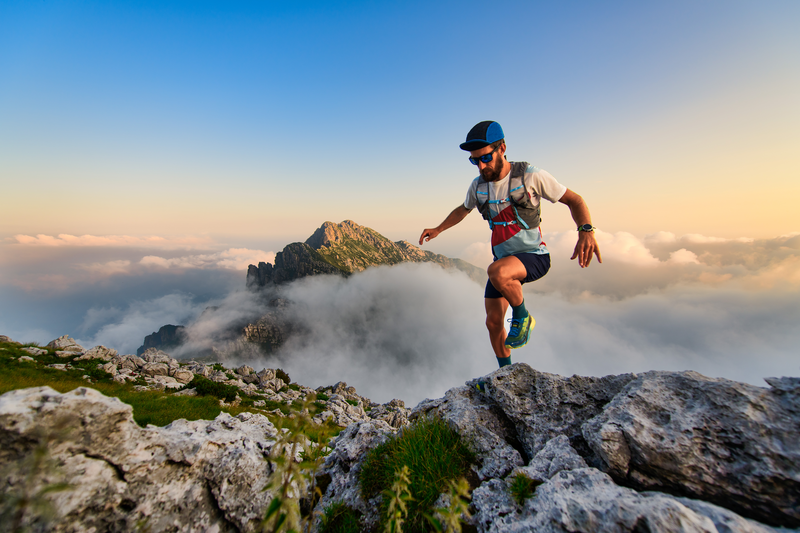
95% of researchers rate our articles as excellent or good
Learn more about the work of our research integrity team to safeguard the quality of each article we publish.
Find out more
ORIGINAL RESEARCH article
Front. Environ. Sci. , 10 May 2022
Sec. Water and Wastewater Management
Volume 10 - 2022 | https://doi.org/10.3389/fenvs.2022.882284
This article is part of the Research Topic Tackling the Presence of Pathogens in Water and Wastewater View all 6 articles
Human urine can be used as fertilizer and technologies, such as alkaline-urine treatment, are being developed to enable easier re-use. There is, however, a risk of pathogens being present in the urine. This hygiene assessment examined inactivation of three model organisms, one parasite (Ascaris suum) and two viruses (coliphages MS2 and ΦX174), during 1) alkaline-urine treatment and 2) drying of the alkalized-urine (A. suum only). Fresh human urine was mixed with calcium hydroxide (10 g Ca(OH)2 L−1 urine) and divided into three fractions (Mixed liquor, Supernate, Precipitates). The factions were inoculated with the model organisms and then subjected to three treatments (Drying-storage, Stored and Thermal treatment) at temperatures between 20 and 50°C. For Ascaris, drying (moisture content (MC) 13–33%) the alkaline-urine proved effective in shortening the time required for a 3 log10 reduction in viable eggs at 20°C, but only Partially drying (MC 73–82%) the urine led to longer inactivation times compared with Wet (MC >90%) or Dry conditions. While virus inactivation took place during the initial addition of Ca(OH)2, the viruses that were embedded in feces survived longer compared to the free viruses. At pH 11.5, contact times of 1.5 and 90.7 min were required to achieve a 4 log10 decay of phages in solution and phages embedded in feces respectively. In areas prone to parasites, Thermal treatment (≥42°C) and/or Storage (111 days at 20°C or 79 days at 35°C) is recommended in order to meet the WHO and USEPA guidelines for unrestricted fertilizer use. Drying (MC 73–82%) can also be used in combination with thermal treatment and/or storage, to accelerate the process.
• In areas prone to parasites, a post-urine-drying-treatment is recommended
• Partial drying led to longer inactivation times for Ascaris compared to dried
• Virus inactivation took place mainly during the initial addition of Ca(OH)2
• Viruses embedded in feces survived longer than free viruses in alkaline solutions
Urine contains the majority of plant-available nutrients found in household wastewater (Jönsson et al., 2005) and is a contributor to eutrophication and hypoxia in aquatic environments (Smil 2002; Spångberg et al., 2014; Steffen et al., 2015; Larsen 2020). As alternative management of these nutrients, urine can be collected, stored and applied as a fertilizer (Harder et al., 2019). However, this poses a logistical challenge, with approximately 350–1000 l urine being produced per person per year (Vinnerås et al., 2006). Storing the urine until application as a fertilizer requires either a large storage tank or frequent emptying to a central storage unit, as fertilizers are generally only applied once or twice per growing season. A solution to this is to concentrate the urine, which is >95% water. Various techniques for concentrating or extracting the nutrients in urine are being developed (Maurer et al., 2006; Antonini et al., 2012; Senecal and Vinnerås 2017; Randall and Naidoo 2018; Volpin et al., 2018; Martin 2020).
When recirculating the nutrients in the urine, there is also a risk of recirculating pathogens. Only a few pathogens are excreted via the urine, compared with feces (Aw 2018). However, there is a risk of cross-contamination from the feces to the urine during excretion and collection (Höglund et al., 2000; Bischel et al., 2015). Feces can potentially contain many pathogenic viruses, bacteria, protozoa, and helminths, many of which are immediately infectious upon excretion (Aw 2018). Cross-contamination of 9.1 mg of feces per liter of urine has been estimated for urine-diverting toilets (Schönning et al., 2002) (no newer data was found). Due to the cross-contamination with feces, there is a potential health risk for the people handling and/or using diverted urine as a fertilizer (WHO 2006; Bischel et al., 2019) hence the importance of hygiene evaluations of emerging technologies.
One method being developed to concentrate urine is the use of alkalizing media to raise the urine’s pH and block the biochemical degradation of urea, which then enables dehydration with minimal loss of nitrogen (N) (Dutta and Vinnerås 2016; Randall et al., 2016; Senecal and Vinnerås 2017; Chipako and Randall 2020). (Randall et al., 2016 #371@@author-year) recommends 10 g Ca(OH)2 L−1 of fresh urine to ensure that the urine is always saturated with calcium hydroxide keeping the pH > 12, which prevents biochemical hydroxylation of urea to ammonia (Randall et al., 2016; Geinzer 2017). The pH must remain >11 to minimize urease enzyme activity and temperature during evaporation shall not exceed 60°C to minimize thermal degradation of urea (Randall et al., 2016 #371).
Helminths have shown to be highly resistant to various chemical treatments (Ghiglietti et al., 1997; Nordin et al., 2009; Naidoo et al., 2016; Senecal et al., 2018; Senecal et al., 2020) and physical treatments (Decrey et al., 2011). Other pathogenic organisms, such as viruses, are more sensitive to high pH (Nyberg et al., 2011; Decrey et al., 2016; Senecal et al., 2018), however they are secreted in greater number. Thus in the present study, the inactivation of one helminth, Ascaris suum, and two virus surrogates, bacteriophage ΦX174 and MS2, were used to assess the effect of the alkaline pre-treatment and (for helminth only) drying of alkalized urine.
Ascaris eggs were used as a conservative model organism, especially during chemical treatment, to assess the efficiency of treatment, as they are one of the most resilient pathogens excreted (Feachem et al., 1983; Jiménez 2006; Pecson et al., 2007; Maya et al., 2010). Bacteriophage ΦX174 (ssDNA virus) and MS2 (ssRNA) were used as conservative surrogates of human and animal viruses in general (Emmoth et al., 2011; Magri et al., 2015; Decrey et al., 2016; Decrey and Kohn 2017).
The overall aim of the study was to determine inactivation rates in relation to composition of sample, treatment temperature, and dry matter content in order to provide hygienic handling recommendations for the urine/Ca(OH)2 solution to produce a urine-based fertilizer that meets the existing guidelines WHO (2006) and USEPA (1994) for unrestricted fertilizer use.
Ascaris eggs and viruses exhibit different sensitivity to the physical-chemical treatment conditions expected in alkaline-urine treatment. Especially, Ascaris eggs’ survival is more affected by temperature increase and moisture reduction whereas virus fate is mainly driven by pH increase. For viruses, the main driver of inactivation in alkaline-urine treatment is high pH, rather than moisture reduction (Decrey et al., 2011; Decrey et al., 2016). In this study, virus inactivation rates were observed in liquids with different alkaline pH at ambient temperature (with and without enmeshment in fecal material), while Ascaris inactivation rates were observed in both liquid and dried urine (all eggs were enmeshed in fecal material). Thus, the following experimental methods differ between Ascaris and the bacteriophages in order to focus on the most relevant parameters to each given organism.
For study of inactivation of Ascaris, >10 urine samples were collected by anonymous donations during 1 week in 1-L sterile containers at the departments toilet and stored at 4°C until use. Human feces (in total 300 g) were collected fresh from two people anonymously at the department toilets and stored frozen until use.
For studies of bacteriophage inactivation, fresh feces were obtained from a male adult and stored at 4°C less than 1 week before experiment. Prior to experiment, the feces were tested and no coliphages detected (detection limit: 100 PFU ml−1).
Ascaris suum eggs were retrieved by sieving feces from inoculated swine (Excelsior Sentinel, Inc., United States) and stored at 4°C in diluted formalin solution during transportation and storage prior to use. Coliphages MS2 (DSMZ 13767) and ΦX174 (DSMZ 4497), and their host Escherichia coli (DSMZ 5695 and DSMZ 13127, respectively) were purchased from the German Collection of Microorganisms and Cell Cultures (DSMZ, Braunschweig, Germany). Phages were propagated and purified as described by Pecson et al. (2009). Sodium carbonate (Na2CO3; Fluka), ammonium chloride (NH4Cl; Acros), sodium chloride (NaCl; Acros), and sodium phosphate (NaH2PO4; Acros) were used to make the experimental stock solutions. Stock solutions were kept in virus dilution buffer (VDB; 5 mmolL−1 NaH2PO4, 10 mmolL−1 NaCl, pH 7.5), and were stored at 4°C. The same stocks were used for all the virus experiments.
The inactivation of Ascaris eggs was assessed in three fractions of urine/Ca(OH)2 solution (Supernatant, Mixed liquor and Precipitates—Figure 1) and in three solution treatments: 1) drying at 20°C with storage at 20 and 35°C in sealed containers; 2) storage (no drying) in sealed containers at 20 and 35°C; and 3) thermal treatment in sealed containers at 42 and 50°C (Table 1 and 2).
FIGURE 1. The three fractions made from the stabilized urine/Ca(OH)2 solution. Mixed liquor being the whole agitated mixture. The Supernate and Precipitate fractions were made by decanting the solution after settling for 3 h.
TABLE 1. Three solution treatments (Drying and Storage, Storage and Thermal treatment) distributed across temperature and moisture content and the different fractions (described in Figure 1).
TABLE 2. Three solution treatments (Drying and Storage, Storage and Thermal treatment) were applied to the three urine/Ca(OH)2 fractions (Mixed liquor, Supernate, and Precipitate) in which inactivation of Ascaris was assessed. Wet conditions had >90% moisture content (MC). Partially wet (P. wet) conditions had 73–82% MC (no free liquid on the surface); and Dried conditions had 13–33% MC. During drying, the containers were open. During storage, the containers were sealed.
The collected urine (>10 L) was pooled and calcium hydroxide (Ca(OH)2; VWR Chemicals, Pennsylvania, United States) was added at a ratio of 10 g Ca(OH)2 per liter urine to achieve pH 12.5. The mixture was agitated for 10 min by magnetic stirrer and then left to stand undisturbed in closed containers for 3 h at 20°C to form what is referred to as stabilized urine.
Urine Fractions
From the stabilized urine/Ca(OH)2 solution, three fractions were formed (Figure 1). The fraction referred to as Mixed liquor was the whole agitated mixture treated and dried together. The Supernate and Precipitate fractions were made by decanting the solution after standing for 3 h. The Supernate represented the situation where after Ca(OH)2 stabilization the top liquid would be pumped out of the reactor for drying, while the precipitates would be removed and dried separately. All three fractions were stored in sealed containers overnight.
The inactivation of Ascaris eggs in the three fractions was assessed by three solution treatments, as described in Table 1 and 2. The volumes of the urine fractions for each treatment are described in Table 3. All treatments had an addition of 0.1 g feces with Ascaris eggs (100 000 eggs per gram epg).
TABLE 3. Amounts of urine/Ca(OH)2 used from the three fractions (Supernate, mixed liquor, and precipitates) and procedure applied to the urine-feces mix to reach the target moisture content (MC) for the treatment.
Solution Treatments
Drying-storage: The effect of drying prior to storage was investigated by drying the three urine/Ca(OH)2 fractions (Mixed liquor, Supernate, and Precipitate) at 20°C to either a Partially wet condition (73–82% moisture content (MC)) or a Dried condition (13–33% MC) followed by storage in sealed containers at 20 or 35°C.
Stored: Urine/Ca(OH)2 fractions were stored directly in sealed containers at 20 and 35°C (>90% MC).
Thermal Treatment: The third aspect investigated was effect of elevated temperature (42 and 50°C) during storage of the Supernate fraction to assess the thermal pre-treatment time required to achieve a 3 log10 reduction in Ascaris egg viability.
Wet Conditions: For setting up the studies of the three urine/Ca(OH)2 fractions under wet conditions (>90% MC), 6 ml from each the three different fractions were aliquoted into 7 ml polypropylene tubes with O-ring lined screw caps (Sarstedt AG & Co., Sweden) already containing 0.1 g of Ascaris eggs in feces. After sealing, the tubes were vortexed for 5 s. The capped tubes were then placed at the target temperatures (in incubators or in an insulated box at room temperature) (Table 2).
Partially Wet Conditions: urine/Ca(OH)2 solution of the three different fractions were divided into 45 ml aliquots, which were transferred to 50 ml centrifuge tubes already containing 0.1 g fecal material and vortexed for 3 s (or to <1 mm lumps). The tubes were then centrifuged for 5 min at 3000 rpm to bring particles, including Ascaris eggs, down from the walls. The tubes were placed without lids on a table and dried with a fan at 20°C until no liquid was visible (73–82% MC) and then the tubes were sealed and stored at target temperature.
Dried conditions: 150 ml of the urine/Ca(OH)2 solution from the Supernate and Mixed liquor fractions and 45 ml of the precipitates fraction were added to 0.1 g feces with Ascaris eggs (the addition of fecal material was made according to the total solids content). After vortexing for 3 s, the fractions were transferred to open foil trays lined with waxed paper, which were kept on a table and dried with a fan at room temperature to the target moisture content of <33%. The dried material was transferred to 7 ml tubes, which were sealed and stored at the target temperature.
The duration of the drying period varied from 2 to 8 days (Table 1) and the time was included in the duration of the treatment. The variation in drying period was due to the tubes from the Partially wet treatment being dried in smaller containers than the open trays in the Dried treatment. The study was conducted as independent singlets using destructive sampling.
Extraction of Eggs
The extraction procedure for the A. suum eggs was a modification of the USEPA (2003) method. To extract the eggs from crystals formed in the three fractions, diluted 0.1 N sulphuric acid was added to the vials and they were vortexed for 30 s and then centrifuged at 4000 rpm for 3 min. The Supernate was decanted over Tyler sieves (⌀ 38 μm, Cat. No. L3-400, and ⌀ 300 μm, Cat. No. L3-50). This was repeated 5–10 times, until the crystals formed by the urine and the Ca(OH)2 were nearly dissolved. Any remaining crystals were decanted over Tyler sieves and further washed with 0.1 N sulphuric acid. Sampling frequency depended on the intensity of the treatment and ranged from every 2 weeks at 20°C to every 8 h at 50 °C.
Experiments were conducted in the presence and absence of feces to assess if fecal matter exerts a protective effect of viruses during alkaline conditions (pH 9, 11.5 and 12.3). Additional control experiment with virus in feces exposed to virus dilution buffer (VDB; 5 mmol L−1 NaH2PO4, 10 mmol L−1 NaCl, pH 7.5) were also performed to examine the effect of feces itself on virus survival. Each experimental condition was tested in independent singlet experiments.
Experiments at pH 11.5 and 12.3 were conducted in phosphate buffer. Experiments at pH were conducted in ammonium carbonate buffer. The specific composition of the buffers to attain a given pH was determined with PHREEQC (version 2.18.00) (Parkhurst and Appelo 2013) and a database using the Pitzer approach for calculating the ion activity. Solutions were made according to SI, Supplamentary Table S1. by mixing salt with sterilized milliQ water, stored at targeted temperature for at least half day, and the solution pH was then measured and adjusted with NaOH/HCl. The pH and electrical conductivity (EC) were measured at experimental temperature with 780 pH Meter with primatrode with NTC no. 6.0228.010 (Metrohm, Herisau, Switzerland) and a Cond315i conductivity meter and a TetraCon 325 probe (WTW, Weilheim, Germany) respectively.
ΦX174 was used as the test organism at pH 11.5 and 12.3, because MS2 inactivation was too rapid to be measured. Conversely, MS2 were used as the test organism at pH 9.0, because ΦX174 did not exhibit measurable inactivation over the timescale considered.
A day prior to the start of the experiment, 50 µL of ∼109 PFU ml−1 virus solution were spiked into ∼5 g of feces in a plastic bag, kneaded for a few minutes, and stored overnight at 4°C. The following day, 0.05–0.2 g of feces were transferred into 1 ml eppendorf tubes. The tubes were then filled with either 1 ml VDB (control sample at circum-neutral pH), 1 ml of ammonium carbonate buffer, 0.9 ml of phosphate buffer at pH 11.5, or 0.8 ml of phosphate buffer at pH 12.3. Sacrificial samples were taken periodically and were mixed with 0.1 and 0.2 ml HCl 1N for phosphate buffer at pH 11.5 and 12.3 respectively to lower the pH to a range between 6.5–7.5. They were then vortexed for 5 min, centrifuged at 10000 g for 1 min, filtered through a 0.22 μm filter (Millipore), diluted in VDB and stored at 4°C for no more than 6 h prior to enumeration. In control samples with VDB, this method showed a 50% recovery of phages.
One milliliter of a virus solution containing 109 PFU ml−1 in VDB was added to airtight 116 ml glass serum flasks (Infochroma) containing 114 ml of experimental solution (as described in Section 2.2). After mixing, 1 ml of ammonium carbonate buffer, 0.9 ml of phosphate buffer at pH 11.5, or 0.8 ml of phosphate buffer at pH 12.3 were taken periodically from each flask with a sterile syringe, mixed in an Eppendorf tube with 0.1 and 0.2 ml HCl 1N for phosphate buffer at pH 11.5 and 12.3 respectively and were filtered through a 0.22 μm filter (Millipore), diluted in VDB and stored at 4°C for no more than 6 h prior to enumeration.
At the start of the experiment, a sample of the A. suum eggs (n = 100) was observed under a microscope to confirm that the eggs were undeveloped. After extraction from the treatments, 100 eggs were directly examined to assess any development or damage occurring during the treatment period. The viability of the eggs was determined by incubating them at 28°C in 0.1 N H2SO4 for 28 days to allow larval development (Arene, 1986). Viability counts were performed under × 10 and × 20 magnification and unfertilized eggs, which constituted a minor proportion of all eggs, were excluded from further counting. Eggs developing to the larval stage were considered viable. Initial viability of the A. suum eggs was 55% in the treatment applying storage temperatures of 20 and 35°C, and 77% in the treatment applying thermal treatment at 42 and 50°C.
Infectivity was assessed using the double agar layer method and was expressed as plaque forming units per mL (PFU ml−1) (Standard Methods 2018). The quantification limit for all phages was 100 PFU ml−1. Number of phages in feces (PFU mg−1) was calculated from the phages in solution PFU mL−1 extracted from feces, multiplied by the total amount of feces in mg mL−1. Natural presence of MS2 and ΦX174 used in this study in feces was assessed to be under the fore-mentioned detection limit.
Confidence intervals for proportions of viable Ascaris eggs were derived using the Wilson score interval (Minitab Inc., US). The logarithmized egg viability data, which showed a two-phase inactivation pattern, were fitted against a non-linear inactivation model (Eq. 1) from (Harm 1980). For the treatments that were repeated, the viability data were modelled as a combined dataset:
where log10NtAscaris is the log(base 10) proportion of viable eggs at time t (in days), log10N0Ascaris is the log proportion of viable eggs at start, kAscaris is the inactivation rate constant, which describes the change in viability over time during the exponential decay phase (log10 proportion viable eggs day−1), and n is a dimensionless parameter determining lag phase duration. The values of kAscaris and n were determined by non-linear regression using the Gauss-Newton algorithm (Minitab 17, Minitab Inc., US). This enabled calculation of the lag phase, i.e., the initial period where there is no significant inactivation (Eq. 2):
Many samples had no lag phase according to this model. For these datasets, the model gave n = 1, which reduced the model to a linear regression with the y-intercept set to zero, i.e., exponential decay.
Virus inactivation was modelled as a log-linear decay process according to Eq. 3:
Where Nvirus and N0,virus are the concentration of infectious viruses at times 0 and t, and kvirus is the first-order inactivation rate constant (day−1). The linear regressions were performed on log10 transformed data with Excel software. The 95% confidence interval (95% CI) of kvirus was calculated from the standard error of the slope from one experiment.
The Ascaris eggs showed both biphasic inactivation with an initial lag phase and log-linear inactivation. In the Drying-storage treatment at 20°C, decreasing the moisture content, from 78 to 82% in the Partially wet condition to 13–16% in the Dried condition, decreased the time required for a 3 log10 reduction by 22 days (Table 4). The Supernate (98 days) and Mixed liquor (102 days) fractions that were stored directly at 20°C (Wet condition) required similar times for a 3 log10 reduction to the Partially wet condition (Supernate, 111 days; Mixed liquor, 95 days). The Precipitates fraction stored directly at 20°C (Wet condition) required a similar time (60 days) for a 3 log10 reduction to that in the Dried condition.
TABLE 4. Ascaris egg inactivation parameters: lag phase (days); inactivation rate constant k (-log10 d−1); time required for a 3 log10 reduction in egg viability (days), derived from Eq. 1, 2, for Ascaris eggs subjected to the different drying, storage and thermal treatments. Wet conditions had >90% MC, Partially wet (P. wet) condition had 73–82% MC (no free liquid on the surface); and Dried conditions had 13–33% MC.
In the Drying-storage treatment at 35°C, the Supernate (12–16 days) and Mixed liquor (18–23 days) fractions were quicker to reach a 3 log10 reduction than in Drying-storage at 20°C. The Precipitates at 35°C were slower to reach a 3 log10 reduction than the other two fractions (59–79 days depending on the moisture content). The Supernate (15 days) and Precipitates (53 days) fractions that were stored directly at 35°C (Wet condition) required a similar time for a 3 log10 reduction as Partially wet conditions (Supernate, 16 days; Precipitates, 59 days). The Mixed liquor fraction required twice the time (48 days) in comparison with the partially wet conditions in the drying-storage treatment (23 days).
In the Thermal treatment, the time required for a 3 log10 reduction in egg viability was <5 days at 42°C and <9 h at 50°C. Due to fast inactivation in relation to sampling frequency at 50°C, the inactivation could only be described by a log-linear relationship and the derived k and the time for 3 log10 reduction in egg viability are conservative values (Table 4).
Differences were observed in the inactivation rate constant (k) with the varying parameters. The k value at 20°C was consistent, with a narrow range (−0.083–−0.035 day−1) across the different treatment conditions. At 35°C, the Supernate fraction was more sensitive to the heat increase (k = −0.65− −0.39 day−1) than the Precipitates fraction (k = −0.11−−0.04 day−1) and the Mixed liquor fraction (k = −0.44−−0.06 day−1). The smaller k-values for the Precipitates at 35°C led to longer inactivation time (up to 80 days for a 3 log10 reduction in egg viability, compared with 16 days for the Supernate).
In mildly alkaline conditions (pH 9.0), the k-value for MS2 was similar for viruses in the bulk and embedded in feces. In more alkaline buffer, the k-value for ΦX174 was faster in the bulk than in feces. At pH 11.5, ΦX174 exhibited k-values of 3.4 ± 1.1 days−1 and 9.7 ± 2.2 days−1 in feces and in the bulk respectively. This effect was shown to increase with pH. At pH 12.5, ΦX174 showed k-value of 64 ± 18 days−1 and 3625 ± 2075 days−1in feces and in the bulk respectively. (Figure 2 and see SI, Supplementary Table S2). At pH 11.5, contact times of 9.8 and 28.5 h are required to achieve a 4 log10 decay of phages in solution and embedded in feces respectively. At pH 12.3, these contact times reduce to 1.5 and 90.7 min for phages in solution and phages embedded in feces respectively. Control experiments at pH 7.5 showed no inactivating effect of feces at 20°C over the course of 4 days (see SI, Supplementary Figure S1 and Supplementary Table S2).
FIGURE 2. Comparison of inactivation rate constant (kvirus) at 20°C measured within the feces (orange bar) or in a homogenous solution (white bar) of corresponding pH, for MS2 (pH 9.0) and ΦX174 (pH 11.5 and 12.3). Error bars depict the 95% CI determined from the fit of the data to Eq. 3. Inactivation curves for each experimental condition can be found in SI, Supplementary Figure S1, and values of kvirus are shown in Supplementary Table S2.
Ascaris eggs were inactivated at 20°C, with times ranging from 53 to 111 days for a 3 log10 reduction in egg viability with shorter time required for inactivation in the dry compared to the wet treatment (Table 4). In a related study high pH (12.5) and drying had no effect on the inactivation of Ascaris eggs kept for 80 days at 20°C under similar conditions in terms of pH, moisture, and temperature, but without urine or NH3 (Senecal et al., 2020). Pecson et al., 2007 confirmed that pH alone will not affect Ascaris egg viability at this temperature whereas Naidoo et al., 2016 showed an effect of moisture content alone, with a 1 log10 reduction in Ascaris egg viability after 6 weeks at MC 20%. It is thus likely that presence of NH3 have contributed to the inactivation in the current study, while any carbonate formation would have been negligible (Fidjeland et al., 2016). At 35°C, the elevated pH of 12.3 and temperature were the driving factors for the inactivation of Ascaris eggs, which took between 12 and 79 days for a 3 log10 reduction in egg viability, depending on the fraction and moisture conditions (Senecal et al., 2020). At 35°C, a higher degree of drying had no clear effect on the inactivation rate, an effect that was observed at 20°C. In the related study with similar conditions pH, moisture, and temperature levels, but without urine or NH3, there was a 3 log10 reduction in egg viability within 23 days (Senecal et al., 2020).
The Ascaris inactivation studies could have been strengthened with controls studying the drying media without urine, to assess whether the medium itself had an effect. Formation and accumulation of ammonia could have been monitored in the different fractions and at different moisture conditions to gain a better understanding of the results.
Ascaris eggs require O2 during development (Pawlowski 1982), but are able to remain viable in anaerobic conditions for >80 days (Gaasenbeek and Borgsteede 1998). In the present study, lack of oxygen should not have affected the egg inactivation rates at 20°C, as demonstrated by no reduction in egg viability after 126 days at pH 12.5 in a sealed container (Senecal et al., 2020). However, the lack of O2 could have been a compounding stress factor for the Ascaris eggs at higher temperatures. The increase in osmotic pressure caused by drying the urine was not likely to have causes stress for the Ascaris eggs (Decrey et al., 2011), as Ascaris eggs have been shown to survive in concentrated urine mixed with ash at 20°C for >100 days (Senecal et al., 2018).
The lag phases were longest in the partially wet condition at 20°C, for two probable reasons: 1) this condition had less NH3 than the Wet and Dried condition (took longer to dry, see Table 1 and 2 this condition had no moisture stress compared with the dried condition. This difference in the lag phase duration between the conditions was not observed at 35°C in this study. However, in another study with similar moisture content (60% MC) and temperature, but no NH3 present, inactivation time was longer compared with wet (>90% MC) and dried (<20% MC) conditions (Senecal et al., 2020). This indicates that partially wet conditions may be ideal for the survival of the Ascaris eggs.
The precipitates at 35°C seemed to have a protective effect on the Ascaris eggs, as the time for a 3 log10 reduction was longer for this fraction than for the other fractions at 35°C (Table 4). For the dried precipitate at 35°C, the MC was higher than in other dried conditions (33%, compared with 18% for the dried Supernate and 24% for the dried mixed liquor fraction), which could have caused the smaller k value. Feces has been shown to have a protective effect on Ascaris eggs (Ghiglietti et al., 1997; Capizzi-Banas et al., 2004), and the precipitates fraction may have provided some similar protection.
Traditionally studies on fecal-oral transmitted virus survival are conducted with viruses suspended in a solution of interest. Although a fraction of excreted viruses may indeed end up in the bulk, most of them are encountered within large to very small pieces of fecal material (Schönning et al., 2002). Viruses are thus embedded in a matrix that can potentially shield them from external stressors, but could also enhance their inactivation.
Our results showed that feces did not enhance virus reduction. On the contrary, viruses embedded in feces survived longer in alkaline solutions compared to free viruses. The higher the pH the greater the difference between survival inside and outside the fecal material (Figure 3 and see SI, Supplementary Table S2). Therefore, in order to accurately assess the fate of viruses through the alkaline-urine treatment, we need to consider that most viruses are embedded, and the survival kinetics estimated based on free viruses thus requires a downward correction.
FIGURE 3. Calculated number of days required for a 3 log10 reduction in Ascaris [red] at the given temperature (pH did not impact the inactivation rate). Calculated number of days for a 4 log10 reduction in virus [green] at the given temperature and pH. Solid green line is for virus enmeshed in fecal material and the dotted line is for virus without enmeshment. As the temperature changes, so does the pH of urine/Ca(OH)2 solution (indicated by the blue dots).
The strong protective role of feces is currently poorly understood and deserves further investigation. For example, the size and the type of feces dependent on diet (structure, pH, ion composition) may influence the protective effect. In this work we only investigated feces from a single source. To better capture the extent of virus protection by the fecal matrix, future work should encompass a more diverse array of sources, and should include a thorough physical-chemical characterization of the samples. In addition, we here used phages to demonstrate the protective effect of feces. While we expect this finding to also apply to human viruses, this assumption remains to be confirmed. And finally, the mechanisms underlying the protective role of feces should be clarified. We here propose possible explanations which remain to be tested. First, the feces may form a physical barrier and prevent the exposure of the virus to the inactivating solution. Second, the feces may act as a chemical barrier that buffers the high pH. Or third, the feces matrix (Robinson et al., 2014; Pérez-Rodriguez et al., 2019) or inner ion composition (Meister et al., 2020) may increase virus stability towards pH and temperature.
When not protected by feces, however, viruses can be expected to be readily inactivated. An increase of pH from neutral to alkaline drastically increased virus inactivation in solution (see SI, Supplementary Figure S2A). Under the pH conditions expected in alkaline-urine treatment (pH > 10.5), MS2 and T4 phages require less than 10 days for a 4 log10 reduction at 20°C. This contact time became even shorter at pH > 11.5 with only a few seconds required. ΦX174 revealed to be stable up to pH 12.0, but was also drastically inactivated beyond that pH. Compared to thermal inactivation expected over the temperature range of 4–50°C, inactivation by alkaline pH is more efficient (Decrey, 2015). However, temperature nevertheless plays an important role, as the virucidal effects of pH and temperature were found to be synergistic. Inactivation at alkaline pH effect was enhanced by increasing temperature, and vice versa, thermal inactivation was more pronounced at higher pH (SI, Supplementary Figure S2B). In addition, the ion composition and ionic strength of the solution may also influence (enhance or inhibit) the virus stability towards high alkaline pH (Decrey 2015; Meister et al., 2020).
When concentrating urine during dehydration, any present pathogens would also be concentrated unless they were inactivated during the dehydration process. Considering the urine-drying temperature applied [0–40°C; (Randall et al., 2016)], the treatment duration and the type of fraction treated (precipitates provided some protection), Ascaris would likely not be inactivated inside the toilet or during drying. Virus inactivation takes place mainly during the addition of Ca(OH)2 and would continue during the drying phase of the end products, however, with slower kinetics (Decrey et al., 2011). Phages are only surrogates of human viruses, but they were more stable in the conditions expected during alkaline-urine treatment and thus serve as a worst-case scenario.
To produce a safe urine-based fertilizer from the alkalized-urine, we modelled the time required for a 4 log10 reduction in viruses and the time required for a 3 log10 reduction in Ascaris egg viability in order to meet both the WHO (2006) and USEPA (1994) guidelines for unrestricted fertilizer (crops consumed raw). The illustration in Figure 3 provides a rather conservative storage time requirement regarding both the Ascaris and the human virus reduction during alkaline-urine treatment. By collecting and treating the urine separately from the feces, for populations with no Ascaris (e.g., the Swedish and the Swiss population), the storage time can be determined by virus inactivation. Assuming a temperature of 20°C and a pH of 10, it would then just take 10 days for a 4 log10 virus reduction. In contrast, in populations prone to Ascaris infections, Ascaris becomes the rate-determining pathogen for alkaline-urine treatment, and 111 days of storage in a sealed bag would be required to ensure a 3 log10 inactivation at 20°C. Ascaris eggs were able to withstand alkaline environments better than bacteria (Senecal et al., 2018) and phages, so if Ascaris eggs are inactivated, then other pathogens, such as Salmonella spp. (Fidjeland et al., 2016), are also likely to be inactivated.
To enhance Ascaris inactivation, two potential post-treatments are thermal treatment and/or storage. In this study, compounding effects of alkaline pH or low moisture content started at temperatures ≥35°C, with pH having more of an effect than decreasing moisture content, but it still required 13–79 days for a 3 log10 reduction in viable eggs, depending on the urine fraction treated. A final thermal treatment (≥42°C) could be used to ensure faster inactivation (<5 days for a 3 log10 reduction) of soil-transmitted helminths. Thermal treatment would be more effective than decreasing the moisture content.
Storing the dried urine in a sealed bag will retain any NH3 formed and thereby ensure further inactivation of microbial contaminants, especially Ascaris spp. In a previous study where Ascaris eggs in urine/ash medium were kept in open containers (MC decreased to 1% by day 102), allowing the NH3 to volatilize, at 20°C an estimated 325 days were required for a 3 log10 reduction in viable eggs (Senecal et al., 2018). In the urine/Ca(OH)2 solution used in this study, where the Ascaris eggs were kept in sealed tubes (no volatilization of NH3 and stable MC%), a 3 log10 reduction at 20°C required a up to 111 days. Additional research has demonstrated that high pH (12.5) and dryness have no effect on the inactivation rate of Ascaris at ≤ 27°C for >70 days (Senecal et al., 2020). Based on the measured inactivation times, a minimal storage time of 111 days at 20°C or 79 days at 35°C in sealed containers would lead to a 3 log10 reduction in any Ascaris present, ensuring a hygiene safety level meeting existing guidelines WHO (2006) and USEPA (1994) guidelines for unrestricted use as fertilizer.
Diverting and collecting urine separately from feces is a good way to retrieve a large fraction of the nutrients from excreta, while excluding the majority of the pathogens. Virus were inactivated during the initial addition of Ca(OH)2, while Ascaris eggs were not. To ensure, and accelerate, the inactivation of parasites in alkaline treated urine, a post-treatment such as thermal treatment (≥42°C) and/or storage in a sealed container (retaining any NH3 formed) is ideal. Drying the alkaline urine can also be effective in shorting the time required for a 3 log10 reduction. However, only partially drying (MC 73–82%) the urine at 20°C can lead to longer inactivation times than in wet (MV >90%) and dried (MC <33%) conditions. Finally, parasites should be considered as worst case pathogen organisms and the alkaline-urine treatment should be designed based on preventing their survival to reduce pathogen risks associated with the end products.
The raw data supporting the conclusions of this article will be made available by the authors, without undue reservation.
JS: Designed the Ascaris experiment with support from BV and performed the experiment. Wrote the Ascaris sections together with AN. JS led the whole paper, with revisions by the co-authors. AN: Wrote the Ascaris sections with JS and contributed with revisions. LD: Designed the virus experiment with support from TK and performed the experiment. Wrote the virus sections together with TK. Contributed with revisions. TK: Designed the virus experiment with LD. Supported the writing of the virus sections. Contributed with revisions. BV: TK: Designed the Ascaris experiment with JS. Contributed with revisions.
Funding source was the Bill and Melinda Gates Foundation (grant number OPP1111293) which was awarded to the Swiss Federal Institute of Aquatic Science and Technology (EAWAG) which subcontracted the co-authors to perform the work.
The authors declare that the research was conducted in the absence of any commercial or financial relationships that could be construed as a potential conflict of interest.
All claims expressed in this article are solely those of the authors and do not necessarily represent those of their affiliated organizations, or those of the publisher, the editors and the reviewers. Any product that may be evaluated in this article, or claim that may be made by its manufacturer, is not guaranteed or endorsed by the publisher.
This work was funded by the Bill and Melinda Gates Foundation as part of the Blue Diversion Autarky project (OPP1111293). Thank you to our productive urine and fecal donors from the Department of Energy and Technology at the Swedish University of Agricultural Science.
The Supplementary Material for this article can be found online at: https://www.frontiersin.org/articles/10.3389/fenvs.2022.882284/full#supplementary-material
Antonini, S., Nguyen, P. T., Arnold, U., Eichert, T., and Clemens, J. (2012). Solar Thermal Evaporation of Human Urine for Nitrogen and Phosphorus Recovery in Vietnam. Sci. Total Environ. 414, 592–599. doi:10.1016/j.scitotenv.2011.11.055
Aw, T. (2018). Global Water Pathogen Project: Part 1 the Health Hazards of Excreta: Theory and Control. Editors B. Rose, and B. Jiménez-Cisneros (E. Lansing, MI: UNESCO, Michigan State University).
Bischel, H. N., Caduff, L., Schindelholz, S., Kohn, T., and Julian, T. R. (2019). Health Risks for Sanitation Service Workers along a Container-Based Urine Collection System and Resource Recovery Value Chain. Environ. Sci. Technol. 53 (12), 7055–7067. doi:10.1021/acs.est.9b01092
Bischel, H. N., Özel Duygan, B. D., Strande, L., McArdell, C. S., Udert, K. M., and Kohn, T. (2015). Pathogens and Pharmaceuticals in Source-Separated Urine in eThekwini, South Africa. Water Res. 85, 57–65. doi:10.1016/j.watres.2015.08.022
Capizzi-Banas, S., Deloge, M., Remy, M., and Schwartzbrod, J. (2004). Liming as an Advanced Treatment for Sludge Sanitisation: Helminth Eggs Elimination--Ascaris Eggs as Model. Water Res. 38 (14–15), 3251–3258. doi:10.1016/j.watres.2004.04.015
Chipako, T. L., and Randall, D. G. (2020). Urine Treatment Technologies and the Importance of pH. J. Environ. Chem. Eng. 8 (1), 103622. doi:10.1016/j.jece.2019.103622
Decrey, L., Kazama, S., and Kohn, T. (2016). Ammonia as an In Situ Sanitizer: Influence of Virus Genome Type on Inactivation. Appl. Environ. Microbiol. 82 (16), 4909–4920. doi:10.1128/aem.01106-16
Decrey, L., and Kohn, T. (2017). Virus Inactivation in Stored Human Urine, Sludge and Animal Manure under Typical Conditions of Storage or Mesophilic Anaerobic Digestion. Environ. Sci. Water Res. Technol. 3 (3), 492–501. doi:10.1039/c6ew00311g
Decrey, L., Udert, K. M., Tilley, E., Pecson, B. M., and Kohn, T. (2011). Fate of the Pathogen Indicators Phage ΦX174 and Ascaris suum Eggs during the Production of Struvite Fertilizer from Source-Separated Urine. Water Res. 45 (16), 4960–4972. doi:10.1016/j.watres.2011.06.042
Decrey, L. (2015). Virus Inactivation Mechanisms in Human Urine and Fecal Sludge. Lausanne, Switzerland: EPFL.
Dutta, S., and Vinnerås, B. (2016). Fertilizer from Dried Human Urine Added to Ash and Lime - a Potential Product from Eco-Sanitation System. Water Sci. Technol. 74 (6), 1436–1445. doi:10.2166/wst.2016.324
Emmoth, E., Ottoson, J., Albihn, A., Belák, S., and Vinnerås, B. (2011). Ammonia Disinfection of Hatchery Waste for Elimination of Single-Stranded RNA Viruses. Appl. Environ. Microbiol. 77 (12), 3960–3966. doi:10.1128/aem.02990-10
Feachem, R., Bradley, D., Garelick, H., and Mara, D. (1983). Sanitation and Disease - Health Aspects of Excreta and Wastewater Management. Chichester, UK: John Wiley & Sons.
Fidjeland, J., Nordin, A., and Vinnerås, B. (2016). Inactivation of Ascaris Eggs and Salmonella Spp. In Fecal Sludge by Treatment with Urea and Ammonia Solution. J. Water, Sanitation Hyg. Dev. 6 (3), 465–473. doi:10.2166/washdev.2016.017
Gaasenbeek, C. P., and Borgsteede, F. H. (1998). Studies on the Survival of Ascaris suum Eggs under Laboratory and Simulated Field Conditions. Vet. Parasitol. 75 (2), 227–234. doi:10.1016/s0304-4017(97)00198-2
Geinzer, M. (2017). Inactivation of the Urease Enzyme by Heat and Alkaline pH Treatment: Retaining Urea-Nitrogen in Urine for Fertilizer useMasters. Uppsala, Sweden: Swedish University of Agricultural Sciences.
Ghiglietti, R., Genchi, C., Matteo, L. D., Calcaterra, E., and Colombi, A. (1997). Survival of Ascaris Suum Eggs in Ammonia Treated Wastewater Sludges. Bioresour. Technol. 59 (1997), 195–198. doi:10.1016/s0960-8524(96)00147-2
Harder, R., Wielemaker, R., Larsen, T. A., Zeeman, G., and Öberg, G. (2019). Recycling Nutrients Contained in Human Excreta to Agriculture: Pathways, Processes, and productsInstitutional Repository. Crit. Rev. Environ. Sci. Technol. 49 (8), 695–743. doi:10.1080/10643389.2018.1558889
Harm, W. (1980). Biological Effects of Ultraviolet Radiation. Cambridge: Cambridge University Press.
Höglund, C., Vinnerås, B., Stenström, T. A., and Jönsson, H. (2000). Variation of Chemical and Microbial Parameters in Collection and Storage Tanks for Source Separated Human Urine. J. Environ. Sci. Health, Part A 35 (8), 1463
Jiménez, B. (2006) Water Reuse New Paradigm towards Integrated Water Resources Management in Encyclopedia of Biological, Physiological and Health Sciences. . Editor W. Grabow. EOLSS Publishers Co. Ltd UNESCO.
Jönsson, H., Baky, A., Jeppsson, U., Hellström, D., and Kärrman, E. (2005). Composition of Urine, Faeces, Greywater and Biowaste: For Utilisation in the URWARE Model. Gothenburg, Sweden: Chalmers University of Technology.
Larsen, T. A. (2020). “Urine Source Separation for Global Nutrient Management,” in Women in Engineering and Science. Women in Water Quality. Investigations by Prominent Female Engineers. Editor D. J. O’Bannon. (Cham: Springer), 99–111. doi:10.1007/978-3-030-17819-2_6
Magri, M. E., Fidjeland, J., Jönsson, H., Albihn, A., and Vinnerås, B. (2015). Inactivation of Adenovirus, Reovirus and Bacteriophages in Fecal Sludge by pH and Ammonia. Sci. Total Environ. 520, 213–221. doi:10.1016/j.scitotenv.2015.03.035
Martin, T. (2020). L’urine humaine en agriculture: des filières variées pour contribuer à une fertilisation azotée durable. Paris: Thesis, Université Paris-Saclay.
Maurer, M., Pronk, W., and Larsen, T. A. (2006). Treatment Processes for Source-Separated Urine. Water Res. 40 (17), 3151–3166. doi:10.1016/j.watres.2006.07.012
Maya, C., Ortiz, M., and Jiménez, B. (2010). Viability of Ascaris and Other Helminth Genera Non Larval Eggs in Different Conditions of Temperature, Lime (pH) and Humidity. Water Sci. Technol. 62 (11), 2616–2624. doi:10.2166/wst.2010.535
Meister, S., Prunotto, A., Dal Peraro, M., and Kohn, T. (2020). Salt Enhances the Thermostability of Enteroviruses by Stabilizing Capsid Protein Interfaces. J. Virol. 94 (11), e02176–02119. doi:10.1128/JVI.02176-19
Naidoo, D., Archer, C., Louton, B., and Rodda, N. (2016). Testing Household Disinfectants for the Inactivation of Helminth Eggs on Surfaces and in Spills during Pit Latrine Emptying. Water sa. 42 (4), 6 doi:10.4314/wsa.v42i4.06
Nordin, A., Nyberg, K., and Vinnerås, B. (2009). Inactivation of Ascaris Eggs in Source-Separated Urine and Feces by Ammonia at Ambient Temperatures. Appl. Environ. Microbiol. 75 (3), 662–667. doi:10.1128/aem.01250-08
Nyberg, K. A., Vinnerås, B., Lewerin, S. S., Kjellberg, E., and Albihn, A. (2011). Treatment with Ca(OH)2 for Inactivation of Salmonella Typhimurium and Enterococcus faecalis in Soil Contaminated with Infected Horse Manure. J. Appl. Microbiol. 110 (6), 1515–1523. doi:10.1111/j.1365-2672.2011.05006.x
Parkhurst, D., and Appelo, C. (2013). PHREEQC (Version 3) - A Computer Program for Speciation, Batch-Reaction, One-Dimensional Transport, and Inverse Geochemical Calculations. Reston, VA: U.S. Geological Survey.
Pawlowski, Z. S. (1982). Ascariasis: Host-Pathogen Biology. Clin. Infect. Dis. 4 (4), 806–814. doi:10.1093/4.4.806
Pecson, B. M., Barrios, J. A., Jiménez, B. E., and Nelson, K. L. (2007). The Effects of Temperature, pH, and Ammonia Concentration on the Inactivation of Ascaris Eggs in Sewage Sludge. Water Res. 41 (13), 2893–2902. doi:10.1016/j.watres.2007.03.040
Pecson, B. M., Martin, L. V., and Kohn, T. (2009). Quantitative PCR for Determining the Infectivity of Bacteriophage MS2 upon Inactivation by Heat, UV-B Radiation, and Singlet Oxygen: Advantages and Limitations of an Enzymatic Treatment to Reduce False-Positive Results. Appl. Environ. Microbiol. 75, 5544–5554. doi:10.1128/aem.00425-09
Pérez-Rodriguez, F. J., Vieille, G., Turin, L., Yildiz, S., Tapparel, C., and Kaiser, L. (2019). Fecal Components Modulate Human Astrovirus Infectivity in Cells and Reconstituted Intestinal Tissues. mSphere 4 (6), e00568–00519. doi:10.1128/mSphere.00568-19
Randall, D. G., Krähenbühl, M., Köpping, I., Larsen, T. A., and Udert, K. M. (2016). A Novel Approach for Stabilizing Fresh Urine by Calcium Hydroxide Addition. Water Res. 95, 361–369. doi:10.1016/j.watres.2016.03.007
Randall, D. G., and Naidoo, V. (2018). Urine: The Liquid Gold of Wastewater. J. Environ. Chem. Eng. 6 (2), 2627–2635. doi:10.1016/j.jece.2018.04.012
Robinson, C. M., Jesudhasan, P. R., and Pfeiffer, J. K. (2014). Bacterial Lipopolysaccharide Binding Enhances Virion Stability and Promotes Environmental Fitness of an Enteric Virus. Cell. Host Microbe 15 (1), 36–46. doi:10.1016/j.chom.2013.12.004
Schönning, C., Leeming, R., and Stenström, T. A. (2002). Faecal Contamination of Source-Separated Human Urine Based on the Content of Faecal Sterols. Water Res. 36 (8), 1965. doi:10.1016/s0043-1354(01)00427-4
Senecal, J., Nordin, A., Simha, P., and Vinnerås, B. (2018). Hygiene Aspect of Treating Human Urine by Alkaline Dehydration. Water Res. 144, 474–481. doi:10.1016/j.watres.2018.07.030
Senecal, J., Nordin, A., and Vinnerås, B. (2020). Fate of Ascaris at Various pH, Temperature and Moisture Levels. J. water health 18 (3), 375–382. doi:10.2166/wh.2020.264
Senecal, J., and Vinnerås, B. (2017). Urea Stabilisation and Concentration for Urine-Diverting Dry Toilets: Urine Dehydration in Ash. Sci. Total Environ. 586, 650–657. doi:10.1016/j.scitotenv.2017.02.038
Smil, V. (2002). Nitrogen and Food Production: Proteins for Human Diets. AMBIO A J. Hum. Environ. 31 (2), 126–131. doi:10.1579/0044-7447-31.2.126
Spångberg, J., Tidåker, P., and Jönsson, H. (2014). Environmental Impact of Recycling Nutrients in Human Excreta to Agriculture Compared with Enhanced Wastewater Treatment. Sci. Total Environ. 493, 209. doi:10.1016/j.scitotenv.2014.05.123
Standard. Methods (2018) Standard Methods for the Examination of Water and Wastewater, American Public Health Association.
Steffen, W., Richardson, K., Rockström, J., Cornell, S. E., Fetzer, I., Bennett, E. M., et al. (2015). Sustainability Planetary Boundaries: Guiding Human Development on a Changing Planet. Science 347, 1259855. doi:10.1126/science.1259855
USEPA (1994) A Plain English Guise to the EPA Part 503 Biosolids Rule, Washington, D.C: U.S. Environmental Protection Agency, Office of Waste Management
Volpin, F., Chekli, L., Phuntsho, S., Cho, J., Ghaffour, N., Vrouwenvelder, J. S., et al. (2018) Simultaneous Phosphorous and Nitrogen Recovery from Source-Separated Urine: A Novel Application for Fertiliser Drawn Forward Osmosis. Chemosphere 203, 482–489. doi:10.1016/j.chemosphere.2018.03.193
Keywords: alkaline, urine, pathogen, nutrient recycling, Ascaris
Citation: Senecal J, Nordin AC, Decrey L, Kohn T and Vinnerås B (2022) Fate of Parasites and Viruses in Calcium Hydroxide-Treated Urine in Relation to Temperature and Moisture Content. Front. Environ. Sci. 10:882284. doi: 10.3389/fenvs.2022.882284
Received: 23 February 2022; Accepted: 19 April 2022;
Published: 10 May 2022.
Edited by:
Susana Segura Munoz, University of São Paulo, BrazilReviewed by:
Marina Smidt Celere Meschede, Federal University of Western Pará, BrazilCopyright © 2022 Senecal, Nordin, Decrey, Kohn and Vinnerås. This is an open-access article distributed under the terms of the Creative Commons Attribution License (CC BY). The use, distribution or reproduction in other forums is permitted, provided the original author(s) and the copyright owner(s) are credited and that the original publication in this journal is cited, in accordance with accepted academic practice. No use, distribution or reproduction is permitted which does not comply with these terms.
*Correspondence: Jenna Senecal, amVubmEuc2VuZWNhbEBzbHUuc2U=
Disclaimer: All claims expressed in this article are solely those of the authors and do not necessarily represent those of their affiliated organizations, or those of the publisher, the editors and the reviewers. Any product that may be evaluated in this article or claim that may be made by its manufacturer is not guaranteed or endorsed by the publisher.
Research integrity at Frontiers
Learn more about the work of our research integrity team to safeguard the quality of each article we publish.