- Department of Atmospheric and Earth Sciences, University of Alabama in Huntsville, Huntsville, AL, United States
Reduced-nitrogen compounds (RNC), such as ammonia and amines, play important roles in atmospheric aerosol nucleation, secondary organic aerosol (SOA), and cloud formation processes. Fast measurements of ammonia and amines are made with a chemical ionization mass spectrometer (CIMS). Clusters containing RNC are measured with an atmospheric pressure interface time of flight mass spectrometer (APi-TOF) or chemical ionization APi-TOF (CI-APi-TOF). Aerosol-phase amines can be detected with a single particle mass spectrometer at real-time, or with offline chemical analytical methods using filter samples. However, the application of these instruments in real atmospheric measurements is still very limited. This perspective article highlights recent measurements of RNC in the atmosphere and discusses their implications in new particle formation (NPF).
Introduction
Hundreds of different RNC have been detected in the gas phase, aerosols, clouds, and fog droplets (Ge et al., 2010a; Ge et al., 2010b; Lee and Wexler, 2013; Nielsen, 2016; Nielsen et al., 2012; Qiu and Zhang, 2013). Ambient concentrations of gas-phase amines are typically at the sub-ppt (parts per trillion in volume mixing ratio) to tens of ppt level, about 3 orders of magnitude lower than ammonia concentrations (Ge et al., 2010b; Yu and Lee, 2012; You et al., 2014). Amines and ammonia are emitted from various natural and anthropogenic sources such as agricultural, animal husbandry, biomass burning, vegetation, soils, oceans, waste incineration, car exhausts, and power plants (Ge et al., 2010b). Recent carbon sequestration techniques also utilize solutions containing amines, representing another source of RNC in the atmosphere (Yu et al., 2017).
Amines have been identified as an important organic component in micron and sub-micron size particles under various atmospheric conditions (Silva et al., 2008; Facchini et al., 2008; Dall’Osto, 2019; Dall’Osto, 2017; Kanawade et al., 2020; Lian et al., 2020; van Pinxteren et al., 2019). Aerosol chamber studies have shown that amines can form SOA either via acid-base reactions with gas-phase inorganic acids or acidic aerosols (Murphy, 2007), or via condensation of products following oxidation reactions with OH, ozone, and NO3 (Silva et al., 2008; Malloy et al., 2009; Erupe et al., 2010). The EUPHORE (European Photochemical Reactor) experiments showed the total aerosol yields from 8 to 14% from oxidation of small amines (C1-C3) (Nielsen, 2016). Field measurements have suggested that amines may be responsible for the high content of RNC found in SOA particles (Sorooshian et al., 2007). Laboratory studies have shown the formation of light-absorbing brown organic aerosols from various amines (Galloway et al., 2014). Amines can participate in aqueous-phase reactions together with glyoxal, methylglyoxal and formaldehyde to form light-absorbing organic aerosols (De Haan et al., 2011; Kua et al., 2011).
Recent CERN CLOUD (Cosmics Leaving Outdoor Droplets) (Kirkby et al., 2011; Almeida et al., 2013) and other laboratory studies (Erupe et al., 2011; Yu et al., 2012; Jen et al., 2014; Glasoe et al., 2015; Jen et al., 2016) investigated the effects of amines on sulfuric acid aerosol nucleation. And it is currently believed that even ppt or sub-ppt levels of amines can significantly enhance nucleation rates of sulfuric acid via acid-base reactions, hence even more effectively than ammonia (Almeida et al., 2013). It has been suggested that ammonia and nitric acid can contribute to the growth of newly formed particles, under specific atmospheric conditions, e.g., with exceedingly low temperature and high concentrations of these nitrogen-containing species (Wang et al., 2020a).
Photochemical products of amines produce various air toxicants and carcinogens such as isocyanic acid, cyanates, nitrosamines, and nitramines (Lee and Wexler, 2013). For example, when amines react with atmospheric oxidants, several secondary products including amide and imine form at low NOx conditions, whereas nitrosamines, nitramines, and amine-nitrogen-oxides form at high NOx conditions (Nielsen, 2016).
Despite these important roles of RNC in air quality, human health, and climate, real-time measurements of RNC are still very limited. In the following sections, we highlight the recent measurements of RNC in the gas phase, clusters, and particle phases, and discuss their implications in the context of aerosol formation and growth.
Gas-Phase Amines and Ammonia
Fast measurements of gas-phase amines and ammonia have been made with CIMS (chemical ionization mass spectrometer) using various reagent ions, such as protonated water ions (Sellegri et al., 2005a; Hanson et al., 2011; Zheng et al., 2015; Wang et al., 2020b; Pfeifer et al., 2020), ethanol or acetone ions (Yu and Lee, 2012; You et al., 2014; Yao et al., 2016), nitrate (Simon et al., 2016), or bisulfate ions (Sipilä et al., 2015). Either a Quadrupole mass detector (Q-MS) or a high-resolution time-of-flight mass spectrometer (HrTOF-MS) is used as the detector (Lee et al., 2019). The main advantage of HrTOF-MS over Q-MS is a mass resolving power >5,000 and up to 12,000 (within m/z from zero to 4,000). CIMS can collect mass spectra at 1 Hz and detect ppt or tens ppt level of amines with the 1-min time resolution.
One of the technical challenges of the detection of nitrogen-containing compounds is the absorption (and desorption) on (and from) inlet tubing inner surfaces (Nowak et al., 2007). Thus, proper measurements of “background” (or blank) measurements are required. For example, in the setup used in Yu and Lee (2012), ambient air is introduced for either ambient or background measurements, via a 3-way valve. During background measurements, ambient air flows through a scrubber (filled with silicon phosphate) where base compounds were removed. Silicon phosphate is prone to humidity and the scrubbing efficiency can decay rapidly in the humid air. In this particular case, 10-min background measurements took place in every 1 h, while during the rest of the time, ambient measurements were made. Additionally, once every 3 h, calibrations were made simultaneously for amines and ammonia. As shown here, background ion signals were not zero and they were relatively high and even comparable to the ambient ion signals and fluctuated with ambient conditions (e.g., relative humidity). Thus, the proper background air is ambient air minus RNC, while other chemical species remain unchanged. Some studies have used synthesized zero air as the background gas. But since such zero air does not have the complex “matrix” of environmental conditions (such as temperature, humidity, and other trace gases that affect the product ion signals), such background signals can be “misleadingly” low, and this can cause an over-estimation of ambient concentrations of RNC.
Table 1 summarizes measured amine concentrations with CIMS at various locations. Using CI-APi-TOF (chemical ionization, atmospheric pressure interface, time-of-flight mass spectrometer) with ethanol ions as a reagent, Yao et al. (2016) measured abundant amines (C1, C2, an C4 were dominant), amides (C2–C4 were dominant), and imines in polluted Shanghai, China (Figure 1). There were abundant amines (C1, C2, an C4 were dominant), amides (C2–C4 were dominant), and imines, ranging from tens of ppt to the ppb level. The CI-APi-TOF can resolve amines and amides, although isomers (e.g., dimethylamine vs. ethylamine) are still not resolved (isomers can be distinguished only with tandem mass analysis). Interestingly, diurnal variations of corresponding amines and amides (e.g., C4-amines and C3-amides) showed different hourly variations, perhaps because amides are secondary products formed from oxidation reactions of amines. Diurnal variations of amines did not follow the ambient temperature, whereas amines were often correlated with the ambient temperature at some other locations, due to gas-to-particle conversion processes (You et al., 2014; Bergen et al., 2016).
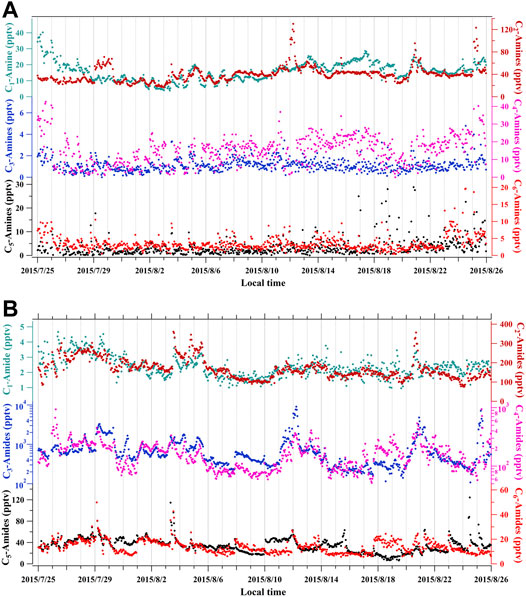
FIGURE 1. Amines (A) and amides (B) measured in the polluted urban Shanghai, China, with the ethanol CI-APi-TOF. Adapted from Yao et al. (2016).
During the winter at a highly polluted site in northern China (Hefei), using protonated water ions as a reagent, Wang et al. (2020b) showed tens of ppt of C2-amines, with the minimum concentration in the afternoon with the higher ambient temperature. With the same instrument, tens of ppb of ammonia and tens of ppt of C2-amines were measured in the polluted urban Nanjing, China (Zheng et al., 2015).
Jen et al. (2016) measured monoamines (e.g., dimethylamine and trimethylamine) and di-amines (e.g., putrescine and cadaverine) in three different environments in the United States: Atlanta, Georgia (a polluted urban city with various pollutant emissions), Lowes, Delaware (a polluted coastal site), and Lamont, Oklahoma (an agricultural site near oil extraction/refinement) with PTR-CIMS (proton-transfer chemical ionization mass spectrometer). At all three sites, these monoamines and diamines co-existed, indicating possible common emission sources for them. The polluted urban site had high concentrations of amines, even comparable to the agricultural site.
During the CLOUD experiments, dimethylamine at the ppt or sub-ppt level was measured with the ion chromatography method using two switchable particle-collection lines to provide a time resolution of about 4 h (Praplan et al., 2012). The off-line IC analytical method has been used to analyze the gas- and aerosol-phase methylamine, dimethylamine, and diethylamine over the tropical Atlantic Ocean (van Pinxteren et al., 2019).
Clusters Containing Amines and Ammonia
There are various molecular clusters in the atmosphere, either electrically charged or neutral, and even thought their concentrations are very low (ppt or even lower), they can play critical roles in aerosol nucleation and growth (Ehn et al., 2014; Bianchi et al., 2016; Yan et al., 2016; Bianchi et al., 2017). Naturally occurring charged clusters are measured with APi-TOF (atmospheric pressure interface time-of-flight mass spectrometer), where a HrTOF-MS is attached to an atmospheric pressure interface (without a CI source) (Junninen et al., 2010). Neutral clusters containing amines are measured with CI-APi-TOF using nitrate ions as s reagent (Jokinen et al., 2012). Clusters containing sulfuric acid and amines are measured with the Cluster-CIMS using nitrate or acetate ions as reagent (Zhao et al., 2010; Jen et al., 2016). The Cluster-CIMS utilizes a Q-MS, and measures small clusters, up to tetramer.
Measurements of clusters have greatly advanced atmospheric NPF studies. For example, Figure 2 shows mass defect plots of clusters containing sulfuric acid, ammonia, and HOMs (highly oxygenated organic molecules) detected with the nitrate CI-APi-TOF, one taken from the CLOUD chamber experiments during the multicomponent nucleation system experiments with sulfuric acid, ammonia, HOMs, and NOx, and another in a Finnish boreal forest during an NPF event (Lehtipalo et al., 2018). Both plots show distinctive, and very similar, clusters containing ammonia. Measurements in CLOUD also show that positive ion clusters containing HOMs always contain ammonia (Kirkby et al., 2016; Frege et al., 2018). These results strongly imply that ammonia is involved in biogenic NPF processes.
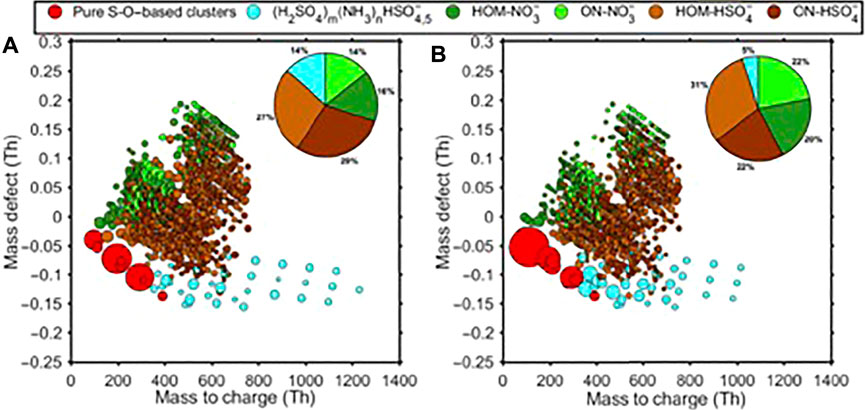
FIGURE 2. CI-Api-TOF mass spectra taken from the CLOUD chamber from a multicomponent nucleation system containing sulfuric acid, ammonia, HOMs, and NOx (A) and in a Finnish boreal forest during an NPF event (B). Adapted from Lehtipalo et al. (Lehtipalo et al., 2018).
Figure 3 shows cluster measurements in urban environments during NPF events at different urban sites: Beijing, China (Yan, 2021), Shanghai, China (Yao et al., 2016), and Barcelona, Spain (Brean et al., 2020). The mass defect plots from the three sites are surprisingly similar, despite vastly differing meteorological and geographical conditions. These studies concluded that sulfuric acid and dimethylamine are important for urban NPF. However, mass defect plots also show abundant unidentified HOMs, and this may indicate that multicomponent NPF processes involving sulfuric acid, base, and HOMs, take place in urban environments.
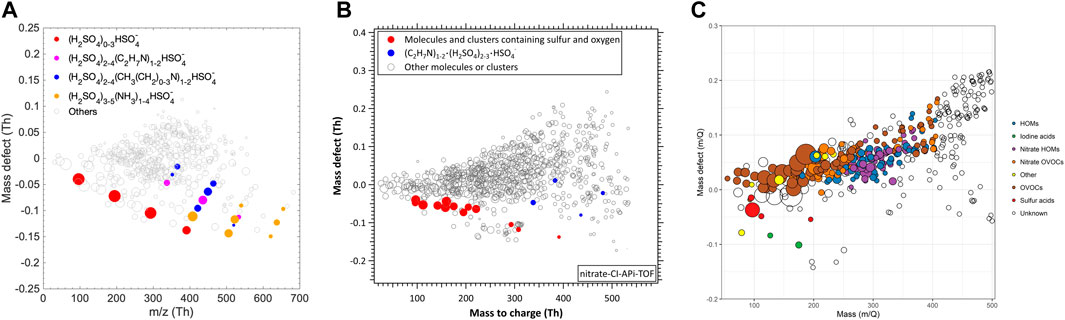
FIGURE 3. Nitrate CI-APi-TOF measurements during the NPF events in Beijing, China (A) (Yan, 2021); Shanghai, China (B) (Yao et al., 2018), and Barcelona, Spain (C) (Brean et al., 2020). Note different axes ranges.
Measurements have shown ammonia and amines in charged or natural clusters in polar regions during NPF events. For example, higher concentrations of negative ion clusters containing sulfuric acid-ammonia were measured during the NPF events than during non-events (Jokinen et al., 2018). Sulfuric acid and ammonia natural clusters were measured at an Arctic coastal site during the spring and summer (Beck, 2021). Their mass defect plots are relatively straightforward (with fewer HOMs), compared to those measured at urban locations. Brean et al. (2021) identified C2 and C4 amines (mono- or di-amines) in neutral clusters at the Antarctic Peninsula during the summer. Interestingly, with the same nitrate CI-APi-TOF instrument, Brean et al. (2021) also measured similar clusters (containing C2- and C4- amines) in urban Barcelona, Spain (Figure 3C).
Amine-containing ion clusters have been detected at a high elevation site in Jungfraujoch, Switzerland, during the NPF events (Bianchi et al., 2016). This cited study also showed distinctively different clusters containing HOMs and sulfuric acid, without ammonia (Bianchi et al., 2016). So far, this is the only study that has provided relatively clear evidence of different NPF processes from cluster chemical composition measurements.
Aerosol-phase Amines
Aerosol-phase amines were detected by fast-response, real-time single particle mass spectrometers (Dall’Osto, 2019; Dall’Osto, 2017; Lian et al., 2020). Single particle instruments are based on laser ablation and laser ionization methods and provide detailed chemical information of a variety of aerosol compositions (Lee and Allen, 2012), but these instruments are less quantitative, for example, compared to off-line chemical analytical methods (e.g., ion chromatography).
Using a SPAMS (single particle aerosol mass spectrometer) instrument, Lian et al. (2020) classified aerosol particles measured in polluted urban Guangzhou in southeastern China into 8 different chemical categories (Figure 4). One category was referred to as “amine-rich,” where various amines co-existed with sulfate and nitrate chemical components; but all other 7 categories of particles also contained some type of amines. Trimethylamine and diethylamine were most common in aerosol particles at this urban site. Amine ions were detected at m/z values corresponding to the amine chemical formula, perhaps indicating that aerosol amines were formed via acid-base reactions (rather than by condensation of amine oxidation products).
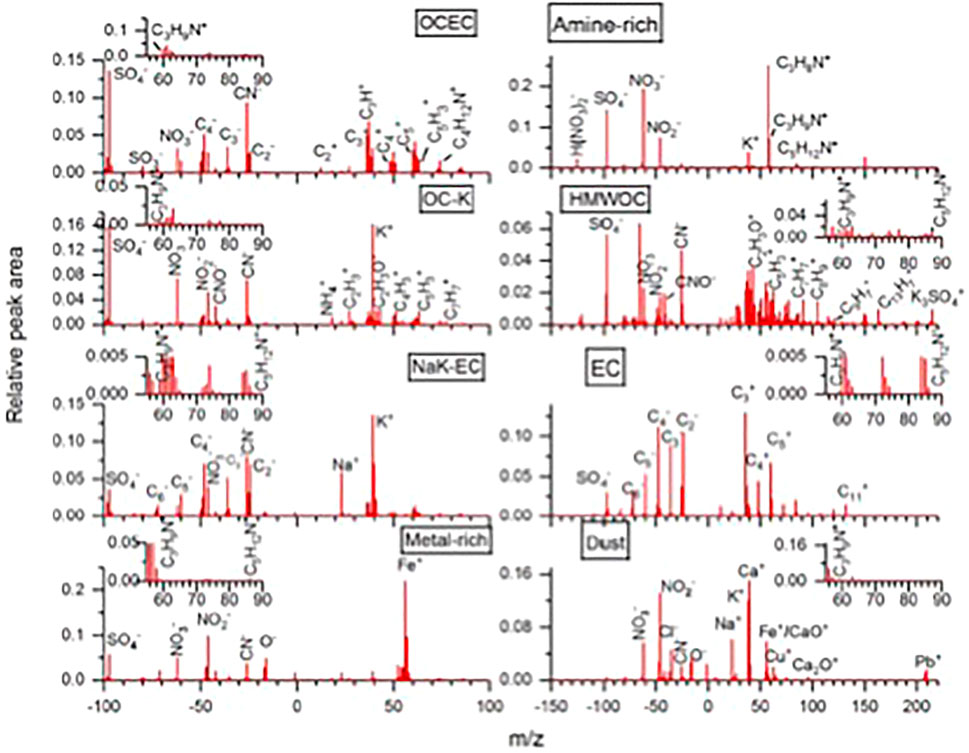
FIGURE 4. Chemical composition of aerosol particles measured with ATOFMS in urban Guangzhou, China (Lian et al., 2020).
Measurements with the single particle ALABAMA (aircraft-based laser ablation aerosol mass spectrometer) instrument showed summertime amine particles in the Canadian Arctic lower troposphere (Köllner et al., 2017). About 20% of the measured particles contained trimethylamine. This cited study showed that aerosol-phase amines formed via gas-to-particle conversion of amines emitted from marine biological sources.
TDCIMS (thermal desorption chemical ionization mass spectrometer) is an in-situ aerosol mass spectrometer, which utilizes thermal desorption to vaporize an ensemble of chemical species from the aerosol phase during an extended time (e.g., hours), followed by chemical ionization (Smith et al., 2004; Hodshire et al., 2016). Although this instrument is not fully calibrated for individual chemical composition, it can provide relatively qualitative information for chemical components because of the soft (chemical) ionization (unlike single particle laser ionization mass spectrometers discussed above). Hodshire et al. (2016) have shown simultaneous measurements of aerosol- and aerosol-phase amines with TDCIMS (Smith et al., 2004) and PTR-MS (Hanson et al., 2011), respectively, along with sulfuric acid clusters with the Cluster-CIMS instrument (Zhao et al., 2010), to study NPF processes at an agricultural site in Southern Great Plains, Oakland.
Aerosol-phase amines have been measured also with off-line methods, such as IC, GC (gas chromatography), LC-MS (liquid chromatography-mass spectrometer), and FT-IR (Fourier transform infrared spectroscopy), for aerosol samples collected on filters. The IC method coupled with MOUDI (micro-orifice uniform deposit impactor) samples were used to make size-resolved aerosol chemical analyses for dimethylamine and trimethylamine in marine environments (Hu et al., 2018; Xie et al., 2018; Zhou et al., 2019). Zhou et al. (2019) made MOUDI-IC measurements at three different sites: very polluted coastal urban Shanghai in China, an island near Shanghai, and over the Yellow Sea and the East China Sea. The aerosol-phase dimethylamine was highest over the remote open sea, whereas ammonium was highest at the polluted urban site. Aliphatic and aromatic amines in aerosol particles have been detected with LC-MS using different ionization methods, such as ESI (electrospray ionization), APCI (atmospheric pressure ionization), and photoionization (Ruiz-Jiménez et al., 2011; Ruiz-Jiménez et al., 2012).
Choi et al. (2020) have used the GC coupled with a tandem mass spectrometer to detect various amines, nitrosamines, and nitramines at an urban site in South Korea. The unique capability of tandem mass spectrometry is that it can identify molecular structures of chemical compounds so that isomers (ethylamine vs. dimethylamine) can be resolved. Additionally, FT-IR has been also used to detect chemical speciation of aerosol-phase amines at various locations (You et al., 2014; Kamruzzaman et al., 2018).
Discussion
Observations show that in general abundant gas-phase amines and ammonia in urban and polluted environments. But there is still a limited number of simultaneous measurements of NPF and these base species. These limited observations usually do not show a strong correlation of dimethylamine (or ammonia) and new particles, although laboratory studies show dimethylamine is important for sulfuric acid nucleation (Almeida et al., 2013). It has been suggested that NPF is initiated by sulfuric acid-dimethylamine nucleation at urban sites (especially, in Chinese mega-cities). Amine-containing clusters have been detected at urban sites, but there were more abundant HOMs, indicating that HOMs also play key roles in urban NPF. The mass defect plots are too complicated and many chemical identities of HOMs are not known, thus it is difficult to understand the roles that HOMs play in urban NPF. An important question related to urban air NPF is what is the role of ammonia in urban nucleation? Considering the synergetic effects of ammonia and amines on sulfuric acid nucleation (Yu et al., 2012), as well as the high potential of di-amines in aerosol nucleation (Jen et al., 2016), it is very likely that the current NPF parameterizations that use only dimethylamine (Sellegri et al., 2005b; Nowak et al., 2006; Benson et al., 2010; Gordon et al., 2017) can under-predict NPF rates in the atmosphere.
Whereas in polar regions, relatively distinctive sulfuric acid-base clusters have been detected during the NPF events. Amines were frequently observed in the aerosol phase in ocean/marine environments. These observations perhaps reinforce the notion that amines are important chemical components for aerosol formation and growth in marine/polar environments.
Chamber studies have shown oxidation products of amines, such as imines, can have a high potential for SOA formation and aerosol nucleation (Nielsen, 2016). Nitrosamines and nitramines (both carcinogens) are important byproducts of amine-based carbon capture facilities (Yu et al., 2017). Analytical methods that can measure these secondary RNC are required to measure them at the atmospheric trace level. Atmospheric measurements of RNC are currently very limited, as these measurements are often very time-consuming and technically challenging. It will be important to develop low-cost, portable, and miniature-size instruments. This will allow measurements in developing countries, indoor environments, remote regions (e.g., high altitudes), and unmanned vehicles (e.g., balloons).
Data Availability Statement
The original contributions presented in the study are included and further inquiries can be directed to the corresponding author.
Author Contributions
S-HL reviewed the literature and wrote the article.
Funding
This work was supported by the National Science Foundation (Nos. 2107916, 2111791 and 1649694).
Conflict of Interest
The author declares that the research was conducted in the absence of any commercial or financial relationships that could be construed as a potential conflict of interest.
Publisher’s Note
All claims expressed in this article are solely those of the authors and do not necessarily represent those of their affiliated organizations, or those of the publisher, the editors and the reviewers. Any product that may be evaluated in this article, or claim that may be made by its manufacturer, is not guaranteed or endorsed by the publisher.
References
Almeida, J., Schobesberger, S., Kürten, A., Ortega, I. K., Kupiainen-Määttä, O., Praplan, A. P., et al. (2013). Molecular Understanding of Sulphuric Acid-Amine Particle Nucleation in the Atmosphere. Nature 502, 359–363. doi:10.1038/nature12663
Beck, L. J. (2021). Differing Mechanisms of New Particle Formation at Two Arctic Sites. Geophys. Res. Lett. 48, e2020GL091334. doi:10.1029/2020gl091334
Benson, D. R., Al-Refai, M., and Lee, S.-H. (2010). Chemical Ionization Mass Spectrometer (CIMS) for Ambient Measurments of Ammonia. Atmos. Meas. Tech. 3, 1133–1162. doi:10.5194/amt-3-1075-2010
Bergen, A. A., Heinritzi, M., Leiminger, M., Lorenz, V., Piel, F., Simon, M., et al. (2016). Observation of New Particle Formation and Measurement of Sulfuric Acid, Ammonia, Amines and Highly Oxidized Organic Molecules at a Rural Site in central Germany. Atmos. Chem. Phys. 16, 12793–12813. doi:10.5194/acp-16-12793-2016
Bianchi, F., Garmash, O., He, X., Yan, C., Iyer, S., Rosendahl, I., et al. (2017). The Role of Highly Oxygenated Molecules (HOMs) in Determining the Composition of Ambient Ions in the Boreal forest. Atmos. Chem. Phys. 17, 13819–13831. doi:10.5194/acp-17-13819-2017
Bianchi, F., Tröstl, J., Junninen, H., Frege, C., Henne, S., Hoyle, C. R., et al. (2016). New Particle Formation in the Free Troposphere: A Question of Chemistry and Timing. Science 352, 1109–1112. doi:10.1126/science.aad5456
Brean, J., Beddows, D. C. S., Shi, Z., Temime-Roussel, B., Marchand, N., Querol, X., et al. (2020). Molecular Insights into New Particle Formation in Barcelona, Spain. Atmos. Chem. Phys. 20, 10029–10045. doi:10.5194/acp-20-10029-2020
Brean, J., Dall’Osto, M., Simó, R., Shi, Z., Beddows, D. C. S., and Harrison, R. M. (2021). Open Ocean and Coastal New Particle Formation from Sulfuric Acid and Amines Around the Antarctic Peninsula. Nat. Geosci. 14, 383–388. doi:10.1038/s41561-021-00751-y
Choi, N. R., Lee, J. Y., Ahn, Y. G., and Kim, Y. P. (2020). Determination of Atmospheric Amines at Seoul, South Korea via Gas Chromatography/tandem Mass Spectrometry. Chemosphere 258, 127367. doi:10.1016/j.chemosphere.2020.127367
Dall’Osto, M. (2017). Antarctic Sea Ice Region as a Source of Biogenic Organic Nitrogen in Aerosols. Scientific Rep. 7, 6047.
Dall’Osto, M. (2019). Simultaneous Detection of Alkylamines in the Surface Ocean and Atmosphere of the Antarctic Sympagic Environment. ACS Earth Space Chem. 3, 854–862.
De Haan, D. O., Hawkins, L. N., Kononenko, J. A., Turley, J. J., Corrigan, A. L., Tolbert, M. A., et al. (2011). Formation of Nitrogen-Containing Oligomers by Methylglyoxal and Amines in Simulated Evaporating Cloud Droplets. Environ. Sci. Technol. 45, 984–991. doi:10.1021/es102933x
Ehn, M., Thornton, J. A., Kleist, E., Sipilä, M., Junninen, H., Pullinen, I., et al. (2014). A Large Source of Low-Volatility Secondary Organic Aerosol. Nature 506, 476–479. doi:10.1038/nature13032
Erupe, M. E., Liberman-Martin, A., Silva, P. J., Malloy, Q. G. J., Yonis, N., Cocker, D. R., et al. (2010). Determination of Methylamines and Trimethylamine-N-Oxide in Particulate Matter by Non-suppressed Ion Chromatography. J. Chromatogr. A 1217, 2070–2073. doi:10.1016/j.chroma.2010.01.066
Erupe, M. E., Viggiano, A. A., and Lee, S.-H. (2011). The Effect of Trimethylamine on Atmospheric Nucleation Involving H2SO4. Atmos. Chem. Phys. 11, 4767–4775. doi:10.5194/acp-11-4767-2011
Facchini, M. C., Decesari, S., Rinaldi, M., Carbone, C., Finessi, E., Mircea, M., et al. (2008). Important Source of marine Secondary Organic Aerosol from Biogenic Amines. Environ. Sci. Technol. 42, 9116–9121. doi:10.1021/es8018385
Frege, C., Ortega, I. K., Rissanen, M. P., Praplan, A. P., Steiner, G., Heinritzi, M., et al. (2018). Influence of Temperature on the Molecular Composition of Ions and Charged Clusters during Pure Biogenic Nucleation. Atmos. Chem. Phys. 18, 65–79. doi:10.5194/acp-18-65-2018
Galloway, M. M., Powelson, M. H., Sedehi, N., Wood, S. E., Millage, K. D., Kononenko, J. A., et al. (2014). Secondary Organic Aerosol Formation during Evaporation of Droplets Containing Atmospheric Aldehydes, Amines, and Ammonium Sulfate. Environ. Sci. Technol. 48, 14417–14425. doi:10.1021/es5044479
Ge, X., Wexler, A. S., and Clegg, S. L. (2010). Atmospheric Amines - Part I: A Review. Atmos. Environ. 45, 524–546.
Ge, X., Wexler, A. S., and Clegg, S. L. (2010). Atmospheric Amines - Part II: Thermodynamic Properties and Gas/particle Partitioning. Atmos. Environ. 45, 561–577.
Glasoe, W. A., Volz, K., Panta, B., Freshour, N., Bachman, R., Hanson, D. R., et al. (2015). Sulfuric Acid Nucleation: an Experimental Study of the Effect of Seven Bases. J. Geophys. Res. Atmos. 120, 1933–1950. doi:10.1002/2014jd022730
Gordon, H., Kirkby, J., Baltensperger, U., Bianchi, F., Breitenlechner, M., Curtius, J., et al. (2017). Causes and Importance of New Particle Formation in the Present-Day and Preindustrial Atmospheres. J. Geophys. Res. Atmos. 122, 8739–8760. doi:10.1002/2017jd026844
Hanson, D. R., McMurry, P. H., Jiang, J., Tanner, D., and Huey, L. G. (2011). Ambient Pressure Proton Transfer Mass Spectrometry: Detection of Amines and Ammonia. Environ. Sci. Technol. 45, 8881–8888. doi:10.1021/es201819a
Hodshire, A. L., Lawler, M. J., Zhao, J., Ortega, J., Jen, C., Yli-Juuti, T., et al. (2016). Multiple New-Particle Growth Pathways Observed at the US DOE Southern Great Plains Field Site. Atmos. Chem. Phys. 16, 9321–9348. doi:10.5194/acp-16-9321-2016
Hu, Q., Qu, K., Gao, H., Cui, Z., Gao, Y., Yao, X., et al. (2018). Large Increases in Primary Trimethylaminium and Secondary Dimethylaminium in Atmospheric Particles Associated with Cyclonic Eddies in the Northwest Pacific Ocean. J. Geophys. Res. Atmospheres 123 (12133-112), 146. doi:10.1029/2018jd028836
Jen, C. N., Bachman, R., Zhao, J., McMurry, P. H., and Hanson, D. R. (2016). Diamine‐sulfuric Acid Reactions Are a Potent Source of New Particle Formation. Geophys. Res. Lett. 43, 867–873. doi:10.1002/2015gl066958
Jen, C. N., McMurry, P. H., and Hanson, D. R. (2014). Stabilization of Sulfuric Acid Dimers by Ammonia, Methylamine, Dimethylamine, and Trimethylamine. J. Geophys. Res. Atmos. 119, 7502–7514. doi:10.1002/2014jd021592
Jokinen, T., Sipilä, M., Kontkanen, J., Vakkari, V., Tisler, P., Duplissy, E. M., et al. (2018). Ion-induced Sulfuric Acid-Ammonia Nucleation Drives Particle Formation in Coastal Antarctica. Sci. Adv. 4, eaat9744. doi:10.1126/sciadv.aat9744
Jokinen, T., Sipilä, M., Junninen, H., Ehn, M., Lönn, G., Hakala, J., et al. (2012). Atmospheric Sulphuric Acid and Neutral Cluster Measurements Using CI-APi-TOF. Atmos. Chem. Phys. 12, 4117–4125. doi:10.5194/acp-12-4117-2012
Junninen, H., Ehn, M., Petäjä, T., Luosujärvi, L., Kotiaho, T., Kostiainen, R., et al. (2010). A High-Resolution Mass Spectrometer to Measure Atmospheric Ion Composition. Atmos. Meas. Tech. 3, 1039–1053. doi:10.5194/amt-3-1039-2010
Kamruzzaman, M., Takahama, S., and Dillner, A. M. (2018). Quantification of Amine Functional Groups and Their Influence on OM/OC in the IMPROVE Network. Atmos. Environ. 172, 124–132. doi:10.1016/j.atmosenv.2017.10.053
Kanawade, V. P., Tripathi, S. N., Chakraborty, A., and Yu, H. (2020). Chemical Characterization of Sub-micron Aerosols during New Particle Formation in an Urban Atmosphere. Aerosol Air Qual. Res. 20, 1294–1305. doi:10.4209/aaqr.2019.04.0196
Kirkby, J., Curtius, J., Almeida, J., Dunne, E., Duplissy, J., Ehrhart, S., et al. (2011). Role of Sulphuric Acid, Ammonia and Galactic Cosmic Rays in Atmospheric Aerosol Nucleation. Nature 476, 429–433. doi:10.1038/nature10343
Kirkby, J., Duplissy, J., Sengupta, K., Frege, C., Gordon, H., Williamson, C., et al. (2016). Ion-induced Nucleation of Pure Biogenic Particles. Nature 533, 521–526. doi:10.1038/nature17953
Köllner, F., Schneider, J., Willis, M. D., Klimach, T., Helleis, F., Bozem, H., et al. (2017). Particulate Trimethylamine in the Summertime Canadian High Arctic Lower Troposphere. Atmos. Chem. Phys. 17, 13747–13766. doi:10.5194/acp-17-13747-2017
Kua, J., Krizner, H. E., and De Haan, D. O. (2011). Thermodynamics and Kinetics of Imidazole Formation from Glyoxal, Methylamine, and Formaldehyde: A Computational Study. J. Phys. Chem. A. 115, 1667–1675. doi:10.1021/jp111527x
Lee, D., and Wexler, A. S. (2013). Atmospheric Amines - Part III: Photochemistry and Toxicity. Atmos. Environ. 71, 95–103. doi:10.1016/j.atmosenv.2013.01.058
Lee, S.-H., and Allen, H. C. (2012). Analytical Measurements of Atmospheric Urban Aerosol. Anal. Chem. 84, 1196–1201. doi:10.1021/ac201338x
Lee, S. H., Gordon, H., Yu, H., Lehtipalo, K., Haley, R., Li, Y., et al. (2019). New Particle Formation in the Atmosphere: From Molecular Clusters to Global Climate. J. Geophys. Res. Atmos. 124, 7098–7146. doi:10.1029/2018jd029356
Lehtipalo, K., Yan, C., Dada, L., Bianchi, F., Xiao, M., Wagner, R., et al. (2018). Multicomponent New Particle Formation from Sulfuric Acid, Ammonia, and Biogenic Vapors. Sci. Adv. 4, eaau5363. doi:10.1126/sciadv.aau5363
Lian, X., Zhang, G., Lin, Q., Liu, F., Peng, L., Yang, Y., et al. (2020). Seasonal Variation of Amine-Containing Particles in Urban Guangzhou, China. Atmos. Environ. 222, 117102. doi:10.1016/j.atmosenv.2019.117102
Yao, L., Garmash, O., Bianchi, F., Zheng, J., Yan, C., Kontkanen, J., et al. (2018). Atmospheric New Particle Formation from Sulfuric Acid and Amines in a Chinese Megacity. Science 361, 278-281. doi:10.1126/science.aao4839
Malloy, Q. G. J., Warren, B., Cocker III, D. R., Erupe, M. E., and Silva, P. J. (2009). Secondary Organic Aerosol Formation from Primary Aliphatic Amines with NO3 Radical. Atmos. Chem. Phys. 9, 2051–2060. doi:10.5194/acp-9-2051-2009
Murphy, S. M. (2007). Secondary Aerosol Formation from Atmospheric Reactions of Aliphatic Amines. Atmos. Chem. Phys. 7, 289–349. doi:10.5194/acp-7-2313-2007
Nielsen, C. J. (2016). Atmospheric Degradation of Amines (ADA) Summary Report: Photo-Oxidation of Methylamine, Dimethylamine and Trimethylamine CLIMIT Project No. Norge: Norsk Institutt for Luftforskning.
Nielsen, C. J., Herrmann, H., and Weller, C. (2012). Atmospheric Chemistry and Environmental Impact of the Use of Amines in Carbon Capture and Storage (CCS). Chem. Soc. Rev. 41, 6684–6704. doi:10.1039/c2cs35059a
Nowak, J. B., Huey, L. G., Russell, A. G., Tian, D., Neuman, J. A., Orsini, D., et al. (2006). Analysis of Urban Gas Phase Ammonia Measurements from the 2002 Atlanta Aerosol Nucleation and Real-Time Characterization Experiment (ANARChE). J. Geophys. Res. 111. doi:10.1029/2006JD007113
Nowak, J. B., Neuman, J. A., Kozai, K., Huey, L. G., Tanner, D. J., Holloway, J. S., et al. (2007). A Chemical Ionization Mass Spectrometry Technique for Airborne Measurements of Ammonia. J. Geophys. Res. 112. doi:10.1029/2006JD007589
Pfeifer, J., Simon, M., Heinritzi, M., Piel, F., Weitz, L., Wang, D., et al. (2020). Measurement of Ammonia, Amines and Iodine Compounds Using Protonated Water Cluster Chemical Ionization Mass Spectrometry. Atmos. Meas. Tech. 13, 2501–2522. doi:10.5194/amt-13-2501-2020
Praplan, A. P., Bianchi, F., Dommen, J., and Baltensperger, U. (2012). Dimethylamine and Ammonia Measurements with Ion Chromatography during the CLOUD4 Campaign. Atmos. Meas. Tech. 5, 2161–2167. doi:10.5194/amt-5-2161-2012
Qiu, C., and Zhang, R. (2013). Multiphase Chemistry of Atmospheric Amines. Phys. Chem. Chem. Phys. 15, 5738–5752. doi:10.1039/c3cp43446j
Ruiz-Jiménez, J., Hautala, S., Parshintsev, J., Laitinen, T., Hartonen, K., Petäjä, T., et al. (2012). Aliphatic and Aromatic Amines in Atmospheric Aerosol Particles: Comparison of Three Ionization Techniques in Liquid Chromatography-Mass Spectrometry and Method Development. Talanta 97, 55–62. doi:10.1016/j.talanta.2012.03.062
Ruiz-Jiménez, J., Parshintsev, J., Laitinen, T., Hartonen, K., Riekkola, M. L., Petäjä, T., et al. (2011). A Complete Methodology for the Reliable Collection, Sample Preparation, Separation and Determination of Organic Compounds in Ultrafine 30 Nm, 40 Nm and 50 Nm Atmospheric Aerosol Particles. Analy. Methods 3, 2501–2509. doi:10.1039/c1ay05362k
Sellegri, K., Hanke, M., Umann, B., Arnold, F., and Kulmala, M. (2005). Measurements of Organic Gases during Aerosol Formation Events in the Boreal forest Atmosphere during QUEST. Atmos. Chem. Phys. 5, 373–384. doi:10.5194/acp-5-373-2005
Sellegri, K., Umann, B., Hanke, M., and Arnold, F. (2005). Deployment of a Ground-Based CIMS Apparatus for the Detection of Organic Gases in the Boreal forest during the QUEST Campaign. Atmos. Chem. Phys. 5, 357–372. doi:10.5194/acp-5-357-2005
Silva, P. J., Erupe, M. E., Price, D., Elias, J., G. J. Malloy, Q., Li, Q., et al. (2008). Trimethylamine as Precursor to Secondary Organic Aerosol Formation via Nitrate Radical Reaction in the Atmosphere. Environ. Sci. Technol. 42, 4689–4696. doi:10.1021/es703016v
Simon, M., Heinritzi, M., Herzog, S., Leiminger, M., Bianchi, F., Praplan, A., et al. (2016). Detection of Dimethylamine in the Low Pptv Range Using Nitrate Chemical Ionization Atmospheric Pressure Interface Time-Of-Flight (CI-APi-TOF) Mass Spectrometry. Atmos. Meas. Tech. 9, 2135–2145. doi:10.5194/amt-9-2135-2016
Sipilä, M., Sarnela, N., Jokinen, T., Junninen, H., Hakala, J., Rissanen, M. P., et al. (2015). Bisulfate - Cluster Based Atmospheric Pressure Chemical Ionization Mass Spectrometer for High-Sensitivity (. Atmos. Meas. Tech. 8, 4001–4011. doi:10.5194/amt-8-4001-2015
Smith, J. N., Moore, K. F., McMurry, P. H., and Eisele, F. L. (2004). Atmospheric Measurements of Sub-20 Nm Diameter Particle Chemical Composition by Thermal Desorption Chemical Ionization Mass Spectrometry. Aerosol Sci. Tech. 38, 100–110. doi:10.1080/02786820490249036
Sorooshian, A., Ng, N. L., Chan, A. W. H., Feingold, G., Flagan, R. C., and Seinfeld, J. H. (2007). Particulate Organic Acids and Overall Water‐soluble Aerosol Composition Measurements from the 2006 Gulf of Mexico Atmospheric Composition and Climate Study (GoMACCS). J. Geophys. Res. 112. doi:10.1029/2007jd008537
van Pinxteren, M., Fomba, K. W., van Pinxteren, D., Triesch, N., Hoffmann, E. H., Cree, C. H. L., et al. (2019). Aliphatic Amines at the Cape Verde Atmospheric Observatory: Abundance, Origins and Sea-Air Fluxes. Atmos. Environ. 203, 183–195. doi:10.1016/j.atmosenv.2019.02.011
Wang, M., Kong, W., Marten, R., He, X. C., Chen, D., Pfeifer, J., et al. (2020). Rapid Growth of New Atmospheric Particles by Nitric Acid and Ammonia Condensation. Nature 581, 184–189. doi:10.1038/s41586-020-2270-4
Wang, Y., Yang, G., Lu, Y., Liu, Y., Chen, J., and Wang, L. (2020). Detection of Gaseous Dimethylamine Using Vocus Proton-Transfer-Reaction Time-Of-Flight Mass Spectrometry. Atmos. Environ. 243, 117875. doi:10.1016/j.atmosenv.2020.117875
Xie, H., Feng, L., Hu, Q., Zhu, Y., Gao, H., Gao, Y., et al. (2018). Concentration and Size Distribution of Water-Extracted Dimethylaminium and Trimethylaminium in Atmospheric Particles during Nine Campaigns - Implications for Sources, Phase States and Formation Pathways. Sci. Total Environ. 631-632, 130–141. doi:10.1016/j.scitotenv.2018.02.303
Yan, C., Nie, W., Äijälä, M., Rissanen, M. P., Canagaratna, M. R., Massoli, P., et al. (2016). Source Characterization of Highly Oxidized Multifunctional Compounds in a Boreal forest Environment Using Positive Matrix Factorization. Atmos. Chem. Phys. 16, 12715–12731. doi:10.5194/acp-16-12715-2016
Yan, C. (2021). The Synergistic Role of Sulfuric Acid, Bases, and Oxidized Organics Governing New-Particle Formation in Beijing. Geophys. Res. Lett. 48, e2020GL091944. doi:10.1029/2020gl091944
Yao, L., Wang, M.-Y., Wang, X.-K., Liu, Y.-J., Chen, H.-F., Zheng, J., et al. (2016). Detection of Atmospheric Gaseous Amines and Amides by a High-Resolution Time-Of-Flight Chemical Ionization Mass Spectrometer with Protonated Ethanol Reagent Ions. Atmos. Chem. Phys. 16, 14527–14543. doi:10.5194/acp-16-14527-2016
You, Y., Kanawade, V. P., de Gouw, J. A., Guenther, A. B., Madronich, S., Sierra-Hernández, M. R., et al. (2014). Atmospheric Amines and Ammonia Measured with a Chemical Ionization Mass Spectrometer (CIMS). Atmos. Chem. Phys. 14, 12181–12194. doi:10.5194/acp-14-12181-2014
Yu, H., and Lee, S.-H. (2012). Chemical Ionisation Mass Spectrometry for the Measurement of Atmospheric Amines. Environ. Chem. 9, 190–201. doi:10.1071/en12020
Yu, H., McGraw, R., and Lee, S.-H. (2012). Effects of Amines on Formation of Sub-3 Nm Particles and Their Subsequent Growth. Geophys. Res. Lett. 39, a–n. doi:10.1029/2011gl050099
Yu, K., Mitch, W. A., and Dai, N. (2017). Nitrosamines and Nitramines in Amine-Based Carbon Dioxide Capture Systems: Fundamentals, Engineering Implications, and Knowledge Gaps. Environ. Sci. Technol. 51, 11522–11536. doi:10.1021/acs.est.7b02597
Zhao, J., Eisele, F. L., Titcombe, M., Kuang, C., and McMurry, P. H. (2010). Chemical Ionization Mass Spectrometric Measurements of Atmospheric Neutral Clusters Using the Cluster-CIMS. J. Geophys. Res. 115. doi:10.1029/2009JD012606
Zheng, J., Ma, Y., Chen, M., Zhang, Q., Wang, L., Khalizov, A. F., et al. (2015). Measurement of Atmospheric Amines and Ammonia Using the High Resolution Time-Of-Flight Chemical Ionization Mass Spectrometry. Atmos. Environ. 102, 249–259. doi:10.1016/j.atmosenv.2014.12.002
Zhou, S., Li, H., Yang, T., Chen, Y., Deng, C., Gao, Y., et al. (2019). Characteristics and Sources of Aerosol Aminiums over the Eastern Coast of China: Insights from the Integrated Observations in a Coastal City, Adjacent Island and Surrounding Marginal Seas. Atmos. Chem. Phys. 19, 10447–10467. doi:10.5194/acp-19-10447-2019
Keywords: amine, ammonia, new particle formation, urban, marine, CIMS, API-TOF, CI-APi-TOF
Citation: Lee S-H (2022) Perspective on the Recent Measurements of Reduced Nitrogen Compounds in the Atmosphere. Front. Environ. Sci. 10:868534. doi: 10.3389/fenvs.2022.868534
Received: 02 February 2022; Accepted: 14 March 2022;
Published: 06 April 2022.
Edited by:
Bin Yuan, Jinan University, ChinaReviewed by:
Lei Yao, University of Helsinki, FinlandHuan Yu, China University of Geosciences Wuhan, China
Copyright © 2022 Lee. This is an open-access article distributed under the terms of the Creative Commons Attribution License (CC BY). The use, distribution or reproduction in other forums is permitted, provided the original author(s) and the copyright owner(s) are credited and that the original publication in this journal is cited, in accordance with accepted academic practice. No use, distribution or reproduction is permitted which does not comply with these terms.
*Correspondence: S.-H. Lee, c2hhbmh1LmxlZUB1YWguZWR1