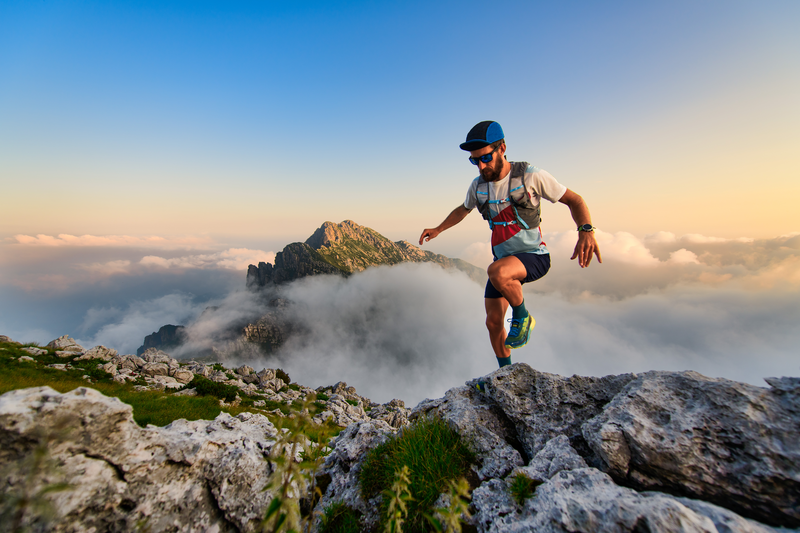
95% of researchers rate our articles as excellent or good
Learn more about the work of our research integrity team to safeguard the quality of each article we publish.
Find out more
ORIGINAL RESEARCH article
Front. Environ. Sci. , 13 April 2022
Sec. Drylands
Volume 10 - 2022 | https://doi.org/10.3389/fenvs.2022.878466
This article is part of the Research Topic Understanding Soil Wind Erosion and Control Practices in Arid and Semiarid Environments View all 10 articles
Dust storms bring a large quantity of dust aerosols from arid and semi-arid regions of the Earth. However, real-time dust concentration data for dust storms in arid regions, important for wind erosion studies, are still limited. Here, temporal variation of airborne dust concentrations in the Mogao Grottoes, Northwest China, during the monitoring period from February to October 2012 and typical dust storms under different wind directions were analyzed. Results reveal that the monthly mean total suspended particle (TSP), particulate matter less than 10 μm (PM10), and particulate matter less than 2.5 μm (PM2.5) concentrations gradually decreased from February to October. The daily mean dust concentrations fluctuated with the day, with extreme values occurring mainly on February, March, and April. The daily mean PM10/TSP ratio ranged from 0.67 to 0.98 (mean of 0.82), and the PM2.5/PM10 ratio ranged from 0.31 to 0.73 (mean of 0.55), indicating PM10 dominated in TSP. In typical dust storms, the maximum real-time concentrations can reach 16, 000–21, 000 μg TSP m−3, ∼11, 000 μg PM10 m−3, and 3000–3500 μg PM2.5 m−3, and extreme real-time dust concentrations of 50358.03 μg TSP m−3, 33100.86 μg PM10 m−3, and 7502.59 μg PM2.5 m−3 were recorded. Coincidence of the dry climate and windy season and sufficient dust sources in the surrounding environment contributed to the high dust concentrations in the Mogao Grottoes. To achieve the goal of minimizing the damage of falling dust to the murals and statues, establishing a dust storm early warning system and improving the existing sand control system are recommended.
Dust storms raise large quantities of dust from arid and semi-arid regions on Earth, which contributes more than half of the total global aerosol burden, and the dust can be transported over 1000s of kilometers and deposited downwind by wet and dry processes (Choobari et al., 2014). Mineral dust affects the Earth and Mars systems through a large variety of interactions (Kok et al., 2012). As an important kind of catastrophic weather, dust storms not only cause great losses to industrial and agricultural production and people’s lives and property, but also pollute the environment and have an important impact on climate change (Goudie, 2009; Choobari et al., 2014). In China, dust storms are common, especially in north and north-west regions (Zhang et al., 1997; Wang X. et al., 2008) and mainly in spring (Zhou and Wang, 2002), which is one of significant global dust sources. For example, from March 14 to 16, 2021, an extremely strong dust storm occurred in the northern region of China, with a distribution area of more than 3.8 million km2, and the peak concentration of PM10 reached over 5000 μg m−3 in many areas of northern China (Yang et al., 2021; Zhang et al., 2022). In addition to Mongolia, the northern and northwestern sandy regions of China are also important dust sources (Liu et al., 2022). As estimated, an annual dust quantity of approximately 800 Tg Chinese desert dust was injected into the atmosphere, and it was almost as much as half of the global production of dust (Zhang et al., 1997).
Much recent advances have been made in our understanding of global dust sources, transport paths, their impacts both on humans and the environment, and their mass concentration, chemical composition, and optical properties (Goudie, 2009), of which the variation characteristics of airborne dust concentrations during dusty weathers is very important in aeolian research, which can help to identify dust source areas (Goudie and Middleton, 2006). However, limited studies have examined the variation of dust concentrations during dusty weathers in arid regions (Gillies et al., 1996; Ganor and Foner, 2001; Shen et al., 2004; Rashki et al., 2012; Dahmardeh Behrooz et al., 2017). Furthermore, due to the limitation of dust concentration measuring instruments, the data of dust concentrations, particularly the real-time monitoring data in northwest of China during dust storm episodes was scarce, especially in dust source areas (Wang Y. Q. et al., 2008). For example, vacuum pump-based devices or high volume samplers (HVS), which can collect the total range of particles in the air, were usually used to measure dust concentrations in wind erosion studies; however, data of dust concentrations are time-integrated over the total sampling period, while real-time dust concentrations cannot be provided by these instruments (Baddock et al., 2014). As a whole, the real-time dust concentrations data during dust storms in arid regions of the world are still limited.
The Mogao Grottoes, Dunhuang, China, one of Buddhism’s most revered shrines (Stone, 2008), and surrounded by Sandy and Gobi Deserts, is a typical study area of extremely arid regions where dust storms are common and severe (Figure 1A). Furthermore, dust hazards caused by dust storms have threatened the protection of murals and statues in the caves. The falling dust particles cause serious discoloration and crispness of murals, which seriously affects their artistic effects. The extremely fine dust particles are easy to invade the gaps of murals and plastic pigments (Figure 1B), which are difficult to be removed without damaging the murals. Dust storms are the dominant source of falling dust in the grottoes. As a famous cultural heritage in China and even in the world, dust hazards have become an urgent and severe problem in the protection of Mogao Grottoes murals. Preliminary studies have been performed on the size and mineral composition of falling dust (Qu et al., 1992), TSP concentration (Wang et al., 2006), and the composition of water-soluble ions of aeolian dust in the Mogao Grottoes (Zhang et al., 2007). However, the monitoring data of dust concentrations during dust storms in the Mogao Grottoes district are limited, and at present, studies regarding aeolian research in the Mogao Grottoes mainly focus on aeolian transport over gobi (Zhang et al., 2014; Liu et al., 2011b; Tan et al., 2013, 2016) and dynamic processes of pyramid dunes atop the Mogao Grottoes (Liu et al., 2011a). Consequently, characteristics of the temporal variation of dust (TSP, PM10, and PM2.5) concentrations in the Mogao Grottoes, especially during dust storms, and the controlling factors are still poorly understood.
FIGURE 1. A severe dust storm in the Mogao Grottoes (A) and the painted sculptures in caves are covered by falling dust particles (B).
This study conducted continuous and synchronous observations of wind speeds and total suspended particle (TSP), particulate matter less than 10 μm (PM10), and particulate matter less than 2.5 μm (PM2.5) concentrations from February to October 2012. Temporal variation characteristics of airborne dust concentrations during the monitoring period (monthly and daily means) and typical dust storms with different wind directions (real-time values) in the Mogao Grottoes were analyzed. Factors influencing dust concentrations in the Mogao Grottoes have also been discussed.
The Mogao Grottoes is located approximately 25 km southeast of Dunhuang City, which is an important way station of the ancient Silk Road in western China (Stone, 2008). The 492 decorated caves with 45,000 square meters of murals were excavated on the vertical cliff surface of the alluvial fan terrace on the west bank of the Daquan River in a length of 1680 m between the 4th and 14th centuries C.E., which are also called the “Museum on the Wall.” The Mogao Grottoes was designated as a World Cultural Heritage site by UNESCO in 1987.
The Mogao Grottoes is adjacent to Sanwei Mountain in the east, Mingsha Mountain in the west, Daquan River in the south, and the open gobi in the north (Figure 2 A, B). The grottoes were built on the west bank of the Daquan River, the length of the cliff body was approximately 1680 m, and the height of the cliff body was generally 10–45 m (Figure 2B). The average annual precipitation in Dunhuang City was 37.9 mm, with an average annual potential evaporation of 2087.3 mm (Ba and Wang, 2010). The multi-year average number of dust days in Dunhuang City was 46.5 days, which mainly occurred in spring (Zhou and Wang, 2002). Gobi and mega-dunes are two main landforms in the surrounding area of the Mogao Grottoes. Mingsha Mountain, composed of huge and complex mega-dunes, is located to the west side atop the grottoes, and it is the main sand source threatening the grottoes.
FIGURE 2. Location of the study areas (A,B), the average sand drift potential during 2005–2007 (C), and the seasonal DP over 10 m s−1 (D).
The annual sand drift potential (DP) was calculated using the method proposed by Fryberger and Dean (1979), and the average annual DP on the top of the Mogao Grottoes in 2005–2007 was 136.8 vector units (VU), indicating a low wind energy environment (Figure 2C) (Tan, 2015). There were three main wind directions atop the Mogao Grottoes, that is, easterly, westerly, and southerly winds. Among them, the DP of westerly winds (W, WSW, WNW, and NW) was 28.3 VU, the DP of easterly winds (NNE, NE, ENE, and E) was 48.8 VU, and the DP of southerly winds was 51.5 VU, accounting for 20.7, 35.7, and 37.6% of the annual DP, respectively. The average annual compound sand drift potential (RDP) at the top of the Mogao Grottoes was 21.0 VU, the compound sand drift direction (RDD) was 299.4°, and the RDP/DP value was 0.15, which was a compound wind regime. However, though the southerly winds have the largest frequency, they were mainly in the speed range of 6–10 m s−1. In the speed range of >10 m s−1, the DP of easterly and westerly winds accounted for 57.9 and 24.3% of the total DP in this speed range, respectively, indicating that easterly winds had the strongest power, and the second was westerly winds. Westerly and easterly winds are two main strong winds that cause dust storms dominating in the spring of each year. The largest DP over 10 m s−1 occurred in spring, accounting for 54.6% of the total DP over 10 m s−1, and it was followed by summer, accounting for 28.2% of the total DP over 10 m s−1 (Figure 2D).
A Metstn weather station on the top of the Mogao Grottoes (Figure 2B) was used to measure wind velocity/direction at a height of 2 m above the ground and precipitation (Figure 3A), which were logged synchronously with data of dust concentrations.
FIGURE 3. The Metstn weather station on the top of the Mogao Grottoes (A) and Thermo Scientific™ Partisol™ 2025i Sequential Air Sampler in front of the Grotto 72 (B).
Dust concentrations were measured using the Thermo Scientific Partisol 2025i Sequential Air Sampler (Figure 3B), which was sited in front of Grotto 72 (Figure 2B). The Partisol 2025i was configured to sample a wide range of ambient PM sizes, including TSP, PM10, and PM2.5. The Partisol has a fully automatic filter exchange mechanism that provides unattended monitoring for up to 16 consecutive days for 24-h sampling prior to filter change, and quartz fibre filters have been used for the collection of particulate matter (Charron et al., 2004). Dust concentration data were logged in 2 min, and the pumping inlet of the Air Sampler was mounted at a height of 1.2 m above the ground. Dust concentrations in front of Grotto 72 during February 2012 to October 2012 were continuously monitored. Unfortunately, due to the harsh aeolian environment and strong dust storms in the study area, the Partisol 2025i Sequential Air Sampler malfunctioned and could not continue to work after October 2012.
The monthly mean TSP, PM10, and PM2.5 concentrations during the monitoring period were 4.90–544.63 μg m−3, 4.29–477.92 μg m−3, and 2.78–234.61 μg m−3, respectively (Table 1). The monthly mean value of dust concentrations gradually decreased from February to October, and showed a maximum in February, with TSP, PM10, and PM2.5 concentrations of 544.63, 477.92, and 234.61 μg m−3. The second high concentration value occurred in March, with the TSP, PM10, and PM2.5 concentrations of 371.71, 316.18, and 197.97 μg m−3. In April, the third high concentration value occurred, and the concentration values of TSP, PM10, and PM2.5 were 117.83, 90.42, and 53.24 μg m−3, respectively. Zhou and Wang (2002) revealed that severe dust storms in northwest China usually occurred in spring, especially in April, and winter was the second high season. Cao et al. (2013) showed that the seasonal variation of PM10 concentration at the Dunhuang station during 2007–2011, and it indicated that the PM10 concentration was the highest in spring, lowest in autumn. The monthly mean dust concentrations in the Mogao Grottoes were consistent with this conclusion, and dust concentrations in summer and autumn significantly decreased compared with those in spring.
The daily variations of TSP, PM10, and PM2.5 concentrations show fluctuation with the day, with extreme peaks on certain days (Figure 4). The extreme values of dust concentrations occurred mainly on February, March, and April, when dusty days were common in the Mogao Grottoes district. The daily mean TSP, PM10, and PM2.5 concentrations were in the range of 2.36–3585.53, 1.95–3056.88, 0.69–1368.60 μg m−3, respectively. However, the daily mean background concentration values of TSP, PM10, and PM2.5 were 14.74, 11.99, and 6.12 μg m−3, respectively. Thus, the maximum daily dust concentration values in dusty days could reach approximately 70 times of those during dust-free days. The maximum daily concentration values occurred in February, while the minimum values in October. Furthermore, the maximum daily mean dust concentrations deceased with each month. For example, the maximum daily mean TSP, PM10, and PM2.5 concentrations in February were 3585.53, 3056.88, 1368.60 μg m−3, respectively, while in March they deceased to 2442.60, 2111.78, and 1495.91 μg m−3, and in April, they decreased to 1185.84, 861.77, and 344.69 μg m−3. Based on daily observations from air quality monitoring stations, Filonchyk et al. (2019) analyzed the PM10 and PM2.5 concentrations in typical cities of the northwest China during winter-spring period of dust storms in 2014–2017, and it showed that the peak daily average PM10 and PM2.5 concentrations exceeded 380 and 150 μg m−3, respectively, and the extreme PM10 concentration value of 977 μg m−3 was recorded on 23 April 2014 in Lanzhou, and in Urumqi, the PM10 concentrations reached to 400 μg m−3. Obviously, the daily mean PM10 concentrations in the Mogao Grottoes district were larger than those values in these cities during dusty days, while the daily dust concentrations in this study were comparable with those during dust storms in Iran, such as Zabol, located in the Sistan basin of southeast Iran (Dahmardeh Behrooz et al., 2017) and Ahvaz, southwestern Iran (Shahsavani et al., 2012).
The relationship among the daily mean concentrations of TSP, PM10, and PM2.5 shows that there was a significant positive correlation between TSP, PM10, and PM2.5, that is, PM10 = 0.84TSP, PM2.5 = 0.42TSP, and M2.5 = 0.50PM10, with a determination coefficient R2 over 0.93 (Figure 5). The PM10/TSP ratio ranged from 0.67 to 0.98 with a mean value of 0.82, and the PM2.5/PM10 ratio ranged from 0.31 to 0.73 (mean of 0.55). This result indicates that PM10 is the main component of TSP, and PM2.5 is dominant in PM10 in the Mogao Grottoes. Gholampour et al. (2016) reported that the daily mean PM10/TSP ratios at two sampling sites in Urmia Lake, Northwest Iran, the second great saline lake in the world, ranged from 0.38 to 0.90 and 0.57 to 0.96, respectively, with mean values of 0.64 and 0.75, which was consistent with the ratios in this study. Dahmardeh Behrooz et al. (2017) analyzed TSP and PM10 concentrations in dust events in Zabol, Southeastern Iran and revealed that the PM10/TSP ratio ranged from 0.27 to 0.99 with a mean of 0.37, indicating that particles above 10 μm were dominant in TSP, which were coarser than those in this study. They attributed the higher fraction of coarse particles to the influence of soil-crust material on the airborne samples.
Westerly and easterly winds are two main strong winds that cause dust storms in the Mogao Grottoes district. An episode of dust storms under westerly winds occurring at 11:50–18:10 on 1 April 2012 in the Mogao Grottoes was selected to show the variation characteristics of real-time dust concentrations (Figure 6). During this dust storm, the average wind direction at 2 m above the ground was 286.2°, belonging to WNW winds. The average wind speed at 2 m above the ground was 11.11 m s−1, and the maximum wind speed was 13.37 m s−1 (Figure 6A). When converting it into the wind speed at 10 m according to the wind profile, the maximum wind speed was 15.78 m s−1, indicating that it reached moderate gale. The average TSP, PM10, and PM2.5 concentrations were 3528.81, 2465.49, and 869.22 μg m−3, respectively. Among them, the average ratio of PM10 to TSP concentration was 0.70, and that of PM2.5 to PM10 concentration was 0.35, implying that PM10 was the main composition of TSP during dust storms of westerly winds. The maximum dust concentrations were 21483.32 μg TSP m−3, 11085.17 μg PM10 m−3, and 3028.08 μg PM2.5 m−3 (Figure 6B).
FIGURE 6. Wind speeds at the height of 2 m atop the Mogao Grottoes (A) and the corresponding dust concentrations (B) changing with time under a typical dust storm of westerly winds on 1 April 2012.
A dust storm under easterly winds occurred at 14:00–19:00 on 13 March 2012 in the Mogao Grottoes, and the variation characteristics of real-time dust concentrations during this period were shown in Figure 7. During this dust storm, the average wind direction at 2 m above the ground was 85.4°, belonging to E winds. The average wind speed at 2 m above the ground was 11.33 m s−1, and the maximum wind speed was 14.09 m s−1 (Figure 7A). The corresponding maximum wind speed at 10 m was 16.93 m s−1, and thus it reached the level of moderate gale. The average TSP, PM10, and PM2.5 concentrations were 5002.71, 3745.33, and 1297.48 μg m−3, respectively. Among them, the average PM10/TSP ratio was 0.75, and the average PM2.5/PM10 ratio was 0.35, showing that PM10 was also dominant in dust storms of easterly winds. The maximum dust concentrations in this episode were 15959.97 μg TSP m−3, 11363.59 μg PM10 m−3, and 3591.52 μg PM2.5 m−3 (Figure 7B).
FIGURE 7. Wind speeds at the height of 2 m atop the Mogao Grottoes (A) and the corresponding dust concentrations (B) changing with time under a typical dust storm of easterly winds on 13 March 2012.
A severe dust storm under easterly winds occurred at 21:00–22:00 on 21 February 2012. During this dust storm, the maximum wind speed at 2 m was 15.46 m s−1 (Figure 8A), which was the maximum wind speed in the whole measurement period. The corresponding wind speed at 10 m was 18.75 m s−1, and it reached the level of fresh gale. The extreme dust concentrations reached 50358.03 μg TSP m−3, 33100.86 μg PM10 m−3, and 7502.59 μg PM2.5 m−3 (Figure 8B). The data of real-time dust concentrations during dust storm episodes in China was scarce due to the limitation of PM concentration measuring instruments. The recorded extreme values of TSP concentration over 20,000 μg m−3 and PM10 over 10,000 μg m−3 in dust storms on Earth was relatively limited in the literature. Ganor and Foner (2001) reported one dust storm in Tel Aviv when a TSP concentration of 24,000 μg m−3 was recorded. Gillies et al. (1996) reported a measured peak dust concentration 13735 μg m−3 during an intense dust plume in the Inland Delta region of Mali. Dahmardeh Behrooz et al. (2017) revealed that an extreme daily TSP concentration could reach 10780 μg m−3 over Sistan, Iran during the summer dusty period. Thus, the dust concentrations reported in this study were comparable to those previously obtained in dust storm events over other arid regions in the literature.
FIGURE 8. Wind speeds at the height of 2 m (A) and dust concentrations (B) changing with time in an extreme dust episode on 21 February 2012.
The Mogao Grottoes is located in the extremely arid region, surrounded by Gobi and Sandy Deserts, and suffered from severe dust storms. Due to the harsh aeolian environment and instrument limitation, real-time dust concentration data in dust storm episodes in the Mogao Grottoes were not easy to obtain. In this study, synchronous observations of wind speeds and concentrations of TSP, PM10, and PM2.5 were performed in the Mogao Grottoes from February to October 2012 using a Partisol 2025i Sequential Air Sampler. This study provides significant real-time concentration data of TSP, PM10, and PM2.5 in a typical dust source area of northwest China, which is important for wind erosion research in arid regions. Meanwhile, the monitoring period is representative because in the latest 10 years the variation of the occurrence frequency of dust storms in Dunhuang, northwest China is limited (Luo et al., 2021). In the following Discussion section, the main factors influencing airborne dust concentrations in the Mogao Grottoes have been discussed.
Wind speed is a dynamic factor influencing dust concentrations. The relationship between the concentrations of TSP, PM10, and PM2.5 and wind speed at 2 m can be expressed by a power function (Figure 9):
Where C is dust concentrations (μg m−3), u is wind speeds at a height of 2 m above the ground (m s−1), and a and b are regression coefficients. Under the two dust storms, the power exponents in the power functions for TSP, PM10, and PM2.5 were 2.99, 2.73, and 2.42, respectively. Ta et al. (2003) analyzed the relationship between TSP concentration and wind speed in seven cities of Gansu Province, China and concluded that TSP concentration increased with wind speed. Wang and Zhang (2021) revealed that the PM10 concentration during a dust storm over a gobi surface was positively correlated with wind speed, and compared with that near the surface the correlation at the heights of 60 and 200 cm was weakened by the PM10 from non-local sources. These results are consistent with the results in this study.
FIGURE 9. The relationship between the concentrations of TSP, PM10 and PM2.5 and wind speed during dust storms.
The area of the Mogao Grottoes is under an extremely dry climate, and the annual precipitation in 2012 was 37.1 mm (Figure 10). Meanwhile, the precipitation was mainly concentrated in summer (August, July and June), accounting for 73.3% of the total value. Spring was the driest season in a year, and there was no precipitation recorded in March and April of the study period. Furthermore, the frequency and strength of wind in these months were the highest (Figure 10). As a result, dust concentrations under dust storms in these months are usually the largest, contributing to the falling dust particles in the caves.
The landforms atop the Mogao Grottoes are of four types, namely, sandy gobi, gravel gobi, flat sand sheets, and mega-dunes (Zhang et al., 2014). Mingsha Mountain is composed of huge and complex mega-dunes, located to the west side atop the grottoes, and it is the main sand source threatening the grottoes. Dust particles (<63 μm) can account for 1.5 and 8.1% in mass percentage for sand dunes and gobi surfaces, respectively (Tan, 2015). Under westerly winds, these landforms atop the Mogao Grottoes supply abundant dust particles to the caves. While in the upwind of easterly winds, sandy gobi is the main landform, and during dust storms of easterly winds, it is the main source for falling dust in the caves. The falling dust in the caves mainly comes from the short distance transport of dust in Mingsha Mountain (Qu et al., 1992) and sandy gobi atop the Mogao Grottoes (Zhang et al., 2014). In addition, aeolian dust transported in a long distance within dust storms from southern Xinjiang and Inner Mongolia partly contributes to aeolian dust in the Mogao Grottoes (Gao et al., 2010).
The falling dust particles in the caves have threatened to the protection of murals and statues. Dust storms are the main sources for these falling dust. In the Mogao Grottoes district, dust storms are mainly caused by westerly and easterly winds (Zhang et al., 2014). In order to control dust hazards, first, the dust storm monitoring and warning system should be established to realize the early warning. A suggestion is that making full use of the resources of the local meteorological department and providing professional real-time forecast services for the dust disaster early warning system in the Mogao Grottoes. Once the dust storm comes, as the concentration of the dust in the caves reaches a certain threshold, the caves should be closed in time. According to the wind speed monitoring at the top of the Mogao Grottoes, when the wind reaches the level of moderate gale and lasts for 30 min, it can usually be identified as a dust storm process, which takes 2–20 h to complete. According to this study, the real-time PM10 concentration in front of Cave 72 reaching 6000 μg m−3 (mean of peak real-time dust concentrations) can be used as the threshold for dust storm warning, and the caves should be closed at this moment. Second, the falling dust in the caves was mainly composed of particles in size of 10–30 μm, accounting for 70–75% of the total falling dust, and this coarse dust particles also indicate the proximity of dust sources (Figure 11). To achieve the goal of minimizing the damage of falling dust to the Mogao Grottoes, improving the existing sand control system is the right thing to do. The previous wind tunnel study showed that the artificial gobi surface with 30% coverage and 30-mm-high gravels was optimal for sand drift control engineering atop the Mogao Grottoes (Zhang et al., 2014). This technique is suggested to control dust emission from gobi surrounding the Mogao Grottoes, especially for gobi in upwind of easterly winds. Besides, further building sand fences and straw checkerboards atop the Mogao Grottoes to control dust emission from mega-dunes is also recommended.
Continuous and synchronous observations of wind speeds and dust concentrations in the Mogao Grottoes were performed from February to October 2012, and temporal variation characteristics of dust concentrations have been analyzed. The following conclusions can be drawn:
(1) The monthly mean TSP, PM10, and PM2.5 concentrations during the monitoring period gradually decreased from February to October. The daily mean TSP, PM10, and PM2.5 concentrations in the Mogao Grottoes area are in the range of 2.36–3585.53, 1.95–3056.88, 0.69–1368.60 μg m−3, respectively, and the maximum daily mean dust concentrations occur mainly on February, March, and April.
(2) PM10 is the main particle size composition of TSP in dust storms of the Mogao Grottoes. The PM10/TSP ratio ranges from 0.67 to 0.98 with a mean value of 0.82, and the PM2.5/PM10 ratio ranges from 0.31 to 0.73 with a mean value of 0.55.
(3) The average TSP, PM10, and PM2.5 concentrations in a dust storm of westerly winds are 3528.81, 2465.49, and 869.22 μg m−3, respectively, with a maximum dust concentration of 21483.32 μg TSP m−3, 11085.17 μg PM10 m−3, and 3028.08 μg PM2.5 m−3. During a dust storm of easterly winds, the average TSP, PM10, and PM2.5 concentrations are 5002.71, 3745.33, and 1297.48 μg m−3, respectively, and the maximum dust concentrations are 15959.97 μg TSP m−3, 11363.59 μg PM10 m−3.
(4) Coincidence of the driest and windy season of spring and sufficient dust sources, such as Mingsha Mountain and the surrounding sandy gobi contribute to the high dust concentrations in the Mogao Grottoes.
(5) A dust storm monitoring and warning system in the Mogao Grottoes should be established to realize the early warning. As the PM10 concentration in front of Cave 72 during dust storms reaches the threshold of 6000 μg m−3, the caves should be closed to prevent dust hazards. Improving the existing sand control system to control dust emission from mega-dunes and sandy gobi is also recommended.
The original contributions presented in the study are included in the article/Supplementary Material, further inquiries can be directed to the corresponding author.
GZ and LT designed the experiment and wrote the manuscript. WZ, HZ, and FQ conducted statistical analysis. All authors contributed to the writing-reviewing and editing.
This work was founded by the National Natural Science Foundation of China (41871018), Key Research and Development Program of Gansu Province (20YF8WF016), and Scientific Research Project of Dunhuang Research Academy (2020-KJ-YB-12).
The authors declare that the research was conducted in the absence of any commercial or financial relationships that could be construed as a potential conflict of interest.
All claims expressed in this article are solely those of the authors and do not necessarily represent those of their affiliated organizations, or those of the publisher, the editors and the reviewers. Any product that may be evaluated in this article, or claim that may be made by its manufacturer, is not guaranteed or endorsed by the publisher.
Ba, J., and Wang, X. (2010). Analysis of Climate Character in Dunhuang in Recent 56 Years. J. Arid Meteorology 28, 304–308. (in Chinese).
Baddock, M. C., Strong, C. L., Leys, J. F., Heidenreich, S. K., Tews, E. K., and McTainsh, G. H. (2014). A Visibility and Total Suspended Dust Relationship. Atmos. Environ. 89, 329–336. doi:10.1016/j.atmosenv.2014.02.038
Cao, L., Cao, H., Yu, H., Yang, Q., Wang, K., and Wang, X. (2013). Concentration Distribution Characteristics of PM10 and the Relation with Meteorological Element in Extreme Arid Areas of the West Part in Hexi Corridor. Ecol. Environ. Sci. 22 (11), 1807–1813. (in Chinese).
Charron, A., Harrison, R. M., Moorcroft, S., and Booker, J. J. A. E. (2004). Quantitative Interpretation of Divergence between PM10 and PM2.5 Mass Measurement by TEOM and Gravimetric (Partisol) Instruments. Atmos. Environ. 38 (3), 415–423. doi:10.1016/j.atmosenv.2003.09.072
Choobari, O. A., Zawar-Reza, P., and Sturman, A. (2014). The Global Distribution of Mineral Dust and Its Impacts on the Climate System: A Review. Atmos. Res. 138, 152–165. doi:10.1016/j.atmosres.2013.11.007
Dahmardeh Behrooz, R., Esmaili-Sari, A., Bahramifar, N., and Kaskaoutis, D. G. (2017). Analysis of the TSP, PM10 Concentrations and Water-Soluble Ionic Species in Airborne Samples over Sistan, Iran during the Summer Dusty Period. Atmos. Pollut. Res. 8 (3), 403–417. doi:10.1016/j.apr.2016.11.001
Filonchyk, M., Yan, H., Shareef, T. M. E., Yang, S. J. T., and Climatology, A. (2019). Aerosol Contamination Survey during Dust Storm Process in Northwestern China Using Ground, Satellite Observations and Atmospheric Modeling Data. Theor. Appl. Climatology 135 (1), 119–133. doi:10.1007/s00704-017-2362-8
Fryberger, S. G., and Dean, G. (1979). “Dune Forms and Wind Regime,” in A Study of Global Sand Seas. Editor E. D. McKee, 1052, 137–169. USGS Professional Paper.
Ganor, E., and Foner, H. A. (2001). Mineral Dust Concentrations, Deposition Fluxes and Deposition Velocities in Dust Episodes over Israel. J. Geophys. Res. 106 (D16), 18431–18437. doi:10.1029/2000JD900535
Gao, Z., Shao, L., and Li, G. (2010). Characteristics Analysis of the Sand-Dust Weather in Dunhuang Area. J. Arid Meteroology 28 (1), 59–64. (in Chinese).
Gholampour, A., Nabizadeh, R., Hassanvand, M. S., Taghipour, H., Rafee, M., Alizadeh, Z., et al. (2016). Characterization and Source Identification of Trace Elements in Airborne Particulates at Urban and Suburban Atmospheres of Tabriz, Iran. Environ. Sci. Pollut. Res. 23 (2), 1703–1713. doi:10.1007/s11356-015-5413-7
Gillies, J. A., Nickling, W. G., and McTainsh, G. H. (1996). Dust Concentrations and Particle-Size Characteristics of an Intense Dust Haze Event: Inland delta Region, Mali, West Africa. Atmos. Environ. 30 (7), 1081–1090. doi:10.1016/1352-2310(95)00432-7
Goudie, A. S., and Middleton, N. J. (2006). Desert Dust in the Global System. Springer Science & Business Media.
Goudie, A. S. (2009). Dust Storms: Recent Developments. J. Environ. Manage. 90 (1), 89–94. doi:10.1016/j.jenvman.2008.07.007
Kok, J. F., Parteli, E. J. R., Michaels, T. I., and Karam, D. B. (2012). The Physics of Wind-Blown Sand and Dust. Rep. Prog. Phys. 75 (10), 106901. doi:10.1088/0034-4885/75/10/106901
Liu, B., Peng, W., Liu, S., and Yang, T. (2022). Estimation on the Dust Lift Amount and Source Contribution of the Heavy Dust Weather in Mid-march 2021 over Central East Asia. J. Desert Res. 42 (13), 79–86. (in Chinese).
Liu, B., Qu, J., Zhang, W., and Qian, G. (2011a). Numerical Simulation of Wind Flow over Transverse and Pyramid Dunes. J. Wind Eng. Ind. Aerodynamics 99 (8), 879–888. doi:10.1016/j.jweia.2011.06.007
Liu, B., Zhang, W., Qu, J., Zhang, K., and Han, Q. (2011b). Controlling Windblown Sand Problems by an Artificial Gravel Surface: A Case Study over the Gobi Surface of the Mogao Grottoes. Geomorphology 134 (3), 461–469. doi:10.1016/j.geomorph.2011.07.028
Luo, X., Li, Y., Yan, Z., Yang, M., and Nie, X. (2021). Evolution Characteristics of Sandstorm and Meteorological Influence Factors in Hexi Corridor in Recent 60 Years. Reserch of Soil and Water Conversation 28 (5), 254–267. (in Chinese).
Qu, J., Ling, Y., Zhang, W., and Wang, X. (1992). Preliminary Studies of Atmopheric Dustfall in Mogao Cave, Duhuang. Sci. Conversation Archeoglogy 4 (2), 19–24. (in Chinese).
Rashki, A., Kaskaoutis, D. G., Rautenbach, C. J. d., Eriksson, P. G., Qiang, M., and Gupta, P. (2012). Dust Storms and Their Horizontal Dust Loading in the Sistan Region, Iran. Aeolian Res. 5, 51–62. doi:10.1016/j.aeolia.2011.12.001
Shahsavani, A., Naddafi, K., Jaafarzadeh Haghighifard, N., Mesdaghinia, A., Yunesian, M., Nabizadeh, R., et al. (2012). Characterization of Ionic Composition of TSP and PM10 during the Middle Eastern Dust (MED) Storms in Ahvaz, Iran. Environ. Monit. Assess. 184 (11), 6683–6692. doi:10.1007/s10661-011-2451-6
Shen, Y. B., Shen, Z. B., Du, M. Y., and Wang, W. F. (2004). Studies on Dust Emission in Gobi and Oasis of Northwest China. China Environ. Sci. 24 (4), 390–394.
Stone, R. (2008). Shielding a Buddhist Shrine from the Howling Desert Sands. Science 321 (5892), 1035. doi:10.1126/science.321.5892.1035
Ta, W., Xiao, Z., Qu, J., Yang, G., and Wang, T. (2003). Characteristics of Dust Particles from the desert/Gobi Area of Northwestern China during Dust-Storm Periods. Env Geol. 43 (6), 667–679. doi:10.1007/s00254-002-0673-1
Tan, L. (2015). Aeolian Sand/dust Flux over Near-Surface Gobi: A Case Study Atop the Mogao Grottoes. Beijing: Doctoral thesis, University of Chinese Academy of Sciences. (in Chinese).
Tan, L., Zhang, W., Qu, J., Wang, J., An, Z., and Li, F. (2016). Aeolian Sediment Transport over Gobi: Field Studies Atop the Mogao Grottoes, China. Aeolian Res. 21, 53–60. doi:10.1016/j.aeolia.2016.03.002
Tan, L., Zhang, W., Qu, J., Zhang, K., An, Z., and Wang, X. (2013). Aeolian Sand Transport over Gobi with Different Gravel Coverages under Limited Sand Supply: A mobile Wind Tunnel Investigation. Aeolian Res. 11, 67–74. doi:10.1016/j.aeolia.2013.10.003
Wang, W., Wang, T., Shen, Z., Xie, J., and Xing, J. (2006). Pollution Status of Harmful Components to Frescos in Dunhuang Mogao Grottoes. Plateau Meteroology 25 (1), 164–168. (in Chinese).
Wang, X., Xia, D., Wang, T., Xue, X., and Li, J. (2008). Dust Sources in Arid and Semiarid China and Southern Mongolia: Impacts of Geomorphological Setting and Surface Materials. Geomorphology 97 (3–4), 583–600. doi:10.1016/j.geomorph.2007.09.006
Wang, X., and Zhang, C. (2021). Field Observations of Sand Flux and Dust Emission above a Gobi Desert Surface. J. Soils Sediments 21 (4), 1815–1825. doi:10.1007/s11368-021-02883-5
Wang, Y. Q., Zhang, X. Y., Gong, S. L., Zhou, C. H., Hu, X. Q., Liu, H. L., et al. (2008). Surface Observation of Sand and Dust Storm in East Asia and its Application in CUACE/Dust. Atmos. Chem. Phys. 8, 545–553. doi:10.5194/acp-8-545-2008
Yang, X., Zhang, Q., Ye, P., Qin, H., Xu, L., Ma, L., et al. (2021). Characteristics and Causes of Persistent Sand-Dust Weather in Mid-march 2021 over Northern China. J. Desert Res. 41 (3), 245–255. (in Chinese).
Zhang, E., Cao, J., Wang, X., Zhang, G., Zhang, Z., Du, N., et al. (2007). Apreliminary Study of Characterizationof Indoor and Outdoor Airquality inDunhuang Mogao Grottoes. J. Graduate Sch. Chin. Acad. Sci. 24 (5), 612–618. (in Chinese).
Zhang, W., Tan, L., Zhang, G., Qiu, F., and Zhan, H. (2014). Aeolian Processes over Gravel Beds: Field Wind Tunnel Simulation and its Application Atop the Mogao Grottoes, China. Aeolian Res. 15, 335–344. doi:10.1016/j.aeolia.2014.07.001
Zhang, X. Y., Arimoto, R., and An, Z. S. (1997). Dust Emission from Chinese Desert Sources Linked to Variations in Atmospheric Circulation. J. Geophys. Res. 102 (D23), 28041–28047. doi:10.1029/97jd02300
Zhang, X., Zhao, S., Mei, H., Zou, Y., Zhou, Z., Zhang, X., et al. (2022). Analysis of Airport Risk Propagation in Chinese Air Transport Network. J. Adv. Transportation 2022 (5), 1–13. (in Chinese). doi:10.1155/2022/9958810
Keywords: dust storms, particulate matter, dust concentration, temporal variation, dust hazards, Mogao Grottoes
Citation: Zhang G, Tan L, Zhang W, Zhan H and Qiu F (2022) Temporal Variation of Airborne Dust Concentrations in the Mogao Grottoes, Dunhuang, China. Front. Environ. Sci. 10:878466. doi: 10.3389/fenvs.2022.878466
Received: 18 February 2022; Accepted: 28 March 2022;
Published: 13 April 2022.
Edited by:
Huawei Pi, Henan University, ChinaReviewed by:
Zhongju Meng, Inner Mongolia Agricultural University, ChinaCopyright © 2022 Zhang, Tan, Zhang, Zhan and Qiu. This is an open-access article distributed under the terms of the Creative Commons Attribution License (CC BY). The use, distribution or reproduction in other forums is permitted, provided the original author(s) and the copyright owner(s) are credited and that the original publication in this journal is cited, in accordance with accepted academic practice. No use, distribution or reproduction is permitted which does not comply with these terms.
*Correspondence: Lihai Tan, dGFubGloYWkwOUBsemIuYWMuY24=
Disclaimer: All claims expressed in this article are solely those of the authors and do not necessarily represent those of their affiliated organizations, or those of the publisher, the editors and the reviewers. Any product that may be evaluated in this article or claim that may be made by its manufacturer is not guaranteed or endorsed by the publisher.
Research integrity at Frontiers
Learn more about the work of our research integrity team to safeguard the quality of each article we publish.