- 1Department of Hydraulic and Sanitary Engineering, Institute of Environmental Engineering, Warsaw University of Life Sciences-SGGW, Warsaw, Poland
- 2Department of Hydraulic and Sanitary Engineering, Poznań University of Life Sciences, Poznań, Poland
- 3Department of Hydrology, Meteorology and Water Management, Institute of Environmental Engineering, Warsaw University of Life Sciences-SGGW, Warsaw, Poland
One of the most commonly used methods of environmental flows assessment is the approach based on Habitat Suitability Modeling (HSM) and habitat preferences curves (HPCs). Relationships between organisms and physical parameters describing the habitat remain strongly nonlinear and vary depending on several factors. The most common comparisons concern analyses between natural and regulated rivers, where the degree of river channel alterations is undefined and usually refers to all forms of hydromorphological degradation. Additionally, spatial scale and computational range of such a research mostly focus on longer river reaches and statistical analysis of HPC transferability, without detailed estimation of environmental flows values. The main aim of our research was assessment and comparison of environmental flows’ values for macroinvertebrates at two reaches of one river with different degrees of hydromorphological alteration. Two research hypotheses were verified: 1) morphological conditions impact on values of environmental flows and weighted usable area; 2) the mean value of environmental flows at the regulated river reach is higher compared to the natural reach. In order to put the obtained values of environmental flows into a broader context, the analyses of hydrological data and calculation of hydrology-based environmental flows were performed. Additionally, before determination of environmental flows, the transferability of habitat suitability criteria between reaches was verified. The obtained results confirmed research hypotheses, revealing strong dependence of environmental flows values to morphological conditions. Additionally, for both studied sections the lower limits of environmental flows were greater than hydrology-based values.
1 Introduction
Habitat suitability models (HSMs) have been recognized as one of the most reliable tools for determining environmental flows and assessing the impact of hydrotechnical and river restoration projects on aquatic habitats (European Commission, 2015; Theodoropoulos et al., 2018a). Despite widespread agreement, it is still problematic to apply HSMs at larger scale due to their high time- and cost-intensity (Pahl-Wostl et al., 2013; Chen and Olden, 2017; Rosenfeld, 2017), making it unfeasible, from a practical and economical point of view, to develop the HSMs for every single river (Millidine et al., 2016; Chen and Olden, 2017). One of the optimization methods to decrease the effort needed to develop habitat suitability models, is the determination of universal habitat suitability criteria describing (in a linear or nonlinear manner) flow-ecology relationships. Data are collected on natural river reaches and subsequently are typically aggregated in three ways: 1) combining samples from different watercourses regardless of the sampling season and no data preprocessing, 2) combining samples from different rivers but only for some specific period, usually low flows, and 3) combining samples from different watercourses regardless of the sampling season, after appropriate data processing (Theodoropoulos et al., 2018b). Regardless of the method, it is necessary to assess the applicability of general criteria to different rivers (Bovee, 1997). This assessment is usually referred to as verification the possibility of transferability of habitat suitability criteria (or flow-ecology relationships) (Bovee, 1997). Based on statistical tests or assumed fit of measures between organisms’ distribution and preferences, a decision is made on the applicability of the criteria to another rivers or sections of the same river (e.g., Millidine et al., 2016; Chen and Olden, 2017). So far, few studies have been conducted that quantify the feasibility to transfer these criteria (Poff and Zimmerman, 2010; Pahl-Wostl et al., 2013; Chen and Olden, 2017). Relationships between organisms and physical parameters describing habitats, may vary with river size, type of hydromorphological degradation, typology, geographic location, life history strategy, season, or stage of the life cycle. Studies have shown poor transferability of habitat suitability criteria between small and large rivers (Jowett, 2003; Mérigoux et al., 2009). At the same time, using the same criteria for rivers with similar typology can yield good measures of fit (Millidine et al., 2016; Chen and Olden, 2017). The possibility of using generalized habitat suitability criteria for macroinvertebrates was analyzed by Theodoropoulos et al. (2018b). The authors considered the applicability of criteria determined on data collected from rivers with different size and during different seasons. Results showed that macroinvertebrates change their habitat preferences with season and geographic location, with season-induced changes being more significant. Furthermore, different values of the fit measures were obtained depending on the data normalization method used, which was consistent with other studies on the transferability of criteria (different computational methods may produce different results) (Huang and Frimpong, 2016; Millidine et al., 2016). Similar results were obtained by Lamouroux et al. (2013), who compared the fit of regional models to the relationship between individual taxa density and hydraulic parameters. The majority of statistical tests demonstrated the applicability of regional models on single watercourses.
Nevertheless, research mostly focus on longer river reaches and statistical analysis of transferability between sections of different regulation status, without detailed estimation of environmental flows values and differences between those reaches. Thus, the analyses typically do not account for local hydromorphological conditions that cause variability in the river channel. Also, such approaches usually refer to fish species, neglecting the broad range of macroinvertebrates that remain an important element of riverine ecosystems. For instance, Chen and Olden (2017) in the study conducted for fish guilds, showed that there was a better possibility of transferability of flow-ecology relationships between rivers with different status of regulation (regulated and free-flowing rivers) compared to rivers of the same status. The study also showed no significant differences in the transferability between the reaches of the same river, between rivers of the same regulation status, and between watercourses with different degrees of regulation.
River regulation remains inevitably related to hydromorphological degradation. According to the Water Framework Directive (WFD), hydromorphological elements include: 1) hydrological status in terms of the amount and dynamics of water flows and the connection to groundwater bodies; 2) river continuity; and 3) morphological conditions, which include river depth and width variability, substrate structure and composition, and riparian zone structure. Hydromorphological degradation is one of the most severe human pressures affecting fish and macroinvertebrates communities in a large proportion of European rivers (Feld and Hering, 2007; EEA, 2012). While the impact of various types of hydromorphological alterations on macroinvertebrates and other aquatic organisms has been identified (e.g., Lorenz et al., 2004; Friberg et al., 2009; Urbanic, 2012), analyses of the requirements of regulated river sections in relation to environmental flows need further research. In that matter, environmental flows with regards to disturbances associated with water damming are most often considered (e.g., Olden and Naiman, 2010; Poff and Zimmerman, 2010; Guareschi et al., 2014; Holt et al., 2014; Leitner et al., 2017). This is due to the fact that flow magnitude is the most noticeable element of modified hydrological regime (Gillespie et al., 2015). Additionally, controlled structures provide the opportunity to match water discharges to environmental flows values (Harman and Stewardson, 2005; Richter and Thomas, 2007; Olden and Naiman, 2010; Renöfält et al., 2010).
However, the research on the influence of morphological conditions and channel modifications on transferability of habitat suitability criteria and values of environmental flows is still insufficient. Such river reaches are characterized by high variability with regards to the degree of hydromorphological alterations and the resulting response of organisms (Feld and Hering, 2007). For that reason taking into account treatments carried out, e.g., as part of so-called water maintenance works (e.g., bed deepening, bank modifications, macrophytes removal, removal of dead organic matter and de-silting) seems to be equally important (Friberg et al., 2009; Leszczyńska et al., 2017). These measures are commonly used on lowland rivers, where catchments have high share of agricultural areas (Bączyk et al., 2018). The purpose of maintenance works is to protect against flooding or to remove its consequences, to maintain the status quo that allows for the water use, to maintain the operating conditions of inland waterways, or to preserve the operation of water facilities (Biedroń et al., 2018). The negative effect of maintenance works may be the loss of biodiversity, and the functioning of river ecosystems depends on the frequency of their performance (Szlauer-Łukaszewska and Zawal, 2014; Grygoruk et al., 2015; Bączyk et al., 2018). A literature review conducted by Bączyk et al. (2018) showed that in 96% of cases a negative impact of maintenance works on the ecological status of rivers was observed. Additionally, the analysis showed that these activities caused a decrease in macrophytes biodiversity by 37% and a decrease in fish and macroinvertebrates abundance by 49 and 42%, respectively. Furthermore, maintenance works can lead to homogenization of the riverbed, drastically changing the diversity of habitats and thus developing a new structure of aquatic organisms, favoring eurytopic species (Szlauer-Łukaszewska and Zawal, 2014; Bączyk et al., 2018). Such altered ecosystems may respond differently to changes in hydrological regime and environmental flows, determined, for example, based on general habitat suitability criteria.
The main aim of our research was the estimation and comparison of environmental flows’ values for macroinvertebrates at two reaches of one river with different degrees of hydromorphological alterations. Before the assessment of environmental flows’ values, the possibility of transferability of habitat suitability criteria was investigated. Analyses were carried out at a very detailed spatial scale, covering two 300 m long river reaches. The first analyzed river section was of the natural hydromorphology conditions whilst the second one was a channelized section under the pressure of regular maintenance works (dredging and macrophyte removal). Two research hypotheses were verified: 1) morphological conditions influence values of environmental flows and weighted usable area index; 2) the mean value of environmental flows at the regulated river reach is higher compared to the natural reach. In order to put the obtained values of environmental flows into a broader context, an analysis of hydrological data, including calculation of hydrology-based environmental flows, was also performed.
2 Materials and Methods
2.1 Study Area
Our study was based on data collected from two sections of the Flinta River. The Flinta River is a small sandy lowland stream located in Western Poland (Figure 1). The total length of the river is 37.8 km and the catchment area is 338.5 km2. The average slope of the riverbed is about 0.75‰, with lower gradients in the upper and middle parts, where the river valley is wide and flat (Gąbka et al., 2014). In the downstream part the valley is narrower, and the channel slope increases. The river is characterized by a snow-rainfall streamflow regime and inflow of cold waters from the Niewiemko Lake, located in the nature reserve “Headwaters of the Flinta River.” One water gauge station is located on the river in Ryczywół.
The first study section (section A) was located about 1.4 km upstream of the river’s confluence to the Wełna River, in the Nature 2000 areas “Noteć Forest” and “Valley of the Wełna River.” The average width of the channel was about 4.5 m, and the slope was equal 0.99‰. The section A was characterized by a good hydromorphological conditions, confirmed by an assessment of hydromorphological elements using the River Habitat Survey method (Szoszkiewicz et al., 2017). The hydromorphological elements were classified the to the I, the highest, class of hydromorphological status (Jakubas et al., 2014). The dominant bottom substrate was sand; however, gravel within the riffles and fine sand and silt within the shelves and pools were also present. The second study section (section B), with a significant degree of morphological degradation, was the regulated river reach located in Ryczywół. In addition to past regulations and channelization of the riverbed, section B was under the pressure of regular maintenance works. These included mowing of the riverbanks, uprooting plants from the river bottom and desludging the bottom with a layer of up to 10 cm. Publicly available data for public contracts indicated that maintenance works were performed during the months of September-October in 2016, 2017, and 2018. Based on the assessment of the hydromorphological elements, the river reach within Ryczywół was classified to the V (the worst) class of river hydromorphological state (Jakubas et al., 2014). Compared to the section A, the section B was characterized by a larger channel width–approximately 8 m on average–and a lower slope of approximately 0.15‰. Technical river training and maintenance works have resulted in a homogeneous riverbed, without distinct morphological forms or mesohabitats. Importantly, the morphological conditions and the lack of shading favored intensive overgrowth of the channel by aquatic macrophytes, which—in the feedback–triggered an intensive process of fine-grained sediment trapping and its deposition, causing channel siltation.
2.2 Development of HSMs
2.2.1 Hydrodynamic Modeling
The analysis were performed using habitat suitability modeling methods. The HSMs were developed for both studied reaches and they consisted of three elements: 2D hydrodynamic models, habitat suitability criteria and the tool for combining modeling results and habitat suitability criteria (Figure 2). The hydrodynamic models were developed in the HEC-RAS 5.0.7. software. The data required for the development of 2D hydrodynamic models consisted of measurements of the riverbed elevations, developed map of mesohabitats (for the section A), photos of the riverbed at the section B, as well as public-domain digital elevation model (DEM) of the floodplains and land cover data from a database of topographic objects (BDOT10k). The riverbed elevations were measured in 2018 using a GPS Sokkia GRX-1 RTK, which allowed for an assumed situational accuracy of 0.03 m and elevation accuracy of 0.05 m. In the section A, survey points were located at cross sections or at locations of changes in channel shape to account for the diversity of morphological forms (Pasternack et al., 2004; Theodoropoulos et al., 2018c). In the section B, where the channel was uniform, points were located in cross sections. Measurements included the target study sections (where point density was high) and approximately 50–100 m sections above and below the study sections (where measurements were taken in several cross sections). This extension was intended to move the upstream and downstream boundary conditions away from the target study areas and thus minimize their influence on the results of hydraulic calculations. At the section A ordinates were measured at a total of 1917 points, and the density of points on the target survey section was 1.17 p/m2. At the river reach B it was 1,615 points and the density of points was 0.79 p/m2. The measured elevation points were used to interpolate the digital elevation models of the riverbed, which in the next step were merged with DEM of the floodplains to the final bathymetries of the models. The DEM consisted of elevation values in a regular 1 m grid.
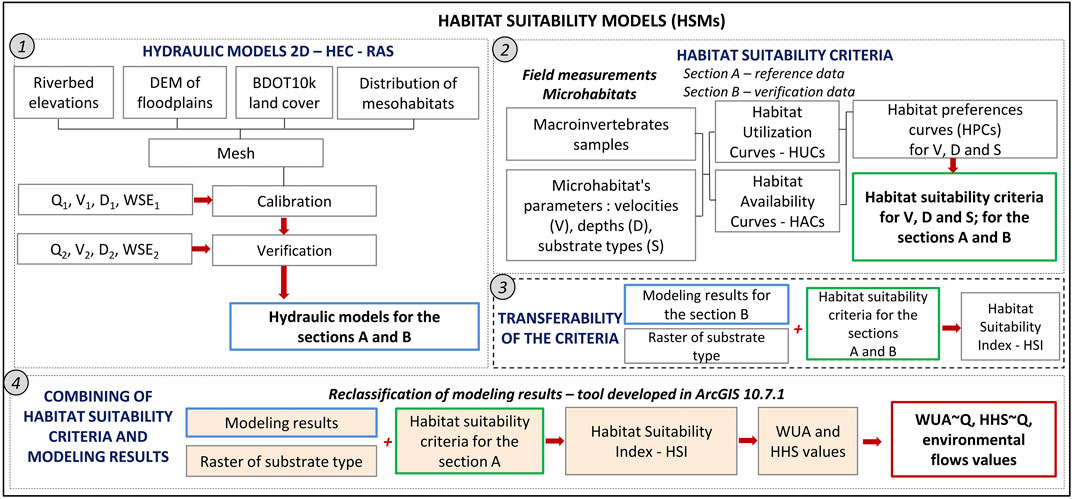
FIGURE 2. Diagram showing the construction of the HSMs developed in the study. V—flow velocities, D—depths, and WSE—water surface elevations, measured at two different flow rates Q1 and Q2.
In the next step initial roughness coefficients were assigned. As Wright et al. stated (2017), during preparing hydraulic models for environmental analysis, it is important to reflect the distribution of vegetation and bottom substrate within the channel using roughness coefficients, which will then condition the occurrence of specific water flow velocities and depths. For this reason, the distribution of mesohabitats was the basis for determining roughness coefficients within the channel in the section A. During field surveys it was observed, analogous to Jowett (2003), that each hydromorphological unit corresponded to a specific water flow velocity dynamics, vegetation structure and debris grain size (e.g., within riffles the highest water flow velocities, gravel and few submerged macrophytes were observed, while within the pools there was fine sand with a high proportion of silt and clay fractions). Due to the uniform character of the riverbed at the section B, roughness coefficients were determined from photographic documentation showing the distribution of macrophytes within the channel. The land cover data were used to assign the coefficient values at the floodplains. The roughness values were defined using Ven Te Chow tables (Chow, 1959) and guidelines developed by Phillips and Tadayon (2007). After that step, the computational mesh was generated. When determining environmental flows, it is important that the resolution of the mesh is sufficient to estimate parameters for the target group of aquatic organisms, in this case to reflect flow parameters at the microhabitat scale (Wright et al., 2017). For this reason, for both studied reaches, a regular (orthogonal) meshes with cell size of 0.5 m were generated.
The prepared hydrodynamic models were calibrated and verified based on measured water surface elevations, depths, and vertically averaged water flow velocities (Tonina and Jorde, 2013; Wright et al., 2017; Theodoropoulos et al., 2018c). Hydrometric measurements were conducted at two different flows (Waddle and Holmquist, 2011; Theodoropoulos et al., 2018c). Data for model calibration were acquired in April 2019. During the measurements, the flow in the section A was 0.537 m3/s, while in the section B it was 0.425 m3/s. A second series of measurements was conducted in June 2019, at the flows of 0.158 m3/s (section A) and 0.048 m3/s (section B). Measurements from 15 randomly selected points within the channel were used for calibration, while verification was based on hydrometric data acquired from 10 points. Vertically averaged water flow velocities were measured using Valeport model 801 flowmeter, at depths of 0.4D when D ≤ 0.75 m or at depths of 0.2D and 0.8D when D > 0.75 m with an accuracy of ±0.5% of the read value (Emery et al., 2003; Jowett, 2003; Pasternack et al., 2006; Theodoropoulos et al., 2018b). A scaled rod of the flowmeter was also used to read the depths at the measurement points. The models were calibrated by modifying the initial roughness coefficients within individual mesohabitats and land cover types, until the coefficients of determination R2 between calculated and observed values of water depths and flow velocities were greater than or equal to 0.8, and until the RMSE error for differences in water surface elevations did not exceed 0.1 m.
2.2.2 Habitat Suitability Criteria
Benthic macroinvertebrates used for determining habitat suitability criteria were collected during two field campaigns carried out during spring seasons (months of May and June) in 2018 and 2019. Total number of macroinvertebrates samples collected from the section A was equal 20 in 2018 and 30 in 2019. The river discharge during field measurements in 2018 was equal to 0.36 m3/s (medium range of flows for the Flinta River) and in 2019–0.11 m3/s (range of low water flows). Data collected at the section A were the basis for determining reference habitat suitability criteria (Dunbar et al., 2011). Macroinvertebrates samples were also collected in 2019 at the section B, however the main purpose of that sampling was to develop separate criteria for the section B. Those were used to verify the transferability of reference criteria between both studied sections. Thus total number of samples from the section B was smaller and was equaled 35. Macroinvertebrates samples were collected from microhabitats using a hydrobiological mesh and then preserved in 95% ethanol. Physical parameters, water depths and velocities at macroinvertebrates sampling points were measured similarly to data acquisition for calibration and verification of hydrodynamic models. Additionally, the bottom substrate was qualitatively determined by visual assessment of the size of mineral fractions. The following categories of bottom substrate were assigned: a–fine sand, c–medium sand, ae–fine sand with gravel, ce–medium sand with gravel, e–gravel, f–silt.
Macroinvertebrates were identified to the lowest possible taxonomic level and counted. In most cases it was genus or species level. Only in two taxa of the order Diptera individuals were assigned to the family level (Simuliideae and Chironomidae). Since data were collected during two seasons, before the development of habitat preference curves, the differences in the structure of macroinvertebrate communities have been investigated using ANOSIM test (Clarke and Warwick, 2001; Graeber et al., 2013). The results of ANOSIM analysis showed that the differences in organism structure between samples collected in 2018 and 2019 were statistically significant (p = 0.002). However, the R-value was low and equaled 0.14 (very small differences between samples). Therefore, it was assumed that the data collected during the two field campaigns can be combined. Subsequently, habitat suitability criteria for water flow velocities, depths, and bottom substrate categories were determined using the method of habitat preferences curves (HPCs) (Bovee, 1986; Jowett et al., 2008). Following Theodoropoulos et al. (2018a), the HPCs were determined for whole group of identified macroinvertebrates. The HPCs are the ratio between habitat utilization curves (HUCs), which are based on field observations and reflect the use of habitats by organisms at the time the measurements were taken; and habitat availability curves (HACs), which determine the amount of available habitats within the analyzed river reach at the time of measurements. The suitability of physical parameters on the curves was expressed by a Suitability Index (SI) that ranges from 0 (low suitability) to 1 (high suitability). Due to fact that for the section A data from different measurement campaigns were pooled, prior to calculations the abundance of organisms in each microhabitat was standardized by dividing by the highest value recorded in a given measurement campaign. Additionally, habitat preferences curves and habitat utilization curves were first analyzed separately for data collected in 2018 and 2019 (Bovee et al., 1998). One of the assumptions associated with the habitat selection function is that organisms have free and equal access to all available resources (Manly et al., 1993) This assumption may be violated if data acquired during low flows are combined with data acquired during higher flow values (the same conditions are not available during both periods). Thus, the final habitat suitability criteria were determined based on preliminary analysis of HPCs and HUCs and curves received for pooled data. For the section A, the highest values of suitability were assigned for water flow velocities in the range 0.05–0.60 m/s, depths in the range 0.07–0.60 m, and the following bottom substrate types: f, a, ce and e. For the section B, the highest values of suitability were obtained for water flow velocities in the range 0.05–0.30 m/s, depths in the range 0.05–0.35 m, and a category of bottom substrate.
2.2.3 Combining Modeling Results With Habitat Suitability Criteria
The final step in preparing HSMs was to develop a tool to link the modeling results to habitat suitability criteria and to calculate the weighted usable area (WUA) and the hydraulic habitat suitability index (HHS) (Figure 2). The tool was created in the Model Builder module of ArcGIS 10.7.1. In the first step, the tool reclassified 2D modeling results (i.e., depths and water flow velocities rasters for each computational scenario) according to the habitat suitability criteria. Since hydrodynamic analyses did not considered sediment transport processes, SI rasters for bottom substrate were created using the location of each mesohabitat type and its association with bottom substrate categories (for the section A), or photographs taken during the field measurements (for the section B). Subsequently, for each flow scenario, the rasters of an overall Habitat Suitability Index (HSI) were calculated based on the SI rasters for water flow velocities (SIV), depths (SID) and bottom substrate categories (SIS), according to the following equation (Ahmadi-Nedushan et al., 2006):
In the last step for each flow scenario the WUA and HHS indices were calculated (Jowett et al., 2008; Stamou et al., 2018):
where Ai was the area of the ith raster cell, and HSIi was the habitat suitability index of the ith raster cell. HHS index was calculated by dividing the WUA value by the total wetted area. This operation eliminated the impact of wetted area on the WUA values and thus facilitated the comparison of results between sections (HHS values ranging from 0 to 1). Following Stamou et al. (2018) and Papadaki et al. (2016), WUA index was only calculated from cells for which the HSI values were greater than 0.5. This minimized the risk of overestimating the WUA values by large areas with low suitability. To estimate the environmental flows values, the flow scenarios in hydrodynamic models consisted of constant values in the range from 0.02 m3/s to 1.00 m3/s, with steps of 0.02 m3/s (for the section A), and from 0.02 to 1.70 m3/s (for the section B). The flows at which the WUA index reached the highest values represented the range of environmental flows.
2.3 Transferability of Habitat Suitability Criteria
Transferability of reference habitat suitability criteria was verified by comparing separate rasters with HSI values calculated using criteria from the section A and B, and results of hydrodynamic model for the section B (whether using different habitat suitability criteria would yield a similar HSI values). Due to fact that section B was sampled only during low water level, transferability was verified for the flow scenario consistent with the flow rate at the time of sampling, which was equal 0.11 m3/s. Analyses were performed based on HSI rasters values at 100 randomly selected points. Statistical significance of difference between habitat suitability index values was tested using the Wilcoxon test. To determine the magnitude of differences between HSI values, RMSE error and Spearman rank correlation coefficient (ρ) were calculated.
2.4 Hydrological Data and Hydrology-Based Environmental Flows
The hydrological data were observed daily water flows at the water gauge Ryczywół from multi-year period 1951–2014. Characteristic water flows were estimated for the entire years and the months of May-June. Those consisted of: LLQ (the lowest of the low periodic flows), MLQ (mean of the low periodic flows), HLQ (the highest of the low periodic flows), LMQ (the lowest of the mean periodic flows), MMQ (mean of the mean periodic flows), HMQ (the highest of the mean periodic flows), LHQ (the lowest of the high periodic flows), MHQ (mean of the high periodic flows), HHQ (the highest of the high periodic flows). The values for the section A were calculated based on the increase of the catchment area. The analysis of hydrological data also included the determination of the flow duration curves and the values of hydrology-based environmental flows for both studied sections. Hydrology-based environmental flows were calculated using the formula of Kostrzewa (Ozga-Zielińska and Brzeziński, 1994), a method commonly used in Poland.
3 Results
The values of characteristic flows were presented in Table 1. The flow duration curves indicated that discharges in the range of 0.10–0.20 m3/s occurred the most frequently (13.6% of all days in the multiyear period). Considering the months of May-June (months, in which macroinvertebrates samples were collected), flows of 0.10–0.20 m3/s were also the most frequent, but the frequency was about 24%. Additionally, a high frequency was observed for flows below 0.10 m3/s and in the range of 0.20–0.40 m3/s (above 10% for the months of May and June as well as for the entire analyzed period). The obtained values of hydrology-based environmental flows were equaled QnA = 0,12 m3/s (for the section A) and QnB = 0,10 m3/s (for the section B).
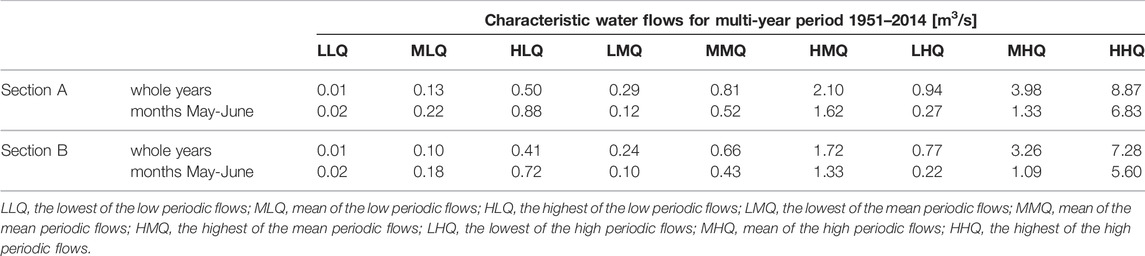
TABLE 1. Characteristic flows from the multi-year period 1951–2014 for the studied sections, based on hydrological data from the Ryczywół water gauge.
Analysis of transferability of habitat suitability criteria indicated that the HSI values obtained for both variants of the criteria showed very similar distributions. The highest differences were noticed for maximum values of the HIS index. For the reference criteria the largest number of points reached values of about 0.95 (Figure 3A). In the variant with the criteria for section B, it was the maximum of suitability value, i.e., 1. The correlation between values of HSI index was statistically significant and Spearman rank correlation coefficient was equaled ρ = 0.86 (Figure 3B). The histogram of differences between HSI index values for both variants of criteria showed that most errors were less than ± 0.10 (Figure 3C). Additionally, the RMSE error was equal 0.06. Statistical analysis showed that the differences between the HSI values calculated based on the criteria from the sections A and B were statistically significant (Wilcoxon test, α = 0.05, p < 0.05). However, taking into account the values of the Spearman rank correlation coefficient, the RMSE error and the magnitude of differences between the HSI index values, it was concluded that habitat suitability criteria from the section A gave relatively good measures of fit to the criteria from the section B, and could be transferred to the degraded river reach.
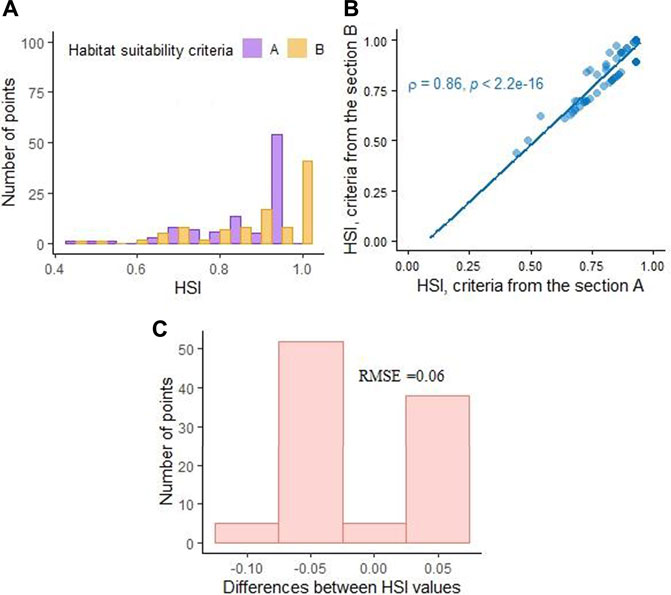
FIGURE 3. Comparison of HSI values. (A)–histogram of HSI values; (B)–relationship between HSI values for criteria from section A and B; (C)–histogram of differences between HSI values.
The shape of the WUA ∼ Q curves for the section A indicated that the area of optimal habitat increases gradually with flows, and approximately reaches maximum values between 0.18–0.43 m3/s, i.e., between MLQV-VI and MMQV-VI (Figure 4A). Thereafter, a gradual decrease was observed, which was probably related to exceeding the water depths and flow velocities limits on the habitat suitability curves. At a flow rate of 1.00 m3/s the WUA index values began to decrease markedly. The different shape of the curve was observed for the section B. The riverbed geometry caused that availability of optimal habitats was maintained even at a flow rate above MMQ. The increase in water depths and velocities with flow rate at the section B, where the channel was wide and had a small slope, was not as rapid as at the section A (Figure 5). In addition, slower increase of hydraulic parameters at the section B could have been affected by the strong overgrowth of the riverbed by macrophytes, which was taken into account in the hydraulic model by means of roughness coefficients. The HHS ∼ Q curves indicated a difference in the maximum values of the HHS index between studied sections (Figure 4B). For the section A it was 0.73, where for the section B 0.92. Additionally, statistical analyses showed that the differences between the HHS index values between sections were statistically significant (Mann-Whitney test for independent groups; α = 0.05; p = 0.82).
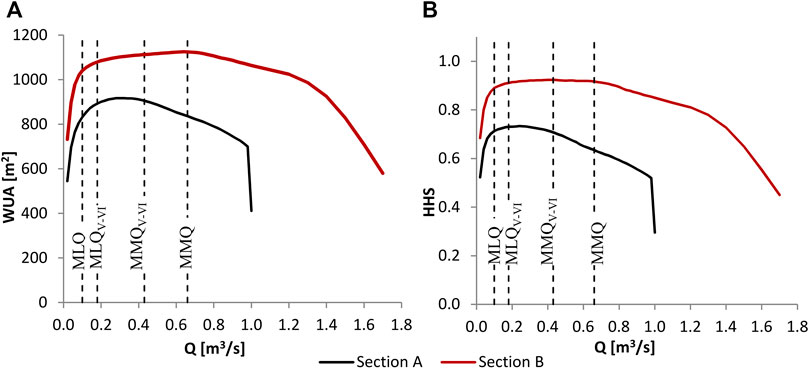
FIGURE 4. WUA ∼ Q (A) and HHS ∼ Q curves (B). MLQ, mean of the low periodic flows; MLQ V-VI, mean of the low periodic flows for the months of May-June; MMQ, mean of the mean periodic flows; MMQ V-VI, mean of the mean periodic flows for the months of May-June.
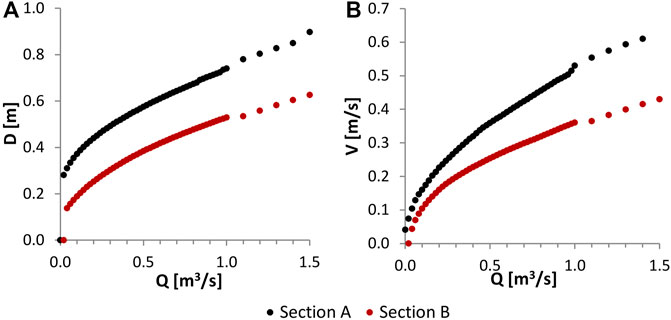
FIGURE 5. Relationships between (A) depths (D) and flow rate, and (B) flow velocities (V) and flow rate.
Environmental flows limits were determined from the WUA ∼ Q curve (Figure 4A). Considering the slopes of the curve, for the section A, the highest values of WUA index occurred in the range of 0.22–0.44 m3/s (mean value of 0.33 m3/s). For the section B it was 0.20–0.80 m3/s, with mean value of 0,50 m3/s (Table 2). The comparison of obtained environmental flows values with hydrology-based environmental flows showed that the lower limits were smaller than QnA and QnB flows by 0.10 m3/s. For the mean values these differences were greater and equaled 0.21 m3/s (section A) and 0.40 m3/s (section B). Comparison to characteristic flows showed that for both river reaches lower limits of the environmental flows were greater than MLQ and were almost the same as MLQV-VI (MLQ for months of May-June; Table 2). For the upper limit of environmental flows for the section A, the value was smaller regards to the MMQ and closer to the MMQV-VI. Conversely, for the section B upper limit was closer to the MMQ flow.
4 Discussion
The statistical analysis showed that differences between HSI values calculated using the criteria from section A and B were statistically significant. However, taking into account the values of the correlation coefficient ρ, the values of the RMSE error and the values of the differences between the HSI index (Figure 3), it was found that the transferability of the criteria could be considered relatively good. The main reason of differences in obtained HSI index values was probably the difference in suitability regards to bottom substrate type. At the section B the bottom substrate was uniform and consisted of category a—fine sand, what is the effect of morphological conditions caused by past regulations and maintenance works. In contrast, in the section A the strong preferences towards fine-grained and coarse-grained sediment were observed (categories f, ce and e). Additionally, during the field measurements, organisms were noticed within a variety of habitats, the availability and diversity of which was much lower at the section B. The obtained result is consistent with other studies, which regards to fish, have shown that the transferability of habitat suitability criteria was better for hydraulic and flow parameters, while considering the cover or bottom substrate caused the values of fit measures to decrease (Millidine et al., 2016; Chen and Olden, 2017).
However, based on the literature, it can be concluded that assessing the transferability of criteria between sections is still a problematic issue in terms of methodology as well as the results obtained, which are strongly dependent on the specificities of the sections analyzed. For example, with regard to fish, Millidine et al. (2016) at two similar sections of a regulated river obtained relatively good values of fit measures, while on sections with different degradation status Moir et al. (2005) showed that the data did not correlate very well with field observations of salmon spawning habitats. Considering macroinvertebrates, studies covered a wide range of samples collected from different rivers and at different seasons showed relatively good (acceptable for the authors) values of fit measures, what may indicate that the large data set allows averaging of habitat suitability criteria regard to environmental parameters (e.g., Dolédec et al., 2007; Jowett et al., 2008; Lamouroux et al., 2013; Theodoropoulos et al., 2018b). The results of transferability obtained in this study are similar to these trends. Nevertheless, Chen and Olden (2017) emphasized that there are currently no guidelines and thresholds that clearly define the lack of transferability of criteria or when the transferability is good. Method proposed by Bovee (1997), which involves performing two χ2 statistical tests for contingency tables, has been repeatedly criticized (Hudson et al., 2003), and with the development of HSMs, other means of testing transferability have been applied, such as the Bayesian Information Criterion (BIC; Millidine et al., 2016), distance functions (Chen and Olden, 2017) and others (Dolédec et al., 2007; Lamouroux et al., 2013; Theodoropoulos et al., 2018b). In this study, the HSI value approach was used, and the final transferability decision was based on several measures of fit.
The differences in HSI index values does not mean that the organisms did not occur at the section B. Manly et al. (1993) stated that in the absence of optimal habitat, organisms are forced to occupy resources that are available, even if they are suboptimal. For this reason, the poor transferability of habitat suitability criteria to regulated river reaches may pose methodological problems. As stated by Bovee et al. (1998), some rivers (or river reaches) are unsuitable for developing habitat suitability criteria because, rather than defining optimal habitats for organisms, they reflect only what species can tolerate. Consequently, in the case of poor transferability it is unclear how to determine habitat suitability criteria for such sections. While literature data for macroinvertebrates in general indicate a fairly universal range of suitability for environmental parameter values and the potential for wider applicability within similar river types (similarity of environmental parameters for highest abundance of organisms and habitat suitability criteria values obtained, e.g., by Jowett et al., 1991; Li et al., 2009; Shearer et al., 2015; Theodoropoulos et al., 2018a), for more specific guilds the criteria may be more diversified. This, coupled with varying environmental conditions in hydromorphologically altered river reaches, can create a great deal of uncertainty associated with estimating optimal environmental flows values.
Following to Papadaki et al. (2017), the shape of the WUA ∼ Q curves is a function of habitat suitability for organisms and reflects the interaction between hydraulic variables and channel geometry as flow increases. The WUA ∼ Q curves indicated how strongly the WUA index values depended on the morphology of the riverbed, which was directly related to differences in the increase of water depths and velocities with flow rate between studied sections (Figure 5). Additionally, beside statistically significant differences between the values of the HHS index, the difference between maximum values was noticed (0.73 for the section A and 0.92 for the section B; Figure 4). This would again confirm the influence of morphological conditions on habitat’s suitability. In the case of the section A, organisms showed greater preferences for bottom substrate categories found primarily within riffles, pools, and shelves (finest and coarsest bottom substrate). The total area of these habitats was smaller than the total area of the channel and thus the maximum HHS index value was much smaller than 1. In the case of the section B, where the channel was uniform and habitat diversity was lower, the maximum of HHS index was also smaller than 1; however the proportion of seemingly useful habitats in the total area of the channel was greater due to habitat suitability criteria for substrate type categories (relatively high value of suitability for the category a—fine sand, which was the only category observed at the section B). Theoretically, that situation could be considered as positive. However, it should be taken into account that in the case of rivers or sections with steeper slopes, it is likely that with increasing of flow rates most habitats would suddenly become non-optimal, because lower habitat diversity may show less buffering capacity relative to increasing hydraulic parameter values (Wyżga et al., 2013). Therefore, it would be advisable to verify the outcomes based on other river sections with similar and different abiotic typology and geometric characteristics. Nevertheless, the obtained results confirmed the hypothesis that morphological conditions impact on values of environmental flow values and weighted usable area.
The analysis of environmental flows showed that the mean value at the section B was higher than that one obtained for the section A (Table 2). This would confirm the hypothesis that the mean value of environmental flow at the regulated river reach is higher compared to the natural reach. However, the obtained differences did not indicate that for the regulated section more water is required to maintain the habitats, since the lower limits of environmental flows for both river reaches were similar (0.22 m3/s and 0.20 m3/s). Due to conflicts of interest, the implemented environmental flows would oscillate around the lower limit rather than the mean values (Theodoropoulos et al., 2018d). It is also worth noting that different ranges of environmental flow values were obtained for the two studied sections (Table 2). Similar results were described by Hudson et al. (2003) and Papadaki et al. (2017). For the Flinta River, the minimum values were similar, whereas this could be a problem if there were large differences. For instance, a flow corresponding to the upper limit for the section B, would result in a decrease in WUA index for the section A of about 13% from the maximum value. For larger rivers that differences may be greater due to additional increase of flow rate along the longitudinal profile and changes of morphometric parameters of the riverbed.
Due to the period of sampling of macroinvertebrates and the definition of environmental flows according to The Brisbane Declaration (Arthington et al., 2018), it should be indicated that environmental flows determined in this study should be referred to the months of May and June, or spring season. According to the flow duration curves, discharges in the range of 0.10–0.40 m3/s are the most frequent in that period. For the section A, the range of environmental flows was close to the most frequent flows, while the upper limit for the section B (i.e., 0.80 m3/s) corresponded to the flows that occurred with a duration of 3% during analyzed multi-year period. Thus, providing that flow rate at the section B would be unlikely. In addition, the range of environmental flows for the section A (0.22–0.44 m3/s) was close to the characteristic flows for the months of May-June MLQV-VI and MMQV-VI (0.22 and 0.52 m3/s) (Tables 1, 2). Therefore, it may be hypothesized that in the case of the natural river reaches, the range of environmental flows coincides with the values that occur most frequently during the analyzed time period. This is consistent with the concept of functional flows (Yarnell et al., 2015) and one of the principle described by Bunn and Arthington (2002), that aquatic species have adapted their life history strategies in direct response to the natural hydrologic regime. In contrast, the results for the section B indicate the divergence that can occur at the regulated river reaches.
Minimum values of environmental flows were greater than hydrology-based environmental flows. Considering lower limits of environmental flows, at the section A hydrology-based value should be higher by 76%, while at the section B by as much as 100%. Furthermore, as mentioned above, environmental flows determined in this study should be referred to the months of May and June, whereas considering the amount and timing of flows (e.g., early spring surges) that discrepancy would be even larger, since hydrology-based flows used in Poland are constant for the entire hydrologic year. Similar results for macroinvertebrates were obtained by Theodoropoulos et al. (2018d), and for fish by Stamou et al. (2018) and Nikghalb et al. (2016), i.e., the lower limits of environmental flows obtained using HSMs, were higher compared to values calculated using classical hydrological methods. This would confirm that values determined using hydrological data alone may not reflect water demands for aquatic organisms (Acreman and Dunbar, 2004). Additionally, Shokoohi and Amini (2014) stated that the use of environmental flows determined based on hydrological methods may lead to degradation of aquatic ecosystems in the long term. Nevertheless, taking into account fact that the values of environmental flows in the section A were close to the most frequent flows and characteristic flows, it might be possible to determine environmental flows based on hydrologic data, but the characteristics used would first have to be extensively verified using HSMs or other methods based on biological elements.
The obtained results highlighted the importance of conducting detailed research on a small spatial scale, especially with regards to river reaches with different degrees of hydromorphological alteration. Estimating the environmental flow values for regulated sections may pose many problems related to transferability of habitat suitability criteria and uncertainty caused by wide range of possible hydromorphological degradations and resultant environmental parameters and organisms’ response. For these reasons, future emphasis should be placed on identifying the broader range of potential diversity and uncertainty associated with assessing environmental flows in regulated river reaches and on developing a framework that would facilitate dealing with discrepancies between natural and regulated rivers. The obtained results indicated potential direction of changes in the values of environmental flows, which were dependent on river geometry and morphological conditions. For that reason, development of abiotic indices for hydromorphologically altered river reaches, based on morphometric parameters, could be helpful in future assessment of environmental flows. Additionally, the range of environmental flows for the natural river reach coincided with the flow values that occurred most frequently in the months of May-June, thus morphometric parameters at the regulated reaches could indicate deviation from natural conditions. Such an observation indicates that wishing to find hydrological proxies of HSM-derived environmental flows one should search beyond the commonly used annual, multi-year river flow statistics, focusing at particular flow hydrogram characteristics that could—most probably—vary depending on river’s hydromorphological and ecological features.
Data Availability Statement
The raw data supporting the conclusion of this article will be made available by the authors, without undue reservation.
Author Contributions
Conceptualization, methodology, investigation, visualization, ES; writing–original draft preparation, ES and MG; writing–review and editing, ES and MG.; supervision, MG and TK. All authors have read and agreed to the published version of the manuscript.
Conflict of Interest
The authors declare that the research was conducted in the absence of any commercial or financial relationships that could be construed as a potential conflict of interest.
Publisher’s Note
All claims expressed in this article are solely those of the authors and do not necessarily represent those of their affiliated organizations or those of the publisher, the editors, and the reviewers. Any product that may be evaluated in this article, or claim that may be made by its manufacturer, is not guaranteed or endorsed by the publisher.
Acknowledgments
Presented results were obtained as a part of a doctoral thesis, carried out at the Department of Hydraulic and Sanitary Engineering of Poznań University of Life Sciences. The publication was co-financed within the framework of Ministry of Science and Higher Education programme as “Regional Initiative Excellence” in years 2019-2022, Project No. 05/RID/2018/19.
References
Acreman, M. C., and Dunbar, M. J. (2004). Defining Environmental River Flow Requirements - a Review. Hydrol. Earth Syst. Sci. 8, 861–876. doi:10.5194/hess-8-861-2004
Ahmadi-Nedushan, B., St-Hilaire, A., Bérubé, M., Robichaud, É., Thiémonge, N., and Bobée, B. (2006). A Review of Statistical Methods for the Evaluation of Aquatic Habitat Suitability for Instream Flow Assessment. River Res. Applic. 22, 503–523. doi:10.1002/rra.918
Arthington, A. H., Bhaduri, A., Bunn, S. E., Jackson, S. E., Tharme, R. E., Tickner, D., et al. (2018). The Brisbane Declaration and Global Action Agenda on Environmental Flows (2018). Front. Environ. Sci. 6, 45. doi:10.3389/fenvs.2018.00045
Bączyk, A., Wagner, M., Okruszko, T., and Grygoruk, M. (2018). Influence of Technical Maintenance Measures on Ecological Status of Agricultural lowland Rivers - Systematic Review and Implications for River Management. Sci. Total Environ. 627, 189–199. doi:10.1016/j.scitotenv.2018.01.235
Biedroń, I., Dubel, A., Grygoruk, M., Pawlaczyk, P., Prus, P., and Wybraniec, K. (2018). Katalog Dobrych Praktyk W Zakresie Robót Hydrotechnicznych I Prac Utrzymaniowych Wraz Z Ustaleniem Zasad Ich Wdrażania. Państwowe Gospodarstwo Wodne Wody Polskie. Warszawa: Krajowy Zarząd Gospodarki Wodnej.
Bovee, K. D. (1997). Data Collection Procedures for the Physical Habitat Simulation System. Fort Collins, CO: U.S.
Bovee, K. D. (1986). Development and Evaluation of Habitat Suitability Criteria for Use in the Instream Flow Incremental Methodology. USDI Fish and Wildlife Service. Instream Flow Information Paper #21 FWS/OBS-86/7. Washington (DC)Fort Collins, Colorado: Geological Survey, Biological Resources Division, Mid-Continent Ecological Science Centre.
Bovee, K. D., Lamb, B. L., Bartholow, J. M., Stalknaker, C. B., Taylor, J., and Henriksen, J. (1998). Stream Habitat Analysis Using the Instream Flow Incremental Methodology. Washington, D.C.: USGS Information and Technology Report1998–0004, 1–130.
Bunn, S. E., and Arthington, A. H. (2002). Basic Principles and Ecological Consequences of Altered Flow Regimes for Aquatic Biodiversity. Environ. Manage. 30 (4), 492–507. doi:10.1007/s00267-002-2737-0
Chen, W., and Olden, J. D. (2017). Evaluating Transferability of Flow-Ecology Relationships across Space, Time and Taxonomy. Freshw. Biol. 63, 817–830. doi:10.1111/fwb.13041
Clarke, K. R., and Warwick, R. M. (2001). in PRIMER-E. 2nd Edition (Plymouth, United Kingdom: Plymouth Marine Laboratory). Changes in Marine Communities: An Approach to Statistical Analysis and Interpretation.
Dolédec, S., Lamouroux, N., Fuchs, U., and Mérigoux, S. (2007). Modelling the Hydraulic Preferences of Benthic Macroinvertebrates in Small European Stream. Freshw. Biol. 52, 145–164.
Dunbar, M. J., Alfredsen, K., and Harby, A. (2011). Hydraulic-habitat Modelling for Setting Environmental River Flow Needs for Salmonids. Fish. Manag. Ecol. 19, 500–517. doi:10.1111/j.1365-2400.2011.00825.x
EEA - European Environment Agency (2012). European Waters – Assessment of Status and Pressures. Copenhagen, Denmark: EEA. EEA Report No 8/2012, ISBN 978-92-9213-339-9.
Emery, J. C., Gurnell, A. M., Clifford, N. J., Petts, G. E., Morrissey, I. P., and Soar, P. J. (2003). Classifying the Hydraulic Performance of Riffle-Pool Bedforms for Habitat Assessment and River Rehabilitation Design. River Res. Applic. 19, 533–549. doi:10.1002/rra.744
European Commission (2015). Ecological Flows in the Implementation of the Water Framework Directive. WFD CIS Guidance Document No. 31.
Feld, C. K., and Hering, D. (2007). Community Structure or Function: Effects of Environmental Stress on Benthic Macroinvertebrates at Different Spatial Scales. Freshw. Biol 52, 1380–1399. doi:10.1111/j.1365-2427.2007.01749.x
Friberg, N., Sandin, L., and Pedersen, M. L. (2009). Assessing the Effects of Hydromorphological Degradation on Macroinvertebrate Indicators in Rivers: Examples, Constraints, and Outlook. Integr. Environ. Assess. Manag. 5 (1), 86–96. doi:10.1897/ieam_2008-042.1
Gąbka, M., Jakubas, E., Janiak, T., and Golski, J. (2014). “Rzeki Wełna I Flinta – Charakterystyka Obiektów Badań, Ich Położenie I Granice Zlewni,” in Koncepcja Lasu Modelowego W Zarządzaniu I Ochronie Różnorodności Biologicznej Rzek Wełny I Flinty (Wielkopolska). Editors J. Batora, M. Gąbka, and E. Jakubas (Bogucki Wydawnictwo Naukowe, Poznań), 21–30. doi:10.1897/ieam_2008-042.1
Gillespie, B. R., Desmet, S., Kay, P., Tillotson, M. R., and Brown, L. E. (2015). A Critical Analysis of Regulated River Ecosystem Responses to Managed Environmental Flows from Reservoirs. Freshw. Biol. 60, 410–425. doi:10.1111/fwb.12506
Graeber, D., Pusch, M. T., Lorenz, S., and Brauns, M. (2013). Cascading Effects of Flow Reduction on the Benthic Invertebrate Community in a lowland River. Hydrobiologia 717, 147–159. doi:10.1007/s10750-013-1570-1
Grygoruk, M., Frąk, M., and Chmielewski, A. (2015). Agricultural Rivers at Risk: Dredging Results in a Loss of Macroinvertebrates. Preliminary Observations from the Narew Catchment, Poland. Water 7, 4511–4522. doi:10.3390/w7084511
Guareschi, S., Laini, A., Racchetti, E., Bo, T., Fenoglio, S., and Bartoli, M. (2014). How Do Hydromorphological Constraints and Regulated Flows Govern Macroinvertebrate Communities along an Entire lowland River? Ecohydrol. 7, 366–377. doi:10.1002/eco.1354
Harman, C., and Stewardson, M. (2005). Optimizing Dam Release Rules to Meet Environmental Flow Targets. River Res. Applic. 21, 113–129. doi:10.1002/rra.836
Holt, C. R., Pfitzer, D., Scalley, C., Caldwell, B. A., and Batzer, D. P. (2014). Macroinvertebrate Community Responses to Annual Flow Variation from River Regulation: An 11-Year Study. River Res. Applic. 31, 798–807. doi:10.1002/rra.2782
Huang, J., and Frimpong, E. A. (2016). Limited Transferability of Stream‐fish Distribution Models Among River Catchments: Reasons and Implications. Freshw. Biol. 61, 729–744. doi:10.1111/fwb.12743
Hudson, H. R., Byrom, A. E., and Chadderton, W. L. (2003). A Critique of IFIM —Instream Habitat Simulation in the New Zealand Context. Wellington, New Zealand: Science for Conservation 231. Published by the Department of Conservation, PO Box 10420.
Jakubas, E., Gąbka, M., Szoszkiewicz, K., Pietruczuk, K., Szwabińska, M., Litka, A., et al. (2014). “Ocena Stanu Ekologicznego I Zmian Hydromorfologicznych Rzek Wełny I Flinty,” in Koncepcja Lasu Modelowego W Zarządzaniu I Ochronie Różnorodności Biologicznej Rzek Wełny I Flinty (Wielkopolska). Editors J. Batora, M. Gąbka, and E. Jakubas (Poznań: Bogucki Wydawnictwo Naukowe), 141–150.
Jowett, I. G., Hayes, J. W., and Duncan, M. J. (2008). A Guide to Instream Habitat Survey Methods and Analysis, 54. Wellington, New Zealand: NIWA Science and Technology Series.
Jowett, I. G. (2003). Hydraulic Constraints on Habitat Suitability for Benthic Invertebrates in Gravel-Bed Rivers. River Res. Applic. 19, 495–507. doi:10.1002/rra.734
Jowett, I. G., Richardson, J., Biggs, B. J. F., Hickey, C. W., and Quinn, J. M. (1991). Microhabitat Preferences of Benthic Invertebrates and the Development of generalised Deleatidium spp. Habitat Suitability Curves, Applied to Four New Zealand Rivers. New Zealand J. Mar. Freshw. Res. 25 (2), 187–199. doi:10.1080/00288330.1991.9516470
Lamouroux, N., Mérigoux, S., Dolédec, S., and Snelder, T. H. (2013). Transferability of Hydraulic Preference Models for Aquatic Macroinvertebrates. River Res. Applic. 29, 933–937. doi:10.1002/rra.2578
Leitner, P., Hauer, C., and Graf, W. (2017). Habitat Use and Tolerance Levels of Macroinvertebrates Concerning Hydraulic Stress in Hydropeaking Rivers - A Case Study at the Ziller River in Austria. Sci. Total Environ. 575, 112–118. doi:10.1016/j.scitotenv.2016.10.011
Leszczyńska, J., Głowacki, Ł., and Grzybkowska, M. (2017). Factors Shaping Species Richness and Biodiversity of Riverine Macroinvertebrate Assemblages at the Local and Regional Scale. Community Ecol. 18 (3), 227–236.
Li, F., Cai, Q., Fu, X., and Liu, J. (2009). Construction of Habitat Suitability Models (HSMs) for Benthic Macroinvertebrate and Their Applications to Instream Environmental Flows: A Case Study in Xiangxi River of Three Gorges Reservior Region, China. Prog. Nat. Sci. 19, 359–367. doi:10.1016/j.pnsc.2008.07.011
Lorenz, A., Hering, D., Feld, C. K., and Rolauffs, P. (2004). A New Method for Assessing the Impact of Hydromorphological Degradation on the Macroinvertebrate Fauna of Five German Stream Types. Hydrobiologia 516, 107–127. doi:10.1023/b:hydr.0000025261.79761.b3
Manly, B. F. J., McDonald, L. L., and Thomas, D. L. (1993). Resource Selection by Animals. London: Chapman & Hall.
Mérigoux, S., Lamouroux, N., Olivier, J-M., and Dolédec, S. (2009). Invertebrate Hydraulic Preferences and Predicted Impacts of Changes in Discharge in a Large River. Freshw. Biol. 54, 1343–1356.
Millidine, K. J., Malcolm, I. A., and Fryer, R. J. (2016). Assessing the Transferability of Hydraulic Habitat Models for Juvenile Atlantic salmon. Ecol. Indicators 69, 434–445. doi:10.1016/j.ecolind.2016.05.012
Moir, H. J., Gibbins, C. N., Soulsby, C., and Youngson, A. F. (2005). PHABSIM Modelling of Atlantic salmon Spawning Habitat in an upland Stream: Testing the Influence of Habitat Suitability Indices on Model Output. River Res. Applic. 21, 1021–1034. doi:10.1002/rra.869
Nikghalb, S., Shokoohi, A., Singh, V. P., and Yu, R. (2016). Ecological Regime versus Minimum Environmental Flow:Comparison of Results for a River in a Semi Mediterranean Region. Water Resour. Manage. 30, 4969–4984. doi:10.1007/s11269-016-1488-2
Olden, J. D., and Naiman, R. J. (2010). Incorporating thermal Regimes into Environmental Flows Assessments: Modifying Dam Operations to Restore Freshwater Ecosystem Integrity. Freshw. Biol. 55, 86–107. doi:10.1111/j.1365-2427.2009.02179.x
Ozga-Zielińska, M., and Brzeziński, J. (1994). Hydrologia Stosowana. Warszawa: Wydawnictwo naukowe PWN.
Pahl-Wostl, C., Arthington, A., Bogardi, J., Bunn, S. E., Hoff, H., Lebel, L., et al. (2013). Environmental Flows and Water Governance: Managing Sustainable Water Uses. Curr. Opin. Environ. Sustainability 5, 341–351. doi:10.1016/j.cosust.2013.06.009
Papadaki, C., Soulis, K., Muñoz-Mas, R., Martinez-Capel, F., Zogaris, S., Ntoanidis, L., et al. (2016). Potential Impacts of Climate Change on Flow Regime and Fish Habitat in Mountain Rivers of the South-Western Balkans. Sci. Total Environ. 540, 418–428. doi:10.1016/j.scitotenv.2015.06.134
Papadaki, C., Soulis, K., Ntoanidis, L., Zogaris, S., Dercas, N., and Dimitriou, E. (2017). Comparative Assessment of Environmental Flow Estimation Methods in a Mediterranean Mountain River. Environ. Manage. 60, 280–292. doi:10.1007/s00267-017-0878-4
Pasternack, G. B., Gilbert, A. T., Wheaton, J. M., and Buckland, E. M. (2006). Error Propagation for Velocity and Shear Stress Prediction Using 2D Models for Environmental Management. J. Hydrol. 328, 227–241. doi:10.1016/j.jhydrol.2005.12.003
Pasternack, G. B., Wang, C. L., and Merz, J. E. (2004). Application of a 2D Hydrodynamic Model to Design of Reach-Scale Spawning Gravel Replenishment on the Mokelumne River, California. River Res. Applic. 20, 205–225. doi:10.1002/rra.748
Phillips, J. V., and Tadayon, S. (2007). Selection of Manning’s Roughness Coefficient for Natural and Constructed Vegetated and Non-vegetated Channels, and Vegetation Maintenance Plan Guidelines for Vegetated Channels in Central Arizona. U.S. Geological Survey Scientific Investigations Report, 2006–5108.
Poff, N. L., and Zimmerman, J. K. H. (2010). Ecological Responses to Altered Flow Regimes: a Literature Review to Inform the Science and Management of Environmental Flows. Freshw. Biol. 55, 194–205. doi:10.1111/j.1365-2427.2009.02272.x
Renöfält, B. M., Jansson, R., and Nilsson, C. (2010). Effects of Hydropower Generation and Opportunities for Environmental Flow Management in Swedish Riverine Ecosystems. Freshw. Biol. 55, 49–67.
Richter, B. D., and Thomas, G. A. (2007). Restoring Environmental Flows by Modifying Dam Operations. E&S 12 (1), 12. doi:10.5751/es-02014-120112
Rosenfeld, J. S. (2017). Developing Flow-Ecology Relationships: Implications of Nonlinear Biological Responses for Water Management. Freshw. Biol. 62, 1305–1324. doi:10.1111/fwb.12948
Shearer, K., Hayes, J., Jowett, I., and Olsen, D. (2015). Habitat Suitability Curves for Benthic Macroinvertebrates from a Small New Zealand River. New Zealand J. Mar. Freshw. Res. 49, 178–191. doi:10.1080/00288330.2014.988632
Shokoohi, A., and Amini, M. (2014). Introducing a New Method to Determine Rivers' Ecological Water Requirement in Comparison with Hydrological and Hydraulic Methods. Int. J. Environ. Sci. Technol. 11, 747–756. doi:10.1007/s13762-013-0404-z
Stamou, A., Polydera, A., Papadonikolaki, G., Martínez-Capel, F., Muñoz-Mas, R., Papadaki, C., et al. (2018). Determination of Environmental Flows in Rivers Using an Integrated Hydrological-Hydrodynamic-Habitat Modelling Approach. J. Environ. Manage. 209, 273–285. doi:10.1016/j.jenvman.2017.12.038
Szlauer-Łukaszewska, A., and Zawal, A. (2014). The Impact of River Dredging on Ostracod Assemblages in the Krąpiel River (NW Poland). Fundam. Appl. Limnol. 185, 295–305.
Szoszkiewicz, K., Jusik, S., Adynkiewicz-Piragas, M., Gebler, D., Achtenberg, K., Radecki-Pawlik, A., et al. (2017). Podręcznik Oceny Wód Płynących W Oparciu O Hydromorfologiczny Indeks Rzeczny. Inspekcja Ochrony Środowiska. Warszawa: Biblioteka Monitoringu Środowiska.
Theodoropoulos, C., Georgalas, S., Mamassis, N., Stamou, A., Rutschmann, P., and Skoulikidis, N. (2018d). Comparing Environmental Flow Scenarios from Hydrological Methods, Legislation Guidelines, and Hydrodynamic Habitat Models Downstream of the Marathon Dam (Attica, Greece). Ecohydrology 11 (8), e2019. doi:10.1002/eco.2019
Theodoropoulos, C., Skoulikidis, N., Rutschmann, P., and Stamou, A. (2018c). Ecosystem-based Environmental Flow Assessment in a Greek Regulated River with the Use of 2D Hydrodynamic Habitat Modelling. River Res. Applic. 34 (6), 538–547. doi:10.1002/rra.3284
Theodoropoulos, C., Skoulikidis, N., Stamou, A., and Dimitriou, E. (2018b). Spatiotemporal Variation in Benthic-Invertebrates-Based Physical Habitat Modelling: Can We Use Generic Instead of Local and Season-specific Habitat Suitability Criteria? Water 10, 1508. doi:10.3390/w10111508
Theodoropoulos, C., Vourka, A., Skoulikidis, N., Rutschmann, P., and Stamou, A. (2018a). Evaluating the Performance of Habitat Models for Predicting the Environmental Flow Requirements of Benthic Macroinvertebrates. J. Ecohydraulics 3 (1), 30–44. doi:10.1080/24705357.2018.1440360
Thomas, J. A., and Bovee, K. D. (1993). Application and Testing of a Procedure to Evaluate Transferability of Habitat Suitability Criteria. Regul. Rivers: Res. Mgmt. 8, 285–294. doi:10.1002/rrr.3450080307
Tonina, D., and Jorde, K. (2013). “Hydraulic Modelling Approaches for Ecohydraulic Studies: 3D, 2D, 1D and Non-numerical Models,” in Ecohydraulics : An Integrated Approach. Editors I. Maddock, A. Harby, P. Kemp, and P. Wood (Hoboken, USA: John Wiley & Sons), 31–74. doi:10.1002/9781118526576.ch3
Urbanic, G. (2012). Hydromorphological Degradation Impact on Benthic Invertebrates in Large Rivers in Slovenia. Hydrobiologia 729 (1), 191–207.
Waddle, T. J., and Holmquist, J. G. (2011). Macroinvertebrate Response to Flow Changes in a Subalpine Stream: Predictions from Two-Dimensional Hydrodynamic Models. River Res. Applic. 29, 366–379. doi:10.1002/rra.1607
Wright, K. A., Goodman, D. H., Som, N. A., Alvarez, J., Martin, A., and Hardy, T. B. (2017). Improving Hydrodynamic Modelling: an Analytical Framework for Assessment of Two-Dimensional Hydrodynamic Models. River Res. Applic. 33, 170–181. doi:10.1002/rra.3067
Wyżga, B., Oglęcki, P., Radecki-Pawlki, A., and Zawiejska, J. (2013). “Diversity of Macroinvertebrate Communities as a Reflection of Habitat Heterogeneity in a Mountain River Subjected to Variable Human Impacts,” in Stream Restoration in Dynamic Fluvial Systems. Editors A. Simon, S. J. Bennett, and J. M. Castro (American Geophysical Union).
Keywords: environmental flows, sustainability, river regulation, macroinvertbrates, habitat preference curves, habitat suitability criteria, habitat suitability curves
Citation: Szałkiewicz E, Kałuża T and Grygoruk M (2022) Environmental Flows Assessment for Macroinvertebrates at the River Reach Scale in Different Degrees of Hydromorphological Alteration. Front. Environ. Sci. 10:866526. doi: 10.3389/fenvs.2022.866526
Received: 31 January 2022; Accepted: 23 March 2022;
Published: 11 April 2022.
Edited by:
Rui Pedro Rivaes, University of Lisbon, PortugalReviewed by:
Ji-Zhong Wan, Qinghai University, ChinaRui Manuel Vitor Cortes, University of Trás-os-Montes and Alto Douro, Portugal
Copyright © 2022 Szałkiewicz, Kałuża and Grygoruk. This is an open-access article distributed under the terms of the Creative Commons Attribution License (CC BY). The use, distribution or reproduction in other forums is permitted, provided the original author(s) and the copyright owner(s) are credited and that the original publication in this journal is cited, in accordance with accepted academic practice. No use, distribution or reproduction is permitted which does not comply with these terms.
*Correspondence: Ewelina Szałkiewicz, c29ubmVuYmVyZ2V3ZWxpbmFAZ21haWwuY29t