- 1Department of Meteorology and Climatology, Nicolaus Copernicus University, Toruń, Poland
- 2Centre of Climate Change Research, Nicolaus Copernicus University, Toruń, Poland
The paper presents results describing the influence of Atmospheric Circulation (AC) on meteorological conditions on Kaffiøyra (NW Spitsbergen, Svalbard Archipelago) in 23 summer seasons (July 21–August 31) in the years 1975, 1977–80, 1982, 1985, 1989, 1997–2000, 2005–15. The analysis covered the diurnal sums or means of major meteorological parameters: total cloudiness, sunshine duration, air temperature, wind speed, relative air humidity, water vapour pressure, and precipitation. Extreme weather events in terms of any given parameter were defined as days whose diurnal values (mean/sum) were in the ≤5th or ≥95th percentiles. The influence of AC on meteorological conditions on Kaffiøyra was analysed using the calendar of circulation types (CT) by T. Niedźwiedź et al. (Calendar of atmospheric circulation types for Spitsbergen–a digital dataset, 2018). In the study area, the variability of individual meteorological parameters depends primarily on air-mass advection direction, while type of baric regime is less important. Our study highlights that the greatest positive anomalies and a significant frequency of extreme values of cloudiness, wind speed, air temperature, humidity and precipitation occurred during air mass advection mainly from the SW and S. It was also demonstrated that sunshine duration correlated statistically significantly with the frequency of the anticyclonic macrotype, and precipitation with the cyclonic macrotype. The results confirmed that atmospheric circulation plays the most important role in shaping weather conditions in Spitsbergen.
Introduction
Meteorological conditions are determined not only by radiative factors, but also by Atmospheric Circulation (AC), which depends on pressure field configuration, baric centre type and frequency of occurrence of air mass types. AC plays a much greater role in shaping weather and climate in the Norwegian Arctic than elsewhere on the globe. Climate model studies have shown that changes in response to climate changes are strongest and quickest in the Arctic (e.g., Turner and Marshall 2011; Przybylak 2016; Łupikasza et al., 2021). A characteristic feature of AC over Spitsbergen is its increased cyclonic activity, conditioned by the island’s location near the Arctic front (Niedźwiedź 2013a). Over the Norwegian Arctic, the Icelandic Low and the Greenland High are the main centres of atmospheric activity shaping the climate. These two semi-permanent centres determine the movement of air masses. The research area lies in the path of deep lows that form in the Iceland region and pass via Bear Island or Spitsbergen towards Novaya Zemlya (Käsmacher and Schneider 2011; Przybylak 2016). This results in a high diversity of incoming air masses in the region. Active lows from temperate latitudes often enter Arctic areas (Niedźwiedź 1992; Turner and Marshall 2011), and local low-pressure centres may also form, especially in the summer (Dzerdzeevskii 1975; Hanssen-Bauer et al., 2019). AC also affects the extent of sea ice in the Arctic (Ding et al., 2017). In addition, changes happening in the Arctic are associated not only with positive feedback mechanisms—“Arctic amplification” (Serreze and Barry 2011)—but also with changes in the extent of sea ice (Ding et al., 2017) and the reshaping of the pressure field and atmospheric circulation (Przybylak 2002; Łupikasza et al., 2021).
On the other hand, radiation factors associated with the phenomenon of polar day and night remain stable for much of the year, so their impact on weather variability stays in the background (Niedźwiedź 1993). Therefore, the most important role in shaping the variability of climatic conditions in the Norwegian Arctic is played by AC (Przybylak 2002; Araźny 2008). However, Isaksen et al. (2016) consider that the strong warming in Spitsbergen in recent decades is not driven by increased frequencies of “warm” AC types but rather by sea-ice decline and high sea-surface temperatures.
Extreme meteorological phenomena have a special place in research on climate change. Changes in the average values of climate parameters also affect the frequency and intensity of extreme events (Przybylak 2016; IPCC 2021). Svalbard witnessed an annual mean temperature rise of 3°C–5°C for the last 50°years (Hanssen-Bauer et al., 2019; Nordli et al., 2020), with winter warm spells becoming a more frequent and extreme weather event (Hansen et al., 2014; Peeters et al., 2019). For example, on Spitsbergen (in Ny-Ålesund) on 7 November 2016, there was an extreme precipitation of 86.8 mm within 24 h. This precipitation value was above the estimated 100°years return period value (Hanssen-Bauer et al., 2019). The emergence of increasingly frequent precipitation is causing, among other things, the formation of avalanches in Spitsbergen (Indreiten and Svarstad 2016).
Many works on Arctic climatic conditions and the mechanisms controlling its climatic processes have been published. An overview of the most important literature in this field can be found in, for example, Przybylak (2002); Bobylev et al. (2003); Turner and Marshall (2011); Lemke and Jacobi (2012); Serreze and Barry (2014); Przybylak (2016).
A particularly large number of studies have related to the Norwegian Arctic, investigating how AC affects: air temperature and humidity (e.g., Niedźwiedź 1987; Gluza and Piasecki 1989; Przybylak 1992; Niedźwiedź 1993; Przybylak 2002; Przybylak and Araźny 2006; Niedźwiedź 2007; Araźny et al., 2010; Bednorz 2010; Przybylak et al., 2012a; Gluza and Siwek 2012; Niedźwiedź 2013b; Przybylak et al., 2014; Isaksen et al., 2016; Araźny et al., 2018; Kendzierski et al., 2018; Przybylak et al., 2018; Araźny 2019; Kejna and Sobota 2019); precipitation (e.g., Niedźwiedź and Ustrnul 1988; Przybylak and Marciniak 1992; Hanssen-Bauer and Førland 1998; Łupikasza and Niedźwiedź 2002; Łupikasza 2010; Dobler et al., 2021); and cloudiness (Niedźwiedź and Ustrnul 1989; Kejna et al., 2012; Lipiński and Łupikasza 2016; Łupikasza and Lipiński 2017; Kolendowicz et al., 2021).
The issue of the impact of AC on meteorological conditions has often also been discussed for the Kaffiøyra region (NW Spitsbergen), where measurements began in 1975, i.e., at the beginning of the current period of global warming (Przybylak 2016). The long series of gathered meteorological data allows changes in climatic conditions in the region to be reliably assessed (Przybylak and Araźny 2006; Przybylak et al., 2009; Przybylak et al. 2011; Przybylak et al. 2012b; Araźny et al., 2016; Przybylak and Araźny 2016). Particular attention has been devoted to the impact of AC on weather conditions in the Kaffiøyra region, and especially on air temperature, air humidity and atmospheric precipitation (Wójcik et al., 1992; Araźny 1998; Przybylak and Araźny 2006; Araźny et al., 2011; Przybylak et al., 2012a; Przybylak and Maszewski 2012; Przybylak et al., 2014; Przybylak et al., 2018; Kejna and Sobota 2019).
The literature lacks any analysis of how AC influences other meteorological parameters not only for the Kaffiøyra area, but for the entire Spitsbergen. This study fills that gap. The main objective of this study is to assess the impact of AC on the occurrence of average and extreme summer weather conditions in NW Spitsbergen around the turn of the 21st century.
Location, Data and Methods
Spitsbergen is the largest island of the Norwegian Svalbard Archipelago (High Arctic). The research area is located in the north-western part (Kaffiøyra, Oscar II Land) of the island (Figure 1). Kaffiøyra is a coastal plain bordered by the Aavatsmark and Dahlbreen glaciers. It is approximately 14 km long, and ranges in width from 1.5 km in the middle to 3.4 km in the north. Six glaciers (the Waldemarbreen, Irenebreen, Elisebreen, Eivindbreen, Andreasbreen and Oliverbreen) reach the plain (Kejna and Sobota 2019). The topography of Kaffiøyra, which is close to the Forland Strait, is very varied. The highest mountains in Oscar II Land are over 1,000 m a.s.l. high, and include Dronningfjella (1,263 m) and TreKroner (1,225 m). Oscar II Land is incised by deep bays and fjords such as St Jonsfjorden, Dahlbukta, Hornbaekbukta, and Engelsbukta, which divide the area along with the glaciers heading towards the sea into coastal plains, such as the Kaffiøyra (Przybylak et al., 2012b). Kaffiøyra is covered with tundra vegetation (Barcikowski et al., 2005). Intensive deglaciation is occurring on Spitsbergen, with the glaciers retreating at a fast pace, e.g., 6–18 m/year in the area of the Kaffiøyra (Sobota and Lankauf 2010; Sobota 2013).
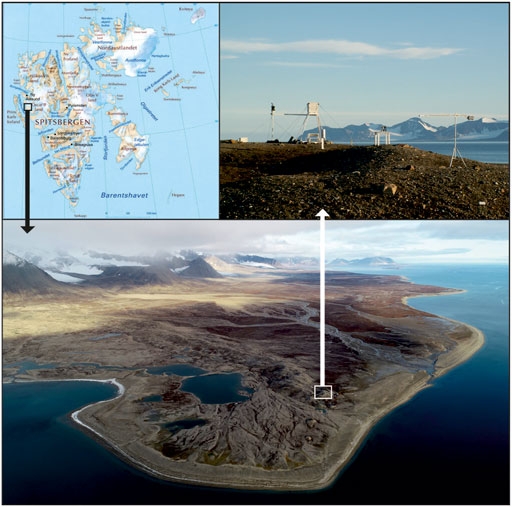
FIGURE 1. Location of Kaffiøyra meteorological station on Spitsbergen (photo by A. Araźny and I. Sobota).
Meteorological measurements and observations on Kaffiøyra began on the beach in 1975 (Leszkiewicz 1977), and in 1978 the station was moved to a nearby terminal-lateral moraine embankment of the Aavatsmark Glacier about 200 m from the Forland Strait (φ = 78°41′N, λ = 11°51′E, h = 11.5 m a.s.l.) (Figure 1). The study was based on meteorological data from Kaffiøyra from summer expeditions in the years 1975, 1977–80, 1982, 1985, 1989, 1997–2000 and 2005–2015. Meteorological data from twenty-three Toruń Polar Expeditions covering 21 July to 31 August (hereinafter referred to as “summer”) of each year were analysed. The analysis used average values or diurnal sums of the main meteorological parameters: total cloudiness (C), sunshine duration (SS), air temperature: diurnal mean (Ti), diurnal maximum (Tmax), diurnal minimum (Tmin), diurnal temperature range (DTR), wind velocity (WV), relative air humidity (RH), water vapour pressure (vp) and precipitation (P). Air temperature, air humidity and wind velocity were measured at a height of 2 m a.g.l.
The influence of AC on meteorological conditions on Kaffiøyra was analysed using the calendar of circulation types by Niedźwiedź (2018). The author of the calendar distinguished 21 types classified by direction of air advection and type of baric regime (Niedźwiedź 2013b). Advective conditions are marked with capital letters indicating direction of air inflow (N, NE, E, SE, S, SW, W and NW). The type of baric regime is marked with the index lowercase “a” for anticyclonic conditions or lowercase “c” for cyclonic conditions. Cyclonic centre (Cc) and anticyclonic centre (Ca), cyclonic trough (Bc) and anticyclonic wedge (Ka) are also distinguished. Hard-to-define baric regimes and barometric cols are marked “X”.
The study uses standard methods from climatology, including synoptic climatology, including presenting anomalies of individual meteorological parameters for CT. Extreme climate phenomena are defined in many ways in the literature, depending on research goals. The IPCC defines an extreme event as a weather phenomenon that occurs at a frequency less than or equal to the 10th percentile or more than or equal to the 90th percentile. A probabilistic criterion is recommended by the European Climate Assessment & Dataset and the IPCC (2001). This study determines extreme conditions using stricter criteria, taking the 5th and 95th percentiles (Supplementary Figure S1). Averages or diurnal totals for meteorological parameters in the period 1975–2015 were used. Relationships between extreme meteorological events and AC were analysed by calculating the average for each meteorological parameter for each of T. Niedźwiedź’s (2018) CT.
Results
Atmospheric Circulation
Over Kaffiøyra, during the summer season (21 July—31 August) in the period 1975–2015, the dominant AC types according to the Niedźwiedź (2018) typology of the synoptic regimes were Ka (15.5%) and Bc (7.5%). The share of no other CT exceeded 7.0%. The least frequent conditions were NWa (1.6%), X (1.8%) and Ca (2.2%). The frequency of the AC macrotypes (cyclonic and anticyclonic) on Kaffiøyra varied greatly from year to year (Figure 2). On average, in the summer seasons, cyclonic types were slightly more common (54.4%) than anticyclonic (43.8%) (Supplementary Figure S2). In the research period, lows most often had their centres to the north-east of Spitsbergen, bringing frequent advection of cool dry air from the northern sector. By contrast, anticyclonic systems were most often centred over the Barents Sea. Our macrotype frequencies support the findings of Niedźwiedź (2013a), and, for July and August, the differences compared to the years 1950–2010 do not exceed 1%–2%.
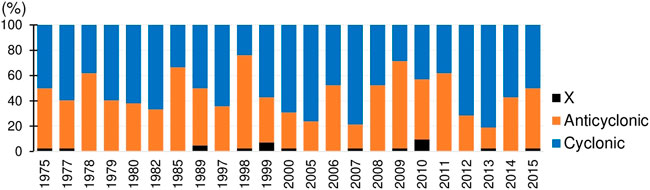
FIGURE 2. Frequency of cyclonic, anticyclonic and indeterminate (X) synoptic conditions over Spitsbergen in the examined summer seasons of 1975–2015.
The Impact of AC on Cloudiness and Sunshine Duration
The Norwegian Arctic is one of the cloudiest areas in the world (Przybylak 2016). The area is also characterised by low insolation (e.g., Budzik 2005; Araźny 2008; Przybylak et al., 2012b; Matuszko et al., 2015). On Kaffiøyra, a significant dependence (Pearson’s linear correlation coefficient 0.90) was found between diurnal total sunshine duration and degree of cloudiness.
The average total cloudiness in the summer seasons on Kaffiøyra was 8.3 on a 0–10 scale (Table 1). For the cyclonic macrotype, cloudiness (C) was 0.3/10 greater than for the anticyclonic macrotype. The lowest C value (7.2) was recorded in the summers of 1985 and 2000. In 1985, anticyclonic situations were very frequent (40.5%), and especially with advection from the E + SE sector, and in 2000 there was an increased frequency of air mass inflows from E. Average C was highest (9.1) in the summers of 1980, 1998 and 2005. In 1980, AC from the S + SW + W sector dominated. In 1998, very high air temperatures occurred, favouring intensive evaporation that increased the development of clouds. By contrast, in the 2005 season, the high C was associated with intense cyclonic activity (76.2%). Average seasonal cloudiness did not show a statistically significant correlation with frequency of AC macrotypes.
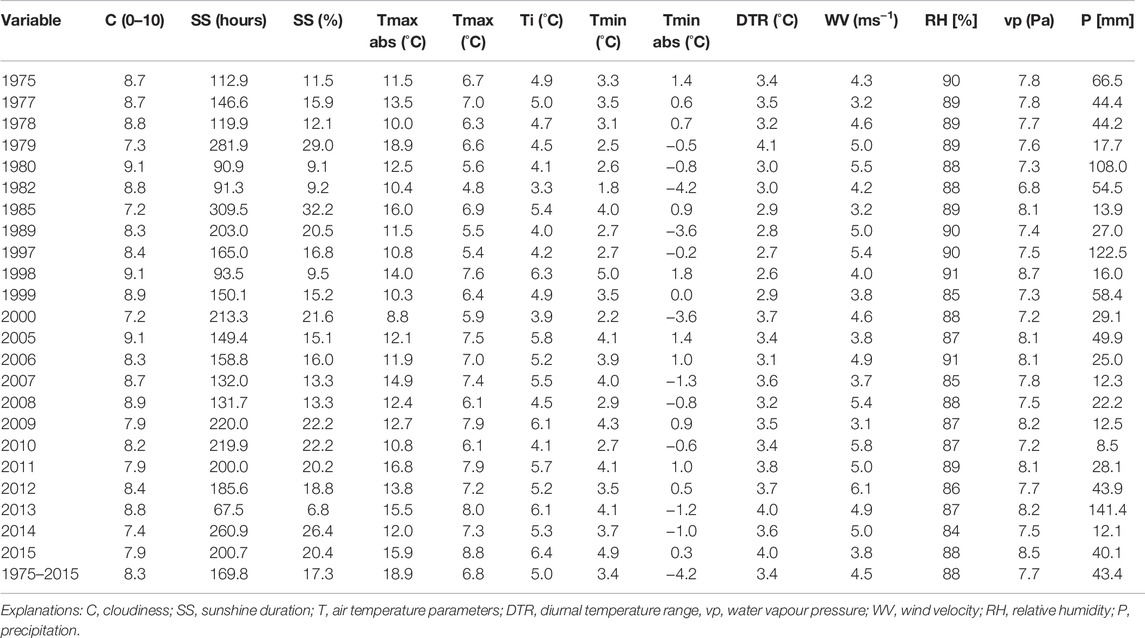
TABLE 1. Average, total and extreme values of meteorological parameters on Kaffiøyra in summer seasons (July 21–August 31) in 1975–2015.
Days of total cloud cover (C = 10) constituted as many as 29% of all days. Over the entire study period, no cloudless day (C = 0) was observed (Figure 3A). Meanwhile, clear days (C < 2) constituted only 2% of all observations on Kaffiøyra and occurred mainly under anticyclonic systems. In the research period, mean diurnal cloudiness (C = 0.3) was lowest on 21 July 1985 during high-pressure circulation from the east. On that day, the heliographs recorded 23.9 h of sunshine duration.
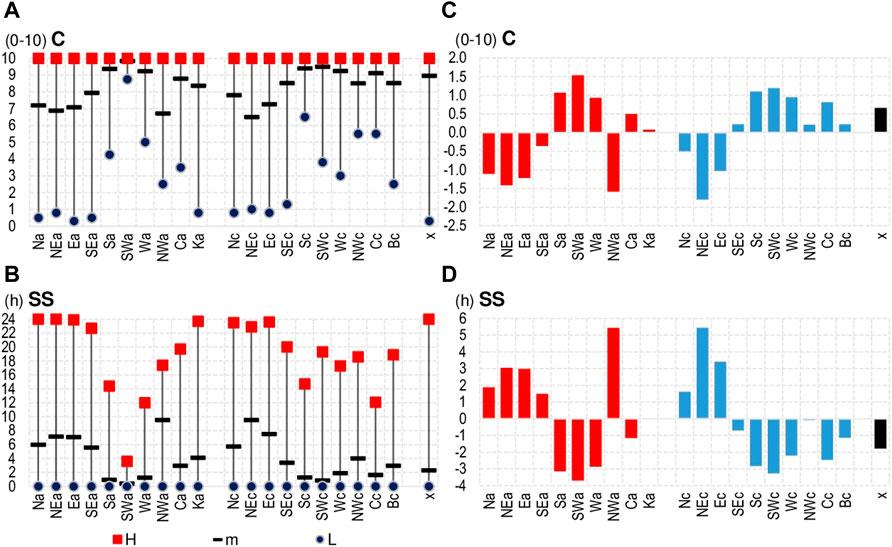
FIGURE 3. Particular synoptic conditions on Kaffiøyra in the summer seasons 1975–2015: (A,B) mean (m) diurnal values of total cloudiness and sunshine duration and their highest (H) and lowest (L) values; and (C,D) their average anomalies.
The highest mean C (>9.0) was associated with the inflow of air masses from the S–W sector under both high- and low-pressure baric regimes. Extreme cloudiness (with a frequency as high as 19.5% placing it above the 95th percentile) is brought by Ka (Figure 5). Conversely, C was lowest C (<7.0) during the inflow of air from the NE, whereas type of baric regime mattered little (Table 2; Figure 3A).
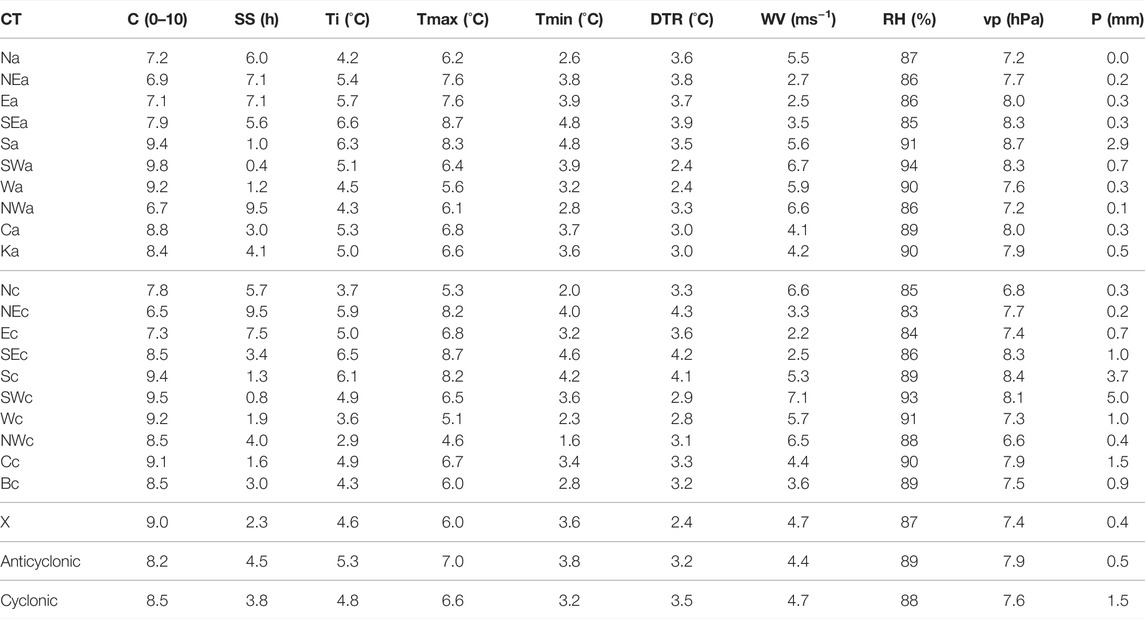
TABLE 2. Mean total cloudiness (C) and sunshine duration (SS); air temperature: diurnal (Ti), maximum (Tmax), minimum (Tmin), range (DTR); wind velocity (WV); relative air humidity (RH); water vapour pressure (vp); and precipitation (P) under various atmospheric circulation types (CT) at the Kaffiøyra station in summer seasons 1975–2015.
In the studied summer seasons, the largest positive C anomalies (>1.0, on a scale of 0–10) were recorded during the inflow of air from the S and SW, during anticyclonic and cyclonic circulation alike. On the other hand, the largest negative anomalies (≥1.4) were recorded during advection from the N sector, i.e., from the centre of the Arctic (NEa, NEc and NWa) (Figure 3C).
In the period 1975–2015, the average total sunshine duration (SS) for the summer season was 169.8 h (Table 1), i.e., 4.1 h/day. The highest SS (309.5 h) occurred in 1985 (Table 1), which was a summer with one of the highest shares of anticyclonic types (66.7%) (Figure 2), during which Ea + SEa circulation predominated (40.5%). Conversely, the poorest summer in terms of insolation was in 2013 (67.5 h). It was characterised by the most intense cyclonic activity (81.0%) (Figure 2). Relative to the mean for the entire period, SS was higher under the anticyclonic macrotype (by 0.4 h) and lower in the cyclonic macrotype (by 0.3 h). A statistically significant correlation was found between seasonal total sunshine durations and frequency of the anticyclonic macrotype (Supplementary Figure S3).
Kaffiøyra’s solar climate is characterised by a large number of sunless days (36%). They occur more often under low-pressure systems than high-pressure ones (53% versus 47%). During the entire measurement period, only 2 days of 24.0 h sunshine duration were recorded; these were 22.07.1979 and 25.07.1997 (Figures 4A,B). On these days, Kaffiøyra was under the influence of anticyclonic circulation, with advection from the northern sector. Extreme SS values (>95th percentile) were most frequent for Ka, NEc and Nc conditions (13%–16%) (Figure 5).
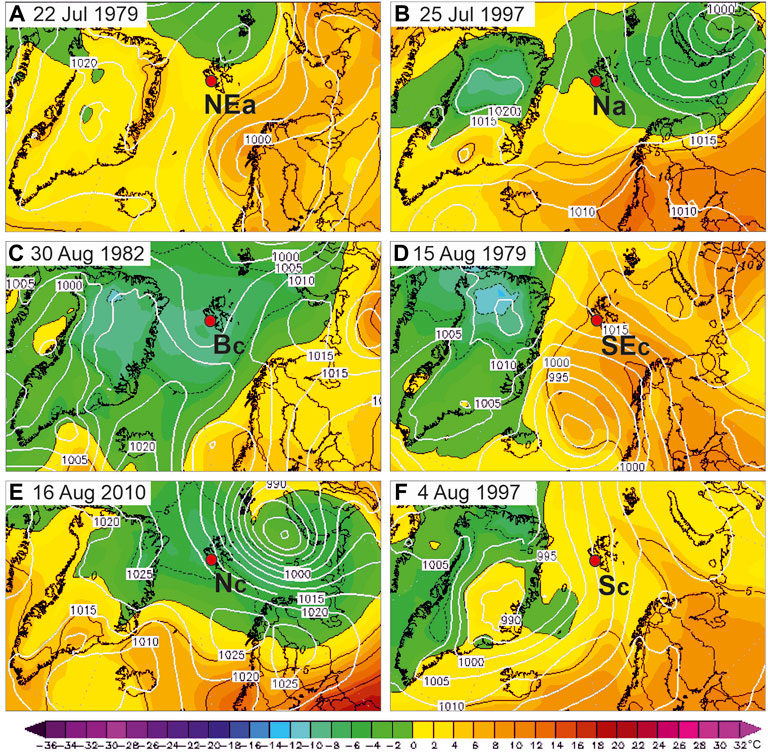
FIGURE 4. Synoptic situations over the research area (red dot) against the background of the atmospheric pressure (hPa) field and air temperature (°C) at the isobaric surface of 850 hPa on selected extreme days: with the highest daily total sunshine duration (A) 22 July 1979, and (B) and 25 July 1997; (C) with the lowest absolute air temperature on 30 August 1982; (D) with the highest absolute air temperature on 15 August 1979; (E) with the highest daily average wind speed on 16 August 2010; and (F) with the highest total daily precipitation on 4 August 1997. Data source: NOAA reanalysis, www.wetterzentrale.
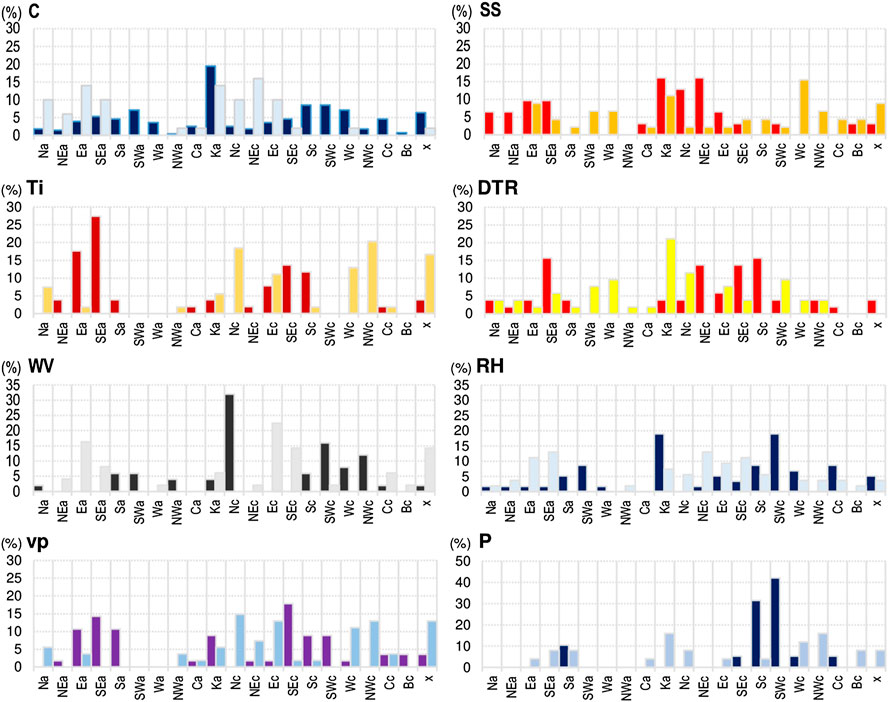
FIGURE 5. Frequency of occurrence of circulation types on days on which meteorological parameter values exceeded thresholds on Kaffiøyra in the summer seasons 1975–2015: days ≥95% (darker bars) and days ≤5% (lighter bars). Explanations: acronyms of meteorological parameters as in Table 2.
Under both baric regimes, the highest average SS (>7.0 h) was recorded with air inflow from the NE and E directions (Table 2; Figure 3B). Conversely, the lowest sunlight durations (<1.0 h) were recorded during the inflow of air from SW, regardless of baric regime. The largest positive SS anomalies were recorded during air inflow from the northern sector, during both high- and low-pressure circulation. The largest positive anomalies were 5.4 h for NWa and NEc types (Figure 3D), and the largest negative anomalies (>2–3 h) were related to air inflow from the S–W sector, which is characterised by high cloudiness.
The Impact of AC on Air Temperature
The most commonly analysed meteorological parameter in the Arctic, including Spitsbergen, is air temperature (Przybylak et al., 2012b, Przybylak, 2016). Its spatial diversity is most influenced by cloudiness, radiation balance, albedo, exposure, altitude above sea level, and advection of air masses (Kejna 2012).
Average Air Temperature (Ti)
Over the 1975–2015 summer seasons, the total average Ti on Kaffiøyra was 5.0°C. It ranged from 3.3°C in 1982 to 6.4°C in 2015 (Table 1). In 1982, the summer season was characterised by a significant share of cyclonic circulation (with a large share of air mass inflows from the north). The low temperature and early onset of winter (i.e., as early as the last decade of August) may have been associated with the eruption of El Chichon volcano in Mexico in spring 1982 (Fujiwara et al., 2020). On the other hand, in the warmest summer season (2015), an increased frequency of anticyclonic circulation was found, with a predominance of wind from southern and eastern sectors.
Over the research period, mean Ti was higher under anticyclonic conditions (at 5.3°C) than cyclonic conditions (4.8°C). This evidences that not only direction of advection but also type of baric regime influenced thermal conditions. In the Kaffiøyra region, significant differences in Ti were found between CTs (Table 2; Figure 6A). Mean Ti (>6°C) was highest during the advection of air from SE and S directions, under both anticyclonic and cyclonic circulation (SEa = 6.6°C, SEc = 6.5°C, Sa = 6.3°C, Sc = 6.1°C). The occurrence of types Ea and SEa was associated with a significant increase in Ti. This is probably due to the appearance of foehn phenomena during advection from these directions (Przybylak et al., 2012b). By contrast, the lowest average Ti was associated with the NWc type (2.9°C). This is confirmed by the frequencies of extreme (≥95th and ≤5th percentiles) Ti values (Figure 5).
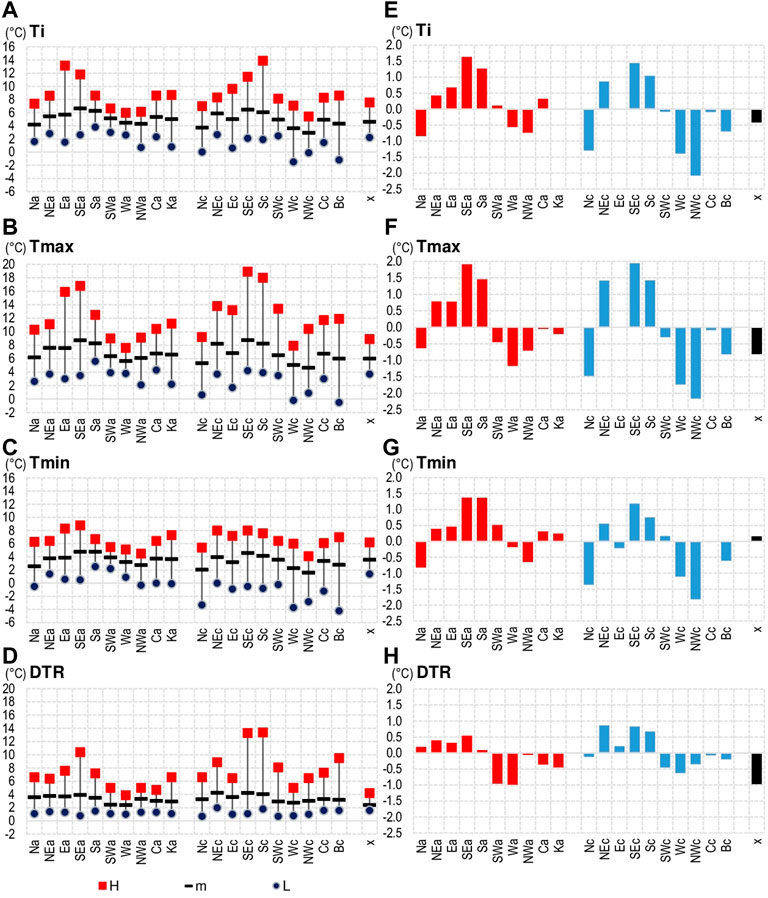
FIGURE 6. Individual circulation types on Kaffiøyra in the summer seasons 1975–2015: (A–D) mean diurnal air temperature (Ti), mean diurnal maximum temperature (Tmax), mean diurnal minimum temperature (Tmin), diurnal temperature range (DTR) and the highest (H) and lowest (L) values; and (E–H) average anomalies of the same parameters.
The highest Ti (13.9°C) occurred on 16 August 1979 during the inflow of warm air from the south (type Sc) and during the occurrence of foehn winds 13.9°C (Wójcik et al., 1981). Meanwhile, it was lowest (1.5°C) during cyclonic circulation from the west on 31 August 1982 (Figure 6A). The standard deviation of mean Ti in the study period was highest during the inflow of air masses from the eastern sector, during both anticyclonic advection (Ea = 2.9°C and SEa = 2.1°C) and cyclonic advection (Ec = 2.1°C).
On Kaffiøyra, during the summer seasons, thermal anomalies of mean Ti are small, at approx. ±2°C (Figure 6E). The largest positive anomalies of Ti (1.0–1.6°C) occur with the inflow of air masses from the SE–E sector, regardless of type of baric regime. By contrast, the largest negative anomalies (1.4–2.1°C) are related to the inflow of air masses from the N–NW sector during cyclonic circulation.
Mean Maximum (Tmax) and Minimum (Tmin) Air Temperature
In this area, the highest Tmax values occurred during the advection of air from the S and SE directions, during high- and low-pressure circulation alike. In such conditions, mean Tmax reached >8°C, and the highest values reached >15°C. The lowest Tmax (4.6°C) and Tmin (1.6°C) values were associated with the NWc type. The highest air temperature in the history of research on Kaffiøyra was 18.9°C, recorded on 15 August 1979 under the SEc type (Figure 4D). The highest Tmin was 8.8°C, recorded on 26 July 1985 under the SEa type and was caused by intense insolation. On the other hand, the lowest Tmax and Tmin (−0.5°C on 30 August 1989 and −4.2°C on 30 August 1982, respectively) were recorded under the Bc type (Figure 4C, 6B,C).
The distribution of Tmax and Tmin anomalies for each CT corresponds to the previously presented anomalies of mean air temperatures (Figures 6E,G). Thermal anomalies were more highly differentiated for Tmax than for Tmin. Tmax ranged on average from −2.2°C (NWc) to 1.9°C (SEc and SEa), while Tmin ranged from −1.8°C (NWc) to 1.4°C (Sa and SEa).
Diurnal Temperature Range
AC also influences DTR. In the summer seasons in 1975–2015, DTR averaged 3.4°C. It ranged from 2.6°C in 1998, when there was significant average cloudiness (9.1), to 4.1°C in 1979, when the average seasonal cloudiness was (7.3) (Table 1). During the cyclonic macrotype, DTR was 0.3°C higher than during the anticyclonic macrotype. In the summer seasons, the lowest mean DTR (2.4°C) occurred for advection from the SW–W sector under anticyclonic conditions. The highest mean DTR (4.2 and 4.1°C) was recorded for SEc and Sc cyclonic advections (Table 2). These two types and SEa had the highest frequencies of high extreme DTRs (approximately 14%–16% each) −16% (Figure 5).
The highest DTR (13.4°C) was recorded on 16 August 1979 for Sc type, when the air temperature increased from 4.6 to 18.0°C as a result of the inflow of warm air with foehn winds (Wójcik et al., 1981). On the other hand, the lowest DTR (0.7°C) occurred twice: on 12 August 1997 (temperature ranged from 2.8 to 3.4°C) and on 2 August 1998 (temperature ranged from 3.6 to 4.3°C). Both these cases occurred during cyclonic situations (SWc and Nc, respectively) (Figure 6D).
DTR thermal anomalies in individual summer seasons are small, at approximately ±1°C (Figure 6H). The largest positive anomalies (0.9°C) were recorded for the NEc type, while the largest negative anomalies (−1.0°C) are associated with the inflow of air masses from the W and SW sectors during anticyclonic and X circulation.
The Impact of AC on Wind Velocity
Wind direction and speed depends on the configuration of the pressure field and the barometric gradient. In the Kaffiøyra region, anemological relations are significantly conditioned by the course and shape of mountain ranges relative to the inflow of prevailing air masses.
The average WV in the summer seasons in the Kaffiøyra region was 4.5 ms−1. In the analysed period, wind velocity was highest (6.1 ms−1) in the summer of 2012, when cyclonic types were prevalent (Figure 2). On the other hand, the lowest velocity (3.1 ms−1) was in 2009, when anticyclonic circulation dominated (69.0%), with Ka and Ca types having the largest share (38.1%). On average, WV was 0.3 ms−1 higher under the cyclonic macrotype than under the anticyclonic macrotype.
Light wind (diurnal average WV ≤ 2.0 ms−1) accounted for 18% of all cases, while strong wind (diurnal average WV ≥ 8.0 ms−1) accounted for 11% of measurements. Both weak and strong winds were both found to occur predominantly under the cyclonic macrotype (55% and 69%, respectively). Characteristic of the anemological conditions in the study area is that the highest mean WVs are associated with air inflow from the SW (7.1 ms−1 for the cyclonic macroptype and 6.7 ms−1 for anticyclonic). Conversely, the lowest mean WV was found for the eastern sector (Ec = 2.2 ms−1, Ea and SEc = 2.5 ms−1) (Table 2).
The highest diurnal mean WV for the study period (14.0 ms−1) was measured on 16 August 2010 during the inflow of air from the north (type Nc) (Figures 4E, 7A). On that day, a gust velocity of 27.7 ms−1 was measured at the nearby summit of Gråfjellet (Przybylak et al., 2012b). The Nc type accounts for as many as 32% of extreme WV values above the 95th percentile (Figure 5). Conversely, the lowest air dynamics (0.1 ms−1) were recorded for anticyclonic conditions from the SE on 5 August 2000. Diurnal mean WVs were least variable during the inflow of air masses with a component from the east (the standard deviation for NEa and SEc = 1.2 ms−1), while they were most variable (3.2 ms−1) during cyclonic advection of air from the N.
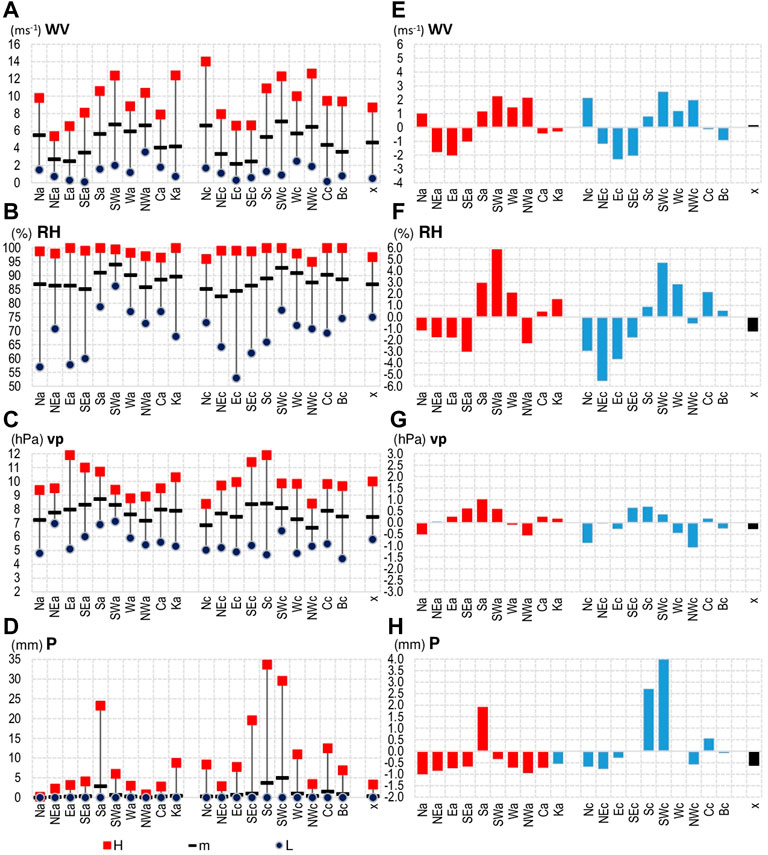
FIGURE 7. Individual circulation types on Kaffiøyra in the summer seasons 1975–2015: (A–D) mean (m) diurnal wind velocity (WV), relative humidity (RH), water vapour pressure (vp), sum of precipitation (P) and their highest (H) and lowest (L) values; and (E–H) their average anomalies.
In the study period, the positive WV anomalies were largest (≥1.2 ms−1) for the Nc type and with air mass inflow from the W sector, and smallest (<1.0 ms−1) for airflow from the eastern sector. In both cases type of baric regime was irrelevant (Figure 7E).
The Impact of AC on Air Humidity
In the summer season, a high degree of water vapour saturation is characteristic of the study area and all of Spitsbergen. This is caused by its insular location, the frequent advection of humid air masses from temperate latitudes under cyclonic systems, and low air temperatures (Przybylak et al., 2012b).
Relative air humidity (RH) The average RH on Kaffiøyra in the summers of 1975–2015 was 88% (Table 1). It ranged from 84% in 2014 (the result, in part, of the high share of advection of air masses from the northern sector) to 91% in 1998 and 2006 (seasons with high air temperature and a high frequency (24%) of anticyclonic wedges).
The mean RH during the cyclonic and anticyclonic macrotypes was similar (with the difference not exceeding 1%) (Table 2). Average diurnal RH values were highest during the inflow of air from the SW (accounting for 94% under the anticyclonic macrotype and 93% for cyclonic), which brought warm humid air masses to Kaffiøyra. The driest air (83%–85%) flowed in from the N–E sector during cyclonic circulation.
Full air saturation (RH = 100%) occurred during half of the AC types (Figure 7B), while RH maxima were lowest during circulation from the eastern sector (Ec = 53% and Ea = 58%) and from the north (Na = 57%).The aforementioned lowest diurnal mean RH of 53% was recorded on 24 August 2012. The standard deviation of the mean diurnal RH was highest during the Ec type (10%) and the lowest during SWa (4%).
In the summer seasons, the largest positive RH anomalies (5%–6%) were associated with types from the SW sector, whereas type of baric regime had no particular significance (Figure 7F). Extreme high RH values were most frequent (19%) for the SWc and Ka types (Figure 5). In the study period, negative RH anomalies were noted with advection of air from the N sector, especially under the NEc type (approx. 6%).
Water vapour pressure (vp) The average vp on Kaffiøyra in the summer seasons of 1975–2015 was 7.7 hPa (Table 1). In the study period, vp fluctuated from 6.8 hPa in 1982 (the coldest season) to 8.7 hPa in 1998 (cloudiest and warmest season). The mean vp was higher under anticyclonic air masses (7.9 hPa) than cyclonic (7.6 hPa). As in the case of RH, no significant relationship was found between vp and the frequency of AC macrotypes (Supplementary Material S3). The highest mean diurnal vp values (>8 hPa) were recorded during the inflow of air from the SE, S and SW directions, regardless of type of baric regime. Also, vp values above the 95th percentile were most frequent for types SEc and SEa (18% and 14%, respectively) (Figure 5). Conversely, the lowest mean vp (<7h Pa) occurred under air masses from N and NW during cyclonic circulation (Table 2).
Mean diurnal vp ranged from 4.4 hPa (30 August 1989) for type Bc to 11.9 hPa for types Sc and Ea (16 August 1979 and 22 July 1998, respectively) (Figure 7C). The standard deviation of mean diurnal vp over the entire measurement period was 1.1 hPa. It was highest (1.4 hPa) during air inflow from the E (both during cyclonic and anticyclonic circulation), and lowest (0.6 hPa) for type SWa.
The largest negative vp anomalies (0.9–1.1 hPa) occurred with air mass inflow from N and NW during cyclonic circulation. Conversely, the largest positive anomalies (0.7 and 1.0 hPa) were recorded during the inflow of air masses from E (regardless of type of baric regime) (Figure 7G).
The Impact of AC on Precipitation
On Kaffiøyra, sums of P depend heavily on AC. In the years 1975–2015, the mean sum of P in the summer season was 43.4 mm (Table 1). Sums ranged from 8.5 mm in 2010 (when anticyclonic circulation predominated) to 141.4 mm in 2013—the summer with the highest frequency (81.0%) of cyclonic baric regimes (Figure 2). Precipitation was highly variable. In individual summer seasons, coefficient of variation ranged from 169% in 2000 to 317% in 1978. A statistically significant correlation was found between seasonal sums of precipitation and frequency of the anticyclonic macrotype (Supplementary Figure S3).
The mean diurnal P of all days in the entire period was 1.0 mm. On days of the cyclonic macrotype, P was 1 mm higher than during the anticyclonic type (Table 2). The highest mean diurnal sums of P occurred during cyclonic situations: SWc (5.0 mm) and Sc (3.7 mm) and under anticyclonic conditions: Sa (2.9 mm). The lowest mean sums of P occurred under anticyclonic conditions, mainly under NE and Ca (Table 2; Figure 7D).
On Kaffiøyra, 61% of days were without precipitation. The occurrence of such days was favoured by anticyclonic situations, and especially Ka. Days with precipitation of ≥0.1 mm accounted for 39%, which includes the 2% of days for which the sums were ≥10.0 mm. These days occurred under the following synoptic situations in particular: SWc (40%): Sc (35%), Sa (10%), and Sc, Wc and Cc (5% each). The largest diurnal sum of P (33.7 mm) was measured on 4 August 1997 during the Sc type (Figures 4F, 7D).
The largest positive P anomalies (from 4.0 to 1.9 mm) were associated with three types from the S–W sector (Sa, Sc and SWc) and with type Cc. As many as 74% of extreme high P occurred during circulation types SWc and Sc (Figure 5). Conversely, negative P anomalies were found during air advection from all other directions (Figure 7H).
Discussion
The analysis of data from the Kaffiøyra station for the summer seasons 1975–2015 showed that AC had a significant impact on all analysed meteorological parameters. Both type of baric regime and direction of advection played an important role.
Cloudiness affects not only SS, but also other climate parameters, including Ti, P and horizontal visibility (Marsz 2013). The influence of AC on cloudiness in Hornsund on Spitsbergen in 1978–87 was presented by Niedźwiedź and Ustrnul (1989). They found that the highest mean diurnal C occurs with air mass advection from southern directions (for types S + SWa and S + SWc). These dependencies have been confirmed by Lipiński and Łupikasza (2016) and Łupikasza and Lipiński (2017) for three Spitsbergen stations (Ny-Ålesund, Svalbard Lufthavn and Hornsund). They also found that C on Spitsbergen is greatest for southerly advection types, regardless of baric regime (S + SWc and S + SWa). By far the lowest C was associated with N + NEa and N + NEc types (Łupikasza and Lipiński 2017). On Kaffiøyra, too, C was highest during air inflow from the S–W sector, under both anticyclonic and cyclonic regimes.
In the Arctic, sunshine duration (SS) is heavily influenced by astronomical factors (polar day and night) and cloudiness caused by air circulation. For Spitsbergen, only one study of how AC influences SS exists (Budzik 2005). For Ny-Ålesund, the highest mean SS values are for types Ka (averaging 178 h a year), NEa (142 h) and Ea (130 h). Conversely, the lowest SS was characteristic of Sa, SWa, Sc, SWc and Wc (less than 20 h a year). On Kaffiøyra, in the summer season, the highest SS values occurred during northerly advection (NWa and NEc averaging 9.5 h a day).
The influence of AC on Ti has mainly been analysed in three regions of Spitsbergen. For the Kaffiøyra area, a number of works exist, including: Wójcik et al., 1992; Araźny 1998; Przybylak and Araźny 2006; Przybylak and Maszewski 2012; Przybylak et al., 2012a, Przybylak et al., 2018; Kejna and Sobota 2019. For Calypsobyen and Petuniabukta in the central part of the island, works include: Gluza and Piasecki 1989; Gluza and Siwek 2012; Kendzierski et al., 2018. And in the Hornsund fjord area, works include: Niedźwiedź 1987, Przybylak 1992; Niedźwiedź 1993, Niedźwiedź 2007, Araźny et al., 2010, Niedźwiedź 2013b; Araźny et al., 2018; Araźny 2019. Circulation conditions relative to air thermics have been studied for all of Spitsbergen by, e.g., Bednorz (2010), Isaksen et al. (2016), and Kejna and Sobota (2019). Most authors found a significant dependence of Ti on AC. The first such research for the summer seasons in the Kaffiøyra area was based on data from 1975 to 89 and carried out by Wójcik et al. (1992). They found Ti to be higher in high-pressure systems (4.8°C) than low-pressure systems (4.3°C). The analysis of data from 1975 to 2015 showed a similar relationship (the difference between macrotypes was 0.5°C), but, as a result of climate warming, the temperature was higher under the anticyclonic macrotype (5.3°C) than the cyclonic type (4.8°C). Wójcik et al. (1992), too, noted that summertime thermal relations depended mainly on direction of advection, and to a lesser extent on type of baric regime. These dependencies have been confirmed by Araźny (1998); Przybylak and Araźny (2006); Przybylak and Maszewski (2012); Przybylak et al. (2012a), Przybylak et al. (2018). Przybylak et al. (2012a) found, based on analysis of 2005–10 data, that the largest positive summer Ti anomalies occurred with the influx of masses from the S–E sector. These dependencies are further confirmed by the results of this study. In advection from this sector, the positive anomalies reached 1.0°C–1.6°C, with type of baric regime being less significant. There were also summer seasons, e.g., 2011, when the highest mean Ti was associated with the Ea + SEa type, e.g., 2011 (Przybylak and Maszewski 2012), which resulted from foehn phenomena during the inflow of air masses over Spitsbergen. Similar dependencies on direction of advection and foehn phenomena have been described for various parts of Spitsbergen, incl. Niedźwiedź (2007), Gluza and Siwek (2012) and Niedźwiedź (2013a). The influence of AC is also marked on glaciers. For the Waldemar Glacier (site at the glacier front and its firn part) in the summer seasons (July 21–August 31) in the period 1975–2014, Przybylak and Araźny (2016) found that anticyclonic and cyclonic types respectively raise and lower the air temperature on the Waldemar Glacier. As the frequency of the anticyclonic and cyclonic types did not change significantly in the summer, it can be assumed that atmospheric circulation was not the reason for the summer temperature increase of recent decades in NW Spitsbergen. Przybylak et al. (2018) investigated the effect of AC on Ti over the annual cycle. Based on 2010–13 data for the Forland Strait area (NW Spitsbergen), they showed that the influence of AC on the distribution of Ti was not uniform across the area. There were differences between coastal and inland stations (on mountains and glaciers). The influence was much smaller in summer than in winter. For Calypsobyen in the central part of Spitsbergen, et al. (2012) analysed data from 2006 to 09 and 2011. They showed that temperatures were highest for the Ea + SEa and Sa + SWa + Wa types, i.e., in anticyclonic conditions. In Hornsund in the south of Spitsbergen, the highest summer temperatures occur for the high-pressure S + SWa type, while the coldest occur for low-pressure N + NEc and W + NWc tyypes (Niedźwiedź 1987; Przybylak 1992). Niedźwiedź (2007) states that, for the period 1978–2006, mean August Ti in Hornsund was 5.2°C and 5.0°C for SEc and Sa, respectively, and 2.8°C for NEa. This was confirmed by Araźny et al. (2010), who for the periods Jun–Sept of 2007 and 2008 found that the largest positive temperature deviations under Sa + SWa + Wa high-pressure circulation reached 1.1°C, and the largest negative ones were of −0.5°C for NWc + Nc + NEc. In another study, covering the period 2014–15, Araźny et al. (2018) showed that mean summer differences in Ti between the Fugleberget peak and the Hornsund station were related to the Sa + SWa + Wa type and amounted to 2.0°C. These are the types that bring the warmest air masses in summer (Niedźwiedź 2013a).
Research by Łupikasza et al. (2021) showed that there were warmer and cooler periods on Spitsbergen in the years 1920–2018. The trend was especially marked in summer and spring. Ti was strongly dependent on southern AC, except in spring, when zonal circulation had the greatest influence.
In Spitsbergen, the highest RH values are associated with cyclonic air advection from the S–SW (e.g., Baranowski and Głowacki 1975; Przybylak 1992). In the 21st century, this has been confirmed by studies from Hornsund, where the highest mean RH occurred for types SWc and SWa, and the lowest for NEa/Na and NEc/Nc during the influx of Arctic air masses (Araźny et al., 2010; Araźny et al., 2018; Araźny 2019). Conversely, in the Kaffiøyra region, according to Araźny et al. (2011) in the summer seasons of 1975–2009, on average, the highest RH value occurred for SWa (94%) and SWc (93%), and the lowest for NEc (83%). These relationships occurred in other years, e.g., in 2010 and 2011 (Araźny et al., 2011; Przybylak and Maszewski 2012). Meanwhile, Przybylak et al. (2014) showed that, over the annual cycle, the air was most humid for types Sc + SWc + Wc and Sa + SWa + Wa (positive anomalies of 7%–9%) and driest for air mass inflows from the E sector, regardless of type of baric regime (negative anomalies of 6%–9%).
On Spitsbergen, the highest P sums occur during air advection from the S and W sectors (Niedźwiedź and Ustrnul 1988; Przybylak and Marciniak 1992; Wójcik et al., 1992; Niedźwiedź 2002; Łupikasza and Niedźwiedź 2002; Łupikasza 2007; Przybylak et al., 2012b). Such a situation results, as reported by Niedźwiedź (2002), from the significant transport of moisture with warmer air masses from above the Atlantic Ocean. Generally, greater sums of precipitation are associated with cyclonic situations more than anticyclonic. Such relationships were found in Hornsund by Łupikasza and Niedźwiedź (2002), and in the Forlandsund by Przybylak and Araźny (2016). In Kaffiøyra, according to Przybylak et al. (2009), the highest diurnal sums of P in the summers of 1980–2008 were recorded during the inflow of air from the S and SW sectors. We obtained similar results in this study. The fact that the lowest p values occurred during the influx of air masses from the N and E was also confirmed, as reported by, inter alia, Przybylak and Marciniak (1992) and Łupikasza and Niedźwiedź (2002).
Summary
The research results confirm the relationship between meteorological conditions and atmospheric circulation. In this study, the impact of AC on average and extreme meteorological conditions in NW Spitsbergen (Svalbard Archipelago) was analysed based on Kaffiøyra data for summer seasons (July 21–August 31) in 1975–2015. The research led to the following conclusions:
• In the studied summer seasons, there was a balanced frequency of anticyclonic and cyclonic macrotypes, and the most frequent synoptic types in the Kaffioyra region were anticyclonic wedge (15.5%) and cyclonic trough (7.5%).
• Circulation factors greatly determined meteorological conditions in the summer seasons in NW Spitsbergen. Direction of advection played the greatest role in determining the type and properties of inflowing air masses, while type of baric regime was less important.
• Extremes and positive anomalies of the analysed weather parameters (including nephological, dynamic, thermal, humidity and precipitation) were strongly favoured by the inflow of air masses from south-western and southern sectors. The highest positive and negative thermal and humidity anomalies were nonetheless associated with the presence of foehn blowing from the island’s interior. Large negative anomalies of all the meteorological parameters (except for SS) were associated with the influx of air masses from the north-east and north.
The results in this study are important to a better understanding of the influence that atmospheric circulation has on meteorological conditions in a time of very dynamic climate change in the Norwegian Arctic. Our results confirm the fact that the role of atmospheric circulation in the shaping of weather and climate conditions in the Arctic is greatest on the Svalbard Archipelago (Przybylak 2002; Przybylak 2016).
Data Availability Statement
The datasets presented in this article are not readily available because The original contributions presented in the study are included in the article/Supplementary Material, further inquiries can be directed to the corresponding author. Requests to access the datasets should be directed to andy@umk.pl.
Author Contributions
AA, RP, and MK formulated the work, AA analysed the data. AA wrote the article. AA, RP, and MK contributed to the analysis and writing. This article is the combined efforts of all the co-authors.
Funding
The work was supported from the funds of the Nicolaus Copernicus University (1665/2020/IDUB.EF – Global environmental changes). N. Copernicus University financed the language correction and open access publication fee.
Conflict of Interest
The authors declare that the research was conducted in the absence of any commercial or financial relationships that could be construed as a potential conflict of interest.
Publisher’s Note
All claims expressed in this article are solely those of the authors and do not necessarily represent those of their affiliated organizations, or those of the publisher, the editors and the reviewers. Any product that may be evaluated in this article, or claim that may be made by its manufacturer, is not guaranteed or endorsed by the publisher.
Acknowledgments
The authors would like to thank the members of the polar expeditions to Kaffiøyra who conducted meteorological measurements and observations in the years 1975–2015. Our gratitude also goes to Tadeusz Niedźwiedź for providing the calendar of circulation types for Spitsbergen.
Supplementary Material
The Supplementary Material for this article can be found online at: https://www.frontiersin.org/articles/10.3389/fenvs.2022.867106/full#supplementary-material
References
Araźny, A. (2008). Bioclimatic Conditions and Their Variability in the Norwegian Arctic for the Period 1971–2000. Toruń: Nicolaus Copernicus University Press.
Araźny, A., Migała, K., Sikora, S., and Budzik, T. (2010). Meteorological and Biometeorological Conditions in the Hornsund Area (Spitsbergen) during the Warm Season. Pol. Polar Res. 31 (3), 217–238. doi:10.2478/v10183-010-0002-4
Araźny, A., Przybylak, R., and Kejna, M. (2016). Ground Temperature Changes on the Kaffiøyra Plain (Spitsbergen) in the Summer Seasons, 1975-2014. Pol. Polar Res. 37 (1), 1–21. doi:10.1515/popore-2016-0011
Araźny, A., Przybylak, R., and Kejna, M. (2011). Zróżnicowanie Wilgotności Względnej I Opadów Atmosferycznych W Rejonie Forlandsundet (NW Spitsbergen) W Sezonie Letnim 2010. Probl. Klim. Polar. 21, 155–172.
Araźny, A., Przybylak, R., Wyszyński, P., Wawrzyniak, T., Nawrot, A., and Budzik, T. (2018). Spatial Variations in Air Temperature and Humidity over Hornsund Fjord (Spitsbergen) from 1 July 2014 to 30 June 2015. Geogr. Ann. Ser. A, Phys. Geogr. 100 (1), 27–43. doi:10.1080/04353676.2017.1368832
Araźny, A. (2019). Temporal and Spatial Variability of Thermal and Humidity Stimuli in the Hornsund Area (Svalbard). Pol. Polar Res. 40 (1), 29–53. doi:10.24425/ppr.2019.126346
Araźny, A. (1998). “The Connection of Air Temperature and Precipitation with the Atmospheric Circulation in the Summer Season 1997 in the Kaffiöyra Plain (Spitsbergen),” in Polish Polar Studies. 25th International Polar Symposium. Editors P. Głowacki, and J. Bednarek (Warszawa: Institute of Geophysics of the Polish Academy of Sciences), 43–50.
Baranowski, S., and Głowicki, B. (1975). “Meteorological and Hydrological Investigations in the Hornsund Region Made in 1970.” in Results of Investigations of the Polish Scientific Spitsbergen Expeditions 1970-1974 (1), Wrocław: Acta Universitatis Wratislaviensis 251, 35–59.
Barcikowski, A., Gugnacka-Fiedor, W., and Zubel, P. (2005). “Charakterystyka Tundry Obszaru Kaffiøyry,” in Kaffiøyra. Zarys Środowiska Geograficznego Kaffiøyry (NW Spitsbergen). Editors M. Grześ, and I. Sobota, (Toruń: Wydawnictwo Mikołaja Kopernika), 35–36.
Bednorz, E. (2010). Occurrence of Winter Air Temperature Extremes in Central Spitsbergen. Theor. Appl. Climatol. 106 (3-4), 547–556. doi:10.1007/s00704-011-0423-y
Bobylev, L. P., Kondratyev, K. Y. A., and Johannessen, O. M. (2003). Arctic Environment Variability in the Context of Global Change. Chichester: PraxisPublishing.
Budzik, T. (2005). Usłonecznienie W Ny-Ålesund (NW Spitsbergen) W Okresie 1993-2004. Probl. Klim. Polar. 15, 103–111.
Ding, Q., Schweiger, A., L’Heureux, M., Battisti, D., Po-Chedley, S., Johnson, N. C., et al. (2017). Influence of High-Latitude Atmospheric Circulation Changes on Summertime Arctic Sea Ice. Nat. Clim. Change 7, 289–295. doi:10.1038/nclimate3241
Dobler, A., Lutz, J., Landgren, O., and Haugen, J. E. (2021). Circulation Specific Precipitation Patterns over Svalbard and Projected Future Changes. Atmosphere 11, 1378. doi:10.3390/atmos11121378
Dzerdzeevskii, B. L. (1975). Cirkulacionnyje Schemy W Troposfierie Centralnoj Arktiki. Moskwa: Izbrannyje Trudy, Izd. Nauka.
Fujiwara, M., Martineau, P., and Wright, J. S. (2020). Surface Temperature Response to the Major Volcanic Eruptions in Multiple Reanalysis Data Sets. Atmos. Chem. Phys. 20, 345–374. doi:10.5194/acp-20-345-2020
Gluza, A., and Piasecki, J. (1989). Rola Cyrkulacji Atmosferycznej W Kształtowaniu Cech Klimatu Południowego Bellsundu Na Przykładzie Sezonu Wiosenno-Letniego 1987r. Lublin: Wypr. Geogr. UMCS na Spitsbergen, 9–28.
Gluza, A., and Siwek, K. (2012). Wpływ Cyrkulacji Atmosferycznej Na Warunki Termiczne Sezonów Letnich (VII-VIII) W Calypsobyen (Zachodni Spitsbergen). Probl. Klim. Polar. 22, 27–34.
Hansen, B. B., Isaksen, K., Benestad, R. E., Kohler, J., Pedersen, Å. Ø., Loe, L. E., et al. (2014). Warmer and Wetter Winters: Characteristics and Implications of an Extreme Weather Event in the High Arctic. Environ. Res. Lett. 9, 114021. doi:10.1088/1748-9326/9/11/114021
Hanssen-Bauer, I., Førland, E. J., Hisdal, H., Mayer, S., Sandø, A. B., and Sorteberg, A. (2019). Climate in Svalbard 2100 -a Knowledge Base for Climate Adaptation. NCCS report no. 1/2019. Norwegian Centre for Climate Services (NCCS) for Norwegian Environment Agency (Miljødirektoratet). doi:10.13140/RG.2.2.10183.75687
Hanssen-Bauer, I., and Førland, E. (1998). Long-term Trends in Precipitation and Temperature in the Norwegian Arctic: Can They Be Explained by Changes in Atmospheric Circulation Patterns? Clim. Res. 10, 143–153. doi:10.3354/cr010143
Indreiten, M., and Svarstad, C. (2016). “The Longyearbyen Fatal Avalanche Accident 19th December 2015, Svalbard - Lessons Learned from Avalanche Rescue inside a Settlement,” in Proceedings of the International Snow Science Workshop (Breckenridge, Colorado. https://arc.lib.montana.edu/snow-science/objects/ISSW16_O16.01.pdf.
IPCC (2001). Climate Change 2001. Th e Scientific Basis. Contribution of the Working Group I to the Third Assessment Report of the Intergovernmental Panel on Climate Change. Cambridge: Cambridge University Press.
Isaksen, K., Nordli, Ø., Førland, E. J., Łupikasza, E., Eastwood, S., and Niedźwiedź, T. (2016). Recent Warming on Spitsbergen - Influence of Atmospheric Circulation and Sea Ice Cover. J. Geophys. Res. Atmos. 121 (11), 913931. doi:10.1002/2016jd025606
Käsmacher, O., and Schneider, C. (2011). An Objective Circulation Pattern Classification for the Region of Svalbard. Geogr. Ann. Ser. A, Phys. Geogr. 93, 259–271. doi:10.1111/j.1468-0459.2011.00431.x
Kejna, M., Przybylak, R., and Araźny, A. (2012). The Influence of Cloudiness and Synoptic Situations on the Solar Radiation Balance in the Area of Kaffiøyra (NW Spitsbergen) in the Summer Seasons 2010 and 2011. Bull. Geogr. Phys. Geogr. Ser. 5, 77–95. doi:10.2478/v10250-012-0005-6
Kejna, M. (2012). “Radiation Conditions,” in Topoclimatic Diversity Min Forlandsundet Region (NW Spitsbergen) in Global Warming Conditions. Editors R. Przybylak, A. Araźny, and M. Kejna Toruń. Turpress: Toruń, 53–76.
Kejna, M., and Sobota, I. (2019). Meteorological Conditions on Kaffioyra (NW Spitsbergen) in 2013-2017 and Their Connection with Atmospheric Circulation and Sea Ice Extent. Pol. Polar Res. 40 (3), 175–204. doi:10.24425/ppr.2019.129670
Kendzierski, S., Kolendowicz, L., and Półrolniczak, M. (2018). The Influence of Synoptic Conditions Patterns on Air Temperature and Humidity in Petuniabukta (Svalbard) in Summer 2016. Pol. Polar Res. 39, 371–392. doi:10.24425/118752
Kolendowicz, L., Półrolniczak, M., Kendzierski, S., Szyga-Pluta, K., and Láska, K. (2021). Influence of Atmospheric Circulation on Cloudiness and Cloud Types in Petuniabukta and Svalbard-Lufthavn in Summer 2016. Atmosphere 12, 724. doi:10.3390/atmos12060724
Leszkiewicz, J. (1977). Meteorological Conditions in the Northern Part of Kaffiøyra Plain during the Period from July 1 to August 21, 1975. Acta Univ. Nic. Copernici, Geogr. 23 (43), 98–111.
Lipiński, O., and Łupikasza, E. (2016). Rola Cyrkulacji Atmosfery W Kształtowaniu Wielkości Zachmurzenia Ogólnego Na Spitsbergenie (1983-2013). Przeg. Geogr. 88 (3), 295–315. doi:10.7163/PrzG.2016.3.2
Łupikasza, E. B., Niedźwiedź, T., Przybylak, R., and Nordli, Ø. (2021). Importance of Regional Indices of Atmospheric Circulation for Periods of Warming and Cooling in Svalbard during 1920-2018. Intl J. Climatol. 41, 3481–3502. doi:10.1002/joc.7031
Łupikasza, E., and Lipiński, O. (2017). Cloud Cover over Spitsbergen and its Relation to Atmospheric Circulation (1983-2015). Geogr. Pol. 90 (1), 21–38. doi:10.7163/GPol.0076
Łupikasza, E. (2010). Long-term Variability of Precipitation Form in Hornsund (Spitsbergen) in Relation to Atmospheric Circulation (1979–2009). Bull. Geogr. Phys. Geogr. Ser. 3, 65–86. doi:10.2478/bgeo-2010-0004
Łupikasza, E., and Niedźwiedź, T. (2002). Wpływ Cyrkulacji Na Opady Atmosferyczne W Hornsundzie, Polish Polar Stud. Poznań: Bogucki Wydawnictwo Naukowe, 203–216.
Łupikasza, E. (2007). Wieloletnia Zmienność Występowania Ekstremów Opadowych W Hornsundzie (Spitsbergen) I Ich Związek Z Cyrkulacją Atmosfery. Probl. Klim. Polar. 17, 87–103.
Marsz, A. A. (2013). Cloudiness and Sunshine Duration, [w:] A. A. Marsz, and A. Styszyńska (red.), Climate and Climate Change in Hornsund, Svalbard, Gdynia Maritime Univ., Gdynia, 101–125.
IPCC (2021). in Climate Change 2021: The Physical Science Basis. Contribution of Working Group I to the Sixth Assessment Report of the Intergovernmental Panel on Climate Change. Editors V. Masson-Delmotte, P. Zhai, A. Pirani, S. L. Connors, C. Péan, S. Bergeret al. (Cambridge: Cambridge University Press).
Matuszko, D., Celiński-Mysław, D., and Soroka, J. (2015). Usłonecznienie W Arktyce Europejskiej I Grenlandii Na Podstawie Danych Z Wybranych Stacji Strefy Polarnej. Probl. Klim. Polar. 25, 127–138.
Niedźwiedź, T. (2018). Calendar of Atmospheric Circulation Types for Spitsbergen – a Digital Dataset. Sosnowiec: Katedra Klimatologii Uniwersytetu Śląskiego.
Niedźwiedź, T. (2013b). “Influence of Atmospheric Circulation on the Air Temperature at Hornsund,” in Climate and Climate Change at Hornsund, Svalbard. Editors A. A. Marsz, and A. Styszyńska, Gdynia: Gdynia Maritime University, 165–172.
Niedźwiedź, T. (1993). Long-term Variability of the Atmospheric Circulation over Spitsbergen and its Influence on the Air Temperature. Lublin: XX Polar Symposium, 17–30.
Niedźwiedź, T. (2013a). “The Atmospheric Circulation,” in Climate and Climate Change at Hornsund, Svalbard. Editors A. A. Marsz, and A. Styszyńska (Gdynia: Gdynia Maritime Univ.), 57–74.
Niedźwiedź, T., and Ustrnul, Z. (1989). Wpływ Sytuacji Atmosferycznej Na Kształtowanie Się Zachmurzenia W Hornsundzie, XVI Symp. Toruń: Polarne, UMK Toruń, 158–160.
Niedźwiedź, T., and Ustrnul, Z. (1988). “Wpływ Sytuacji Synoptycznych Na Stosunki Opadowe W Hornsundzie (Spitsbergen),” in XV Sympozjum Polarne (Wrocław: Wydawnictwo Uniwersytetu Wrocławskiego), 196–202.
Niedźwiedź, T. (1987). Wpływ Cyrkulacji Atmosfery Na Temperaturę Powietrza W Hornsundzie, Spitsbergen, XIV Symp. Lublin: Polarne, 174–180.
Niedźwiedź, T. (2002). Wpływ Cyrkulacji Atmosfery Na Wysokie Opady W Hornsundzie (Spitsbergen). Probl. Klim. Polar. 12, 65–75.
Niedźwiedź, T. (2007). “Wpływ Cyrkulacji Atmosferycznej Na Temperaturę Powietrza W Hornsundzie,” in Klimat Rejonu Polskiej Stacji Polarnej W Hornsundzie – Stan, Zmiany I Ich Przyczyny. A. A. Marsz, and A. Styszyńska. Editors (Gdynia: Akademii Morskiej), 45–63.
Niedźwiedź, T. (1992). Wybrane Problemy Klimatologii Synoptycznej Spitsbergenu. Probl. Klim. Polar. 2, 77–84. Gdynia.
Nordli, Ø., Wyszyński, P., Gjelten, H. M., Isaksen, K., Łupikasza, E., Niedźwiedź, T., et al. (2020). Revisiting the Extended Svalbard Airport Monthly Temperature Series, and the Compiled Corresponding Daily Series 1898-2018. Polar Res. 39, 3614. doi:10.33265/polar.v39.3614
Peeters, B., Pedersen, Å. Ø., Loe, L. E., Isaksen, K., Veiberg, V., Stien, A., et al. (2019). Spatiotemporal Patterns of Rain-On-Snow and Basal Ice in High Arctic Svalbard: Detection of a Climate-Cryosphere Regime Shift. Environ. Res. Lett. 14, 015002. doi:10.1088/1748-9326/aaefb3
Przybylak, R., and Araźny, A. (2006). Climatic Conditions of the North-Western Part of Oscar II Land (Spitsbergen) in the Period between 1975 and 2000. Pol. Polar Res. 27 (2), 133–152.
Przybylak, R., Araźny, A., Kejna, M., Maszewski, R., and Wyszyński, P. (2009). Zróżnicowanie Opadów Atmosferycznych W Rejonie Kaffioyry (NW Spitsbergen) W Sezonie Letnim W Latach 1980-2008. Probl. Klim. Polar 19, 189–202.
Przybylak, R., Araźny, A., and Kejna, M. (2012b). “Topoclimatic Diversity in Forlandsundet Region (NW Spitsbergen) in Global Warming Conditions,” in . (Toruń: Oficyna Wyd. Turpress).
Przybylak, R., Araźny, A., and Kejna, M. (2012a). “Wpływ Cyrkulacji Atmosferycznej Na Temperaturę Powietrza W Regionie Kafiøyry (NW Spitsbergen) W Okresie Od Lipca 2005 R. Do Sierpnia 2010 R,” in Rola Cyrkulacji Atmosfery W Kształtowaniu Klimatu. Editors Z. Bielec-Bąkowska, E. Łupikasza, and A. Widawski, Sosnowiec: Wydawnictwo Uniwersytetu Śląskiego, 195–208.
Przybylak, R., Araźny, A., and Ulandowska-Monarcha, P. (2018). The Influence of Atmospheric Circulation on the Spatial Diversity of Air Temperature in the Area of Forlandsundet (NW Spitsbergen) during 2010-2013. Int. J. Climatol. 38 (1), 230–251. doi:10.1002/joc.5172
Przybylak, R., Araźny, A., and Ulandowska-Monarcha, P. (2014). Zróżnicowanie Przestrzenne Wilgotności Względnej Powietrza W Północnej Części Równiny Kaffiøyra I Na Lodowcu Waldemara (NW Spitsbergen) W Okresie Od Września 2010 Do Sierpnia 2013. Probl. Klim. Polar. 24, 25–36.
Przybylak, R., and Araźny, A. (2016). Warming and Drying of Tundra and Glacier Summer Climate in NW Spitsbergen from 1975 to 2014. Pol. Polar Res. 37 (2), 173–192. doi:10.1515/popore-2016-0011
Przybylak, R., Kejna, M., and Araźny, A. (2011). Air Temperature and Precipitation Changes in the Kaffiøyra Region (NW Spitsbergen) from 1975 to 2010. Pap. Glob. Change IGBP 18 (1), 7–22. doi:10.2478/v10190-010-0001-10
Przybylak, R., and Marciniak, K. (1992). Opady a Cyrkulacja Atmosferyczna Na Zachodnim Wybrzeżu Spitsbergenu W Okresie 1979-1985. Problem. Klim. Polar. 2, 84–95.
Przybylak, R., and Maszewski, R. (2012). “The Influence of Atmospheric Circulation on Temperature and Humidity Conditions,” in Topoclimatic Diversity in Forlandsundet Region (NW Spitsbergen) in Global Warming Conditions. Editors R. Przybylak, A. Araźny, and M. Kejna (Toruń: Oficyna Wyd. Turpress), 139–145.
Przybylak, R. (1992). Stosunki Termiczno-Wilgotnościowe Na Tle Warunków Cyrkulacyjnych W Hornsundzie (Spitsbergen) W Okresie 1978-1983. Warszawa: Dok. Geogr., 2.
Przybylak, R. (2016). The Climate of the Arctic. Second edition, 52. Heidelberg/New York/Dordrecht/London: Atmospheric and Oceanographic Sciences LibrarySpringer.
Przybylak, R. (2002). Variability of Air Temperature and Atmospheric Precipitation in the Arctic. Dordrecht/Boston/London: Atmospheric and Oceanographic Sciences Library, Kluwer Academic Pub., 25.
Serreze, M. C., and Barry, R. G. (2011). Processes and Impacts of Arctic Amplification: A Research Synthesis. Glob. Planet 77 (1–2), 85–96. doi:10.1016/j.gloplacha.2011.03.004
Serreze, M. C., and Barry, R. G. (2014). The Arctic Climate System. 2nd ed. Cambridge: Cambridge University Press.
Sobota, I., and Lankauf, K. R. (2010). Recession of Kaffiøyra Region Glaciers, Oscar II Land, Svalbard. Bull. Geogr. Phys. Geogr. Ser. 3, 27–45. doi:10.2478/bgeo-2010-0002
Sobota, I. (2013). Współczesne Zmiany Kriosfery Północno-Zachodniego Spitsbergenu Na Przykładzie Rejonu Kaffiøyry. Toruń: Naukowe UMK.Wyd.
Turner, J., and Marshall, G. J. (2011). Climate Change in the Polar Regions. Cambridge: Cambridge University Press.
Wójcik, G., Marciniak, K., Przybylak, R., and Kejna, M. (1992). Temperatura I Opady a Cyrkulacja Atmosferyczna W Regionie Kaffiöyry (NW Spitsbergen) W Sezonie Letnim W Okresie 1975–1989. Probl. Klim. Polar. 2, 96–102.
Keywords: atmospheric circulation, climate changes, extreme weather, meteorological conditions, Kaffiøyra, Spitsbergen
Citation: Araźny A, Przybylak R and Kejna M (2022) The Influence of Atmospheric Circulation on Mean and Extreme Weather Conditions on Kaffiøyra (NW Spitsbergen, Svalbard Archipelago) in the Summer Seasons 1975–2015. Front. Environ. Sci. 10:867106. doi: 10.3389/fenvs.2022.867106
Received: 31 January 2022; Accepted: 21 June 2022;
Published: 11 July 2022.
Edited by:
Hong Liao, Nanjing University of Information Science and Technology, ChinaReviewed by:
Nicola Scafetta, University of Naples Federico II, ItalyKristaps Lamsters, University of Latvia, Latvia
Copyright © 2022 Araźny, Przybylak and Kejna. This is an open-access article distributed under the terms of the Creative Commons Attribution License (CC BY). The use, distribution or reproduction in other forums is permitted, provided the original author(s) and the copyright owner(s) are credited and that the original publication in this journal is cited, in accordance with accepted academic practice. No use, distribution or reproduction is permitted which does not comply with these terms.
*Correspondence: Andrzej Araźny, andy@umk.pl