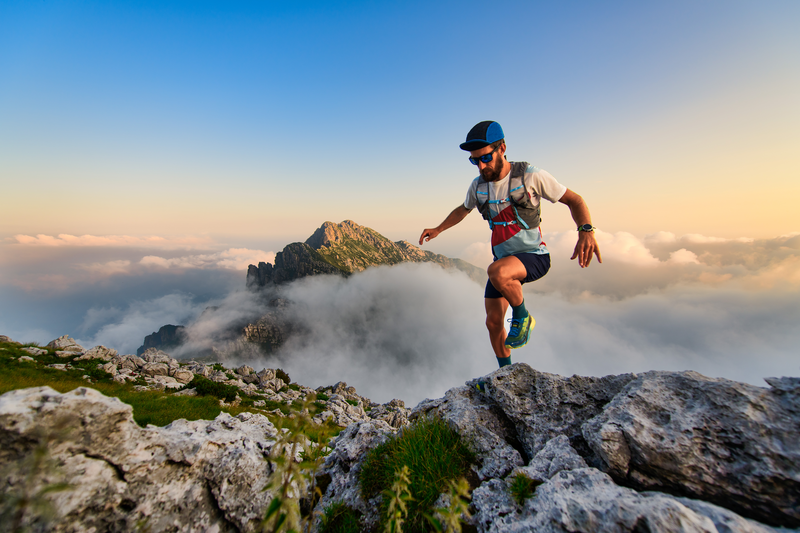
94% of researchers rate our articles as excellent or good
Learn more about the work of our research integrity team to safeguard the quality of each article we publish.
Find out more
BRIEF RESEARCH REPORT article
Front. Environ. Sci. , 06 April 2022
Sec. Freshwater Science
Volume 10 - 2022 | https://doi.org/10.3389/fenvs.2022.863508
Macrophyte communities have major role in the functioning of freshwater ecosystems. However, there is gap in knowledge about how natural and anthropogenic hydro-morphological disturbances affect their functional diversity and trait structure, particularly in the temperate large rivers. In this study we investigated the effect of hydro-morphology on functional diversity and trait structure of macrophyte communities in the middle section of the Danube course. We collected macrophyte and environmental data from 947 sampling units in the main river channel and connected side waterbodies. We extracted data on 18 traits with 65 trait states and calculated seven functional diversity metrics and cumulative weighted means of trait states (CWMs). We applied redundancy analysis (RDA) to investigate the response of functional diversity metrics to the environmental variables, and Variation Partitioning to determine whether natural, or anthropogenic subset of hydro-morphological factors is more important predictor of functional diversity. To relate CWMs and environmental variables, we performed RLQ and fourth-corner analysis, followed by false discovery rate procedure. Hydro-morphological variables explained 36.7% of the variability in the functional diversity metrics. Combined effect of two subsets of environmental variables explained largest part of the variability in functional diversity metrics. Six associations between traits and environmental variables were found. We found that functional diversity metrics indicate prevailing ecological processes, from environmental to biotic filtering, along the natural—anthropogenic hydro-morphological gradient. We concluded that functional diversity metrics are potentially useful tools in the identification of the causes of ecological degradation, and could be applied in river bioassessments and management.
Ecological theory suggests that multiple environmental factors shape local species assemblages by progressively filtering species from the regional species pool to local communities (Baattrup-Pedersen et al., 2015), and one of the main challenges in community ecology is understanding the processes driving the functional structure of the species assemblage (Villégér et al., 2010). Three types of ecological processes, or filters, produce non-random patterns in the community structure, acting on species traits, rather than on species themselves: the abiotic environment, biotic interactions, and dispersal (McGill et al., 2006; Violle et al., 2007; Lukács et al., 2019). Species traits are morphological, physiological, and phenological features measurable at the species or individual level (Violle et al., 2007). Abiotic environment acts as filter selecting species with suitable traits that can persist in the given habitat; therefore, increasing similarity in trait composition and reducing functional diversity (MacArthur and Levins, 1967; Kraft et al., 2008). Biotic interactions, or niche differentiation, usually prevail on a finer scale where co-existing species have small overlap in their functional niches, usually by competitive exclusion; leading to divergent traits and increased functional diversity (Mason et al., 2007). The third filter influences the community structure by selecting species according to their ability to disperse to a site (Paz et al., 2021). Interaction of these three processes is complex, and can lead to shifts in the community structure, changing the abundance and/or presence of species (Cadotte and Tucker, 2017). Disturbances could lead to changes in the interaction and relative importance of filters in the community structure, acting directly through the loss of species, or indirectly by modifying environmental conditions (Baattrup-Pedersen et al., 2003; Rambaud et al., 2009). According to the stress-dominance hypothesis, environmental filtering is important under stressful, disturbed conditions, while biotic interactions are more important in structuring communities in less disturbed environments (Weiher and Keddy, 1995; Swenson and Enquist, 2007; Lukács et al., 2019).
The establishment and structure of macrophyte communities in rivers are largely determined by physical factors (i.e., water current, light availability, bottom substrate) that combine the effects of water and sediment chemistry on community composition (Butcher, 1933; Canfield and Hoyer, 1988; Baattrup-Pedersen and Riis, 1999; Riis et al., 2001; Riis and Biggs, 2003; Daniel et al., 2006; Janauer et al., 2010; O'Hare et al., 2010; Steffen et al., 2013). Macrophyte communities are strongly influenced by river hydrology, reflecting both anthropogenic and natural disturbances, where these environmental factors have stronger effect on the trait composition than on the species composition of the community (Papastergiadou et al., 2016; Göthe et al., 2017; Baattrup-Pedersen et al., 2018; Bejarano et al., 2018). Due to the great potential that functional diversity and trait composition of macrophyte communities might have in bioindication of hydro-morphological disturbances in running water-bodies, and its potential importance in river management, there is a growing number of studies investigating the influence of these factors on the trait distribution and/or functional diversity of macrophyte communities (e.g., Baattrup-Pedersen et al., 2016; Göthe et al., 2017; Lukács et al., 2019; Manolaki et al., 2020; Paz et al., 2021; Stefanidis et al., 2021). However, there is still a lack of data on the effects that hydro-morphological disturbances have on the functional diversity and trait composition of macrophyte communities in a large, heavily modified river, such as Danube. After the millennia of human activities on its banks, Danube is far from being natural river (Olson and Krug, 2020; Janauer et al., 2021). The most extensive interferences with Danube course are dams of the hydro-power plants, causing discontinuities in river flow and disruption of longitudinal connectivity, and consequently a series of environmental changes, including decreased water flow, increased sedimentation, increased transparency, etc. (Birk et al., 2012). It was found that free-running sections of the Danube River are poorer in number and abundance of macrophyte species than the impounded reaches (Janauer et al., 2021).
In the middle section of the Danube River (the same section that was analyzed here), it was found that species diversity and the abundance of macrophyte assemblages increased in the downstream direction towards the dams (i.e., in the run of the river reservoirs), and were negatively correlated to the riparian zone width (Vukov et al., 2018). In the impoundments, discontinuity in flow imposed by anthropogenic alteration—damming, and loss of riparian shading, alleviate many of the constrains to the plant growth found in the free-running section (water velocity is lower, sedimentation is increased, transparency is higher), while the higher retention time leads to accumulation of nutrients and eutrophication that contribute to macrophyte biomass production (Smith et al., 2006; O’Hare et al., 2010; Nõges et al., 2016). It was concluded that macrophytic cover and species diversity in the main channel of the Danube River in its middle course indicates major anthropogenic impact—damming, and consequent eutrophication (Vukov et al., 2018). Since the environmental filters act on species traits, rather than on species themselves, the main idea behind this study was to analyze the response of the trait based functional diversity indices and trait structure of macrophyte communities to the set of environmental variables used in previous study (Vukov et al., 2018) in order to compare it to the response observed previously for the diversity of macrophyte communities on a species level.
Therefore, the aims of this study were: 1) to investigate the effects that hydro-morphological disturbances, both natural and anthropogenic, have on the functional diversity of macrophyte communities in the middle section of the Danube River; and 2) to identify macrophyte traits that respond to the analyzed hydro-morphological factors. Achieving these objectives will provide an insight into the prevailing ecological processes shaping macrophyte communities in the heavily modified water bodies, with possible implications regarding the river bioassessment and management.
Original data on the abundance and distribution of 49 macrophyte species (Supplementary Table S1), and on eleven environmental parameters (Table 1, Supplementary Table S2), in 947 sampling units were collected during the survey of the Danube River in Serbia done in the summer of 2014 (Vukov et al., 2018). A survey of macrophytes was conducted according to European Standard EN 14184:2014 (2014), using a five-level scale for species abundance estimation (1—very rare; 2—rare; 3—frequent; 4—abundant; 5—very abundant) in relation to the total volume and length of the sampling unit. Studied section of the Danube River is located between river km 1433 and 846. Survey was done in the river’s main channel and in the side channels and side arms with surface inflow. Studied river reach was divided into 1081 contiguous sampling units that were 1 km long in the main channel, and of varying length in the side channels and side arms. Left and right riversides were surveyed separately. Survey was done in the whole length of the sampling unit visually and/or by raking, using small boat. In this study we have used data from 947 sampling units in which macrophytes have been recorded.
TABLE 1. Environmental variables used in data analyses, with grouping applied for the Variation Partitioning (VarPart) procedure.
Studied section of the Danube River is located between river km 1433 and 846. The upstream part of the surveyed reach (Pannonian Plain Danube) runs through a floodplain landscape (avg. slope is 0.04‰), with an average width of approximately 750 m, and a mean depth of 6 m. At km 1071, the Danube enters Đerdap gorge (Iron Gate, slope gradient of the riverbed ranges from 0.04‰ to 0.25‰, average width of the river is 750 m). From km 931 the river again runs through a floodplain landscape (avg. slope 0.04‰). Average width is 830 m, and mean depth is 8.5 m. In the Đerdap section, two hydropower plants with large dams were built: Iron Gate 1 at km 943 (60 m high, 1278 m bank to bank, operational since 1972); Iron Gate 2 at km 863 (35 m high, 412 m bank to bank, operational since 1984). These modifications resulted in creation of two contiguous runs of river reservoirs. The entire studied river reach is part of the Danube navigation corridor.
Data on 18 macrophyte traits and 65 trait states were extracted from the literature (Borhidi, 1993; Willby et al., 2000; Table 2). Traits: Rooting at nodes, High below: above-ground biomass, Evergreen leaf, and Amphibius, had binary states (0 meaning the trait is absent, and 1 meaning the trait is present). States of other traits had values: 0 for absence, 1 for occasional but not general presence, and 2 for general presence of the trait state (Willby et al., 2000; Baattrup-Pedersen et al., 2016).
Macrophyte community data and macrophyte trait states data were used to compute distance-based multi-trait diversity indices: functional richness (FRic—represents the amount of functional space filled by a community); functional evenness (FEve—measures the regularity of the abundance distribution of the species along the minimum spanning tree that links the species points in multidimensional functional space); functional divergence (FDiv—relates to how species abundances are distributed within the functional trait space); functional dispersion (FDis—represents the mean distance of individual species to the centroid of all species in the multidimensional trait space, taking into account the species relative abundances); Rao quadratic entropy (RaoQ—the sum of the dissimilarities between all possible pairs of species, weighted by the product of species proportions) (Botta-Dukát, 2005; Villéger et al., 2008; Laliberté and Legendre, 2010; Borcard et al., 2018). Also, the computation of the community-level weighted means of trait states values (CWMs) was added to the framework, although CWMs are not strictly diversity indices but represent the functional composition of the community (Lavorel et al., 2008). Since the trait states values were not quantitative and continuous, functional trait space is obtained from a PCoA (Principal Coordinate Analysis) of the Gower dissimilarity matrix computed on the trait states matrix (Borcard et al., 2018). de Bello et al. (2010) proposed a unified framework for computing community diversity indices, based on the Rao quadratic entropy. In this framework, taxonomic (species) diversity (TD) reduces to the inverse Simpson diversity, while the functional diversity (FD) is the sum of dissimilarities in functional traits between all possible pairs of species, weighted by the product of species proportions (Borcard et al., 2018). Since the inverse Simpson diversity (TD) represents the potential maximum value of FD, the difference between TD and FD is a measure of functional redundancy (FRed). Computation of functional diversity metrics was done using “FD” package (Laliberté et al., 2014), with descriptive statistics done using “summarytools” (Comtois, 2021) in R software environment (R version 4.1.2).
In this paper, we will refer to functional diversity indices: functional richness (FRic), functional evenness (FEve), functional divergence (FDiv), functional dispersion (FDis), Rao quadratic entropy (RaoQ), functional diversity (FD), and functional redundancy (FRed) as functional diversity metrics, while cumulated weighted means of macrophyte trait states will be referred to as CWMs and/or functional composition.
In the Redundancy analysis (RDA), obtained functional diversity metrics (except CWMs) were constrained by the environmental variables. Prior to the analysis, both functional metrics and environmental variables were standardized. Linear dependencies between constraining variables were checked using Variance Inflation Factor indices, and the correlation between explanatory and response variables was extracted from the RDA model. The statistical significance of the RDA (global model) and that of the individual canonical axes were tested using permutation tests. The unbiased amount of variation in functional diversity measures explained by the environmental variables was measured as adjusted R2 and was used later in the Variation Partitioning. Forward Selection was performed to determine the order of importance of the environmental variables in the variation of functional diversity metrics, with the adjusted R2 value as a threshold. To distinguish between the amount of variation in the functional diversity metrics explained by hydro-morphological variables, on one side, and their alterations on the other, Variation Partitioning was performed. For that purpose, environmental variables were divided into: Hydro-morphology (river_km, flw_vlc, trnspr, bnk_txt, sdm_txt, ripz_w) and Alterations (dam_dst, prt_bnk, trn_str, ripz_hem). Analyses were done using R package “vegan” (Oksanen et al., 2020).
Relating CWM matrices to environmental variables representing gradients through linear models (e.g., correlation, regression, RDA) has been strongly criticized (Peres-Neto et al., 2017). When only the traits or only the environmental variables are important in structuring the species distributions, tests of correlations based on this approach have strongly inflated type I error, therefore the fourth-corner approach is recommended (Borcard et al., 2018). To evaluate the relationships between species traits (Q) and environmental data (R), mediated by the species abundance data (L), two methods were applied: RLQ (Dolédec et al., 1996) and fourth-corner analysis (Legendre et al., 1997; Dray and Legendre, 2008; Legendre and Legendre, 2012). These two methods are complementary (Dray et al., 2014), where RLQ is an ordination method allowing the visualization of the joint structure resulting from the three data tables, but with a single global test, while the fourth-corner analysis consists in a series of statistical tests of individual trait-environment relationships. Since the four-corner method involves multiple tests, the overall rate of type I error is increased, correction for multiple testing should be performed (Legendre et al., 1997; Dray et al., 2014). Here, the false discovery rate method with α = 0.05 (FDR; Benjamini and Hochberg, 1995; Borcard et al., 2018) was applied. RLQ and fourth-corner analysis were done using the “ade4” R package (Dray et al., 2021).
The environmental variables included in the RDA model (Figure 1A) explained 36.7% of the variation in functional diversity metrics (R2 adjusted = 0.367). The first RDA axis alone explained 35.48% of variation in the response data. It is worth mentioning that the first residual eigenvalue (PC1 representing 46.13% of the variance in response data) is larger than the first canonical eigenvalue (RDA1) in the obtained RDA model, indicating that there could be other factors responsible for the variability in the response data. Variables: river_km (r = −0.793), dam_dst (r = −0.752), and ripz_w (r = −0.545) were strongly correlated with the first RDA axis, whereas the highest correlation with the second axis was detected for trnspr (r = −0.595) and sdm_txt (r = −0.653). Permutation tests of RDA results showed that the RDA model, as well as the first RDA axis, was statistically highly significant. According to the forward selection procedure, eight variables are enough to reach the R2 adjusted threshold. Their order of importance is: river_km, trnspr, dam_dst, sdm_txt. cnnctv, bnk_txt, ripz_w, prt_bnk. Highest absolute values (above 0.30) of the correlation coefficients extracted from the RDA were recorded between all functional diversity metrics and variables: river_km, dam_dst, and ripz_w (Supplementary Table S3). The strongest correlation was found between river_km and FDiv (−0.518), followed by FRed (−0.514), FEve (−0.513), FDis (−0.490), RaoQ (−0.475), FD (−0.452), and FRic (−0.378). The correlation coefficient between dam_dst and FDiv was −0.486; followed by FRed (−0.484), FEve (−0.473), FDis (−0.448), RaoQ (−0.439), FD (−0.429), and FRic (−0.410). Correlation between ripz_w and diversity metrics was: FRed (−0.376), RaoQ (−0.332), FDis (−0.328), FD (−0.325), FDiv (−0.315), FRic (−0.310), and FEve (−0.304).
FIGURE 1. (A) RDA plot of the environmental predictors and functional diversity metrics as response variables, scaling 2 applied. (B) Venn diagrams of the variation partitioning. Functional diversity metrics: FRic—functional richness; FEve—functional evenness; FDiv—functional divergence; FDis—functional dispersion; RaoQ—Rao quadratic entropy; FRed—functional redundancy.
Variation Partitioning revealed that the hydro-morphological features of the environment explained 33.1% of the functional diversity metrics in total; 19.8% was the part of the variability jointly explained with anthropogenic alterations, while for 13.3% hydro-morphology was solely responsible. Anthropogenic alterations of the river habitat explained 23.3% of the variability in the functional diversity metrics in total; 19.8% of the variability was explained in combination with hydro-morphology, and for 3.5%, anthropogenic alterations were solely responsible (Figure 1B). Permutation tests of all fragments indicate their high significance.
Fourth-corner tests revealed a total of 83 statistically significant associations (out of 704 possible) between macrophyte trait states and environmental variables. Thirty-one of them were positive and 52 were negative correlations (Figure 2A). Output of the fourth-corner analysis was corrected for multiple testing using the FDR (false discovery rate) procedure, leaving six out of 83 associations (Figure 2B). Trait state plinsct (pollination by insects) was positively associated with the environmental variable trnspr (water transparency), and negatively associated with river_km (distance from the river’s mouth) and dam_dst (distance from the dam). Trait state G (generalists) was negatively associated with dam_dst (distance from the dam). BF3 (high body flexibility) was negatively associated with cnnctv (level of connectivity to the river’s main channel), as well as trait state rhzm (dominant mode of reproduction is by rhizomes).
FIGURE 2. (A) Results of the fourth-corner tests, significant (at p < 0.05) positive associations are represented by blue cells and negative ones by red cells. (B) Biplot of the macrophyte trait states and environmental variables, inherited from the RLQ analysis (Supplementary Figure S1), with significant (at p < 0.05) negative and positive associations coded red and blue, respectively, inherited from the fourth-corner analysis and corrected for multiple testing using the FDR procedure. Associations are the result of fourth-corner analysis, therefore the lengths of lines that represent them does not indicate the strength of the relationship.
Our study assessed the effects of hydro-morphology and hydro-morphological alterations on functional diversity and trait structure of macrophyte communities in the Danube River in its middle course. The results showed that the examined predictors were responsible for substantial portion of the explained variance of functional diversity metrics, indicating the importance of hydro-morphological disturbances, both natural and anthropogenic, in shaping the macrophyte communities in running waters. This finding corresponds with the results of previous studies done in different types of running water-bodies (Lukács et al., 2019; Manolaki et al., 2020; Paz et al., 2021). Also, our results indicated that there could be other important environmental factors, since the effects of e.g., nutrient levels and other physicochemical factors, were out of scope of this study.
We have found that distance from the mouth, distance from the dam and riparian zone width, along the first RDA axes, and water transparency and riverbed granulometry, along the second RDA axes, were most important factors constraining functional diversity metrics. The values of all functional diversity indices increased in the downstream direction, towards the dam, in the river reach with narrow/none riparian zone, and with fine material on the riverbed. Similar result was found for the species diversity in the same reach of the Danube, where the abundance and species diversity of macrophyte communities showed the same pattern (Vukov et al., 2018). The Variation Partitioning analysis showed that the combined effect of morpho-hydrological factors (according to RDA, river’s directional flow as the most important) and anthropogenic alterations (according to RDA, damming as the most important) explained largest part of the variability in functional diversity metrics, larger than any of them explained separately. Individual relation of each of the functional diversity metrics revealed their similar response to the variables representing the distance from the mouth and the distance from the dam. In both cases the highest correlation was found between those factors and Functional Divergence (FDiv), Functional Redundancy (FRed), and Functional Evenness (FEve). Higher values of FDiv, found in the downstream direction, toward the dam indicate higher degree of niche differentiation, and consequent low resource competition. It is suggested that communities with high functional divergence may have increased ecosystem function as a result of more efficient resource use (Mason et al., 2005). Higher FRed indicates the similarity in trait states, meaning that some species within the community perform similar functions, improving its resilience to the loss of species and ecosystem functions (Ricotta et al., 2016). High FEve indicates the high degree of the trait abundance distribution of the community in the niche space, allowing effective utilization of the entire range of resources available to it, hence providing increased productivity and stability of the community (Mason et al., 2005). Width of the riparian zone was also found to be the important environmental factor constraining functional diversity metrics, with the strongest correlation to Functional Redundancy, as well as with two metrics of functional dissimilarity—Rao quadratic entropy (RaoQ) and functional dispersion (FDis). Manolaki et al. (2020) found that low values of functional dissimilarity related to the riparian zone width are consequence of habitat homogeneity. Therefore, its high values found here, might be the result of habitat heterogeneity, and consequent heterogeneity of niches (wide riparian zone—shaded, narrow/none riparian zone—open) found in the impoundments (Vukov et al., 2018).
Distance from the mouth is both spatial and typological factor, indicating the catchment size and the position of the studied section in the context of the whole river, it comprises the river flow parameters—discharge and flow velocity, which provide longitudinal connectivity for the river reaches (Vannote et al., 1980; Franklin et al., 2008). In contrast, dams are disruption of longitudinal connectivity of the river, and cause a series of environmental changes, including decreased water flow, increased sedimentation and increased water transparency, accumulation of nutrients and eutrophication, contributing to macrophyte biomass production (O’Hare et al., 2010; Birk et al., 2012). Increased values of all functional diversity metrics in the downstream direction and towards dam indicate shift from predominant effect of abiotic environment to the prevalence of biotic interactions as filters shaping structure of macrophyte communities (MacArthur and Levins, 1967; Stubbs and Wilson, 2004; Mason et al., 2007; Kraft et al., 2008; Lukács et al., 2019). In the impoundments, high values of functional diversity metrics confirm the findings of previous studies, that biotic filters—competition and limiting similarity (i.e., niche differentiation) play role in aquatic ecosystems under high productivity (Engelhardt and Ritchie, 2001; Fu et al., 2014). According to the stress-dominance hypothesis, environmental filtering is important under stressful conditions, while competitive interactions are more important in the benign environments (Weiher and Keddy, 1995; Swenson and Enquist, 2007). Therefore, the conditions in the impoundments found in the surveyed section of the Danube River, although the result of major anthropogenic modification of the river habitat, enabled the development of rich and functionally diverse aquatic vegetation.
Although fourth-corner tests revealed quite large number of significant associations between cumulated weighted means of macrophyte trait states (CWMs) and environmental variables, after performing FDR correction, only five of them remained. Pollination by insects was trait positively associated with higher water transparency, and negatively associated with the distance from the mouth and distance from the dam. This association indicates the assemblages of entomophilous, also mainly amphibious species that are established in the impoundments, were the water transparency is higher. In this case the association with the higher water transparency is indirect, while the conditions in the impoundments (narrow/none riparian zone, shallows along the banks), enable their growth as well as pollination by various insects preferring open, sunny habitats. Generalist strategy was negatively associated with the distance from the dam. Generalists are group of stress-tolerant widely distributed plants that are able to thrive in a wide variety of environmental conditions and to use different resources, and therefore dominate the communities (Borhidi, 1993). Having the wide variety of traits, they contribute to the functional diversity, and consequent resilience of the communities (Zografou et al., 2020). High body flexibility and dominant mode of reproduction by rhizomes were negatively associated with the level of connectivity to the river’s main channel, placing them in the side channels and side arms. Rhizomes provide clonal growth, which can theoretically lead to unlimited horizonal spreading of the population, competition avoidance, and exploitation and saving of resources (Klimešová, 2018; Manolaki et al., 2020). They also provide the lateral mobility and the capacity to survive unfavorable conditions (Baattrup-Pedersen et al., 2016). Since the surveyed side water-bodies dry up during the low water level, plants with rhizomes are fit to survive, and due to their capacity to reproduce by rhizomes, particularly when the water level is too high during the flowering periods, enable their populations to thrive. High body flexibility is essentially related to stronger water currents (Baattrup-Pedersen et al., 2016) which are not found in the side arms and side channels. Species assemblages with this trait are abundant in the side water-bodies with frequent and intense changes in the water level due to the hydropeaking in the hydropower plant, and are resilient to the vertical movement of water.
Our study confirmed that macrophyte functional diversity metrics respond well to the natural and anthropogenic hydro-morphological disturbances, and are valuable indicators of prevailing ecological processes shaping macrophyte communities in the large rivers. These metrics might prove to be useful diagnostic tools that are needed to identify cause(s) of ecological degradation and to guide the choice of relevant management measures. The potential of CWMs as indicators of environmental stressors is relatively low. According to Lukács et al. (2019) this shortcoming might be improved by applying more precise continuous traits and more specific and relevant gradients in the future.
The data analyzed in this study is subject to the following licenses/restrictions: The data set generated/analyzed in this study is available from the corresponding author on reasonable request. Requests to access these datasets should be directed to DV, ZHJhZ2FuYS52dWtvdkBkYmUudW5zLmFjLnJz.
DV and MI designed and performed analyses; DV, MI, MĆ, and RI collected and prepared the data; DV performed the analyses; all authors contributed to preparing the manuscript.
This study was funded by the Ministry for education, science and technological development of the Republic of Serbia, through Scientific Research Program in 2021, No. 451-03-68/2021-14/200125.
The authors declare that the research was conducted in the absence of any commercial or financial relationships that could be construed as a potential conflict of interest.
All claims expressed in this article are solely those of the authors and do not necessarily represent those of their affiliated organizations, or those of the publisher, the editors and the reviewers. Any product that may be evaluated in this article, or claim that may be made by its manufacturer, is not guaranteed or endorsed by the publisher.
We thank the reviewers for their time, effort and insightful comments.
The Supplementary Material for this article can be found online at: https://www.frontiersin.org/articles/10.3389/fenvs.2022.863508/full#supplementary-material
Baattrup-Pedersen, A., Garssen, A., Göthe, E., Hoffmann, C. C., Oddershede, A., Riis, T., et al. (2018). Structural and Functional Responses of Plant Communities to Climate Change-Mediated Alterations in the Hydrology of Riparian Areas in Temperate Europe. Ecol. Evol. 8, 4120–4135. doi:10.1002/ece3.3973
Baattrup-Pedersen, A., Göthe, E., Larsen, S. E., O'Hare, M., Birk, S., Riis, T., et al. (2015). Plant Trait Characteristics Vary with Size and Eutrophication in European lowland Streams. J. Appl. Ecol. 52 (6), 1617–1628. doi:10.1111/1365-2664.12509
Baattrup-Pedersen, A., Göthe, E., Riis, T., and O'Hare, M. T. (2016). Functional Trait Composition of Aquatic Plants Can Serve to Disentangle Multiple Interacting Stressors in lowland Streams. Sci. Total Environ. 543, 230–238. doi:10.1016/j.scitotenv.2015.11.027
Baattrup-Pedersen, A., Larsen, S. E., and Riis, T. (2003). Long-term Effects of Stream Management on Plant Communities in Two Danish lowland Streams. Hydrobiologia 48, 33–45. doi:10.1023/A:1021296519187
Baattrup-Pedersen, A., and Riis, T. (1999). Macrophyte Diversity and Composition in Relation to Substratum Characteristics in Regulated and Unregulated Danish Streams. Freshw. Biol. 42, 375–385. doi:10.1046/j.1365-2427.1999.444487.x
Bejarano, M. D., Jansson, R., and Nilsson, C. (2018). The Effects of Hydropeaking on Riverine Plants: a Review. Biol. Rev. 93, 658–673. doi:10.1111/brv.12362
Benjamini, Y., and Hochberg, Y. (1995). Controlling the False Discovery Rate: a Practical and Powerful Approach to Multiple Testing. J. R. Stat. Soc. Ser. B (Methodological) 57, 289–300. doi:10.1111/j.2517-6161.1995.tb02031.x
Birk, S., van Kouwen, L., and Willby, N. (2012). Harmonising the Bioassessment of Large Rivers in the Absence of Near-Natural Reference Conditions - a Case Study of the Danube River. Freshw. Biol. 57, 1716–1732. doi:10.1111/j.1365-2427.2012.02831.x
Borhidi, A. (1993). Social Behaviour Types of the Hungarian flora, its Naturalness and Relative Ecological Indicator Values. Pécs: Janus Pannonius Tudományegyetem Kiadványa.
Botta-Dukát, Z. (2005). Rao’s Quadratic Entropy as a Measure of Functional Diversity Based on Multiple Traits. J. Veg. Sci. 16, 533–540. doi:10.1658/1100-9233(2005)16[533:RQEAAM]2.0.CO;2
Butcher, R. W. (1933). Studies on the Ecology of Rivers: I. On the Distribution of Macrophytic Vegetation in the Rivers of Britain. J. Ecol. 21, 58–91. doi:10.2307/2255874
Cadotte, M. W., and Tucker, C. M. (2017). Should Environmental Filtering Be Abandoned? Trends Ecol. Evol. 32 (6), 429–437. doi:10.1016/j.tree.2017.03.004
Canfield Jr., D. E., and Hoyer, M. V. (1988). Influence of Nutrient Enrichment and Light Availability on the Abundance of Aquatic Macrophytes in Florida Streams. Can. J. Fish. Aquat. Sci. 45, 1467–1472. doi:10.1139/f88-171
Comtois, D. (2021). Summarytools: Tools to Quickly and Neatly Summarize Data. R Package Version 1.0.0.
Daniel, H., Bernez, I., and Haury, J. (2006). Relationships between Macrophytic Vegetation and Physical Features of River Habitats: the Need for a Morphological Approach. Hydrobiologia 570, 11–17. doi:10.1007/s10750-006-0156-6
de Bello, F., Lavergne, S., Meynard, C. N., Lepš, J., and Thuiller, W. (2010). The Partitioning of Diversity: Showing Theseus a Way Out of the Labyrinth. J. Veg. Sci. 21, 992–1000. doi:10.1111/j.1654-1103.2010.01195.x
Dolédec, S., Chessel, D., ter Braak, C. J. F., and Champely, S. (1996). Matching Species Traits to Environmental Variables: a New Three-Table Ordination Method. Environ. Ecol. Stat. 3, 143–166.
Dray, S., Choler, P., Dolédec, S., Peres-Neto, P. R., Thuiller, W., Pavoine, S., et al. (2014). Combining the Fourth‐corner and the RLQ Methods for Assessing Trait Responses to Environmental Variation. Ecology 95, 14–21. doi:10.1890/13-0196.1
Dray, S., Dufour, A.-B., and Thioulouse, J. (2021). ade4: Analysis of Ecological Data: Exploratory and Euclidean Methods in Environmental Sciences. R Package Version 1.7-18.
Dray, S., and Legendre, P. (2008). Testing the Species Traits-Environment Relationships: the Fourth-Corner Problem Revisited. Ecology 89, 3400–3412. doi:10.1890/08-0349.1
Engelhardt, K. A. M., and Ritchie, M. E. (2001). Effects of Macrophyte Species Richness on Wetland Ecosystem Functioning and Services. Nature 411, 687–689. doi:10.1038/35079573
European Standard EN 14184:2014 (2014). Water Quality − Guidance Standard for the Surveying of Macrophytes in Running Waters. Bruxelles: Comité Européen de Normalisation.
Franklin, P., Dunbar, M., and Whitehead, P. (2008). Flow Controls on lowland River Macrophytes a Review. Sci. Total Environ. 400, 369–378. doi:10.1016/j.scitotenv.2008.06.018
Fu, H., Zhong, J., Yuan, G., Ni, L., Xie, P., and Cao, T. (2014). Functional Traits Composition Predict Macrophytes Community Productivity along a Water Depth Gradient in a Freshwater lake. Ecol. Evol. 4, 1516–1523. doi:10.1002/ece3.1022
Göthe, E., Baattrup‐Pedersen, A., Wiberg‐Larsen, P., Graeber, D., Kristensen, E. A., and Friberg, N. (2017). Environmental and Spatial Controls of Taxonomic versus Trait Composition of Stream Biota. Freshw. Biol. 62, 397–413. doi:10.1111/fwb.12875
Grime, J. P. (1988). “The C-S-R model of primary plant strategies—origins, implications and tests,” in Plant Evolutionary Biology. Editors L. D. Gottlieb, and S. K. Jain (Dordrecht: Springer). doi:10.1007/978-94-009-1207-6_14
Janauer, G. A., Schmidt-Mumm, U., and Schmidt, B. (2010). Aquatic Macrophytes and Water Current Velocity in the Danube River. Ecol. Eng. 36, 1138–1145. doi:10.1016/j.ecoleng.2010.05.002
Janauer, G., Exler, N., Anačkov, G., Barta, V., Berczik, Á., Boža, P., et al. (2021). Distribution of the Macrophyte Communities in the Danube Reflects River Serial Discontinuity. Water 13, 918. doi:10.3390/w13070918
Klimešová, J. (2018). Horizontal Growth: an Overlooked Dimension in Plant Trait Space. Perspect. Plant Ecol. Evol. Syst. 32, 18–21. doi:10.1016/j.ppees.2018.02.002
Kraft, N. J. B., Valencia, R., and Ackerly, D. D. (2008). Functional Traits and Niche-Based Tree Community Assembly in an Amazonian Forest. Science 322, 580–582. doi:10.1126/science.1160662
Laliberté, E., and Legendre, P. (2010). A Distance-Based Framework for Measuring Functional Diversity from Multiple Traits. Ecology 91, 299–305. doi:10.1890/08-2244.1
Laliberté, E., Legendre, P., and Shipley, B. (2014). FD: Measuring Functional Diversity from Multiple Traits, and Other Tools for Functional Ecology. R package version 1.0-12.
Lavorel, S., Grigulis, K., McIntyre, S., Williams, N. S. G., Garden, D., Dorrough, J., et al. (2008). Assessing Functional Diversity in the Field: Methodology Matters. Funct. Ecol. 22, 134–147. doi:10.1111/j.1365-2435.2007.01339.x
Legendre, P., Galzin, R., and Harmelin-Vivien, M. L. (1997). Relating Behavior to Habitat: Solutions to Thefourth-Corner Problem. Ecology 78, 547–562. doi:10.1890/0012-9658(1997)078[0547:rbthst]2.0.co;2
Lukács, B. A., E-Vojtkó, A., Erős, T., Molnár, A. V., Szabó, S., and Götzenberger, L. (2019). Carbon Forms, Nutrients and Water Velocity Filter Hydrophyte and riverbank Species Differently: a Trait-Based Study. J. Veg. Sci. 30 (3), 471–484. doi:10.1111/jvs.12738
MacArthur, R., and Levins, R. (1967). The Limiting Similarity, Convergence, and Divergence of Coexisting Species. The Am. Naturalist 101 (921), 377–385. doi:10.1086/282505
Manolaki, P., Guo, K., Vieira, C., Papastergiadou, E., and Riis, T. (2020). Hydromorphology as a Controlling Factor of Macrophytes Assemblage Structure and Functional Traits in the Semi-arid European Mediterranean Streams. Sci. Total Environ. 703, 134658. doi:10.1016/j.scitotenv.2019.134658
Mason, N. W., Lanoiselée, C., Mouillot, D., Irz, P., and Argillier, C. (2007). Functional Characters Combined with Null Models Reveal Inconsistency in Mechanisms of Species Turnover in Lacustrine Fish Communities. Oecologia 153, 441–452. doi:10.1007/s00442-007-0727-x
Mason, N. W. H., Mouillot, D., Lee, W. G., and Wilson, J. B. (2005). Functional Richness, Functional Evenness and Functional Divergence: the Primary Components of Functional Diversity. Oikos 111, 112–118. doi:10.1111/j.0030-1299.2005.13886.x
McGill, B., Enquist, B., Weiher, E., and Westoby, M. (2006). Rebuilding Community Ecology from Functional Traits. Trends Ecol. Evol. 21, 178–185. doi:10.1016/j.tree.2006.02.002
Nõges, P., Argillier, C., Borja, Á., Garmendia, J. M., Hanganu, J., Kodeš, V., et al. (2016). Quantified Biotic and Abiotic Responses to Multiple Stress in Freshwater, marine and Ground Waters. Sci. Total Environ. 540, 43–52. doi:10.1016/j.scitotenv.2015.06.045
O′Hare, M. T., Clarke, R. T., Bowes, M. J., Cailes, C., Henville, P., Bissett, N., et al. (2010). Eutrophication Impacts on a River Macrophyte. Aquat. Bot. 92 (3), 173–178. doi:10.1016/j.aquabot.2009.11.001
Oksanen, J., Blanchet, G., Friendly, M., Kindt, R., Legendre, P., McGlinn, D., et al. (2020). Vegan: Community Ecology Package. R package version 2.5-7.
Olson, K. R., and Krug, E. (2020). The Danube, an Empire Boundary River: Settlements, Invasions, Navigation, and Trade Pathway. Jwarp 12, 884–897. doi:10.4236/jwarp.2020.1210051
Papastergiadou, E., Stefanidis, K., Dorflinger, G., Giannouris, E., Kostara, K., and Manolaki, P. (2016). Exploring Biodiversity in Riparian Corridors of a Mediterranean Island: Plant Communities and Environmental Parameters in Cyprus Rivers. Plant Biosyst. - Int. J. Dealing all Aspects Plant Biol. 150, 91–103. doi:10.1080/11263504.2014.941032
Paz, L. E., Altieri, P., Ferreira, A. C., Ocon, C., Rodrigues Capítulo, A., and Cortelezzi, A. (2021). Macrophyte Functional Traits in Channelized Streams. Aquat. Bot. 175, 103434. doi:10.1016/j.aquabot.2021.103434
Peres-Neto, P. R., Dray, S., and ter Braak, C. J. F. (2017). Linking Trait Variation to the Environment: Critical Issues with Community-Weighted Mean Correlation Resolved by the Fourth-Corner Approach. Ecography 40, 806–816. doi:10.1111/ecog.02302
Rambaud, M. P., Machon, N., Moret, J., Combroux, I., and Combroux, I. (2009). Does Channelization Alter Spatial and Temporal Dynamics of Macrophyte Communities and Their Physical Habitat? fal 174 (2), 159–172. doi:10.1127/1863-9135/2009/0174-0159
Ricotta, C., Bello, F., Moretti, M., Caccianiga, M., Cerabolini, B. E. L., and Pavoine, S. (2016). Measuring the Functional Redundancy of Biological Communities: a Quantitative Guide. Methods Ecol. Evol. 7, 1386–1395. doi:10.1111/2041-210x.12604
Riis, T., and Biggs, B. J. F. (2003). Hydrologic and Hydraulic Control of Macrophyte Establishment and Performance in Streams. Limnol. Oceanogr. 48, 1488–1497. doi:10.4319/lo.2003.48.4.1488
Riis, T., Sand-Jensen, K., and Larsen, S. E. (2001). Plant Distribution and Abundance in Relation to Physical Conditions and Location within Danish Stream Systems. Hydrobiologia 448, 217–228. doi:10.1023/a:1017580424029
Smith, V. H., Joye, S. B., and Howarth, R. W. (2006). Eutrophication of Freshwater and marine Ecosystems. Limnol. Oceanogr. 51 (12), 351–355. doi:10.4319/lo.2006.51.1_part_2.0351
Stefanidis, K., Oikonomou, A., and Papastergiadou, E. (2021). Responses of Different Facets of Aquatic Plant Diversity along Environmental Gradients in Mediterranean Streams: Results from Rivers of Greece. J. Environ. Manage. 296, 113307. doi:10.1016/j.jenvman.2021.113307
Steffen, K., Becker, T., Herr, W., and Leuschner, C. (2013). Diversity Loss in the Macrophyte Vegetation of Northwest German Streams and Rivers between the 1950s and 2010. Hydrobiologia 713, 1–17. doi:10.1007/s10750-013-1472-2
Stubbs, W. J., and Bastow Wilson, J. (2004). Evidence for Limiting Similarity in a Sand Dune Community. J. Ecol. 92, 557–567. doi:10.1111/j.0022-0477.2004.00898.x
Swenson, N. G., and Enquist, B. J. (2007). Ecological and Evolutionary Determinants of a Key Plant Functional Trait: wood Density and its Community-wide Variation across Latitude and Elevation. Am. J. Bot. 94, 451–459. doi:10.3732/ajb.94.3.451
Vannote, R. L., Minshall, G. W., Cummins, K. W., Sedell, J. R., and Cushing, C. E. (1980). The River Continuum Concept. Can. J. Fish. Aquat. Sci. 37, 130–137. doi:10.1139/f80-017
Villéger, S., Mason, N. W. H., and Mouillot, D. (2008). New Multidimensional Functional Diversity Indices for a Multifaceted Framework in Functional Ecology. Ecology 89, 2290–2301. doi:10.1890/07-1206.1
Villéger, S., Miranda, J. R., Hernández, D. F., and Mouillot, D. (2010). Contrasting Changes in Taxonomic vs. Functional Diversity of Tropical Fish Communities after Habitat Degradation. Ecol. Appl. 20 (6), 1512–1522. doi:10.1890/09-1310.1
Violle, C., Navas, M.-L., Vile, D., Kazakou, E., Fortunel, C., Hummel, I., et al. (2007). Let the Concept of Trait Be Functional!. Oikos 116, 882–892. doi:10.1111/j.0030-1299.2007.15559.x
Vukov, D., Ilić, M., Ćuk, M., Radulović, S., Igić, R., and Janauer, G. A. (2018). Combined Effects of Physical Environmental Conditions and Anthropogenic Alterations Are Associated with Macrophyte Habitat Fragmentation in Rivers - Study of the Danube in Serbia. Sci. Total Environ. 634, 780–790. doi:10.1016/j.scitotenv.2018.03.367
Walz, U., and Stein, C. (2014). Indicators of Hemeroby for the Monitoring of Landscapes in Germany. J. Nat. Conserv. 22, 279–289. doi:10.1016/j.jnc.2014.01.007
Weiher, E., and Keddy, P. A. (1995). Assembly Rules, Null Models, and Trait Dispersion: New Questions from Old Patterns. Oikos 74, 159–164. doi:10.2307/3545686
Willby, N. J., Abernethy, V. J., and Demars, B. O. L. (2000). Attribute-based Classification of European Hydrophytes and its Relationship to Habitat Utilization. Freshw. Biol. 43, 43–74. doi:10.1046/j.1365-2427.2000.00523.x
Keywords: functional traits, aquatic plants, Danube River, RDA and variation partitioning analysis, RLQ and fourth-corner analysis
Citation: Vukov D, Ilić M, Ćuk M and Igić R (2022) The Effect of Hydro-Morphology and Habitat Alterations on the Functional Diversity and Composition of Macrophyte Communities in the Large River. Front. Environ. Sci. 10:863508. doi: 10.3389/fenvs.2022.863508
Received: 27 January 2022; Accepted: 21 March 2022;
Published: 06 April 2022.
Edited by:
Mateja Germ, University of Ljubljana, SloveniaReviewed by:
Eva Socrates Papastergiadou, University of Patras, GreeceCopyright © 2022 Vukov, Ilić, Ćuk and Igić. This is an open-access article distributed under the terms of the Creative Commons Attribution License (CC BY). The use, distribution or reproduction in other forums is permitted, provided the original author(s) and the copyright owner(s) are credited and that the original publication in this journal is cited, in accordance with accepted academic practice. No use, distribution or reproduction is permitted which does not comply with these terms.
*Correspondence: Dragana Vukov, ZHJhZ2FuYS52dWtvdkBkYmUudW5zLmFjLnJz
Disclaimer: All claims expressed in this article are solely those of the authors and do not necessarily represent those of their affiliated organizations, or those of the publisher, the editors and the reviewers. Any product that may be evaluated in this article or claim that may be made by its manufacturer is not guaranteed or endorsed by the publisher.
Research integrity at Frontiers
Learn more about the work of our research integrity team to safeguard the quality of each article we publish.