- 1Department of Biological, Geological and Environmental Sciences, Alma Mater Studiorum University of Bologna, Bologna, Italy
- 2School of Environmental Studies, China University of Geosciences, Wuhan, China
- 3Department of Environmental Sciences, Kohat University of Science and Technology, Kohat, Pakistan
- 4Department of Land Surveying and Geo-Informatics, The Hong Kong Polytechnic University, Hong Kong, Hong Kong SAR, China
- 5Zoology Department, College of Science, King Saud University, Riyadh, Saudi Arabia
- 6Research Centre for Food and Nutrition, Council for Agricultural Research and Economics, Rome, Italy
- 7Zoology Department, Faculty of Science, Cairo University, Giza, Egypt
- 8Department of Botany, Abdul Wali Khan University Mardan, Mardan, Pakistan
- 9Natural and Medical Sciences Research Center, University of Nizwa, Nizwa, Oman
Arsenic (As) contamination in drinking groundwater sources is a common environmental problem in Pakistan. Therefore, we collected a total of (n = 81) drinking groundwater samples from various groundwater sources, from two districts’ namely Ghotki (n = 44) and Nawab Shah (n = 37) of Sindh, Province, Pakistan. The current research aimed to investigate the hydrogeochemistry of groundwater with elevated arsenic (As), its effect on the health of local population living in the study area, potential sources of groundwater variables, and the suitability of groundwater for ingestion motive. The results showed that groundwater sample variables of both districts had moderate to high concentration levels. The cations concentrations were observed in decreasing order of Na+ > Ca+ > Mg+ > K+, for both districts, while anions abundance was HCO3− > Cl− > SO42− > F− > NO3− in the Ghotki, and HCO3− > SO42− > Cl− > NO3− > F− in the Nawab Shah, respectively. Arsenic (As) had low to high concentration levels in both districts, observed with average values of 10.1 μg/L and 21.0 μg/L in the Ghotki and Nawab Shah, respectively. The water type was mixed CaMgCl type in both districts, while the groundwater sources were saturated for CO3 minerals indicated by the saturation indices results. Principal component analysis showed the geogenic source of ion exchange, dissolution of minerals, weathering of host rocks and anthropogenic input of industrial activities, agricultural practices and domestic waste for groundwater contamination. The water quality index (WQI) exhibits that majority of groundwater samples (73.6% of Ghotki and 65.7% of Nawab Shah) were suitable for drinking. The non-carcinogenic risk (HQ) values of As were (>1) in groundwater samples of the Ghotki and Nawab Shah, while a high mean HQ value (8.78 × 100) was recorded for children in Nawab Shah. The carcinogenic risk (CR) values of As were significantly higher than the maximum threshold CR value (1 × 10–4). This study emphasized that monitoring strategies are substantially needed to mitigate the As contamination to reduce the potential health risk.
Introduction
Groundwater is a vital source of freshwater resources which is used for drinking and other domestic purposes in many developing countries (Chen et al., 2020). However, groundwater could be contaminated due to anthropogenic and natural processes (Huang et al., 2013; Huang et al., 2014). The factors including weathering of rocks, water-rock interaction, ion exchange, soil mineralization, atmospheric precipitation, and evaporation process are the most important mechanisms as well as the impact of anthropogenic activities that could influence the geochemical composition of groundwater (Singh et al., 2017; Liu et al., 2020). The over-exploitation of groundwater, leaching of chemical fertilizers and wastewater discharge, sewage spills, land-use changes, landfills, and associated anthropogenic activities affect the groundwater quality (Kumar and Singh 2015; Chung et al., 2018; Selvam et al., 2018; Zaki et al., 2019; Huang et al., 2020b). Rapid industrialization and urbanization have contributed to anthropogenic inputs in recent decades, which may affect groundwater quality (Liu et al., 2020). The contamination in groundwater intensifies and persists for a long period of time because of the low proportion of groundwater flowing rate in the aquifer system. A comprehensive evaluation of groundwater quality is needed to configure the hydrogeochemistry by numerous methods. For this purpose, researchers have strategically adopted various techniques to investigate the hydrochemical characteristics of groundwater chemistry. Various geo-statistical approaches and multivariate statistical analysis have been implied to comprehensively understand the geochemistry of groundwater, spatial distribution of contaminants level, water quality index, and health risk assessment in many developing countries (Singh et al., 2011a; Singh et al., 2011b; Avtar et al., 2013; Dehghanzadeh et al., 2015). For instance, nitrate, fluoride, and arsenic were frequently recorded as the main contributors to groundwater contamination worldwide (Zhang Q. et al., 2020; Adimalla 2021; Natasha et al., 2021; Mwiathi et al., 2022). Research work has been conducted in the past, concerning groundwater quality and its hydrochemistry worldwide (Huang et al., 2018a; van Geen et al., 2019; Kumar and Singh 2020; Mallick et al., 2021a; Mallick et al., 2021b; Dashora et al., 2022; Khattak et al., 2022). Thus, it is very crucial to investigate and monitor the groundwater quality and detail hydrochemistry, to protect the health of the exposed local population, especially in highly contaminated areas of Sindh, Pakistan.
Although arsenic (As) can be released into the groundwater through geogenic sources, anthropogenic activities could enhance As contamination in the aquifers (Vithanage et al., 2017; Mukherjee et al., 2019; Tweed et al., 2020; Mushtaq et al., 2021; Saeed et al., 2021). High As content might also be derived from oxidative desorption in groundwater along with the evaporative process (Naseem and McArthur 2018; Natasha et al., 2020). Humans could be exposed to As through ingestion, inhalation, and dermal contact routes. Drinking As-contaminated water poses a potential risk to human health among the aforementioned exposure routes (Tabassum et al., 2019; Wei et al., 2019). Exposure to elevated As may cause multi-organs cancer, pigmentation, hand palm hardness, and soles of feet, as proposed by WHO, (2001). The majority of the people are exposed to As-toxicity across the globe via the As-enriched drinking water consumption. It has been reported that arsenic can cause cancer through consumption even at low concentrations (Ghosh and Mukhopadhyay, 2019). Elevated levels of As may disrupt the stomach, cause vomiting and diarrhea, and disfunction the nerves with the sensation of “needles and pins” in the hands and feet, skin deformations, resulting in wart-like growths (Caussy, 2005). Untreated and contaminated drinking water has a detrimental effect on human health deteriorates and harms different body organs, creating heart diseases and hypertension, kidney disorder, and malfunction of the peripheral nerve system (Mohamed et al., 2018; Lkr et al., 2020). Therefore, the local population of an area should be more aware of drinking water quality and its suitability for domestic and industrial usage (Chung et al., 2019; Li and Hou, 2020). Hence, the assessment of groundwater quality needs to be explored for hydrochemistry and its suitability for ingestion motive.
Pakistan is also facing the same problem regarding groundwater contamination with As and other heavy metals have affected the groundwater quality in many regions of Pakistan, especially in Sindh, Province (Ali et al., 2019b; Talib et al., 2019; Siddique et al., 2020; Iqbal et al., 2021; Ullah et al., 2021). According to a survey by the Pakistan Council of Research in Water Resources (PCRWR), arsenic was detected in the aquifers of Sindh Province, such as Manchar lake, Hyderabad, Jamshoro, and Sanghar, 16–36% of people were affected due to ingestion of As contaminated drinking groundwater as tap water. In the district of Hyderabad, 40 people died in 2004 due to ingestion of water contaminated with heavy metals (Ullah et al., 2021). The groundwater contamination has recently been increased with an increased population in Lower Indus plains regions in Sindh province. In this context, it is necessary to investigate the drinking water sources to protect the health risk of the local inhabitants. To our knowledge, limited work has been conducted on arsenic contamination in drinking groundwater sources from Lower Indus residential areas of Ghotki and Nawab Shah.
Hence, a detailed study was conducted to investigate the geochemical behavior of elevated As, and its source of mobilization into the groundwater sources of the study area using multivariate statistical approaches and geochemical modeling. Therefore, the objectives of the present study were 1) to elucidate the hydrogeochemical characteristics of groundwater variables with a main emphasis on the spatial distribution of arsenic contamination, and 2) to assess the suitability of groundwater for drinking with relative World Health Organization (WHO) guidelines, and 3) to evaluate carcinogenic and non-carcinogenic risks in the groundwater of Ghotki and Nawab Shah districts.
Materials and Methods
Study Area Description
Ghotki district is situated (27° 49′ N and 69° 39′ E) in upper Sindh province, Pakistan (Figure 1). District Ghotki is extended to 6975 km2 and geographically divided into three regional parts, i.e., cultivable area, white deserted area, and flooded area.
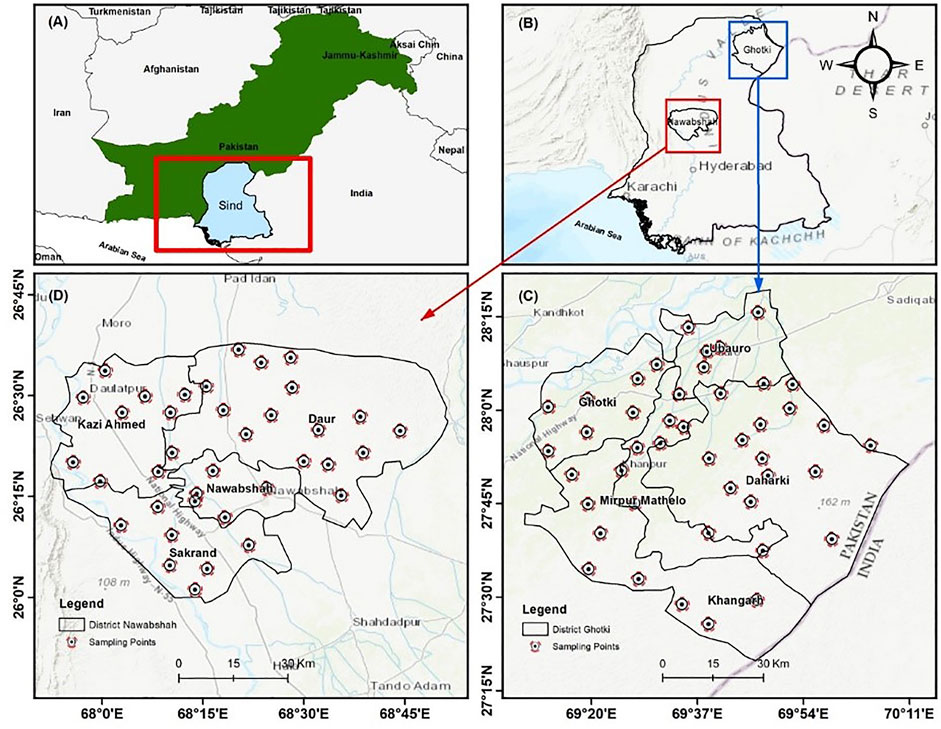
FIGURE 1. Groundwater sampling points in the study area of the (C) Ghotki and (D) Nawab Shah districts, in Sindh Province (B), Pakistan.
The white desert area is comprised of peaks of high wind-blown sand, also known as Achro Thar, and is extended from Sanghar district to the Cholistan desert of Punjab province and is connected with the border of the Rajasthan state in India. The Indus River continuously flows in the Ghotki region from northeast to southwest. The flood plain (also known as Katcha is bounded by safety bunds and forests, a total area of 1.6 km2, and covers 25.8% of the total area (PESA, 2014). The cultivable area is situated in the center of the district between the flood and desert areas. This land is fertile and is irrigated through the Ghotki canal irrigation system. District Nawab Shah is located between 26° 35′ N and 68° 10′ E in the center of the Sindh province, Pakistan and Nawab Shah city lies in the center of the Sindh province (Figure 1). Most of the soil is fertile and suitable for growing crops, whereas some saline soil and uncultivated soil are also included in the region. Rohri canal provides the essential water source for drinking and irrigation purposes in the study area. The village people mostly use groundwater resources for drinking purposes in Nawab Shah district. The climate of the area is dry and hot but the temperature falls to −40°C from (December to February). The lower Indus plain of both districts is covered by sedimentary rocks having geological tertiary and Cretaceous formations, which contain the dolomite, limestone, chert and marl rocks, and chalky limestone. The main source of fresh water in the study area includes boreholes, tube wells, hand pumps, spring water, and the Indus River. The fresh water of the lower Indus aquifer extends to 50 m deep, and becomes saline in the deep water table. Thus, the groundwater aquifers may possibly be affected in Ghotki and Nawab Shah districts. Furthermore, the Chemi Visco Fiber and Habib Sugar Mills industries discharge the effluents into the Gajra Wah Canal flowing along the Nawab Shah adjacent to Ghotki, which deteriorates the surface and groundwater quality (Khuhawar and Majidano, 2011). Due to this reason, the present study has been conducted to comparatively investigate the groundwater contamination of the Ghotki and Nawab Shah.
Groundwater Sampling
The groundwater sampling campaign was conducted during September 2018. The groundwater samples were randomly collected from districts Ghotki (n = 44) and Nawab Shah (n = 37) of Sindh Province, Pakistan. Total samples (n = 81) were collected from boreholes, tube wells, hand pumps, and dug wells in the Ghotki and Nawab Shah, that can be actively used for drinking and domestic purposes. The samples were taken from hand pumps (12), boreholes (13), tube wells (12), and dug wells (7) in three different geographical regional parts, i.e., cultivable area (n = 18), white deserted area (n = 9), and flooded area (n = 17) in Ghotki district whereas the groundwater samples were randomly collected from hand pumps (10), boreholes (12), tube wells (9), and dug wells (6) in domestic regions of the Nawab Shah district.
The groundwater table depth in the Ghotki area varies between 1.48 and 16.2 m, with an average of 5.24 m. While the water table depth in the Nawab Shah area varies between 1.39 and 15.4 m, with an average of 5.10 m. The groundwater samples were filtered through a filtration process on-site using a 0.45 μm membrane. All the groundwater samples were collected in two sets of polyethylene 1.5 L plastic bottles after filtration, following the procedure adopted by Natasha et al. (2021). To avoid any kind of contamination from the pipes, water was continuously purged and pumped for 4–6 min through the taps before the groundwater samples were collected, this procedure was adopted by Tabassum et al. (2019). All the samples were clear with no suspended particles and were kept in Ice-bath and immediately transported to the PCRWR laboratory. The samples were then stored at 4°C in a dark place until analysis.
Chemical Analysis
The physiochemical parameters such as TDS, EC, and pH were measured during the sample collected by the electrochemical analyzer and pH meter (Hac 44600-00, Loveland, CO, United States (Rehman et al., 2020). Major cations such as Na+ and K+ were measured using a flame photometer (PFP7, Cambridge, United Kingdom). A volumetric titration method was used to achieve Ca2+ and Mg2+ values with ethylene diamine tetra acetic acid (EDTA, 0.05 N) of (<2%) analytical error (Gao et al., 2020). Anions such as NO3− and SO42− were examined by ultraviolet-visible (UV-VIS) spectrophotometer (Germany). Bicarbonate (HCO3−) and chloride (Cl−) ions were measured by titration method. Arsenic was analyzed in groundwater samples by atomic absorption spectrophotometer (AAS Vario 6, Analytik Jena, Jena, Germany).
Quality Control
The reagent blanks, standards, and duplicate samples were used for quality control. The reproducibility of analytical data was 5% and analytical error estimation was 10%. Standard reference material of NIST (SRM; 1640) was used to validate the arsenic results in groundwater by hydride generation atomic absorption spectrophotometry (HG-AAS). The analyzed As concentration by NIST SRM was also closed to the certified As concentration on an average (25.29 ± 0.43 μg/L) with 102.3% recovery. All analyses were performed in triplicate.
Mineral Phases
Saturation indices (SI) were calculated to investigate the equilibrium status of minerals phases by PHREEQC 3.0 (Parkhurst and Appelo, 2013). The SI can be identified by the following equation:
where IAP is the ion activity product, K is the equilibrium constant. The SI was classified based on equilibrium conditions (SI = 0), oversaturation (SI > 0), and undersaturation (SI < 0).
Water Quality Assessment
The water quality index (WQI) was calculated to assess the groundwater suitability for drinking purposes, as recommended by WHO (2017). For this purpose, WQI was computed for 81 Ghotki and Nawab Shah districts' groundwater quality samples in the present study. WPI can be calculated by a series of steps, as followed by Ullah et al. (2021), and WHO standards for each parameter were used in mg/L (Supplementary Table S1). WQI was computed for selected parameters using the summation of sub-indices in each sample (Eq. 2):
Health Risk Assessment
The health risk assessment is a useful quantitative method that can be used to quantify the relationship among toxic elements in drinking water and human health. Health risk assessment is internationally recognized by USEPA (2005), and includes hazard assessment, dose-effect potential analysis, exposure identification, and risk characterization (Adimalla and Qian, 2019; Liu et al., 2021). The chronic daily intake (CDI) was first estimated (Eq. 3) for As to evaluate the carcinogenic and non-carcinogenic risks via ingestion exposure route. The non-carcinogenic risk of hazard quotient (HQ) was calculated to divide the CDI values on reference dose (RfD) (Eq. 4), and the carcinogenic risk (CR) was then calculated by multiplying the CDI’s with cancer slope factor (CSF) (Eq. 5) (Shah et al., 2020).
In the above equations, C represents As concentration (μg/L) in both the Ghotki and Nawab Shah districts’ groundwater, IR shows ingestion rate of 2 L/day, BW is body weights for adults (70 kg), and for children the BW is 32.7, RfD is oral reference dose of As (0.3 μg/kg/day), while CSF refers to cancer slope factor of As (1500 μg/kg/day−1) (USEPA, 2011).
Statistical Data Analysis
Descriptive statistics such as minimum and the maximum level, mean and standard deviation were calculated (IBM-SPSS software version 25). Principal component analysis was used to identify the pollution sources, while Pearson correlation analysis was applied to evaluate the correlations between water quality parameters. A Piper diagram was made to interpret the hydrochemical facies by Aqua-Chem (version 2010.1). Saturation indices and geological study area map were computed by geochemical modeling of PHREEQC (version 3.1), whereas water quality map and arsenic distribution level map were made by inverse distance weightage (IDW) interpolation method, using ARC GIS (version 9.3).
Results and Discussion
Characteristics of Groundwater Chemistry
Table 1 shows the geochemical composition of groundwater samples collected from the study area. The pH of groundwater samples was in the range of 7–8.3 with a mean value of 7.63 in the district Ghotki, whereas pH was varied from 6.4–8.2 with a mean value of 7.25 in the aquifers of Nawab Shah district. The pH level indicates the alkaline nature of groundwater sources in the Ghotki and Nawab Shah. pH has a significant impact as an important water quality parameter on water chemistry, alkalinity, and solubility of arsenic (Selvakumar et al., 2017). In the present study, the leaching of rain water in soil may enhance the alkaline characteristics in the groundwater of the study areas. Moreover, the cation exchange process and silicate weathering are also subjected to an increase in the alkaline nature of the groundwater pH (Mushtaq et al., 2021). Electrical conductivity (EC) of groundwater samples ranged from 416–2210 μs/cm, and 310–2148 μs/cm with mean values of 1133 μs/cm and 1083 μs/cm in the groundwater sources of Ghotki and Nawab Shah, respectively. Furthermore, the total dissolved solids (TDS) concentration of the samples was in the range of 309–1954 mg/L with an average value of 807 mg/L in the Ghotki, while in Nawab Shah, the TDS values of the samples ranged from 405–2524 mg/L with a mean value of 1182 mg/L. The presence of high TDS could be attributed to ions leaching into the groundwater system (Loh et al., 2020). The turbidity values ranged from 0.06 to 2 Nephelometric Turbidity Units (NTUs) with a mean value of 0.94 NTUs in Ghotki, while the turbidity values varied between 0 and 7 NTUs with a mean value of 2.92 NTUs in Nawab Shah, respectively. The higher turbidity results in shallow-depth wells due to contamination exposure as compared to deep and mid-depth aquifers in the groundwater system. Moreover, in the present study, the occurrence of high turbidity in some samples could have resulted from shallow and poorly constructed wells in the study area (Boonkaewwan et al., 2021). Among cations, Na+ was predominantly observed with high concentration, ranging from 32–410 mg/L, and 31–593 mg/L with mean values of 107 mg/L, and 143 mg/L in Ghotki and Nawab Shah, respectively. High occurrence of Na+ concentration in the groundwater along with high TDS values from both districts' samples could be possibly associated with the dissolution of halite and water-rock interaction (Roy et al., 2021). In addition, weathering of silicate, halite, and plagioclase minerals dissolution is subjected to the release of Na+ in adjacent waterbodies (Rashid et al., 2019). In contrast, K+ had low concentrations ranging from 1.5–5.5 mg/L, and 2–17 mg/L, with mean values of 2.77 mg/L and 5.56 mg/L in the samples from Ghotki and Nawab Shah, respectively. Furthermore, Ca2+ and Mg2+ showed moderate concentrations level with average values of 58.3 and 83.2 mg/L, 49.3 and 53.9 mg/L in Ghotki and Nawab Shah, respectively.
Similarly, HCO3− was the most dominant among anions and it varied between 122 and 640 mg/L, and 61–815 mg/L with average values of 297 mg/L, and 285 mg/L in Ghotki and Nawab Shah, respectively (Table 1). While SO42− was the second most abundant anion with concentrations varying between 31–398 mg/L, and 11–593 mg/L, with average values of 103 mg/L, and 119 mg/L, respectively. HCO3− and SO42− were enriched in the study area of the district Ghotki and Nawab Shah. HCO3− prevalence in both districts suggests carbonate solubilization, and it could be also derived from naturally occurring CO2 dissolved with carbonate-containing minerals or results from atmospheric deposition of CO2 with rainwater (Ismail et al., 2018). While high SO42− in the present study could be possibly originated from halite, anhydrite, and gypsum dissolutions (Roy et al., 2021). Cl− also exhibit high concentration levels ranging from 38–497 mg/L, and 14–183 mg/L, with mean values of 123 mg/L and 69.6 mg/L in the above-mentioned study areas, respectively. High Cl− contamination could be particularly influenced by industrial and agricultural activities as well as municipal waste (Adimalla, 2021). However, F− and NO3− had the lowest mean concentrations of 0.34 mg/L, and 0.26 mg/L in the district Ghotki and Nawab Shah, respectively. The concentrations of cations were observed in decreasing order of Na+ > Ca+ > Mg+ > K+, and the concentrations of anions were HCO3− > Cl− > SO42− > F− > NO3− in the Ghotki, whereas the concentrations of cations and anions showed decreasing order: Na+ > Ca+ > Mg+ > K+, and HCO3− > SO42− > Cl− > NO3− > F− in the district Nawab Shah, respectively. Fe had low mean concentrations of 0.05, and 0.13 mg/L in the Ghotki and Nawab Shah, respectively. Arsenic (As) had low to high concentration levels from 0.5–42 μg/L, and 2–70 μg/L with average concentrations of 10.1 μg/L and 21.0 μg/L in the Ghotki and Nawab Shah, respectively (Table 1). Elevated As contents emphasized and influenced the groundwater chemistry in both districts’ regions. High arsenic enrichment could be associated with excessive use of pesticides and water-logging (Çiner et al., 2021), which can be introduced into the Ghotki and Nawab Shah groundwater systems. Furthermore, natural arsenic mobilization can be enhanced in the groundwater due to a decrease in the dissolution of pH-based iron hydroxide (FeOH) and maximum sorption of bicarbonate minerals in the presence of microorganisms with evaporation enrichment (Talib et al., 2019). In comparison, concentrations of parameters were high in groundwater samples of the Ghotki as compared to Nawab Shah.
Hydrochemical Types
Piper diagram is used for the identification of hydrochemical evolution regarding the chemical composition of groundwater (Piper, 1944) in the study area. The piper plots have been previously used to identify high similarities and differences in classification in groundwater and chemical types composition (Jehan et al., 2019). The trilinear Piper’s plots of major ions for the Ghotki and Nawab Shah are shown in Figures 2A,B. It shows that the majority of groundwater samples (88%) are classified into mixed CaMgCl water type in the Ghotki, while five samples (12%) were observed in the CaCl type in the Ghotki groundwater samples. The cation triangle revealed that sample points were mostly found in zone B (no dominant type), and a calcium type was observed in zone A in the piper diagram of Ghotki. The anion triangle showed that the groundwater samples points were mainly distributed in zone B of no dominant type, and in F zone of sulfate type in the Ghotki. (Figure 2A).
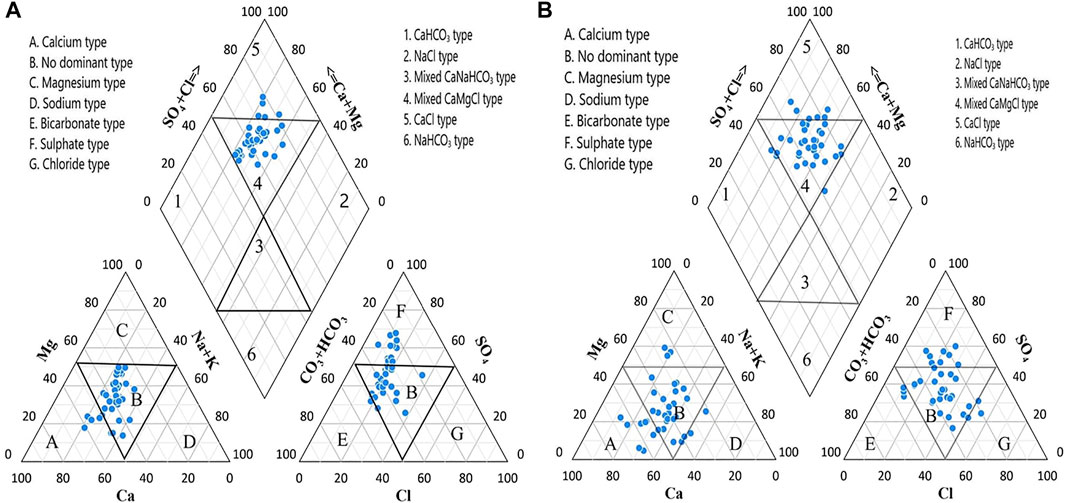
FIGURE 2. Piper diagram shows the groundwater type categories in the study area of the (A) Ghotki and (B) Nawab Shah.
Similar results were found in the groundwater samples of the Nawab Shah, Piper plot. Most of the groundwater samples (86%) were classified into mixed CaMgCl water types with few samples (14%) found in the CaCl type in the Nawab Shah, groundwater samples (Figure 2B). These water types suggest the mixed dissolved saline water resulted from surface water, and the addition of high evaporates widely dispersed in the aquifer system (Siddique et al., 2020). The cation triangle exhibit that sample points of groundwater are primarily placed in zone B, demonstrating no dominant type, and a calcium type in zone A, and in zone C with magnesium type, and few samples (2 samples) in sodium type. The anion triangle showed that the water sample points were mainly distributed in zone B (no dominant type), and in F zone of sulfate type, with few samples (three samples) in zone E of Bicarbonate type, and two samples in zone G of chloride type in the Nawab Shah, as shown in Figure 2B. Hence, the hydrochemical facies results showed the aforementioned mixed water types, influencing the chemical composition of the Ghotki and Nawab Shah groundwater sources.
Factors Controlling the Groundwater Chemistry in the Ghotki and Nawab Shah
The evaporation and precipitation process, rock weathering, and crystallization are primary contributors that influence and control the formation of groundwater chemistry (Kimbi et al., 2021). In the present study, the Gibbs ratio was used to investigate the mechanisms controlling groundwater chemistry (Gibbs, 1970). Gibbs diagrams can be considered as a significant method to determine groundwater chemistry by distinguishing the natural processes. It has been revealed in the Gibbs plot that almost all of the groundwater samples of the Ghotki district fall in the rock dominance zone, as shown in Supplementary Figures S1A,B. However, the evaporation and precipitation were found negligible to influence the groundwater chemistry in the study area.
Similarly, the majority of samples (86%) from all aquifers in the Nawab Shah district, the plot fell into the rock dominance zone, while (14%) of the samples fell into the evaporation dominance zones (see Supplementary Figures S1C,D), indicating that rock-forming weathered minerals and infiltration of precipitation were the primary factors that influenced groundwater chemistry (Kumaravel et al., 2014). Some groundwater samples from the Nawab Shah fall in the evaporation dominance zone, demonstrating the impact of surface river water on the groundwater system, which could be possibly trapped during the sedimentation process (Mani et al., 2008), as well as the importance of evaporation in the depth zone of shallow groundwater. Anthropogenic activities may also affect the groundwater samples that fell beyond the plots (Srinivasamoorthy et al., 2008). The process of minerals dissolution and dissolved salts become assimilated with groundwater aquifers under the influence of weathering of parent rocks. In addition, the cation exchange mechanism also plays a critical role in controlling groundwater chemistry composition in the study area (Rashid et al., 2020; Haji et al., 2021; Noor et al., 2022). This observation recommends that rock weathering is the foremost driving factor controlling the groundwater chemistry in the study area of both Ghotki and Nawab Shah districts. The Gibbs plots results were found consistent with the recent relevant studies conducted in Pakistan (Talib et al., 2019; Iqbal et al., 2021; Ullah et al., 2021).
Saturation Indices for Minerals Phases
Saturation indices (SI) is an important standard that can be used to quantify the equilibrium potential status of the contribution of various minerals in groundwater aquifer system (Yang et al., 2020). In this study, the SI values were calculated for minerals phases of calcite, dolomite, gypsum, and halite. Positive SI values show mineral supersaturation, whereas negative SI values represent mineral undersaturation in groundwater. It was shown that the SI values for calcite and dolomite were high (>0) for most of the samples in both districts, as shown in Figures 3A,B. The SI values of dolomite were found higher (SI: >1) in the Ghotki and Nawab Shah with mean values of 2.30 and 1.50, respectively, while most SI values of calcite were also observed to be higher than (SI: >1) in the Ghotki, and remained higher than zero in the Nawab Shah, with an average value of 1.13 and 0.77, as shown in Figure 3B. Thus, groundwater samples of Ghotki and Nawab Shah districts were oversaturated relative to dolomite and calcite minerals. The high SI’s of calcite and dolomite in groundwater may imply the phenomenon of maximum precipitation of calcite/dolomite deposition in water/H2O (Yu et al., 2021). In addition, a higher pH in the present study is subjected to enhance calcite mineral precipitation (Dahiphale et al., 2020). Additionally, Ca2+, Mg2+, and HCO3− were also highly observed in the present study, which could be released from dissolution containing calcium magnesium carbonate, ideally CaMg-(CO3)2, and carbonate-containing minerals or could result from atmospheric deposition of CO2 with rainwater (Ismail et al., 2018; Natasha et al., 2021). Moreover, calcite dissolution could derive from cretaceous calcareous and Jurassic sandstone (Zhang et al., 2021).
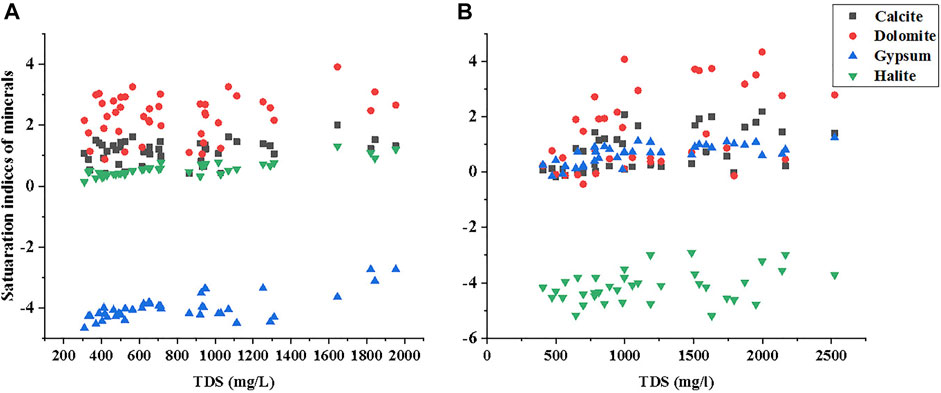
FIGURE 3. Saturation indices of Calcite, Dolomite, Gypsum, and Halite against TDS in the study area of (A) Ghotki and (B) Nawab Shah.
Similarly, Brahman et al. (2016) reported high SI of calcite (SI > 0) in the groundwater aquifers of two districts of Tharparkar, Sindh Pakistan, proposing precipitation of calcite and its dissolution during As release process. It shows that these minerals could occur in groundwater aquifers with primary basis of high As (Kaleem et al., 2021), due to the water–rock interaction. The SI’s of gypsum were above zero with mean values of (0.57) and (0.66) for all groundwater samples in both districts Ghotki and Nawab Shah, respectively (Figures 3A,B). While the SI’s of halite mineral were below zero with mean values of (−3.97), and (−4.14), in the Ghotki and Nawab Shah respectively, indicating a mineral unsaturated state in groundwater and inclination in continuous dissolution. The above findings revealed that the groundwater chemistry of Ghotki and Nawab Shah regions was dominated by dolomite/calcite dissolution and cation exchange relatively with correlations of major ions and saturation indices (Zhang et al., 2021). In the current study, the formation of carbonate-containing minerals, influenced by the As discharge with favorable alkaline conditions that could be observed in relevance to pH level in groundwater in the majority of water samples.
Source Identification and Correlation Analysis of Groundwater Parameters
Principal component analysis (PCA) was implied to find and classify the sources that influenced the groundwater variables (Talpur et al., 2020). Thus, the significant factors were obtained in PCA results, followed by the varimax rotation. Five factors were obtained including PC1, PC2, PC3, PC4 and PC5 for groundwater parameters, with eigenvalues of 8.16, 1.80, 1.37, 1.26, and 1.04 and total variance of 47.62, 10.46, 8.35, 6.65 and 6.11% for the Ghotki. While the eigenvalues of 6.29, 2.81, 1.48, 1.33, and 1.18 with total variance of 37.02, 16.55, 8.49, 7.80 and 6.91% for the Nawab Shah, respectively as shown in Table 2. The total loading factors and the first two factors of PC1 and PC2 are shown in Figures 4A–D.
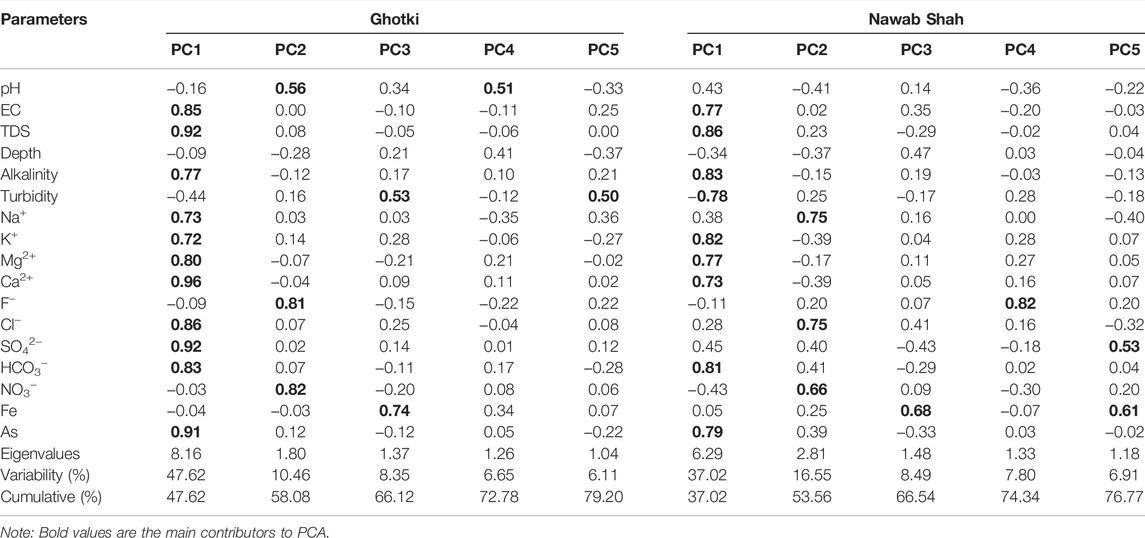
TABLE 2. Principal component analysis of selected groundwater parameters for the Ghotki and Nawab Shah districts.
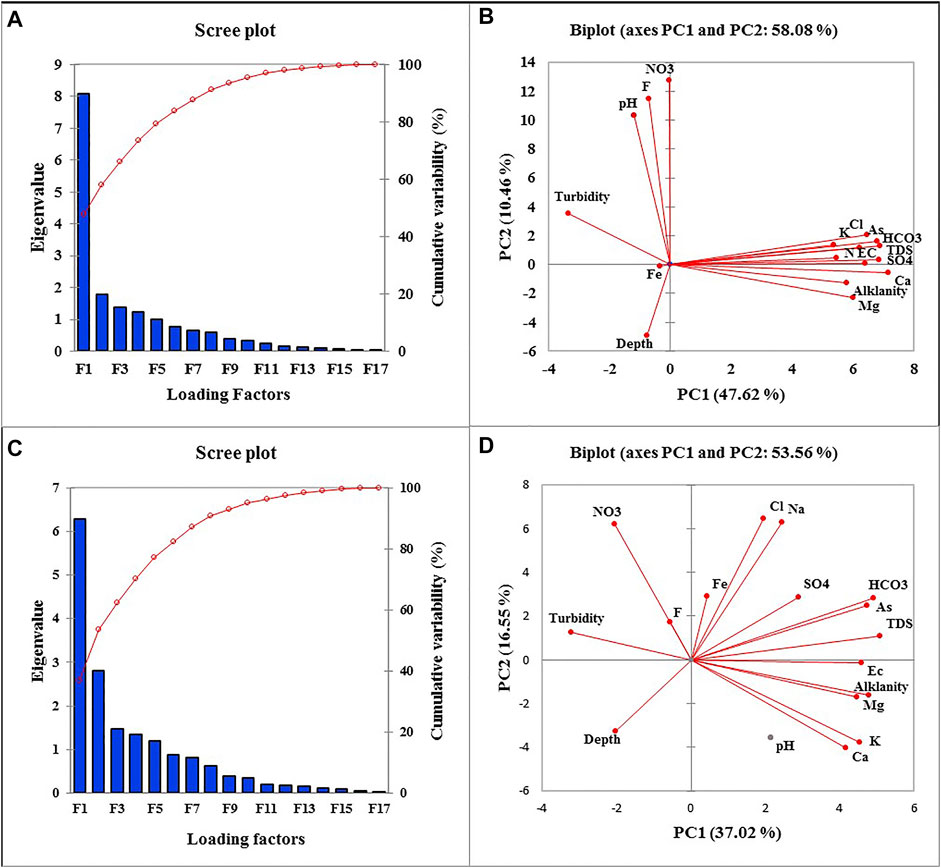
FIGURE 4. (A) Sum of all the calculated factors, (B) first and second factors shows 58.02% contribution for Ghotki, and (C) shows sum of all the calculated factors, (D) first and second factors shows 53.57% contribution for Nawab Shah.
PC1 had 47.62% of variability with an eigenvalue of 8.16. The strong loadings factors of EC, TDS, Alkalinity, Na+, K+, Mg+, Ca+, HCO3−, Cl−, SO42−, and As were calculated to be 0.85, 0.92, 0.77, 0.73, 0.72, 0.80, 0.96, 0.86, 0.92, 0.83, and 0.91, respectively in the Ghotki district. Similarly, PC1 had 37.02% of variability with an eigenvalue of 6.29 in the Nawab Shah. The significant loadings values in PC1 for EC, TDS, Alkalinity, Turbidity, K+, Mg+, Ca+, HCO3− and As were observed to be 0.77, 0.86, 0.83, -0.78, 0.82, 0.77, 0.73, 0.81, and 0.79, respectively in the Nawab Shah district. Thus, PC1 showed the highest contribution of strong loading factors in PCA results, demonstrating the geogenic and anthropogenic sources in the study area. The PC1 results indicate the ionic formation in groundwater, resulting from the ion exchange process, dissolution of minerals, and weathering of host rocks (Supplementary Figure S2) (Edmunds and Smedley, 2013; Huang et al., 2018b; Rashid et al., 2018; Huang et al., 2022).
PC2 exhibits 10.46% variability with an eigenvalue of 1.80. The moderate loadings factors of groundwater variables were observed to be pH (0.56), F− (0.81), and NO3− (0.82) in the Ghotki. Although for Nawab Shah, PC2 showed a contribution of 16.55% variability with an eigenvalue of 2.81. The moderate loading factors were calculated to be Na+ (0.75), Cl− (0.75), NO3− (0.66). The PC3, PC4, and PC5 showed the lowest contribution in PCA results with 8.35%, 6.65%, 6.11%, 8.49%, 7.80%, and 6.91% variability, and eigenvalues of 1.42, 1.13, 1.04 for the Ghotki and 1.36, 1.25, 1.11 for Nawab Shah. The significant moderate loading factors in PC3 were observed to be Turbidity (0.53), Fe (0.74) for the Ghotki, and Fe (0.68) for the Nawab Shah. In PC4, loading factors were pH (0.51) for the Ghotki, and F (0.82) for the Nawab Shah. While in PC5, moderate loading factors were Turbidity (0.50) calculated for the district Ghotki, whereas SO42− (0.53) and Fe (0.61) were recorded for the Nawab Shah, respectively (Table 2). High loading factors of Cl− and SO42− in PC2 may also be attributed to dissolution of evaporites (gypsum and halite), sulfide oxidation, and anthropogenic input of industrial activities, whereas NO3− in PC2 and Fe in PC3 and PC5 could be related to agricultural practices and inputs of domestic waste (Edmunds and Smedley, 2013; Xiao et al., 2016; Hou et al., 2020; Rashid et al., 2021) (see Supplementary Figure S2). The moderate factors of variables in PC3, PC4, and PC5 could be associated with anthropogenic activities leading to influence the hydrochemical characterization of groundwater aquifers (Fan et al., 2014; Zhang et al., 2019; Huang et al., 2020a; Zhang M. et al., 2020).
Pearson’s correlation analysis was applied to identify the significant correlations among the groundwater variables (Supplementary Table S2). Significant correlation pairs were identified between numerous variables of groundwater sources. The EC, TDS, and Alkalinity had significant positive correlations with the rest of the parameters. The Na+ had significant correlations with Cl−, SO42−, HCO3−, NO3−, and As in the groundwater of Ghotki and Nawab Shah, indicating that these elements possibly originated from the same sources. Na+ had positive correlations with Cl− (r = 0.51) and HCO3− (r = 0.48). Furthermore, K+ had significant positive correlations with Mg+ (r = 0.69), Ca+ (r = 0.75), and HCO3− (r = 0.39). In addition, Mg+−HCO3−, Ca+−Mg+, Cl−−SO42−, Cl−−HCO3−, and SO42−−HCO3− were significantly, positively correlated indicating that these hydrochemical parameters could be originated from the same sources in the study area of the Ghotki and Nawab Shah. The significant correlation between cations and anions demonstrates the same source of composition in the groundwater aquifer that primarily originates from the ions-exchange process during the water-rock interactions and chemical weathering (Mushtaq et al., 2021). The high concentration of these major ions and their correlations in the present study reflect the dissolution of evaporitic minerals in the regions (Singh and Mukherjee, 2015). Furthermore, ionic composition increases in the groundwater due to the dissolution of evaporite minerals and sulfate salts (Alaya et al., 2014). Arsenic had also significant positive correlations with major ions including Na+ (r = 0.55), K+ (r = 0.59), Mg+ (r = 0.55), Ca+ (r = 0.57), SO42− (r = 0.61), and HCO3 (r = 0.79) in the groundwater of Ghotki and Nawab Shah. These significant moderate correlations indicate the competitive effect of ions subjected to As desorption by the ions exchange process (SunBaek and XiaoGuang, 2004). The correlation of observed parameters was further supported by PCA results in the groundwater of Ghotki and Nawab Shah. Hence, the positive correlation values proposed that variables have a strong effect on each other, while inverse correlation shows that groundwater variables are not depending on each other (Supplementary Table S2).
Arsenic Mechanism in Groundwater of Ghotki and Nawab Shah
Overall, Arsenic concentrations in groundwater of both districts were varied from low to high enrichments level, as shown in Figure 5. The results showed that 27% of the groundwater samples exceeded the WHO permissible limit of As (10 μg/L) in Ghotki, whereas 65% of the groundwater samples were substantially above the WHO limit in Nawab Shah. In contrast, the groundwater samples (73% and 35%) had low enrichment contents and didn’t exceed the WHO permissible limit in the Ghotki and Nawab Shah, respectively. The arsenic concentrations in the present study were considerably higher in the Nawab Shah than in the Ghotki district (Figure 5).
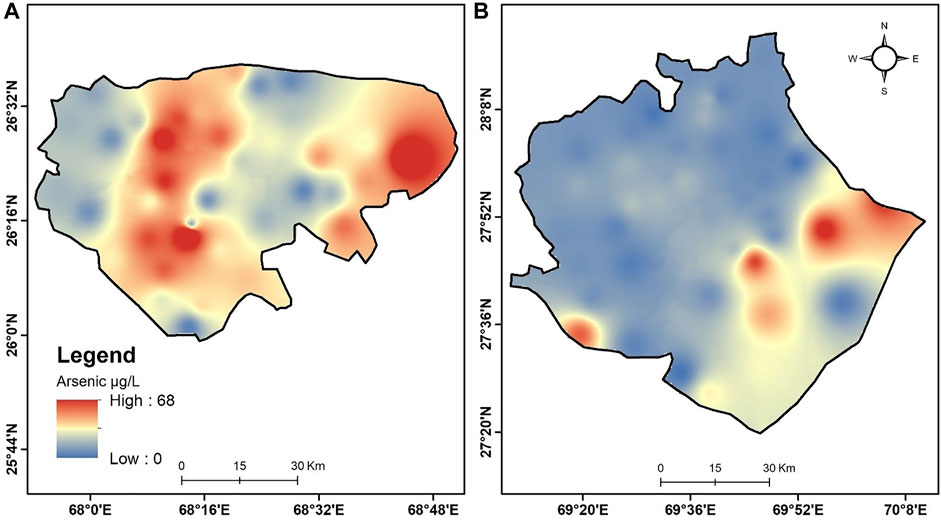
FIGURE 5. Arsenic distribution level (low to high) in the study area of the (A) Nawab Shah, and (B) Ghotki.
An increase in concentrations of HCO3, SO42−, Na+, K+, Mg+, Ca+, TDS, and EC were subjected resulting in moderate to high correlations between As and aforementioned parameters in the groundwater of both districts (Supplementary Table S2). This demonstrated that Arsenic concentrations were greatly influenced by the ions-exchange process and its adsorption with ions, high TDS and EC contents in the study area. For instance, cation such as Ca+ was previously reported to potentially compete with As adsorption under the impact of ion reaction on mineral surfaces (Xie et al., 2008). Thus, As desorption can either be enhanced by high competing ionic compositions (HCO3, SO42−, Na+, K+, Mg+, and Ca+). The results were found in agreement with the previous studies of Brahman et al. (2016) and Shahab et al. (2019) with high As concentration in the lower Indus plain of Sindh province, Pakistan. In addition, soil salinization and subsurface environmental conditions had a potential cause of variation in As enrichment in groundwater under high pH conditions (Li et al., 2020). In the present study, the low correlation of As with pH could be related to alkaline desorption that may influence As release into the groundwater. Moreover, it has also been reported that the aquifers of lower Indus regions are naturally alluvial, composed of silt, sand, and gravel containing an elevated level of As in Sindh province (Shahab et al., 2019). A high rate of evaporation, i.e., 74–80% of the groundwater is highly evaporated in Sindh province (Van Steenbergen et al., 2015), resulting in an upsurge of salinity and ions which could probably boost up As enrichment in the groundwater (Yu et al., 2015). No statistically significant correlation of As with F−, NO3−, and Fe was observed with no impacts in the groundwater samples of Ghotki and Nawab Shah since their concentrations were very low in almost all the samples.
Groundwater Quality Assessment for Drinking Purposes
WQI is one of the most crucial methods to quantify the overall drinking water quality of groundwater (Sadat-Noori et al., 2014). The WQI has been broadly used to estimate the inclusive effects of hydrochemical variables on drinking water quality (Hasan and Rai, 2020). The WQI signifies the groundwater quality on the basis of considerable standards used for drinking purposes, recommended by the WHO (2011).
The WQI results based on groundwater quality categories are presented in Table 3. The WQI values for Ghotki range from 35.15 to 231.46. In district Ghotki, 32 samples (73.68%) are considered “excellent”, 5 samples (13.74%) were estimated as “good” while 3 samples (9.27%) were classified as “poor”, whereas 2 samples (2.15%) were classified as “very poor”, and 1 sample (1.16%) was estimated as “water unsuitable for drinking”. In district Nawab Shah, 23 samples (65.72%) are considered “excellent”, 7 samples (20.67%) were estimated as “good” while 3 samples (8.66%) were classified as “poor”, whereas 2 samples (4.42%) were classified as “very poor”, and 1 sample (0.53%) was estimated as “water unsuitable for drinking”. The results showed that 70% of groundwater samples presented the Rank 1 level (excellent category), as shown in Figure 6, and were found well consistent with recommended drinking water WHO standard. Therefore, it has been observed that the majority of groundwater samples of the Ghotki and Nawab Shah were classified in the excellent category. Although a comparison of the water quality evaluation results between the Ghotki and Nawab Shah showed little difference.
Human Health Risk Assessment
The chronic daily intake (CDI), adverse non-carcinogenic (HQ), and carcinogenic risk (CR) result from As exposure in study areas of the Ghotki and Nawab Shah is presented in Table 4. Based on statistical results, the As exceeded the permissible limit for drinking purposes, hence resulting in non-carcinogenic health risks. In this study, the non-carcinogenic risk as hazard quotient (HQ) and carcinogenic risk (CR) for an individual by ingestion of arsenic for adults and children were compared for the Ghotki and Nawab Shah.
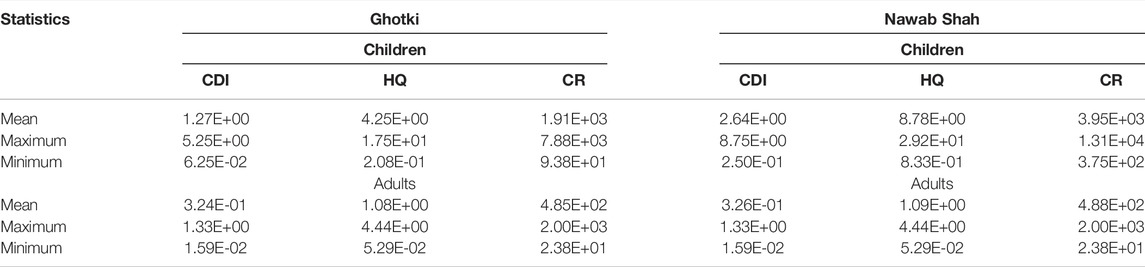
TABLE 4. Health risk assessment of arsenic (As) in groundwater sources of the Ghotki and Nawab Shah districts.
For non-carcinogenic risk, the mean HQ values of As were observed to be (>1) in groundwater samples of the Ghotki and Nawab Shah. A high mean HQ value (8.78E+00) was recorded for children in the Nawab Shah, while a low mean HQ value (5.29E−02) was recorded for the adults in the Ghotki and Nawab Shah. Recently, some studies highlighted high health risks related to ingestion of As-contaminated water in different regions of Pakistan (Bibi et al., 2021; Mushtaq et al., 2021; Ullah et al., 2021). Likewise, the order of CR values of As calculated for Ghotki and Nawab Shah had a similar trend as recorded for HQ (Table 4). If CR is greater than 1 × 10–4, the local population could be considered at cancer risk. The CR of As was highly observed for the groundwater of the Ghotki with mean values of 4.85E+02 and 1.91E+03 for adults and children respectively, whereas in the Nawab Shah high mean values of 4.88E+02 and 3.95E+03 were recorded for adults and children, respectively. Hence, average CR values of As in the present study were significantly higher in drinking water samples of Ghotki and Nawab Shah than the maximum threshold CR value (i.e., 1 × 10–4), recommended by USEPA (2011). However, previously the carcinogenic risk was mostly resulted from high As concentration in the groundwater (Ali et al., 2019a). The health risk results exhibit that groundwater can pose potential health risks in the local inhabitants of the Ghotki and Nawab Shah areas. As a result, it has been substantially noted that drinking water sources of the Ghotki and Nawab Shah can pose high non-carcinogenic and carcinogenic risks to the local population of the study areas due to ingestion of As-contaminated water.
Conclusion
• It is concluded that groundwater samples of the Ghotki were more contaminated based on a high concentration of arsenic and other parameters in comparison with the Nawab Shah.
• Hydrochemistry of the groundwater samples by Piper plot revealed mixed CaMgCl and CaCl type. Gibbs plots revealed that rock dominance has a major impact on groundwater chemistry with little contribution to evaporation in both districts, signifying the evaporation importance in the shallow groundwater depth zone. Carbonate dissolution implies a substantial influence on the hydrochemistry evolution of groundwater in the study area. Consequently, the hydrogeochemistry of the groundwater is highly affected by water-rock interaction, evaporation, carbonate weathering, and anthropogenic sources in the study area.
• Furthermore, geochemical modeling of saturation indices showed that most of the groundwater in the Ghotki and Nawab Shah were oversaturated relative to dolomite and calcite minerals, which implies the calcite/dolomite dissolution and carbonate minerals. while gypsum and halite had negative values, indicating a mineral unsaturated state in groundwater and inclination in continuous dissolution.
• According to WQI results, the majority of the studied water samples were observed in excellent water quality for both the Ghotki and Nawab Shah.
• The health risk assessment results showed that children are more susceptible to high HQ and CR risk of As than the adults in the studied regions of Ghotki and Nawab Shah. The present study provides significant information on the context of hydrochemistry and arsenic contamination in the groundwater that needs to be monitored for sustainable management of drinking water quality for local inhabitants.
Data Availability Statement
The raw data supporting the conclusions of this article will be made available by the authors, without undue reservation.
Author Contributions
JG: conceptualization, methodology, writing original draft, ZU: methodology, JN: software and validation, JI: validation, MW: software and result analysis, AA: reviewing and editing, MA: visualization, and funding acquisition, IP: formal analysis and investigation, HM: methodology and formal analysis, MS: data curation. All authors have read and agreed to the published version of the manuscript.
Funding
This project was supported by the Researchers Supporting Project number (RSP-2021/191), King Saud University, Riyadh, Saudi Arabia.
Acknowledgments
The authors extend their appreciation to the Researchers Supporting Project number (RSP-2021/191), King Saud University, Riyadh, Saudi Arabia.
Conflict of Interest
The authors declare that the research was conducted in the absence of any commercial or financial relationships that could be construed as a potential conflict of interest.
Publisher’s Note
All claims expressed in this article are solely those of the authors and do not necessarily represent those of their affiliated organizations, or those of the publisher, the editors and the reviewers. Any product that may be evaluated in this article, or claim that may be made by its manufacturer, is not guaranteed or endorsed by the publisher.
Supplementary Material
The Supplementary Material for this article can be found online at: https://www.frontiersin.org/articles/10.3389/fenvs.2022.874464/full#supplementary-material
References
Adimalla, N. (2021). Application of the Entropy Weighted Water Quality Index (EWQI) and the Pollution Index of Groundwater (PIG) to Assess Groundwater Quality for Drinking Purposes: a Case Study in a Rural Area of Telangana State, India. Arch. Environ. Contam. Toxicol. 80 (1), 31–40. doi:10.1007/s00244-020-00800-4
Adimalla, N., and Qian, H. (2019). Groundwater Quality Evaluation Using Water Quality Index (WQI) for Drinking Purposes and Human Health Risk (HHR) Assessment in an Agricultural Region of Nanganur, South India. Ecotoxicol. Environ. Saf. 176, 153–161. doi:10.1016/j.ecoenv.2019.03.066
Alaya, M. B., Saidi, S., Zemni, T., and Zargouni, F. (2014). Suitability Assessment of Deep Groundwater for Drinking and Irrigation Use in the Djeffara Aquifers (Northern Gabes, South-Eastern Tunisia). Environ. earth Sci. Earth Sci. 7171 (88), 33873387–34213421. doi:10.1007/s12665-013-2729-9
Ali, W., Aslam, M. W., Feng, C., Junaid, M., Ali, K., Li, S., et al. (2019a). Unraveling Prevalence and Public Health Risks of Arsenic, Uranium and Co-occurring Trace Metals in Groundwater along Riverine Ecosystem in Sindh and Punjab, Pakistan. Environ. Geochem. Health 41 (5), 2223–2238. doi:10.1007/s10653-019-00278-7
Ali, W., Mushtaq, N., Javed, T., Zhang, H., Ali, K., Rasool, A., et al. (2019b). Vertical Mixing with Return Irrigation Water the Cause of Arsenic Enrichment in Groundwater of District Larkana Sindh, Pakistan. Environ. Pollut. 245, 77–88. doi:10.1016/j.envpol.2018.10.103
Avtar, R., Kumar, P., Singh, C. K., Sahu, N., Verma, R. L., Thakur, J. K., et al. (2013). Hydrogeochemical Assessment of Groundwater Quality of Bundelkhand, India Using Statistical Approach. Water Qual. Expo. Health 5 (3), 105–115. doi:10.1007/s12403-013-0094-2
Bibi, I., Shahid, M., Niazi, N. K., Younas, F., Naqvi, S. R., et al. (2021). Hydrogeochemical and Health Risk Evaluation of Arsenic in Shallow and Deep Aquifers along the Different Floodplains of Punjab, Pakistan. J. Hazard. Mater. 402, 124074. doi:10.1016/j.jhazmat.2020.124074
Boonkaewwan, S., Sonthiphand, P., and Chotpantarat, S. (2021). Mechanisms of Arsenic Contamination Associated with Hydrochemical Characteristics in Coastal Alluvial Aquifers Using Multivariate Statistical Technique and Hydrogeochemical Modeling: a Case Study in Rayong Province, Eastern Thailand. Environ. Geochem. Health 43 (1), 537–566. doi:10.1007/s10653-020-00728-7
Brahman, K. D., Kazi, T. G., Afridi, H. I., Arain, S. S., Kazi, A. G., Talpur, F. N., et al. (2016). Toxic Risk Assessment of Arsenic in Males through Drinking Water in Tharparkar Region of Sindh, Pakistan. Biol. Trace Elem. Res. 172 (1), 61–71. doi:10.1007/s12011-015-0567-1
Caussy (2005). A Field Guide for Detection, Management and Surveillance of Arsenicosis Cases. South-East Asia: World Health Organization.
Chen, X., Zeng, X. C., Kawa, Y. K., Wu, W., Zhu, X., Ullah, Z., et al. (2020). Microbial Reactions and Environmental Factors Affecting the Dissolution and Release of Arsenic in the Severely Contaminated Soils under Anaerobic or Aerobic Conditions. Ecotoxicol. Environ. Saf. 189, 109946. doi:10.1016/j.ecoenv.2019.109946
Chung, S. Y., Rajesh, R., and Venkatramanan, S. (2019). Chapter 24 - Evaluation of Heavy-Metal Contamination in Groundwater Using Hydrogeochemical and Multivariate Statistical Analyses. South Korea: Elsevier.
Chung, S. Y., Senapathi, V., Sekar, S., and Kim, T. H. (2018). Time Series Analyses of Hydrological Parameter Variations and Their Correlations at a Coastal Area in Busan, South Korea. Hydrogeol. J. 26 (6), 1875–1885. doi:10.1007/s10040-018-1739-9
Çiner, F., Sunkari, E. D., and Şenbaş, B. A. (2021). Geochemical and Multivariate Statistical Evaluation of Trace Elements in Groundwater of Niğde Municipality, South-Central Turkey: Implications for Arsenic Contamination and Human Health Risks Assessment. Arch. Environ. Contam. Toxicol. 80 (1), 164–182. doi:10.1007/s00244-020-00759-2
Ruiz-Agudo, E., Putnis, C. V., Rodriguez-Navarro, C., and Putnis, A. (2011). Effect of pH on calcite growth at constant aCa2+/aCO32-ratio and supersaturation. Geochim. Cosmochim. Acta 75 (1), 284–296. doi:10.1016/j.gca.2010.09.034
Dashora, M., Kumar, A., Kumar, S., Kumar, P., Kumar, A., and Singh, C. K. (2022). Geochemical Assessment of Groundwater in a Desertic Region of India Using Chemometric Analysis and Entropy Water Quality Index (EWQI). Nat. Hazards 112, 747–782. doi:10.1007/s11069-021-05204-8
Dehghanzadeh, R., Safavy Hir, N., Shamsy Sis, J., and Taghipour, H. (2015). Integrated Assessment of Spatial and Temporal Variations of Groundwater Quality in the Eastern Area of Urmia Salt Lake Basin Using Multivariate Statistical Analysis. Water Resour. Manage 29 (4), 1351–1364. doi:10.1007/s11269-014-0877-7
Edmunds, W. M., and Smedley, P. L. (2013). Essentials of Medical Geology. Berlin: Springer, 311–336.
Fan, B.-L., Zhao, Z.-Q., Tao, F.-X., Liu, B.-J., Tao, Z.-H., Gao, S., et al. (2014). Characteristics of Carbonate, Evaporite and Silicate Weathering in Huanghe River Basin: A Comparison Among the Upstream, Midstream and Downstream. J. Asian Earth Sci. 96, 17–26. doi:10.1016/j.jseaes.2014.09.005
Gao, Y., Qian, H., Ren, W., Wang, H., Liu, F., and Yang, F. (2020). Hydrogeochemical Characterization and Quality Assessment of Groundwater Based on Integrated-Weight Water Quality Index in a Concentrated Urban Area. J. Clean. Prod. 260, 121006. doi:10.1016/j.jclepro.2020.121006
Ghosh, G., and Mukhopadhyay, D. K. (2019). Human Health Hazards Due to Arsenic and Fluoride Contamination in Drinking Water and Food Chain. Groundw. Dev. Manag., 351–369. doi:10.1007/978-3-319-75115-3_15
Gibbs, R. J. (1970). Mechanisms Controlling World Water Chemistry. Science 170170 (39623962), 10881088–10901090. doi:10.3390/w11081577
Haji, M., Karuppannan, S., Qin, D., Shube, H., and Kawo, N. S. (2021). Potential Human Health Risks Due to Groundwater Fluoride Contamination: A Case Study Using Multi-Techniques Approaches (GWQI, FPI, GIS, HHRA) in Bilate River Basin of Southern Main Ethiopian Rift, Ethiopia. Arch. Environ. Contam. Toxicol. 80 (1), 277–293. doi:10.1007/s00244-020-00802-2
Hasan, M. S. U., and Rai, A. K. (2020). Groundwater Quality Assessment in the Lower Ganga Basin Using Entropy Information Theory and GIS. J. Clean. Prod. 274, 123077. doi:10.1016/j.jclepro.2020.123077
Hou, Q., Zhang, Q., Huang, G., Liu, C., and Zhang, Y. (2020). Elevated Manganese Concentrations in Shallow Groundwater of Various Aquifers in a Rapidly Urbanized Delta, South China. Sci. Total Environ. 701, 134777. doi:10.1016/j.scitotenv.2019.134777
Huang, G., Liu, C., Sun, J., Zhang, M., Jing, J., and Li, L. (2018a). A Regional Scale Investigation on Factors Controlling the Groundwater Chemistry of Various Aquifers in a Rapidly Urbanized Area: A Case Study of the Pearl River Delta. Sci. Total Environ. 625, 510–518. doi:10.1016/j.scitotenv.2017.12.322
Huang, G., Zhang, M., Liu, C., Li, L., and Chen, Z. (2018b). Heavy Metal(loid)s and Organic Contaminants in Groundwater in the Pearl River Delta that Has Undergone Three Decades of Urbanization and Industrialization: Distributions, Sources, and Driving Forces. Sci. Total Environ. 635, 913–925. doi:10.1016/j.scitotenv.2018.04.210
Huang, G., Chen, Z., Liu, F., Sun, J., and Wang, J. (2014). Impact of Human Activity and Natural Processes on Groundwater Arsenic in an Urbanized Area (South China) Using Multivariate Statistical Techniques. Environ. Sci. Pollut. Res. 21 (22), 13043–13054. doi:10.1007/s11356-014-3269-x
Huang, G., Han, D., Song, J., Li, L., and Pei, L. (2022). A Sharp Contrasting Occurrence of Iron-Rich Groundwater In the Pearl River Delta During the Past Dozen Years (2006–2018): The Genesis and Mitigation Effect. Sci. Total Environ. 829, 154676. doi:10.1016/j.scitotenv.2022.154676
Huang, G., Liu, C., Li, L., Zhang, F., and Chen, Z. (2020a). Spatial Distribution and Origin of Shallow Groundwater Iodide in a Rapidly Urbanized Delta: A Case Study of the Pearl River Delta. J. Hydrology 585, 124860. doi:10.1016/j.jhydrol.2020.124860
Huang, G., Liu, C., Zhang, Y., and Chen, Z. (2020b). Groundwater Is Important for the Geochemical Cycling of Phosphorus in Rapidly Urbanized Areas: A Case Study in the Pearl River Delta. Environ. Pollut. 260, 114079. doi:10.1016/j.envpol.2020.114079
Huang, G., Sun, J., Zhang, Y., Chen, Z., and Liu, F. (2013). Impact of Anthropogenic and Natural Processes on the Evolution of Groundwater Chemistry in a Rapidly Urbanized Coastal Area, South China. Sci. Total Environ. 463-464, 209–221. doi:10.1016/j.scitotenv.2013.05.078
Iqbal, J., Su, C., Rashid, A., Yang, N., Baloch, M. Y. J., Talpur, S. A., et al. (2021). Hydrogeochemical Assessment of Groundwater and Suitability Analysis for Domestic and Agricultural Utility in Southern Punjab, Pakistan. Water 13 (24), 3589. doi:10.3390/w13243589
Ismail, A. H., Shareef, M. A., and Mahmood, W. (2018). Hydrochemical Characterization of Groundwater in Balad District, Salah Al-Din Governorate, Iraq. J. Groundw. Sci. Eng. 6 (4), 306. doi:10.19637/j.cnki.2305-7068.2018.04.006
Jehan, S., Khan, S., Khattak, S. A., Muhammad, S., Rashid, A., and Muhammad, N. (2019). Hydrochemical Properties of Drinking Water and Their Sources Apportionment of Pollution in Bajaur Agency, Pakistan. Measurement 139, 249–325. doi:10.1016/j.measurement.2019.02.090
Kaleem, M., Naseem, S., Bashir, E., Shahab, B., and Rafique, T. (2021). Discrete Geochemical Behavior of Sr and Ba in the Groundwater of Southern Mor Range, Balochistan, a Tracer for Igneous and Sedimentary Rocks Weathering and Related Environmental Issues. J. Appl. Geochem. 130, 104996. doi:10.1016/j.apgeochem.2021.104996
Khattak, J. A., Farooqi, A., Hussain, I., Kumar, A., Singh, C. K., Mailloux, B. J., et al. (2022). Groundwater Fluoride across the Punjab Plains of Pakistan and India: Distribution and Underlying Mechanisms. Sci. Total Environ. 806, 151353. doi:10.1016/j.scitotenv.2021.151353
Khuhawar, M. A., and Majidano, S. A. (2011). An Investigation of Quality of Groundwater of Taluka Nawabshah. Pak. J. Chem. 1 (2), 65–71. doi:10.1016/j.apgeochem.2021.104996
Kimbi, S. B., Kimbi, S. B., Nozaki, S., Tomozawa, Y., Wang, K., Rusydi, A. F., et al. (2021). Impact of Citrus Agriculture on the Quality of Water Resource in a Small Steep Island, Seto Inland Sea, Japan. Geomate 20 (82), 109–114. doi:10.21660/2021.82.6166
Kumar, A., and Singh, C. K. (2020). Arsenic Enrichment in Groundwater and Associated Health Risk in Bari Doab Region of Indus Basin, Punjab, India. Environ. Pollut. 256, 113324. doi:10.1016/j.envpol.2019.113324
Kumar, A., and Singh, C. K. (2015). Characterization of Hydrogeochemical Processes and Fluoride Enrichment in Groundwater of South-Western Punjab. Water Qual. Expo. Health 7 (3), 373–387. doi:10.1007/s12403-015-0157-7
Kumaravel, S., Gurugnanam, B., Bagyaraj, M., Venkatesan, S., Suresh, M., Chidambaram, S., et al. (2014). Mapping of Groundwater Quality Using GIS Technique in the East Coast of Tamilnadu State and Pondicherry Union Territory, India. Magnes. (Mg) 2, 30–150. doi:10.14419/ijag.v2i2.2213
Li, H., and Hou, E. (2020). Groundwater Dynamic Response Mechanism and Quantity Vulnerability Assessment under the Influence of Human Activities. Environ. Sci. Pollut. Res. 27 (17), 22046–22064. doi:10.1007/s11356-020-08645-z
Li, Z., Yang, Q., Yang, Y., Xie, C., and Ma, H. (2020). Hydrogeochemical Controls on Arsenic Contamination Potential and Health Threat in an Intensive Agricultural Area, Northern China. Environ. Pollut. 256, 113455. doi:10.1007/s10661-020-08356-5
Liu, J., Gao, Z., Wang, Z., Xu, X., Su, Q., Wang, S., et al. (2020). Hydrogeochemical Processes and Suitability Assessment of Groundwater in the Jiaodong Peninsula, China. Environ. Monit. Assess. 192, 1–17. doi:10.1007/s10661-020-08356-5
Liu, J., Peng, Y., Li, C., Gao, Z., and Chen, S. (2021). Characterization of the Hydrochemistry of Water Resources of the Weibei Plain, Northern China, as Well as an Assessment of the Risk of High Groundwater Nitrate Levels to Human Health. Environ. Pollut. 268, 115947. doi:10.1016/j.envpol.2020.115947
Lkr, A., Singh, M. R., and Puro, N. (2020). Assessment of Water Quality Status of Doyang River, Nagaland, India, Using Water Quality Index. Appl. Water Sci. 10 (1), 1–13. doi:10.1007/s13201-019-1133-3
Loh, Y. S. A., Akurugu, B. A., Manu, E., and Aliou, A.-S. (2020). Assessment of Groundwater Quality and the Main Controls on its Hydrochemistry in Some Voltaian and Basement Aquifers, Northern Ghana. Groundw. Sustain. Dev. 10, 100296. doi:10.1016/j.gsd.2019.100296
Mallick, J., Talukdar, S., Alsubih, M., Ahmed, M., Islam, A. R. M. T., Shahfahad, M., et al. (2021a). Proposing Receiver Operating Characteristic-Based Sensitivity Analysis with Introducing Swarm Optimized Ensemble Learning Algorithms for Groundwater Potentiality Modelling in Asir Region, Saudi Arabia. Geocarto Int. 14 (3), 1–28. doi:10.1080/10106049.2021.1878291
Mallick, J., Singh, C. K., AlMesfer, M. K., Singh, V. P., and Alsubih, M. (2021b). Groundwater Quality Studies in the Kingdom of Saudi Arabia: Prevalent Research and Management Dimensions. Water 13 (9), 1266. doi:10.14419/ijag.v2i2.2213
Mani, S. A., Naing, L., John, J., and Samsudin, A. R. (2008). Comparison of Two Methods of Dental Age Estimation in 7-15-Year-Old Malays. Int. J. Paediatr. Dent. 18 (5), 380–388. doi:10.1111/j.1365-263X.2007.00890.x
Mohamed, A. K., Liu, D., Mohamed, M. A., and Song, K. (2018). Groundwater Quality Assessment of the Quaternary Unconsolidated Sedimentary Basin Near the Pi River Using Fuzzy Evaluation Technique. Appl. Water Sci. 8 (2), 1–12. doi:10.1111/j.1365-263X.2007.00890.x
Mukherjee, A., Gupta, S., Coomar, P., Fryar, A. E., Guillot, S., Verma, S., et al. (2019). Plate Tectonics Influence on Geogenic Arsenic Cycling: From Primary Sources to Global Groundwater Enrichment. Sci. Total Environ. 683, 793–807. doi:10.1016/j.scitotenv.2019.04.255
Mushtaq, N., Masood, N., Khattak, J. A., Hussain, I., Khan, Q., and Farooqi, A. (2021). Health Risk Assessment and Source Identification of Groundwater Arsenic Contamination Using Agglomerative Hierarchical Cluster Analysis in Selected Sites from Upper Eastern Parts of Punjab Province, Pakistan. Hum. Ecol. Risk Assess. Int. J. 27 (4), 999–1018. doi:10.1080/10807039.2020.1794787
Mwiathi, N. F., Gao, X., Li, C., and Rashid, A. (2022). The Occurrence of Geogenic Fluoride in Shallow Aquifers of Kenya Rift Valley and its Implications in Groundwater Management. Ecotoxicol. Environ. Saf. 229, 113046. doi:10.1016/j.ecoenv.2021.113046
Naseem, S., and McArthur, J. M. (2018). Arsenic and Other Water-Quality Issues Affecting Groundwater, Indus Alluvial Plain, Pakistan. Hydrol. Process. 32 (9), 1235–1253. doi:10.1002/hyp.11489
Natasha, B. I., Bibi, I., Shahid, M., Niazi, N. K., Younas, F., Naqvi, S. R., et al. (2021). Hydrogeochemical and Health Risk Evaluation of Arsenic in Shallow and Deep Aquifers along the Different Floodplains of Punjab, Pakistan. J. Hazard. Mater. 402, 124074. doi:10.1016/j.jhazmat.2020.124074
Natasha, N., Shahid, M., Imran, M., Khalid, S., Murtaza, B., Niazi, N. K., et al. (2020). Arsenic Environmental Contamination Status in South Asia. Environ. Sci., 13–39. doi:10.1007/978-981-13-8587-2_2
Noor, S., Rashid, A., Javed, A., Khattak, J. A., and Farooqi, A. (2022). Hydrogeological Properties, Sources Provenance, and Health Risk Exposure of Fluoride in the Groundwater of Batkhela, Pakistan. Environ. Technol. Innovation 25, 102239. doi:10.1016/j.eti.2021.102239
Parkhurst, D. L., and Appelo, C. (2013). Description of Input and Examples for PHREEQC Version 3: A Computer Program for Speciation, Batch-Reaction, One-Dimensional Transport, and Inverse Geochemical Calculations. Denver, Colorado: US Geological Survey.
Piper, A. M. (1944). A Graphic Procedure in the Geochemical Interpretation of Water-Analyses. Trans. AGU 25 (6), 914–928. doi:10.1029/tr025i006p00914
Rashid, A., Ayub, M., Javed, A., Khan, S., Gao, X., Li, C., et al. (2021). Potentially Harmful Metals, and Health Risk Evaluation in Groundwater of Mardan, Pakistan: Application of Geostatistical Approach and Geographic Information System. Geosci. Front. 12 (3), 101128. doi:10.1016/j.gsf.2020.12.009
Rashid, A., Farooqi, A., Gao, X., Zahir, S., Noor, S., and Khattak, J. A. (2020). Geochemical Modeling, Source Apportionment, Health Risk Exposure and Control of Higher Fluoride in Groundwater of Sub-district Dargai, Pakistan. Chemosphere 243, 125409. doi:10.1016/j.chemosphere.2019.125409
Rashid, A., Guan, D.-X., Farooqi, A., Khan, S., Zahir, S., Jehan, S., et al. (2018). Fluoride Prevalence in Groundwater Around a Fluorite Mining Area in the Flood Plain of the River Swat, Pakistan. Sci. Total Environ. 635, 203–215. doi:10.1016/j.scitotenv.2018.04.064
Rashid, A., Khattak, S. A., Ali, L., Zaib, M., Jehan, S., Ayub, M., et al. (2019). Geochemical Profile and Source Identification of Surface and Groundwater Pollution of District Chitral, Northern Pakistan. Microchem. J. 145, 1058–1065. doi:10.1016/j.scitotenv.2018.04.064
Rehman, F., Cheema, T., Azeem, T., Naseem, A. A., Khan, I., Iqbal, N., et al. (2020). Groundwater Quality and Potential Health Risks Caused by Arsenic (As) in Bhakkar, Pakistan. Environ. Earth Sci. 79 (24), 1–13. doi:10.1007/s12665-020-09270-2
Roy, P. D., Selvam, S., Venkatramanan, S., Logesh, N., Lakshumanan, C., and Sánchez-Zavala, J. L. (2021). Identification of Sources and Groundwater Recharge Zones from Hydrochemistry and Stable Isotopes of an Agriculture-Based Paleo-Lacustrine Basin of Drought-Prone Northeast Mexico. Geochemistry 18(2), 125742. doi:10.1016/j.chemer.2021.125742
Sadat-Noori, S. M., Ebrahimi, K., and Liaghat, A. M. (2014). Groundwater Quality Assessment Using the Water Quality Index and GIS in Saveh-Nobaran Aquifer, Iran. Environ. Earth Sci. 71 (9), 3827–3843. doi:10.1007/s12665-013-2770-8
Saeed, M., Rehman, M. Y. A., Farooqi, A., and Malik, R. N. (2021). Arsenic and Fluoride Co-exposure through Drinking Water and Their Impacts on Intelligence and Oxidative Stress Among Rural School-Aged Children of Lahore and Kasur Districts, Pakistan. Environ. Geochem. Health 4, 1–23. doi:10.1007/s10653-021-01141-4
Selvakumar, S., Ramkumar, K., Chandrasekar, N., Magesh, N. S., and Kaliraj, S. (2017). Groundwater Quality and its Suitability for Drinking and Irrigational Use in the Southern Tiruchirappalli District, Tamil Nadu, India. Appl. Water Sci. 7 (1), 411–420. doi:10.1007/s13201-014-0256-9
Selvam, S., Singaraja, C., Venkatramanan, S., and Chung, S. Y. (2018). Geochemical Appraisal of Groundwater Quality in Ottapidaram Taluk, Thoothukudi District, Tamil Nadu Using Graphical and Numerical Method. J. Geol. Soc. India 92 (3), 313–320. doi:10.1007/s12594-018-1013-8
Shah, A. H., Shahid, M., Khalid, S., Natasha, Z., Shabbir, Z., Bakhat, H. F., et al. (2020). Assessment of Arsenic Exposure by Drinking Well Water and Associated Carcinogenic Risk in Peri-Urban Areas of Vehari, Pakistan. Environ. Geochem. Health. 42 (1), 121–133. doi:10.1007/s10653-019-00306-6
Shahab, A., Qi, S., and Zaheer, M. (2019). Arsenic Contamination, Subsequent Water Toxicity, and Associated Public Health Risks in the Lower Indus Plain, Sindh Province, Pakistan. Environ. Sci. Pollut. Res. 26 (30), 30642–30662. doi:10.1007/s11356-018-2320-8
Siddique, J., Menggui, J., Shah, M. H., Shahab, A., Rehman, F., and Rasool, U. (2020). Integrated Approach to Hydrogeochemical Appraisal and Quality Assessment of Groundwater from Sargodha District, Pakistan. Geofluids 2020, 1–15. doi:10.1155/2020/6621038
Singh, C. K., Kumar, A., Shashtri, S., Kumar, A., Kumar, P., and Mallick, J. (2017). Multivariate Statistical Analysis and Geochemical Modeling for Geochemical Assessment of Groundwater of Delhi, India. J. Geochem. Explor. 175, 59–71. doi:10.1016/j.gexplo.2017.01.001
Singh, C. K., and Mukherjee, S. (2015). Aqueous Geochemistry of Fluoride Enriched Groundwater in Arid Part of Western India. Environ. Sci. Pollut. Res. 22 (4), 2668–2678. doi:10.1007/s11356-014-3504-5
Singh, C. K., Shashtri, S., and Mukherjee, S. (2011a). Integrating Multivariate Statistical Analysis with GIS for Geochemical Assessment of Groundwater Quality in Shiwaliks of Punjab, India. Environ. Earth Sci. 62 (7), 1387–1405. doi:10.1007/s12665-010-0625-0
Singh, C. K., Shashtri, S., Mukherjee, S., Kumari, R., Avatar, R., Singh, A., et al. (2011b). Application of GWQI to Assess Effect of Land Use Change on Groundwater Quality in Lower Shiwaliks of Punjab: Remote Sensing and GIS Based Approach. Water Resour. Manage 25 (7), 1881–1898. doi:10.1007/s12665-010-0625-0
Srinivasamoorthy, K., Chidambaram, S., Prasanna, M. V., Vasanthavihar, M., Peter, J., and Anandhan, P. (2008). Identification of Major Sources Controlling Groundwater Chemistry from a Hard Rock Terrain - A Case Study from Mettur Taluk, Salem District, Tamil Nadu, India. J. Earth Syst. Sci. 117 (1), 49–58. doi:10.1007/s12040-008-0012-3
SunBaek, B., and XiaoGuang, M. (2004). A Review of Arsenic Interactions with Anions and Iron Hydroxides. Environ. Eng. Res. 9 (4), 184–192. doi:10.4491/eer.2004.9.4.184
Tabassum, R. A., Shahid, M., Dumat, C., Niazi, N. K., Khalid, S., Shah, N. S., et al. (2019). Health Risk Assessment of Drinking Arsenic-Containing Groundwater in Hasilpur, Pakistan: Effect of Sampling Area, Depth, and Source. Environ. Sci. Pollut. Res. 26 (20), 20018–20029. doi:10.1007/s11356-018-1276-z
Talib, M., Tang, Z., Shahab, A., Siddique, J., Faheem, M., and Fatima, M. (2019). Hydrogeochemical Characterization and Suitability Assessment of Groundwater: a Case Study in Central Sindh, Pakistan. Int. J. Environ. Res. Public Health 16 (5), 886. doi:10.3390/ijerph16050886
Talpur, S. A., Noonari, T. M., Rashid, A., Ahmed, A., Jat Baloch, M. Y., Talpur, H. A., et al. (2020). Hydrogeochemical Signatures and Suitability Assessment of Groundwater with Elevated Fluoride in Unconfined Aquifers Badin District, Sindh, Pakistan. SN Appl. Sci. 2 (6), 11–1515. doi:10.1007/s42452-020-2821-1
Tweed, S., Massuel, S., Seidel, J. L., Chhuon, K., Lun, S., Eang, K. E., et al. (2020). Seasonal Influences on Groundwater Arsenic Concentrations in the Irrigated Region of the Cambodian Mekong Delta. Sci. Total Environ. 728, 138598. doi:10.1016/j.scitotenv.2020.138598
Ullah, Z., Talib, M. A., Rashid, A., Ghani, J., Shahab, A., Irfan, M., et al. (2021). Hydrogeochemical Investigation of Elevated Arsenic Based on Entropy Modeling, in the Aquifers of District Sanghar, Sindh, Pakistan. Water 13 (23), 3477. doi:10.3390/w13233477
USEPA (2005). Quick Guide to Drinking Water Sample Collection. ?Colorado: USR Laboratory and Editors.
USEPA (2011). Exposure Factors Handbook 2011 Edition (Final). Washington, DC: US Environmental Protection.
van Geen, A., Farooqi, A., Kumar, A., Khattak, J. A., Mushtaq, N., Hussain, I., et al. (2019). Field Testing of over 30,000 Wells for Arsenic across 400 Villages of the Punjab Plains of Pakistan and India: Implications for Prioritizing Mitigation. Sci. Total Environ. 654, 1358–1363. doi:10.1016/j.scitotenv.2018.11.201
Van Steenbergen, F., Basharat, M., and Lashari, B. (2015). Key Challenges and Opportunities for Conjunctive Management of Surface and Groundwater in Mega-Irrigation Systems: Lower Indus, Pakistan. Resources 4 (4), 831–856. doi:10.3390/resources4040831
Vithanage, M., Herath, I., Joseph, S., Bundschuh, J., Bolan, N., Ok, Y. S., et al. (2017). Interaction of Arsenic with Biochar in Soil and Water: a Critical Review. Carbon 113, 219–230. doi:10.1016/j.carbon.2016.11.032
Wei, Y., Jia, C., Lan, Y., Hou, X., Zuo, J., Li, J., et al. (2019). The Association of Tryptophan and Phenylalanine Are Associated with Arsenic-Induced Skin Lesions in a Chinese Population Chronically Exposed to Arsenic via Drinking Water: a Case-Control Study. BMJ open 9 (10), e025336. doi:10.1136/bmjopen-2018-025336
Xiao, J., Zhang, F., and Jin, Z. (2016). Spatial Characteristics and Controlling Factors of Chemical Weathering of Loess in the Dry Season in the Middle Loess Plateau, China. Hydrol. Process. Process. 3030 (2525), 48554855–48694869. doi:10.1002/hyp.10959
Xie, X., Wang, Y., Su, C., Liu, H., Duan, M., and Xie, Z. (2008). Arsenic Mobilization in Shallow Aquifers of Datong Basin: Hydrochemical and Mineralogical Evidences. J. Geochem. Explor. 98 (3), 107–115. doi:10.1016/j.gexplo.2008.01.002
Yang, J., Ye, M., Tang, Z., Jiao, T., Song, X., Pei, Y., et al. (2020). Using Cluster Analysis for Understanding Spatial and Temporal Patterns and Controlling Factors of Groundwater Geochemistry in a Regional Aquifer. J. Geochem. Explor. 98 (3), 107–115. doi:10.1016/j.gexplo.2008.01.002
Yu, Q., Wang, Y., Xie, X., Currell, M., Pi, K., and Yu, M. (2015). Effects of Short-Term Flooding on Arsenic Transport in Groundwater System: A Case Study of the Datong Basin. J. Geochem. Explor. 158, 1–9. doi:10.1016/j.gexplo.2015.05.015
Yu, Z., Wu, G., Li, F., Huang, J., Xiao, X., and Liu, K. (2021). Small-catchment Perspective on Chemical Weathering and its Controlling Factors in the Nam Co Basin, Central Tibetan Plateau. J. Hydrology 598, 126315. doi:10.1016/j.jhydrol.2021.126315
Zaki, S. R., Redwan, M., Masoud, A. M., and Abdel Moneim, A. A. (2019). Chemical Characteristics and Assessment of Groundwater Quality in Halayieb Area, Southeastern Part of the Eastern Desert, Egypt. Geosci. J. 23 (1), 149–164. doi:10.1007/s12303-018-0020-5
Zhang, F., Huang, G., Hou, Q., Liu, C., Zhang, Y., and Zhang, Q. (2019). Groundwater Quality in the Pearl River Delta after the Rapid Expansion of Industrialization and Urbanization: Distributions, Main Impact Indicators, and Driving Forces. J. Hydrology 577, 124004. doi:10.1016/j.jhydrol.2019.124004
Zhang, M., Huang, G., Liu, C., Zhang, Y., Chen, Z., and Wang, J. (2020). Distributions and Origins of Nitrate, Nitrite, and Ammonium in Various Aquifers in an Urbanized Coastal Area, South China. J. Hydrology 582, 124528. doi:10.1016/j.jhydrol.2019.124528
Zhang, Q., Xu, P., Qian, H., and Yang, F. (2020). Hydrogeochemistry and Fluoride Contamination in Jiaokou Irrigation District, Central China: Assessment Based on Multivariate Statistical Approach and Human Health Risk. Sci. Total Environ. 741, 140460. doi:10.1016/j.scitotenv.2020.140460
Keywords: groundwater, arsenic, geochemical modeling, water quality index, health risk, indus plain
Citation: Ghani J, Ullah Z, Nawab J, Iqbal J, Waqas M, Ali A, Almutairi MH, Peluso I, Mohamed HRH and Shah M (2022) Hydrogeochemical Characterization, and Suitability Assessment of Drinking Groundwater: Application of Geostatistical Approach and Geographic Information System. Front. Environ. Sci. 10:874464. doi: 10.3389/fenvs.2022.874464
Received: 12 February 2022; Accepted: 17 June 2022;
Published: 14 July 2022.
Edited by:
Chuan Wu, Central South University, ChinaReviewed by:
Chander Kumar Singh, TERI School of Advanced Studies (TERI SAS), IndiaGuanxing Huang, Chinese Academy of Geological Sciences, China
Abdur Rashid, Quaid-i-Azam University, Pakistan
Copyright © 2022 Ghani, Ullah, Nawab, Iqbal, Waqas, Ali, Almutairi, Peluso, Mohamed and Shah. This is an open-access article distributed under the terms of the Creative Commons Attribution License (CC BY). The use, distribution or reproduction in other forums is permitted, provided the original author(s) and the copyright owner(s) are credited and that the original publication in this journal is cited, in accordance with accepted academic practice. No use, distribution or reproduction is permitted which does not comply with these terms.
*Correspondence: Zahid Ullah, MjIwMTg5MDA0OEBjdWcuZWR1LmNu