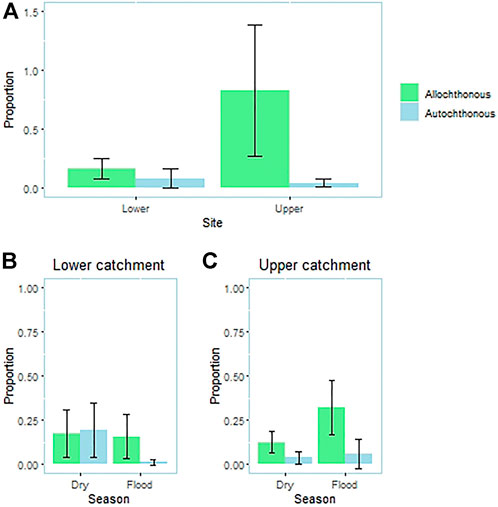
FIGURE 2. Comparison between the volume of resources of aquatic and terrestrial origin (riparian vegetation) between (A) sites and seasons (flood and dry season) in the (B) lower catchment and (C) upper catchment). Although we used a permutational ANOVA, mean values and error bars are shown for clarity.
The bipartite graphs in Figure 3 represent the consumer-resource networks for the upper and lower catchment populations. Not surprisingly, both networks were sensitive to the removal of common resources (i.e., highly connected items) and presented low values of a (upper catchment = 0.55; lower catchment = 0.69). The estimated value of a in the upper catchment was smaller than what would be expected by chance (a̅null = 0.87; sd = 0.21; p < 0.001), but in the lower catchment, it was not significantly different from a random pattern of resource elimination (a̅null = 0.71; sd = 0.064; p = 0.31). This suggests that the population from the upper catchment would be more sensitive than the lower catchment population to eventual riparian deforestation, as many individuals in the former are highly dependent on allochthonous input, especially from terrestrial plants. However, the networks exhibited low robustness values (Rupper catchment = 0.34; Rlower catchment = 0.21), which points to the fragility of both populations to the loss of a small fraction of food items. These robustness values were smaller than those expected by chance for both upper (
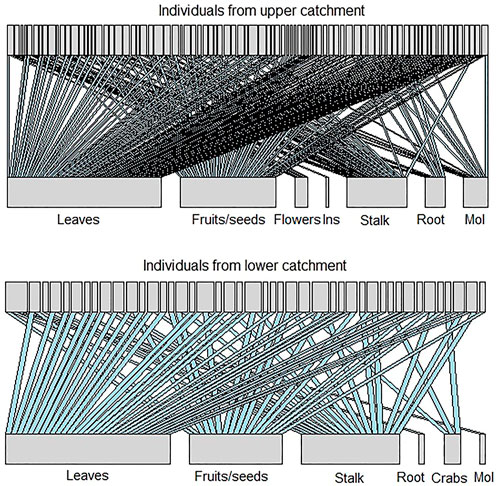
FIGURE 3. Bipartite graph showing the relationship between Piaractus mesopotamicus individuals and their diet resource in the upper catchment and in the lower catchment populations. The thickness of the resources bars expresses the frequency of interactions.
We found a relatively high value for coefficient a when the simulation started removing less common food items for the upper catchment (aupper catchment = 12.71). These resources were mostly autochthonous, and the high values for the bipartite-slope exponent suggest that this population would persist if aquatic prey were locally extinct (Rupper catchment = 0.91). However, starting the simulation of elimination for the lower catchment with the less common items made no difference (alower catchment = 0.693) and that the network is also more robust to elimination of aquatic items (Rlower catchment = 0.653). The a and R estimates did not differ from those that would be expected by chance at either site. These results suggest that the elimination of the less common dietary items has little effect on both upper (a̅null = 0.74; sd = 2.4; p = 0.99;
Discussion
Our study on the spatiotemporal pattern of resource consumption showed that P. mesopotamicus populations are highly dependent on terrestrial resources year-round, despite seasonal shifts in diet. The seasonal shift in the P. mesopotamicus diet was higher in the upper catchment population than in the lower catchment population. In addition, simulations to assess the sensitivity of their consumer-resource network suggested that the population dwelling in the upper catchment is more dependent on riparian vegetation resources than the population dwelling in the lower catchment.
The gut content analysis provided strong evidence that P. mesopotamicus is predominantly herbivorous (mainly leaves and fruits), although a few individuals also ate small animals. Their dietary versatility may be associated with resource abundance, which is well known to drive diet shifting in tropical freshwater ichthyofauna (Goulding 1980; Hahn et al., 1997; Lowe-Mcconnell, 1999). Fish species are able to shift their diet according to changes in resource availability (Goulding 1980; Hahn et al., 1997; Agostinho et al., 2007). In river floodplains, resource abundances are regulated by spatial and temporal variations in environmental conditions due to variations in forest cover and seasonal hydrological changes in the lateral extent of the river channel (Junk and Wantzen, 2004; Arantes et al., 2019; Larsen et al., 2019).
We found a greater volume of leaves in pacu stomachs than other types of resources. While 21% of the leaf content was only identified to the family level, all of them were from species belonging to the terrestrial ecosystem, as we did not find any leaves from aquatic macrophytes. However, P. mesopotamicus did consume aquatic macrophyte stalks, being the second and third most consumed item in the lower and upper catchments, respectively. Conversely, a low consumption of macrophyte leaves by fish was also reported for the Amazon basin (Merona, 1987; Araujo-Lima et al., 1995) and may be due to toxicity, low nutritional value and/or low digestibility (Goulding et al., 1988; Araujo-Lima et al., 1995).
The consumption of fruits was higher, as expected, during the wet season, when fruiting is more intense (Goulding, 1980; Lucas, 2008; Correa and Winemiller 2014). However, this was the case only for the upper catchment population. This is surprising, as lateral expansion of the littoral zone, which expands the opportunity for fishes to find a fruiting tree, is higher in the floodplain than in the upper catchment. Apart from differences in plant composition between the upper and lower catchments, the higher consumption of fruits in the upper catchment could also be explained by the higher aggregation of fleshy-fruited plants in the riparian forest. Unfortunately, we did not find available data to test these hypotheses. Regardless, in flooded systems, the synchrony between hydrological seasons and fish diet has been shown to be a key characteristic of the feeding ecology of several species (Lucas 2008; Costa-Pereira et al., 2011; Freitas and Almeida, 2011; Correa and Winemiller, 2014), especially in tropical floodplain forests (Goulding 1980). For example, Colossoma macropomum is frugivorous during the wet season but detrivorous during the dry season (Anderson et al., 2009, 2011). In addition, the abundance of seeds and fruits in fish diets is strongly associated with their availability (Davis et al., 2010), which depends on the flowering and fruiting phenology of plant species in the landscape (Correa et al., 2007; Correa and Winemiller 2014), although Araujo et al. (2020) recently showed that frugivorous fishes can actively select fruits.
Furthermore, we observed that the consumption of other plant parts decreased in the upper catchment population during the flood season when individuals consumed more fruits. However, there were no significant differences in the consumption of different food items between seasons in the lower catchment population, with the exception of a slight decrease in plant items in their diet when they fed more on animal prey during the dry season. The quantity and quality of available resources is important because it regulates the amount of energy available to the organism and will consequently affect their energy allocation process (Adams et al., 1982). Thus, the negative correlation between fruit and animal resources that we found in stomach contents may be advantageous for the species because the higher consumption of fruit when consumption of animals is low may improve their nutritional status. In turn, nutritional status affects growth and reproductive activity (Li et al., 2009). For example, gonadal development, fecundity and egg quality are all affected by the nutritional status of breeders and by specific essential nutrients (Brooks et al., 1997; Izquierdo et al., 2001). Moreover, migratory fish invest in energy storage prior to migration, and this energy is stored mainly as fat that will subsequently be converted into reproductive tissue (Arrington et al., 2006). Therefore, a decline in nutritional status may have negative consequences for individual reproduction that will result in reduced recruitment and thus smaller fish stocks.
Riparian vegetation items were the most common in the P. mesopotamicus diet in the upper catchment population during the flood season due to the higher availability of fruits and during the dry season due to the consumption of leaves. This observation suggests that riparian forests in the upper catchment are the main providers of resources for the pacu population in this river system. Allochthonous production in riparian ecosystems has been recognized as the most important basis for the diets of stream fishes (Esteves and Aranha 1999; Claro et al., 2004; Claro et al., 2004). Pacu consumed more plants in the lower catchment during the flood season. We think this has resulted from higher seasonal change in the availability of plant-derived food resources in the lower catchment than in the upper catchment. River channels overflow during the flood seasons and submerge larger adjacent areas with a higher abundance of trees (fruiting or not) in the lower catchment, which allows many fish to feed on abundant plant food sources during this season. Seasonal changes in the lateral extent of the river are lower for the upper catchment, which, in turn, gives rise to lower fluctuations in the availability of plant-derived food sources.
Goulding (1980) highlighted the crucial role of allochthonous subsidies from riparian vegetation to aquatic food webs in the floodplains of the Amazon River basin. Subsequent studies in other Neotropical river-floodplain systems have shown the depth and complexity of these coevolutionary relationships and have revealed an aquatic-terrestrial connection that is not paralleled anywhere in the world (Horn 1997; Drewe et al., 2004; Correa et al., 2007; Horn et al., 2011). The pacu is a large species that connects terrestrial and aquatic ecosystems because the energy that they incorporate from their allochthonous food sources becomes available to other large aquatic consumers, including gilded catfish (Zungaro zungaro) and spotted surubim (Pseudoplatystoma corruscans), which are large enough to prey on small-sized pacus. Conversely, this species is a potential prey for large vertebrates such as the giant otters Pteronuras brasiliensis (Rosas-Ribeiro et al., 2012) and caimans Caiman crocodillus yacare (Santos et al., 1996).
The results from our network simulations suggest that neither the upper nor the lower catchment networks are tolerant of the reduction of allochthonous food items. Additionally, the interaction between pacu and plants from the upper catchment may be more sensitive to deforestation than that from the lower catchment. However, pacu is an omnivorous species, and the reduction in terrestrial item input may force individuals toward a dietary shift (for an example, see Melo et al., 2019). A dietary shift is expected when deforestation occurs (Baxter et al., 2005; Bojsen 2005; Ferreira et al., 2012), as demonstrated by the deforestation caused by dams (Albrecht et al., 2009; Melo et al., 2019). However, since nutritional status affects growth and reproductive activity (Li et al., 2009), it is possible to imagine scenarios when fitness would be affected by poor diet. For example, if deforestation occurs upstream of a dam, and individuals cannot move downstream to find the most suitable habitat, diets can shift toward suboptimal resources, affecting the nutritional status of the individuals trapped upstream of the dam.
P. mesopotamicus and riparian plant species are likely to form a mutualistic relationship as the fish act as dispersal vectors (i.e., ichthyochoric dispersal) of their seeds (Galetti et al., 2008; Donatti et al., 2011; Correa et al., 2015; Correa et al., 2016). These interactions are common among frugivore fish and riparian plants (Horn 1997; Lucas 2008; Horn et al., 2011; Pollux 2011), and it has been recognized that frugivore fish may affect the recruitment and distributional patterns of trees, as they have different waste deposition patterns than other vertebrate frugivores (Chick, 2003; Correa et al., 2007; Galetti et al., 2008; Anderson et al., 2011; Horn et al., 2011). Although the role of pacu in the recruitment of riparian plants remains to be elucidated, the high degree of interdependence between fish and trees in tropical forests provides a strong argument for the conservation of both to maintain ecosystem functioning (Horn 1997).
Our results demand a cautionary note, as the method we used in our study (stomach content analysis) has limitations for the identification of several items (e.g., soft parts of animals, digested vegetable organic matter), which may compromise the quantification of their relative importance. Additionally, stomach content analysis represents a snapshot of food consumption, not of energy and nutrient absorption. In fact, it is plausible that food items that arise as important from the use of stomach content analysis may have contributed little of the energy and nutrient needed for somatic growth and reproduction. Therefore, a definitive conclusion about long term dependency between pacu and forest food resources can only emerge from the use of a more integrative methodology, such as stable isotope analysis. We recommend such studies as a further development of the analysis described in this paper. In summary, we showed that pacu is strongly dependent on riparian and floodplain vegetation as food resources. Consequently, it seems likely to be very sensitive to the degradation and deforestation of marginal forested lands. A recent study has shown the importance of conserving forests to maintain high fish catches and fisheries productivity in the Amazon (Barros et al., 2020). In this way, the conservation of riparian and floodplain forests should be considered essential for the maintenance of viable populations of pacu and probably other frugivorous fishes in the Pantanal.
Data availability statement
The raw data supporting the conclusions of this article will be made available by the authors, without undue reservation.
Ethics statement
Ethical review and approval was not required for the animal study because the specimens were collected under the legal authority permits issued by the Chico Mendes Institute for Biodiversity Conservation (ICMBio) number 16313.
Author contributions
LM and JP: ideas, analysis, and manuscript preparation. RC: data collection and laboratory analysis.
Acknowledgments
We warmly acknowledge the National Council for Scientific and Technological Development (CNPq)/Long-Term Ecological Research Program–PELD–Site 12 and the Pantanal Research Center (CPP)/Ministry of Science and Technology (MCT) for their financial support. We are also indebted to the Private Natural Heritage Reserve SESC Pantanal and their park guards for logistic support. JP thanks the Conselho Nacional de Desenvolvimento Científico e Tecnológico for research fellowships (CNPq #307002/2019–5) and the Instituto Nacional de Pesquisas do Pantanal (INPP) for logistical support. We thank all reviewers and the editor for their constructive review, which was very helpful in improving our manuscript. The specimens were collected under the legal authority permits (# 16313) issued by the Chico Mendes Institute for Biodiversity Conservation (ICMBio).
Conflict of interest
The authors declare that the research was conducted in the absence of any commercial or financial relationships that could be construed as a potential conflict of interest.
Publisher’s note
All claims expressed in this article are solely those of the authors and do not necessarily represent those of their affiliated organizations, or those of the publisher, the editors and the reviewers. Any product that may be evaluated in this article, or claim that may be made by its manufacturer, is not guaranteed or endorsed by the publisher.
Supplementary material
The Supplementary Material for this article can be found online at: https://www.frontiersin.org/articles/10.3389/fenvs.2022.883298/full#supplementary-material
References
Adams, S. M., Mclean, R. B., and Parrotta, J. A. (1982). Energy partitioning in largemouth bass under conditions of seasonally fluctuating prey availability. Trans. Am. Fish. Soc. 111, 549–558. doi:10.1577/1548-8659(1982)111<549:EPILBU>2.0.CO;2
Agostinho, A. A., Gomes, L. C., and Pelicice, F. M. (2007). Ecologia e manejo de recursos pesqueiros em reservatórios do Brasil. Maringá: Editora da Universidade Estadual de Maringá EDUEM.
Albrecht, M. P., Caramaschi, É. P., and Horn, M. H. (2009). Population responses of two omnivorous fish species to impoundment of a Brazilian tropical river. Hydrobiologia 627, 181–193. doi:10.1007/s10750-009-9727-7
Alvares, C. A., Stape, J. L., Sentelhas, P. C., Moraes Gonçalves, J. L., and Sparovek, G. (2013). Köppen’s climate classification map for Brazil. metz. 22, 711–728. doi:10.1127/0941-2948/2013/0507
Anderson, J. T., Nuttle, T., Saldaña Rojas, J. S., Pendergast, T. H., and Flecker, A. S. (2011). Extremely long-distance seed dispersal by an overfished Amazonian frugivore. Proc. R. Soc. B 278, 3329–3335. doi:10.1098/rspb.2011.0155
Anderson, J. T., Saldaña Rojas, J., and Flecker, A. S. (2009). High-quality seed dispersal by fruit-eating fishes in Amazonian floodplain habitats. Oecologia 161, 279–290. doi:10.1007/s00442-009-1371-4
Anderson, M., and Ter Braak, C. (2003). Permutation tests for multi-factorial analysis of variance. J. Stat. Comput. Simul. 73, 85–113. doi:10.1080/00949650215733
Arantes, C. C., Winemiller, K. O., Asher, A., Castello, L., Hess, L., Petrere, M., et al. (2019). Floodplain land cover affects biomass distribution of fish functional diversity in the Amazon River. Sci. Rep. 9, 16684. doi:10.1038/s41598-019-52243-0
Araujo, J. M., Correa, S. B., Anderson, J., and Penha, J. (2020). Fruit preferences by fishes in a Neotropical floodplain. Biotropica 52, 1131–1141. doi:10.1111/btp.12790
Araujo, J. M., Correa, S. B., Penha, J., Anderson, J., and Traveset, A. (2021). Implications of overfishing of frugivorous fishes for cryptic function loss in a Neotropical floodplain. J. Appl. Ecol. 58, 1499–1510. doi:10.1111/1365-2664.13891
Araujo-Lima, C. A., Agostinho, A. A., and Fabré, N. N. (1995). “Trophic aspects of fish communities in brazilian rivers and reservoirs,” in Limnology in Brazil. Editors J. G. Tundisi, C. E. Bicudo, and T. Matsumura-Tundisi (Rio de Janeiro: Academia Brasileira de Ciências e Sociedade Brasileira de Limnologia), 105–136.
Araujo-Lima, C. A., and Goulding, M. (1997). So fruitful a fish: Ecology, conservation and aquaculture of the amazon’s tambaqui. New York: Columbia University Press.
Arrington, D., Davidson, B. K., Winemiller, K. O., and Layman, C. A. (2006). Influence of life history and seasonal hydrology on lipid storage in three neotropical fish species. J. Fish. Biol. 68, 1347–1361. doi:10.1111/j.0022-1112.2006.00996.x
Barros, D., Petrere, M., Lecours, V., Butturi-Gomes, D., Castello, L., and Isaac, V. J. (2020). Effects of deforestation and other environmental variables on floodplain fish catch in the Amazon. Fish. Res. 230, 105643. doi:10.1016/j.fishres.2020.105643
Bascompte, J., Jordano, P., Melián, C. J., and Olesen, J. M. (2003). The nested assembly of plant-animal mutualistic networks. Proc. Natl. Acad. Sci. U. S. A. 100, 9383–9387. doi:10.1073/pnas.1633576100
Baxter, C. V., Fausch, K. D., and Saunders, W. C. (2005). Tangled webs: Reciprocal flows of invertebrate prey link streams and riparian zones. Freshw. Biol. 50, 201–220. doi:10.1111/j.1365-2427.2004.01328.x
Boecklen, W. J., Yarnes, C. T., Cook, B. A., and James, A. C. (2011). On the use of stable isotopes in trophic ecology. Annu. Rev. Ecol. Evol. Syst. 42, 411–440. doi:10.1146/annurev-ecolsys-102209-144726
Bojsen, B. H. (2005). Diet and condition of three fish species (Characidae) of the Andean foothills in relation to deforestation. Environ. Biol. Fishes 73, 61–73. doi:10.1007/s10641-004-5330-y
Britski, H. A., Silimon, K. Z. S., and Lopes, B. S. (2007). Peixes do pantanal. Manual de identificação. Brasília: Embrapa.
Brooks, S., Tyler, C., and Sumpter, J. (1997). Egg quality in fish: What makes a good egg? Rev. Fish. Biol. Fish. 7, 387–416. doi:10.1023/a:1018400130692
Burgos, E., Ceva, H., Perazzo, R. P. J., Devoto, M., Medan, D., Zimmermann, M., et al. (2007). Why nestedness in mutualistic networks? J. Theor. Biol. 249, 307–313. doi:10.1016/j.jtbi.2007.07.030
Chick, J., Cosgriff, R. J., and Gittinger, L. S. (2003). Fish as potential dispersal agents for floodplain plants: First evidence in north America. Can. J. Fish. Aquat. Sci. 1439, 1437–1439. doi:10.1139/F03-155
Claro, L., Ferreira, E., Zuanon, J., and Araújo-Lima, C. (2004). O efeito da floresta alagada na alimentação de três espécies de peixes onívoros em lagos de várzea da Amazônia Central, Brasil. Acta Amaz. 34, 133–137. doi:10.1590/S0044-59672004000100018
Correa, S. B., Araujo, J. M., Penha, J., Nunes da Cunha, C., Bobier, K. E., and Anderson, J. T. (2016). Stability and generalization in seed dispersal networks: A case study of frugivorous fish in neotropical wetlands. Proc. R. Soc. B 283, 20161267. doi:10.1098/rspb.2016.1267
Correa, S. B., Araujo, J. M., Penha, J., Nunes da Cunha, C., Stevenson, P., and Anderson, J. T. (2015). Overfishing disrupts an ancient mutualism between frugivorous fishes and plants in Neotropical wetlands. Biol. Conserv. 191, 159–167. doi:10.1016/j.biocon.2015.06.019
Correa, S. B., Oliveira, P. C., Nunes da Cunha, C., Penha, J., and Anderson, J. T. (2018). Water and fish select for fleshy fruits in tropical wetland forests. Biotropica 50, 312–318. doi:10.1111/btp.12524
Correa, S. B., Winemiller, K. O., López-Fernández, H., and Galetti, M. (2007). Evolutionary perspectives on seed consumption and dispersal by fishes. BioScience 57, 748–756. doi:10.1641/B570907
Correa, S. B., and Winemiller, K. O. (2014). Niche partitioning among frugivorous fishes in response to fluctuating resources in the Amazonian floodplain forest. Ecology 95, 210–224. doi:10.1890/13-0393.1
Costa, R., and Mateus, L. A. F. (2009). Reproductive biology of pacu Piaractus mesopotamicus (holmberg, 1887) (teleostei: Characidae) in the Cuiabá River basin, Mato Grosso, Brazil. Neotrop. Ichthyol. 7, 447–458. doi:10.1590/S1679-62252009000300012
Costa-Pereira, R., Severo-Neto, F., Yule, T. S., and Pereira, A. P. T. (2011). Fruit-eating fishes of Banara arguta (salicaceae) in the miranda river floodplain, pantanal wetland. Biota Neotrop. 11, 373–376. doi:10.1590/S1676-06032011000400033
Cristescu, B., and Boyce, M. S. (2013). Focusing ecological research for conservation. Ambio 42, 805–815. doi:10.1007/s13280-013-0410-x
Davis, A. M., Pusey, B. J., Thorburn, D. C., Dowe, J. L., Morgan, D. L., and Burrows, D. (2010). Riparian contributions to the diet of terapontid grunters (Pisces: Terapontidae) in wet-dry tropical rivers. J. Fish. Biol. 76, 862–879. doi:10.1111/j.1095-8649.2010.02541.x
Donatti, C. I., Guimarães, P. R., Galetti, M., Pizo, M. A., Marquitti, F. M. D., and Dirzo, R. (2011). Analysis of a hyper-diverse seed dispersal network: Modularity and underlying mechanisms. Ecol. Lett. 14, 773–781. doi:10.1111/j.1461-0248.2011.01639.x
Dormann, C. F., Gruber, B., and Fruend, J. (2008). Introducing the bipartite package: Analysing ecological networks. R. news 8, 8–11.
Dormann, C. F., Gruber, B., and Fruend, J. (2013). Package “bipartite”. R package version. Available online at: http://CRAN.R-project.org/package=bipartite.
Dormann, C., Fründ, J., Blüthgen, N., and Gruber, B. (2009). Indices, graphs and null models: Analyzing bipartite ecological networks. Open Ecol. J. 2, 7–24. doi:10.2174/1874213000902010007
Drewe, K. E., Horn, M. H., Dickson, K. A., and Gawlicka, A. (2004). Insectivore to frugivore: Ontogenetic changes in gut morphology and digestive enzyme activity in the characid fish Brycon guatemalensis from Costa Rican rain forest streams. J. Fish Biol. 64, 890–902. doi:10.1111/j.1095-8649.2004.0357.x
Esteves, K. E., and Aranha, J. M. R. (1999). Ecologia trófica de Peixes de Riachos. Oecol. Austr. 6, 157–182. doi:10.4257/oeco.1999.0601.05
Fantin-Cruz, I., Pedrollo, O., Castro, N. M. R., Girard, P., Zeilhofer, P., and Hamilton, S. K. (2011). Historical reconstruction of floodplain inundation in the Pantanal (Brazil) using neural networks. J. Hydrology 399, 376–384. doi:10.1016/j.jhydrol.2011.01.014
Ferraz de Lima, J. A., and Chabalin, E. (1981). A pesca no pantanal de Mato Grosso (rio Cuiabá: Biologia e ecologia pesqueira). Recife: Anais II Congresso Brasileiro de Engenharia de Pesca.
Ferreira, A., Paula, F. R., Barros Ferraz, S. F., Gerhard, P., Kashiwaqui, E. A. L., Cyrino, J. E. P., et al. (2012). Riparian coverage affects diets of characids in neotropical streams. Ecol. Freshw. Fish. 21, 12–22. doi:10.1111/j.1600-0633.2011.00518.x
Figueiredo, D. M., and Salomão, F. X. T. (2009). Bacia do rio Cuiabá: uma abordagem socioambiental. Cuiabá: Entrelinhas/Editora da Universidade Federal de Mato Grosso.
Freitas, T., Almeida, V., Valente, R. d. M., and Montag, L. F. d. A. (2011). Feeding ecology of Auchenipterichthys longimanus (siluriformes: Auchenipteridae) in a riparian flooded forest of eastern amazonia, Brazil. Neotrop. Ichthyol. 9, 629–636. doi:10.1590/S1679-62252011005000032
Galetti, M., Donatti, C., Pizo, M., and Giacomini, H. (2008). Big fish are the best: Seed dispersal of Bactris glaucescens by the pacu fish (Piaractus mesopotamicus) in the pantanal, Brazil. Biotropica 40, 386–389. doi:10.1111/j.1744-7429.2007.00378.x
Goulding, M., Carvalho, M. L., and Ferreira, E. G. (1988). Rio Negro, rich life in poor water: Amazonian diversity and foodchain ecology as seen through fish communities. The Hague, Netherlands: SPB Academic Publishing.
Goulding, M. (1980). The fishes and the forest: Explorations in amazonian natural. History. Berkeley: University of California Press.
Hagen, M., Kissling, D., Rasmussen, C., Aguiar, M. A., Brown, L. E., Carstensen, D., et al. (2012). Biodiversity, species interactions and ecological networks in a fragmented world. Adv. Ecol. Res. 46, 89–210. doi:10.1016/B978-0-12-396992-7.00002-2
Hahn, N. S., Andrian, I. F., Fugi, R., and Almeida, V. L. L. (1997). “Ecologia trófica,” in A planície de inundação do alto rio Paraná: Aspectos físicos, biológicos e socioeconômicos. Editors A. E. A. M. Vazzoler, A. A. Agostinho, and N. S. Hahn (Maringá: Editora da Universidade Estadual de Maringá EDUEM), 209–228.
Helfield, J. M., and Naiman, R. J. (2006). Keystone interactions: Salmon and bear in riparian forests of Alaska. Ecosystems 9, 167–180. doi:10.1007/s10021-004-0063-5
Higgins, J. (2004). Introduction to modern nonparametric statistics. Belmont: Brooks⁄Cole Cengage Learning.
Hilderbrand, G., Hanley, T., Robbins, C., and Schwartz, C. (1999). Role of Brown bears (Ursus arctos) in the flow of marine nitrogen into a terrestrial ecosystem. Oecologia 121, 546–550. doi:10.1007/s004420050961
Horn, M. H. (1997). Evidence for dispersal of fig seeds by the fruit-eating characid fish Brycon guatemalensis Regan in a Costa Rican tropical rain forest. Oecologia 109, 259–264. doi:10.1007/s004420050081
Horn, M. H., Correa, S. B., Parolin, P., Pollux, B. J. A., Anderson, J. T., Lucas, C., et al. (2011). Seed dispersal by fishes in tropical and temperate fresh waters: The growing evidence. Acta Oecol. 37, 561–577. doi:10.1016/j.actao.2011.06.004
Izquierdo, M. S., Fernández-Palacios, H., and Tacon, A. G. J. (2001). Effect of brood stock nutrition on reproductive performance of fish. Aquaculture 197, 25–42. doi:10.1016/S0044-8486(01)00581-6
Junk, W. J., Bayley, P. B., and Sparks, R. E. (1989). The flood pulse concept in river-floodplain systems. Can. Special Publ. Fish. Aquatic Sci. 106, 110–127.
Junk, W. J., Soares, M. G. M., and Saint-Paul, U. (1997). “The fish,” in The central Amazon floodplain, ecology of a pulsing system. Editor W. J. Junk (Berlin, Germany: Springer-Verlag), 85–408.
Junk, W. J., and Wantzen, K. M. (2004). “The Flood Pulse Concept: New aspect, approaches and application- an update,” in Proceedings of the second international symposium on the management of large rivers for fisheries. Editors R. L. Welcomme, and A. T. Petr (Bangkok: Food and Agriculture Organization and Mekong River Commission, FAO Regional Office for Asia and the Pacific), 117–132.
Kubitzki, K., and Ziburski, A. (1994). Seed dispersal in flood plain forests of Amazonia. Biotropica 26, 30–43. doi:10.2307/2389108
Larsen, S., Karaus, U., Claret, C., Sporka, F., Hamerlı´k, L., and Tockner, K. (2019). Flooding and hydrologic connectivity modulate Community assembly in a dynamic river-floodplain ecosystem. PLoS ONE 14, e0213227. doi:10.1371/journal.pone.0213227
Li, P., Mai, K., Trushenski, J., and Wu, G. (2009). New developments in fish amino acid nutrition: Towards functional and environmentally oriented aquafeeds. Amino Acids 37, 43–53. doi:10.1007/s00726-008-0171-1
Lowe-Mcconnell, R. H. (1999). Estudos ecológicos de Comunidades de Peixes tropicais. São Paulo: Edusp.
Lucas, C. M. (2008). Within flood season variation in fruit consumption and seed dispersal by two characin fishes of the Amazon. Biotropica 40, 581–589. doi:10.1111/j.1744-7429.2008.00415.x
Makrakis, M. C., Miranda, L. E., Makrakis, S., Xavier, A. M. M., Fontes, H. M., and Morlis, W. G. (2007). Migratory movements of pacu, Piaractus mesopotamicus, in the highly impounded Paraná River. J. Appl. Ichthyol. 23, 700–704. doi:10.1111/j.1439-0426.2007.01006.x
Masese, F. O., Abrantes, K. G., Gettel, G. M., Bouillon, S., Irvine, K., and Mcclain, M. E. (2015). Are large herbivores vectors of terrestrial subsidies for riverine food webs? Ecosystems 18, 686–706. doi:10.1007/s10021-015-9859-8
Masese, F. O., Abrantes, K. G., Gettel, G. M., Irvine, K., Bouillon, S., and McClain, M. E. (2018). Trophic structure of an african savanna river and organic matter inputs by large terrestrial herbivores: A stable isotope approach. Freshw. Biol. 63, 1365–1380. doi:10.1111/fwb.13163
Massaroli, B. A. R., Araújo, J. M., Ortega, J. C., Nunes, A, V., Mateus, L., Silva, S. E., et al. (2021). Temporal dynamic and economic valuation of recreational fisheries of the lower Cuiabá River, Brazilian Pantanal. Fish. Manag. Ecol. 28, 328–337. doi:10.1111/fme.12479
Mateus, L. A. F., Vaz, M., and Catella, A. A. (2011). in Fishery and fishing resources in the pantanal”, inThe pantanal: Ecology and sustainable management of a large neotropical seasonal wetland. Editors W. J. Junk, C. J. Da Silva, C. Nunes, and Wantzen (Sofia-Moscow: Pensoft Publishers), 621–647.
Melo, T., Torrente-Vilara, G., and Röpke, C. P. (2019). Flipped reducetarianism: A vegan fish subordinated to carnivory by suppression of the flooded forest in the Amazon. For. Ecol. Manag. 435, 138–143. doi:10.1016/j.foreco.2018.12.050
Memmott, J., Waser, N. M., and Price, M. V. (2004). Tolerance of pollination networks to species extinctions. Proc. R. Soc. Lond. B Biol. Sci. 271, 2605–2611. doi:10.1098/rspb.2004.2909
Merona, B. (1987). Aspectos ecológicos da ictiofauna no Baixo Tocantins. Acta Amaz. 17, 109–160. doi:10.1590/1809-43921987171124
Nakano, S., and Murakami, M. (2001). Reciprocal subsidies: Dynamic interdependence between terrestrial and aquatic food webs. Proc. Natl. Acad. Sci. U. S. A. 98, 166–170. doi:10.1073/pnas.98.1.166
Pires, M. M., Guimarães, P. R., Araújo, M. S., Giaretta, A. A., Costa, J. C. L., and dos Reis, S. F. (2011). The nested assembly of individual-resource networks. J. Anim. Ecol. 80, 896–903. doi:10.1111/j.1365-2656.2011.01818.x
Polis, G. A., Anderson, W. B., and Holt, R. D. (1997). Toward an integration of landscape and food web ecology: The Dynamics of Spatially Subsidized Food Webs. Annu. Rev. Ecol. Syst. 28, 289–316. doi:10.1146/annurev.ecolsys.28.1.289
G. A. Polis, M. E. Power, and G. R. Huxel (Editors) (2004). Food webs at the landscape level (Chicago: University of Chicago Press).
Pollux, B. J. A. (2011). The experimental study of seed dispersal by fish (ichthyochory). Freshw. Biol. 56, 197–212. doi:10.1111/j.1365-2427.2010.02493.x
Pringle, R. M. (2017). How large herbivores subsidize aquatic food webs in African savannas. Proc. Natl. Acad. Sci. U. S. A. 18, 7489–7491. doi:10.1073/pnas.1708571114
Pusey, B., and Arthington, A. (2003). Importance of the riparian zone to the conservation and management of freshwater fish: A review. Mar. Freshw. Res. 54, 1–16. doi:10.1071/MF02041
R Core Team (2020). R: A language and environment for statistical computing. Vienna: R Foundation for Statistical Computing. Available online at: http://www.R-project.org/.
Rauber, R. G., Strictar, L., Gomes, L. C., Suzuki, H. I., and Agostinho, A. A. (2021). Spatial segregation in the reproductive activity of Neotropical fish species as an indicator of the migratory trait. J. Fish. Biol. 98, 694–706. doi:10.1111/jfb.14614
Reis, R. E., Kullander, S. O., and Ferraris, C. J. J. (2003). Check list of the freshwater fishes of south and Central America. Porto Alegre: Editora da Pontifícia Universidade Católica do Rio Grande do Sul.
Reys, P., Sabino, J., and Galetti, M. (2009). Frugivory by the fish Brycon hilarii (characidae) in Western Brazil. Acta Oecol. 35, 136–141. doi:10.1016/j.actao.2008.09.007
Romagosa, E., Paiva, P., Godinho, H. M., and Storfer, E. B. (1988). Desenvolvimento dos ovócitos de Piractus mesopotamicus (Holmberg, 1887) (= Colossoma mitrei Berg, 1895) em condições de cultivo intensivo. Ciência Cult. 40, 60–64.
Rosas-Ribeiro, P. F., Rosas, F. C. W., and Zuanon, J. (2012). Conflict between fishermen and giant otters Pteronura brasiliensis in western Brazilian Amazon. Biotropica 44, 437–444. doi:10.1111/j.1744-7429.2011.00828.x
Sado, R., Souza, F. C., Behr, E. R., Mocha, P., and Baldisserotto, B. (2020). “Anatomy of teleosts and elasmobranchs,” in Biology and physiology of freshwater neotropical fish. Editors B. Baldisserotto, E. C. Urbinati, and J. E. P Cyrino (Cambridge, Massachusetts, United States: Academic Press), 21–47. doi:10.1016/B978-0-12-815872-2.00002-6
Santos, S. A., Nogueira, M. S., Pinheiro, M. S., Campos, Z., Magnusson, W. E., and Mourão, G. M. (1996). Diets of Caiman crocodilus yacare from different habitats in the Brazilian Pantanal. Herpetological J. 6, 111–117.
Urbinati, E. C., Gonçalves, F. D., and Takahashi, L. S. (2010). “Pacu (Piaractus mesopotamicus),” in Espécies nativas para piscicultura no Brasil. Editors B. Baldisserotto, and L. C. Gomes (Santa Maria: UFSM), 205–244.
Wantzen, K. M. (2006). Physical pollution: Effects of gully erosion on benthic invertebrates in a tropical clear-water stream. Aquat. Conserv. 16, 733–749. doi:10.1002/aqc.813
Wheeler, B. (2010). lmPerm: Permutation tests for linear models. R package version 1.1-2. Available online at: http://CRAN.R-project.org/package=lmPerm.
Keywords: Piaractus mesopotamicus, frugivory, pantanal, wetlands, bipartite network, floodplain
Citation: Mateus L, Rodrigues Costa RM and Penha J (2022) Spatiotemporal diet shifting in a neotropical fish species: The role of riparian vegetation and seasonal inundation. Front. Environ. Sci. 10:883298. doi: 10.3389/fenvs.2022.883298
Received: 24 February 2022; Accepted: 20 September 2022;
Published: 04 October 2022.
Edited by:
Angela Helen Arthington, Griffith University, AustraliaReviewed by:
Frank Onderi Masese, University of Eldoret, KenyaRaphael Ligeiro, Federal University of Pará, Brazil
André Guaraldo, Federal University of Paraná, Brazil
Copyright © 2022 Mateus, Rodrigues Costa and Penha. This is an open-access article distributed under the terms of the Creative Commons Attribution License (CC BY). The use, distribution or reproduction in other forums is permitted, provided the original author(s) and the copyright owner(s) are credited and that the original publication in this journal is cited, in accordance with accepted academic practice. No use, distribution or reproduction is permitted which does not comply with these terms.
*Correspondence: Lucia Mateus, bGFmbWF0ZXVzQGdtYWlsLmNvbQ==