- 1Botany and Ecology Department, Federal University of Mato Grosso (UFMT), Cuiabá, Brazil
- 2Pantanal Research Center, Cuiabá, Brazil
- 3Department of General Biology, State University of Ponta Grossa, Paraná, Brazil
- 4Multidisciplinary Institute for Environmental Studies (MIES), University of Alicante, Alicante, Spain
- 5Department of Ecology, University of Alicante, Alicante, Spain
- 6Department of Oceanography of the Federal University of Pernambuco, Recife, Brazil
Microplastics are an emerging global threat to freshwater ecosystems. There is a growing number of microplastic studies in wetlands and floodplains such as the Pantanal. Microplastics in the Pantanal’s water courses, lakes, and floodplains were sampled with a plankton net in 16 locations in the Pantanal lowlands at varying distances from their potential urban sources over 2 years. Microplastics from the water samples were separated from sediments and organic matter and examined under a stereomicroscope to be identified and counted. Visual determination was then confirmed by RAMAN spectrometry. We found 730 microplastics. The average ± std microplastic concentration was 38 ± 16 x/m3, ranging between 10 and 100 x/m3. Fibres, fragments, and films represented 68%, 28%, and 4%, respectively, of the total microplastics. Surprisingly, a synthetic blue fibre of non-identified composition accounted for 51.0% of the microplastics. Other identified polymers included PP (18.2%), PET (16.9%), PVC (5.1%), PVA (4.4%) and LDPE (4.4%). The blue fibres, PP, and PET were found at all sampling locations. Microplastic concentrations varied from 27 ± 12 x/m3 to 48 ± 21 x/m3 across the sampling sites. Microplastics’ average size was 0.51 ± 0.21 mm, ranging from 0.06 to 0.97 mm. In the study area, the average seasonal concentrations varied from 34 ± 11 to 43 ± 18 x/m3 during the 2 years study period (2018–2019). In lentic environments, the median concentrations (30 x/m3) were significantly lower than in the lotic ones (40 x/m3). Urban human activities are a potential source of microplastics as the concentration of these increased significantly with the population of the nearest human agglomeration. These results indicate widespread contamination of the Pantanal waters by microplastics. Furthermore, a microsynthetic of indeterminate composition is found everywhere in the Pantanal, and more research is urgently needed to determine its composition and sources. The contamination by micropolymers and other microsynthetics will likely impact the Pantanal biota and, eventually, humans living in these floodplains.
Introduction
Currently, the improper management of vast amounts of plastic waste has become a global concern (Henderson and Green, 2020), especially considering that there is much more plastic in the environment than visible to the naked eye. Within the environment, these plastics are mechanically fragmented, submitted to the action of water and Sun, and slowly degrade into microplastic particles (MPs) (Backhaus and Wagner, 2020; Mao et al., 2020). These are polymer fragments with sizes up to 5 mm, in various shapes such as granules, fragments, fibres, and films (Carr et al., 2016; Jahnke et al., 2017; Frias and Nash, 2019). This is without counting the plastic particles already produced in sizes under 5mm, such as microbeads for paints or cosmetics (Horton and Dixon, 2018).
Due to their small size, MPs are present in the air, soil, sewage, and water bodies such as lakes, rivers, and oceans (Andrady, 2017; Koelmans et al., 2019; Li C. et al., 2020; De Faria et al., 2021). The most recent studies have shown the presence of MPs even in remote locations, such as remote mountain areas (Free et al., 2014; Pastorino et al., 2021; Padha et al., 2022) or frozen water lakes in the Arctic (González-Pleiter et al., 2020). MPs have different compositions; micro polymers such as PET, PVA, and PVC are often found in water bodies (Fahrenfeld et al., 2019). In addition, the literature reports other particles of anthropogenic origin whose composition is complex and may include different polymers and metals, such as paint particles, cellulose, dyed particles, rubber, or those that are mixtures of different materials and difficult to identify (Hartmann et al., 2019; Bikker et al., 2020; Huntington et al., 2020; Felismino et al., 2021; Fragão et al., 2021).
As they are present in almost all types of environments and due to their small size, MPs can be found at various trophic levels (Cole et al., 2016). One of the most relevant impacts is the introduction of these polymers into the food chain through ingestion by invertebrates directly from the water (Ivar Do Sul and Costa, 2014). Different studies have observed the effects of the ingestion of microplastics on marine invertebrates and vertebrates, and the effects are diverse (Ivar Do Sul and Costa, 2014; Cole et al., 2016; Green et al., 2017). Furthermore, this type of coexistence with MPs focuses on their role as vectors of microorganisms, pathogens, and pollutants (Graca et al., 2017).
A recent review by Li et al. (2019) indicated that the quantification of MPs in freshwater ranges from more than 1 million pieces per cubic meter to less than 1 piece in 100 cubic metres. The variation in total MP concentration for different studies is likely due to some combination of differences in MP size classes, study site, temporal variation, and whether MPs were identified visually (considered to be prone to false positives and negatives) or with confirmation of the chemical structure (Ziajahromi et al., 2017). The studies report different results about the number of MPs found, but it is worth mentioning that a greater abundance was found in sites closer to cities and more populated places. Rivers are the main routes for microplastics to the ocean (Lebreton et al., 2017). Their transport is affected by processes such as sedimentation that depend mainly on the hydraulic characteristics of water courses and the size of the microplastics (Nizzetto et al., 2016; Horton and Dixon, 2018; Kumar et al., 2021a; Yan et al., 2021). Microplastics are also found in the air and can affect people living in cities with rapid urbanization and high industrialization but can reach remote locations by atmospheric transport (Liu et al., 2015; Li et al., 2019; Truong et al., 2021).
In wetlands, studies on the presence and factors controlling the distribution of MPs are recent and need to be better understood (Qian et al., 2021). Helcoski et al. (2020) showed that in a wetland area of Washington (USA), the types and amounts of microplastics differed between muddy and densely vegetated habitats. Scheurer and Bigalke (2018) found correlations between the number of microplastics and densely populated river basins in Switzerland. Studies on floodplains and humid environments have reported microplastics in Europe, China, North America, and South America (Ballent et al., 2016; Sutton et al., 2016; Leads and Weinstein, 2019; Jian et al., 2020; Bertoldi et al., 2021; Wang et al., 2021). They are reported in the Danube Alluvial Zone National Park (Lechner et al., 2014) and the floodplain soils from Switzerland (Scheurer and Bigalke, 2018).
In Brazil, microplastics have been reported in shoreline sediments of a lake in the Paraná River floodplain (Bletter et al., 2017) and the upper, middle, and lower parts of the Goiana Estuary (Lima et al., 2015). In this estuary, microplastics contaminated the life cycle of Acoupa (Cynoscion acoupa) (Ferreira et al., 2016). Microplastics were also ingested by Hoplosternum littorale, a common freshwater fish widely consumed by humans in South America (Silva-Cavalcanti et al., 2017). However, there are few published data in South America (Li C. et al., 2020; Li et al., 2020 Y.). In the Brazilian Pantanal, there is only one published study on microplastics in surface waters that specifically analysed one of the rivers that form the Pantanal: the Cuiabá River and related water bodies in its floodplain (De Faria et al., 2021).
The Pantanal is a wetland of international importance, more extensive than England. It is known for its mega biodiversity and for providing several environmental services. As it is relatively remote, it has remained reasonably pristine until now (Wantzen et al., 2008). This was made explicit by the Brazilian Constitution, which recognized the Pantanal as a “National Heritage,” by the Ramsar Convention that listed it as a Wetland of International Importance, and by UNESCO, which designated it a Biosphere Reserve in 2000.
This work aims to describe the spatial and temporal distribution of microplastics in the surface waters of the Pantanal to provide an overview of contamination by microplastics in the various aquatic environments that make up this immense wetland. It also discusses the potential sources of these pollutants and possible mechanisms of dispersal in this territory. It proposes to test some hypotheses: i—the concentration/composition and sizes of microplastics in the Pantanal vary according to geography since the potential sources of microplastics are not homogeneously distributed; ii—the concentration/composition and sizes of microplastics vary according to lotic and lentic environments, as these favour differential deposition of microplastics; iii—the concentration/composition of microplastics varies seasonally; iv—the concentration/composition of microplastics is influenced by the distance from human agglomerations and by the population of these agglomerations; v—the mean size of microplastics varies according to their polymer composition.
Materials and methods
Pantanal wetlands
The Pantanal (Figure 1) is a large mosaic of wetlands of ∼160,000 km2 in central South America within the Upper Paraguay River Basin - UPRB. The hydrological cycle of this large floodplain is characterized by an annual, monomodal, and predictable flood pulse. Flood dynamics and geomorphological heterogeneity generate a diversity of macrohabitats supporting high biodiversity. The quality, together with the number of available habitats, favours the maintenance of the high biodiversity in the naturally fragmented landscape of the Pantanal (Regolin et al., 2021). In the Pantanal, there are more than 2,000 species of plants, more than 580 birds, 271 fish, 174 mammals, 131 reptiles and 57 amphibians, and countless species of invertebrates and microorganisms. It is also home to considerable populations of endangered species such as jaguar (Panthera onca), giant otter (Pteronura brasiliensis), marsh deer (Blastocerus dichotomus), pampas deer (Ozotoceros bezoarticus) and hyacinth macaw (Anodorhynchus hyacinthinus) (Tomas et al., 2019).
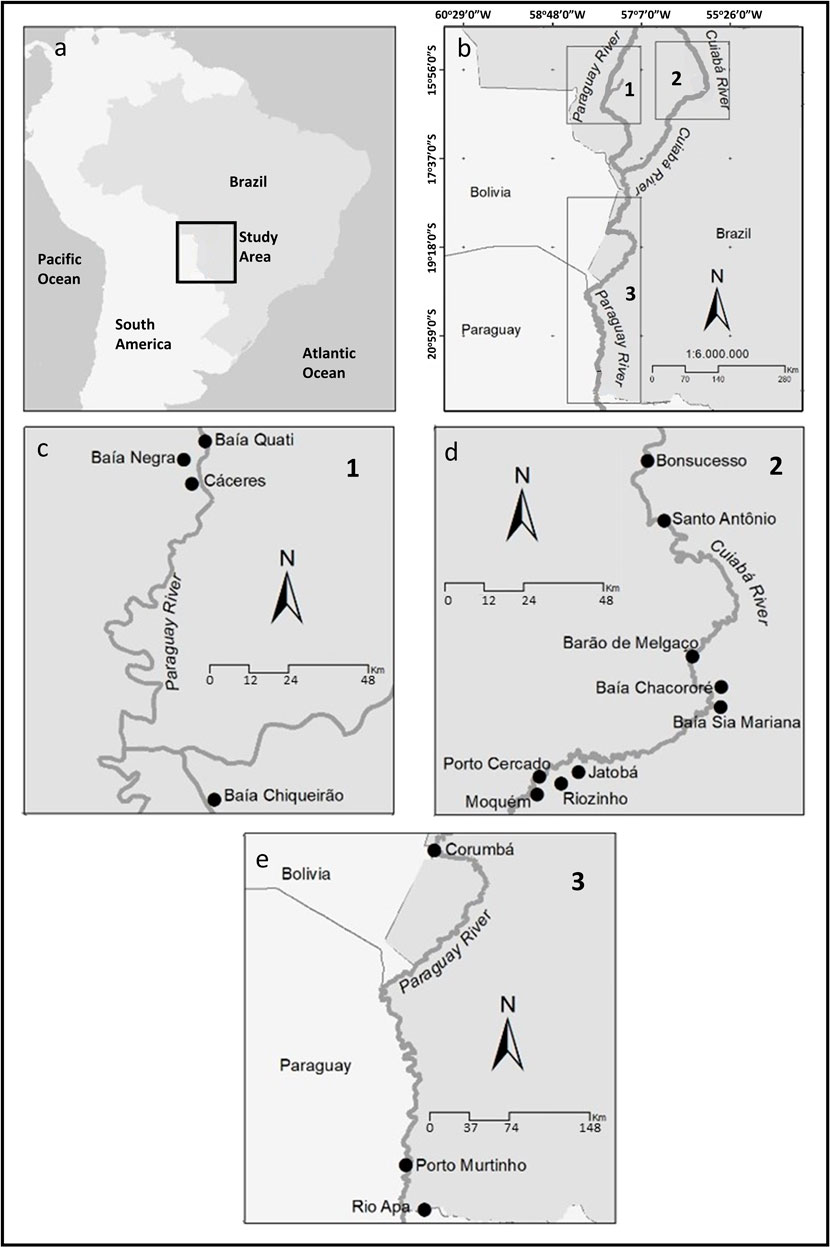
FIGURE 1. Study area and microplastic sampling sites in the Pantanal. (A) Location of the study area in South America. (B) Location of the sampling zones in The Pantanal: 1- Upper Paraguay Zone; 2- Cuiabá River Zone; 3- Lower Paraguay Zone. (C) Sampling locations of the Upper Paraguay Zone. (D) Sampling locations of the Cuiabá River Zone. (E) Sampling locations of the Lower Paraguay Zone. Dots represent the sampling sites.
The Pantanal floodplain (100–180 m asl) is enclosed to the east by a plateau (250–750 m asl) and to the west by the Bolivian and Paraguayan Chaco depression (Girard, 2011). The largest tributaries of this plain—the Paraguay, Cuiabá, and Taquari rivers—spring in the plateau and drain the more-or-less open Cerrado, where a large fraction of the vegetation cover has been converted into cultivated pastures and cultivated areas where highly mechanized agriculture is practiced. The same pattern is developing in the Paraguayan Chaco and less intensely in the Chiquitano Forest of Bolivia, both in the immediate region on the western edge of the Pantanal (Tomas et al., 2019). On the plateau, there are also important cities, such as Cuiabá on the banks of the river of the same name, the capital of the state of Mato Grosso, which, together with its sister city, Várzea Grande, has a population of around 865,000 people (De Faria et al., 2021). Another important city on the plateau of the Upper Paraguay River Basin is Cáceres, on the banks of the Paraguay River, with ∼95,000 inhabitants (IBGE 2021). The largest urban agglomeration in the plain is Corumbá, located on the banks of the Paraguay River and has a total of 145,000 people (IBGE 2021).
In these cities, the population density can be relatively high, as is the case for the metropolitan area of Cuiabá, a density of around 200 inhabitants km−2. In all of these, the treatment of effluents is deficient. For example, in Cuiabá, 47% of effluents are neither collected nor treated. In Várzea Grande, this reaches 55%. The sewage treatment deficit is even greater in smaller cities with a lower population density. For example, in Santo Antônio de Leverger, a town of around 18,000 people (1.51 inhabitants km−2), only 10.4% of wastewater is appropriately treated (De Faria et al., 2021). Figure 1 illustrates the position of cities in the vicinity of the sampling sites of this study and Table 1 provides some more information about these cities.
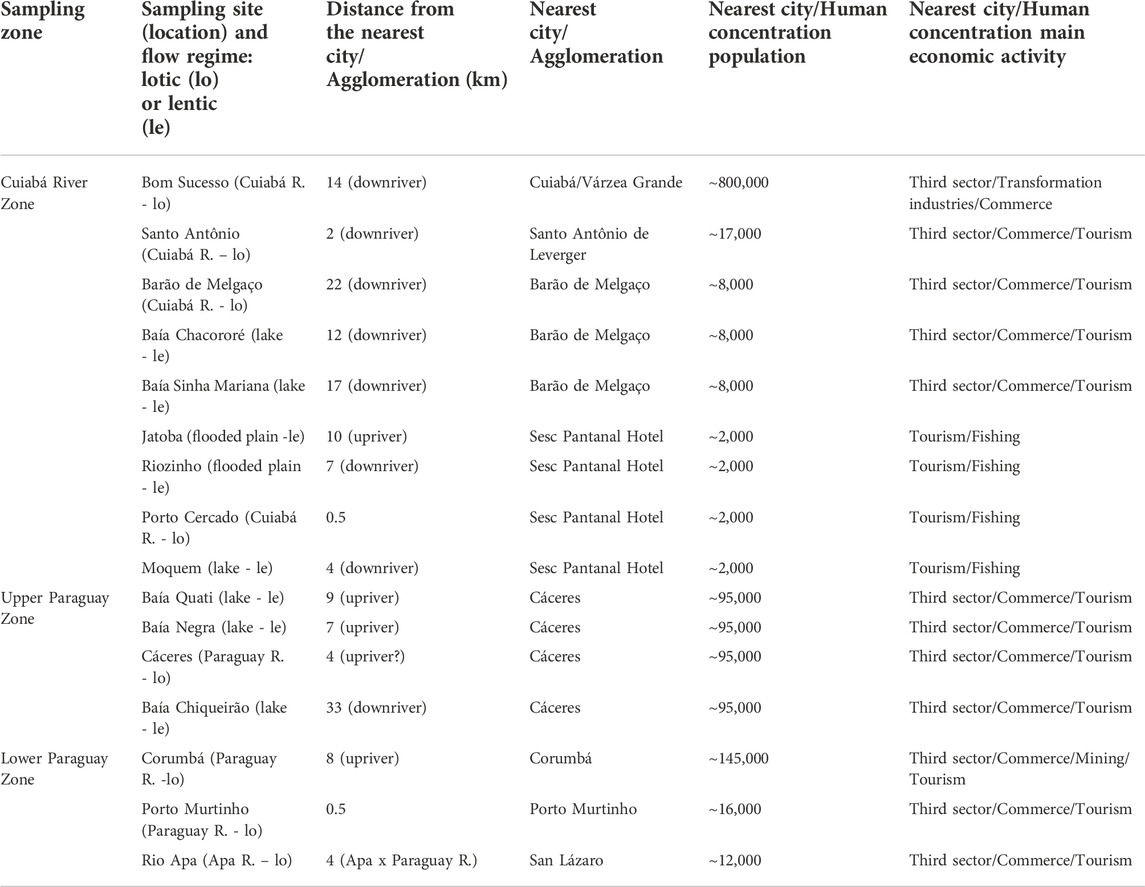
TABLE 1. Sampling sites’ characteristics. Populations of Brazilian cities from IBGE (2021). Estimated population at Sesc Pantanal Hotel and surroundings from Marinha (2011). Population of San Lázaro, Paraguay from DGEEC (2015).
Sampling sites and frequency
Sixteen sites were sampled, 9 along the Cuiabá River, 4 along the Upper Paraguay River, and 3 along the Lower Paraguay River (Figure 1). These sites include points within the main course of rivers and the bays and streams connecting to the main channel. Logistics were a determining factor in the selection of sampling sites. The Cuiabá River Zone is located near the Federal University of Mato Grosso in Cuiabá, the institution where the research was carried out. Most sampling sites along this river are accessible by land. Cáceres, 219 km from Cuiabá by road, is the Upper Paraguay zone’s main city. In this zone, all the sites are accessible only by boat. The most important city in the Lower Paraguay Zone is Corumbá, 1,031 km by road from Cuiabá. All sampling sites in this zone are accessible only by boat, from Corumbá or Porto Murtinho, 1,141 km from Cuiabá. Table 1 summarizes the information regarding the sampling sites.
Each of these sampling sites was sampled 4 times during 2 consecutive years, between 2018 and 2019. Two samples were taken at the end of the rainy season (March/April 2018 and 2019) and two others at the beginning of the rainy season (October/November 2018 and 2019), always respecting a short interval in sampling between one site and another. On each visit to a sampling site, 3 water samples were taken. In summary, the 16 sampling sites were sampled 4 times, and each collection was carried out in triplicate for 192 water samples.
Microplastic sampling
A 30 cm diameter, 70 cm long, 68 µm mesh size nylon plankton net with a 150 ml collection flask was used to sample microplastics following a methodology previously described by De Faria et al. (2021). A volume of water of 100 L was passed through this net. Once filtered, the residual 150 ml obtained in the collection bottle was transferred to the sampling bottle. The plankton net was used because the water bodies sampled in this study were shallower than the coastal and oceanic areas where a neuston net is generally employed. In the Pantanal region, most water bodies are shallow, and a neuston net would not be suitable. All samples were obtained from a boat.
The following procedure was adopted to minimize the potential for cross-contamination between sampling sites and sampling events. Before each sampling event, the plankton net was thoroughly washed. The collection flask and sampling bottles were rinsed 3 times (filled and then emptied). At all sampling sites, the plankton net was rinsed with 3 times its volume with local water. The collection bottle and sample bottles were rinsed 3 times with local water. After a sampling event, the plankton net and its collection flask were thoroughly washed.
Microplastics observation
The use of a cotton lab coat was mandatory during the experimental process. Clean Petri dishes were left open in the lab during the analytical procedures and examined for air background contamination. No microplastic items were found. To assess potential contamination of laboratory containers or air, 10 laboratory blanks were collected and analysed along with environmental samples. They consisted of distilled water stored in open sample vials for 5 days. These blanks were then processed according to the procedure described below. One (1) microplastic fibre was found in one of the blanks, indicating a low potential for contamination in the laboratory.
Once the samples had been acquired, they were taken to the laboratory for microplastic determination. First, a density separation was performed using a saline solution with sodium bromide (40% solution, density ∼1.4 g/cm3) to separate the microplastics, most of which have a lower density. Once prepared, the saline solution was filtered through a 1 μm fiberglass filter 47 mm diameter. The water from the sampling bottles was transferred to a beaker, and the filtered saline solution was added. The samples were left to rest for 24 h, sufficient for sediments to settle. The water was then carefully poured directly into a glass filtration apparatus (Buchner funnel and vacuum flask) and pumped under vacuum through a 0.45 μm filter (glass fibre microfilter, 47 mm diameter). Then, any organic matter in the filters was digested by spraying a solution with 30% hydrogen peroxide until there was no more visible reaction with the organic matter. About 5 to 10 drops of hydrogen peroxide per filter was sufficient. The digestion process was carried out in an oven at 40°C. Once there was no more visible reaction, the filters were left in the oven (turned off) to dry for 24 h.
Visual assessments were used to identify microplastics according to the physical characteristics of the particles using a ×45 magnification under a Zeiss stereo microscope (Yang et al., 2015). Particles down to 10 μm (0.01 mm) were assessed. We recorded the shape, colour, and number of microplastic particles. Based on their shapes, microplastics were classified into three types: fibres, fragments, and films (Su et al., 2018). The colour of each microplastic was also recorded. Six main types of microplastics were determined: blue fragments, blue fibres, red and pink fibres, green fragments, and transparent films.
The size distribution of the microplastics was obtained from a subsample (n = 48). In this subsample, the particle size was measured by analyzing the pictures of each microplastic confirmed by RAMAN (Chen et al., 2019; Prata et al., 2019; De Faria et al., 2021). A total of 202 size measurements were obtained.
Microplastic identification (RAMAN)
We analysed all the filters under a binocular magnifying glass (MOTIC SMZ 143 N2GG Trinocular) with a transparent circular template divided into eight sections placed at the top of the Petri dish. Each filter was analysed section by section.
Microplastics were characterized by RAMAN spectrometry, a spectroscopic technique based on the RAMAN effect/inelastic light scattering (NRS-5100, Jasco, Madrid, Spain) equipped with an LMU-20X-UVB lens. The laser excitation frequency and energy used were 784.79 nm and 11.8 mW, respectively. RAMAN spectra were recorded with a charge-coupled device camera (UV-NIR range, 1,024 × 255 pixels) electrically cooled to −70°C. RAMAN spectra were obtained between 162 and 1886 cm−1 with a spectral resolution of 2.47 cm−1. We considered the observed RAMAN spectra similar to the Open Specy spectra database when the Pearson correlation coefficient was 0.7 or higher and peaks of both spectra were coincident. SloPP-E, a spectra database for polymers aging in environmental conditions, was also used (Munno et al., 2020).
Only particles from types confirmed by RAMAN as synthetic material (single polymer or polymer mixture, or polymer-metal mixture) were counted as microplastics. Results are expressed as the number of microplastics (x); abundance of microplastics, which is x divided by the total number of microplastic (x/N), expressed as a percentage; frequency, which is the number of times a microplastic type was present over the number of samples (192), expressed as a percentage; or average or median microplastic concentration expressed in x/m3.
Data treatment
MS Excel was used for data tabulation and calculation, as well as to perform statistical tests. A two-way ANOVA was run for sampling site (16: see Figure 1) vs. sampling moment - wet and dry seasons of 2018 and 2019. For the sampling zone/sampling sites and flow regime/sampling site, assumptions of two-way ANOVA were not met (non-normality of residuals). A Kruskal Wallis test was applied to check for significant differences between sampling zones (Cuiabá River; Upper Paraguay; Lower Paraguay; Figure 1). Similarly, a Mann Whitney U-test was used to check for significant differences in concentrations between flow regimes (lentic or lotic, see Table 1). The Mann-Whitney U-test was applied because data were not normally distributed and because it is more robust than the t-test in the presence of outliers.
To check the influence of human agglomeration on the concentration of microplastics, a correlation analysis between distances from major urban centres (Cuiabá/Várzea Grande, Cáceres, Corumbá) or nearest city/agglomeration (see Table 1) and the average concentration of microplastics at the sampling site was carried out. A correlation between the population of the nearest human agglomeration and the average microplastic concentration was also done. The log of distance and population was used in these correlations. Pearson correlation (r) was first calculated and tested for null correlation. If H0 could not be rejected, the Spearman correlation (rs) was calculated and tested. In these analyses, residuals followed a normal distribution. When neither correlation coefficients were significant, the larger (absolute value) of the two coefficients was reported.
Microplastic sizes followed a normal distribution. ANOVA was used to check if there were significant differences in sizes between sampling sites and microplastic types. To check if there is a difference in size between lotic and lentic environments, a t-test was applied.
Results
Microplastic composition and sizes
In the 192 samples collected, a total of 730 microplastics were identified. There was at least one (1) microplastic piece in all water samples. Fibres were the most abundant microplastics (67.8%) recorded in 99.5% of the samples (Table 2). Fragments constituted 27.8% of microplastics and were recorded in 60.4% of the samples. Films were the least abundant (4.4%), but they were still found in 12% of the samples. The maximum number of different polymers in 1 single sampling event was 4.
RAMAN analysis revealed six different compositions among the analysed MPs. Of these six, five polymers were positively identified. The most abundant polymers were polypropylene (PP), in the form of blue fragments, with an abundance of 18.2% of the total found microplastics and present in 43.8% of the collected samples. PP was most abundant at the Bom Sucesso site (2018 rainy season), in the proximity of the largest urban agglomeration of the study region (Cuiabá/Várzea Grande). The following polymer in abundance was polyethylene terephthalate (PET), red and pink fibres, with an abundance of 16.9% and a frequency of 41.7%. Next, polyvinyl chloride (PVC), the transparent fragments, were found to have an abundance of 5.1% and a frequency of 15.6%. The remaining ones were polyvinyl alcohol (PVA), green fragments, and low-density polyethylene (LDPE), the transparent films, each with an abundance of 4.4% and frequency of 11.5% and 12.0%, respectively.
The sixth composition indicates a synthetic material, probably a mixture containing metallic/rare Earth compounds. Non-identified synthetic compounds were recently described by Felismino et al. (2021). These undetermined compounds (NIS = non-identified synthetic), which in the samples appear as blue fibres, were the most abundant (51.0%) in the study region and present in 99.0% of samples (Table 2). They were also the most abundant at all sampling sites, their relative abundance at each sampling site varied between 41% and 62% of the total microplastics at any particular sampling site. Photos displaying examples of the encountered microplastics are shown in Figure 2.
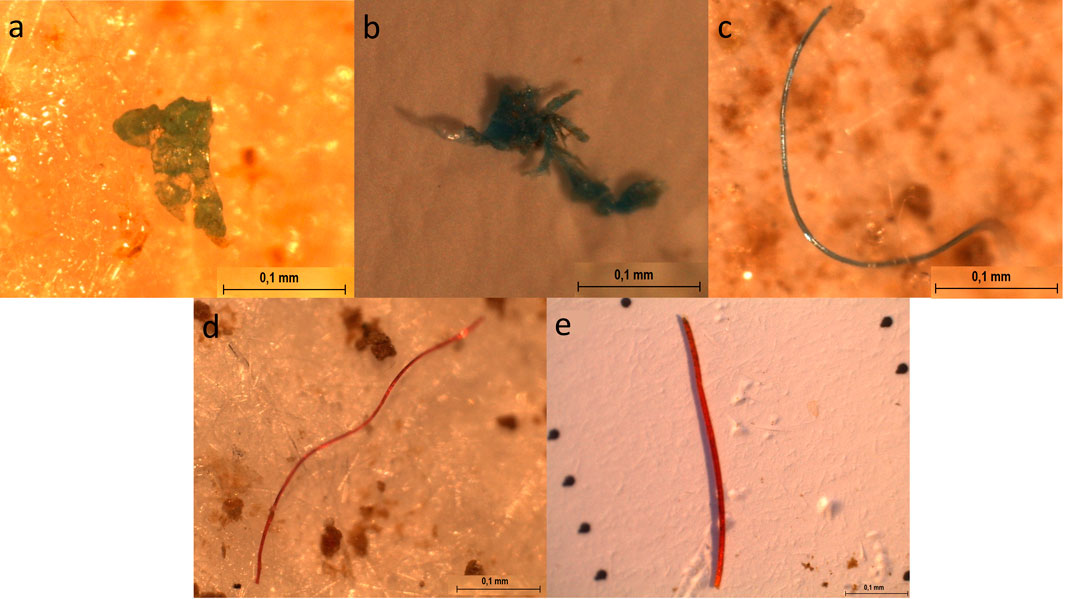
FIGURE 2. Examples of microplastics identified in the Pantanal wetlands. (A) PVA (green fragment); (B) PP (blue fragment); (C) NIS (blue fibre); (D) PET (red fibre); (E) PET (pink fibre).
Visually, these blue fibres of indeterminate composition have the characteristics common to other microplastics (no visible cellular/organic structure; clear and homogeneously coloured; equally thick fibres) (Yang et al., 2021), but surprisingly their RAMAN spectra did not correspond to a polymer spectrum reported by Open Specy. Some RAMAN spectra corresponding to these NIS are shown in Supplementary Figure S1. Due to their appearance under the microscope and the fact that no organic compound or dye (such as indigo blue) known in the extensive Open Specy library was identified, we deemed them synthetic. In the rest of the text, for convenience, they will be treated as microplastics, even if their composition is still unknown.
In terms of concentration in water, the average (± standard deviation) concentration of microplastics in the study region was 38 ± 16 x/m3, ranging between 10 and 100 x/m3. The average concentrations of NIS particles (19 ± 12 x/m3) were the highest, while those of PVC, PVA, and LDPE (2 ± 5 x/m3) were the lowest found (Table 3).
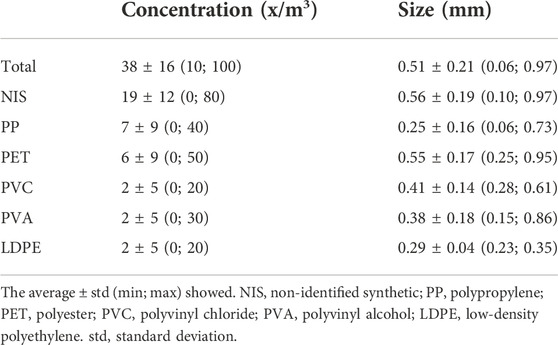
TABLE 3. Average microplastic concentrations (x/m3) and average size (mm) in the Pantanal wetland surface waters.
Regarding sizes, the average microplastic size was 0.51 ± 0.21 mm, varying from 0.06 to 0.97 mm. The sizes were significantly different among polymers (F = 12.02; p < 0.01). The Tukey HSD post-hoc test showed that NIS was significantly larger than PP, PVA, and LDPE, while PET was significantly larger than PP.
Microplastic spatio-temporal distribution in the Pantanal
Figure 3 illustrates the microplastic composition at each sampling site in the Pantanal. The lowest average concentration of microplastics was 27 ± 12 x/m3, found in Jatoba, on the floodplain. The largest (48 ± 21 x/m3) was found in Bom Sucesso, close to the metropolitan area of Cuiabá/Várzea Grande, the largest urban population in the study region. An ANOVA was performed between average concentrations at sampling sites indicating significant differences between the concentrations (F = 3.15; p < 0.01). The Tukey HSD post-hoc test revealed significant differences only for the Jatoba site, where the samples occurred in the floodplain only during the 2018 and 2019 rainy seasons: Jatobá/Bom Sucesso (p = 0.03); Jatoba/Apa River (p < 0.01); Jatoba/Cáceres (p < 0.03); Jatobá/Santo Antônio (p = 0.02). Other than these, there were no other significant differences between mean concentrations at the different sampling sites.
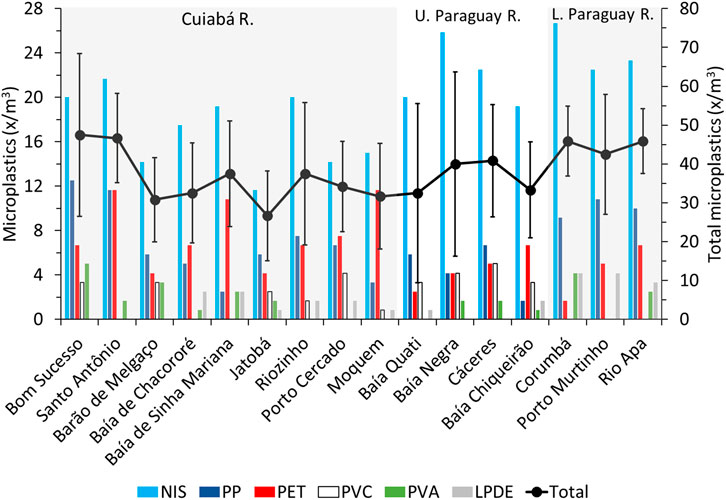
FIGURE 3. Average microplastic composition at sampling sites. Total microplastic concentration on the right vertical axis. Microplastics concentration for each specie on the left vertical axis. Cuiabá R. = Cuiabá River Zone; U. Paraguay R. = Upper Paraguay Zone; L. Paraguay R. = Lower Paraguay Zone. See Figure 1 for localization. NIS: non-identified synthetic; PP: polypropylene; PET: polyester; PVC: polyvinyl chloride; PVA: polyvinyl alcohol; LDPE: low-density polyethylene. The vertical bar associated with each average total microplastic composition is ± the standard deviation.
Over time, between the dry season of 2018 and the wet season of 2019, within the study area, the average microplastic concentration varied from 34 ± 11 (rainy season 2019) to 43 ± 18 x/m3 (dry season 2019). An ANOVA was performed between average concentrations at sampling moments (dry season 2018, rainy season 2018, dry season 2019, rainy season 2019), indicating significant differences between the concentrations (F = 3.46; p = 0.02). According to the Tukey HSD post-hoc test, only the average concentration of microplastics collected in the dry season of 2019 significantly differed from the average concentration in the rainy season of 2019 (p = 0.04). There were no significant differences between the 2018 wet (39 ± 16 x/m3) and dry (36 ± 1.4 x/m3) seasons. Finally, a two-way ANOVA results between the sampling moment and sampling site revealed that the sampling moment had no significant interaction with the sampling site (F = 1.26; p = 0.16).
Concerning the differences between sampling zones, a significant Kruskal Wallis test (H = 24.6343; p < 0.01) revealed that the mean ranks of the following pairs were different: Cuiabá River (36 ± 15 x/m3)/Lower Paraguay (46 ± 11 x/m3) and Lower Paraguay/Upper Paraguay (37 ± 19 x/m3). Finally, the Mann Whitney U-Test showed that the median concentrations found in the lotic regime (40 x/m3) were significantly (Z = −4.32; p < 0.01) higher than in the lentic regime (30 x/m3). Figure 4 displays the average concentration at each sampling site, and those from lentic sampling sites are generally lower than those from lotic sampling sites.
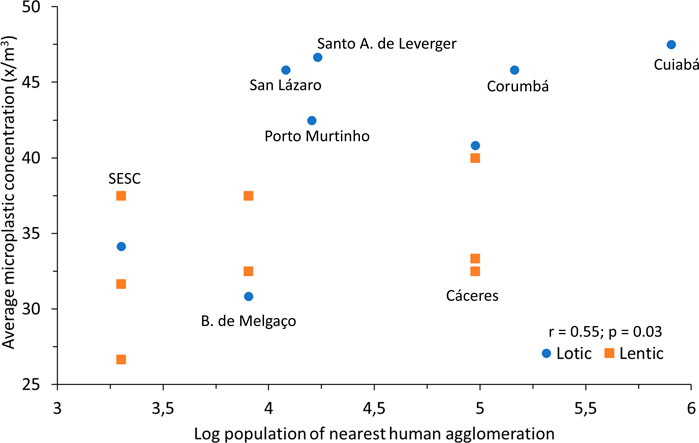
FIGURE 4. Correlation between the average concentration of microplastics and the population of the nearest city/human agglomeration (in Log10). The correlation coefficient (r) and the significance probability (p) are also given. For each sampling site, the nearest human agglomeration is indicated. When more than a sampling site is closest to the same nearest human agglomeration, the name of the agglomeration is indicated above or below. The legend also indicates whether the sampling site is under lotic or lentic flow conditions. More information about human agglomerations can be obtained in Table 1.
No significant correlations were detected between total MPs and the distance (in Log10) from larger cities (r = −0.17; p = 0.19) and distance from agglomerations closer to the sampling sites (r = −0.23; p = 0.07). However, there was a significant correlation between the population (in Log10) of the urban centre closest to the sampling site and the concentration of microplastics (r = 0.33; p < 0.01), which indicates that the concentration of microplastics tends to increase together with the population from the local urban centre. (Figure 4).
As for sizes, there were no significant differences between sampling locations (F = 1.75; p = 0.09) and between microplastic from lentic and lotic environments (T = −1.64; p = 0.10).
Discussion
The concentrations of microplastics found in the Pantanal fall within the wide ranges of microplastics encountered in freshwater wetlands. For example, in Guaíba Lake, Southern Brazil, comparable concentrations ranging from 11.9 ± 0.6 to 61.2 ± 6.1 microplastics per m3 were found. There, PP and polyethylene (PE) together comprised most of the polymer types (98%) (Bertoldi et al., 2021). In the Xijin Wetland Park, the largest wetland park in South China, total aquatic microplastics were from the same order of magnitude ranging from 150 to 1,067 x/m3. Rayon (14.55%), followed by polyester (PES) (9.09%) and PE (8.18%), were the most frequently detected microplastic polymers in the Wetland Park along with PP (7.27%) (Wang et al., 2021). In a recent compilation regarding microplastics in wetlands, concentrations as low as 3.5 x/m3 and as high as 11,250 x/m3 were reported. In the Ebro River Delta (3.5 x/m3), the main microplastics found were polyamide (24%), PE (16%), polymethyl methacrylate (12%), and PET (12%). At Wuliangsu Lake, northern China (11,250 x/m3), the main polymers were polystyrene (39%), PP (28%), and PE (17%) (Qian et al., 2021). Note that in this study, no PE particles were found in the Pantanal.
Concerning fragments found in the Pantanal waters, blue PP fragments were the most abundant fragments in the Pantanal. This polymer is commonly found in wetlands (Kumar et al., 2021b). In estuaries of South Carolina (USA), blue PP fragments of secondary origin were also found (Gray et al., 2018). In Lake Victoria (East Africa) waters, PP fragments of secondary origin make up around 30% of microplastics (Egessa et al., 2020).
PVC, the transparent fragments in waters of the Pantanal, is not frequently reported in freshwater wetlands. Some analysis protocols are not efficient in isolating this relatively high-density compound (1.388 g/cm3; Gray et al., 2018), which was not the case in the present study. Similar abundances of PVC (4%) were found in Veeranam in Tamil Nadu, India (Bharath et al., 2021). On the contrary, in Lake Poyang (China) waters, PVC is one of the main microplastics found (Jian et al., 2020). In this same lake, PVA is also one of the dominant polymers in the microplastics load (Jian et al., 2020), while in the Pantanal, where it appears as green fragments, its concentration was low. PVA in the filament form has also been reported in fish from the Setiu wetland, Malaysia (Kumar et al., 2021b).
The LDPE, the film fragment in the Pantanal waters, was also recently reported in freshwater and wetlands of China (Jian et al., 2020; Kumar et al., 2021b; Wang et al., 2021; Yang et al., 2021), India (Bharath et al., 2021) and Lake Victoria (Egessa et al., 2020). In Lake Victoria where the microplastic concentration in the water column is lower than in the Pantanal (∼0.73 x/m3), film fragments represented 15% microplastics and were mainly composed of LDPE.
Regarding microfibres, red and pink ones composed of PET were found in the waters of the Pantanal as elsewhere worldwide in freshwaters (Wang et al., 2021). In wetland waters, PET is not frequently mentioned. For example, there is no mention of PET by Kumar et al. (2021a), neither Abbasi (2021) or Qian et al. (2021).
Finally, the NIS blue fibres found everywhere in the Pantanal waters are not, to our knowledge, described in the literature. Their RAMAN spectra did not correspond to any of the spectra included in the Open Specy Library. We also used SLOPP-E (Munno et al., 2020) but could not find a good match. However, the SLOPP-E still does not contain all possible RAMAN spectra for weathered polymers. These spectra of weathered microplastics are distinctly different from the spectra of fresh standard samples, as many peaks in the RAMAN spectra of weathered microplastics are weakened and even invisible (Dong et al., 2020). The same applies to paint (Simon et al., 2021). Furthermore, the existence of composite plastic products reduced the reliability of traditional 2D Raman spectroscopy (Jiao et al., 2021).
The sources of microplastics can be elucidated by considering differences in particle morphology and polymer type (Ballent et al., 2016). The presence of fragments suggests the fragmentation of larger plastics. Secondary MPs, such as films and fragments, are associated with wear, abrasion, or decomposition of plastic packaging (bottles, paint) or plastic bags and may be associated with terrestrial sources such as improper solid waste disposal (Enyoh et al., 2019; Liu et al., 2019; Wang et al., 2019). These can be transported by rainwater to water bodies (Talvitie et al., 2015; Sutton et al., 2016; Horton et al., 2017). Polymers such as PP, PVC, PVA, and LDPE) are used for many consumer products in urban settings and might be the source of the fragments encountered in the Pantanal (Wang et al., 2019; Egessa et al., 2020; Park et al., 2020).
PET-type plastics are widely used in the textile industry (Hidalgo-Ruz et al., 2012; Liu et al., 2019). They are likely associated with wastewater from laundry and washing machines (Vaughan et al., 2017). When introduced into the washing machine, the friction produced during the wash cycle may release up to 6 million microfibres that are likely to end up in the wastewater (Napper et al., 2020). The abundance of red and pink fibres (polyester) may be related to the presence of cities close to sampling sites and the improper disposal of sewage water. Browne et al. (2011) identified a predominance of polyester and acrylic fibres in coastal sediments and wastewater effluents, suggesting that wastewater inflows were the predominant source of fibres at these sites.
Several studies have linked the abundance of microplastics to population densities (Ballent et al., 2016; Sruthy and Ramasamy, 2017). In the Pantanal, the results indicate that microplastic concentrations increase with the populations of local agglomerations, which suggests that they are a source of microplastics. However, this correlation is weak even if significant, indicating that diffuse sources such as tourism, fishing, subsistence agriculture, or livestock farming could significantly contribute to the microplastic loads found (Wang et al., 2018; Zhou et al., 2020). PVC, PVA, and LDPE are absent from sampling sites even if they are close to large human agglomerations. For example, no LDPE was detected in Bom Sucesso, and just a few km downstream of Cuiabá/Várzea Grande with 800,000 inhabitants, but it is present in Jatobá or Moquem, near an agglomeration of around 2,000 people (Figure 3), which are among the most pristine sites sampled in this study, which suggests that rural or sporadic activities in these sites contribute to the microplastic load. Alternatively, the transport of these polymers (PVC, PVA, and LPDE) is not as efficient as NIS, PP, and PET, which are present in all samples. Alternatively, differential settlement rates under lentic conditions may generally lower the concentration of microplastics, as found in this study (Figure 4). They may also influence the microplastic composition in the water column (Kumar et al., 2021a).
Among these synthetics, the concentration of NIS is systematically the highest at all sampling sites, which indicates a pervasive penetration of the parent material throughout the Pantanal. In South Carolina, the presence of blue fibres in intertidal sediments has been associated with wastewater treatment effluents carrying textile fibres. However, their composition is not reported (Gray et al., 2018; Leads and Weinstein, 2019). Blue fibres with similar RAMAN spectra were also reported in Pantanal beach sediments and associated with e-waste (Camargo et al., 2022). Abundances and concentrations of plastic debris in soil from an abandoned e-waste site in China were at the high end in results from the literature. However, at this site, Acrylonitrile-butadiene-styrene (ABS) particle was the main plastic (Zhang et al., 2021).
Nonetheless, the fate of e-waste in Brazil is still not defined (Echegaray and Valeria, 2017), and reuse or informal collection is the most likely handling of electronic waste, as only a few companies are specialized in recycling electronic devices in Brazil (Reis et al., 2012). It is common to observe televisions, computers, and other electronic materials along the Cuiabá River banks. Thus, E-waste is a possible source of microplastics in the Pantanal even though no clear link could be established with the presence of the NIS blue fibres in the Pantanal waters. It is known that e-waste recycling activities bring heavy input of metals and organic pollutants into the local environment (Zhang et al., 2021). In a recent literature review, metals’ concentration on microplastics was sometimes 800 times higher than in the surrounding environment. Combined toxic effects include endocrine disruption and reduced predatory behaviour of aquatic carnivores (Naqash et al., 2020). Finally, microplastics and associated contaminants such as heavy metals may threaten food safety, transferring chemicals to human bodies and causing adverse health effects (Huang et al., 2021). They could become an entry route for these contaminants in the Pantanal ecosystem, which could affect the biota and ecosystem functions, which needs to be investigated (Baláž et al., 2017).
Data availability statement
The original contributions presented in the study are included in the article/Supplementary Materials, further inquiries can be directed to the corresponding author.
Author contributions
EF and PG contributed to the conception and design of the study. EF sampled all locations. EF isolated microplastics in the lab, made first visual observations and determined sizes. EF and PG organized the database. CS-L and NC identify and quantify microplastics and perform RAMAN analyses. EF wrote the first draft of the manuscript. AG, AM, SC, and MC wrote sections of the manuscript. PG wrote the final draft of the manuscript. All authors contributed to the review of the manuscript, read and approved the submitted version.
Conflict of interest
The authors declare that the research was conducted in the absence of any commercial or financial relationships that could be construed as a potential conflict of interest.
The reviewer PS is currently organizing a Research Topic with the author PG.
Publisher’s note
All claims expressed in this article are solely those of the authors and do not necessarily represent those of their affiliated organizations, or those of the publisher, the editors and the reviewers. Any product that may be evaluated in this article, or claim that may be made by its manufacturer, is not guaranteed or endorsed by the publisher.
Supplementary material
The Supplementary Material for this article can be found online at: https://www.frontiersin.org/articles/10.3389/fenvs.2022.878152/full#supplementary-material
References
Abbasi, S. (2021). Prevalence and physicochemical characteristics of microplastics in the sediment and water of Hashilan Wetland, a national heritage in NW Iran. Environ. Technol. Innov. 23, 101782. doi:10.1016/j.eti.2021.101782
Andrady, A. L. (2017). The plastic in microplastics: A review. Mar. Pollut. Bull. 119, 12–22. doi:10.1016/j.marpolbul.2017.01.082
Backhaus, T., and Wagner, M. (2020). Microplastics in the environment: Much ado about nothing? A debate. Glob. Chall. 4 (6), 1900022. doi:10.1002/gch2.201900022
Baláž, P., Baláž, M., Sayagués, M. J., Škorvánek, I., Zorkovská, A., Dutková, E., et al. (2017). Mechanochemical solvent-free synthesis of quaternary semiconductor Cu-Fe-Sn-S nanocrystals. Nanoscale Res. Lett. 12, 256. doi:10.1186/s11671-017-2029-5
Ballent, A., Corcoran, P. L., Madden, O., Helm, P. A., and Longstaffe, F. J. (2016). Sources and sinks of microplastics in Canadian Lake Ontario nearshore, tributary and beach sediments. Mar. Pollut. Bull. 110 (1), 383–395. doi:10.1016/j.marpolbul.2016.06.037
Bertoldi, C., Lara, L. Z., Fernanda, A. D. L., Martins, F. C., Battisti, M. A., Hinrichs, R., et al. (2021). First evidence of microplastic contamination in the freshwater of lake Guaíba, Porto alegre, Brazil. Sci. Total Environ. 759, 143503. doi:10.1016/j.scitotenv.2020.143503
Bharath, M. K., Srinivasalu, S., Natesan, U., Ayyamperumal, R., Kalam, N., Anbalagan, S., et al. (2021). Microplastics as an emerging threat to the freshwater ecosystems of Veeranam lake in south India: A multidimensional approach. Chemosphere 264, 128502. doi:10.1016/j.chemosphere.2020.128502
Bikker, J., Lawson, J., Wilson, S., and Rochman, C. M. (2020). Microplastics and other anthropogenic particles in the surface waters of the Chesapeake Bay. Mar. Pollut. Bull. 156, 111257. doi:10.1016/j.marpolbul.2020.111257
Bletter, M. C. M., Ulla, M, A., Rabuffetii, A, P., and Garello, N. (2017). Plastic pollution in freshwater ecosystems: Macro-meso-and microplastic debris in a floodplain lake. Environ. Monit. Assess. 189, 581. doi:10.1007/s10661-017-6305-8
Browne, M. A., Crump, P., Niven, S. J., Teuten, E., Tonkin, A., Galloway, T., et al. (2011). Accumulation of microplastic on shorelines woldwide: Sources and sinks. Environ. Sci. Technol. 45, 9175–9179. doi:10.1021/es201811s
Camargo, A. L. G., Girard, P., Sanz-Lazaro, C., Silva, A. C. M., de Faria É, , Figueiredo, B. R. S., et al. (2022). Microplastics in sediments of the pantanal wetlands, Brazil. Front. Environ. Sci. 10, 1017480. doi:10.3389/fenvs.2022.1017480
Carr, S. A., Liu, J., and Tesoro, A. G. (2016). Transport and fate of microplastic particles in wastewater treatment plants. Water Res. 91, 174–182. doi:10.1016/j.watres.2016.01.002
Chen, X., Xiong, X., Jiang, X., Shi, H., and Wu, C. (2019). Sinking of floating plastic debris caused by biofilm development in a freshwater lake. Chemosphere 222, 856–864. doi:10.1016/j.chemosphere.2019.02.015
Cole, M., Lindeque, P. K., Fileman, E., Clark, J., Lewis, C., Halsband, C., et al. (2016). Microplastics alter the properties and sinking rates of zooplankton faecal pellets. Environ. Sci. Technol. 50 (6), 3239–3246. doi:10.1021/acs.est.5b05905
De Faria, E., Girard, P., Nardes, C. S., Moreschi, A., Christo, S. W., Junior, A. L. F., et al. (2021). Microplastics pollution in the South American pantanal. Case Stud. Chem. Environ. Eng. 3, 100088. doi:10.1016/j.cscee.2021.100088
DGEEC – Dirección General de Estadística, Encuestas y Censos (2015). Paraguay. Proyección de la Población por Sexo y Edad, según Distrito, 2000-2025. Available at: https://www.ine.gov.py/publication-single.php?codec=MTQz (Accessed on Nov 16th 2021.
Dong, M., Zhang, Q., Xing, X., Chen, W., She, Z., and Luo, Z. (2020) Raman spectra and surface changes of microplastics weathered under natural environments. Sci. Total Environ., 739, 139990. doi:10.1016/j.scitotenv.2020.139990
Echegaray, F., and Valeria, F. (2017). Assessing the intention-behavior gap in electronic waste recycling : The case of Brazil. J. Clean. Prod. 142, 180–190. doi:10.1016/j.jclepro.2016.05.064
Egessa, R., Nankabirwa, A., Ocaya, H., and Pabire, W. G. (2020). Microplastic pollution in surface water of Lake Victoria. Sci. Total Environ. 741, 140201. doi:10.1016/j.scitotenv.2020.140201
Enyoh, C. E., Verla, A. W., Verla, E. N., Ibe, F. C., and Amaobi, C. E. (2019). Airborne microplastics: A review study on method for analysis, occurrence, movement and risks. Environ. Monit. Assess. 191 (11), 668–684. doi:10.1007/s10661-019-7842-0
Fahrenfeld, N. L., Arbuckle-Keil, G., Beni, N. N., and Bartelt-Hunt, S. L. (2019). Source tracking microplastics in the freshwater environment. TrAC Trends Anal. Chem. 112, 248–254. doi:10.1016/j.trac.2018.11.030
Felismino, M. E. L., Helm, P. A., and Rochman, C. M. (2021). Microplastic and other anthropogenic microparticles in water and sediments of Lake Simcoe. J. Gt. Lakes. Res. 47 (1), 180–189. doi:10.1016/j.jglr.2020.10.007
Ferreira, G. V. B., Barletta, M., Lima, A. R. A., Dantas, D. V., Justino, A. K. S., and Costa, M. F. (2016). Plastic debris contamination in the life cycle of Acoupa weakfish (Cynoscion acoupa) in a tropical estuary. ICES J. Mar. Sci. 73 (10), 2695–2707. doi:10.1093/icesjms/fsw108
Fragão, J., Bessa, F., Otero, V., Barbosa, A., Sobral, P., Waluda, C. M., et al. (2021). Microplastics and other anthropogenic particles in Antarctica: Using penguins as biological samplers. Sci. Total Environ. 788, 147698. doi:10.1016/j.scitotenv.2021.147698
Free, C. M., Jensen, O. P., Mason, S. A., Eriksen, M., Williamson, N. J., and Boldgiv, B. (2014). High-levels of microplastic pollution in a large, remote, mountain lake. Mar. Pollut. Bull. 85 (1), 156–163. doi:10.1016/j.marpolbul.2014.06.001
Frias, J. P. G. L., and Nash, R. (2019). Microplastics: Finding a consensus on the definition. Mar. Pollut. Bull. 138, 145–147. doi:10.1016/j.marpolbul.2018.11.022
Girard, P. (2011). “Hydrology of surface and ground waters in the Pantanal floodplains,” in The pantanal: Ecology, biodiversity and sustainable management of a large neotropical seasonal wetland, 103–126.
González-Pleiter, M., Edo, C., Velázquez, D., Casero-Chamorro, M. C., Leganés, F., Quesada, A., et al. (2020). First detection of microplastics in the freshwater of an antarctic specially protected area. Mar. Pollut. Bull. 161, 111811. doi:10.1016/j.marpolbul.2020.111811
Graca, B., Szewc, K., Zakrzewska, D., Dołęga, A., and Szczerbowska-Boruchowska, M. (2017). Sources and fate of microplastics in marine and beach sediments of the southern baltic sea—A preliminary study. Environ. Sci. Pollut. Res. 24 (8), 7650–7661. doi:10.1007/s11356-017-8419-5
Gray, A. D., Wertz, H., Leads, R. R., and Weinstein, J. E. (2018). Microplastic in two South Carolina Estuaries: Occurrence, distribution, and composition. Mar. Pollut. Bull. 128, 223–233. doi:10.1016/j.marpolbul.2018.01.030
Green, D. S., Boots, B., O’Connor, N. E., and Thompson, R. (2017). Microplastics affect the ecological functioning of an important biogenic habitat. Environ. Sci. Technol. 51 (1), 68–77. doi:10.1021/acs.est.6b04496
Hartmann, N. B., Huffer, T., Thompson, R. C., Hassellöv, M., Verschoor, A., Daugaard, A. E., et al. (2019). “Are we speaking the same language?,” in Recommendations for a definition and categorization framework for plastic debris, 1039–1047. doi:10.1021/acs.est.8b05297
Helcoski, R., Yonkos, L. T., Sanchez, A., and e Baldwin, A. H. (2020). Wetland soil microplastics are negatively related to vegetation cover and stem density. Environ. Pollut. 256, 113391. doi:10.1016/j.envpol.2019.113391
Henderson, L., and Green, C. (2020). Making sense of microplastics? Public understandings of plastic pollution. Mar. Pollut. Bull. 152, 110908. doi:10.1016/j.marpolbul.2020.110908
Hidalgo-Ruz, V., Gutow, L., Thompson, R. C., and Thiel, M. (2012). Microplastics in the marine environment: A review of the methods used for identification and quantification. Environ. Sci. Technol. 46 (6), 3060–3075. doi:10.1021/es2031505
Horton, A. A., and Dixon, S. J. (2018). Microplastics: An introduction to environmental transport processes. WIREs Water 5, 1–10. doi:10.1002/wat2.1268
Horton, A. A., Walton, A., Spurgeon, D. J., Lahive, E., and Svendsen, C. (2017). Microplastics in freshwater and terrestrial environments: Evaluating the current understanding to identify the knowledge gaps and future research priorities. Sci. Total Environ. 586, 127–141. doi:10.1016/j.scitotenv.2017.01.190
Huang, W., Song, B., Liang, J., Niu, Q., Zeng, G., Shen, M., et al. (2021). Microplastics and associated contaminants in the aquatic environment: A review on their ecotoxicological effects, trophic transfer, and potential impacts to human health. J. Hazard. Mat. 405, 124187. doi:10.1016/j.jhazmat.2020.124187
Huntington, A., Corcoran, P. L., Jantunen, L., Thaysen, C., Bernstein, S., Stern, G. A., et al. (2020). A first assessment of microplastics and other anthropogenic particles in Hudson Bay and the surrounding eastern Canadian Arctic waters of Nunavut. Facets 5, 432–454. doi:10.1139/facets-2019-0042
IBGE – INSTITUTO BRASILEIRO DE GEOGRAFIA E ESTATÍSTICA (2021). Estimativas da população 2021; Estimativas da população residente para os municípios e para as unidades da federação com data de referência em 1º de julho de 2021; Nota metodológica n. 01. Available at: https://biblioteca.ibge.gov.br/index.php/biblioteca-catalogo?view=detalhes&id=2101849 (Accessed on Nov. 16th 2021.
Ivar Do Sul, J. A., and Costa, M. F. (2014). The present and future of microplastic pollution in the marine environment. Environ. Pollut. 185, 352–364. doi:10.1016/j.envpol.2013.10.036
Jahnke, A., Arp, H. P. H., Escher, B. I., Gewert, B., Gorokhova, E., Kühnel, D., et al. (2017). Reducing uncertainty and confronting ignorance about the possible impacts of weathering plastic in the marine environment. Environ. Sci. Technol. Lett. 4 (3), 85–90. doi:10.1021/acs.estlett.7b00008
Jian, M., Zhang, Y., Yang, W., Zhou, L., Liu, S., and Xu, E. G. (2020). Occurrence and distribution of microplastics in China’s largest freshwater lake system. Chemosphere 261, 128186. doi:10.1016/j.chemosphere.2020.128186
Jiao, M., Cao, S., Ren, L., and Li, R. (2021). Analysis of composite microplastics in sediment using 3D Raman spectroscopy and imaging method. J. Hazard. Mater. Adv. 3, 100016. doi:10.1016/j.hazadv.2021.100016
Koelmans, A. A., Nor, N. H. M., Hermsen, E., Kooi, M., Mintenig, S. M., and De France, J. (2019). Microplastics in freshwaters and drinking water: Critical review and assessment of data quality. Water Res. 155, 410–422. doi:10.1016/j.watres.2019.02.054
Kumar, R., Sharma, P., Verma, A., Jha, P. K., Singh, P., Gupta, P. K., et al. (2021a). Effect of physical characteristics and hydrodynamic conditions on transport and deposition of microplastics in riverine ecosystem. Water 13, 2710. doi:10.3390/w13192710
Kumar, R., Sharma, P., and Bandyopadhyay, S. (2021b). Evidence of microplastics in wetlands: Extraction and quantification in Freshwater and coastal ecosystems. J. Water Process Eng. 40, 101966. doi:10.1016/j.jwpe.2021.101966
Leads, R. R., and Weinstein, J. E. (2019). Occurrence of tire wear particles and other microplastics within the tributaries of the Charleston Harbor Estuary, South Carolina, USA. Mar. Pollut. Bull. 145, 569–582. doi:10.1016/j.marpolbul.2019.06.061
Lebreton, L. C. M., van der Zwet, J., Damsteeg, J.-W., Slat, B., Andrady, A., and Reisser, J. (2017). River plastic emissions to the world’s oceans. Nat. Commun. 8, 15611. doi:10.1038/ncomms15611
Lechner, A., Keckeis, H., Lumesberger-Loisl, F., Zens, B., Krusch, R., Tritthart, M., et al. (2014). The Danube so colourful: A potpourri of plastic litter outnumbers fish larvae in europe's second largest river. Environ. Pollut. 188, 177–181. doi:10.1016/j.envpol.2014.02.006
Li, Q., Jiang, N., Yu, X., Dong, Z., Duan, S., Zhang, L., et al. (2019). Sources and spatial distribution of PM2. 5-bound polycyclic aromatic hydrocarbons in Zhengzhou in 2016. Atmos. Res. 216, 65–75. doi:10.1016/j.atmosres.2018.09.011
Li, C., Busquets, R., and Campos, L. C. (2020a). Assessment of microplastics in freshwater systems: A review. Sci. Total Environ. 707, 135578. doi:10.1016/j.scitotenv.2019.135578
Li, Y., Zhang, H., and Tang, C. (2020b). A review of possible pathways of marine microplastics transport in the ocean. Anthr. Coasts 3 (1), 6–13. doi:10.1139/anc-2018-0030
Lima, A. R. A., Barletta, M., and Costa, M. F. (2015). Seasonal distribution and interactions between plankton and microplastics in a tropical estuary. Estuar. Coast. Shelf Sci. 165, 213–225. doi:10.1016/j.ecss.2015.05.018
Liu, J., Man, R., Ma, S., Li, J., Wu, Q., and Peng, J. (2015). Atmospheric levels and health risk of polycyclic aromatic hydrocarbons (PAHs) bound to PM2. 5 in Guangzhou, China. Mar. Pollut. Bull. 100 (1), 134–143. doi:10.1016/j.marpolbul.2015.09.014
Liu, S., Jian, M., Zhou, L., and Li, W. (2019). Distribution and characteristics of microplastics in the sediments of Poyang Lake, China. Water Sci. Technol. 79 (10), 1868–1877. doi:10.2166/WST.2019.185
Mao, R., Lang, M., Yu, X., Wu, R., Yang, X., and Guo, X. (2020). Aging mechanism of microplastics with UV irradiation and its effects on the adsorption of heavy metals. J. Hazard. Mat. 393, 122515. doi:10.1016/j.jhazmat.2020.122515
Marinha, M. S. S. (2011). Níveis de cádmio em sangue e urina dos habitantes do entorno de uma reserva ecológica no Pantanal Mato-grossense. Master 's Dissertation. Rio de Janeiro: Escola Nacional de Saúde Pública Sergio Arouca.
Munno, K., De Frond, H., O’Donnell, B., and Rochman, C. M. (2020). Increasing the accessibility for characterizing microplastics: Introducing new application-based and spectral libraries of plastic particles (SLoPP and SLoPP-E). Anal. Chem. 92, 2443–2451. doi:10.1021/acs.analchem.9b03626
Napper, I. E., Barrett, A. C., and Thompson, R. C. (2020). The efficiency of devices intended to reduce microfibre release during clothes washing. Sci. Total Environ. 738, 140412. doi:10.1016/j.scitotenv.2020.140412
Naqash, N., Prakash, S., Kapoor, D., and Singh, R. (2020). Interaction of freshwater microplastics with biota and heavy metals: A review. Environ. Chem. Lett. 18 (6), 1813–1824. doi:10.1007/s10311-020-01044-3
Nizzetto, L., Bussi, G., Futter, M. N., Butterfield, D., and Whitehead, P. G. (2016). A theoretical assessment of microplastic transport in river catchments and their retention by soils and river sediments. Environ. Sci. Process. Impacts 18 (8), 1050–1059. doi:10.1039/C6EM00206D
Padha, S., Kumar, R., Dhar, A., and Sharma, P. (2022). Microplastic pollution in mountain terrains and foothills: A review on source, extraction, and distribution of microplastics in remote areas. Environ. Res. 207, 112232. doi:10.1016/j.envres.2021.112232
Park, H. J., Oh, M. J., Kim, P. G., Kim, G., Jeong, D. H., Ju, B. K., et al. (2020). National reconnaissance survey of microplastics in municipal wastewater treatment plants in Korea. Environ. Sci. Technol. 54 (3), 1503–1512. doi:10.1021/acs.est.9b04929
Pastorino, P., Pizzul, E., Bertoli, M., Anselmi, S., Kušće, M., Menconi, V., et al. (2021). First insights into plastic and microplastic occurrence in biotic and abiotic compartments, and snow from a high-mountain lake (Carnic Alps). Chemosphere 265, 129121. doi:10.1016/j.chemosphere.2020.129121
Prata, J. C., Reis, V., Matos, J. T. V., da Costa, J. P., Duarte, A. C., and Rocha-Santos, T. (2019). A new approach for routine quantification of microplastics using Nile Red and automated software (MP-VAT). Sci. Total Environ. 690, 1277–1283. doi:10.1016/j.scitotenv.2019.07.060
Qian, J., Tang, S., Wang, P., Lu, B., Li, K., Jin, W., et al. (2021). From source to sink: Review and prospects of microplastics in wetland ecosystems. Sci. Total Environ. 758, 143633. doi:10.1016/j.scitotenv.2020.143633
Regolin, A. L., Oliveira-Santos, L. G., Ribeiro, M. C., and Bailey, L. L. (2021). Habitat quality, not habitat amount, drives mammalian habitat use in the Brazilian Pantanal. Landsc. Ecol. 36, 2519–2533. doi:10.1007/s10980-021-01280-0
Reis, C., Oliveira, D., Moura, A., and Engel, A. (2012). Collection and recycling of electronic scrap : A worldwide overview and comparison with the Brazilian situation. Waste Manag. 32, 1592–1610. doi:10.1016/j.wasman.2012.04.003
Scheurer, M., and Bigalke, M. (2018). Microplastics in Swiss floodplain soils. Environ. Sci. Technol. 52, 3591–3598. doi:10.1021/acs.est.7b06003
Silva-Cavalcanti, J. S., Silva, J. D. B., França, E. J. d., Araújo, M. C. B. d., and Gusmão, F. (2017). Microplastics ingestion by a common tropical freshwater fishing resource. Environ. Pollut. 221, 218–226. doi:10.1016/j.envpol.2016.11.068
Simon, M., Vianello, A., Shashoua, Y., and Vollertsen, J. (2021). Accelerated weathering affects the chemical and physical properties of marine antifouling paint microplastics and their identification by ATR-FTIR spectroscopy. Chemosphere 274, 129749. doi:10.1016/j.chemosphere.2021.129749
Sruthy, S., and Ramasamy, E. V. (2017). Microplastic pollution in vembanad lake, Kerala, India: The first report of microplastics in Lake and estuarine sediments in India. Environ. Pollut. 222, 315–322. doi:10.1016/j.envpol.2016.12.038
Su, L., Cai, H., Kolandhasamy, P., Wu, C., Rochman, C. M., and Shi, H. (2018). Using the Asian clam as an indicator of microplastic pollution in freshwater ecosystems. Environ. Pollut. 234, 347–355. doi:10.1016/j.envpol.2017.11.075
Sutton, R., Mason, S. A., Stanek, S. K., Willis-Norton, E., Wren, I. F., and Box, C. (2016). Microplastic contamination in the san francisco bay, California, USA. Mar. Pollut. Bull. 109, 230–235. doi:10.1016/j.marpolbul.2016.05.077
Talvitie, J., Heinonen, M., Pääkkönen, J. P., Vahtera, E., Mikola, A., Setälä, O., et al. (2015). Do wastewater treatment plants act as a potential point source of microplastics? Preliminary study in the coastal gulf of Finland, baltic sea. Water Sci. Technol. 72 (9), 1495–1504. doi:10.2166/wst.2015.360
Tomas, W. M., de Oliveira Roque, F., Morato, R. G., Medici, P. E., Chiaravalloti, R. M., Tortato, F. R., et al. (2019). Sustainability agenda for the pantanal wetland: Perspectives on a collaborative interface for science, policy, and decision-making. Trop. Conserv. Sci. 12, 194008291987263. doi:10.1177/1940082919872634
Truong, T., Strady, E., Kieu-Le, T., Tran, Q., Le, T., and Thuong, Q. (2021). Microplastic in atmospheric fallouts of a developing Southeast Asian megacity under tropical climate. Chemosphere 272, 129874. doi:10.1016/j.chemosphere.2021.129874
Vaughan, R., Turner, S. D., and Rose, N. L. (2017). Microplastics in the sediments of a UK urban lake. Environ. Pollut. 229, 10–18. doi:10.1016/j.envpol.2017.05.057
Wang, T., Zou, X., Li, B., Yao, Y., Zang, Z., Li, Y., et al. (2019). Preliminary study of the source apportionment and diversity of microplastics: Taking floating microplastics in the South China sea as an example. Environ. Pollut. 245, 965–974. doi:10.1016/j.envpol.2018.10.110
Wang, Q., Huang, K., Li, Y., Zhang, Y., Yan, L., Xu, K., et al. (2021). Microplastics abundance, distribution, and composition in freshwater and sediments from the largest Xijin Wetland Park, Nanning, South China. Gondwana Res. 108, 13–21. doi:10.1016/j.gr.2021.07.009
Wantzen, K. M., da Cunha, C. N., Junk, W. J., Girard, P., Rossetto, O. C., Penha, J. M., et al. (2008). Towards a sustainable management concept for ecosystem services of the Pantanal wetland. Ecohydrol. Hydrobiology 8 (2), 115–138. doi:10.2478/v10104-009-0009-9
Yan, M., Wang, L., Dai, Y., Sun, H., and Liu, C. (2021). Behavior of microplastics in inland waters: Aggregation, settlement, and transport. Bull. Environ. Contam. Toxicol. 107, 700–709. doi:10.1007/s00128-020-03087-2
Yang, D., Shi, H., Li, L., Li, J., Jabeen, K., and Kolandhasamy, P. (2015). Microplastic pollution in table salts from China. Environ. Sci. Technol. 49 (22), 13622–13627. doi:10.1021/acs.est.5b03163
Yang, L., Zhang, Y., Kang, S., Wang, Z., and Wu, C. (2021). Microplastics in freshwater sediment: A review on methods, occurrence, and sources. Sci. Total Environ. 754, 141948. doi:10.1016/j.scitotenv.2020.141948
Zhang, J., Wu, X., Guo, H., Zheng, X., and Mai, B. (2021). Pollution of plastic debris and halogenated flame retardants (HFRs) in soil from an abandoned e-waste recycling site: Do plastics contribute to (HFRs) in soil? J. Hazard. Mater. 410, 124649. doi:10.1016/j.jhazmat.2020.124649
Zhou, B., Wang, J., Zhang, H., Shi, H., Fei, Y., Huang, S., et al. (2020). Microplastics in agricultural soils on the coastal plain of Hangzhou Bay, east China: Multiple sources other than plastic mulching film. J. Hazard. Mat. 388, 121814. doi:10.1016/j.jhazmat.2019.121814
Keywords: microplastics, Pantanal, microsynthetics, pollution, floodplains, wetlands
Citation: De Faria É, Girard P, Gomes Camargo AL, Moreschi Silva AC, Christo SW, Casado Coy N, Sanz-Lazaro C and Costa MF (2022) Microsynthetics in waters of the South American Pantanal. Front. Environ. Sci. 10:878152. doi: 10.3389/fenvs.2022.878152
Received: 17 February 2022; Accepted: 02 November 2022;
Published: 18 November 2022.
Edited by:
Sandra Olga Brizga, Brizga Environmental, AustraliaReviewed by:
Prabhakar Sharma, Nalanda University, IndiaQinghui Huang, Tongji University, China
Lars Hildebrandt, Helmholtz Centre for Materials and Coastal Research (HZG), Germany
Copyright © 2022 De Faria, Girard, Gomes Camargo, Moreschi Silva, Christo, Casado Coy, Sanz-Lazaro and Costa. This is an open-access article distributed under the terms of the Creative Commons Attribution License (CC BY). The use, distribution or reproduction in other forums is permitted, provided the original author(s) and the copyright owner(s) are credited and that the original publication in this journal is cited, in accordance with accepted academic practice. No use, distribution or reproduction is permitted which does not comply with these terms.
*Correspondence: Pierre Girard, pierregirard1301@gmail.com