- Department of Life and Environmental Sciences, University of Cagliari, Cagliari, Italy
Diadromous fish, like the European eel Anguilla anguilla (L., 1758), are highly threatened by dams that disrupt river connectivity, consequently impeding fish movements to reach feeding and spawning habitats. In this study, variation in eel occurrence between a historical period (1940–1970) and recent data (2016–2020) was assessed throughout the Sardinian rivers’ network (more than 450 sites). Using Boosted Regression Trees (BRT) we investigated relationships between eel’s occurrence and a set of spatial and temporal environmental variables including a set of dams’ construction features for each period. An overall decrease by 65% of eel’s occurrence was noticed during the ca. 80-years period under scrutiny. Considering a subset (105 and 88 sites for the historical and the recent period, respectively) characterized by the presence of larger dams (height >15 m), eel’s occurrence dropped by 85%. Conversely, eel’s occurrence dropped only by ca. 44% in dam-free sites. During the historical period, eel’s occurrence was mostly affected by time since the initial habitat fragmentation, flow, distance to dams, connectivity, and dams’ height. In the most recent period, eel’s occurrence is mostly affected by dams’ building year, dam-to-sea distance, and, again, dams’ height. Results pinpoint that dams’ construction features and the time from their construction have significant negative effects on eel’s occurrence. Addition of future effective eel restoration practices, apart any other adverse environmental stressor, must consider dams’ removal, wherever socially sustainable or alternatively, the modification of construction features of dams (like excessive height) and the addition of fish ladders.
Introduction
Freshwaters are biodiversity hotspots with 13,000 fish species inhabiting rivers and lakes (Lévêque et al., 2008). Free-flowing rivers provide migration routes for aquatic and riparian species, allow the transportation of sediments and nutrients, enable groundwater recharge, and mitigate flooding (Poff et al., 1997; Tickner et al., 2020). At the same time, rivers are essential elements for biodiversity and humans’ wellbeing (Addams et al., 2009; Russi et al., 2013). Rivers are among the most threatened ecosystems by anthropogenic disturbances (Vörösmarty et al., 2010) including a large variety of obstacles (e.g., dams and weirs, road crossings, hydroelectric power plants, water abstraction for irrigation, flood control systems for municipal water security) (Welcomme, 1995; Jungwirth et al., 2000; Nilsson et al., 2005). Dams’ construction has seen an acceleration worldwide during 1950/1960s (Dynesius and Nilsson, 1994; Postel and Richter, 2003; MacGregor et al., 2009), and more than two thirds of larger rivers have been fragmented (Grill et al., 2015). At present, ca. 2.8 million dams are operating and roughly 3,700 major dams are being constructed or planned (Zarfl et al., 2015; Grill et al., 2019).
Environmental alterations caused by anthropogenic obstacles affect the natural hydrology and connectivity of stream networks, and their ability to transport sediments (Bednarek 2001; Fullerton et al., 2010; Grill et al., 2015; Rincón et al., 2017). Modifying the river flow (Grill et al., 2015), dams pose increasing threats to freshwater ecosystems and mobile biota, particularly fish (Arthington et al., 2016). These barriers play a role in the degradation of water quality (Chowdhury and Kipgen, 2013; Galipeau et al., 2013; Opperman et al., 2019; Barbarossa et al., 2020), cause variations in temperature and water flows (Poff et al., 1997; Richter et al., 2010; Opperman et al., 2017), impair biodiversity, and in particular impede migration, threat the spawning habitats and alter the natural gene flows (Silva et al., 2018; Wilkes et al., 2018; Birnie-Gauvin and Aarestrup, 2019), ultimately increasing the risk of extinction of fish (Lucas and Baras, 2001; Ding et al., 2018). In addition, to adequate habitat for spawning and development, fish require connectivity to migrate freely between different areas of the river and to lakes and to the sea. High connectivity between freshwater and marine habitats facilitates the exchange of matter, energy, and nutrients, with species contributing to the longitudinal transfer and supporting important ecosystem services (Holmlund and Hammer, 1999). For instance, anthropogenic intervention in river discharge result in reduced flushing up to unnaturally extended periods of estuary mouth closure and reduce the connectivity between freshwater habitats and the sea (Potter et al., 2010; Lloyd et al., 2012; Podda et al., 2020). In many estuarine systems, especially those with Mediterranean climate, sand bars are deposited during low flow periods at the river mouth, resulting in truncation of the connection between freshwater and marine habitats (Potter et al., 2010; Suari et al., 2019; Podda et al., 2020). These interruptions and the river flow alteration can have severe implications especially for diadromous species (Gillanders et al., 2003), like the European eel Anguilla anguilla (L., 1758). This diadromous species demonstrates high plasticity in habitat use (Daverat et al., 2006), and is currently exposed to numerous threats along the migratory routes in both marine and freshwater realms (Culurgioni et al., 2014, 2015; Bevacqua et al., 2015; Aalto et al., 2016; Baltazar-Soares et al., 2014; Dekker and Beaulaton, 2016; Drouineau et al., 2018; Podda et al., 2021). Because of these hazards, A. anguilla is progressively declining since the 1970s (ICES, 2021), and is currently classified as Critically Endangered (CR) (IUCN, 2014; Pike et al., 2020) and protected according to the European Council regulation 1100/2007 (EC, 2007).
One of the major threats to the European eel is the presence of large instream barriers restricting access to juveniles leaving the sea after recruitment (Dekker, 2003; Piper et al., 2013; Tamario et al., 2019). The more so as the natural recruitment is the only source of supply of the species (Pedersen and Rasmussen, 2016). Despite their extraordinary climb ability, only a minor proportion of eels can successfully overcome large dams (White and Knights, 1997). Furthermore, direct mortality and sublethal injuries can occur during obstacle passages because of impingements on hard structures, even in the absence of turbines (Bruijs and Durif, 2009). Moreover, increased costs of metabolic energy caused by obstacle passage may have a delayed impact on eel’s migration success and fecundity (Van Ginneken and van den Thillart, 2000). Delays induced by dams can impair escapement, especially when the migration suitability window is limited (Verbiest et al., 2012; Drouineau et al., 2017).
In this study, the impacts of large dams on the occurrence of the European eel A. anguilla in the Sardinian hydrographic district (Central-Western Mediterranean) have been investigated considering how the pre and post dams’ building can affect the long-term presence of eels. We studied relationships among current and historical occurrence of the European eel hypothesizing potential negative effects of a set of temporal, spatial, and dams’ related descriptors using a multivariate approach.
Materials and Methods
Study Area
Sardinia (Italy) is the second largest island in the Mediterranean (area = 24,106 km2). Its hydrographic district coincides with the entire regional territory (Figure 1). The Sardinian hydrographic network is characterized by a reduced number of perennial rivers and the prevalence of intermittent streams (Palmas et al., 2022). Mediterranean climate is typically bi-seasonal with severe drought summer and rainy autumn/winter that determine irregular flow and strong seasonal hydrological fluctuations (Sabatini et al., 2011, 2018; Palmas et al., 2020; Podda et al., 2020). Average annual precipitations range from 500 to 900 mm (De Waele et al., 2010; Moccia et al., 2020). Artificial interruptions of longitudinal river continuity, like dams, strongly influence the annual hydrological cycle of these streams (Naselli-Flores and Lugliè, 2014). An increasing construction of artificial barriers has been observed since the end of the 19th century to provide water for human use (Marchetto et al., 2009; Montaldo and Sarigu, 2017).
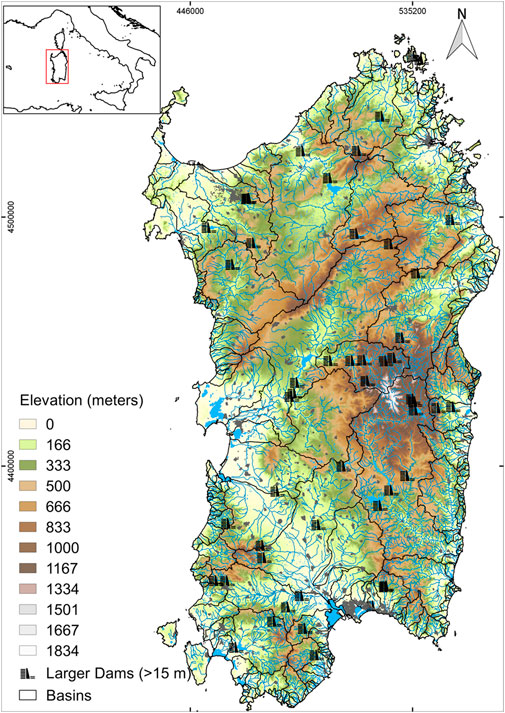
FIGURE 1. Study area, location of rivers and larger dams (height >15 m) in the Sardinian hydrographic district.
Eels’ Data
Two datasets were collected in the frame of the monitoring program for the official Fish Inventory of the Sardinian region (VV.AA., 2022).
The point-to-point historical occurrence data available from 1940 (1940–1970, hereafter historical period), derive from scientific and informative publications of regional origin for a total of 238 sites (Supplementary Table S1), and were georeferenced in a Geographical Information System (GIS) through the Open-Source Software Quantum Gis 2.18.3 (QGIS) (http://www.qgis.org/).
Recent occurrence data (2016–2020; hereafter current period) were collected for 214 sites. Surveys were conducted mainly during the dry season using electrofishing (0-4 Ampere, 0-600 Volt) in habitats ranging from sea level to 1,262 m a.s.l. For comparisons, current data were then integrated into the GIS layer including historical data. Sites have been selected in proportion to the amplitude of the hydrographic basin and in order to have a historical continuity with the data deriving from the previous historical period allowing an appropriate spatial coverage in relation to the extension of the whole hydrographic network of Sardinia (VV.AA., 2022).
Datasets were analyzed separately, and sites influenced by at least one downstream dam were identified. In each dataset, sites located on free-flow rivers or under dams have been analyzed separately from those located above dams.
Data Analysis
Twelve variables, ten continuous and two dummies, have been investigated as potential factors explaining the eel’s occurrence related to dams’ effects. The variables were subdivided into four categories: temporal, spatial, dams’ features, and site-specific, respectively (Table 1). Geospatial information on dams were acquired from the Regional Land Information System of Sardinia (RAS, 2021).
Differences between the percentage of eel’s occurrence during the historical and recent period were evaluated using the Χ2 test (p-value < 0.05). Pairwise collinearity in explanatory variables was examined by scatter plots to exclude redundancy between paired variables disregarding combinations with Spearman’s rho >0.7. Variables were discarded from the pairwise combination based on the Variance Inflation Factor (VIF) discarding observation with VIF > 3 (Zuur et al., 2010).
The set of dams’ descriptors for the two datasets was fitted using Boosted Regression Tree models (BRT) (Friedman 2001; Elith et al., 2008). BRT is a machine-learning method for data exploration and analysis recently introduced into the fields of ecology and conservation biology (Déath, 2007; Elith et al., 2008). BRTs are suited to select the most relevant predictors from a large set of candidate variables, do not depend on the normality and homoscedasticity of the data, integrate nonlinear responses, and reduce the problem of ‘overfitting’ (Elith et al., 2008). BRTs allow to calculate multiple regression models (regression trees) and include an adaptive method to combine many simple models to give improbe predictive performance (boosting). Within the BRT model, terms that are used to optimize predictive performance are represented by the learning rate, tree complexity, and bagging factor (Friedman, 2001; Elith et al., 2008). BRTs were adjusted with a learning rate to return an optimal number of regression trees (1,000–1,500), which has been associated with data overfitting. Trees’ complexity of two refers to the number of nodes in a tree that has been selected (Elith et al., 2008). We use tree complexity to control the number of nodes, to set the maximum number of interactions between predictor variables that are possible, and we used a bagging factor of 0.5 (Friedman, 2001). Variable selection is not necessary for constructing BRTs because they generally ignore non-informative predictors (Elith et al., 2008). The BRT analysis was conducted using the Bernoulli family of occurrence. For visualizing the results, we calculated the partial dependencies that depict the relationships between the response and each predictor variable while controlling for the average effects of the remaining predictors (Friedman, 2001; Friedman and Meulman, 2003).
Our approach did not allow to assess confidence intervals for BRTs, used when a large number of BRT sub-models are fitted. The partial dependence plots for parameters with a contribution >10% were used to visualize the effect of each variable on eel’s occurrence. As this method does not deliver p-values, but uses internal validation processes, BTRs performance were evaluated using the amount of total deviance explained (%) and cross-validated correlation between model prediction and observed data (CV correlation) (Derville et al., 2016; Nieto and Mélin, 2017; Ju et al., 2021; Saha et al., 2021). Statistical analyses were conducted using the open-source R software (R Core Team, 2021). Specifically, gbm (Greenwell et al., 2020), and dismo (Hijmans et al., 2011) packages for BRT analyses, and partial dependence plots with smoothing for each variable.
Results
Dams in the Sardinian Hydrographic District
Although the first dam in Sardinia date back to the end of the 19th century, a rapid increase in dams’ construction has been observed from the late 1960s to the late 1990s (Figure 2A). More than 50% of the 54 larger dams (>15 m height, mean height ± Standard Deviation—SD, 42.5 ± 22.3 m) were built between 1940 and 1960 (Figure 2B), with two of the most recent dams not completely built yet. Furthermore, Sardinia is currently lacking river flow regulations, and fishways or fish ladders are mainly absent or not working with one single exception (i.e., Casteldoria dam, height = 26.6 m, 40°53.3843 N–08°53.7534 E, recently built, 2015).
Eel’s Occurrence in Sardinian Rivers
Comparing sites correspondence (one observation per site for each dataset) between the historical and the current datasets, a total of 54 sites matched (23% and 25% of number of sites, respectively). The analysis of the historical period (1940–1970) revealed that eel’s occurrence amounted of ca. 95% (Figure 3A). In the current period (2016–2020) we observed a strong contraction (ca. 65%, Χ2, p < 0.001) of eel’s occurrence, which decreased to only 30% (Figure 3B).
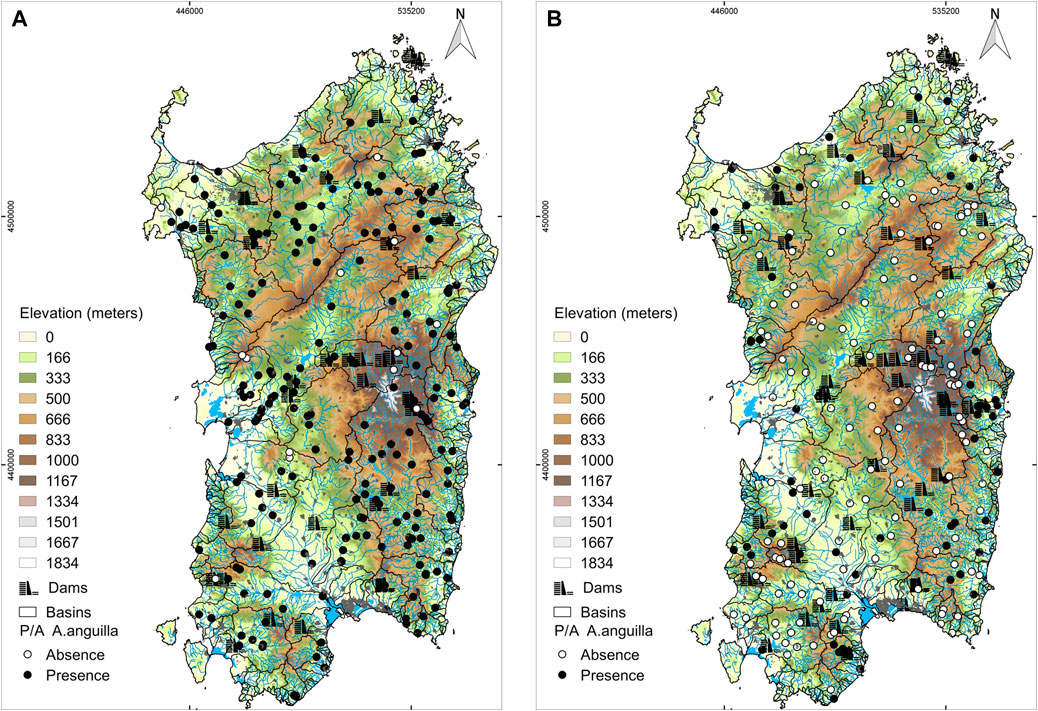
FIGURE 3. (A) Historical European eel’s occurrence (1940–1970). (B) Current European eel’s occurrence (2016–2020)
Considering only sites with downstream dams (88 and 105 sites for the historical and current period, respectively), eel’s occurrence dropped from 92% to 7% respectively, with a total (statistically significant) decrease of ca. 85% (Χ2, p < 0.001). In this subset, the proportion of sites influenced by downstream dams was ca. 37% and 49% for the two periods, respectively.
During the historical period, 96% of free flow sites (i.e., sites without dams or sites without downstream dams) had eels, whereas in the current period this value dropped to 52%, with a decreasing rate between the two periods of ca. 44% (Χ2, p < 0.001).
Effect of Larger Dams on Eel’s Occurrence
After analyzing dams’ descriptors through Spearman correlation (Supplementary Figures S1, S2) and using VIF score >3 as a threshold (Supplementary Tables S2, S3), only eight descriptors were included for the modeling of the data from the historical period, and seven for the current one. Five descriptors were common between the datasets: dendritic connectivity index (DCI), height (HEIGHT) and flow (FLOW) of dams, river mouth condition (MOUTH), and fishing pressure (FISH). The predictors in the modeling of the historical period also included the temporal fragmentation (FRAGMENTATION), the distance of dams from each site (D_STA) and the dams’ elevation (ELEV), while the year of dams’ building (YEAR) and the distance of dams from the sea (D_SEA) were included in the modeling of the current period.
The historical BRT model shows that five descriptors cumulatively explain 88.4% of the model: FRAGMENTATION (relative contribution = 22.3%), FLOW (22.2%), D_STA (14.7%), DCI (14.6%), HEIGHT (14.6%). Explained deviance (51%) and CV correlation (0.40) suggest that the relationships between dams’ characteristics and the eel’s occurrence are quite robust. The partial dependence plots obtained after BRT models (Figure 4) show that the contribution of FRAGMENTATION to eel’s occurrence is greatest 20 years after dams’ building. The difference across that threshold is very narrow, with a value of 91% and 94% occurrence, below and above downstream dams, respectively. The contribution of FLOW to eel’s occurrence is maximum over a threshold of ca. 1,300 m3s−1, with a value of 87% below and 100% above the threshold. The highest effect of D_STA is observed more than 5 km far from dams, where mean eel’s occurrence is 75%, whereas at closer sites this value is 96%. The HEIGHT effect was highest above ca. 60 m, where eel’s occurrence (100%) was higher than that (90%) in sites with downstream dams <60 m height. The index of river connectivity (DCI) has the highest effect on eel’s occurrence within the interval of 60%–80% DCI with mean eel’s occurrence of 86% below 60% of DCI, and 100% above this threshold.
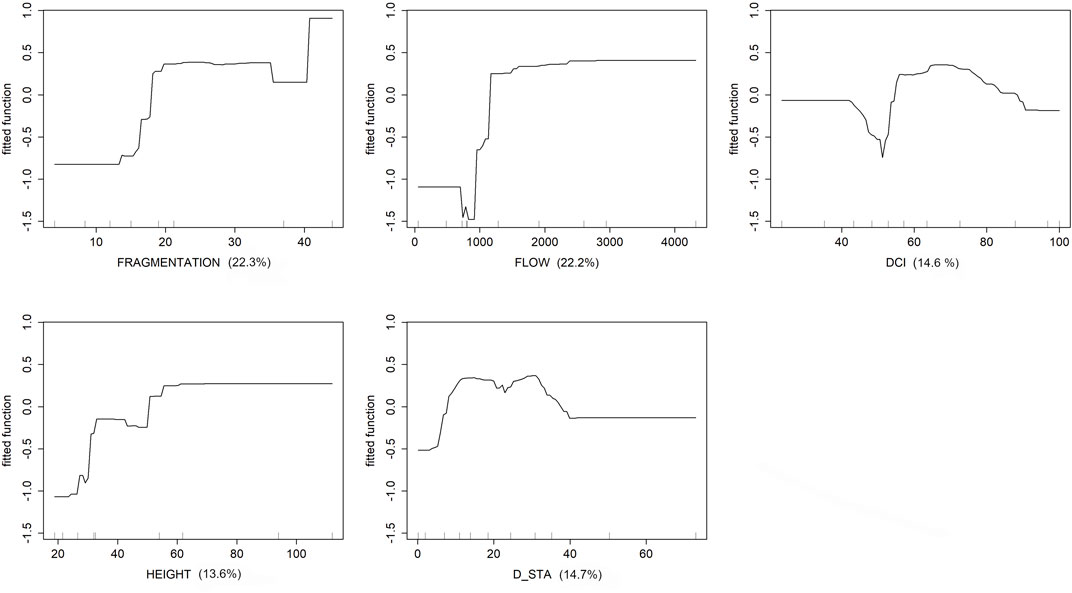
FIGURE 4. Partial dependence plots of the five variables (FRAGMENTATION, FLOW, D_STA, DCI, and HEIGHT) affecting eels ‘occurrence in Sardinian rivers during the historical period (1940–70). The relative contribution of each variable is reported within parenthesis. Black lines refer to the raw model output.
The BRT model for the most recent period reveals that three descriptors, YEAR (54.6% of relative contribution), D_SEA (21.3%), and HEIGHT (13.4%), explain cumulatively 91% of the model. There is well goodness of fit of the BRT with an explained deviance of 62% and a CV correlation of 0.67. The partial dependence plots obtained after BRT models (Figure 5) show that the effect of dams’ age is highest (25%) after 1980 (i.e., dams with less than ca. 40 years) and drops to 2% in more recent years. The contribution of D_SEA to eel’s occurrence is highest below 20 km, with the highest mean occurrence (13%) in sites close to the sea and the lowest (2%) in the farthest ones. The highest contribution of HEIGHT is observed for dams between 30 and 80 m, which have a mean eel’s occurrence of 5%. In sites with dams <30 m occurrence is 10%, whereas in sites with dams >80 m (some of which are still not completed) the mean eel’s occurrence is 7%.
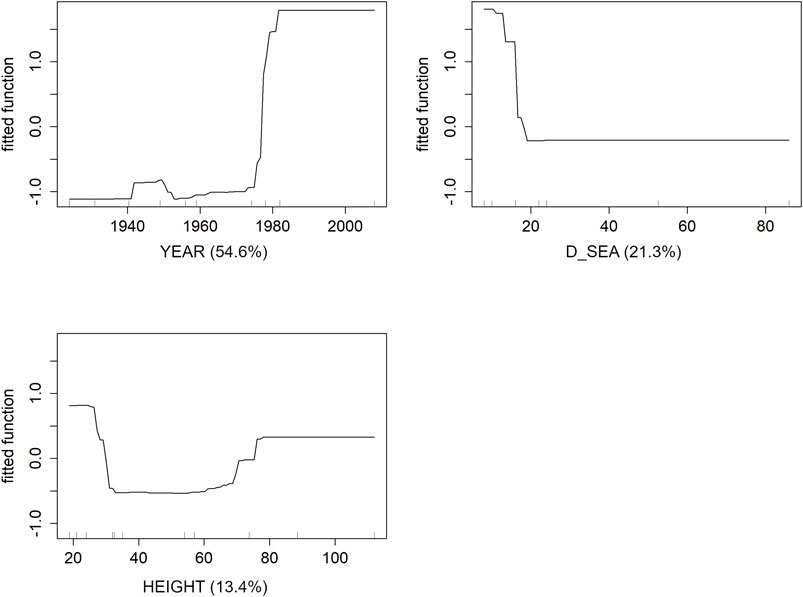
FIGURE 5. Partial dependence plots of the three variables (YEAR, D_SEA, and HEIGHT) affecting eels ‘occurrence in Sardinian rivers during the recent period (2016–20). The relative contribution of each variable is reported within parenthesis. Black lines refer to the raw model output.
Discussion
Impacts of Large Dams on Eel’s Occurrence
A. anguilla is worldwide ranked as critically endangered species due to an ample array of often synergistic, anthropogenic threats (Jacoby et al., 2015; Miller et al., 2016; Drouineau et al., 2018). Moreover, the presence of dams, causing a physical interruption of river connectivity, river flow reduction or modification, and loss and deterioration of habitats, may also critically affect eel’s survival during their freshwater life stage (Feunteun, 2002; Tamario et al., 2019; Watz et al., 2019).
The dramatic decline of the European eel’s recruitment reported worldwide (Dekker, 2003, 2004; ICES, 2021) also affects the Sardinian rivers’ network, where we observed a huge decrease in eel’s occurrence. Indeed, considering only free-flow sites (i.e., sites in dam-free rivers or sites without downstream dams), the European eel occupied most of the main Sardinian rivers until the 1970s (ca. 95%). The overall 44% reduction in species’ occurrence between the two periods in these sites suggests that a relevant proportion of such a decline is independent by dams. Nevertheless, the decline of eel’s occurrence in Sardinian rivers is also severely affected by damming with eel’s occurrence in sites above dams dropping by 85% between the two periods. Our results, ultimately, confirm that dams have a severe impact on eels due to the fragmentation of migration habitats and the alteration of river flow (Poff and Schmidt, 2016; Dias et al., 2017).
We hypothesized that dams’ height (Larinier, 2001; Merg et al., 2020), dams’ age (Atkinson et al., 2020) and the decreased river flow due to the oldest dams (Legault et al., 2003) could negatively affect eel’s migration.
In both periods under scrutiny, as expected, the height of dams could have had a negative effect on eel’s occurrence and such an impact was lower during the old period but severely exacerbated in recent years. In the most recent period, the eel’s occurrence above dams >80 m high is, counterintuitively, higher than that in lower dams. This apparent discrepancy can be ascribed to the fact that especially some of the very tall dams (>80 m) were built only very recently, thus the presence of eels above the dam stems from times before the dam was finalized or filled. For catadromous fish, including eels, climbing out of the water to surpass a barrier is challenging and linked to high metabolic costs (Edeline et al., 2004; Briand et al., 2005; Bult and Dekker 2007).
The age of the dams (estimated using either year of construction–in the most recent period—or temporal fragmentation—in the old one) negatively influence eel’s occurrence. We contend that the oldest dams installed in Sardinia, possibly due to technical and financial limitations, have been built in rivers of lower order or in the upper part of the rivers, thus having a minor negative effect on the overall river connectivity (Segurado et al., 2013). In the most recent period, the strongest impact of fragmentation by dams could be attributable to the technological progress and modernization that allowed building dams with more effective water retention and, thus, the stronger capacity of interrupting the river flow in more downstream river sections (Haidvogl, 2018; Merg et al., 2020). Nevertheless, the effect of river flow is a major factor influencing eel’s occurrence only in the old period. The minimum or nihil effect of river flow and the DCI index on eel’s occurrence in the most recent period could be attributable to the very low river flow in the last 30 years, caused by prolonged drought, which, most probably impaired eels’ ability to migrate upstream. The negative effect of dams appears more evident during the old period, when either river flow or the DCI index explains significant proportions of eel’s occurrence variations. The major role of river flow and the DCI index, in the old period only, and their ininfluence in the most recent one, is corroborated by the intensity of water flow involved in the route choice (Legault et al., 2003), causing the silencing of environmental stimuli when it is too low (Trancart et al., 2018, 2020) and, in the most extreme cases, interrupting downstream (Durif, 2003) and upstream migration (Podda et al., 2020).
In both periods, the distance from the sea explains significant proportions of eel’s occurrence. The more distant from the sea the more probable is the presence of natural (and artificial) obstacles to be crossed by fish (Merg et al., 2020), which, indeed, becomes a severe impediment to migration, especially when river connectivity is limited due to low river flow.
Giving Future to the European Eel
Our study reveals that different dams’ attributes can explain the current decline of A. anguilla. Along with damming, other factors, associated with excessive human pressure but also with climate change, are severely impairing eel’s occurrence. To foster the conservation of the European eel, many authors have pushed to mitigate its commercial exploitation (Nielsen and Prouzet, 2008; Henderson et al., 2011; Aalto et al., 2016; ICES, 2021). In addition, we contend that a strong effort should be paid to eliminate highly impacting artificial barriers or, at least, to mitigate their impacts with more sustainable actions, tools, and devices. While creating unobstructed migration routes between the spawning area in the sea and freshwater growth habitats could be preferable (Feunteun, 2002; Drouineau et al., 2018; Rodeles et al., 2021), the removal of artificial barriers could be, locally, socially, and economically impracticable. Some attempts have been made and succeeded with other catadromous endangered species (Graf, 2003; Stanley and Doyle, 2003; Harris et al., 2016), including the American eel Anguilla rostrata (Lesueur, 1817) (Hitt et al., 2012; Turner et al., 2018). The removal of artificial barriers could be not exempt from collateral negative effects, including, for instance, the facilitation of biological invasions (Rahel, 2013; Milt et al., 2018), the spill of toxic sediments, and sudden changes in hydromorphology (Stanley and Doyle, 2003; Gangloff, 2013).
Except these general suggestions, in our context it could be crucial to control and reducing the uptake of water for human use above dams and, at the same time, ensuring a Minimum Vital Flow (MVF; Moccia et al., 2020) that guarantees the morphological, hydrological, physical-chemical, and biological integrity downstream will enable the protection of eel’s habitats in the short and long term (Dudgeon et al., 2006). A potentially compensative solution for partial restoration of dammed river connectivity, without eliminating dams, could also profit of the construction of artificial fishways to allow eels’ passage beyond dams (Seliger and Zeiringer, 2018), management measures both still lacking in Sardinia. Moreover, it would be advisable that any management, operation, and maintenance of large dams in Sardinia would be implemented within a regional control system aimed at harmonizing and standardizing information to support a correct use and management of these structures. Although this study was conducted on a purely regional scale, our results highlighted the major impact of dams on the European eel’s occurrence in Sardinian rivers and its apparent worsening in the last 10 years. We conclude that, based on the general current crisis of this species, urgent actions are needed worldwide to restore their habitats by reducing humans’ pressure, ensuring a minimum vital flow, abating, wherever possible, artificial barriers to river connectivity, or, at least, implementing natural migration routes with artificial fishways.
Data Availability Statement
The raw data supporting the conclusions of this article will be made available by the authors, without undue reservation.
Author Contributions
CP led conceptualization, field sampling, data curation, software realization, statistical analysis, formal writing, and original draft. FP conducted field sampling, software realization, writing, review, and editing. AP contributed for the supervision, writing, review, and editing. AS led the project administration, supervision, writing, review, and editing.
Funding
This study has been carried out in the context of the agreements: “Rep. RAS n. 27002–1 A.D.A. of 18.12.2015 and Rep. RAS n. 7304–12 A.D.A. of 29.03.2018,” Sardinian Regional Government. CP gratefully acknowledges Sardinian Regional Government for the financial support of her PhD scholarship (P.O.R. Sardegna F.S.E.—Operational Program of the Autonomous Region of Sardinia, European Social Fund 2014–2020—Axis III Education and training, Thematic goal 10, Investment Priority 10ii), Specific goal 10.5.
Conflict of Interest
The authors declare that the research was conducted in the absence of any commercial or financial relationships that could be construed as a potential conflict of interest.
Publisher’s Note
All claims expressed in this article are solely those of the authors and do not necessarily represent those of their affiliated organizations, or those of the publisher, the editors and the reviewers. Any product that may be evaluated in this article, or claim that may be made by its manufacturer, is not guaranteed or endorsed by the publisher.
Supplementary Material
The Supplementary Material for this article can be found online at: https://www.frontiersin.org/articles/10.3389/fenvs.2022.876369/full#supplementary-material
References
Aalto, E., Capoccioni, F., Terradez Mas, J., Schiavina, M., Leone, C., De Leo, G., et al. (2016). Quantifying 60 Years of Declining European Eel (Anguilla anguilla L., 1758) Fishery Yields in Mediterranean Coastal Lagoons. ICES J. Mar. Sci. 73, 101–110. doi:10.1093/icesjms/fsv084
Addams, L., Boccaletti, G., Kerlin, M., and Stuchtey, M. (2009). Charting OurWater Future. Economic Frameworks to Inform Decision-Making. https://www.mckinsey.com/∼/media/mckinsey/dotcom/client_service/sustainability/pdfs/charting%20our%20water%20future/charting_our_water_future_full_report_.ashx.
Arthington, A. H., Dulvy, N. K., Gladstone, W., and Winfield, I. J. (2016). Fish Conservation in Freshwater and Marine Realms: Status, Threats and Management. Aquat. Conserv. Mar. Freshw. Ecosyst. 26, 838–857. doi:10.1002/aqc.2712
Atkinson, S., Bruen, M., O' Sullivan, J. J., Turner, J. N., Ball, B., Carlsson, J., et al. (2020). An Inspection-Based Assessment of Obstacles to Salmon, Trout, Eel and Lamprey Migration and River Channel Connectivity in Ireland. Sci. Total Environ. 719, 137215. doi:10.1016/j.scitotenv.2020.137215
Baltazar-Soares, M., Biastoch, A., Harrod, C., Hanel, R., Marohn, L., Prigge, E., et al. (2014). Recruitment Collapse and Population Structure of the European Eel Shaped by Local Ocean Current Dynamics. Curr. Biol. 24 (1), 104–108. doi:10.1016/j.cub.2013.11.031
Barbarossa, V., Schmitt, R. J. P., Huijbregts, M. A. J., Zarfl, C., King, H., and Schipper, A. M. (2020). Impacts of Current and Future Large Dams on the Geographic Range Connectivity of Freshwater Fish Worldwide. Proc. Natl. Acad. Sci. U.S.A. 117, 3648–3655. doi:10.1073/pnas.1912776117
Bednarek, A. T. (2001). Undamming Rivers: a Review of the Ecological Impacts of Dam Removal. Environ. Manag. 27, 803–814. doi:10.1007/s002670010189
Bevacqua, D., Melià, P., Gatto, M., and De Leo, G. A. (2015). A Global Viability Assessment of the European Eel. Glob. Change Biol. 21, 3323–3335. doi:10.1111/gcb.12972
Birnie‐Gauvin, K., and Aarestrup, K. (2019). A Call for a Paradigm Shift: Assumed‐to‐be Premature Migrants Actually Yield Good Returns. Ecol. Freshw. Fish. 28, 62–68. doi:10.1111/eff.12431
Briand, C., Fatin, D., Feunteun, E., and Fontenelle, G. (2005). Estimating the Stock of Glass Eels in an Estuary by Mark-Recapture Experiments Using Vital Dyes. Bull. Fr. Pêche Piscic. 378, 23–46. doi:10.1051/kmae:2005002
Bruijs, M. C. M., and Durif, C. M. F. (2009). “Silver Eel Migration and Behaviour,” in Spawning Migration of the European Eel. Editors G. Thillart, S. Dufour, and J. C. Rankin (Dordrecht: Fish & Fisheries SeriesSpringer), 30, 65–95. doi:10.1007/978-1-4020-9095-0_4
Bult, T. P., and Dekker, W. (2007). Experimental Field Study on the Migratory Behaviour of Glass Eels (Anguilla anguilla) at the Interface of Fresh and Salt Water. ICES J. Mar. Sci. 64 (7), 1396–1401. doi:10.1093/icesjms/fsm105
Chowdhury, A. R., and Kipgen, N. (2013). Deluge amidst Conflict: Hydropower Development and Displacement in the North-east Region of India. Prog. Dev. Stud. 13, 195–208. doi:10.1177/1464993413486545
Cote, D., Kehler, D. G., Bourne, C., and Wiersma, Y. F. (2009). A New Measure of Longitudinal Connectivity for Stream Networks. Landsc. Ecol. 24 (1), 101–113. doi:10.1007/s10980-008-9283-y
Cottiglia, M. (1968). La Distribuzione Dell’ittiofauna Dulciacquicola in Sardegna. Riv. Idrob. 7 (1), 64–115.
Culurgioni, J., Figus, V., Cabiddu, S., De Murtas, R., Cau, A., and Sabatini, A. (2015). Larval Helminth Parasites of Fishes and Shellfishes from Santa Gilla Lagoon (Sardinia, Western Mediterranean), and Their Use as Bioecological Indicators. Estuaries Coasts 38, 1505–1519. doi:10.1007/s12237-014-9894-2
Culurgioni, J., Sabatini, A., De Murtas, R., Mattiucci, S., and Figus, V. (2014). Helminth Parasites of Fish and Shellfish from the Santa Gilla Lagoon in Southern Sardinia, Italy. J. Helminthol. 88, 489–498. doi:10.1017/S0022149X13000461
Déath, G. (2007)., 88. 0.CO, 2432–251. doi:10.1890/0012-9658(2007)88[243Boosted Trees for Ecological Modeling and PredictionEcologyBTFEMA]2
Daverat, F., Limburg, K., Thibault, I., Shiao, J., Dodson, J., Caron, F., et al. (2006). Phenotypic Plasticity of Habitat Use by Three Temperate Eel Species, Anguilla anguilla, A. Japonica and A. rostrata. Mar. Ecol. Prog. Ser. 308, 231–241. doi:10.3354/meps308231
De Waele, J., Martina, M. L. V., Sanna, L., Cabras, S., and Cossu, Q. A. (2010). Flash Flood Hydrology in Karstic Terrain: Flumineddu Canyon, Central-East Sardinia. Geomorphology 120, 162–173. doi:10.1016/j.geomorph.2010.03.021
Dekker, W., and Beaulaton, L. (2016). Climbing Back up what Slippery Slope? Dynamics of the European Eel Stock and its Management in Historical Perspective. ICES J. Mar. Sci. 73, 5–13. doi:10.1093/icesjms/fsv132
Dekker, W. (2003). Did Lack of Spawners Cause the Collapse of the European Eel, Anguilla anguilla ? Fish. Manag. Ecol. 10, 365–376. doi:10.1111/j.1365-2400.2003.00352.x
Dekker, W. (2004). Slipping through Our Hands – Population Dynamics of the European Eel. PhD Thesis. Amsterdam, Netherlands: University of Amsterdam. Available at: http://www.diadfish.org/doc/these_2004/dekker_thesis_eel.pdf.
Derville, S., Constantine, R., Baker, C., Oremus, M., and Torres, L. (2016). Environmental Correlates of Nearshore Habitat Distribution by the Critically Endangered Maūi Dolphin. Mar. Ecol. Prog. Ser. 551, 261–275. doi:10.3354/meps11736
Dias, M. S., Tedesco, P. A., Hugueny, B., Jézéquel, C., Beauchard, O., Brosse, S., et al. (2017). Anthropogenic Stressors and Riverine Fish Extinctions. Ecol. Indic. 79, 37–46. doi:10.1016/j.ecolind.2017.03.053
Ding, L., Chen, L., Ding, C., and Tao, J. (2018). Global Trends in Dam Removal and Related Research: A Systematic Review Based on Associated Datasets and Bibliometric Analysis. Chin. Geogr. Sci. 29, 1–12. doi:10.1007/s11769-018-1009-8
Drouineau, H., Bau, F., Alric, A., Deligne, N., Gomes, P., and Sagnes, P. (2017). Silver Eel Downstream Migration in Fragmented Rivers: Use of a Bayesian Model to Track Movements Triggering and Duration★. Aquat. Living Resour. 30, 5–9. doi:10.1051/alr/2017003
Drouineau, H., Carter, C., Rambonilaza, M., Beaufaron, G., Bouleau, G., Gassiat, A., et al. (2018). River Continuity Restoration and Diadromous Fishes: Much More Than an Ecological Issue. Environ. Manag. 61, 671–686. doi:10.1007/s00267-017-0992-3
Dudgeon, D., Arthington, A. H., Gessner, M. O., Kawabata, Z.-I., Knowler, D. J., Lévêque, C., et al. (2006). Freshwater Biodiversity: Importance, Threats, Status and Conservation Challenges. Biol. Rev. 81, 163–182. doi:10.1017/S1464793105006950
Durif, C. M. F. (2003). La migration d’avalaison de l’anguille européenne Anguilla anguilla: Caractérisation des fractions dévalantes, phénoméne de migration et franchissement d’obstacles. PhD Thesis. Toulouse: University of Paul Sabatier.
Dynesius, M., and Nilsson, C. (1994). Fragmentation and Flow Regulation of River Systems in the Northern Third of the World. Science 266 (5186), 753–762. doi:10.1126/science.266.5186.753
E.C. (2007). Council Regulation (EC) No. 1100/2007 of 18 September 2007, Establishing Measures for the Recovery of the Stock of European Eel. Official J. Eur. Union L 248, 17–23.
Edeline, E., Dufour, S., Briand, C., Fatin, D., and Elie, P. (2004). Thyroid Status Is Related to Migratory Behavior in Anguilla anguilla Glass Eels. Mar. Ecol. Prog. Ser. 282, 261–270. doi:10.3354/meps282261
Elith, J., Leathwick, J. R., and Hastie, T. (2008). A Working Guide to Boosted Regression Trees. J. Anim. Ecol. 77, 802–813. doi:10.1111/j.1365-2656.2008.01390.x
Feunteun, E. (2002). Management and Restoration of European Eel Population (Anguilla anguilla): An Impossible Bargain. Ecol. Eng. 18, 575–591. doi:10.1016/S0925-8574(02)00021-6
Friedman, J. H. (2001). Greedy Function Approximation: A Gradient Boosting Machine. Ann. Stat. 29 (5), 1189–1232. doi:10.1214/aos/1013203451
Friedman, J. H., and Meulman, J. J. (2003). Multiple Additive Regression Trees with Application in Epidemiology. Stat. Med. 22, 1365–1381. doi:10.1002/sim.1501
Fullerton, A. H., Burnett, K. M., Steel, E. A., Flitcroft, R. L., Pess, G. R., Feist, B. E., et al. (2010). Hydrological Connectivity for Riverine Fish: Measurement Challenges and Research Opportunities. Freshw. Biol. 55, 2215–2237. doi:10.1111/j.1365-2427.2010.02448.x
Galipeau, B. A., Ingman, M., and Tilt, B. (2013). Dam-Induced Displacement and Agricultural Livelihoods in China's Mekong Basin. Hum. Ecol. 41, 437–446. doi:10.1007/s10745-013-9575-y
Gangloff, M. M. (2013). Taxonomic and Ecological Tradeoffs Associated with Small Dam Removals. Aquat. Conserv. Mar. Freshw. Ecosyst. 23, 475–480. doi:10.1002/aqc.2383
Graf, W. L. (2003). “Dam Removal Research – Status and Prospects,” in Proceedings of the Heinz Center’s Dam Removal Research Workshop (Pennsylvania Avenue, Washington D.C: The H. John Heinz III Center for Science, Economics and the Environment), 23–24.
Greenwell, B., Boehmke, B., and Cunningham, J. (2020). Gbm: Generalized Boosted Regression Models. R Package Version 2.1.5. Available online: https://CRAN.R-project.org/package=gbm.
Grill, G., Lehner, B., Lehner, B., Lumsdon, A. E., Zarfl, C., MacDonald, G. K., et al. (2015). An Index-Based Framework for Assessing Patterns and Trends in River Fragmentation and Flow Regulation by Global Dams at Multiple Scales. Environ. Res. Lett. 10 (1), 015001. doi:10.1088/1748-9326/10/1/015001
Grill, G., Lehner, B., Thieme, M., Geenen, B., Tickner, D., Antonelli, F., et al. (2019). Mapping the World's Free-Flowing Rivers. Nature 569, 215–221. doi:10.1038/s41586-019-1111-9
Haidvogl, G. (2018). “Historic Milestones of Human River Uses and Ecological Impacts,” in Riverine Ecosystem Management. Editors S. Schmutz, and J. Sendzimir (Cham: Springer), 8, 19–39. Aquatic Ecology Series. doi:10.1007/978-3-319-73250-3_2
Harris, J. H., Kingsford, R. T., Peirson, W., and Baumgartner, L. J. (2017). Mitigating the Effects of Barriers to Freshwater Fish Migrations: The Australian Experience. Mar. Freshw. Res. 68, 614–696. doi:10.1071/MF15284
Henderson, P. A., Plenty, S. J., Newton, L. C., and Bird, D. J. (2011). Evidence for a Population Collapse of European Eel (Anguilla anguilla) in the Bristol Channel. J. Mar. Biol. Ass. 92 (4), 843–851. doi:10.1017/s002531541100124x
Hijmans, R. J., Phillips, S., Leathwick, J., and Elith, J. (2011). Dismo: Species Distribution Modeling. R Package Version 1.1-4. Available online: https://CRAN.R-project.org/package=dismo.
Hitt, N. P., Eyler, S., and Wofford, J. E. B. (2012). Dam Removal Increases American Eel Abundance in Distant Headwater Streams. Trans. Am. Fish. Soc. 141, 1171–1179. doi:10.1080/00028487.2012.675918
Holmlund, C. M., and Hammer, M. (1999). Ecosystem Services Generated by Fish Populations. Ecol. Econ. 29 (2), 253–268. doi:10.1016/S0921-8009(99)00015-4
ICES (2021). Joint EIFAAC/ICES/GFCM Working Group on Eels (WGEEL). ICES Sci. Rep. 3 (85). doi:10.17895/ices.pub.8143
IUCN (2014). The IUCN Red List of Threatened Species. Anguilla anguilla. Available at: http://www.iucnredlist.org.
Jacoby, D. M. P., Casselman, J. M., Crook, V., DeLucia, M.-B., Ahn, H., Kaifu, K., et al. (2015). Synergistic Patterns of Threat and the Challenges Facing Global Anguillid Eel Conservation. Glob. Ecol. Conservation 4, 321–333. doi:10.1016/j.gecco.2015.07.009
Ju, M.-C., Zhang, T.-L., Li, X.-K., Li, B.-Y., Li, Y.-P., Liu, Q.-Y., et al. (2021). Large Scale Environmental Drivers of Biocrust Distribution and Development across a Sandy Desert in China. Catena 200, 105137. doi:10.1016/j.catena.2020.105137
Jungwirth, M., Muhar, S., and Schmutz, S. (2000). Fundamentals of Fish Ecological Integrity and Their Relation to the Extended Serial Discontinuity Concept. Hydrobiologia 422, 85–97. doi:10.1023/A:101704552723310.1007/978-94-011-4164-2_7
Lévêque, C., Oberdorff, T., Paugy, D., Stiassny, M. L. J., and Tedesco, P. A. (2008). Global Diversity of Fish (Pisces) in Freshwater. Hydrobiologia 595, 545–567. doi:10.1007/s10750-007-9034-0
Larinier, M. (2001). Dams, Fish and Fisheries: Opportunities, Challenges and Conflict Resolution. FAO fisheries technical paper.Environmental Issues, Dams and Fish Migration
Legault, A., Acou, A., Guillouët, J., and Feunteun, E. (2003). Suivi De La Migration D'avalaison Des Anguilles Par Une Conduite De Debit Reserve. Bull. Fr. Pêche Piscic. 368, 43–54. doi:10.1051/kmae:2003035
Lloyd, L. N., Anderson, B. G., Cooling, M., Gippel, C. J., Pope, A. J., and Sherwood, J. E. (2012). Estuary Environmental Flows Assessment Methodology for Victoria. Melbourne, Victoria, Australia: Victorian Government Department of Sustainability and Environment.
Lucas, M. C., and Baras, E. (2001). Migration of Freshwater Fishes. Oxford GBR: Blackwell Science Ltd.
Mac Gregor, R., Casselman, J., Allen, W., Haxton, T., Dettmers, J., Mathers, A., et al. (2009). Natural Heritage, Anthropogenic Impacts, and Biopolitical Issues Related to the Status and Sustainable Management of American Eel: a Retrospective Analysis and Management Perspective at the Population Level. Am. Fish. Soc. Symp. 69, 713–740. doi:10.1158/0008-5472.sabcs-09-2053
Manca, G. (1965). “Contributo Alla Conoscenza Della Salmo trutta Macrostigma Dum,” in Sardegna (University of Cagliari), 92.
Marchetto, A., Padedda, B. M., Mariani, M. A., Lugliè, A., and Sechi, N. (2009). A Numerical Index for Evaluating Phytoplankton Response to Changes in Nutrient Levels in Deep Mediterranean Reservoirs. J. Limnol. 68 (1), 106–121. doi:10.4081/jlimnol.2009.106
Merg, M.-L., Dézerald, O., Kreutzenberger, K., Demski, S., Reyjol, Y., Usseglio-Polatera, P., et al. (2020). Modeling Diadromous Fish Loss from Historical Data: Identification of Anthropogenic Drivers and Testing of Mitigation Scenarios. Plos One 15 (7), e0236575. doi:10.1371/journal.pone.0236575
Miller, M. J., Feunteun, E., and Tsukamoto, K. (2016). Did a “Perfect Storm” of Oceanic Changes and Continental Anthropogenic Impacts Cause Northern Hemisphere Anguillid Recruitment Reductions? ICES J. Mar. Sci. 73, 43–56. doi:10.1093/icesjms/fsv063
Milt, A. W., Diebel, M. W., Doran, P. J., Ferris, M. C., Herbert, M., Khoury, M. L., et al. (2018). Minimizing Opportunity Costs to Aquatic Connectivity Restoration while Controlling an Invasive Species. Conserv. Biol. 32, 894–904. doi:10.1111/cobi.13105
Moccia, D., Salvadori, L., Ferrari, S., Carucci, A., and Pusceddu, A. (2020). Implementation of the EU Ecological Flow Policy in Italy with a Focus on Sardinia. Adv. Ocean. Limnol. 11 (1). doi:10.4081/aiol.2020.8781
Montaldo, N., and Sarigu, A. (2017). Potential Links between the North Atlantic Oscillation and Decreasing Precipitation and Runoff on a Mediterranean Area. J. Hydrology 553, 419–437. doi:10.1016/j.jhydrol.2017.08.018
Naselli-Flores, L., and Lugliè, A. (2014). Laghi artificiali dell’Italia meridionale e delle isole maggiori. Biol. Ambient. 28, 1–8.
Nielsen, T., and Prouzet, P. (2008). “Capture-based Aquaculture of the Wild European Eel (Anguilla anguilla),” in Capture-based Aquaculture. Global Overview. Editors A. Lovatelli, and P. F. Holthus (Rome: FAO Fisheries Technical Paper).
Nieto, K., and Mélin, F. (2017). Variability of Chlorophyll-A Concentration in the Gulf of Guinea and its Relation to Physical Oceanographic Variables. Prog. Oceanogr. 151, 97–115. doi:10.1016/j.pocean.2016.11.009
Nilsson, C., Reidy, C. A., Dynesius, M., and Revenga, C. (2005). Fragmentation and Flow Regulation of the World's Large River Systems. Science 308 (5720), 405–408. doi:10.1126/science.1107887
Opperman, J., Baruch-Mordo, S., Carvallo, J., Kammen, D., Kiesecker, J., and Weber, C. (2019). Sustaining the Last Rivers. Amer. Sci. 107, 302. doi:10.1511/2019.107.5.302
Opperman, J. J., Moyle, P. B., Larsen, E. W., Florsheim, J. L., and Manfree, A. D. (2017). Floodplains: Processes and Management for Ecosystem Services. Berkeley, CA, USA: University of California Press.
Palmas, F., Cau, A., Podda, C., Musu, A., Serra, M., Pusceddu, A., et al. (2022). Rivers of Waste: Anthropogenic Litter in Intermittent Sardinian Rivers, Italy (Central Mediterranean). Environ. Pollut. 302, 119073. doi:10.1016/j.envpol.2022.119073
Palmas, F., Righi, T., Musu, A., Frongia, C., Podda, C., Serra, M., et al. (2020). Pug-Headedness Anomaly in a Wild and Isolated Population of Native Mediterranean Trout Salmo trutta L., 1758 Complex (Osteichthyes: Salmonidae). Diversity 12 (9), 353. doi:10.3390/d12090353
Pedersen, M. I., and Rasmussen, G. H. (2016). Yield Per Recruit from Stocking Two Different Sizes of Eel (Anguilla anguilla) in the Brackish Roskilde Fjord. ICES J. Mar. Sci. 73, 158–164. doi:10.1093/icesjms/fsv167
Pike, C., Crook, V., and Gollock, M. (2020). “Anguilla anguilla,” in The IUCN Red List of Threatened Species. e.T60344A152845178.
Piper, A. T., Wright, R. M., Walker, A. M., and Kemp, P. S. (2013). Escapement, Route Choice, Barrier Passage and Entrainment of Seaward Migrating European Eel, Anguilla anguilla, within a Highly Regulated Lowland River. Ecol. Eng. 57, 88–96. doi:10.1016/j.ecoleng.2013.04.030
Podda, C., Palmas, F., Frau, G., Chessa, G., Culurgioni, J., Diciotti, R., et al. (2020). Environmental Influences on the Recruitment Dynamics of Juvenile European Eels, Anguilla anguilla , in a Small Estuary of the Tyrrhenian Sea, Sardinia, Italy. Aquat. Conserv. Mar. Freshw. Ecosyst. 30 (8), 1638–1648. doi:10.1002/aqc.3362
Podda, C., Sabatini, A., Palmas, F., and Pusceddu, A. (2021). Hard Times for Catadromous Fish: the Case of the European Eel (Anguilla anguilla, L. 1758). Adv. Ocean. Limnol. 12 (2), 9997. doi:10.4081/aiol.2021.9997
Poff, N. L., Allan, J. D., Bain, M. B., Karr, J. R., Prestegaard, K. L., Richter, B. D., et al. (1997). The Natural Flow Regime. BioScience 47, 769–784. doi:10.2307/1313099
Poff, N. L., and Schmidt, J. C. (2016). How Dams Can Go with the Flow. Science 353, 1099–1100. doi:10.1126/science.aah557110.1126/science.aah4926
Postel, S., and Richter, B. D. (2004). Rivers for Life: Managing Water for People and Nature, 41. Washington DC: Island Press, 41–4059. doi:10.5860/choice.41-4059Rivers for Life: Managing Water for People and NatureChoice Rev. Online
Potter, I. C., Chuwen, B. M., Hoeksema, S. D., and Elliott, M. (2010). The Concept of an Estuary: a Definition that Incorporates Systems Which Can Become Closed to the Ocean and Hypersaline. Estuar. Coast. Shelf Sci. 87, 497–500. doi:10.1016/j.ecss.2010.01.021
R Core Team (2021). R: A Language and Environment for Statistical Computing. [WWW Document]. R Found. Stat. Comput. Vienna. URL: https://www.r-project.org.
Rahel, F. J. (2013). Intentional Fragmentation as a Management Strategy in Aquatic Systems. Bioscience 63, 362–372. doi:10.1525/bio.2013.63.5.9
Regione Autonoma della Sardegna (2021). Sardegna Geo-Portale. Available at: http://www.sardegnageoportale.it/index.html.
Richter, B. D., Postel, S., Revenga, C., Scudder, T., Lehner, B., Churchill, A., et al. (2010). Lost in Development’s Shadow: The Downstream Human Consequences of Dams. Water Altern. 3 (2), 14–42.
Rincón, G., Solana-Gutiérrez, J., Alonso, C., Saura, S., and García de Jalón, D. (2017). Longitudinal Connectivity Loss in a Riverine Network: Accounting for the Likelihood of Upstream and Downstream Movement across Dams. Aquat. Sci. 79, 573–585. doi:10.1007/s00027-017-0518-3
Rodeles, A. A., Galicia, D., and Miranda, R. (2021). A Simple Method to Assess the Fragmentation of Freshwater Fish Meta-Populations: Implications for River Management and Conservation. Ecol. Indic. 125, 107557. doi:10.1016/j.ecolind.2021.107557
Russi, D., Ten Brink, P., Farmer, A., Badura, T., Coates, D., Förster, J., et al. (2013). The Economics of Ecosystems and Biodiversity for Water and Wetlands. Available at: https://www.cbd.int/financial/values/g-ecowaterwetlands-teeb.pdf.
Sabatini, A., Cannas, R., Follesa, M. C., Palmas, F., Manunza, A., Matta, G., et al. (2011). Genetic Characterization and Artificial Reproduction Attempt of Endemic Sardinian troutSalmo truttaL., 1758 (Osteichthyes, Salmonidae): Experiences in Captivity. Italian J. Zoology 78, 20–26. doi:10.1080/11250003.2010.497171
Sabatini, A., Podda, C., Frau, G., Cani, M. V., Musu, A., Serra, M., et al. (2018). Restoration of Native Mediterranean Brown Trout Salmo Cettii Rafinesque, 1810 (Actinopterygii: Salmonidae) Populations Using an Electric Barrier as a Mitigation Tool. Eur. Zoological J. 85, 137–149. doi:10.1080/24750263.2018.1453554
Saha, S., Arabameri, A., Saha, A., Blaschke, T., Ngo, P. T. T., Nhu, V. H., et al. (2021). Prediction of Landslide Susceptibility in Rudraprayag, India Using Novel Ensemble of Conditional Probability and Boosted Regression Tree-Based on Cross-Validation Method. Sci. Total Environ. 764, 142928. doi:10.1016/j.scitotenv.2020.142928
Segurado, P., Branco, P., and Ferreira, M. T. (2013). Prioritizing Restoration of Structural Connectivity in Rivers: a Graph Based Approach. Landsc. Ecol. 28 (7), 1231–1238. doi:10.1007/s10980-013-9883-z
Seliger, C., and Zeiringer, B. (2018). “Riverine Ecosystem Management,” in Riverine Ecosystem Management. Science for Governing towards a Sustainable Future. Editors S. Schmutz, and J. Sendzimir (Amsterdam: Springer). doi:10.1007/978-3-319-73250-3
Silva, A. T., Lucas, M. C., Castro-Santos, T., Katopodis, C., Baumgartner, L. J., Thiem, J. D., et al. (2018). The Future of Fish Passage Science, Engineering, and Practice. Fish. Fish. 19, 340–362. doi:10.1111/faf.12258
Stanley, E. H., and Doyle, M. W. (2003). Trading off: the Ecological Effects of Dam Removal. Front. Ecol. Environ. 1, 15–22. doi:10.1890/1540-9295(2003)001[0015:toteeo]2.0.co;2
Suari, Y., Amit, T., Gilboa, M., Sade, T., Krom, M. D., Gafny, S., et al. (2019). Sandbar Breaches Control of the Biogeochemistry of a Micro-estuary. Front. Mar. Sci. 6, 1–15. doi:10.3389/fmars.2019.00224
Tamario, C., Calles, O., Watz, J., Nilsson, P. A., and Degerman, E. (2019). Coastal River Connectivity and the Distribution of Ascending Juvenile European Eel ( Anguilla anguilla L.): Implications for Conservation Strategies Regarding Fish‐passage Solutions. Aquat. Conserv. Mar. Freshw. Ecosyst. 29, 612–622. doi:10.1002/aqc.3064
Tickner, D., Opperman, J. J., Abell, R., Acreman, M., Arthington, A. H., Bunn, S. E., et al. (2020). Bending the Curve of Global Freshwater Biodiversity Loss: an Emergency Recovery Plan. BioScience 70, 330–342. doi:10.1093/biosci/biaa002
Trancart, T., Carpentier, A., Acou, A., Charrier, F., Mazel, V., Danet, V., et al. (2020). When “Safe” Dams Kill: Analyzing Combination of Impacts of Overflow Dams on the Migration of Silver Eels. Ecol. Eng. 145, 105741. doi:10.1016/j.ecoleng.2020.105741
Trancart, T., Tétard, S., Acou, A., Feunteun, E., Schaeffer, F., and de Oliveira, E. (2018). Silver Eel Downstream Migration in the River Rhine, Route Choice, and its Impacts on Escapement: A 6-year Telemetry Study in a Highly Anthropized System. Ecol. Eng. 123, 202–211. doi:10.1016/j.ecoleng.2018.09.002
Turner, S. M., Chase, B. C., and Bednarski, M. S. (2018). Evaluating the Effect of Dam Removals on Yellow‐Phase American Eel Abundance in a Northeastern U.S. Watershed. North Am. J. Fish. Manage 38, 424–431. doi:10.1002/nafm.10040
VV, A. A. (2022). Carta Ittica Della Sardegna – D.G.R. N. 2/28, 428. del 20/01/2022. Regione Autonoma della Sardegna (ADA/STNPF)/Università degli Studi di Cagliari (DISVA)Available online: https://delibere.regione.sardegna.it/protected/58916/0/def/ref/DBR58914/ (accessed on march 21, 2022).
Vörösmarty, C. J., McIntyre, P. B., Gessner, M. O., Dudgeon, D., Prusevich, A., Green, P., et al. (2010). Global Threats to Human Water Security and River Biodiversity. Nature 467, 7315555–561. doi:10.1038/nature09440
Van Ginneken, V. J. T., and van den Thillart, G. E. E. J. M. (2000). Eel Fat Stores Are Enough to Reach the Sargasso. Nature 403, 156–157. doi:10.1038/35003110
Verbiest, H., Breukelaar, A., Ovidio, M., Philippart, J.-C., and Belpaire, C. (2012). Escapement Success and Patterns of Downstream Migration of Female Silver Eel Anguilla anguilla in the River Meuse. Ecol. Freshw. Fish. 21, 395–403. doi:10.1111/j.1600-0633.2012.00559.x
Watz, J., Nilsson, P. A., Degerman, E., Tamario, C., and Calles, O. (2019). Climbing the Ladder: an Evaluation of Three Different Anguillid Eel Climbing Substrata and Placement of Upstream Passage Solutions at Migration Barriers. Anim. Conserv. 22 (5), 452–462. doi:10.1111/acv.12485
Welcomme, R. L. (1995). Relationships between Fisheries and the Integrity of River Systems. Regul. Rivers Res. Mgmt. 11, 121–136. doi:10.1002/rrr.3450110110
White, E. M., and Knights, B. (1997). Environmental Factors Affecting Migration of the European Eel in the Rivers Severn and Avon, England. J. Fish Biol. 50, 1104–1116. doi:10.1111/j.1095-8649.1997.tb01634.x
Wilkes, M. A., Mckenzie, M., and Webb, J. A. (2018). Fish Passage Design for Sustainable Hydropower in the Temperate Southern Hemisphere: An Evidence Review. Rev. Fish. Biol. Fish. 28 (1), 117–135. doi:10.1007/s11160‐017‐9496‐8
Zarfl, C., Lumsdon, A. E., Berlekamp, J., Tydecks, L., and Tockner, K. (2015). A Global Boom in Hydropower Dam Construction. Aquat. Sci. 77, 161–170. doi:10.1007/s00027-014-0377-0
Keywords: Diadromous species, European eel, freshwater ecosystems, damming, river fragmentation
Citation: Podda C, Palmas F, Pusceddu A and Sabatini A (2022) When the Eel Meets Dams: Larger Dams’ Long-Term Impacts on Anguilla anguilla (L., 1758). Front. Environ. Sci. 10:876369. doi: 10.3389/fenvs.2022.876369
Received: 15 February 2022; Accepted: 23 June 2022;
Published: 18 July 2022.
Edited by:
Robert Ptacnik, Wasser Cluster Lunz, AustriaReviewed by:
Jeyaraj Antony Johnson, Wildlife Institute of India, IndiaMaria Filomena Magalhães, Faculty of Sciences, University of Lisbon, Portugal
Copyright © 2022 Podda, Palmas, Pusceddu and Sabatini. This is an open-access article distributed under the terms of the Creative Commons Attribution License (CC BY). The use, distribution or reproduction in other forums is permitted, provided the original author(s) and the copyright owner(s) are credited and that the original publication in this journal is cited, in accordance with accepted academic practice. No use, distribution or reproduction is permitted which does not comply with these terms.
*Correspondence: Cinzia Podda, cpodda@unica.it