- 1Department of Geology, University at Buffalo, Buffalo, NY, United States
- 2Department of Geosciences, Austin Peay State University, Clarksville, TN, United States
- 3Department of Geoscience, Winona State University, Winona, MN, United States
- 4Department of Geological Engineering, Ankara University, Ankara, Turkey
- 5Department of Geosciences, Union College, Schenectady, NY, United States
- 6Department of Geology, San Jose State University, San Jose, CA, United States
- 7School of Earth and Environmental Sciences, Cardiff University, Cardiff, United Kingdom
- 8School of Civil and Environmental Engineering, The University of New South Wales, Kensington, NSW, Australia
The training of geological scientists, more so than any other natural science, is dependent on how students learn to visualize and interpret complex three-dimensional problems at scales from micrometers to kilometers over time scales that span from seconds to centuries. Traditionally, our classrooms are at a disadvantage due to our standard two-dimensional use of whiteboards or slide decks. We are at an even bigger disadvantage when courses go to online education. While computer simulations and three-dimensional visualizations are used, they can lack the flexibility for students to perform free-form exploration. The novelty of this research is in the use of paper aquifer models and their implementation across seven academic institutions to provide three-dimensional physical examples for students to visualize subsurface geologic structure and quantify fluid flow through porous media. Students can cut, fold, and build three-dimensional hydrologic problems at home or in the classroom. Our methodology allows students to physically rotate their aquifer models to visualize cross-sectional areas, layer thicknesses, heterogeneity, and confining units. These foldable paper models provide a low barrier of entry for students to understand and quantify the relationships between water levels and geologic structure. Our experience using these models in both in-person and online classrooms highlights the advantages and disadvantages of these models. Results, although mostly anecdotal, suggest the paper models improve students’ learning and enhance their engagement with the material. The formal evaluations of pre- and post-model implementation show that low-scoring students had the most significant gains after being introduced to the paper aquifer models. At the same time, there was no change in the number of students in the highest scoring group. Our experience in the classroom points to new opportunities to engage with remote learners and tools for supporting flipped classroom activities. Our vision for the paper aquifer models is to provide the hydrologic community with an additional tool to help bridge the virtual classroom gap, engage students, and help them develop mastery of three-dimensional problem-solving.
Introduction
Of all the natural sciences, the geological sciences arguably have the strongest association with multidimensional research problems (King, 2008). This can lead to difficulty in the training of future geoscientists as visualizing multidimensional problems forces students away from fact-based crystallized intelligence to more malleable learning using their fluid intelligence (Jaeger et al., 2017; Bresciani Ludvik, 2021). An example includes students learning to map dipping and striking geologic strata in order to build geologic maps, where they integrate fact-based identification of rock types and field observations to create complex three-dimensional representations of the subsurface (Ishikawa and Kastens, 2005; Clark et al., 2008; Kuiper, 2008; Chenrai, 2021). These are open-ended problems where students must integrate their knowledge across a range of information (Dickerson et al., 2005). Similar examples in the hydrologic sciences include interpreting geologic wellbore data to create aquifer maps or fence diagrams. In these problems, students integrate scientific knowledge from point locations to create three-dimensional models where flexibility in interpolation is led by expert knowledge and exact solutions are unknown. In such contexts, three-dimensional visualization is a core student skill and must be further developed in order to be successful professionals. Yet, as educators, we spend much of our time teaching in a two-dimensional world on whiteboards or using slide decks. We continue to evaluate students on their fact-based crystallized intelligence and do not open the opportunity for fluidity in student exploration of geologic problems. This gap increased as our classroom settings drastically changed in the face of online and hybrid instruction.
Difficulties in teaching three-dimensional thinking were exacerbated due to the COVID-19 pandemic as the majority of our educational system, for at least some period of time, was moved to online learning. This change forced a cohort of students to follow video lectures with limited hands-on or field opportunities. In addition, faculty were given limited time and resources to change the historical ways in which we teach (Garcia-Vela et al., 2020). The situation, although challenging, provided room for educational opportunities (Andrews et al., 2020) and the potential for a more inclusive community (Bursztyn et al., 2022). The abrupt shift to virtual learning set the stage for the advancement of new off-the-shelf teaching tools to reconnect students to hands-on opportunities to visualize and solve three-dimensional problems in the geosciences. Here we present the use of low-technology origami-inspired paper aquifer models and describe their use across seven academic institutions.
The motivation for these paper aquifer models is rooted in teaching the governing equations for groundwater flow. The application of three-dimensionality in groundwater science is directly connected to the formulation of Darcy’s Law, accounting for the change in hydraulic head through space within a geologic unit and the cross-sectional area over which groundwater flows. While these concepts can be simple for students when dealing with visible sediment-filled columns, it becomes more difficult when translating to flow through hidden, subsurface, regional aquifer systems. These large regional aquifer systems represent a scale that can be difficult to visualize by the novice student and typically poorly illustrated in scientific reports, presentations, and on a classroom whiteboard. Our experience with students has shown across institutions that simple ideas like defining the cross-sectional area that groundwater flows through an aquifer can be extremely difficult to visualize by students. This cross-sectional area is often confused as being parallel to the hydraulic gradient, not perpendicular. This has led our group to think beyond two-dimensional illustrations and develop origami inspired three-dimensional paper aquifer models.
The origami inspired models were therefore developed to support the instructional need for students to visualize three-dimensional groundwater problems. The use of these models acts to slow students down in their attempt to search for an equation with the same number of variables given in a homework problem. The concept of students slowing down their minds to think and solve problems during COVID-19 pandemic instruction is a theme that may be a bright spot emerging in the literature (Phillips et al., 2021). Models are designed for students to make actual measurements using the model geometry and then apply these measurements to arrive at a solution. The value of these interactive teaching tools became increasingly apparent as many classrooms transitioned to online learning. Our group discovered that through these simple models, students are able to physically build models and explore concepts of three-dimensional groundwater flow in a much more open and tangible environment as compared to traditional assignments. Importantly, these paper models focus the students’ attention on the physical conceptualization of the problem of interest—something that is easy for students to lose sight of when dealing with new concepts in solely text-based problems.
While computer generated three-dimensional visualizations are impressive, it is highly effective to supplement these visualizations with low-tech paper aquifer models. Additionally, in most computer models, two-dimensional projections of three-dimensional models are presented, which can be difficult to understand (Kuiper, 2008). On a practical level, these paper models take less time to prepare when compared to computer models and can provide flexibility in the types of problems that can be assigned to students. They can also easily be distributed to students as handouts or through email, removing technological barriers to access and use.
The motivation of this work is to present a new set of tools to help students visualize three-dimensional groundwater flow through a series of hands-on exercises, and to demonstrate and discuss their pedagogical effectiveness based on reflective practice in the classroom across multiple institutions and teaching settings. These models are simple to use and easy to adapt to a range of groundwater related assignments both in traditional and online classrooms. Our goal is to provide instructors with descriptions of where these models have been used and when these methods have been most useful. The contribution of this work can be applied to traditional and online classroom environments. We do this using an open science framework that allows users to take and adapt our models to a wide range of hydrologic problems.
Classroom methodology
The foldable aquifer models are based on rectangular cuboid paper models that are cut and glued together by the students (Figure 1). Each model has an associated problem set that can be used by the instructor, or instructors can choose to use the paper model by itself with their own specific set of questions. The current suite of models includes problems related to porosity/sediment packing, Darcy flux, radial flow, pumping tests, and image wells, with approximately 20 models in the initial offerings (Supplementary Table S1). Across most institutions, online students are expected to print out a given model and construct the model at home based on the provided instructions (Figure 1). In some cases, faculty were able to provide paper copies of the models to students in advance, and in one instance these were printed on card-stock and pre-cut. Unlike traditional hydrologic assignments, students must use these models to measure geologic thickness, hydraulic heads, and distance to boundary conditions, which are all drawn to scale. This reduces the ability of students to simply look at an assignment-provided set of variables and then find an equation where all variables are applied. Through building and then making measurements of a given model, students must use their full knowledge base to solve these assignments.
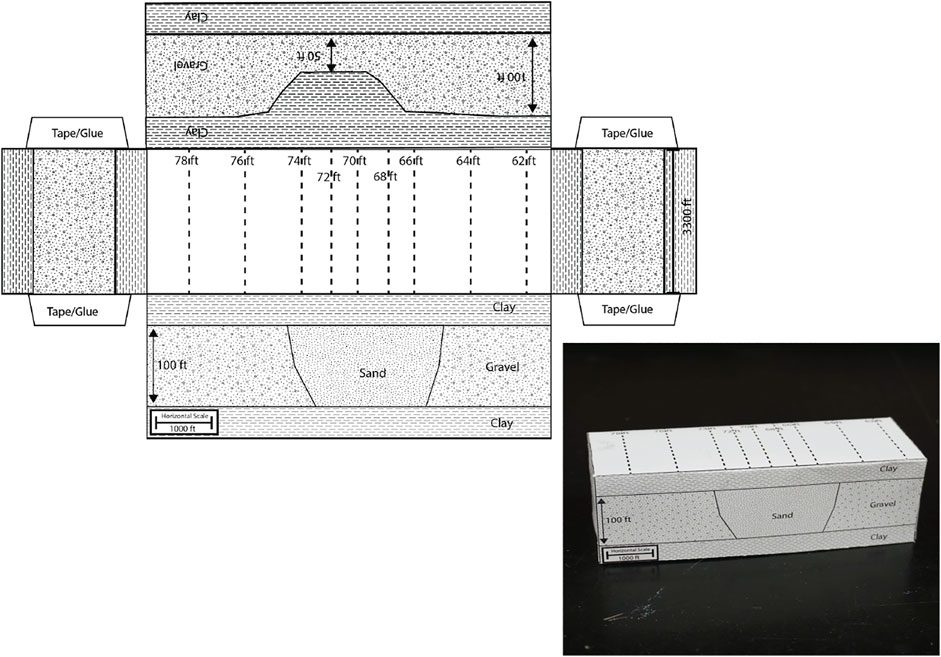
FIGURE 1. Example of a paper aquifer model used to illustrate the relationship between hydraulic conductivity, aquifer thickness, and hydraulic head in confined aquifers.
The barrier of entry for these models is designed to be low but can still create challenges for implementation. Students are given these models as single sheets of paper or are asked to print them out, then cut and glue/tape the models into their three-dimensional form. Limited instructions are given as to the direction to fold the paper as the aquifer images are only printed on one side of the sheet. During the COVID-19 pandemic, limits to the adoption of these models occurred as some universities did not expect students to have access to printers at home (Becker, M. personal communication 9/28/2021). The literature shows that during the COVID-19 pandemic, the lack of printers in students’ homes can be as high as 20% (Kanetaki et al., 2021). While this implementation is not perfect, most students had the resources and ability to construct these models with limited extra help from the faculty over various institutions (Table 1).
Across the institutions represented here, these aquifer models were applied to four general categories of course instruction: homework, flipped classroom, breakout rooms, and class projects (Table 1). Homework is defined here as a problem set designed to be completed outside the formal classroom environment. While flipped classrooms do not have a standard definition (Song et al., 2017), in this context, this type of classroom is defined by students learning terminology and baseline knowledge on their own at home through online lecture videos and readings outside of the scheduled course time, and then applying and developing that knowledge during course time through activities and discussions guided by the faculty in the classroom. Breakout rooms represent small group assignments embedded in traditional lecture format. Class projects are defined as comprehensive assignments designed to incorporate multiple modes of knowledge to solve a multi-step problem. Models were used in both in-person classrooms and remote learning from 2019 through 2022 and of varying course enrollments from eight students in-person to 101 students online. The majority of models were downloaded from the Foldable Aquifer Project web page (http://aquifer.geology.buffalo.edu) with the exception of those used at Cardiff University, which was specifically designed for an integrated class project. Not all faculty used the associated problem sets supplied with each aquifer model, some faculty chose to write their own problems based on a specific aquifer model.
The implementation across seven academic institutions represents a heterogeneous group of faculty implementing these paper models in the classroom. This project was not designed to be a pedagogical study. This implementation is a reaction to the need to engage students as many institutions went online due to the COVID-19 pandemic and, as such, describes our experience across a diverse range of institutions. This project should not be confused with the full-scale implementation of a curriculum and instruction educational research paper. In general, a qualitative assessment of the effectiveness of the aquifer models in the classroom is based on anecdotal feedback from students, except for the implementation of a formal evaluation of the flipped classroom implementation pre- and post-model use at Austin Peay State University. Anecdotal feedback comes from informal conversations with students, primarily during office hours, which is typically biased toward students struggling with particular concepts or problems. Feedback from students helped the instructors gauge the usefulness of these models and allow for modification of the implementation of these models in the classroom.
Results and discussion
The implementation of paper aquifer models across institutions represents a variety of applications; all focused on maintaining engagement. These simple models increase student participation in hands-on activities while retaining the universal desire during the COVID-19 pandemic to keep workloads affordable for teachers and students (Lepp et al., 2021). Challenges across institutions have addressed formal classroom assessment techniques through the faculty transition to online education. This lack of classroom assessment was the norm during the pandemic, not the exception (Fisher and Tatomir, 2022). The results and discussion presented herein describe how these paper aquifer models were used and, when possible, document students’ reactions and outcomes.
Homework
The original use of these models was as homework assignments, implemented by three of the seven institutions represented here, where students build and solve these problems on their own. Across the institutions, adoption of the models as homework assignments ranged from no use to using one model per homework assignment. As expected, participation among students was mixed, with some students folding every model while others did not fold the models but took measurements off the unfolded templates. In the case of students who did not cut out and fold the models, some students could still easily solve the problem. These students represent a group of students who already have the skills to interpret three-dimensional problems given a two-dimensional example. The rationale for not folding the models was to save time in completing the homework assignments, as described by one student during office hours. However, a group of students could not visualize the unfolded models in three dimensions and were unable to arrive at the correct solution early on in the course. In most cases, students learned from their early mistakes and took the time to fold the models.
In general, students enjoyed using the paper aquifer models used in homework assignments. All models were simple enough for students to construct independently without instructions. Those students who had the skills to visualize the problems without folding were not required to fold the model. Students who needed the models to visualize the problem fully did not complain about their use. An unforeseen advantage of using these models was the pride students took in building the models and then displaying them. This created a touch point where students were reminded of information presented in class long after a homework assignment was turned in.
Flipped classroom
The flipped classroom methodology was used in three of the institutions represented here. After watching the online videos and reading prior to class, students were then given the models and quantitative problems centered around the aquifer models to work with during class. These activities occurred several times throughout the semester at multiple institutions and students had positive responses to faculty members and teaching assistants actively interacting with students using the aquifer models in the flipped classroom format, including a willingness to be photographed with their models for social media. Written student feedback at the end of the course focused on the usefulness of the paper models; a representative example response stated “3D aquifer modeling proved its usefulness during the build-up toward our final project, which included an in-depth analysis of possible future locations for municipal wells in a fictional city….models helped us visualize the aquifers’ cross-section, thickness, and hydraulic gradient in a three-dimensional format, which was significant when studying the underground geology and analyzing the groundwater flow of the city...” At one institution, Austin Peay State University, formal evaluations of student learning pre- and post-use of the aquifer models in a flipped classroom format were evaluated. The instructor of the flipped hydrogeology course collected significant amounts of qualitative and quantitative data in order to determine the effectiveness of this flipped pedagogical change, with preliminary results indicating the flipped classroom increased students’ persistence, learning, and attitudes toward the course (Dunkle and Yantz, 2021). The instructor has continued to collect these data, which allows for comparisons between the pre-paper model (Fall 2015; Spring 2017; and Fall 2018; n = 35) and post-paper model (Spring 2020 and Fall 2021; n = 29) iterations of the course. Students’ learning was assessed through their scores on homework, quiz, and exam questions, while student persistence was defined as the motivation to complete all assignments. Results indicate that both learning and persistence increased with the use of the paper models for Darcy’s Law and Storage concepts.
Signs of increased engagement in homework were observed as the percentage of students who attempted homework assignments increased. The largest gains in persistence occurred with a homework assignment addressing the concept of groundwater storage, with an increase from 80% of students’ pre-paper models who attempted these groundwater storage problems to 93% post-paper models. Students’ perceptions of their own learning for Darcy’s Law were measured through Anonymous Learning Surveys, which showed minimal change from pre- to post-paper model implementation across all students (Supplementary Table S2). Results from the Likert scale questions for Darcy’s Law (Supplementary Table S3) also indicated similarities in learning perception pre- and post-paper models, with minimal increases 1 week after the activity. Increased learning observations include gains in mean scores and a decrease in the number of students with low scores in homework, quiz, and exam questions (Supplementary Table S4). Most notably, the mean scores from three multiple choice final exam questions related to Darcy’s Law and requiring calculations also increased from 60% (pre-paper models) to 76% (post-paper models). While there are limitations to this study given the small number of participants, the variety of data collected and overall results (Supplementary Material) indicate the use of these paper models may increase student’s persistence and learning, especially in the longer term as indicated by larger gains in the final exam questions. Results of perceptions of learning indicate minimal differences, with the exception of an increase in topics related to Darcy’s Law being least understood initially. This is likely due to an increased awareness of the topic due to the use of the paper models for calculating discharge and ultimately average linear groundwater velocity.
In-class example and lab prep
At San José State University, the foldable porosity model was used in an in-person setting to cover the general description of porosity as well as the associated problem set to derive porosity geometrically. While the use of the 3D model was not formally assessed, the scores for the subsequent lab assignment on porosity (the first lab of the semester) were higher in the Spring of 2020 (96 ± 9%, n = 9) when the model was not used than in the Fall of 2021 when the model was used (89 ± 7%, n = 8). While the grades did not show an improvement in the lab using the paper aquifer model, anecdotally, the model allowed students to visualize the concept of grain packing, review volume calculations, and also give them a hands-on 3D model to work with.
Zoom breakout rooms
One of the more challenging aspects of remote teaching is identifying ways to effectively engage students to actively participate in learning activities rather than simply turning off their cameras and tuning out. Additionally, for at least some students, the normal fear and anxiety of participating in a traditional classroom setting seemed to be amplified by the transition to virtual learning and the knowledge that if you contributed to a discussion or answered a question all eyes were looking directly at you.
At Winona State University, to promote an active learning environment where students felt comfortable participating, the paper models were used as part of Zoom breakout room exercises. After introducing a concept either with a short lecture over Zoom or following more of a flipped classroom approach, three to four students were sent to each of the breakout rooms (students picked up or were mailed hard copies of the foldable aquifers at the beginning of the semester) and asked to work on one or more of the problems that accompanied the model (students were told in advance which models needed to be cut out for class). After allowing the students an appropriate amount of time to begin to work on the problem, the instructor rotated through each of the breakout rooms to check on progress and answer any questions. In many cases when the instructor entered each room the students were chatting with each other and collaboratively working through the problem. Anecdotally, the models appeared to help students visualize important hydrogeological concepts. Perhaps more importantly, the models were a truly valuable mechanism by which students could connect with each other in the virtual environment.
Integrated class project
For use in two different MSc level classes (n = 24) at Cardiff University, a bespoke origami problem was designed to test students’ ability to link a number of hydrogeological concepts together based on learning materials given in introductory lectures on various aspects of physical hydrogeology. The problem was based on the movement of a conservative contaminant within a layered aquifer system separated by a layered aquitard. This required them to first conceptualize, in 3-D, the overall qualitative nature of the likely flowpaths of a contaminant moving through the system both horizontally and vertically, based on supplied borehole data. They then broke the problem down into separate components for calculation by different individuals in their groups which included: a 3-point flow problem in different directions in each aquifer, effective hydraulic conductivity of the aquitard, and the direction and advective velocity through each layer. A final solution was required to be presented as to the likely location and timescale of the solute breakthrough at the edge of the model. Each group annotated their paper models and presented them at the front of the class for ranking as to the best solution, before a class discussion about the learning experience and clarification of any concepts which were not yet clear. The students clearly enjoyed the competitive and interactive nature of the exercise, seemed proud of their models, and showed a discernible development of their understanding throughout the class of the important concepts. Written student feedback at the end of the course described the use of the paper aquifer models as “...very helpful for visualizing the spread of contamination.” General student evaluation comments described the use of these models as “fun,” “interactive,” and “engaging.” In the subsequent mid-term anonymous module feedback this class activity was the one that the cohort singled out most often as positively facilitating their learning experience.
Conclusion
There are challenges associated with teaching the directly unobservable concepts of groundwater science due to the fact that groundwater is a hidden resource. This became even more difficult during a global pandemic where courses at many institutions were forced online. The role of visualization in learning basic hydrogeological concepts like Darcy’s law is incontrovertible. Being novice learners of groundwater science, students need help while dealing with three-dimensional visualization of these concepts, no matter in which environment the courses are carried out. According to the experiences gained by seven different faculty members in different institutions during Hydrogeology or related courses, the paper models presented in this study help with three-dimensional visualization and filled a much-needed deficiency in hands-on activities when courses went to online instruction. A combination of anecdotal and formal course evaluations showed that student learning is enhanced with the utilization of these models. The faculty noted the added value of students slowing down to take time to fold the paper aquifer models, slowing down to take more time to think about problems has been noted in the literature as a beneficial change during the COVID-19 pandemic. Results also show these models provided a second touch point as students took ownership in building these models resulting in the paper models sitting on desks and shelves. Across all seven institutions, there is clearly variability in participation rates. Based on interactions during office hours and informal discussions, many students who struggled with basic concepts seemed to appreciate the models. Some students who attended office hours did admit to not taking the time to fold the model. However, when these students were shown the folded models during office hours, all attendees appeared to recognize the value in visualizing the problems in 3D, and many went back and took the time to fold the paper model. As faculty, we are not naive enough to think that students do not take shortcuts, but it is nice to have evidence that struggling students went back and took the time to execute the problems as originally designed.
Across the institutions represented here, the overall perception was that the paper aquifer models were a constructive tool to increase student learning independent of the classroom format, assuming students took the time to participate. These results are primarily based on anecdotal evidence, with the exception of one institution that performed pre- and post-assessments. This generally low level of formal assessments by the faculty also follows literature trends during the COVID-19 pandemic, when faculty were just trying to survive. The formal evaluations of pre- and post-model implementation show that low-scoring students had the most significant gains after being introduced to the paper aquifer models. At the same time, there was no change in the number of students in the highest scoring group. These results need further investigation with large sample sizes but follow anecdotal evidence that students at the highest level did not need to fold the paper aquifers models to solve the assignments.
The open-ended nature of these paper models allows for highly flexible implementation, including customization. The paper models can easily be modified and designed to fit different classroom projects. They can be used as homework or implemented via flipped classrooms. In an online environment, the models were a valuable mechanism for students to connect within breakout rooms. They are small and easy to transport and add a novel and fun element to teaching. These models can even be employed in the field to aid students’ understanding of the hidden groundwater resources if representative sites can be found. Most importantly, they are open to everyone.
Data availability statement
The original contributions presented in the study are included in the article/Supplementary Material, further inquiries can be directed to the corresponding author.
Author contributions
CL developed the paper aquifer models and framed the original manuscript. KD conducted the data collection and analysis of outcomes of the paper aquifer model use in the flipped classroom. All authors implemented the use of the paper aquifer models in the classroom. CK-B designed and implement the use of the paper aquifer models in zoom break out rooms. CL, SA, and CK-B implemented the use of the paper aquifer models in homework assignments. MS and NB implemented the use of the paper aquifer models using in class examples/demonstrations. MC designed and implemented the use of class specific paper aquifer models for use in an integrated class project. All authors participated in the writing and editing of the manuscript.
Acknowledgments
Mark Cuthbert gratefully acknowledges funding from the UK Natural Environment Research Council (NE/P017819/1).
Conflict of interest
The authors declare that the research was conducted in the absence of any commercial or financial relationships that could be construed as a potential conflict of interest.
Publisher’s note
All claims expressed in this article are solely those of the authors and do not necessarily represent those of their affiliated organizations, or those of the publisher, the editors and the reviewers. Any product that may be evaluated in this article, or claim that may be made by its manufacturer, is not guaranteed or endorsed by the publisher.
Supplementary material
The Supplementary Material for this article can be found online at: https://www.frontiersin.org/articles/10.3389/fenvs.2022.876853/full#supplementary-material
References
Andrews, G. D. M., Labishak, G. D., Brown, S. R., Isom, S. L., Pettus, H. D., Byers, T., et al. (2020). Teaching with digital 3D models of minerals and rocks. GSA Today 30 (9), 42–43. doi:10.1130/gsatg464gw.1
Bresciani Ludvik, M. (2021). Equity-drive, high achievement, assessment of student learning and development. Washington, DC: Barker & Taylor Publisher Services, 254. Student Affairs Administrators of Higher Education.
Bursztyn, N., Sajjadi, P., Riegel, H., Huang, J., Wallgrun, J. O., Zhao, J., et al. (2022). Virtual strike and dip - advancing inclusive and accessible field geology. Geosci. Commun. 5, 29–53. doi:10.5194/gc-5-29-2022
Chenrai, P. (2021). Case study on geoscience teaching innovation: Using 3D printing to develop structural interpretation skill in higher education levels. Front. Earth Sci. (Lausanne). 8, 1–6. doi:10.3389/feart.2020.590062
Clark, D., Reynolds, S., Lemanowski, V., Stiles, T., Yasar, S., Proctor, S., et al. (2008). University students’ conceptualization and interpretation of topographic maps. Int. J. Sci. Educ. 30, 377–408. doi:10.1080/09500690701191433
Dickerson, D., Callahan, T. J., Van Sickle, M., and Hay, G. (2005). Students' conceptions of scale regarding groundwater. J. Geosci. Educ. 53 (4), 374–380. doi:10.5408/1089-9995-53.4.374
Dunkle, K. M., and Yantz, J. L. (2021). Intentional design and implementation of a “flipped” upper division geology course: Improving student learning outcomes, persistence, and attitudes. J. Geosci. Educ. 69 (1), 55–70. doi:10.1080/10899995.2020.1787808
Fisher, B. M. C., and Tatomir, A. (2022). A snapshot sample of how COVID-19 impacted and holds up a minor to European water education. Geoscience Communication. doi:10.5194/gc-2022-5A
Garcia-Vela, M., Zambrano, J. L., Falquez, D. A., Pincay-Musso, W., Duque, K. B., Zumba, N. V., et al. (2020). “Management of virtual laboratory experiments in the geosciences field in the time of COVID-19 pandemic,” in Proceeding of ICERI2020 Conference, Online Conference, 9–10 November, 2020, 8702–8711.9
Ishikawa, T., and Kastens, K. A. (2005). Why some students have trouble with maps and other spatial representations. J. Geosci. Educ. 53 (2), 184–197. doi:10.5408/1089-9995-53.2.184
Jaeger, A. J., Shipley, T. F., and Reynolds, S. J. (2017). The roles of working memory and cognitive load in geoscience learning. J. Geosci. Educ. 65 (4), 506–518. doi:10.5408/16-209.1
Kanetaki, Z., Stergiou, C., Bekas, G., Troussas, C., and Sgouropoulou, C. (2021). Analysis of engineering student data in online higher education during the COVID-19 pandemic. Int. J. Eng. Ped. 11 (6), 27–49. doi:10.3991/ijep.v11i6.23259
King, C. (2008). Geoscience education: an overview. Stud. Sci. Educ. 44 (2), 187–222. doi:10.1080/03057260802264289
Kuiper, Y. D. (2008). The dry-erase cube: Making three-dimensional visualization easy. J. Geoscience Educ. 56 (3), 261–268. doi:10.5408/1089-9995-56.3.261
Lepp, L., Aaviku, T., Leijen, A., Pedaste, M., and Saks, K. (2021). Teaching during COVID-19: The decisions made in teaching. Educ. Sci. 11, 47. doi:10.3390/educsci11020047
Phillips, C., Sunderlin, D., and Addy, T. (2021). Adapting to new modes of teaching during COVID-19: Developing instructional approaches that empower learners and facilitate virtual learning experiences. J. Transformative Learn. 8 (1), 10–21.
Keywords: groundwater science, 3D visualization, instructional resources, online learning, origami
Citation: Lowry CS, Dunkle KM, Kairies-Beatty CL, Arslan S, Stahl M, Bogie N and Cuthbert MO (2022) Groundwater origami: Folding paper models to visualize groundwater flow. Front. Environ. Sci. 10:876853. doi: 10.3389/fenvs.2022.876853
Received: 15 February 2022; Accepted: 28 June 2022;
Published: 05 August 2022.
Edited by:
Adam Scott Ward, Indiana University, United StatesReviewed by:
Christos Troussas, University of West Attica, GreeceBridget Mulvey, Kent State University, United States
Copyright © 2022 Lowry, Dunkle, Kairies-Beatty, Arslan, Stahl, Bogie and Cuthbert. This is an open-access article distributed under the terms of the Creative Commons Attribution License (CC BY). The use, distribution or reproduction in other forums is permitted, provided the original author(s) and the copyright owner(s) are credited and that the original publication in this journal is cited, in accordance with accepted academic practice. No use, distribution or reproduction is permitted which does not comply with these terms.
*Correspondence: Christopher S. Lowry, Y3Nsb3dyeUBidWZmYWxvLmVkdQ==