- 1Grassland Ecosystem Key Laboratory of Ministry of Education, Sino-US Research Centre for Sustainable Grassland and Livestock Management, Grassland Science College of Gansu Agricultural University, Lanzhou, China
- 2Faculty of Natural Resources and Environment, University for Development Studies, Tamale, Ghana
- 3Gansu Agricultural University, College of Food Science and Engineering, Lanzhou, China
- 4Key Laboratory of Development of Forage Germplasm in the Qinghai-Tibetan Plateau of Qinghai Province, Qinghai Academy of Animal Science and Veterinary Medicine of Qinghai University, Xining, China
- 5Gansu Agricultural University, College of Plant Protection, Lanzhou, China
Vegetation patchiness is common in degraded grasslands. Vegetation patchiness enhances the spatial variability of grassland soil organic carbon and total nitrogen. Stripped vegetation patches have a great impact on ecosystem carbon (C) and nitrogen (N) storage. Using field surveys, we examined the effects of patches on the ecosystem carbon and nitrogen storage of four typical alpine grass species patches (viz: Leymus secalinus, Koeleria pers, Stipa aliena, and Leontopodium nanum). The results indicated that ecosystem C, N, and respiration were significantly higher in intact vegetation patches than in stripped vegetation patches. Also, stripped vegetation patches recorded higher quantities of soil gravel content than the intact patches. In Leymus secalinus and Koeleria pers species patches, soil approximately contributed about 62% and vegetation about 38% to ecosystem carbon and nitrogen storage, whereas in Stipa aliena and Leontopodium nanum species patches, close to 80% of ecosystem carbon and nitrogen were found in the soil while close to 20% were stored in the vegetation. Soil total phosphorus (TP), total potassium (TK), available phosphorus (AP), soil microbial biomass carbon (MBC), and soil microbial biomass nitrogen (MBN) were higher in intact vegetation patches than in the stripped vegetation patches. Ecosystem carbon and nitrogen were observed to have a significant correlation with soil gravel content and vegetation productivity. Stripped vegetation patches resulted in decreased plant biomass input and an increased rate of soil erosion. We conclude that grassland patchiness resulted in the decline of ecosystem carbon and nitrogen storage due to a reduction in vegetation input and an increase in soil erosion. Grasslands are likely to have a higher possibility of serving as a C sink if the input of organic matter exceeds its output via sustainable management practices.
Introduction
Grasslands are a key part of the global ecosystem that account for a significant portion of Earth’s area and have a major function in influencing climate change by serving as a C sink (Li et al., 2013; Qin et al., 2015). They also have a substantial role in food security by serving as feed for beef and dairy livestock (O’Mara, 2012; Gang et al., 2014). Grassland ecosystems are experiencing increasingly unsustainable land use and climate change which could have catastrophic shifts in their structure and the services they provide (Scheffer et al., 2009; Dakos et al., 2019). Throughout the world, grasslands are said to store carbon in the soil at an estimated rate of 0.5 Pg C per year and this is about one-quarter of the possible soil C sequestration globally (Wu et al., 2014a). The accumulation of carbon is dependent on vegetation productivity, and nitrogen is one of the key nutrients that enhance a plant’s photosynthetic ability (Freschet et al., 2018). Therefore, C sequestration is usually closely linked to soil nitrogen. Hence, studies on soil organic carbon (SOC) and soil N storage are vital for the determination of their possibility for sequestration or emission (Qi et al., 2021). A little change in carbon storage could have a significant effect on CO2 in the atmosphere, and hence, the global C cycle and balance. The enhancement of carbon storage is a vital factor in ensuring grassland ecosystems' sustainability (Wang et al., 2014). All the same, grasslands are considered one of the most altered biomes on Earth (Pineiro et al., 2006). Grasslands are likely to have a higher possibility of serving as a high C sink if the input of organic matter exceeds its output via sustainable management practices; however, many grasslands have low carbon inputs and tend to be poorly managed and degraded (Shrestha and Stahl, 2008).
The Alpine grassland is one of the most vital grassland types and accounts for more than 66% of the grasslands on the Qinghai–Tibetan Plateau (QTP) (Miehe et al., 2019). Over the decades, these grasslands have experienced widespread degradation, leading to a decrease in water retention ability, biodiversity, carbon sequestration, grassland productivity, soil nutrients, and value for recreation (Bao et al., 2019; Li et al. ,2013; Lü et al., 2016; Qin et al., 2014; Zhang et al., 2016). However, high-altitude terrestrial ecosystems are regarded as major constituents of the global cycles of carbon and nitrogen (Lozano-García et al., 2016; Zhang et al., 2018). The top soils of these ecosystems have substantial amounts of SOC, soil nitrogen (TN), root carbon, and nitrogen (Jia et al., 2017). These ecosystems, to a high degree, are fragile and susceptible to external effects such as overgrazing, burrowing activities of mammals, and soil erosion which often lead to patchiness (Gao et al., 2009; Qin et al., 2014). As a result of these disturbances, intact vegetation would be isolated into archipelagos of small fragments and regenerating bald patches embedded in matrixes (Akiyama and Kawamura, 2007). This patchiness results in a reduction in ecosystem carbon and nitrogen storage (Nie et al., 2013; Haddad et al., 2015) and increased spatial variability of the distribution of carbon and nitrogen (Yan et al., 2016).
Patchiness can indicate the state and functionality of ecosystems (Heras et al., 2011); patches with different sizes and types indicate major differences in biomass, soil moisture, and properties (Chen et al., 2017; Qin et al., 2019). Therefore, obtaining an insight into the effects of patchiness on ecosystem N and C storage is a vital step in disclosing the process and mechanisms of the degradation of grasslands (Lin et al., 2010). Multi-scale research of patch patterns is one of the central directions of landscape ecology. Even though field surveys and remote sensing have been used to a greater extent in studies of patch patterns at plot and landscape scales, there is a lack of research on small-scale patchiness and its effect on ecosystem N and C accumulation. It would be pragmatic to identify signals for grassland state shifts by exactly discovering pertinent patch attributes and their specific variations along the entire stages of degradations. This would serve as an essential monitoring tool if it could be done by direct and simple vegetation measurements at small spatial scales.
Many studies have been conducted on species composition, biomass, and nutrient concentrations during the degradation succession of grasslands (Chen et al., 2013; Dong et al., 2013; Babel et al., 2014; Hopping et al., 2018). All the same, few studies have been conducted to provide information on the effects of patchiness on grassland ecosystem carbon and nitrogen storage which play vital roles in regional and global C and N cycles. This study aimed to examine the patchiness characteristics of four typical alpine grass species patches and their effect on ecosystem carbon and nitrogen storage. This work, thus, shows how a ground-based small-scale vegetation survey can provide easy-to-use ways for determining patch characteristics and effects of patchiness on grassland C and N storage.
Materials and methods
Study area
The study area is located in the alpine grasslands of the Qilian Mountains in Zhuaxixiulong Township in the Tianzhu Tibetan Autonomous County of Gansu Province of China. The area has a typical alpine climate and is usually cold and wet for most parts of the year. It also has weather conditions such as thin air with low oxygen concentrations, high solar and high ultraviolet radiation, and an annual temperature of about −4.0°C (Zhang et al., 2015). The site has big mountains with steep slopes (Yao and Zhen, 2017). Four typical alpine grass patches were selected, namely, Koeleria pers patch (KP), Leymus secalinus patch (LS), Leontopodium nanum patch (LN), and Stipa aliena patch (SA). The area has four typical alpine grassland types, viz, alpine swamp meadow, alpine meadow, alpine steep meadow, and alpine steep (Qin et al., 2014). A map indicating the study area and sampling location is shown in Figures 1, 2 is a picture of the four typical alpine grass species patches and a bare patch.
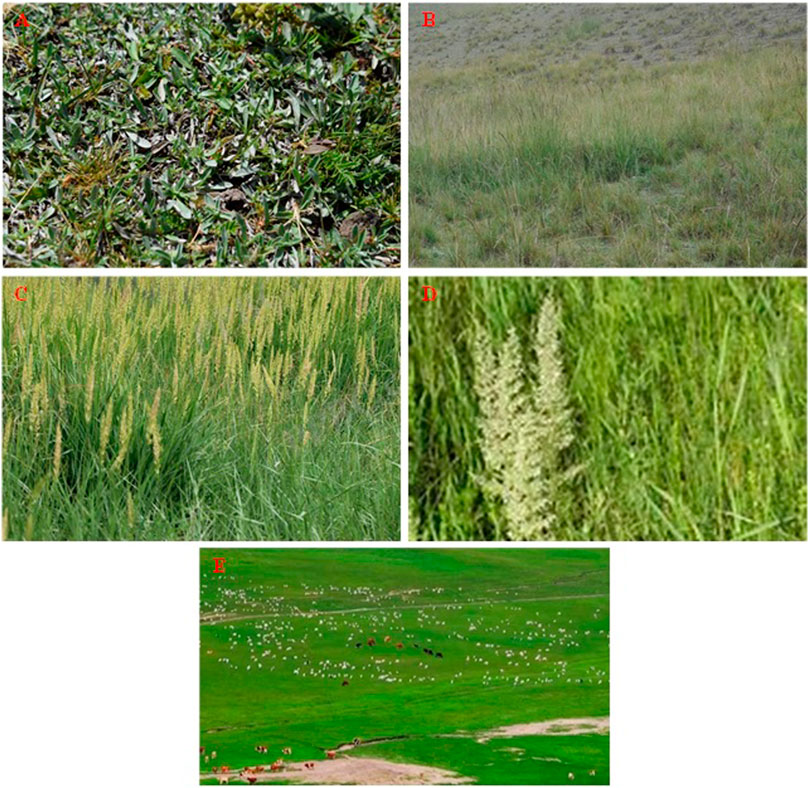
FIGURE 2. Picture of selected alpine grass species patches; (A) Leontopodium nanum (B) Stipa aliena, (C) Leymus secalinus, (D) Koeleria pers, and (E) the grassland showing a bare patch.
Field sampling
Field sampling was conducted along a transect that traversed the typical vegetation within the area. A total of 12 locations were selected among the KP, LS, LN, and SA patches with each having three locations. Locations with flat slopes of <4° were selected. The grasslands within these locations are used for grazing, usually from May to June and September to October. For each grassland type, three types of patches were delineated: intact vegetation patches (IGP), large stripped vegetation patches (LBP) with a diameter of 4–10 m, and medium stripped patches (MBP) with a diameter of <1–3.5 m. A total of 12 sampling locations were established and 90 soil core samples were taken in each location encompassing the three different patches in the selected types of grasslands. In total, 1,080 soil samples were taken at depths 0–10, 10–20, 20–30, and 30–40 cm with a core sampler (5 × 5 cm). Sampling in each patch was conducted between the end of July and the middle of August when vegetation growth was at its peak. Soil bulk density was estimated using the same core sampler (undisturbed soil). A total of three 50 cm × 50 cm quadrats were randomly established in each patch type and above and below-ground biomass was taken. Patches with different sizes were measured along the transect in each sampling location and on each surface type, and cover fractions of the patches were then calculated. Ecosystem respiration was estimated using an automated soil CO2 flux system (LI-800A, LI-COR Inc., Lincoln, United States). PVC collars (20 cm diameter and 15 cm height) were inserted into the soil to a depth of about 10–12 cm. Measurements were made among the four typical alpine grasslands with a total of 12 subplots within each patch surface. All measurements were made on sunny days.
Laboratory analysis
Soil samples were sent to the laboratory, air-dried, sieved via a wire mesh to remove unwanted particles such as roots and debris, and used for the determination of SOC and TN. Samples for below-ground biomass were washed in a fine wire mesh after which roots were then oven-dried and weighed. Oven-dried vegetation samples and root samples were grounded and sieved for analyzing organic C and TN. Organic C quantity in all samples was determined via dichromate oxidation using Walkley–Black acid digestion. TN in all the samples was determined by digestion and then tested using a flow injection analyses system (FIAstar, 5000, Foss Inc., Sweden) (Sparks et al., 1996).
Soil microbial biomass C and N were determined using the fumigation-extraction method (Wu et al., 1990). A total of 20 g of wet soil (dry weight basis) was fumigated by placing it in a sealed vacuum desiccator containing alcohol-free CHCl3 vapor for 24 h. The fumigated base was repeatedly discharged in an aseptic, empty desiccator until the scent of CHCl3 was no longer detectable, and then extracted with 80 ml of 0.5 M K2SO4 (soil: K2SO4 = 1:4) for 30 min. The extraction of non-fumigated soil was the same as that of fumigated soil. Soil microbial biomass C and N were calculated as the difference between total organic C and total N in the fumigated and non-fumigated extracts, respectively, with a conversion factor (KEC) of 0.38 and (KEN) of 0.45 (Jenkinson, 1988; Joergensen, 1996).
Estimation of SOC, TN, EC, and EN
The soil organic carbon and nitrogen were calculated as follows:
where SOC is soil organic C (kg m−2), TN is soil total N stocks (kg m−2), ρ is the soil bulk density (g cm−3), σ gravel is the relative amount of gravel (% w/w), CSOC is the soil organic C concentration (g kg−1), CTN is the soil total N concentration (g kg−1), and Di is the soil thickness (cm) at depth i, i = 1……….4.
The plant and root carbon and plant and root nitrogen stocks were calculated as
where PC/RC is the plant/root C stocks, PN/RN is the plant/root N stocks, PB/RB is the plant/root biomass (g m−3/kg m−2), C P/R is the plant/root C concentration (g kg−1), and N P/R is the plant/root N concentration (g kg−1).
Total ecosystem carbon and nitrogen stocks (kg m−2) were calculated as
where EC is ecosystem C (kg m−2), SOC is soil organic C (kg m−2), PC is plant carbon (kg m−2), RC is root carbon (kg m−2), EN is ecosystem nitrogen (kg m−2), TN is soil total nitrogen (kg m−2), PN is plant nitrogen, and RN is root nitrogen (kg m−2).
Data analysis
Data on gravel content, cover fraction, plant and root biomass, soil nitrogen, plant and root N, ecosystem carbon and nitrogen stocks, and soil physicochemical parameters were analyzed via a one-way ANOVA with the least significant difference at 5%. The relationship between ecosystem carbon and nitrogen with plant and root biomass and gravel content was analyzed via regression analysis. All statistical analyses were carried out using SPSS software version 21 (IBM Corp., Chicago, IL, United States). Principal component analysis was carried out to evaluate the effect of vegetation patchiness on ecosystem C and N. Figures and tables were drawn using Microsoft Office Word 365 and GraphPad Prism 6 (GraphPad Software, Inc., San Diego, CA, United States).
Results
Cover fraction of patch types, soil gravel, and plant biomass
Vegetation patterns and patch features varied amongst the various grassland types. The intact and stripped vegetation patches mosaic co-existed within the various grassland types. The average cover fractions of intact, large, and medium stripped vegetation patches constituted approximately 58–80%, 10–12%, and 11–30%, respectively, of the total area of KP and LS grass patches (Figure 3A). On the other hand, intact, large, and medium stripped vegetation patches contributed close to 34, 58, and 8%, respectively, of the total field of LN and SA grass patches (Figure 3A). In all, the KP patch recorded significantly low amounts of gravel content than the rest. For each of the selected grass types, the intact vegetation patch had less gravel content than those in stripped vegetation patches (Figure 3B). In each of the different patches, there were sharply varied productivity levels in all grass types. Intact vegetation patches recorded significantly higher biomass of both plants and roots than the rest of the patches (Figures 3C,D).
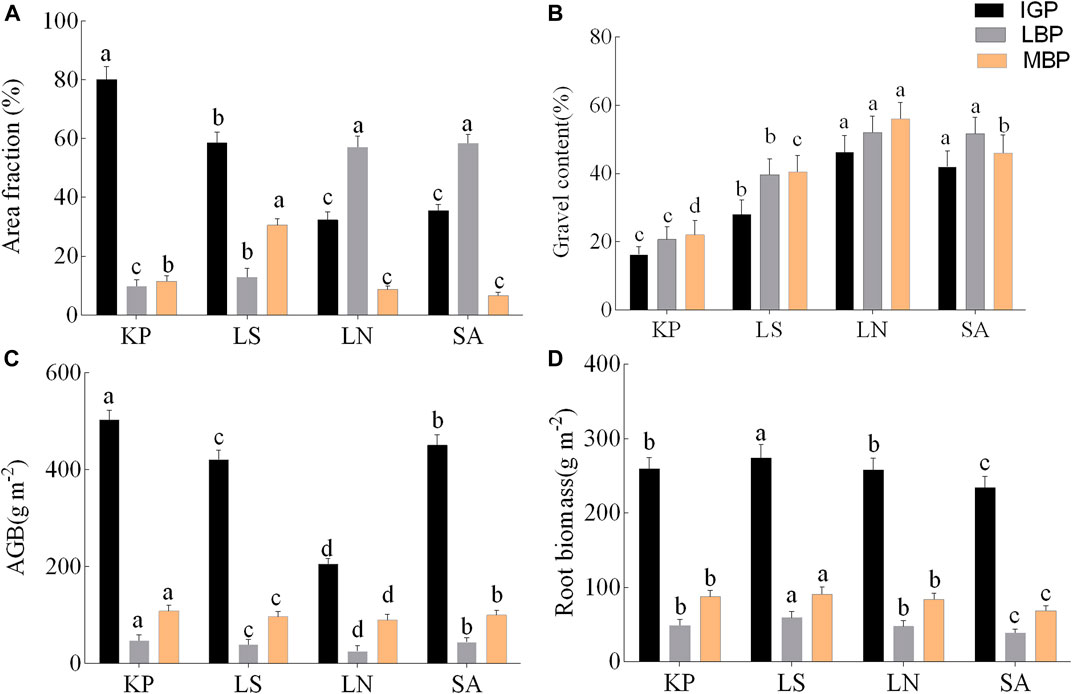
FIGURE 3. Cover fraction (A), soil gravel (B), plant biomass (C), and root biomass (D) in each of the various patches of the four alpine grass species types. Bars with different letters show significance at p < 0.001. IGP; intact vegetation patch, LBP; large stripped vegetation patch, MBP; medium stripped vegetation patch, KP; Koeleria pers patch, LS; Leymus secalinus patch, LN; Leontopodium nanum patch, SA; Stipa aliena patch.
Ecosystem C and N storage and respiration
The intact vegetation patches in all the grassland types recorded higher SOC and TN stocks than the other patches (Figures 4A, 5A). In addition, the intact vegetation patches in all the selected grass types had significantly higher above-ground biomass C and N, root C and N, ecosystem carbon, and nitrogen than that of the large and medium stripped vegetation patches (Figures 4B–D, Figures 5A–D). Also, ecosystem respiration was higher in the intact vegetation patches than in the stripped ones. KP and LS grass patches recorded significantly high amounts of soil respiration as compared to the rest (Figure 6).
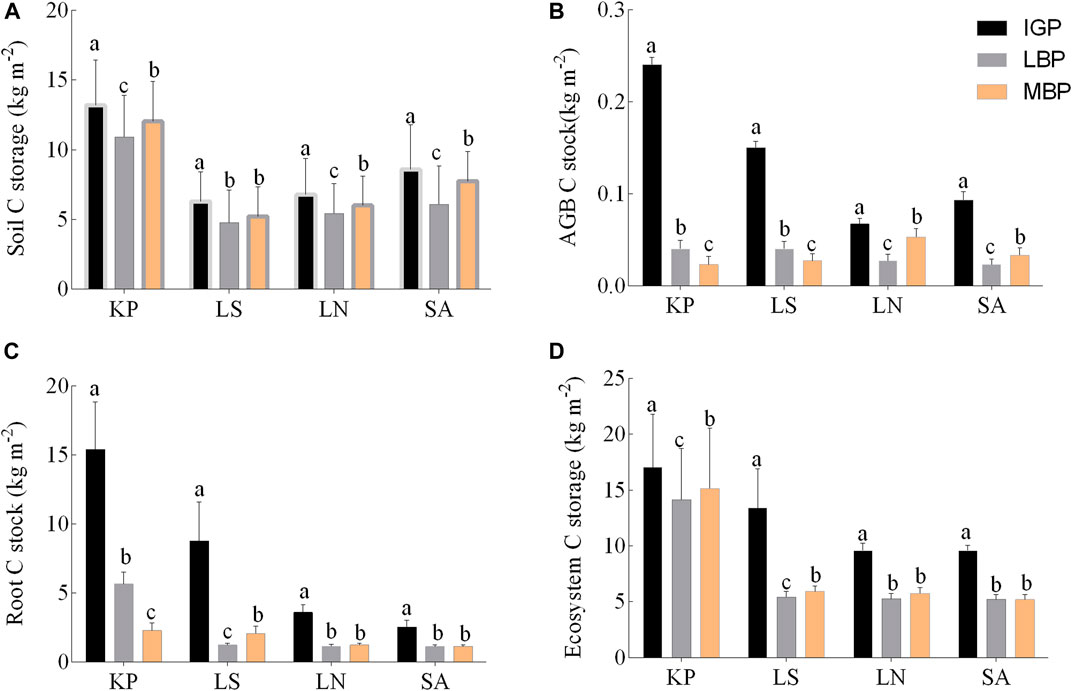
FIGURE 4. Soil organic carbon (A), plant carbon (B), root carbon (C), and ecosystem carbon (D) in each of the selected patches. Bars with different letters show significance at p < 0.001. IGP; intact vegetation patch, LBP; large stripped vegetation patch, MBP; medium stripped vegetation patch, KP; Koeleria pers patch, LS; Leymus secalinus patch, LN; Leontopodium nanum patch, SA; Stipa aliena patch.
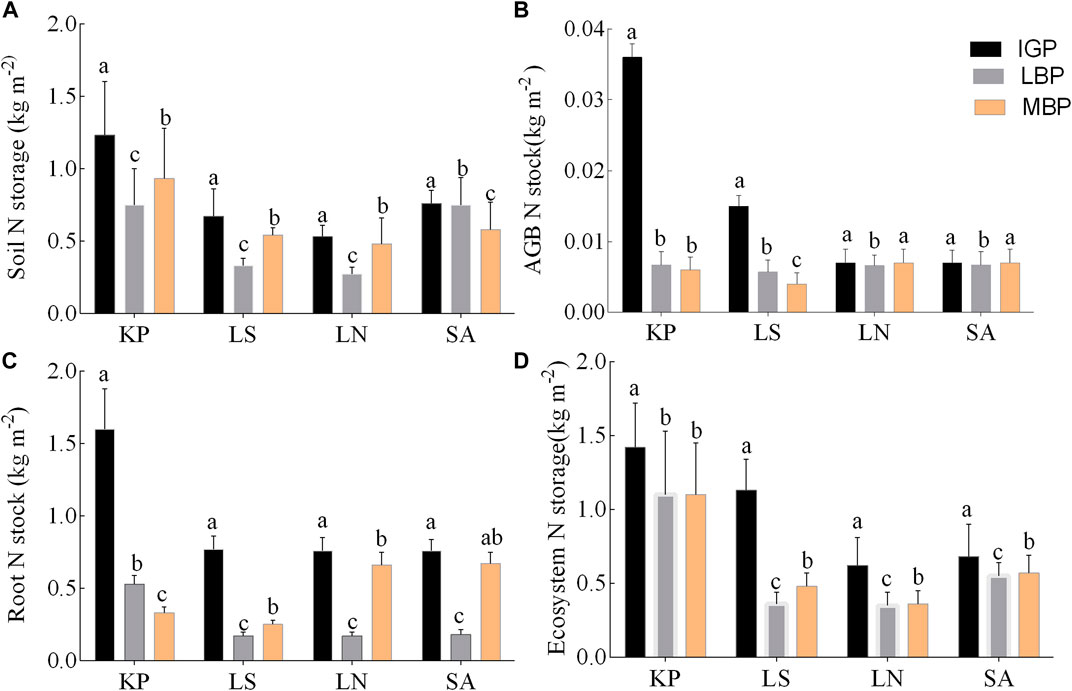
FIGURE 5. Soil total nitrogen (A), plant nitrogen (B), root nitrogen (C), and (D) ecosystem nitrogen storage in the selected patches. Bars with different letters show significance at p < 0.001. IGP; intact vegetation patch, LBP; large stripped vegetation patch, MBP; medium stripped vegetation patch, KP; Koeleria pers patch, LS; Leymus secalinus patch, LN; Leontopodium nanum patch, SA; Stipa aliena patch.
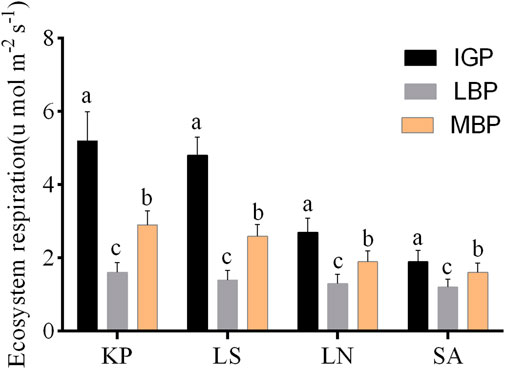
FIGURE 6. Ecosystem respiration of the various grass species patches. Bars with different letters show significance at p < 0.001. IGP; intact vegetation patch, LBP; large stripped vegetation patch, MBP; medium stripped vegetation patch, KP; Koeleria pers patch, LS; Leymus secalinus patch, LN; Leontopodium nanum patch, SA; Stipa aliena patch.
Soil C and N added approximately 78% and 83% of total ecosystem C in LN and SA, respectively, and 69% and 77% of total ecosystem N in LN and SA, respectively (Table 1). Above-ground biomass contributed the lowest to ecosystem total C and N in all types of grasslands. Similarly, root C and N contributed closely to 38% for KP and LS, 21% and 29% for LN, and 15% and 22% for C and N in SA (Table 1).
Relationship between ecosystem C and N storage, gravel content, and vegetation productivity
The study indicated that ecosystem carbon and nitrogen had a strong relation with soil gravel and above and below-ground biomass. There was a significant negative correlation between ecosystem carbon and nitrogen and soil gravel content (Figures 7A,B). However, ecosystem carbon and nitrogen stocks had a positive correlation between plant biomass (Figures 7C,D) and root biomass (Figures 7E,F), indicating that vegetation productivity had an impact on ecosystem carbon and nitrogen storage. Principal component analysis (PCA) was conducted to examine the effects of vegetation patchiness on ecosystem C and N storage (Figures 8A,B). Principal component 1 (PC 1) explained 73.27% while principal component 2 (PC 2) explained 15.38% of the total variations. Principal components 1 and 2 explained 88.65% of the variance. All vegetation and soil parameters within the intact and medium grass vegetation patches were on the positive side of PC 1 except for the cover fraction in LBP and MBP and gravel. SOC, root, and plant C and N were strongly positively correlated with ecosystem C and N. Gravel content, cover fraction in LBP, and MBP were negatively correlated with ecosystem C and N. The KP patch had strong positive loadings while the LS patch had strong negative loadings on PC 1. On PC 2, all patches except the LS patch had strong positive loadings, indicating that vegetation patchiness strongly influenced ecosystem C and N storage.
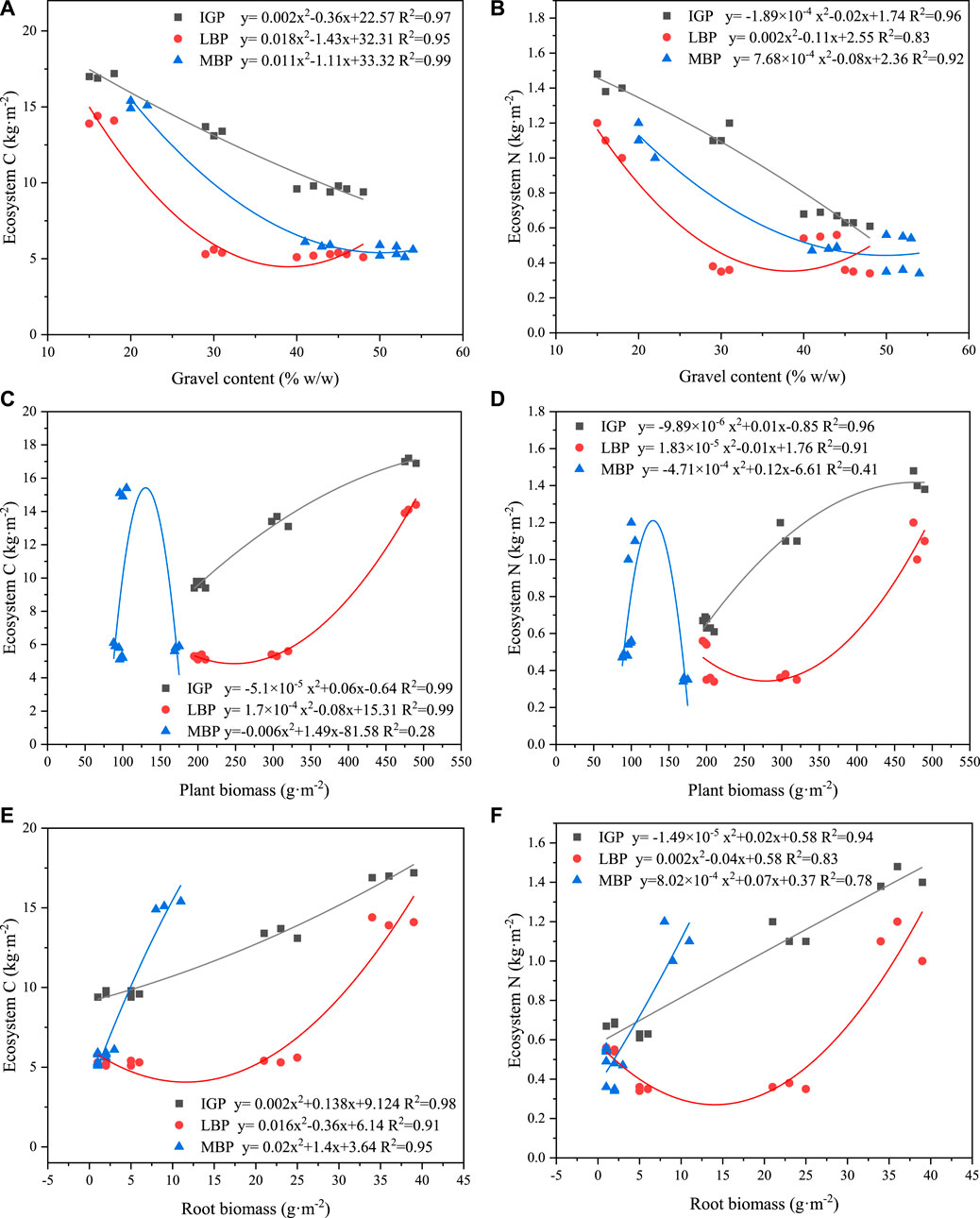
FIGURE 7. Relationship between ecosystem carbon (A) and nitrogen storage (B) with gravel, ecosystem carbon (C) and nitrogen storage (D) with plant biomass and ecosystem carbon (E) and nitrogen storage (F) with root biomass. p < 0.001. IGP; intact vegetation patch, LBP; large stripped vegetation patch, MBP; medium stripped vegetation patch.
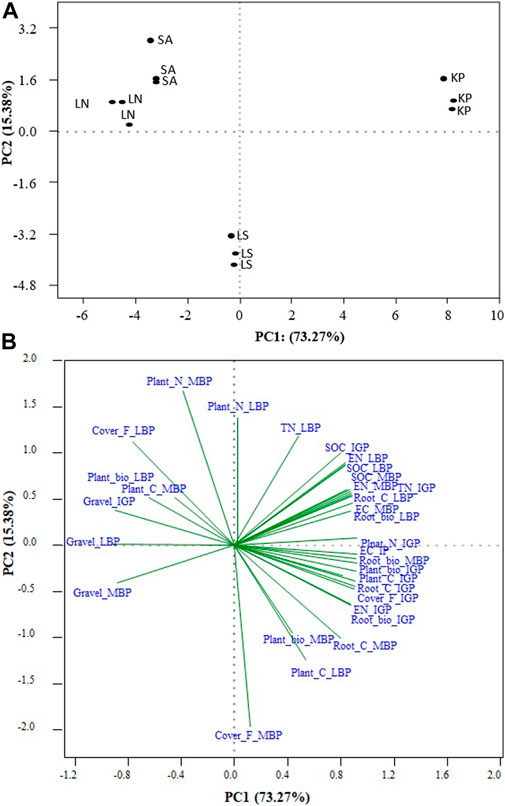
FIGURE 8. Principal component analysis (A) score plot and (B) biplot based on the effect of grassland patchiness on ecosystem C and N storage in four typical alpine grass species patches. KP; Koeleria pers patch, SA; Stipa aliena patch, LN; Leontpodium nanum patch, LS; Leymus secalinus patch. Cover F LBP; cover fraction in large stripped patch, Cover F IGP; cover fraction in intact grass patch, Cover F MBP; cover fraction in medium stripped patch, SOC IGP; soil organic carbon in intact grass patch, SOC LBP; soil organic carbon in large stripped patch, Plant bio IGP; plant biomass in intact grass patch, Plant N IGP; plant nitrogen in intact grass patch, EN IGP; ecosystem nitrogen in intact grass patch.
Variations in soil physicochemical parameters
Soil bulk density was lowest in the intact vegetation patches than in the medium and large stripped vegetation patches across all grassland types. Soil TP, TK, and AP were highest in the intact vegetation patches than in the medium and large stripped vegetation patches within all the selected grassland types and decreased with increasing depth. Soil AK did not follow a similar trend as that of TP, TK, and AP; it varied across the selected patches. The KP grass patch recorded the highest values of soil TP, TK, AP, and AK than the rest of the grassland types. It equally recorded the lowest value of soil bulk density. Similarly, soil MBC and MBN were equally higher in the vegetation patch than in the medium and large stripped vegetation (Table 2).
Discussion
Factors responsible for vegetation patchiness
The alpine grassland in the QTP is composed of three defined attributes which are vital for its ecosystem’s grassland sequence: a mattic epipedon layer (Dai et al., 2020), an active permafrost layer (Yin et al., 2017), and a non-uniform rainfall distribution (You et al., 2014). These distinct attributes lead to a unique eco-hydrological process (Wang et al., 2012). These features can increase the degradation of alpine grasslands in the QTP. They could also lead to the expansion of bald patches via the destruction of the mattic epipedon layer at the interface between bald and vegetation patches (Chen et al., 2017) to form desertification lands and thermokarst lakes (Wang et al., 2018).
In arid regions, self-organized patchiness is the resultant effect of positive feedback between vegetation growth and water availability (Chen et al., 2017). Higher vegetation density enhances water infiltration and lowers its evaporation (Qin et al., 2019). On the Qilian Mountains, alpine swamp meadow and alpine meadow grasslands are primarily located within sub-stable and transition permafrost fields which have high soil moisture and low temperature while alpine steppe meadow and alpine steppe grasslands are mainly situated in unstable permafrost and seasonal frozen soil areas which have low soil moisture and high soil temperature (Wang et al., 2016). The modification of soil hydrothermal attributes induced by surface permafrost-free thaw processes (You et al., 2017) may account for the form and patchiness of the vegetation. In addition, the major kind of land use of the alpine grassland is grazing which also serves as a habitat for wild mammals. The overall effect of grazing, trampling by livestock, and burrowing activities of small mammals are regarded to be contributing factors to the formation of patchiness (Gao et al., 2010; Qin et al., 2018). Soil texture and topography can alter the redistribution of soil water content and hence affect vegetation patterns (Hais et al., 2016; Zhang and Yao, 2016). Therefore, soils with drier surfaces are not optimal for the survival of continuous vegetation patches (Qin et al., 2015; Chen et al., 2017).
Effects of patchiness on ecosystem C and N storage
The results of the study indicated that patchiness in the typical alpine grass species led to a considerable decrease in ecosystem carbon and nitrogen storage which are in agreement with studies by Yi et al. (2016) and Zhang et al. (2019). The increasing soil output via erosion exceeds the input of organic matter which may have accounted for the decline in ecosystem carbon and nitrogen storage. This is so because stripped vegetation patches result in increased erosion and gravel content in the soil which is not useful for vegetation growth (Chen et al., 2017). There were significant variations in the decline of ecosystem carbon and nitrogen within the selected patches. Furthermore, there was a substantial decline in ecosystem carbon and nitrogen in KP and LS than in LN and SA (Figure 4D, Figure 5D). The results indicated that ecosystem carbon and nitrogen distribution and storage are mainly reliant on the form and structure of the vegetation. Close to 62% of ecosystem carbon and nitrogen was found in the soil and 38% in vegetation for KP and LS. On the other hand, more than 80% of ecosystem carbon and nitrogen was found in the soil while less than 20% in vegetation for LN and SA. Studies have shown that alpine grassland ecosystem C is highly vulnerable with a significant quantity of easy cycling carbon but has a lesser quantity of resistant carbon (Chen et al., 2016b). Vegetation degradation leads to increased organic matter decomposition rates as a result of limited moisture and greater availability of oxygen (Wu et al., 2012; Chen et al., 2017). It is a fact that vegetation productivity impacts ecosystem carbon and nitrogen greatly (De Deyn et al., 2009; Zhang et al., 2019) and our findings are consistent with this fact. Our findings show there was a significant positive correlation between ecosystem carbon and nitrogen and plant and root biomass (Figures 7C–F). The decomposition of litter is considered the major source of soil C and N. A substantial decrease in vegetation production due to patchiness accounted for the decline of ecosystem carbon and nitrogen storage. However, there was a significant negative correlation between ecosystem carbon and nitrogen and soil gravel content (Figures 7A,B). These results are in sync with previous studies (Qin et al., 2015; Qin et al., 2018). Also, the results indicate there were significantly high levels of gravel in stripped vegetation patches compared to that of intact vegetation patches (Figure 3B) which are consistent with the findings by Qin et al. (2015). The literature shows that most of the alpine grasslands of QTP have thin soils and contain a significant quantity of gravel (Qin et al., 2015). Grassland soils contain a significant amount of soil organic carbon and total nitrogen in the topsoil and for that matter, ecosystem carbon and nitrogen are susceptible to soil erosion once the soil is exposed (Nie et al., 2013; Liu et al., 2012). As soon as the topsoil is carried away by water and wind erosion, ecosystem carbon and nitrogen are lost (Lin et al., 2014). During the growing season, runoff is capable of carrying away significant nutrients and top tiny pieces of soil due to less plant biomass in stripped vegetation patches (Bertol et al., 2003). This leads to the expansion of stripped vegetation patches and its resultant effect on vegetation productivity, hence, is a decline in ecosystem carbon and nitrogen storage. Bald patches facilitate the establishment of biological and physical crusts (Assouline et al., 2015). The bald patches reduce water infiltration ability and worsen water stress of the remaining vegetation (Thompson et al., 2010). Degraded grasslands are extremely fragmented with the area of grassland patches endlessly reducing while their spatial isolations increase (Krauss et al., 2004). Habitat fragmentation is a threat to biodiversity and leads to a reduction in species richness within small and isolated habitat patches (Ouborg et al., 2006). Large intact vegetation patches are certainly a vital key for the maintenance of some vital ecological processes (Watson et al., 2018) and biodiversity conservation (Gibson et al., 2011). Nonetheless, Wintle et al. (2018) indicated that several species could be lost if small, isolated patches of remnant habitats were ignored and conservation efforts centered mainly on large, intact, and highly connected areas. The make-up and functionality of the soil microbiome are governed by soil abiotic factors such as pH, texture, and nutrient and moisture availabilities (Maestre et al., 2012; Maestre et al., 2015; Ochoa-Hueso et al., 2018), which are susceptible to soil erosion. Erosion is regarded to negatively affect microbial biomass abundance, and make-up by modifying natural soil features and taking away the vegetation protective cover (Mabuhay et al., 2004; Li et al., 2015; Hou et al., 2014). Successively, all of these modifications may change the turnover and availability of soil nutrients and soil functionality.
To combat degradation and promote restoration, socio-ecological solutions are required. Increasing grassland awareness in global policy; generating standardized degradation indicators; leveraging scientific innovation for effective restoration at regional and landscape sizes; and improving information transfer and data sharing on restoration experiences are all important measures. The possible ecosystem service trade-offs in degraded and regenerated grasslands can be balanced by systematic assessment and common awareness of stakeholder needs. Integrating these efforts into a sustainability strategy will help to slow degradation and improve restoration success, while also protecting the socioeconomic, cultural, and ecological benefits that grasslands bring (Bardgett et al., 2021).
To improve the assessment of grassland deterioration and propose unique methods for restoration and sustainable grassland management, environmental research innovations must be created and implemented. Remote sensing advances on a global and regional scale have the ability to assess the extent and condition of grasslands and inform the spatial targeting of large-scale restoration operations. For example, maps of general degradation indicators, such as primary productivity, standing biomass, soil moisture, phenology, and soil organic carbon (Bardgett et al., 2021; Schweiger et al., 2018) could be combined with spatial information on climate, edaphic, and socio-economic data to identify national and global patterns of grassland degradation and pinpoint locations where restoration efforts could have the greatest impact.
The accumulation of SOC with grassland development promotes ecosystem nitrogen retention, resulting in the accumulation of soil total nitrogen. The increased vegetation biomass and decreased soil erosion in long-term vegetation restoration lead to an increase in above-ground and underground carbon inputs, which may be the main factors contributing to soil carbon and nitrogen sequestration (Bardgett et al., 2021). Paddock-scale measurements over 10 years with variable weather conditions in Switzerland also highlighted the need to minimize fallow periods following sward renewal to avoid carbon losses (Ammann et al., 2020). McNally et al. (2015) estimated that increased root mass and rooting depth for a sward with seven species compared with conventional ryegrass/clover could increase soil carbon inputs to a depth of 0.3 m by up to 1.2 tC ha−1. Moderate grazing, dung returns, introducing legumes, increasing sward diversity rotational grazing, and lower grazing or cutting intensity can minimize carbon losses, maintain carbon stocks, and mitigate greenhouse gas emissions (Wang and Fang, 2009; Whitehead, 2020).
Effect of stripped vegetation on soil physicochemical properties
Stripped vegetation could cause micro-climate features such as soil temperature, water, availability of light, airflow, and nutrients, affect plant physiological processes, and finally influence the growth of individual plant communities (Anderson and Leopold, 2002). The micro-climate is modified by stripped vegetation which in turn influence above and below-ground productivity and soil microbial decomposers, which may finally affect soil chemical properties (Sariyildiz, 2008). Our results show that soil TP, TK, and AP were higher in vegetation patches than in the medium and large stripped vegetation patches. The decreased litter decomposition in the large stripped vegetation patches could account for the reduction in soil P and K. Furthermore, the decrease in litter/root decomposition and the enhanced nutrient volatilization in large stripped vegetation patches tend to decrease soil P, K, and N concentrations (Scharenbrock and Bockheim, 2008). The results of this study are in line with that of Zhou et al. (2017). The lower plant biomass and its attendant low SOC in the stripped vegetation patches could account for the low MBC and MBN. Soil erosion takes away the top fertile soil and nutrients which support microbial habitats and soil ecosystem services resulting in a reduction in productivity (Van Oost and Bakker, 2012; FAO, ITPS, 2015). Grassland degradation can influence soil microbes in several ways. It often decreases SOC greatly (Liu et al., 2018), which in turn decreases microbial abundance and subdues soil microbial activity.
Conclusion
The research examined the features of grassland patches and estimated the effects of patchiness on ecosystem carbon and nitrogen storage via a field survey. The results indicated that there were variations in patchiness among the different typical alpine grass species patches. Our results suggested that stripped vegetation patches led to an increase in soil gravel content. Also, patchiness led to a substantial decline in ecosystem carbon and nitrogen. The reduction in plant biomass input and the increase in soil erosion due to stripped vegetation patches accounted for the vast decline in ecosystem carbon and nitrogen.
Data availability statement
The raw data supporting the conclusion of this article will be made available by the authors, without undue reservation.
Author contributions
TA, WC, and SH formulated the idea and designed the study. TA and SH performed the fieldwork. TA, CW, WL, and FS performed the data analysis. TA wrote the manuscript and RO and CW revised the manuscript with advice from all authors. All authors contributed to the manuscript and approved the submitted version.
Funding
This study was funded by the National Natural Science Foundation of China (32060269) and China Agricultural Research System of MOF and MARA (CARS-34).
Acknowledgments
The authors would like to thank the research team of the College of Grassland Science of the Gansu Agricultural University.
Conflict of interest
The authors declare that the research was conducted in the absence of any commercial or financial relationships that could be construed as a potential conflict of interest.
Publisher’s note
All claims expressed in this article are solely those of the authors and do not necessarily represent those of their affiliated organizations, or those of the publisher, the editors, and the reviewers. Any product that may be evaluated in this article, or claim that may be made by its manufacturer, is not guaranteed or endorsed by the publisher.
References
Akiyama, T., and Kawamura, K. (2007). Grassland degradation in China: Methods of monitoring, management and restoration. Grassl. Sci. 53 (1), 1–17. doi:10.1111/j.1744-697x.2007.00073.x
Ammann, C., Neftel, A., Jocher, M., Fuhrer, J., and Leifeld, J. (2020). Effect of management and weather variations on the greenhouse gas budget of two grasslands during a 10-year experiment. Agric. Ecosyst. Environ. 292, 106814. doi:10.1016/j.agee.2019.106814
Anderson, K. L., and Leopold, D. J. (2002). The role of canopy gaps in maintaining vascular plant diversity at a forested wetland in New York State. J. Torrey Bot. Soc. 129 (3), 238–250. doi:10.2307/3088774
Assouline, S., Thompson, S. E., Chen, L., Svoray, T., Sela, S., and Katul, G. G. (2015). The dual role of soil crusts in desertification. J. Geophys. Res-Biogeo. 120 (10), 2108–2119. doi:10.1002/2015JG003185
Babel, W., Biermann, T., Coners, H., Falge, E., Seeber, E., Ingrisch, J., et al. (2014). Pasture degradation modifies the water and carbon cycles of the Tibetan highlands. Biogeosciences 11, 6633–6656. doi:10.5194/bg-11-6633-2014
Bao, G. S., Wang, Y. Q., Song, M. L., Wang, H. S., Yin, Y. L., Liu, S. C., et al. (2019). Effects of Stellera chamaejasme patches on the surrounding grassland community and on soil physical-chemical properties in degraded grasslands susceptible to S. chamaejasme invasion. Acta Prataculturae Sin. 28, 51–61. doi:10.11686/cyxb2018205
Bardgett, R. D., Bullock, J., M., Lavorel, S., Manning, P., Schaffner, U., Ostle, N., et al. (2021). Combatting global grassland degradation. Nat. Rev. Earth Environ. 2, 720–735. doi:10.1038/s43017-021-00207-2
Bertol, I., Mello, E. L., Guadagnin, J. C., Zaparolli, A. L. V., and Carrafa, M. R. (2003). Nutrient losses by water erosion. Sci. Agric. 60 (3), 581–586. doi:10.1590/s0103-90162003000300025
Chen, H., Zhu, Q., Peng, C., Wu, N., Wang, Y., Fang, X., et al. (2013). The impacts of climate change and human activities on biogeochemical cycles on the Qinghai-Tibetan Plateau. Glob. Chang. Biol. 19, 2940–2955. doi:10.1111/gcb.12277
Chen, J., Yi, S., and Qin, Y. (2017). The contribution of plateau pika disturbance and erosion on patchy alpine grassland soil on the Qinghai-Tibetan plateau: Implications for grassland restoration. Geoderma 297, 1–9. doi:10.1016/j.geoderma.2017.03.001
Chen, L., Liang, J., Qin, S., Liu, L., Fang, K., Xu, Y., et al. (2016b). Determinants of carbon release from the active layer and permafrost deposits on the Tibetan Plateau. Nat. Commun. 7, 13046. doi:10.1038/ncomms13046
Dai, L., Yuan, Y., Guo, X. W., Du, Y., Ke, X., Zhang, F., et al. (2020). Soil water retention in alpine meadows under differentdegradation stages on the northeastern Qinghai-Tibet. J. Hydrol. 590 (1), 125397. doi:10.1016/j.jhydrol.2020.125397
Dakos, V., Matthews, B., Hendry, A. P., Levine, J., Loeuille, N., Norberg, J., et al. (2019). Ecosystem tipping points in an evolving world. Nat. Ecol. Evol. 3, 355–362. doi:10.1038/s41559-019-0797-2
De Deyn, G. B., Quirk, H., Yi, Z., Oakley, S., Ostle, N. J., and Bardgett, R. D. (2009). Vegetation composition promotes carbon and nitrogen storage in model grassland communities of contrasting soil fertility. J. Ecol. 97 (5), 864–875. doi:10.1111/j.1365-2745.2009.01536.x
Dong, Q. M., Zhao, X. Q., Wu, G. L., Shi, J. J., and Ren, G. H. (2013). A review of formation mechanism and restoration measures of black-soil-type degraded grassland in the QinghaiTibetan Plateau. Environ. Earth Sci. 70, 2359–2370. doi:10.1007/s12665-013-2338-7
FAO, ITPS (2015). Status of the world’s soil resources - main report. Rome, Italy. Food and Agriculture Organization of the United Nations and Intergovernmental Technical Panel on Soils. Available at: https://www.fao.org/3/a-i5199e.pdf (Accessed December 31, 2021).
Freschet, G. T., Violle, C., Bourget, M. Y., Scherer-Lorenzen, M., and Fort, F. (2018). Allocation, morphology, physiology, architecture: The multiple facets of plant above- and below-ground responses to resource stress. New Phytol. 219 (4), 1338–1352. doi:10.1111/nph.15225
Gang, C., Zhou, W., Chen, Y., Wang, Z., Sun, Z., Li, J., et al. (2014). Quantitative assessment of the contributions of climate change and human activities on global grassland degradation. Environ. Earth Sci. 72, 4273–4282. doi:10.1007/s12665-014-3322-6
Gao, Q., Li, Y., Wan, Y., Qin, X., Jiangcun, W., and Liu, Y. (2009). Dynamics of alpine grassland NPP and its response to climate change in Northern Tibet. Clim. Change 97, 515–528. doi:10.1007/s10584-009-9617-z
Gao, Q. Z., Wan, Y. F., Li, Y., Qin, X. B., Jiangcun, W. Z., and Xu, H. M. (2010). Spatial and temporal pattern of alpine grassland condition and its response to human activities in Northern Tibet. China. Rangel. J. 32, 165–173. doi:10.1071/rj08061
Gibson, L., Lee, T. M., Koh, L. P., Brook, B. W., Gardner, T. A., Barlow, J., et al. (2011). Primary forests are irreplaceable for sustaining tropical biodiversity. Nature 478, 378–381. doi:10.1038/nature10425
Haddad, N. M., Brudvig, L. A., Clobert, J., Davies, K. F., Gonzalez, A., Holt, R. D., et al. (2015). Habitat fragmentation and its lasting impact on earth's ecosystems. Sci. Adv. 1, e1500052. doi:10.1126/sciadv.1500052
Hais, M., Chytrý, M., and Horsak, M. (2016). Exposure-related forest-steppe: Adiverse landscape type determined by topography and climate. J. Arid. Environ. 135, 75–84. doi:10.1016/j.jaridenv.2016.08.011
Heras, M. M., Saco, P. M., Willgoose, G. R., and Tongway, D. (2011). Assessing landscape structure and pattern fragmentation in semiarid ecosystems using patch-size distributions. Ecol. Appl. 21, 2793–2805. doi:10.1890/10-2113.1
Hopping, K. A., Knapp, A. K., Dorji, T., and Klein, J. A. (2018). Warming and land use change concurrently erode ecosystem services in Tibet. Glob. Chang. Biol. 24, 5534–5548. doi:10.1111/gcb.14417
Hou, S., Xin, M., Wang, L. L., Jiang, H., Li, N., and Wang, Z. (2014). The effects of erosion on the microbial populations and enzyme activity in black soil of northeastern China. Acta Ecol. Sin. 34, 295–301. doi:10.1016/j.chnaes.2014.10.001
Jenkinson, D. S. (1988). “The determination of microbial biomass carbon and nitrogen in soil,” in Advances in nitrogen cycling in agricultural ecosystems. Editor J. R. Wilson (Wallingford: CAB international).
Jia, J., Feng, X., He, J. S., He, H., Lin, L., and Liu, Z. (2017). Comparing microbial carbon sequestration and priming in the subsoil versus topsoil of a Qinghai-Tibetan alpine grassland. Soil Biol. Biochem. 104, 141–151. doi:10.1016/j.soilbio.2016.10.018
Joergensen, R. G. (1996). The fumigation-extraction method to estimate soil microbial biomass: Calibration of the KEC value. Soil Biol. Biochem. 28, 25–31. doi:10.1016/0038-0717(95)00102-6
Krauss, J., Klein, A-M., Stefan-Dewenter, I., and Tscharntke, T. (2004). Effects of habitat area, isolation, and landscape diversity on plant species richness of calcareous grasslands. Biodivers. Conserv. 13, 1427–1439. doi:10.1023/b:bioc.0000021323.18165.58
Li, X. L., Gao, J., Brierley, G., Qiao, Y. M., Zhang, J., and Yang, Y. W. (2013). Rangeland degradation on the Qinghai-Tibet plateau: Implications for rehabilitation. Land Degrad. Dev. 24, 72–80. doi:10.1002/ldr.1108
Li, Z., Xiao, H., Tang, Z., Huang, J., Nie, X., Huang, B., et al. (2015). Microbial responses to erosion-induced soil physico-chemical property changes in the hilly red soil region of southern China. Eur. J. Soil Biol. 71, 37–44. doi:10.1016/j.ejsobi.2015.10.003
Lin, H. L., Wang, Z. Q., and Shang, Z. H. (2010). Features on fractal dimension of barren patch and mouse hole among different degenerated succession stages on alpine meadow in the Source Region of the Yangtze and Yellow River, Qinghai-Tibetan Plateau. China. Acta Agrestia Sin. 18, 477–484. doi:10.11733/j.issn.1007-0435.2010.04.001
Lin, Y., Cui, P., Ge, Y., Chen, C., Wang, D., Wu, C., et al. (2014). The succession characteristics of soil erosion during different vegetation succession stages in dry-hot river valley of Jinsha River upper reaches of Yangtze River. Ecol. Eng. 62, 13–26. doi:10.1016/j.ecoleng.2013.10.020
Liu, S. B., Zamanian, K., Schleuss, P. M., Zarebanadkouki, M., and Kuzyakov, Y. (2018b). Degradation of Tibetan grasslands: Consequences for carbon and nutrient cycles. Agric. Ecosyst. Environ. 252, 93–104. doi:10.1016/j.agee.2017.10.011
Liu, W., Chen, S., Qin, X., Baumann, F., Scholten, T., Zhou, Z., et al. (2012). Storage, patterns, and control of soil organic carbon and nitrogen in the northeastern margin of the Qinghai-Tibetan Plateau. Environ. Res. Lett. 7, 035401. doi:10.1088/1748-9326/7/3/035401
Lozano-García, B., Parras-Alcántara, L., and Brevik, E. C. (2016). Impact of topographic aspect and vegetation (native and reforested areas) on soil organic carbon and nitrogen budgets in Mediterranean natural areas. Sci. Total Environ. 544, 963–970. doi:10.1016/j.scitotenv.2015.12.022
Lü, L., Wang, R., Liu, H., Yin, J., Xiao, J., Wang, Z., et al. (2016). Effect of soil coarseness on soil base cations and available micronutrients in a semi-arid sandy grassland. Solid 7, 549–556. doi:10.5194/se-7-549-2016
Mabuhay, J. A., Nakagoshi, N., and Isagi, Y. (2004). Influence of erosion on soil microbial biomass, abundance and community diversity. Land Degrad. Dev. 15, 183–195. doi:10.1002/ldr.607
Maestre, F. T., Delgado-Baquerizo, M., Jeffries, T. C., Eldridge, D. J., Ochoa, V., Gozalo, B., et al. (2015). Increasing aridity reduces soil microbial diversity and abundance in global drylands. Proc. Natl. Acad. Sci. U. S. A. 112, 15684–15689. doi:10.1073/pnas.1516684112
Maestre, F. T., Quero, J. L., Gotelli, N. J., Escudero, A., Ochoa, V., Delgado-Baquerizo, M., et al. (2012). Plant species richness and ecosystem multifunctionality in global drylands. Science 335, 214–218. doi:10.1126/science.1215442
McNally, S. R., Laughlin, D. C., Rutledge, S., Dodd, M. B., Six, J., and Schipper, L. A. (2015). Root carbon inputs under moderately diverse sward and conventional ryegrass -clover pasture: Implications for soil carbon sequestration. Plant Soil 392, 289–299. doi:10.1007/s11104-015-2463-z
Miehe, G., Schleuss, P. M., Seeber, E., Babel, W., Biermann, T., Braendle, M., et al. (2019). The Kobresia pygmaea ecosystem of the Tibetan highlands – origin, functioning and degradation of the world's largest pastoral alpine ecosystem. Sci. Total Environ. 648, 754–771. doi:10.1016/j.scitotenv.2018.08.164
Nie, X. J., Zhao, T. Q., and Qiao, X. N. (2013). Impacts of soil erosion on organic carbon and nutrient dynamics in an alpine grassland soil. Soil Sci. Plant Nutr. 59, 660–668. doi:10.1080/00380768.2013.795475
O'Mara, F. P. (2012). The role of grassland in food security and climate change. Ann. Bot. 110 (6), 1263–1270. doi:10.1093/aob/mcs209
Ochoa-Hueso, R., Collins, S. L., Delgado-Baquerizo, M., Hamonts, K., Pockman, W. T., Sinsabaugh, R. L., et al. (2018). Drought consistently alters the composition of soil fungal and bacterial communities in grasslands from two continents. Glob. Chang. Biol. 24, 2818–2827. doi:10.1111/gcb.14113
Ouborg, N. J., Vergeer, P., and Mix, C. (2006). The rough edges of the conservation genetics paradigm for plants. J. Ecol. 94, 1233–1248. doi:10.1111/j.1365-2745.2006.01167.x
Pineiro, G., Paruelo, J. M., and Oesterheld, M. (2006). Potential long-term impacts of livestock introduction on carbon and nitrogen cycling in grasslands of Southern South America. Glob. Chang. Biol. 12, 1267–1284. doi:10.1111/j.1365-2486.2006.01173.x
Qi, Q., Zhang, D., Zhang, M., Tong, S., Wang, W., and An, Y. (2021). Spatial distribution of soil organic carbon and total nitrogen in disturbed Carex tussock wetland. Ecol. Indic. 120, 106930. doi:10.1016/j.ecolind.2020.106930
Qin, Y., Yi, S., Chen, J., Ren, S., and Ding, Y. (2015). Effects of gravel on soil and vegetation properties of alpine grassland on the Qinghai-Tibetan Plateau. Ecol. Eng. 74, 351–355. doi:10.1016/j.ecoleng.2014.10.008
Qin, Y., Yi, S., Ding, Y., Zhang, W., Qin, Y., Chen, J., et al. (2019). Effect of plateau pika disturbance and patchiness on ecosystem carbon emissions in alpine meadow in the northeastern part of Qinghai-Tibetan Plateau. Biogeosciences 16, 1097–1109. doi:10.5194/bg-16-1097-2019
Qin, Y., Yi, S., Ren, S., Li, N., and Chen, J. (2014). Responses of typical grasslands in a semi-arid basin on the Qinghai-Tibetan Plateau to climate change and disturbances. Environ. Earth Sci. 71, 1421–1431. doi:10.1007/s12665-013-2547-0
Qin, Y., Yia, S., Ding, Y., Xua, G., Chen, J., and Wang, Z. (2018). Effects of small-scale patchiness of alpine grassland on ecosystem carbon and nitrogen accumulation and estimation in northeastern Qinghai-Tibetan Plateau. Geoderma 318, 52–63. doi:10.1016/j.geoderma.2017.12.007
Sariyildiz, T. (2008). Effects of gap-size classes on long-term litter decomposition rates of beech, oak and chestnut species at high elevations in Northeast Turkey. Ecosystems 11, 841–853. doi:10.1007/s10021-008-9164-x
Scharenbroch, B. C., and Bockheim, J. G. (2008). The effects of gap disturbance on nitrogen cycling and retention in late-successional northern hardwood–hemlock forests. Biogeochemistry 87, 231–245. doi:10.1007/s10533-008-9180-0
Scheffer, M., Bascompte, J., Brock, W. A., Brovkin, V., Carpenter, S. R., Dakos, V., et al. (2009). Early-warning signals for critical transitions. Nature 461, 53–59. doi:10.1038/nature08227
Schweiger, A. K., Cavender-Bares, J., Townsend, P. A., Hobbie, S. E., Madritch, M. D., Wang, R., et al. (2018). Plant spectral diversity integrates functional and phylogenetic components of biodiversity and predicts ecosystem function. Nat. Ecol. Evol. 2, 976–982. doi:10.1038/s41559-018-0551-1
Shrestha, G., and Stahl, P. D. (2008). Carbon accumulation and storage in semi-arid sagebrushsteppe: Effects of long-term grazing exclusion. Agric. Ecosyst. Environ. 125, 173–181. doi:10.1016/j.agee.2007.12.007
Sparks, D. L., Page, A. L., Helmke, P. A., Loeppert, R. H., Soltanpour, P. N., Tabatabai, M. A., et al. (1996). Methods of soil analysis. Part 3 – chemical methods. Madison: Soil Science Society of America, American Society of Agronomy.
Thompson, S. E., Harman, C. J., Heine, S., and Katul, G. G. (2010). Vegetation-infiltration relationships across climatic and soil type gradients. J. Geophys. Res. 115, G02023. doi:10.1029/2009JG001134
Van Oost, K., and Bakker, M. M. (2012). “Soil productivity and erosion,” in Soil ecology and ecosystem services. D. H. Wall, R. D. Bardgett, V. Behan-Pelletier, J. E. Herrick, H. Jones, and K. Ritz (Oxford, UK: Oxford University Press), 301–314.
Wang, D., Wu, G. L., Zhu, Y. J., and Shi, Z. H. (2014). Grazing exclusion effects on above- and below-ground C and N pools of typical grassland on the Loess Plateau (China). Catena 123, 113–120. doi:10.1016/j.catena.2014.07.018
Wang, S., Wang, X., and Ouyang, Z. (2012). Effects of land use, climate, topography and soil properties on regional soil organic carbon and total nitrogen in the Upstream Watershed of Miyun Reservoir, North China. J. Environ. Sci. 24, 387–395. doi:10.1016/S1001-0742(11)60789-4
Wang, W., and Fang, J. (2009). Soil respiration and human effects on global grasslands. Glob. Planet. Change 67, 20–28. doi:10.1016/j.gloplacha.2008.12.011
Wang, X., Yi, S., Wu, Q., Yang, K., and Ding, Y. (2016). The role of permafrost and soil water in distribution of alpine grassland and its NDVI dynamics on the Qinghai-Tibetan Plateau. Glob. Planet. Change 147, 40–53. doi:10.1016/j.gloplacha.2016.10.014
Wang, Y., Sun, Z., and Sun, Y. (2018). Effects of a thaw slump on active layer in permafrost regions with the comparison of effects of thermokarst lakes on the Qinghai-Tibet plateau, China. Geoderma. 314, 47–57. doi:10.1016/j.geoderma.2017.10.04
Watson, J. E. M., Evans, T., Venter, O., Williams, B., Tulloch, A., Stewart, C., et al. (2018). The exceptional value of intact forest ecosystems. Nat. Ecol. Evol. 2, 599–610. doi:10.1038/s41559-018-0490-x
Whitehead, D. (2020). Management of grazed landscapes to increase soil carbon stocks in temperate, dryland grasslands. Front. Sustain. Food Syst. 4, 585913. doi:10.3389/fsufs.2020.585913
Wintle, B. A., Kujala, H., Whitehead, A., Cameron, A., Veloz, S., Kukkala, A., et al. (2018). Global synthesis of conservation studies reveals the importance of small habitat patches for biodiversity. Proc. Natl. Acad. Sci. U. S. A. 116, 909–914. doi:10.1073/pnas.1813051115
Wu, J., Joergensen, R. G., Pommerening, B., Chaussod, R., and Brookes, P. C. (1990). Measurement of soil microbial biomass C by fumigation-extraction—An automated procedure. Soil Biol. Biochem. 20, 1167–1169. doi:10.1016/0038-0717(90)90046-3
Wu, X., Z., Li, B., Fu, W., Zhou, H., and Liu, G. (2014a). Restoration of ecosystem carbon and nitrogen storage and microbial biomass after grazing exclusion in semi-arid grasslands of Inner Mongolia. Ecol. Eng. 73, 395–403. doi:10.1016/j.ecoleng.2014.09.077
Wu, X. D., Zhao, L., Chen, M. J., Fang, H. B., Yue, G. Y., Chen, J., et al. (2012). Soil organic carbon and its relationship to vegetation communities and soil properties in permafrost areas of the central Western Qinghai-Tibet plateau, China. Permafr. Periglac. Process. 23, 162–169. doi:10.1002/ppp.1740
Yan, Y., Xin, X., Xu, X., Wang, X., Yan, R., and Murray, P. J. (2016). Vegetation patches increase wind-blown litter accumulation in a semi-arid steppe of northern China. Environ. Res. Lett. 11, 124008. doi:10.1088/1748-9326/11/12/124008
Yao, Y., and Zhen, C. (2017). Qilian Mountains in the Northern Qinghai-Tibet Plateau with glaciers in the distance sustaining an intermittent stream. Geophys. Res. Lett. doi:10.1002/grl.54866
Yi, S., Chen, J., Qin, Y., and Xu, G. (2016). The burying and grazing effects of plateau pika on alpine grassland are small: A pilot study in a semiarid basin on the qinghai-tibet plateau. Biogeosciences 13, 6273–6284. doi:10.5194/bg-13-6273-2016
Yin, G., Niu, F., Lin, Z., Luo, J., and Liu, M. (2017). Effects of local factors and climate on permafrost conditions and distribution in Beiluhe basin, Qinghai-Tibet Plateau, China. Sci.Total Environ. 581-582, 472–485. doi:10.1016/j.scitotenv.2016.12.155
You, Q., Xue, X., Peng, F., Dong, S., and Gao, Y. (2017). Surface water and heat exchange comparison between alpine meadow and bare land in a permafrost region of the Tibetan Plateau. Agric. For. Meteorol. 232, 48–65. doi:10.1016/j.agrformet.2016.08.004
You, Y., Wang, J., Huang, X., Tang, Z., Liu, S., and Sun, O. J. (2014). Relating microbial community structure to functioning in forest soil organic carbon transformation and turnover. Ecol. Evol. 4, 633–647. doi:10.1002/ece3.969
Zhang, B. P., and Yao, Y. H. (2016). Implications of mass elevation effect for the altitudinal patterns of global ecology. J. Geogr. Sci. 26, 871–877. doi:10.1007/s11442-016-1303-2
Zhang, L., Unteregelsbacher, S., Hafner, S., Xu, X., Schleuss, P. M., Miehe, G., et al. (2016). Fate of organic and inorganic nitrogen in crusted and non-crusted Kobresia grasslands. Land Degrad. Dev. 28, 166–174. doi:10.1002/ldr.2582
Zhang, X. L., Wang, S. J., Zhang, J. M., Wang, G., and Tang, X. Y. (2015). Temporal and spatial variability inprecipitation trends in the Southeast Tibetan Plateau during 1961–2012. Clim. Past Discuss. 11 (1), 447–487. doi:10.5194/cpd-11-447-2015
Zhang, X. Y., Liu, M. Z., Zhao, X., Li, Y. Q., Zhao, W., Li, A., et al. (2018). Topography and grazing effects on storage of soil organic carbon and nitrogen in the northern China grasslands. Ecol. Indic. 93, 45–53. doi:10.1016/j.ecolind.2018.04.068
Zhang, Y., Tang, S., Xie, S., Liu, K., Li, J., Chen, Q., et al. (2019). Effects of species-dominated patches on soil organic carbon and total nitrogen storage in a degraded grassland in China. Peer. J. 7, e6897. doi:10.7717/peerj.6897
Keywords: alpine grassland, stripped vegetation patches, ecosystem C and N, ecosystem respiration, intact patch, vegetation input
Citation: Abalori TA, Cao W, Atogi-Akwoa Weobong C, Sam FE, Li W, Osei R and Wang S (2022) Effects of vegetation patchiness on ecosystem carbon and nitrogen storage in the alpine grassland of the Qilian Mountains. Front. Environ. Sci. 10:879717. doi: 10.3389/fenvs.2022.879717
Received: 20 February 2022; Accepted: 21 July 2022;
Published: 01 September 2022.
Edited by:
Jane Addison, James Cook University, AustraliaReviewed by:
Yang Yu, Beijing Forestry University, ChinaPeng Shi, Xi’an University of Technology, China
Copyright © 2022 Abalori, Cao, Atogi-Akwoa Weobong, Sam, Li, Osei and Wang. This is an open-access article distributed under the terms of the Creative Commons Attribution License (CC BY). The use, distribution or reproduction in other forums is permitted, provided the original author(s) and the copyright owner(s) are credited and that the original publication in this journal is cited, in accordance with accepted academic practice. No use, distribution or reproduction is permitted which does not comply with these terms.
*Correspondence: Wenxia Cao, Y2Fvd2VueGlhQGZveG1haWwuY29t