- 1Environmental Engineering Group, Civil Engineering Department, Indian Institute of Technology Roorkee (IITR), Roorkee, India
- 2Department of Environmental Science, Tezpur University, Tezpur, India
- 3Department of Civil Engineering, College of Engineering, King Saud University, Riyadh, Saudi Arabia
- 4Department of Civil Engineering, Z.H. College of Engineering and Technology, AMU, Aligarh, India
Slaughterhouse plants, especially meat and poultry products (MPPs), generate significant quantities of wastewater during the slaughtering process and the cleaning of machinery. The handling and final disposal of wastewater from the slaughterhouse is a public and environmental health issue due to its pollution potential. The monitoring of three full-scale buffalo meat-based abattoir effluent treatment plants (ETPs) to remove organic matter and nutrients was conducted at three separate sites in India. Rotary and static screens, an equalization tank, a dissolved air flotation (DAF) system, aeration tanks, and clarifiers were the component unit operations/processes of the wastewater treatment systems. All the treatment plants were operating at an average flow rate of 254 m3/d Meem Agro (designed capacity 500 m3/d); 427 m3/d Al Noor (designed capacity 500 m3/d), and 353 m3/d International Agro Foods (IAF) plant (designed capacity 750 m3/d) for the treatment of slaughterhouse wastewater. The Al Noor and Meem Agro’s ETP consisted of a DAF unit working at the surface-loading rates of 5.7 and 1.48 m3/m2 h, respectively, applied during the analysis to the DAF system, resulting in 46.5 and 56.8% efficiencies of O&G and SS removal. The ETP of Al Noor and Meem Agro and IAF was loaded at an organic loading rate of 276 kg COD/day, 746 kg COD/day, and 629 kg COD/day, respectively. Performance of ETPs showed that the efficiency of total COD, total BOD, and TSS removal was 97, 98, and 99%; 94, 94, and 93%, and 95, 98, and 99% for Al Noor, Meem Agro, and IAF plants, respectively. The treatment plants’ efficiencies in all three plants for the removal of organic matter surpassed the effluent discharge level (as per Indian standards). Still, it is concluded that the ETPs need to be upgraded by incorporating the advanced wastewater treatment methods for the removal of nutrient concentration from treated effluents.
Introduction
Enhanced population and industrialization in recent times have resulted in reduced water quality. Wastewaters with high concentrations of pollutants are discharged from slaughterhouses, food processing plants, dairies, breweries, pharmaceutical, and tanning industries. Slaughterhouse wastewater, in particular, contains high content of organic matter, suspended solids, oil and grease, and nutrients (Mittal, 2006; Bustillo-Lecompte and Mehrvar, 2015). Different industrial processes, namely, slaughtering, refining, and washing, contribute different kinds of waste streams which combine to generate various components of pollution slaughterhouse wastewater.
The highly hazardous and polluting nature of the wastewater demands its proper treatment before final disposal. A variety of treatment techniques have been in use for the treatment of slaughterhouse wastewater such as physicochemical processes, biological treatment, and filtration (Avery et al., 2005; Mittal, 2006).
The potential of integrated poultry slaughterhouse wastewater treatment systems has been tested in many studies (Bustillo-Lecompte and Mehrvar, 2017; Debik and Coskun, 2009; and De Nardi et al., 2011). In integrated wastewater systems, the elimination of pollutants was accomplished by using multi-stage systems for recovery where multiple parameters, including heavy metals, grease and oils, color, demand for biochemical oxygen demand (BOD), total suspended solids (TSS), and chemical oxygen demand (COD) were monitored (Schwarzenbach et al., 2010; Oller et al., 2011; Ganzenko et al., 2014). Studies were also critical in providing more available information on wastewater for poultry slaughterhouses, citing various successful technologies in terms of their effectiveness. (Meiramkulova et al., 2018). The development of a full-scale model requires the installation and operation of laboratory-scale procedures (Zheng et al., 2019). However, the degree to which laboratory-scale studies are implemented on a full scale depends on a case-to-case basis (Malato et al., 2002). The composition of the wastewater, that can be used and the combination of technologies depend on the actual situation at the full-scale plant site. Treatment procedures on the laboratory scale have normally characterized by low ability and high versatility (Bakare et al., 2017). Treatment plants on a pilot-scale installations are part of the early stages of the development of an integrated full-scale treatment facility. However, it has not extensively explored the degree to which a full-size poultry slaughterhouse wastewater plant can be a good approximation of the laboratory scale. Pilot-scale plants are widely used during the first stage of process design before an industrial-scale treatment plant is designed (Han et al., 2012). Therefore, obtained results through research on the comparison between lab-scale and full-scale plants are of specific interest in order to understand the extent of scale-up of laboratory-scale reactor to full-scale.
Poultry slaughterhouses’ processing technological processes display significant organic matter composition variations, which accounts for a high load of wastewater contaminants (Salminen, & Rintala, 2002). The high organic loading of poultry slaughterhouses is conditioned by significant quantities of biodegradable substances, such as fat, loose meat, colloidal particles, soluble proteins, undigested foods, and suspended solids. The aerobic and anaerobic treatment methods, which are also subject to certain drawbacks, appear to be prevalent methods of treatment of slaughterhouse wastewaters. The high energy requirement for aeration is the most important drawback of the aerobic process in addition to high excess sludge generation (Davarnejad, and Nasiri, 2017). Similarly, low rate or slow process, sludge buoyancy due to high-fat content, sludge wash-out, and less efficiency in comparison to aerobic process is the well-documented demerits of anaerobic wastewater treatment process (EPA, 2008; Rajakumar, et al., 2011).
Therefore, aerobic treatment processes in tropical countries like India, Bangladesh, etc. (Baker et al., 2020) incur very high costly due to the high energy consumption required for aeration and post-treatment of a high quantity of excess sludge. On the other side the anaerobic digestion (AD) process offers low energy consumption, reduced sludge formation, and a smaller area (Harun et al., 2011; Cheng et al., 2014), but is inefficient in nutrient removal. Therefore, an anaerobic process followed by an aerobic process is the alternative combination (Li et al., 2018). Another option would be to increase the hydraulic retention time (HRT) to ensure more significant organic removal. However, this requirement would increase reactor size and investment costs (Aziz et al., 2019; Tong et al., 2020).
Moreover, different technologies on lab and pilot-scale were studied to treat slaughterhouse wastewater for the removal of organic carbon and nitrogen (Rahimi et al., 2011; Rajakumar et al., 2011; Bazrafshan et al., 2012; Sunder and Satyanarayan, 2013). However, inadequate information is available so far on the design criteria, evaluation, and performance of abattoir effluent treatment plants in India (Aziz et al., 2019). More detailed descriptions, including design adequacy and understanding, are lacking for the abattoir wastewater treatment plants. Therefore, this study focus on the performance and design suitability of abattoir wastewater treatment plant, based on the biological treatment process in northern India. Subsequently, the study also suggested the upgrade to improve the effectiveness of the abattoir wastewater treatment process.
Materials and Methods
Site Location and Description of Effluent Treatment Plants
For this study, three abattoir ETPs in the northern India were selected to evaluate the efficiency of the wastewater treatment plants. The descriptions of the plants are given below:
1) Meem Agro, Foods Pvt. Ltd., Kandla road, located at Kairana, district Shamli, Uttar Pradesh, India the factory occupies 11,000 sqm. area. It has an infrastructure for a slaughtering capacity of 600 buffalo/day for the total production of 72 MT/day of frozen meat, poultry feed supplement (PES), and 8 MT/day of tallow meat (Figure 1).
Al Noor Exports slaughterhouse plant is located at Shernagar, Jansath road, district Mujaffarnagar, Uttar Pradesh, India. The plant is spread in an area of 8 ha. It has infrastructure for a maximum slaughtering capacity of 600 buffalo/day and frozen meat production of 100 MT/day, by-product meat bone meal and Tallow 60 MT/day (Figure 2).
2) International Agro Foods slaughterhouse is located at Bhoor Garhi, Dasna, district Ghaziabad, Uttar Pradesh, India. It is spread over an area of 36,126 m2 with an effluent treatment plant spread in a 1900 m2 area. It has an infrastructure for the slaughtering capacity of 750 buffalo/day. The plant has a processing capacity of frozen meat 142 MT/per and by-product Meat Bone 29.4 MT/day, Tallow 9.8 MT/day, and Blood meal 1.125 MT/day (Figure 3; Tables 1–3).
Process Description of Effluent Treatment Plants
All ETPs scheme comprises of screen chamber, oil and grease trap, equalization tank and dissolved air floatation (DAF) unit, biological reactors (aeration tank), disinfection tank, pressure sand filter (PSF) or multi-grade filter (MGF), and activated carbon filter (ACF) followed by disinfection tank. However, the DAF unit was present only in ETPs of Meem Agro and Al Noor factory. Effluent from various meat process units was fed to the equalization tank followed by a fine screen and oil and grease trap. The mixing was done in an equalization tank by diffused aeration system. Mixed and homogenized effluent was then transferred at a steady rate to the DAF unit in which effluent with coagulants was mixed with compressed air to reduce the density. The treated/underflow of the DAF unit is then transferred to aeration tanks, where the mineralization of organic matter takes place using the activated sludge process. The treated effluent after disinfection was collected in a clear water tank followed by pumped to a multi-media filter (MF) and activated carbon filter (ACF). The clear water after MF and ACF was discharged on the land and was then used for irrigation and industrial purposes. Sludge from the DAF and aeration tanks was collected in the sludge-holding tank which was further dewatered by using the centrifuge and sludge drying beds. Dried sludge was used as fertilizer aid on agricultural land.
Sampling and Analysis
Samples in replicates obtained from different stages of ETP were frozen at 4°C in an icebox, and transported to the IIT Roorkee, India laboratory within 24 h of sampling. Samples were analyzed for physicochemical parameters such as pH, temperature, oil and grease, total alkalinity, turbidity, total biochemical oxygen demand (BOD), total chemical oxygen demand (COD), total suspended solids (TSS), ammonium nitrogen (NH+4-N), nitrate-nitrogen (NO−3-N) using standard methods for water and wastewater review (APHA, 2012). Analyzed on-site parameters for biological reactor such as some parameters namely; pH, DO, sludge volume index (SVI), oxygen uptake rate (OUR) were analyzed on-site. Mixed liquor samples from the aeration tank were also obtained for mixed liquor suspended solids (MLSS), mixed liquor volatile suspended solid (MLVSS), and microbiota analysis.
Analysis of Microbiota
For microbiota analysis, 25 sub-samples of mixed liquor were taken by automated micropipette, and a minimum of four replicates of this amount were counted under phase-contrast illumination.(×100 magnifications). The sludge was kept continuously homogenized and aerated to keep all the suspended solids during the entire analysis period.
Results and Discussion
Water Balance of Water Consumption and Wastewater Discharge in the Abattoir Processes
The water balance is a numerical audit of the water enters in a plant, the fraction used in the process followed by the fraction of discharge from the plant. A preliminary analysis of existing data was analyzed to assess the current water balance in order to improve the use of water in the three slaughterhouse industries under study.
The water consumption of processes and other potential uses namely; treated water for cooling processes, tank washing, trucks, floors, gardening, etc. Detailed information about the amount of water that each application used is included. It was found that groundwater was used as a source in all three abattoir industries for the slaughtering process.
In the Meem Agro industry, 575 m3/d of fresh water was actually required out of 620 m3/d for most of the purposes such as slaughtering (450 m3/d), laundry (15 m3/d), domestic canteen (20 m3/d), refrigeration and cooling, and boiler (50 m3/d). For the remaining use in lairage and rendering processes the treated water from the storage tank was taken, which was 15 m3/d and 30 m3/d, respectively (Figure 4A). About 470 m3/d of treated water was used for gardening and irrigation purposes.
In the case of Al Noor Pvt. Ltd., total of 300 m3/d of freshwater was required for all the processes (Figure 4B). Fraction of freshwater used for slaughtering such as slaughter hall (230 m3/d), tray washing 10 m3/d, debarking 5 m3/d, rendering (15 m3/d), machine room (15 m3/d), and for sanitary purposes (2 m3/d). About 264 m3/d of wastewater was fed to ETP for the treatment and after treatment 10 m3/d was used for gardening and the remaining 254 m3/d for irrigation purposes. Wastewater from toilet and kitchen is sent to septic tank followed by its discharge to the drain.
In international Agro Food Industries, 500 m3/d of freshwaters was required on average (Figure 4C). Out of this 490 m3/d of water was consumed for the slaughtering process and 10 m3/d for domestic purposes. The 260 m3/d of treated water was used for rendering and floor washing purposes. Hence, a total of 500 m3/d of wastewater was sent to ETP for the treatment. Approximately, 290 m3/d of treated water was used for irrigation purposes and 10 m3/d and 20 m3/d of treated water were used for cooling and landscaping purposes. Water used for domestic purposes such as toilet and kitchen wastewater sent to the septic tank.
Performance Evaluation of Effluent Treatment Plants
Carbon Removal
Organic carbon, which is the source of energy for heterogenic and denitrifying microorganisms, was estimated as chemical oxygen demand (COD). All three ETPs were running on an average flow of 254 m3/d (Meem Agro, 500 m3/d), 427 m3/d (Al Noor, 500 m3/d), and 353 m3/d (IAF, 750 m3/d), respectively. The treated effluent from the DAF unit or primary settling unit is fed to aeration tanks, where suspended biomass (MLSS/MLVSS) degrades the organic matter. DAF unit was installed in the ETP of Al Noor and Meem Agro designed at the surface-loading rates of 5.7 and 1.48 m3/m2 h, respectively, which resulted in O&G and SS removal efficiencies of 95 and 99% and 57 and 83%, respectively (Table 4). In addition to O&G and suspended solids (SS) significant BOD and TSS removal was observed in the DAF unit.
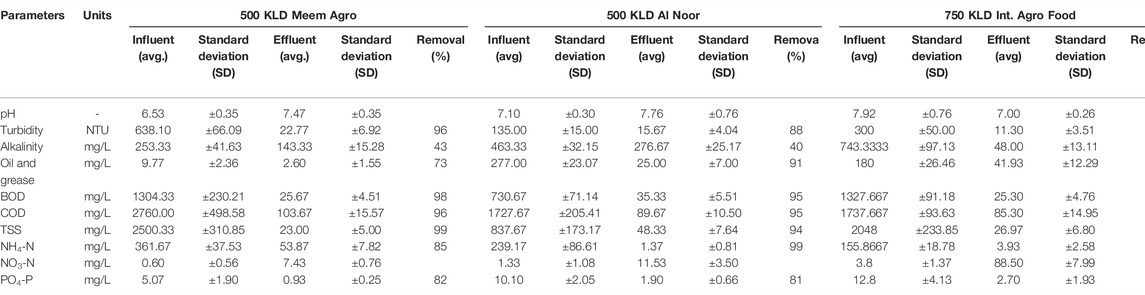
TABLE 4. Performance of ETPs including average unit process data, standard deviation, and removal efficiencies.
Organic matter is removed in the aeration basin in which microorganisms metabolize the suspended and soluble organic matter. Part of the organic matter was synthesized into new cells (MLSS) and part was oxidized to CO2. The ETP of Meem Agro comprised of single aeration tanks (SBR) for BOD removal, was operating at an average F/M ratio of 0.05 gBOD/gVSS.d. While, the ETP of Al Noor comprised three aeration tanks in parallel, operating at an average F/M ratio of 0.13 gBOD/gVSS.d. The observed F/M was slightly higher due to the presence of low biomass in aeration tanks. The ETP of International Agro Food comprised two aeration tanks in series and were operating at an F/M ratio of 0.3 gBOD/gVSS.d in aeration Tank −1 and 0.1 gBOD/gVSS.d in aeration Tank −2. The higher F/M enabled aeration Tank −1 to absorb higher BOD shock loads. The aeration Tank −2 operating in series acted as a polishing treatment unit. The removal efficiency of secondary treatment of Meem Agro, Al Noor, and IAF IS shown in Table 4.
Observed values for COD, BOD, and TSS in the treated effluent of Meem Agro were 89, 79, and 85 mg/L, Al Noor was 26, 41, and 25 mg/L and IAF were 29, 50, and 26 mg/L, respectively (Figure 5). The organic loading rate was 726.4, 747.2, and 629.4 kg COD/m3 day and BOD load of 328.4, 298.9, and 501.9 kg/BOD/m3 Day, respectively. However, COD and BOD removal efficiency were 97, 98, 94, 94, and 83%, respectively. Whereas TSS load to ETP was 617.4, 345.8, and 810.1 kgTSS days and removal efficiency of 95, 98, and 99%, respectively. Based on on-site measurements the sizes of aeration tanks of all the ETPs were found adequate.
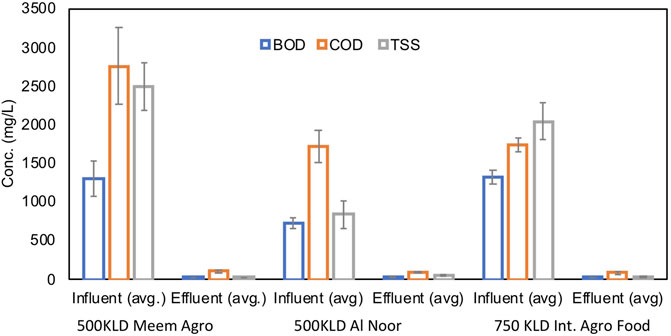
FIGURE 5. Organic and suspended solids removal in terms of COD, BOD, and TSS in the ETPs at Meem Agro, Al Noor and IAF Pvt., Ltd.
Nitrogen and Phosphorus Removal
The nitrogen loading to the ETP of Al Noor, Meem Agro, and IAF Pvt. Ltd. was 82.5, 118.5, and 62.4 kgNH4-N/day, and the results indicated that the ammonia removal efficiencies were 83, 99.8, and 99%, respectively. However, significant nitrification was observed during the process in all three ETPs but high NO3-N values observed in the final effluent sample of Al Noor, IAF, and Meem Agro were 15.5 mg/L, 96 mg/L, and 6.9 mg/L, respectively (Figure 6).
While, phosphorus load on Al Noor, Meem Agro, and IAF Pvt., Ltd., ETPs were 0.78 kg PO4-P/day, 3.4 kg PO4-P/day and 4.9 kg PO4-P/day. The observed removal efficiency was 77, 75, and 65%, respectively. In the final treated effluent sample, the observed PO4-P values were 0.7 mg/L, 2.0 mg/L, and 4.9 mg/L in Meem Agro, Al Noor, and IAF respectively (Figure 6).
pH, Alkalinity, Ammonia-N, and Nitrate-N Profiles
A biological system’s pH and alkalinity values are critical for the microbial activity for concurrent nitrification and denitrification (SND). Therefore, alkalinity provides essential stability through the maintenance of the desired level of alkalinity. Alkalinity was found to have a close connection with the activity based on the activated sludge phase, as different levels of nitrification (alkalinity consumption) and denitrification (alkalinity production) in all ETPs lead to the alkalinity variation in the system.
In the case of Meem Agro, pH value decreased to slightly acidic in DAF (5.6) due to the mixing of coagulant and compressed air then increased in SBR effluent (7.6), and remained uniform at 7.5 in the final effluent. Similarly, alkalinity reduced from 240 mg/L to 135 mg/L in DAF and did not reduce further below 130 mg/L in final outlet. While good nitrification occurred in the DAF unit and aeration tank and NH4-N reduced from 325 mg/L to 243 mg/L. It was then further reduced to 68 mg/L, respectively, due to aeration, simultaneously significant denitrification also occurred during the settling and observed NO3-N value of 6.9 mg/L in the final effluent. Results indicated that alkalinity was consumed during nitrification, however still a high amount of NH4-N was observed in the final effluent sample (Kundu et al., 2013). However, NH4-N decreased from 277 mg/L to 0.5 mg/L and the NO3-N value was observed at 15.5 mg/L in the final effluent sample which showed significant simultaneous nitrification and denitrification in the ETP of Al Noor samples (Figure 7). Similarly, very high nitrification and denitrification were observed in ETP of IAF and alkalinity reduced from 650 mg/L to 34 in the final effluent sample and NH4-N reduced from 176.8 mg/L to 1.6 mg/L, while Nitrate was increased from 0.3 to 5.5 mg/L, respectively.
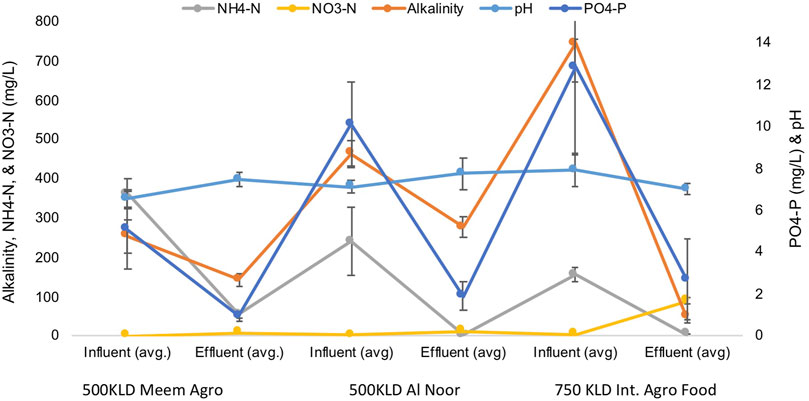
FIGURE 7. pH, alkalinity, and NH4-N and NO3-N profiles during the abattoir wastewater treatment process.
Operational Parameters During the Treatment Process
Different levels of MLSS were observed in aeration tanks of all three plants, i.e., 4,385 mg/L in the ETP of Meem Agro, 1,563 mg/L in the ETP of Al Noor, and in the ETP of IAF was 2,764 mg/L (Tank-1) and 1,682 (Tank-2), while the organic fraction in the ETPs in the form of VSS/SS ratio was 0.64, 0.60, and, 0.57, respectively. The sludge volume index (SVI) in the secondary or final clarifier of the triggered sludge was measured. Lower SVI values demonstrate better sludge settle-ability. Excellent zone settling can be interpreted for SVI measuring cylinders with Meem Agro and Al Noor aeration tank sludge values of 103 ml/g and 147 ml/g and SVI 94 ml/g and 89 ml/g in tank−1 and tank−2, respectively in IAF and it demonstrated the non-bulking nature of the sludge (Table 5).
The specific oxygen uptake rate (SOUR) in terms of the quantity of oxygen used by microorganisms while consuming 1 g of food is an indicator of the activity of the sludge. The mixed liquor sample was filled into a 1,000 ml capacity flask containing a magnetic stirring system for analyzing OUR (Table 4). The sample was aerated for several minutes in order to obtain a saturation DO concentration of around 7.8 mg/L. Then a DO probe was inserted into the flask immediately. An appropriate sample was pushed with the electrode-containing probe to fill the bottle’s flared top to isolate its atmospheric contents. The sample was then stirred and the DO was measured at an interval of 1 min for 10 min. The resulting oxygen uptake rate (SOUR) was determined by obtaining the graphical slope of the previously measured OUR values as 5.71, 6.26 mgO2/g.VSS.h, 17.5–5.6 (in tank-1 and tank-2) mgO2/g.VSS.h Meem Agro and Al Noor aeration tanks, while 17.5 and 5.6 mgO2/g.VSS.h in tank-1 and tank-2, respectively in the IAF industry, Table 4 summarizes the all relevant aeration tank sludge characteristics.
The cellular composition on the basis of dry weight is carbon 50%, oxygen 20%, nitrogen 15%, hydrogen 8%, phosphorus 3%, and <1% each of sulfur, potassium, sodium, calcium, iron, and magnesium (Droste, 1997). The average carbon to nitrogen to phosphorus ratio (C: N: P ratio) evolved through empirical forms of activated sludge biomass are expressed as C5H7NO2 and C42H100N11O13P (Metcalf & Eddy, 2003) is reported as 100:5:1 (Droste, 1997). However, the actual requirement of nutrients for net cell synthesis of biomass depends on the influent concentration of nitrogen and phosphorus. However, the actual concentration of nutrients shall be sufficiently more than stoichiometric requirements to prevent the nutrient content as a rate-limiting concentration. The C:N:P ratio is conflictingly reported in the literature as around 100:17:5 and 100:19:6 (Peter Spencer Davies, 2005).
The C:N:P ratio at the inlet of the aeration tank of Al Noor was 100:29.8:1.2, Meem Agro was 100:16.6:0.4, and IAF Pvt., Ltd., was 100:23.3:0.5. The inadequate growth of sludge in the aeration tank of Al Noor (1,563 mg TSS/L) was due to inadequate phosphorus content and poor equalization (2.35 times less volume) causing shock loads.
The performance of rapid sand filter (RSF) followed by activated carbon filter (ACF) as a tertiary treatment unit was variable. The BOD removal efficiency in Meem, Al Noor and IAF ETP final effluent was 52, 8, and 7%, respectively, while the TSS was 38, 50, and 47% respectively. These efficiencies were much below the desired level. The design filtration rate of RSF and ACF were 12 m3/m2 h. and 15 m3/m2 h, respectively, however, the actual rate of filtration in Meem, Al Noor, and IAF ETPs was 4.68 m3/m2 h. identical in both RSF and ACF; 5.14 and 10.11 m3/m2 h. and 7.13 m3/m2 h. identical in both RSF and ACF, therefore an inference can be drawn that the RSF and ACF units require further reduced design rate of filtration for slaughterhouse wastewater and this aspect needs careful reconsideration otherwise frequent replacement of the media of RSF and ACF may be required for better polishing of effluent.
Microbiota Observations
Protozoa are single-layered species ranging from 10 microns to more than 300 microns in size. Their primary role in the treatment process is to eliminate non-flocculent bacteria and tiny flocks that will not settle. Protozoans are responsible for the mechanism of flocculation that results in the organic particle biosorption phenomenon n addition to the improvement in sludge setting properties. In treating traditional contaminants and micro-contaminant degradation, these processes are essential. The presence or absence of protozoa is also an indication of the number of bacteria in the sludge and the extent of treatment (Eikelboom, 2000). Ciliated protozoa are the significant bio-indicator of the activated sludge process. It shows the plant’s operational characteristics (Supplementary Figure S1 in supplementary figures), and comparative observations of protozoans growing in the aeration basins of three ETPs as shown in Supplementary Figure S2 in supplementary figures.
Microbiota prevailing in the aeration tanks’ sludge of all the ETPs differ in their numbers depicting the different conditions of the sludge. Amoeba species and Flagellates were found slightly more in number in the IAF sludge, whereas these species were less in the Meem Agro ETPs. Ciliates were similar in their number in three plants depicting the satisfactory effluent quality. However, the number of worms species was higher in the IAF ETP sludge. Filamentous bacteria enumerated in three ETPs varied relating to the different F/M ratios, i.e., high substrate condition was prevailing in the IAF ETP as compared to the Meem and Al Noor ETPs. The presence of these protozoans in the sludge of all three ETPs, whether more or less in their numbers reflected the good health of the biomass in aeration basins and was responsible for the satisfactory quality of treated effluent by virtue of efficient settling.
Conclusion
The present experimental investigation demonstrated that the activated sludge process is an adaptable and efficient biological method to treat slaughterhouse wastewater in a single unit. Current research also supports that some pre-treatment processes are required, such as chemical dosing, PST, and DAF, to reduce oil and grease and TSS load on the subsequent biological unit processes. It is concluded that ETPs of Meem Agro and International Agro Food (IAF) were performing well with respect to carbon and nitrogen removal, while high BOD was observed in the treated effluent of Al Noor ETP due to the prevalence of low biomass (MLSS) in the aeration tank and poor settling in a secondary sedimentation tank. The low density of microbiota in Al Noor and Meem Agro’s aeration tank sludge revealed the unbalanced quality of sludge than the sludge in IAFs. This is due to the DAF unit not reducing the solids, oil, and grease. Overall, biological treatment efficiency for the ETPs of Meen Agro and IAF was found better and effluent quality satisfied the effluent discharge standards compared to the ETP of Al Noor due to the system’s unproportioned food and biomass ratio. However, C:N:P ratio was found deficient with respect to phosphorus content. The supplementation of phosphorus may further stabilize and improve the process. The performances of rapid sand filter followed by activated carbon filter units as final effluent polishing were inconsistent and demands revision of reduced design rates in comparison to the prevailing adopted standards. However, further long-term investigation for all the previously mentioned aspects is suggested to overcome the challenges during abattoir wastewater treatment.
Data Availability Statement
The original contributions presented in the study are included in the article/Supplementary Material, further inquiries can be directed to the corresponding author.
Author Contributions
The study relates to Abattoir wastewater full-scale treatment plants; therefore, the major tasks include formulation of the methodology of monitoring of plants, sample collection and analysis, result compilation, result interpretation, manuscript writing, figure and table preparation, literature review, manuscript correction, and final proof reading. AK as the principal investigator got this project and conceived the idea and developed a methodology for monitoring plants. AR was responsible for sample collection and analysis and result compilation, discussed the results and commented on the manuscript, and performed literature review. MC was responsible for sample collection and analysis, result compilation, and microbiological tests. MA was the head of the field team responsible for sample collection and analysis, result compilation, and final submission of the manuscript as the corresponding author after necessary discussion. AIA, AFA, and FM each verified the analytical methods, performed the analytic calculations, and checked the results of one ETP each preparation of tables and figures for the final manuscript. AK supervised the findings of this work, discussed the results of each ETP with co-authors, checked the final manuscript, and concluded the results before final submission. All authors discussed the results and commented on the manuscript.
Conflict of Interest
The authors declare that the research was conducted in the absence of any commercial or financial relationships that could be construed as a potential conflict of interest.
Publisher’s Note
All claims expressed in this article are solely those of the authors and do not necessarily represent those of their affiliated organizations, or those of the publisher, the editors, and the reviewers. Any product that may be evaluated in this article, or claim that may be made by its manufacturer, is not guaranteed or endorsed by the publisher.
Supplementary Material
The Supplementary Material for this article can be found online at: https://www.frontiersin.org/articles/10.3389/fenvs.2022.881623/full#supplementary-material
References
Avery, L. M., Killham, K., and Jones, D. L. (2005). Survival of E. coli O157:H7 in Organic Wastes Destined for Land Application. J. Appl. Microbiol. 98, 814–822. doi:10.1111/j.1365-2672.2004.02524.x
Aziz, A., Basheer, F., Sengar, A., Irfanullah, K. S. U., Khan, S. U., and Farooqi, I. H. (2019). Biological Wastewater Treatment (Anaerobic-aerobic) Technologies for Safe Discharge of Treated Slaughterhouse and Meat Processing Wastewater. Sci. Total Environ. 686, 681–708. doi:10.1016/j.scitotenv.2019.05.295
Bakare, B. F., Shabangu, K., and Chetty, M. (2017). Brewery Wastewater Treatment Using Laboratory Scale Aerobicsequencing Batch Reactor. S. Afr. J. Chem. Eng. 83, 13–26. [CrossRef]. doi:10.1016/j.sajce.2017.08.001
Baker, B. R., Mohamed, R., Al-Gheethi, A., and Aziz, H. A. (2020). Advanced Technologies for Poultry Slaughterhouse Wastewater Treatment: A Systematic Review. J. Dispersion Sci. Technol. 42, 880–899. doi:10.1080/01932691.2020.1721007
Bazrafshan, E., Mostafapour, F. K., Farzadkia, M., Ownagh, K. A., and Mahvi, A. H. (2012). Slaughterhouse Wastewater Treatment by Combined Chemical Coagulation and Electrocoagulation Process. PLoS One 7 (6).
Bustillo-Lecompte, C. F., and Mehrvar, M. (2015). Slaughterhouse Wastewater Characteristics, Treatment, and Management in the Meat Processing Industry: a Review on Trends and Advances. J. Environ. Manag. 161, 287–302. doi:10.1016/j.jenvman.2015.07.008
Bustillo-Lecompte, C., and Mehrvar, M. (2017). “Slaughterhouse Wastewater: Treatment, Management and Resource Recovery,” in Physico-Chemical Wastewater Treatment and Resource Recovery (London, UK: InTech), 153–174. doi:10.5772/65499
Cheng, S., Li, Z., Mang, H.-P., Huba, E.-M., Gao, R., and Wang, X. (2014). Development and Application of Prefabricated Biogas Digesters in Developing Countries. Renew. Sustain. Energy Rev. 34, 387–400. doi:10.1016/j.rser.2014.03.035
Davarnejad, R., and Nasiri, S. (2017). Slaughterhouse Wastewater Treatment Using an Advanced Oxidation Process: Optimization Study. Environ. Pollut. 223, 1–10. [CrossRef] [PubMed]. doi:10.1016/j.envpol.2016.11.008
De Nardi, I. R., Del Nery, V., Amorim, A. K. B., dos Santos, N. G., and Chimenes, F. (2011). Performances of SBR,chemical–DAF and UV Disinfection for Poultry Slaughterhouse Wastewater Reclamation. Desalination 269, 184–189. [CrossRef].
Debik, E., and Coskun, T. (2009). Use of the Static Granular Bed Reactor (SGBR) with Anaerobic Sludge to Treat Poultry Slaughterhouse Wastewater and Kinetic Modeling. Bioresour. Technol. 100, 2777–2782. [CrossRef][PubMed]. doi:10.1016/j.biortech.2008.12.058
Eikelboom, D. H. (2000). Process Control of Activated Sludge Plants by Microscopic Investigation.London, UK: IWA Publishing.
EPA (2008). Water and Energy: Leveraging Voluntary Programs to Save Both Water and Energy. Washington, DC: Environmental Protection Agency (Accessed November 11, 2020).
Ganzenko, O., Huguenot, D., van Hullebusch, E. D., Esposito, G., and Oturan, M. A. (2014). Electrochemical Advanced Oxidation and Biological Processes for Wastewater Treatment: a Review of the Combined Approaches. Environ. Sci. Pollut. Res. 21, 8493–8524. [CrossRef]. doi:10.1007/s11356-014-2770-6
Han, B., Kyu Kim, J., Kim, Y., Seung Choi, J., and Young Jeong, K. (2012). Operation of Industrial-Scale Electron Beam Wastewater Treatment Plant. Radiat. Phys. Chem. 81, 1475–1478. [CrossRef]. doi:10.1016/j.radphyschem.2012.01.030
Harun, R., Davidson, M., Doyle, M., Gopiraj, R., Danquah, M., and Forde, G. (2011). Technoeconomic Analysis of an Integrated Microalgae Photobioreactor, Biodiesel and Biogas Production Facility. Biomass Bioenergy 35, 741–747. doi:10.1016/j.biombioe.2010.10.007
Kundu, P., Debsarkar, A., and Mukharjee, S. (2013). Treatment of Slaughterhouse Wastewater in Sequencing Batch Reactor: Performance Evaluation and Biodegradation Kinetics. J. Biomed Res. Int. (9), 134872. doi:10.1155/2013/134872
Li, J., Wei, J., Ngo, H. H., Guo, W., Liu, H., Du, B., et al. (2018). Characterization of Soluble Microbial Products in a Partial Nitrification Sequencing Batch Biofilm Reactor Treating High Ammonia Nitrogen Wastewater. Bioresour. Technol. 249, 241–246. doi:10.1016/j.biortech.2017.10.013
Malato, S., Blanco, J., Vidal, A., and Richter, C. (2002). Photocatalysis with Solar Energy at a Pilot-Plant Scale: An Overview. Appl. Catal. B Environ. 37, 1–15. [CrossRef]. doi:10.1016/s0926-3373(01)00315-0
Meiramkulova, K., Userbayev, M., Aubakirova, K., Saiyabayev, K., and Suleimenova, B. (2018). Electrochemical Treatmentof Poultry Industry Wastewater. Int. J. Mech. Eng. Technol. 09, 10.
Mittal, G. (2006). Treatment of Wastewater from Abattoirs before Land Application-A Review. Bioresour. Technol. 97, 1119–1135. doi:10.1016/j.biortech.2004.11.021
Oller, I., Malato, S., and Sánchez-Pérez, J. A. (2011). Combination of Advanced Oxidation Processes and Biological Treatments for Wastewater Decontamination-A Review. Sci. Total Environ. 409, 4141–4166. [CrossRef]. doi:10.1016/j.scitotenv.2010.08.061
Peter Spencer Davies (2005). The Biological Basis of Wastewater Treatment. Glasgow, UK: Strathkelvin Instruments Ltd.
Rahimi, Y., Torabian, A., Mehrdadi, N., and Shahmoradi, B. (2011). Simultaneous Nitrification-Denitrification and Phosphorus Removal in a Fixed Bed Sequencing Batch Reactor (FBSBR). J. Hazard. Mater. 185 (2-3), 852–857.
Rajakumar, R., Meenambal, T., Banu, J. R., and Yeom, I. T. (2011). Treatment of Poultry Slaughterhouse Wastewater in Upflow Anaerobic Filter under Low Upflow Velocity. Int. J. Environ. Sci. Technol. 8, 149–158. [CrossRef]. doi:10.1007/bf03326204
Salminen, E., and Rintala, J. (2002). Anaerobic Digestion of Organic Solid Poultry Slaughterhouse Waste-Aa Review. Bioresour. Technol. 83, 13–26. [CrossRef]. doi:10.1016/s0960-8524(01)00199-7
Schwarzenbach, R. P., Egli, T., Hofstetter, T. B., von Gunten, U., and Wehrli, B. (2010). Global Water Pollution and HumanHealth. Annu. Rev. Environ. Resour. 35, 109–136. [CrossRef). doi:10.1146/annurev-environ-100809-125342
Sunder, G. C., and Satyanarayan, S. (2013). Efficient Treatment of Slaughter House Wastewater by Anaerobic Hybrid Reactor Packed with Special Floating Media. Int. J. Phys. Chem. Sci. 2, 73–81.
Tong, S., Zhao, Y., Zhu, M., Wei, J., Zhang, S., Li, S., et al. (2020). Effect of the Supernatant Reflux Position and Ratio on the Nitrogen Removal Performance of Anaerobic-Aerobic Slaughterhouse Wastewater Treatment Process. Environ. Eng. Res. 25, 309–315. doi:10.4491/eer.2019.091
Keywords: abattoir wastewater, activate sludge process, ETP, microbiota, COD, BOD, TSS
Citation: Rajpal A, Ali M, Choudhury M, Almohana AI, Alali AF, Munshi FMA, Khursheed A and Kazmi AA (2022) Abattoir Wastewater Treatment Plants in India: Understanding and Performance Evaluation. Front. Environ. Sci. 10:881623. doi: 10.3389/fenvs.2022.881623
Received: 22 February 2022; Accepted: 14 April 2022;
Published: 30 May 2022.
Edited by:
Sheena Kumari, Durban University of Technology, South AfricaReviewed by:
Ioannis Vyrides, Cyprus University of Technology, CyprusElena Neverova-Dziopak, AGH University of Science and Technology, Poland
Copyright © 2022 Rajpal, Ali, Choudhury, Almohana, Alali, Munshi, Khursheed and Kazmi. This is an open-access article distributed under the terms of the Creative Commons Attribution License (CC BY). The use, distribution or reproduction in other forums is permitted, provided the original author(s) and the copyright owner(s) are credited and that the original publication in this journal is cited, in accordance with accepted academic practice. No use, distribution or reproduction is permitted which does not comply with these terms.
*Correspondence: Muntjeer Ali, muntazir.ce@sric.iitr.ac.in