- 1Departamento de Geología, Universidad de Sonora, Hermosillo, Mexico
- 2Departamento de Investigación en Física, Universidad de Sonora, Hermosillo, Mexico
- 3Environment and Climate Change Canada, Subdury, ON, Canada
- 4Departamento de Ciencias del Agua y Medio Ambiente, Instituto Tecnológico de Sonora, Ciudad Obregón, Mexico
- 5Programa de Doctorado en Nanotecnología, Universidad de Sonora, Hermosillo, Mexico
- 6Departamento de Ciencias Químico-Biológicas, Universidad de Sonora, Hermosillo, Mexico
- 7Colección Nacional de Ácaros, Instituto de Biología, Universidad Nacional Autónoma de, Mexico city, Mexico
Despite the documented effects on human and animal health, particles smaller than 0.1 µm in diameter found in soils, sediments, and the atmosphere remain unregulated. Yet, cerium and titanium oxide nanoparticles associated with traffic increase mortality, cause behavioral changes, and inhibit the growth in amphibians. Mites of the genus Hannemania spend their early stages in the soil before becoming exclusive parasites of amphibians. Unlike other mites, Hannemania is found inside the epidermis of amphibians, thus facilitating the intake of particles, and leading to direct and chronic exposure. To better understand this exposure path, we sampled amphibians hosting mites in a river potentially polluted by traffic sources. Particles collected from mites were studied by scanning electron microscopy and Raman spectroscopy while sediment samples were analyzed for total metal content by portable X-ray fluorescence. Our results indicate that sediment samples showed significant correlations between elements (Zr, Mn, Ti, Nb, Fe) often associated with components in catalytic converters and a level of Zr that exceeded the local geochemical background, thus suggesting an anthropic origin. Furthermore, particles adhered to mites exhibited the characteristic Raman vibrational modes of ceria (CeO2, 465 cm−1), ceria-zirconia (CeO2-ZrO2, 149, 251, and 314 cm−1), and rutile (TiO2, 602 cm−1), pointing out to the deterioration of catalytic converters as the most likely source. This research highlights both the importance of unregulated catalytic converters as a source of ultrafine Ce-Ti particle pollution and the role of sub-cutaneous mites as a vector of these particles for amphibian exposure.
1 Introduction
The global amphibian population decline is estimated at 3.79% per year (USGS, 2021). Together with freshwater fish, amphibians are the most endangered class among the vertebrate groups, with a functional loss of 27% (Toussaint et al., 2021). Causes for these declines are diverse including habitat destruction (Calderón et al., 2019; Borzée et al., 2021), disease (Kriger and Hero, 2009; Herczeg et al., 2021), exposure to pesticides and chemicals (Pinto-Vidal et al., 2021; Goessens et al., 2022), and climate change (Velasco et al., 2021; Villamizar-Gomez et al., 2021). Even in natural protected areas, the steady decline of amphibian populations calls for more studies to be undertaken in pristine or rural areas to assess the extent of the threats worldwide (Green et al., 2020).
Amphibians are indicator species of ecosystem stress, as they are twice as sensitive to environmental factors due to their biphasic phases (Leduc et al., 2016; Velasco et al., 2021). Early life-history stages of semiaquatic or fully aquatic amphibians develop in water bodies where pollutants from urban runoff, vehicular traffic, agriculture, or mining effluents are common (Pounds et al., 2006; Brühl et al., 2013; Sievers et al., 2019). Under such environmental stress, amphibians may modify their behavior and physiology (Wong and Candolin, 2015), causing erratic swimming and altering their survival, growth, and reproduction (Sievers et al., 2018). For instance, in mining environments, high levels of copper (Cu) increase tadpole time spent swimming at the water surface (Hayden et al., 2015; Azizishirazi et al., 2021). Consequently, amphibians living in Cu-polluted environments show a higher-level of mortality (Azizishirazi et al., 2021) with disruptions in thyroid hormone metabolic pathways during metamorphosis (Thambirajah et al., 2019). In addition, Amphibians exposed to intensive vehicle traffic have a significant reduction in body size, body condition, and lower corticosterone concentrations compared to unexposed individuals (Cayuela et al., 2017). Yet, our understanding of the indirect effects associated with toxic exposure resulting from traffic on Amphibian life stages is still limited.
Catalytic converters have exhaustive and non-exhaustive emissions, the latter emissions associated with traffic are released from the catalytic converters, leading to contamination into soils and sediments (Meza-Figueroa et al., 2021). Chemical elements (Zr and Ce) used to manufacture three-way catalytic converters (TWCC) have low crustal abundances making the associated compounds valuable tracers of such sources in the environment (Meza-Figueroa et al., 2021; Navarro-Espinoza et al., 2021). A TWCC contains a honeycomb structure cordierite (2MgO·2Al2O3·5SiO2) monolith, with a coating made of cerium oxide (CeO2 or nanoceria) and zirconium oxide (ZrO2 or zirconia). A layer of platinoid nanoparticles, generally platinum or palladium, covers the Ce-Zr´s refractory washcoat (Aruguete et al., 2020). A typical TWCC operates at temperatures up to 800°C that can cause its deterioration and the potential release of platinoids and ZrO2-CeO2 compounds into the environment (Rinkovec, 2019; Meza-Figueroa et al., 2021) leading to the TWCC deactivation. Catalytic converters can then expel ultrafine particles (UFP) of compounds (ZrO2-CeO2) that are less than 0.1 µm in diameter and may be deposited in soils and water sources which in turn become a direct exposure path for living organisms. While this issue is assessed by regulatory vehicle emission policies in major cities, such policies are lacking in rural areas making UFP released from the TWCC refractory washcoat impossible to prevent.
Mites are a good model for assessing the pollution of both aquatic and terrestrial ecosystems due to their close relationship with environment, high abundance, high diversity of species, their cosmopolitan distribution, and the wide range of environments they inhabit (Walter and Proctor, 2013; Goldschmidt, 2016). Oribatid mites have often been used as bioindicators of soil contamination and toxicity (Eeva and Penttinen, 2009; Skubala and Zaleski, 2012; Manu et al., 2019). This characteristic is attributed to their diet made of fungal hyphae (primary food resource), which accumulates heavy metals, and to their ability to regulate concentrations of metals through low intestinal absorption and rapid excretion (Skubala and Zaleski, 2012). In particular, mites of the genus Hannemania Oudemans, 1911 (Acariformes: Prostigmata: Leeuwenhoekiidae) enter Amphibians’ skin during their early life stage (i.e., larva) and stay in the spongy layer of the epidermis for months, sometimes over a year, thus becoming a potential vector for UFP if these were accumulated beforehand (Hyland, 1961; Duszynski and Jones, 1973; Westfall et al., 2008). Among UFP, Amphibians are particularly susceptible to the effect of cerium compounds, with the in vitro toxicity of ceria particles being related to their chemical composition, aerodynamic size, and morphology (Forest et al., 2017).
Despite the extensive literature on the impacts of various pollutants on amphibians (Ferreira do Amaral et al., 2019; Slaby et al., 2019; Azizishirazi et al., 2021), information about the environmental occurrence of nanomaterials or UFP related to traffic sources is scarce (Ferreira do Amaral et al., 2019; Gallego-Hernández et al., 2020; Meza-Figueroa et al., 2021). In the context of the global decline of amphibian populations, novel paths of exposure to emerging pollutants must be explored, particularly in pristine areas. The objectives of our pilot study were: 1) to determine the occurrence of traffic-related Ce-Ti UFP in the rural environments, and 2) to determine in mites constitute a potential exposure route for such UFP in Amphibians.
2 Materials and Methods
2.1 Study Area
Amphibians and mites were collected near the town of Bacoachi is in the mining district of Cananea, Sonora, near the Mexico-United States border (Figure 1). The area hosts one of the most polluted open-pit copper mines worldwide (Barra et al., 2005) leading to high levels of soil and water pollution in the river have been attributed to these mining activities (Guzmán et al., 2019; Archundia et al., 2021; Morales-Pérez et al., 2021); yet no other potential sources for pollutants have been explored. While high levels of motor vehicle traffic occur on the riverbank due to recreational activities, there are no emission testing programs for automobiles in this region of Mexico, and several cars circulate with degraded catalytic converters most likely emitting UFP (pers. obs). As a reference for areas unaffected by either mining or traffic, we also obtained the local geochemical background (Calmus et al., 2018).
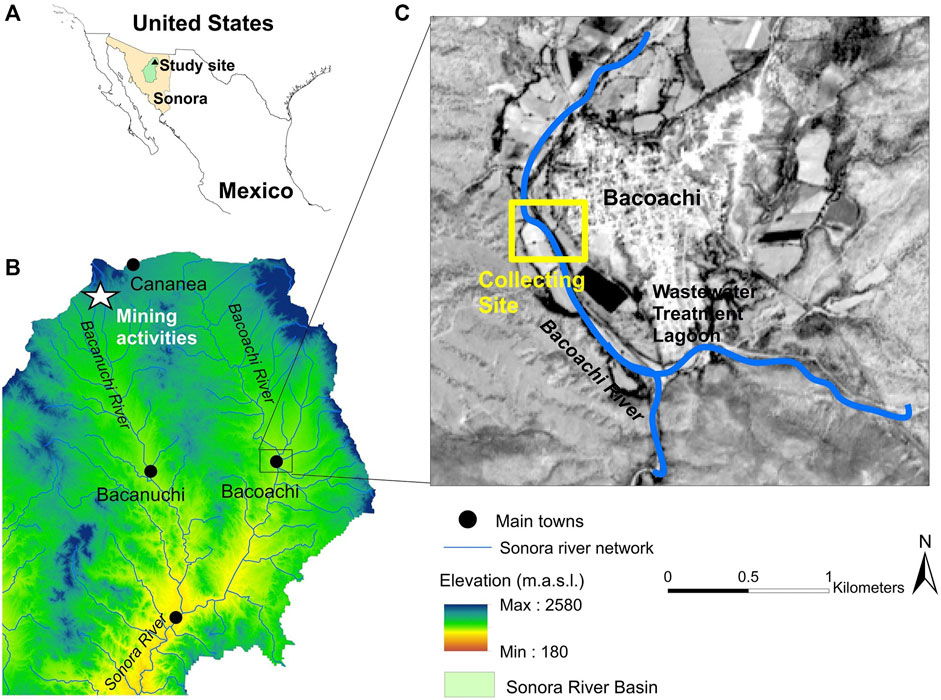
FIGURE 1. (A) Map of the study site, (B) the location of the Cananea mining area (Bacanuchi river), and (C) Bacoachi site and town.
2.2 Sediment, Amphibian, and Mite Sampling and Preparation
To distinguish pollution from traffic or mining sources, we also sampled sediments near the mining area. Sediment samples were collected from the riverbed in the areas of Bacoachi (hereafter our study area) and the area near the Cananea mine (Bacanuchi). Sediment samples were taken on one side of the river and in two sites of the flooding zone (1 kg approximately was taken at each point). All samples were collected with a stainless-steel shovel and kept in high-density plastic bags. Samples were homogenized and dried in an oven at 37°C for 24 h, then sieved through #18, 35, 60, and 120 mesh to obtain particulate matter with diameters of 51 mm, 500 μm, 250 μm, and 20 μm, respectively, for analysis by a portable X-ray fluorescence equipment.
Seven individuals of Lowland leopard frog, Lithobates yavapaiensis (Platz and Frost, 1984) were collected from the riverbank near Bacoachi town but we did not find any amphibians at the Bacanuchi site. Lowland leopard frogs are common in our study area and could thus represent a sentinel species for this pilot study. Amphibians were manually collected wearing vinyl gloves. Each specimen was weighed, measured, and inspected for mites. Specimens hosting mites (n = 5) were anesthetized with isoflurane and immersion baths of gel and water before removing the mites following procedures described in Doss et al. (2021). Mites were carefully extracted using a microscope and a small needle by opening the intradermal capsules and extracting the parasites. Mites were counted and preserved in 100 and 70% ethanol. They were further cleared with lactophenol and then mounted with PVA medium in a semi-permanent microscope slide for taxonomic identification (Hoffmann, 1990; Krantz and Walter, 2009). Following removal of the mite(s), the area was disinfected with hyper oxidation solution, and Lowland leopard frogs remained in observation for 10 min until released at the same location they were found. No individuals were lost or injured in this procedure. All animal capture and handling protocols for the scientific purpose at national territory were approved by the Secretariat of the Environment and Natural Resources in Mexico (SEMARNAT Permit No: FAUT-0027). The experimental procedure for mite´s collection permit was approved under permit: SGPA/DGVS/04418/21. All specimens were deposited in the National Mite Collection (CNAC) at the Biology Institute of the National Autonomous University of Mexico with access number CNAC012393-CNAC012402.
2.3 Total Metal Content in Sediments
We used a portable X-ray fluorescence NitonTM FXL 950 instrument (ThermoFisher Scientific Inc., Boston, Massachusetts, U.S.) with an X-ray tube: silver anode, 50kV/200 μA/4W, and a geometrically optimized area drift detector (GOLDD). We analyzed soil samples using the TestAllGeo mode for a fixed period of 120 s. We used seven replicates of the reference material standard NIST 2710a and a blank made of pure SiO2 for quality control following method 6200 (United States Environmental Protection Agency). Our pilot study was restricted to analyzing the elements: Zr, Sr, Rb, Pb, As, Ce, Zn, Cu, Ni, Co, Mn, Cr, V, Ti, K, Sb, Nb, Y, Fe, and Ca. Each sample was analyzed in triplicate, and we reported the recovery range from 100 to 110% for all analyzed elements, but Ce whose concentration was below the detection limit. The detection of Ce was obtained from Raman and SEM analysis.
2.4 SEM and Raman Analysis in Mites
Mites were analyzed both superficially and internally after the dissection. We obtained the particle size, semiquantitative chemical analysis, and morphology of UFP using scanning electron microscopy and energy dispersive spectroscopy (SEM-EDS) Phenom ProX desktop (ThermoFisher Scientific Inc., Boston, Massachusetts, U.S.). We used the analytical conditions of 5 kV for particle morphology and at 15kV for chemical compositions by EDS. We used an Alpha300 RA Raman confocal microspectrometer (WITec, Ulm, Germany) to identify crystalline particles in mites. The instrument has a Nd:YAG frequency-doubled laser excitation of 532 nm. We resuspended the samples using ethanol and placed them on a calcium fluoride substrate for 2h in a desiccator (CaF2, 13 mm Ø × 1.0 mm, Crystan Ltd.,United Kingdom). We used a cubic zirconia solid standard (ASTIMEX MINM25-53 Serial 1Al) for checking the instrument performance of both Raman and SEM.
2.5 Multivariate Statistical Analysis
We used a Principal Component Analysis (PCA) to evaluate the chemical differences among sediments collected within the mining area and our study site where amphibians and mites were sampled. PCA has been employed for source apportionment of metals (Shi et al., 2022) and to assess chemical differences between used and new TWCC (Navarro-Espinoza et al., 2021). The suitability of the dataset was evaluated by the Kaiser-Meyer-Olkin (KMO) and Bartlett´s test of sphericity. The obtained KMO (0.732) value was >0.7 and we considered a Spearman correlation coefficient >0.5 as significant to determine a common source of metals (Liu et al., 2020). All statistical analyses were performed using XLSTAT 2021.4.1 (Addinsoft, 2022).
3 Results
3.1 Total Metal Content in Sediments
We observed a range of total metal content in sediments across the mining and our study sites (Table 1). The elements that exceeded the LGB around mining activities were in the following order: Co > Sb > Cu > Zr > Nb > Zn > Ni > Sr > Pb while the ranking was slightly different in the study site: Co > Sb > Zr > Cu > Nb > Y > V > Zn > Fe. Due to its crustal values, and conservative behavior, Zr has been recommended as a reference element in environmental studies and it is commonly assumed to have a natural origin (i.e., geogenic; Calmus et al., 2018). However, the average content of Zr in our study area (838 mg kg−1) was more than twice the mean content in sediments from the mining area (364.5 mg kg−1), and more than four times higher than the LGB (193 mg kg−1) uggesting an anthropic origin unrelated to mining.
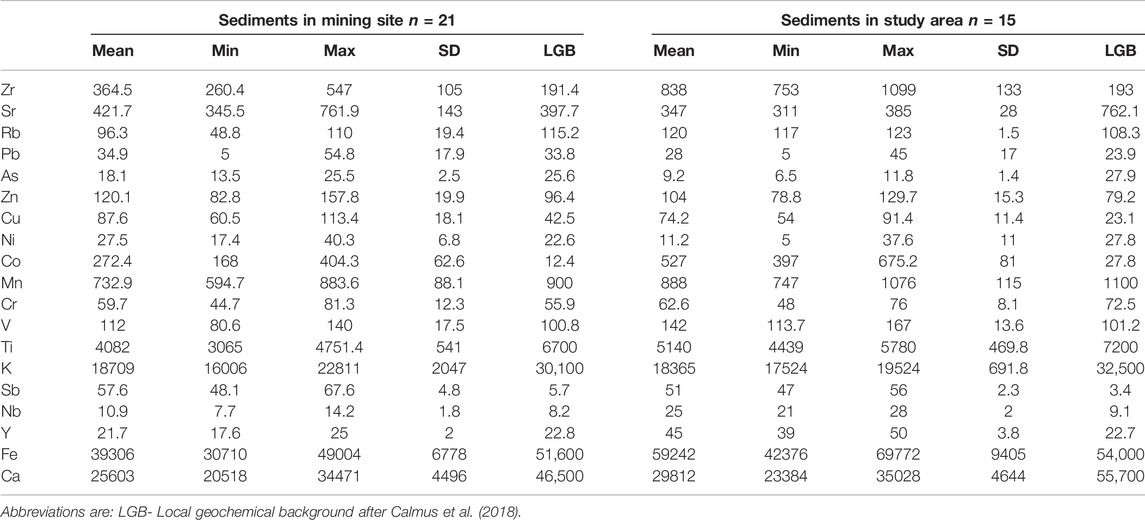
TABLE 1. Geochemistry of riverbank sediments collected from 1) active mining area of Bacanuchi and 2) study area where amphibian and mites were collected.
The following elements occurred in higher concentrations at the study site than those found in the mining area: Zr-Cu-V-Sb-Nb-Y-Fe. The concentration of Ti and Zr is remarkably different in the study area compared to the mining site (Figure 2) indicating a source different to mineralization.
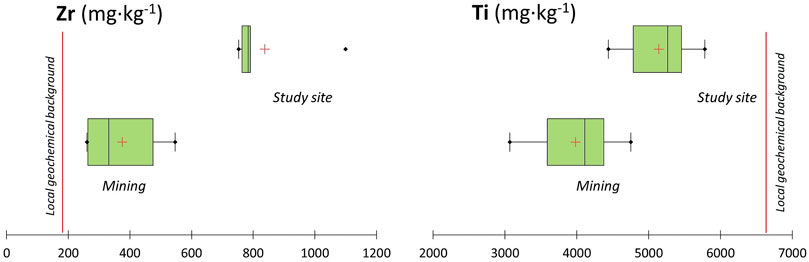
FIGURE 2. Comparison of Ti and Zr contents in sediments from the study site of Bacochi (where amphibians were collected) and the mining area. Local geochemical background is shown for reference as the red dotted line (after Calmus et al., 2018). The box plots show the average, maximum, minimum, and median values (red cross) for each metal in the <20 µm fraction of sediments of our study.
Table 2 contains a correlation matrix for sediments collected at both sites. The element Zr is correlated at an alpha = 0.05 significance level with Pb-Zn-Cu-Ni-Mn-V-Ti-K, and Fe at the site where amphibians were collected. The geochemical signatures of riverbank sediments were different among sites (Figure 3) and the first two principal components explained 68.02% of the variability. The first principal component (PC1) explained 47.76% of total variance, defined by the contributions (%) of the following elements: Zr, Co, V, Ti, Nb, Y, Mn, and Fe, thus separating the group of sediments impacted by traffic sources in Bacoachi from the mining site. The second principal component (PC2) explained the 20.27% of the total variance and it is defined by the contributions of Pb, Zn, Cu, which follow the mineralization of the area. The third component (PC3) contributed 10.63% of the total variance and contained geogenic elements such as Rb, Cr, K, and Ca. Cerium, titanium, and zirconium content in riverbank sediments collected at the mining area are similar to those reported worldwide (Table 3).
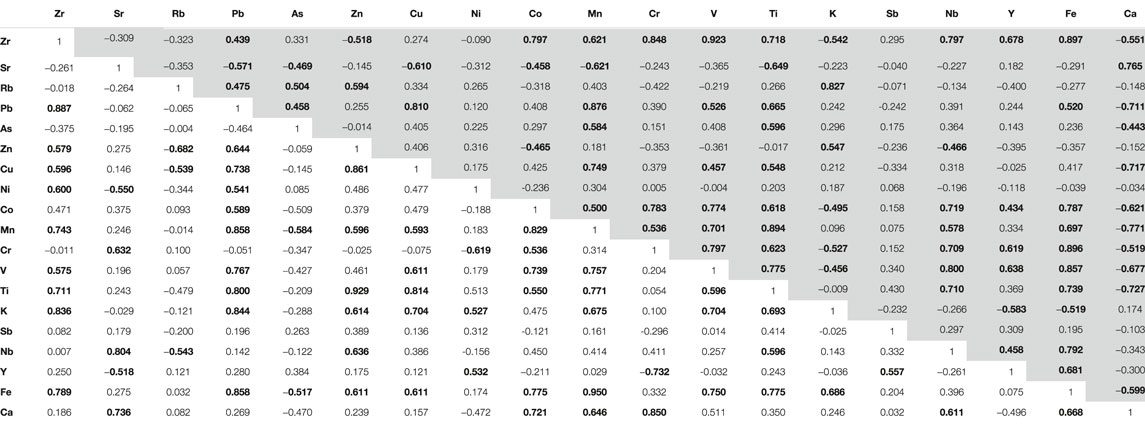
TABLE 2. Values different from zero at alpha=0.05 significance level are shown in bold. Shaded area corresponds to sediments collected in the active mining site, white area corresponds to the Bacanuchi riverbank.

TABLE 3. Cerium, titanium, and zirconium content reported in riverbank sediments near mining zones worldwide. Concentrations in mg.kg−1, unless otherwise stated. Not reported (nr).
3.2 Characterization of UFP Adhered to Mites
The mites had an average size of 270 by 530 µm (Figure 4A), and the UFP adhered to mites consisted of polycrystalline agglomerates with particle sizes varying from 850 nm to 5 µm (Figure 4B). The analysis of UFP revealed the presence of Zr, Ce, Sb, and Ti with traces of La, Mo, and Br. We also identified particles smaller than 1 µm composed of Zr, Sb, Ce, La, Br, and Ti (Figures 5A,B) similar to those associated with TWCC emissions (Meza-Figueroa et al., 2021).
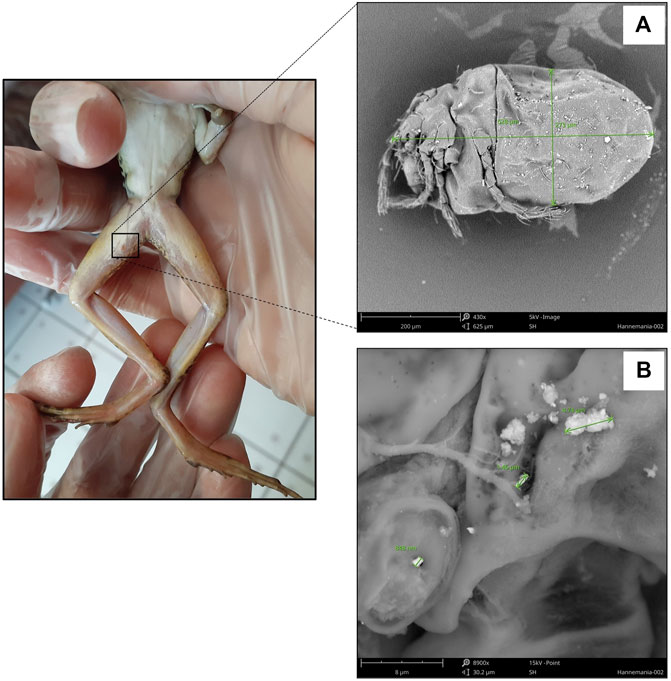
FIGURE 4. Lithobates yavapaiensis parasitized by Hannemania mites (A) Backscattered electron micrographs of a Hannemania mite, and (B) ultrafine particles containing Ce, Sb, and Zr, among other traffic-related elements.
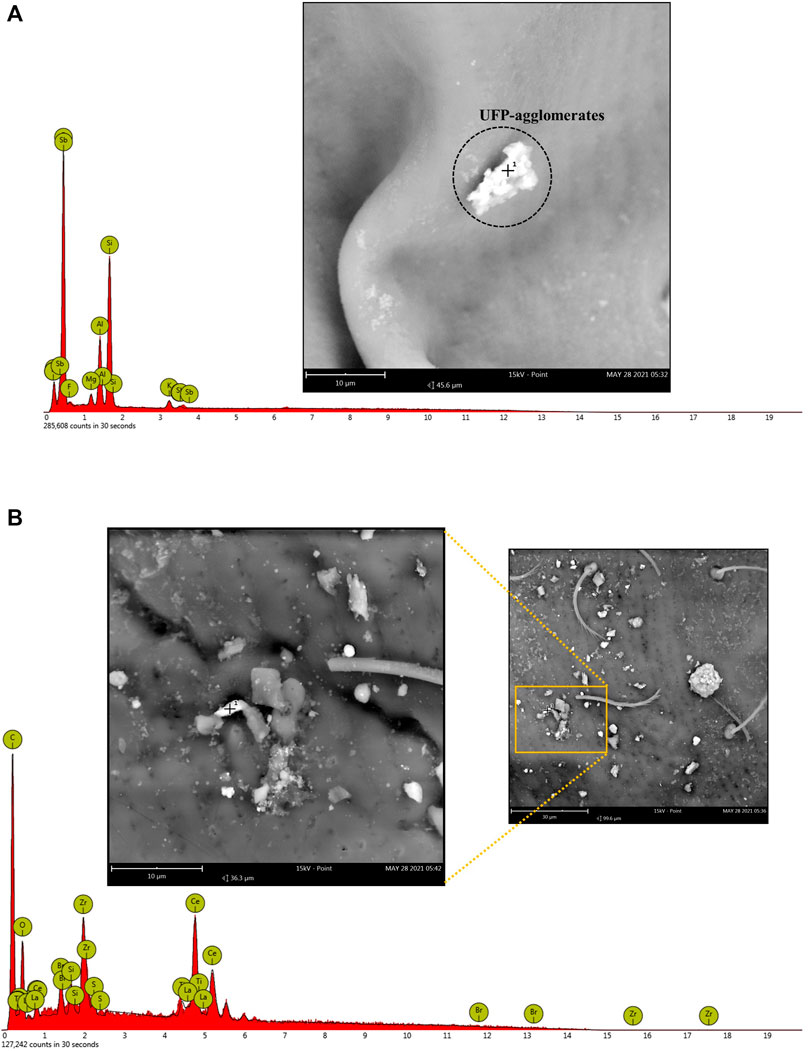
FIGURE 5. Scanning electron microscopy images and energy dispersive spectroscopy analysis of (A) UFP agglomerates on Hannemania mites, and (B) semiquantitative analysis of UFP.
The Raman spectra of the TWCC washcoat reported by Meza-Figueroa et al. (2021) and Navarro-Espinoza et al. (2021), and the particles in the mites showed a peak for the TWCC washcoat at 465 cm−1 attributed to the symmetric stretching mode of the vibratory unit Ce-O (Kosacki et al., 2002). The peaks at 149, 251, and 314 cm−1 were ascribed to the vibrational model Eg, A1g, and B1g of the O-Zr-O stretches. The particles on the mites showed peaks at 149 cm−1, and 251 cm−1 of tetragonal ZrO2, and 339 cm−1 of monoclinic ZrO2 (Quintard et al., 2002). The peak at 465 cm−1 in mite particles corresponds to CeO2 and the peak at 602 cm−1 is attributed to the A1g vibrational mode of tetragonal TiO2 rutile (Frank et al., 2012; Gallego-Hernández et al., 2020).
Additionally, three well-defined peaks revealed the presence of albite mineral (Figure 6). Albite can arise from natural sources, such as dust from local soils. The peak at 83 cm−1 is assigned to tetrahedral cage shear displacements in conjunction with Na environment breathing-rotation motions. The peak at 720 cm−1 was a signature of four fundamental modes: O-Al-O bend. Si-Al tetrahedral deformation, Na-OA1 and Na-OB(o) stretch. The peak at 1032 cm−1 was created by internal tetrahedral vibrations dominated by Si-O stretch (McKeown, 2005).
3.3 Hannemania Mites
The prevalence of Hannemania infesting Lithobates yavapaiensis was 71.42% (n = 7). On macroscopic examination, mites were found within the skin of the ventral abdomen and femoral areas of the amphibians. The taxonomic identification of the mite genus was based on characters with taxonomic importance, such as the shape of the scutum, the cheliceral, multiple genualae on all legs, and the number of branched setae in palpal tarsus. These characteristics were evaluated in 10 specimens (Brennan and Goff, 1977; Hoffmann, 1990; Alvarado-Rybak et al., 2018). The average value for Hannemania mites among the infested individuals was 2.8 ± 1.11.
4 Discussion
Our study highlights the importance of considering the study of endoparasitic mites (e.g., Hannemnia mites) within the biotic factors as an alternative route of exposure of amphibian species to pollutants. Regarding this topic, Ferreira do Amaral and collaborators (2019) mention amphibians are able to absorb nanomaterials through their skin. Hagens et al. (2007) also mention the nanoparticles in the dermis can migrate to the central blood circulation and from there to the entire body.
The elements Zr-Cu-V-Sb-Nb-Y-Fe have been previously described by Navarro-Espinoza et al. (2021) as contaminant elements in TWCC that promote the detachment of the refractory washcoat made of Zr-Ce. This process is crucial for the release of UFP to the environment. An important finding of this pilot study is identifying the presence of Zr, Ce, and Ti UFP in the mites collected from amphibians. These elements are commonly considered as conservative, i.e., their concentrations have not been substantially modified by human activity and therefore, can be used as reference elements in the estimating of pollution indices (Calmus et al., 2018). However, our results show that Ti and Zr concentrations in sediments can be affected by traffic sources, even in pristine areas. The presence of traffic-related elements in levels above the LGB is relevant because of the toxicity of Ti to amphibians and the association of Zr with Ce in TWWC. Polycrystalline agglomerates identified in mites also contain particles with Al, Si, Mg, and K most likely derived from geogenic sources.
The presence of Zr at concentrations above LGB in the riverbank is relevant since there are no Zr-sources other than traffic in the area. Previous studies have shown the strong correlation of Ce and Zr in road dust collected at high traffic areas (Meza-Figueroa et al., 2021) and the Raman signature of ceria-zirconia particles (Navarro-Espinoza et al., 2021). Even though we did not obtain the total Ce composition of sediments, the UFP composed of Ce-Zr was identified by Raman spectroscopy and supported by the SEM-EDS analysis.
The Raman results of UFP analysis show peaks at 149, 251, and 314 cm−1 that result from the division of F2g (465 cm−1) because of the doping of cubic CeO2 with high concentrations of Zr (>20 mol% Zr; Kuhn et al., 2013). The introduction of Zr generates the transition of cubic crystals of CeO2 to a tetragonal structure (Bolon and Gentleman, 2011; Kuhn et al., 2013). The distortion of this crystal lattice produces oxygen vacancies (Vö) that are essential for the catalysis process (peak at 617 cm−1; Kosacki et al., 2002). ZrO2-CeO2 compounds found in a TWCC can be released and incorporated into environmental matrices (Meza-Figueroa et al., 2021).
Bour et al. (2015) found that exposure, particle size, and the concentration of CeO2 could produce high mortality, growth inhibition, and genotoxicity in amphibians. CeO2 toxicity is species-dependent, and the route of exposure is a relevant variable influencing toxicity that should be further studied. Previous studies showed that bare CeO2 nanoparticles (mainly found in the water column) induced high genotoxicity on amphibian larvae (Bour et al., 2017). Furthermore, Keller et al. (2010) and Quik et al. (2010) found that CeO2 nanoparticles tend to form aggregates with consequent sedimentation, potentially entering the trophic chain through their integration to organisms such amphibian. Most of the published research was performed under controlled conditions at the laboratory and this is one of the few studies reporting CeO2-ZrO2 UFP in natural systems.
Our results validate the data obtained by the elemental determination from the SEM-EDS, showing high concentrations of titanium (Ti) and oxygen (O) in the mite samples (Silva et al., 2021). On the other hand, the small size of TiO2 nanoparticles allows them to penetrate cells and accumulate therein (Nations, 2009) leading to alterations of the cellular metabolism or even apoptosis (Galdiero et al., 2017). Zhang et al. (2012), and Zhang (2011) reported that the increase of TiO2 (10 nm) and UVA light co-exposition decreased Xexopus laevis survival and the exposure to particles of different size (5,10, and 32 nm) with or without ultraviolet light and high concentrations of nano-TiO2, significantly affected tadpole growth. UV-A radiation can pass through the translucent skin of amphibians and interact with nano TiO2 particles damaging amphibian tissues and impacting growth (Nations et al., 2011; Zhang et al., 2012; Vijayaraj et al., 2018). Furthermore, Hammond et al. (2013) reported a risk of hormone disruption (thyroxine, and triiodothyronine) and cellular stress in amphibians exposed to different sizes and concentrations of TiO2 nanoparticles. Therefore, endoparasite mites such as Hannemania sp. may indirectly increase the risk in juvenile amphibians or species with very light skin coloration (i.e., the family Centrolenidae).
To our knowledge, this is the first record of Hannemania mites on Lithobates yavapaiensis although Hannemania mites have been reported in other species of juvenile and adults amphibians living in the Americas and New Caledonia (Silva-De la Fuente et al., 2016; Bassini-Silva et al., 2021). The prevalence reported in this study (71.42%) is within the range observed in other amphibians species parasitized by Hannemania mites in Sonora state with prevalences from 20 to 95% (Hoffmann, 1969; Loomis and Welbourn, 1969; Goldberg et al., 2002). While the number of mites per individual was low in this study, the infestation level is a key factor in the damage they can cause to their host, especially when abundances are as high as those observed in Bassini-Silva et al. (2021). Therefore, we suggest conducting further experimental research on the concentrations of metals that Hannemania mites may harbor and expose Amphibians to.
5 Conclusion
The UFP identified in mites were derived from polluted sediments impacted by traffic suggesting the likelihood for intradermal Hannemania mites to be a pathway for UFP exposure in Amphibians. The presence of Ce and Ti oxides on mites is thus potentially toxic to amphibians and further research should continue addressing the potential synergy of biotic and abiotic factors in threatening species (Carrasco et al., 2021). Given the prolonged period in which parasites remain within their host, the potentially detrimental effects of chronic exposure on amphibians are high (Welbourn and Loomis, 1975; Westfall et al., 2008). Therefore, we recommend future work to investigate different exposure times and assess the role of parasites load in increasing the toxicity in all life stages of the host. Ultimately, regulations should also be developed to reduce the threat of traffic derived UFP to amphibians and ecological consequences even in rural areas.
Data Availability Statement
The original contributions presented in the study are included in the article/supplementary material, further inquiries can be directed to the corresponding authors.
Ethics Statement
Ethical review and approval was not required for the animal study because all animal capture and handling protocols for the scientific purpose at national territory were approved by the Secretariat of the Environment and Natural Resources in Mexico (SEMAR Permit No: FAUT-0027). The experimental procedure for mite ́s collection permit was approved under permit: SGPA/DGVS/04418/21. All specimens were deposited in the National Mite Collection (CNAC) at the Biology Institute of the National Autonomous University of Mexico with access number CNAC012393-CNAC012402. No amphibians were lost or injured in this procedure, and after mite ́s extraction, the specimens were placed in the exact place where we found them.
Author Contributions
MJ-M: Investigation, conceptualization, formal analysis, Writing-original draft, Review and Editing. DM-F: Funding acquisition, Conceptualization, Investigation, Writing-original draft, Review and Editing. MP-M: Investigation, Methodology, Writing-review and Editing. DL: Investigation, Writing-review and Editing. AR-M: Methodology, Writing-review and editing. SN-E: Formal analysis, Data curation, Resources, Writing-review and Editing. BG-G: Formal analysis, Methodology, Data curation, Resources, Writing-review and Editing. EP-S: Conceptualization, Investigation. ES-C: Writing-review and Editing. AA-M: Writing-review and Editing. RP-L: Investigation, Resources, Data curation, Editing.
Funding
This research was funded by the National Council for Science and Technology in Mexico (CONACYT) Grant 309959 PRONACES Toxic Agents, and Grant A1-S-29697 to DM-F, and a postdoctoral scholarship to M. Jacinto-Maldonado I1200/94/2020.
Conflict of Interest
The authors declare that the research was conducted in the absence of any commercial or financial relationships that could be construed as a potential conflict of interest.
Publisher’s Note
All claims expressed in this article are solely those of the authors and do not necessarily represent those of their affiliated organizations, or those of the publisher, the editors and the reviewers. Any product that may be evaluated in this article, or claim that may be made by its manufacturer, is not guaranteed or endorsed by the publisher.
Acknowledgments
We gratefully acknowledge Iván Guillermo Souffle Lamphar, Lourdes Gabriela Canizalez Juárez, and Miguel Ernesto Rosas Morales from the Herpetology Club at the University of Sonora for field assistance and discussions. We also thank Daisy López, Jay Taylor, Enrique de la Re Vega, and Griselda Montiel Parra for their valuable comments and technical support.
References
Addinsoft (2022). XLSTAT Statistical and Data Analysis Solution. Available at: https://www.xlstat.com/es (Accessed February 05, 2022).
Alvarado-Rybak, M., Valenzuela-SÁnchez, A., Cevidanes, A., Peñafiel-Ricaurte, A., Uribe-Rivera, D. E., Flores, E., et al. (2018). High prevalence of chigger mite infection in a forest-specialist frog with evidence of parasite-related granulomatous myositis. Parasitol. Res 117, 1–16. doi:10.1007/s00436-018-5822-x
Akinyemi, S. A., Nyakuma, B. B., Jauro, A., Olanipekun, T. A., Mudzielwana, R., Gitari, M. W., et al. (2021). Rare Earth Elements Study of Cretaceous Coals from Benue Trough Basin, Nigeria: Modes of Occurrence for Greater Sustainability of Mining. Fuel 304, 121468. doi:10.1016/j.fuel.2021.121468
Archundia, D., Prado-Pano, B., González-Méndez, B., Loredo-Portales, R., and Molina-Freaner, F. (2021). Water Resources Affected by Potentially Toxic Elements in an Area under Current and Historical Mining in Northwestern Mexico. Environ. Monit. Assess. 193, 1–20. doi:10.1007/s10661-021-08998-z
Aruguete, D. M., Wallace, A., Blakney, T., Kerr, R., Gerber, G., and Ferko, J. (2020). Palladium Release from Catalytic Converter Materials Induced by Road De-icer Components Chloride and Ferrocyanide. Chemosphere 245, 125578. doi:10.1016/j.chemosphere.2019.125578
Azizishirazi, A., Klemish, J. L., and Pyle, G. G. (2021). Sensitivity of Amphibians to Copper. Environ. Toxicol. Chem. 40, 1808–1819. doi:10.1002/etc.5049
Barra, F., Ruiz, J., Valencia, V. A., Ochoa-Landín, L., Chesley, J. T., and Zurcher, L. (2005). Laramide Porphyry Cu-Mo Mineralization in Northern Mexico: Age Constraints from Re-os Geochronology in Molybdenite. Econ. Geol. 100, 1605–1616. doi:10.2113/gsecongeo.100.8.1605
Bassini-Silva, R., Huang-Bastos, M., Morais, D. H., Alcantara, E. P., Ávila, R. W., Welbourn, C., et al. (2021). A New Species of Hannemania Oudemans, 1911 (Trombidiformes: Leeuwenhoekiidae) from Brazil. J. Nat. Hist. 55, 1277–1287. doi:10.1080/00222933.2021.1944687
Bolon, A. M., and Gentleman, M. M. (2011). Raman Spectroscopic Observations of Ferroelastic Switching in Ceria-Stabilized Zirconia. J. Am. Ceram. Soc. 94, 4478–4482. doi:10.1111/j.1551-2916.2011.04737.x
Borzée, A., Kielgast, J., Wren, S., Angulo, A., Chen, S., Magellan, K., et al. (2021). Using the 2020 Global Pandemic as a Springboard to Highlight the Need for Amphibian Conservation in Eastern Asia. Biol. Conserv. 255, 108973. doi:10.1016/j.biocon.2021.108973
Bour, A., Mouchet, F., Verneuil, L., Evariste, L., Silvestre, J., Pinelli, E., et al. (2015). Toxicity of CeO2 Nanoparticles at Different Trophic Levels - Effects on Diatoms, Chironomids and Amphibians. Chemosphere 120, 230–236. doi:10.1016/j.chemosphere.2014.07.012
Bour, A., Mouchet, F., Cadarsi, S., Silvestre, J., Baqué, D., Gauthier, L., et al. (2017). CeO2 Nanoparticle Fate in Environmental Conditions and Toxicity on a Freshwater Predator Species: a Microcosm Study. Environ. Sci. Pollut. Res. 24, 17081–17089. doi:10.1007/s11356-017-9346-1
Brühl, C. A., Schmidt, T., Pieper, S., and Alscher, A. (2013). Terrestrial Pesticide Exposure of Amphibians: An Underestimated Cause of Global Decline? Sci. Rep. 3, 1–4. doi:10.1038/srep01135
Brennan, J. M., and Goff, M. L. (1977). Keys to the Genera of Chiggers of the Western Hemisphere (Acarina: Trombiculidae) J. Parasitol 63, 554–566. doi:10.2307/3280021
Calderón, M. R., Almeida, C. A., González, P., and Jofré, M. B. (2019). Influence of Water Quality and Habitat Conditions on Amphibian Community Metrics in Rivers Affected by Urban Activity. Urban Ecosyst. 22, 743–755. doi:10.1007/s11252-019-00862-w
Calmus, T., Valencia-Moreno, M., Del Río-Salas, R., Ochoa-Landín, L., and Mendivil-Quijada, H. (2018). A Multi-Elemental Study to Establish the Natural Background and Geochemical Anomalies in Rocks from the Sonora River Upper Basin, NW Mexico. revmexcg 35, 158–167. doi:10.22201/cgeo.20072902e.2018.2.605
Carrasco, G. H., de Souza, M. B., and de Souza Santos, L. R. (2021). Effect of Multiple Stressors and Population Decline of Frogs. Environ. Sci. Pollut. Res. 28, 59519–59527. doi:10.1007/s11356-021-16247-6
Cayuela, H., Quay, L., Dumet, A., Léna, J.-P., Miaud, C., and Rivière, V. (2017). Intensive Vehicle Traffic Impacts Morphology and Endocrine Stress Response in a Threatened Amphibian. Oryx 51, 182–188. doi:10.1017/s0030605315000812
Doss, G. A., Mans, C., and Sladky, K. K. (2021). “Analgesia, Anesthesia, and Monitoring,” in Exotic Animal Emergency and Critical Care Medicine (John Wiley & Sons), 746–757. doi:10.1002/9781119149262.ch45
Duszynski, D. W., and Jones, K. L. (1973). The Occurrence of Intradermal Mites, Hannemania Spp. (Acarina: Trombiculidae), in Anurans in New Mexico with a Histological Description of the Tissue Capsule. Int. J. Parasitol. 3, 531–538. doi:10.1016/0020-7519(73)90050-7
Eeva, T., and Penttinen, R. (2009). Leg Deformities of Oribatid Mites as an Indicator of Environmental Pollution. Sci. Total Environ. 407, 4771–4776. doi:10.1016/j.scitotenv.2009.05.013
Ferreira do Amaral, D., Guerra, V., Motta, A. G. C., de Melo e Silva, D., and Rocha, T. L. (2019). Ecotoxicity of Nanomaterials in Amphibians: A Critical Review. Sci. Total Environ. 686, 332–344. doi:10.1016/j.scitotenv.2019.05.487
Forest, V., Leclerc, L., Hochepied, J.-F., Trouvé, A., Sarry, G., and Pourchez, J. (2017). Impact of Cerium Oxide Nanoparticles Shape on Their In Vitro Cellular Toxicity. Toxicol. Vitro 38, 136–141. doi:10.1016/j.tiv.2016.09.022
Frank, O., Zukalova, M., Laskova, B., Kürti, J., Koltai, J., and Kavan, L. (2012). Raman Spectra of Titanium Dioxide (Anatase, Rutile) with Identified Oxygen Isotopes (16, 17, 18). Phys. Chem. Chem. Phys. 14, 14567–14572. doi:10.1039/c2cp42763j
Fuente, M. C. S.-D. L., Moreno-Salas, L., and Castro-Carrasco, C. (2016). Review of the Genus Hannemania (Acari: Leeuwenhoekiidae) with Description the Two New Species in Amphibians from Chile. Zootaxa 4200, 580–590. doi:10.11646/zootaxa.4200.4.8
Galdiero, E., Falanga, A., Siciliano, A., Maselli, V., Guida, M., Carotenuto, R., et al. (2017). Daphnia Magna and Xenopus laevis as In Vivo Models to Probe Toxicity and Uptake of Quantum Dots Functionalized with gH625. Int. J. Nanomedicine 12, 2717–2731. doi:10.2147/ijn.s127226
Gallego-Hernández, A. L., Meza-Figueroa, D., Tanori, J., Acosta-Elías, M., González-Grijalva, B., Maldonado-Escalante, J. F., et al. (2020). Identification of Inhalable Rutile and Polycyclic Aromatic Hydrocarbons (PAHs) Nanoparticles in the Atmospheric Dust. Environ. Pollut. 260, 114006–114010. doi:10.1016/j.envpol.2020.114006
Godwyn-Paulson, P., Jonathan, M. P., Rodríguez-Espinosa, P. F., and Rodríguez-Figueroa, G. M. (2022). Rare Earth Element Enrichments in Beach Sediments from Santa Rosalia Mining Region, Mexico: An Index-Based Environmental Approach. Mar. Pollut. Bull. 174, 113271. doi:10.1016/j.marpolbul.2021.113271
Goessens, T., De Baere, S., Deknock, A., De Troyer, N., Van Leeuwenberg, R., Martel, A., et al. (2022). Agricultural Contaminants in Amphibian Breeding Ponds: Occurrence, Risk and Correlation with Agricultural Land Use. Sci. Total Environ. 806, 1–13. doi:10.1016/j.scitotenv.2021.150661
Goldberg, S. R., Wrenn, W. J., and Bursey, C. R. (2002). Bufo Mazatlanensis (Sinaloa Toad), Rana Tarahumarae (Tarahumara Frog). Ectoparasites. Herpetol. Rev. 33, 301–302.
Goldschmidt, T. (2016). Water Mites (Acari, Hydrachnidia): Powerful but Widely Neglected Bioindicators - a Review. Neotropical Biodivers. 2, 12–25. doi:10.1080/23766808.2016.1144359
Green, D. M., Lannoo, M. J., Lesbarrères, D., and Muths, E. (2020). Amphibian Population Declines: 30 Years of Progress in Confronting a Complex Problem. Herpetologica 76, 97–100. doi:10.1655/0018-0831-76.2.97
Guzmán, H. M., Gómez-Álvarez, A., Valenzuela-García, J. L., Encinas-Romero, M. A., Villalba-Atondo, A. I., and Encinas-Soto, K. K. (2019). Assessment of the Impact on Sediment Quality from Abandoned Artisanal Mine Runoffs in a Semi-arid Environment (The Sonora River Basin—Northwestern Mexico). Environ. Earth Sci. 78, 1–14. doi:10.1007/s12665-019-8131-5
Hagens, W. I., Oomen, A. G., de Jong, W. H., Cassee, F. R., and Sips, A. J. A. M. (2007). What do we (need to) know about the kinetic properties of nanoparticles in the body?. Regul. Toxicol. Pharmacol 49, 217–229. doi:10.1016/J.YRTPH.2007.07.006
Hammond, S. A., Carew, A. C., and Helbing, C. C. (2013). Evaluation of the Effects of Titanium Dioxide Nanoparticles on Cultured Rana catesbeiana Tailfin Tissue. Front. Genet. 4, 251–258. doi:10.3389/fgene.2013.00251
Hayden, M. T., Reeves, M. K., Holyoak, M., Perdue, M., King, A. L., and Tobin, S. C. (2015). Thrice as Easy to Catch! Copper and Temperature Modulate Predator‐prey Interactions in Larval Dragonflies and Anurans. Ecosphere 6, 1–17. doi:10.1890/es14-00461.1
Herczeg, D., Ujszegi, J., Kásler, A., Holly, D., and Hettyey, A. (2021). Host–multiparasite Interactions in Amphibians: a Review. Parasites Vectors 14, 1–20. doi:10.1186/s13071-021-04796-1
Hoffmann, A. (1990). Los trombicúlidos de México (Acarida: Trombiculidae): Parte taxonómica. Mexico City: Universidad Nacional Autónoma de México Press.
Hoffmann, L., and Koriath, G. (1969). NEFr-Aufwand und Verwertung der Futterenergie für Milchproduktion. Arch. für Tierernaehrung 19, 209–222. doi:10.1080/17450396909424526
Hyland, K. E. (1961). Parasitic Phase of Chigger Mite, Hannemania Hegeneri, on Experimentally Infested Amphibians. Exp. Parasitol. 11, 212–225. doi:10.1016/0014-4894(61)90027-3
Keller, A. A., Wang, H., Zhou, D., Lenihan, H. S., Cherr, G., Cardinale, B. J., et al. (2010). Stability and Aggregation of Metal Oxide Nanoparticles in Natural Aqueous Matrices. Environ. Sci. Technol. 44, 1962–1967. doi:10.1021/es902987d
Kosacki, I., Suzuki, T., Anderson, H. U., and Colomban, P. (2002). Raman Scattering and Lattice Defects in Nanocrystalline CeO2 Thin Films. Solid State Ionics 149, 99–105. doi:10.1016/s0167-2738(02)00104-2
Krantz, G. W., and Walter, D. E. (2009). A Manual of Acarology. 3rd ed.. Lubbock (TX): Texas Tech University Press.
Kriger, K. M., and Hero, J.-M. (2009). Chytridiomycosis, Amphibian Extinctions, and Lessons for the Prevention of Future Panzootics. Ecohealth 6, 6–10. doi:10.1007/s10393-009-0228-y
Kuhn, M., Bishop, S. R., Rupp, J. L. M., and Tuller, H. L. (2013). Structural Characterization and Oxygen Nonstoichiometry of Ceria-Zirconia (Ce1−xZrxO2−δ) Solid Solutions. Acta Mater. 61, 4277–4288. doi:10.1016/j.actamat.2013.04.001
Leduc, J., Echaubard, P., Trudeau, V., and Lesbarrères, D. (2016). Copper and Nickel Effects on Survival and Growth of Northern Leopard Frog (Lithobates Pipiens) Tadpoles in Field-Collected Smelting Effluent Water. Environ. Toxicol. Chem. 35, 687–694. doi:10.1002/etc.3227
Liu, H., Guo, H., Pourret, O., Wang, Z., Liu, M., Zhang, W., et al. (2022). Geochemical Signatures of Rare Earth Elements and Yttrium Exploited by Acid Solution Mining Around an Ion-Adsorption Type Deposit: Role of Source Control and Potential for Recovery. Sci. Total Environ. 804, 150241. doi:10.1016/j.scitotenv.2021.150241
Liu, L., Dong, Y., Kong, M., Zhou, J., Zhao, H., Tang, Z., et al. (2020). Insights into the Long-Term Pollution Trends and Sources Contributions in Lake Taihu, China Using Multi-Statistic Analyses Models. Chemosphere 242, 125272. doi:10.1016/j.chemosphere.2019.125272
Loomis, R. B., and Welbourn, W. C. (1969). A New Species of Hannemania (Acarina, Trombiculidae) from Bufo punctatus of Western North America, with Comments on Hannemania Hylae (Ewing). Bull. South. Calif. Acad. Sci. 68, 161–169.
Manu, M., Honciuc, V., Neagoe, A., Băncilă, R. I., Iordache, V., and Onete, M. (2019). Soil Mite Communities (Acari: Mesostigmata, Oribatida) as Bioindicators for Environmental Conditions from Polluted Soils. Sci. Rep. 9, 1–13. doi:10.1038/s41598-019-56700-8
McKeown, D. A. (2005). Raman spectroscopy and vibrational analyses of albite: From 25°C through the melting temperature. Am. Mineral 90, 1506–1517. doi:10.2138/am.2005.1726
Meza-Figueroa, D., Pedroza-Montero, M., Barboza-Flores, M., Navarro-Espinoza, S., Ruiz-Torres, R., Robles-Morúa, A., et al. (2021). Identification of Refractory Zirconia from Catalytic Converters in Dust: An Emerging Pollutant in Urban Environments. Sci. Total Environ. 11, 559–564. doi:10.1016/j.scitotenv.2020.143384
Morales-Pérez, A., Moreno-Rodríguez, V., Del Rio-Salas, R., Imam, N. G., González-Méndez, B., Pi-Puig, T., et al. (2021). Geochemical Changes of Mn in Contaminated Agricultural Soils Nearby Historical Mine Tailings: Insights from XAS, XRD and, SEP. Chem. Geol. 573, 1–12. doi:10.1016/j.chemgeo.2021.120217
Munemoto, T., Solongo, T., Okuyama, A., Fukushi, K., Yunden, A., Batbold, T., et al. (2020). Rare Earth Element Distributions in Rivers and Sediments from the Erdenet Cu-Mo Mining Area, Mongolia. Appl. Geochem. 123, 104800. doi:10.1016/j.apgeochem.2020.104800
Nations, S. L. (2009). Acute and Developmental Toxicity of Metal Oxide Nanoparticles (ZnO, TiO2, Fe2O3, and CuO) in Xenopus laevis. [dissertation/ master’s thesis]. [Texas]: Texas Tech University.
Nations, S., Wages, M., Cañas, J. E., Maul, J., Theodorakis, C., and Cobb, G. P. (2011). Acute Effects of Fe2O3, TiO2, ZnO and CuO Nanomaterials on Xenopus laevis. Chemosphere 83, 1053–1061. doi:10.1016/j.chemosphere.2011.01.061
Navarro-Espinoza, S., Meza-Figueroa, D., Guzmán, R., Duarte-Moller, A., Esparza-Ponce, H., Paz-Moreno, F., et al. (2021). Release of Nanoparticles in the Environment and Catalytic Converters Ageing. Nanomater 11, 8–15. doi:10.3390/nano11123406
Pinto-Vidal, F. A., Carvalho, C. D. S., Abdalla, F. C., Ceschi-Bertoli, L., Moraes Utsunomiya, H. S., Henrique da Silva, R., et al. (2021). Metabolic, Immunologic, and Histopathologic Responses on Premetamorphic American Bullfrog (Lithobates Catesbeianus) Following Exposure to Lithium and Selenium. Environ. Pollut. 270, 116086–116089. doi:10.1016/j.envpol.2020.116086
Pounds, J. A., Bustamante, M. R., Coloma, L. A., Consuegra, J. A., Fogden, M. P. L., Foster, P. N., et al. (2006). Widespread amphibian extinctions from epidemic disease driven by global warming. Nature 439, 161–167. doi:10.1038/nature04246
Platz, J. E., and Frost, J. S. (1984). Rana yavapaiensis, a New Species of Leopard Frog (Rana pipiens Complex). Copeia 1984, 940–948. doi:10.2307/1445338
Quik, J. T. K., Lynch, I., Hoecke, K. V., Miermans, C. J. H., Schamphelaere, K. A. C. D., Janssen, C. R., et al. (2010). Effect of Natural Organic Matter on Cerium Dioxide Nanoparticles Settling in Model Fresh Water. Chemosphere 81, 711–715. doi:10.1016/j.chemosphere.2010.07.062
Quintard, P. E., Barbéris, P., Mirgorodsky, A. P., and Merle-Méjean, T. (2002). Comparative Lattice-Dynamical Study of the Raman Spectra of Monoclinic and Tetragonal Phases of Zirconia and Hafnia. J. Am. Ceram. Soc. 85, 1745–1749. doi:10.1111/j.1151-2916.2002.tb00346.x
Rinkovec, J. (2019). Platinum, Palladium, and Rhodium in Airborne Particulate Matter. Arch. Ind. Hyg. Toxicol. 70, 224–231. doi:10.2478/aiht-2019-70-3293
Sandu, M. C., Soroaga, L. V., Balaban, S. I., Chelariu, C., Chiscan, O., Iancu, G. O., et al. (2021). Trace Elements Distribution in Stream Sediments of an Abandoned U Mining Site in the Eastern Carpathians, Romania, with Particular Focus on REEs. Geochemistry 81, 125761. doi:10.1016/j.chemer.2021.125761
Schneider, L., Haberle, S. G., Maher, W. A., Krikowa, F., Zawadzki, A., and Heijnis, H. (2016). History of Human Impact on Lake Kutubu, Papua New Guinea: The Geochemical Signatures of Oil and Gas Mining Activities in Sediments. Chemosphere 148, 369–379. doi:10.1016/j.chemosphere.2015.12.086
Shi, W., Li, T., Feng, Y., Su, H., and Yang, Q. (2022). Source Apportionment and Risk Assessment for Available Occurrence Forms of Heavy Metals in Dongdahe Wetland Sediments, Southwest of China. Sci. Total Environ. 815, 1–11. doi:10.1016/j.scitotenv.2021.152837
Sievers, M., Hale, R., Swearer, S. E., and Parris, K. M. (2018). Contaminant Mixtures Interact to Impair Predator-Avoidance Behaviours and Survival in a Larval Amphibian. Ecotoxicol. Environ. Saf. 161, 482–488. doi:10.1016/j.ecoenv.2018.06.028
Sievers, M., Hale, R., Parris, K. M., Melvin, S. D., Lanctôt, C. M., and Swearer, S. E. (2019). Contaminant-induced Behavioural Changes in Amphibians: A Meta-Analysis. Sci. Total Environ. 693, 1–11. doi:10.1016/j.scitotenv.2019.07.376
Silva, L. F. O., Hower, J. C., Dotto, G. L., Oliveira, M. L. S., and Pinto, D. (2021). Titanium Nanoparticles in Sedimented Dust Aggregates from Urban Children’s Parks Around Coal Ashes Wastes. Fuel 285, 1–6. doi:10.1016/j.fuel.2020.119162
Skubała, P., and Zaleski, T. (2012). Heavy Metal Sensitivity and Bioconcentration in Oribatid Mites (Acari, Oribatida) Gradient Study in Meadow Ecosystems. Sci. Total Environ. 414, 364–372. doi:10.1016/j.scitotenv.2011.11.006
Slaby, S., Marin, M., Marchand, G., and Lemiere, S. (2019). Exposures to Chemical Contaminants: What Can We Learn from Reproduction and Development Endpoints in the Amphibian Toxicology Literature? Environ. Pollut. 248, 478–495. doi:10.1016/j.envpol.2019.02.014
Thambirajah, A. A., Koide, E. M., Imbery, J. J., and Helbing, C. C. (2019). Contaminant and Environmental Influences on Thyroid Hormone Action in Amphibian Metamorphosis. Front. Endocrinol. 10, 1–29. doi:10.3389/fendo.2019.00276
Toussaint, A., Brosse, S., Bueno, C. G., Pärtel, M., Tamme, R., and Carmona, C. P. (2021). Extinction of Threatened Vertebrates Will Lead to Idiosyncratic Changes in Functional Diversity across the World. Nat. Commun. 12, 1–12. doi:10.1038/s41467-021-25293-0
USGS (2021). “Why Are Frog and Toad Populations Declining?,” in Science for a Changing World. Biology and Ecosystems. Available at: https://www.usgs.gov/faqs/why-are-frog-and-toad-populations-declining?qt-news_science_products=0#qt-news_science_products (Accessed February 03, 2022).
Velasco, J. A., Estrada, F., Calderón-Bustamante, O., Swingedouw, D., Ureta, C., Gay, C., et al. (2021). Synergistic Impacts of Global Warming and Thermohaline Circulation Collapse on Amphibians. Commun. Biol. 4, 141–147. doi:10.1038/s42003-021-01665-6
Vijayaraj, V., Liné, C., Cadarsi, S., Salvagnac, C., Baqué, D., Elger, A., et al. (2018). Transfer and Ecotoxicity of Titanium Dioxide Nanoparticles in Terrestrial and Aquatic Ecosystems: A Microcosm Study. Environ. Sci. Technol. 52, 12757–12764. doi:10.1021/acs.est.8b02970
Villamizar-Gomez, A., Wang, H., Peterson, M., Grant, W., and Forstner, M. (2021). Environmental Determinants of Batrachochytrium Dendrobatidis and the Likelihood of Further Dispersion in the Face of Climate Change in Texas, USA. Dis. Aquat. Org. 146, 29–39. doi:10.3354/dao03613
Walter, D. E., and Proctor, H. C. (2013). Mites: Ecology, Evolution and Behaviour. New York London: Springer Press.
Welbourn, W. C., and Loomis, R. B. (1975). Hannemania (Acarina: Trombiculidae) and Their Anuran Hosts at Fortynine Palms Oasis, Joshua Tree National Monument, Califonia. Bull. South. Calif. Acad. Sci. 74, 15–18.
Westfall, M. C., Cecala, K. K., Price, S. J., and Dorcas, M. E. (2008). Patterns of Trombiculid Mite (Hannemania Dunni) Parasitism Among Plethodontid Salamanders in the Western Piedmont of North Carolina. J. Parasitol. 94, 631–634. doi:10.1645/ge-1260r1.1
Wong, B. B. M., and Candolin, U. (2015). Behavioral Responses to Changing Environments. Behav. Ecol. 26, 665–673. doi:10.1093/beheco/aru183
Zhang, J., Wages, M., Cox, S. B., Maul, J. D., Li, Y., Barnes, M., et al. (2012). Effect of Titanium Dioxide Nanomaterials and Ultraviolet Light Coexposure on African Clawed Frogs (Xenopus laevis). Environ. Toxicol. Chem. 31, 176–183. doi:10.1002/etc.718
Keywords: mites, amphibian, vehicle emissions, ultrafine particles, cerium, titanium
Citation: Jacinto-Maldonado M, Meza-Figueroa D, Pedroza-Montero M, Lesbarrères D, Robles-Morúa A, Navarro-Espinoza S, González-Grijalva B, Pérez-Segura E, Silva-Campa E, Angulo-Molina A and Paredes-León R (2022) Mites as a Potential Path for Ce-Ti Exposure of Amphibians. Front. Environ. Sci. 10:870645. doi: 10.3389/fenvs.2022.870645
Received: 07 February 2022; Accepted: 27 May 2022;
Published: 24 June 2022.
Edited by:
Haiyan Wang, Zhejiang University, ChinaReviewed by:
Damian Lettoof, Curtin University, AustraliaShule Zhang, Nanjing University of Science and Technology, China
Muhammad Shahid, University of the Punjab, Pakistan
Copyright © 2022 Jacinto-Maldonado, Meza-Figueroa, Pedroza-Montero, Lesbarrères, Robles-Morúa, Navarro-Espinoza, González-Grijalva, Pérez-Segura, Silva-Campa, Angulo-Molina and Paredes-León. This is an open-access article distributed under the terms of the Creative Commons Attribution License (CC BY). The use, distribution or reproduction in other forums is permitted, provided the original author(s) and the copyright owner(s) are credited and that the original publication in this journal is cited, in accordance with accepted academic practice. No use, distribution or reproduction is permitted which does not comply with these terms.
*Correspondence: Diana Meza-Figueroa, diana.meza@unison.mx; Martín Pedroza-Montero, martin.pedroza@unison.mx