- 1Pesticides Chemistry and Toxicology Department, Faculty of Agriculture, Kafrelsheikh University, Kafrelsheikh, Egypt
- 2Department of Anatomy, Faculty of Medicine, University of Jeddah, Jeddah, Saudi Arabia
- 3Department of Biochemistry and Clinical Biochemistry, Faculty of Veterinary Medicine, Kafrelsheikh University, Kafrelsheikh, Egypt
- 4Department of Pathology, Faculty of Veterinary Medicine, Kafrelsheikh University, Kafrelsheikh, Egypt
- 5Department of Clinical Laboratory Sciences, College of Applied Medical Sciences, Taif University, Taif, Saudi Arabia
- 6Department of Zoonoses, Faculty of Veterinary Medicine, Sohag University, Sohag, Egypt
The toxicity of organophosphorus insecticides is considered a major global health problem, and the target of the toxic action of these compounds in humans and pests is the same. Malathion is the most commonly used organophosphate, and its danger lies in prolonged exposure to low doses. Based on a review of the literature, little is known about the toxicological and clinicopathological effects of low doses of malathion on animal enzyme activity, such as acetylcholinesterase (AChE), alkaline phosphatase (ALP), glutamic-pyruvic transaminase (GPT), glutamic-oxaloacetic transaminase (GOT), and glutathione S-transferase (GST). Furthermore, the histopathological changes in the organs being studied (liver, kidney, brain, and lung) in treated rats were described. Three groups of experimental animals were created (each with eight rats): two experimental groups and one control group. The first group of rats received a dose of 5 mg/kg malathion orally for 24 h, the second received a dose of 5 mg/kg malathion for 21 days, and the third served as a control. Surprisingly, ALP, GPT, GOT, and GST enzymatic activities increased significantly in both malathion-treated groups (24 h or 21 days), while those of AChE significantly decreased. The histopathological changes were minimal and almost negligible in rats treated with malathion for 24 h. However, multiple histopathological changes were reported in rats treated with malathion for 21 days, including focal hepatocellular necrosis, chronic pyelonephritis, cerebral malaria, interstitial pneumonia, and testicular degeneration. Interestingly, there was a direct correlation between the alterations in biochemical parameters and histopathological lesions with the prolonged time of low malathion dose administration in rats. The study highlights the importance of research involving malathion’s chronic toxicity by non-lethal low concentrations of malathion to which most people and animals are exposed, whether as residues in water, air, or food.
1 Introduction
Pesticides have been used for several decades to improve agricultural productivity, reduce economic losses caused by controlling unwanted insects, worms, and animals, and eradicate disease vectors (Bhardwaj and Saraf, 2014). However, pesticides are not entirely beneficial since they are considered a significant source of pollution and have negative health consequences, some of which are irreversible (LaVerda et al., 2015; Navarrete-Meneses et al., 2017; Zhou et al., 2022). Therefore, the long-term effects of low doses of pesticides on human health, non-target species, and the environment raise serious concerns (Massoud et al., 2010; Ahmed et al., 2020). It is, therefore, not surprising to mention that many researchers are interested in implementing advanced methods for detoxification of these insecticides from their major resources, primarily aquatic media (Derbalah et al., 2021; Massoud et al., 2021; Massoud et al., 2022).
Pesticides, even at sub-lethal doses, are among the major hazards for humans and environments (Ahmed et al., 2020). Malathion is an insecticide that is widely used worldwide because of its effectiveness in controlling insects in field crops, fruits, vegetables, and livestock (Rettich, 1980; Chamber, 1992; Barlas, 1996; Tchounwou et al., 2015). Organophosphates (malathion, diazinon, and chlorpyrifos) have been detected in samples of water collected from vegetable and paddy fields in Bangladesh (Chowdhury et al., 2012). Similarly, another previous study conducted in Punjab, Pakistan (Ahmad et al., 2008), showed several pesticide residues in different rice samples, including karate, padan, malathion, and novacran. In addition, Hamid et al. (2020) reported that the concentration of malathion was 18.26 mg/kg in rice from India, which is higher than the maximum limit, suggesting a significant health risk for rice consumers. Therefore, the United States Environmental Protection Agency (USEPA and other studies; US Department of Health and Human Services, 1999; Zheng and Hwang, 2006) classified malathion as a very hazardous compound in Group 2A. Despite its low environmental persistence, high-level exposure to malathion toward animals and other higher vertebrates is considered neurotoxic in animals (US Department of Health and Human Services, 1999; Gurushankara et al., 2007; Budischak et al., 2009; Kumar et al., 2010; International Agency for Research on Cancer, 2015). Several international organizations, notably the Food and Drug Administration and USEPA, have established maximum residual concentrations of malathion in various food crops, such as 8 mg/kg in rice (US Department of Health and Human Services, 1999). In addition, the toxicity of several organophosphorus insecticides, for example, malathion, is increased by their breakdown products, such as oxygen analogs (oxons), which can be bio-activated within an organism or when exposed to sunlight (Derbalah and Ismail, 2013). The influence of pesticides on biochemical parameters, tissues, and organs is commonly used to assess their toxicity toward humans and animals (Enan et al., 1982; Ghanem et al., 2006). Measuring the enzymatic activity in the blood is commonly carried out to measure pesticide toxicity because of its high sensitivity and it is less time consuming. Furthermore, investigating the histopathological changes is considered a reliable diagnostic measure of the toxic effects of pesticides (Cornelius et al., 1959; Adeel et al., 2020; Adeel et al., 2021). The liver is the primary target of xenobiotic toxicity and is an essential organ for metabolizing many chemicals by biotransformation. The kidneys participate in the disposal of toxic metabolites (Neal, 1972). Hepatocytes secrete transaminases (glutamic-pyruvic transaminase [GPT] and glutamic-oxaloacetic transaminase [GOT]), which play an important role in amino acid biosynthesis and are, thus, specific indicators of liver injury. GPT and GOT are normally found in hepatocytes; however, when liver tissue is damaged, they leak into the bloodstream, leading to an increase in their level and activity in the plasma (Banaee et al., 2011). The alkaline phosphatase enzyme degrades different phosphorous esters in an alkaline medium, and its increased activity has been associated with liver cell damage (Sayim, 2007). Collectively, these organs, liver and kidney, are considered the target for malathion toxins (Yang et al., 2000). These organs are frequently used as a key indicator of the toxicity of various substances (Massoud et al., 2010). Although humans and animals are mostly exposed to pesticides at low doses for long-term periods, most of the previous studies evaluated the toxicity of pesticides at high doses, and very limited information is available regarding the effects of low doses (Derbalah and Ismail, 2013; Ahmed et al., 2020). Given the aforementioned information, this study evaluated the toxic effects of malathion at a low dose (5 mg/kg) in rats. Malathion toxicity was determined by examining its effects on numerous biochemical parameters combined with an assessment of the histopathological changes in some organs (liver, kidney, brain, lung, and testes) 24 h and 21 days after treatment versus the untreated control.
2 Materials and Methods
2.1 Chemicals
Malathion (purity = 99.9%) was purchased from Kafr El-Zayat Company for chemicals and pesticides, (Kafr El-Zayat, Egypt). All chemical kits used for the determination of some biochemical parameters were bought from Biogenic Co., Ltd. (United States).
2.2 Toxicity Experiments
2.2.1 Ethical Statement
This study’s experimental protocol and animal care followed the ethical standards of the Faculty of Veterinary Medicine, Kafrelsheikh University, Egypt. The study’s ethical approval code number is KFS-2019/3.
2.2.2 Animals
In this study, adult Wister male rats (Rattus norvegicus) were procured from the Animal House of the High Institute of Public Health, Tanta, Egypt, at 8 weeks of age and weighing 100–120 g. The animals were kept in wire cages under standard conditions, with unrestricted access to food and water. The rats were kept in a temperature-controlled room with 14 h of light and 10 h of dark cycles, standard temperature of 24 ± 4 °C, and relative humidity of 60 ± 10%. The animals were acclimatized for 2 weeks under these conditions before starting the treatment (Korsrud et al., 1972).
2.2.3 Animal Treatment
This experiment was performed in the Department of Pesticide Chemistry and Toxicology, Faculty of Agriculture, Kafrelsheikh University, Egypt. The experimental animals were divided into three groups (eight animals per group). The first and second groups were used to test the pesticide’s effects after 24 h (G1: Group 1) and 21 days (G2: Group 2), respectively, while the third group (G3: Group 3) served as a control (no malathion administered). Malathion was given to the rats at a dose of 5 mg/kg (volume, 1 ml) (1/316 LD50 for malathion). The rats were checked daily for any clinical signs of toxicity, moribund status, and mortality throughout the testing period (Ahmed et al., 2020).
2.2.4 Biochemical Assays
Blood samples were collected from each treatment group (one sample per animal), centrifuged at 4,500 rpm for 20 min, and serum was extracted and stored at −20°C until the enzymatic activities were measured. Colorimetric methods were performed as described previously (Waber and Dtsch, 1966; Gornal AC and David., 1949; Belfield A, 1971; Reitman and Frankel, 1957; and; Rose and Wallbank, 1986) to determine the activity of AChE, total protein, ALP, GPT, GOT, and GST. A spectrophotometer (SPECTRO MASTER Model 415; Fisher Scientific) was used to determine the level of enzymes after treatment with malathion. The specific activity of the selected enzymes was calculated by dividing the number of units/mL by the protein concentration in mg/mL to obtain μmol/min/mg (Manole et al., 2008).
2.2.5 Histopathological Examination
Animals were sacrificed under anesthesia and all lesions were recorded after the post-mortem examination. To investigate possible histopathological changes, specimens from the liver, kidneys, brain, lungs, and testes were collected and preserved in neutral buffered 10% formalin. Later on, the samples were dehydrated in ascending concentrations of alcohol, cleared in xylene, embedded in paraffin wax, cut at a thickness of 4 μm, stained with hematoxylin and eosin, and examined under a light microscope (Bancroft and Stevens, 1996). Histopathological lesions were scored according to the set criteria: marked [massive] (41–100% of tissue involved), moderate (21–40% of tissue involved), mild [slight] (11–20% of tissue involved), and minimal (0–10% of tissue involved) by recording the nature and extent of the lesion and its frequency of occurrence in randomly selected sites in the tissue (Shackelford et al., 2002).
2.3 Statistical Analysis
One-way analysis of variance (ANOVA) was used to analyze the biochemical data using the SPSS statistical software package for Windows version 11.0. Duncan’s multiple range test (Duncan, 1955) was also used to determine significant differences between the means. The significance level was set at p ≤ 0.05 (Duncan, 1955).
3 Results
3.1 Biochemical Effects in Rats Treated With Malathion
Figure 1 shows that the ALP, GPT, GOT, and GST activities were significantly increased after 24 h of treatment with malathion, whereas those of AChE were significantly decreased compared with those of the untreated rats. After 21 days of treatment, the assessed enzymes (ALP, GPT, GOT, and GST) showed the same trend and were significantly increased, while the activity of the AChE enzyme was significantly decreased (Figure 2).
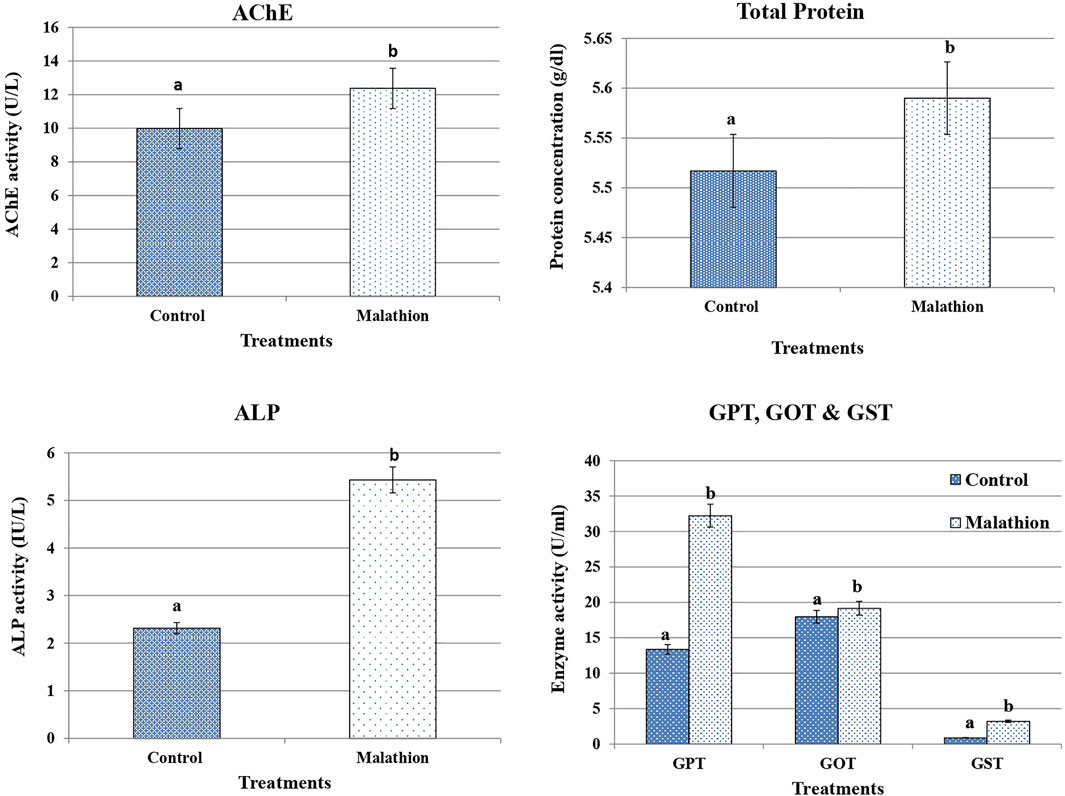
FIGURE 1. Effect of malathion on AChE, total protein, ALP GPT, GOT, and GST in male rats after 24 h of treatment compared to control. Bars represent means ± SD. *p < 0.05 versus control. Different letters (A,B) indicate the significant differences between means according to Duncan’s multiple range test.
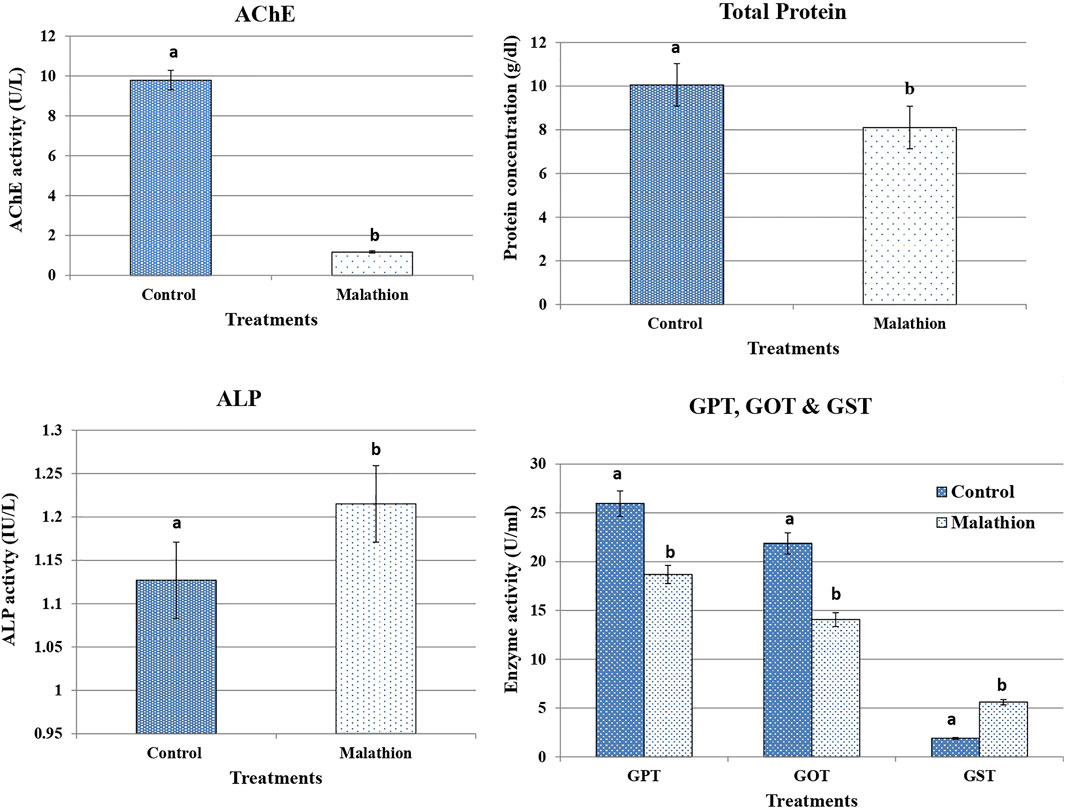
FIGURE 2. Effect of malathion on AChE, total protein, ALP GPT, GOT, and GST in male rats after 21 days of treatment compared to control. Bars represent means ± SD. *p < 0.05 versus control. Different letters (A,B) indicate the significant differences between means according to Duncan’s multiple range test.
3.2 Histopathological Changes in Rats Treated With Malathion
3.2.1 Histopathological Changes in the Liver
As shown in Figure 3, there was slight sinusoidal cell activation, mainly with macrophages and the presence of single hepatocytes cell necrosis among rats treated with malathion for 24 h. Meanwhile, the livers of rats which received the same dose of malathion for 21 days showed congestion and dilatation of the hepatic sinusoids, moderate inflammatory reaction in the form of periportal mononuclear cell infiltration, and focal hepatocellular necrosis.
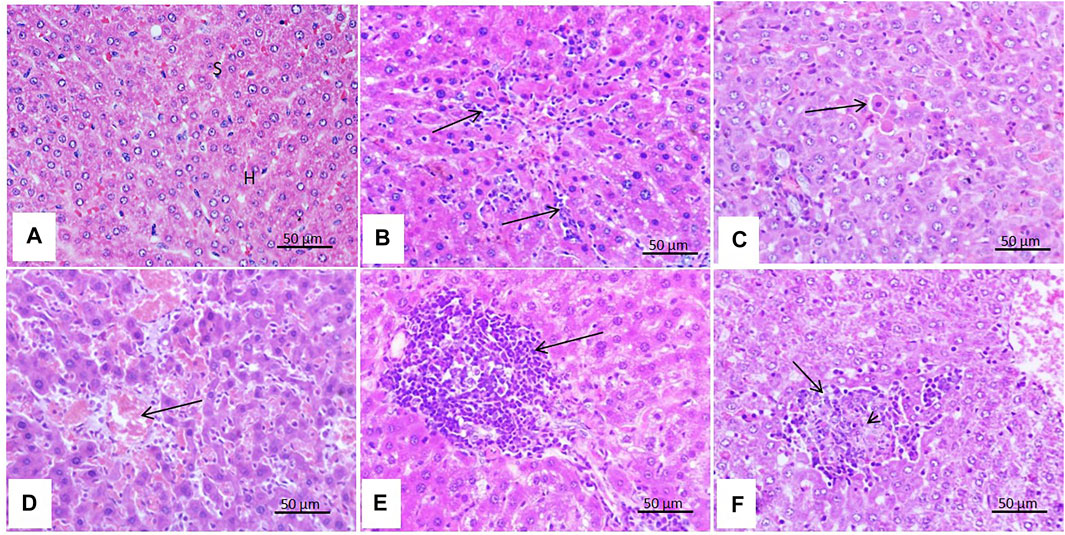
FIGURE 3. Effect of malathion on the liver. (A) Liver of the control rats showing intact hepatic parenchyma with normal arrangement of hepatocytes (H), sinusoids (S), and central vein (C). (B) Liver of rats treated with malathion for 24 h showing mild sinusoidal cell activation (arrow) and (C) single cell necrosis of hepatocytes (arrow). (D) Liver of rats treated with malathion for 21 days showing severe congestion (arrow), (E) massive periportal mononuclear cell infiltration (arrow), and (F) focal hepatocytic necrosis (arrow) with the infiltration of mononuclear cells within and at the margin of the necrosis areas (arrow head).
3.2.2. Histopathological Changes in the Kidney
The kidneys of rats treated with malathion for 24 h showed no histopathological changes and had normal renal tubules and glomeruli. However, those rats treated for 21 days had moderate vacuolar degeneration in the renal tubules lining the epithelium, presence of proteinaceous casts in the lumens of renal tubules, massive pyelonephritis with mononuclear cell infiltration in the pelvis, and squamous metaplasia of the renal pelvis lining epithelium and tubular basophilia (Figure 4).
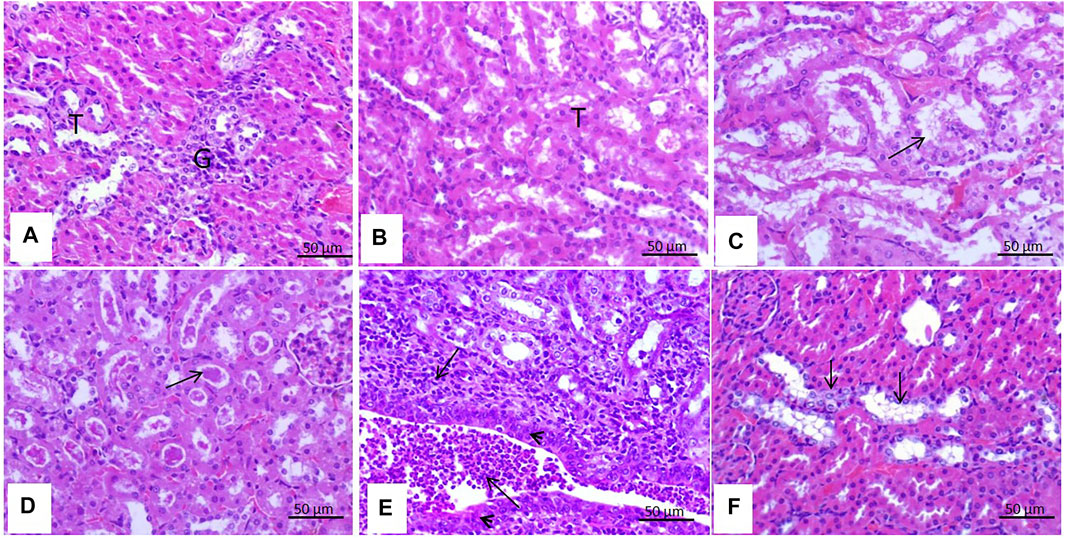
FIGURE 4. Effect of malathion on the kidneys. (A,B) Kidneys of control rats and those treated with malathion for 24 h appeared with normal renal tubules (T) and glomeruli (G). (C) Rats treated with malathion for 21 days showing a moderate degree of vacuolar degeneration (arrow) in the lining epithelium of the renal tubules, (D) presence of proteinaceous casts (arrow) in the lumens of renal tubules, (E) massive pyelonephritis with mononuclear cell infiltration (arrow) in the pelvis and squamous metaplasia (arrow head) of the renal pelvis lining epithelium, and (F) tubular basophilia (arrow) of the tubular lining epithelium.
3.2.3 Histopathological Changes in the Brain
There were few changes in the brain of rats treated with malathion for 24 h in the form of a slight perivascular cuff. However, there was focal cerebral malacia with infiltration of many glial cells in rats treated with malathion for 21 days (Figure 5).
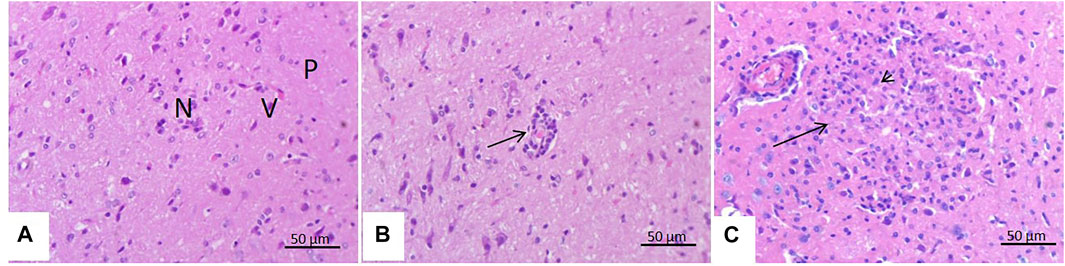
FIGURE 5. Effect of malathion on the brain. (A) Brain of control rats showing intact neuronal cells (N) and blood vessels (V) in the neuropil (P) of the cerebrum. (B) Brain of rats treated with malathion for 24 h showing a slight perivascular cuff (arrows). (C) Brain of rats treated with malathion for 21 days showing cerebral malacia (arrow) with the infiltration of large number of glial cells (arrow head).
3.2.4. Histopathological Changes in the Lungs
There were few microscopic changes noticed in the lungs of rats treated with malathion for 24 h in the form of congestion of the inter-alveolar blood vessels with perivascular eosinophilic infiltration. The lungs of rats treated with malathion for 21 days showed moderate interstitial pneumonia with thickened inter-alveolar septa and infiltration of numerous mononuclear inflammatory cells (Figure 6).
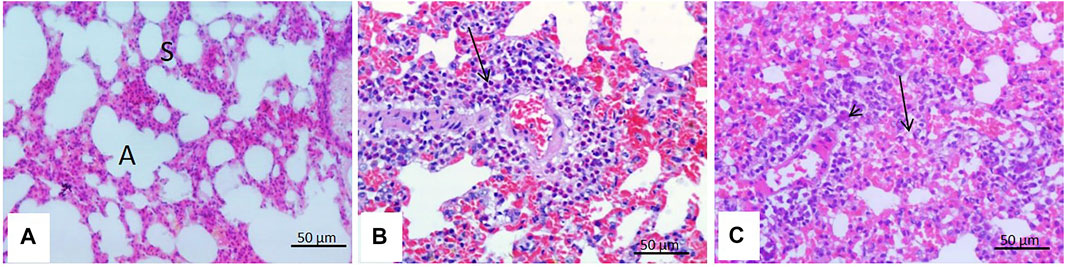
FIGURE 6. Effect of malathion on the lungs. (A) Lungs of control rats with clear and intact alveoli (A) with thin inter-alveolar septa (S). (B) Lungs of rats treated with malathion for 24 h showing congestion of the inter-alveolar blood vessels and perivascular eosinophilic infiltration (arrow). (C) Lungs of rats treated with malathion for 21 days showing thickening of the inter-alveolar septa infiltration of a large number of mononuclear inflammatory cells (arrow).
3.2.5. Histopathological Changes in the Testes
In this work, no microscopic changes in the testes of rats treated with malathion for 24 h were noticed and the testes appeared similar to those of the control rats. However, rats treated with malathion for 21 days showed testicular degeneration with complete necrosis of the germinal lining epithelium of some seminiferous tubules and massive interstitial edema (Figure 7).
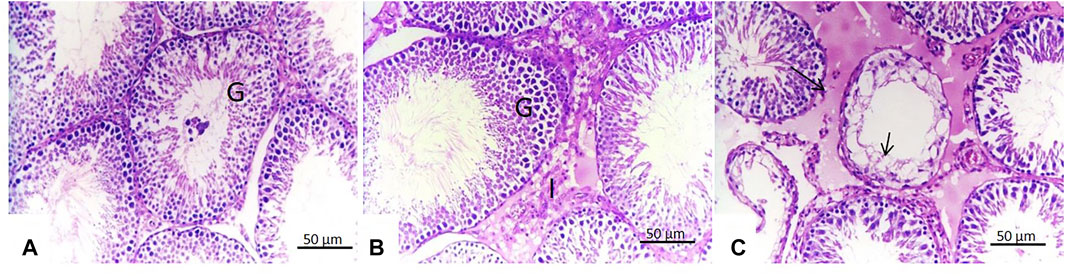
FIGURE 7. Effect of malathion on the testis. (A,B) Testis of control rats and those treated with malathion for 24 h showing normal testicular structures with germinal epithelium (G) in the seminiferous tubules and interstitial (Leydig) cells (L). (C) Testis of rats treated with malathion for 21 days showed testicular degeneration with complete necrosis of the germinal lining epithelium (arrow) of some seminiferous tubules and massive interstitial edema (short arrow).
3.2.6 Quantification of Histopathological Changes in Rats Treated With Malathion
The quantification and scores of histopathological lesions recorded for the liver, kidneys, brain, lungs and testis in rats treated with malathion are shown in Table 1.
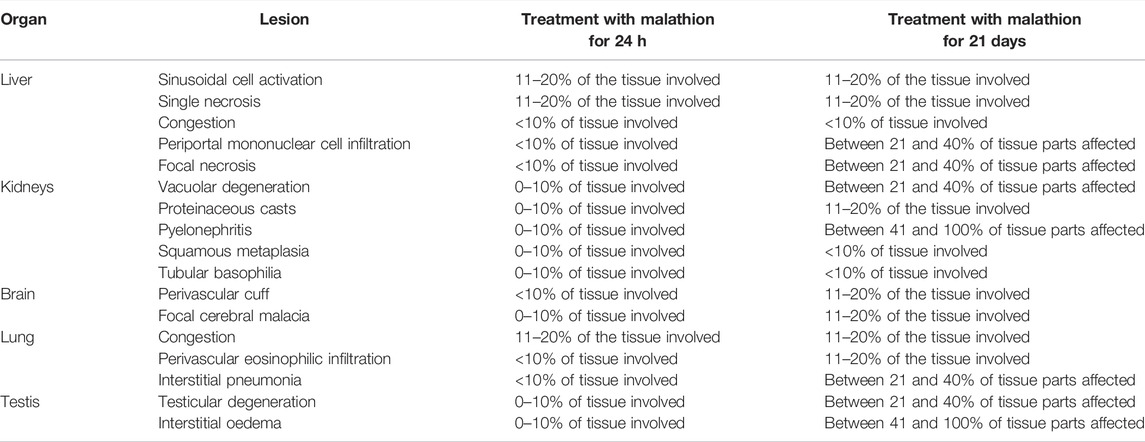
TABLE 1. Lesions and scoring criteria for reported histopathological lesions of the liver, kidneys, brain, lung, and testis scored in the rats treated with malathion.
4 Discussion
This study reveals interesting biochemical and histopathological findings evaluating the toxic effects of malathion at a low dose (5 mg/kg) in rats after 24 h and 21 days of treatment. The study measured several biochemical enzymes followed by assessing the histopathological changes in the liver, kidney, brain, lung, and testes. In the present work, we observed a significant increase in the activity of liver enzymes (GOT and GPT) after treatment with malathion, which is in agreement with previous reports (Bogusz, 1968; Menratha et al., 1973; and Abdel-Rahman et al., 1985). Notably, the elevated levels of the GPT and GOT enzymes indicate the damage of hepatic tissue (Massoud et al., 2010; Banaee et al., 2011).
It should be stressed that the liver is considered one of the most important organs affected by malathion toxicity, resulting in hepatocellular damage and increased liver enzymatic activity (Badr, 2020). In the present work, high levels of ALT and AST were reported in the treated groups compared with the control animals, which is in harmony with the histological changes found in liver tissue and is in agreement with previous studies (Agrahari et al., 2007). The reported alteration in the activity of hepatic enzymes in malathion-treated mice could be attributed to early hepatocyte damage induced by malathion (Banaee et al., 2011) (Ncibi et al., 2008). It is noteworthy to mention that ALT, AST, and ALP are considered markers of liver damage (Gokcimen et al., 2007). In addition, it has been shown that organophosphorus insecticides can elevate the enzymatic activities of ALT, AST, and ALP (Khan et al., 2005; Rezg et al., 2007; Ncibi et al., 2008; Ogutcu et al., 2008; Celik et al., 2009). The ALP enzyme is involved in various biological processes, including the removal of toxic compounds, metabolism, and biosynthesis, all required for cell function. As a result, any disruption in this enzyme contributes to tissue and cell function disruption (Rezg et al., 2007). In the present study, there was an increase in the activity of this enzyme in rats treated with malathion compared with the control group which might be attributed to the damage that occurred in liver tissues with the disturbance of the normal liver function. This interpretation is consistent with several previous studies showing that the damage of the cell membrane of the liver is followed by the release of many enzymes into the blood, including ALT, AST, and ALP (Abdel-Rahman et al., 1985; Rezg et al., 2007; Ncibi et al., 2008).
Taken into account, the GST enzyme targets the detoxification of organophosphate insecticides. This enzyme acts by either rapidly metabolizing the insecticide into non-toxic products or rapid binding to the insecticide (Hemingway et al., 1998; Kostaropoulos et al., 2001). In this work, rats treated with malathion after 24 h had reduced hepatic GSH activity, which is in agreement with previous reports (Lasram et al., 2014; Selmi et al., 2015). In stark contrast, rats treated with malathion for 21 days exhibited an increase in GST activity, which was also observed in a previous study (Hazarika et al., 2003).
Furthermore, the amount of GSH present in the cell as a co-substrate of GST appears adequate to cope with the enzyme’s increased activity (data not shown). Importantly, the inhibition of AChE remains among the main mechanisms of malathion toxicity (Esen and Uysal, 2018); AChE activity could be used as a surrogate marker of oxidative stress in health monitoring and, therefore, is considered an indicator of brain function. In this work, the activity of the cholinesterase enzyme decreased in rats treated with malathion compared with the control group. The present findings agree with many researchers who revealed that poisoning with organophosphorus pesticides, such as malathion, inhibits the enzyme cholinesterase in the blood (Hazarika et al., 2003; Timur et al., 2003). In a previous study, workers exposed to organophosphorus pesticides had significantly lower AChE activity (Hernández et al., 2013). The significant decrease in acetylcholinesterase activity after malathion administration can be explained because organophosphate insecticides inhibit AChE irreversibly by the phosphorylation of the enzyme’s serine-OH group (Dumschat et al., 1991; Mortensen et al., 1998).
Regarding the histopathological changes in this work, a slight inflammatory reaction was observed in the liver tissue of rats treated with malathion as a single dose. In contrast, an advanced inflammatory reaction was represented by focal hepatic necrosis with periportal infiltration of mononuclear cells in the rats treated with malathion for 21 days. These later lesions might arise from the toxic effects of malathion and their influence on the liver’s detoxification mechanisms and inflammatory response (Gokcimen et al., 2007; Yehia et al., 2007). As mentioned previously, the liver is a well-known detoxifying organ which mainly performs through p450-mediated enzymatic catalysis (Ahmed et al., 2020). In this study, it seems that prolonged treatment with malathion inhibited p450-mediated biostimulants or negatively affected the mitochondrial membrane transport system of hepatocytes of treated rats, resulting in hepatocyte damage and death, and these findings are consistent with several previous studies (Guengerich and Avadhani, 2018; He et al., 2020). The observed mild degenerative changes in the liver of rats after 24 h of treatment are consistent with previous studies (Toś-Luty et al., 2003). It was discovered that exposure of male Wister rats to a single dose of malathion caused degenerative changes in the liver and induced parenchymatous degeneration. Similarly, after 21 days of treatment, the observed hepatic changes were correlated with increased levels of liver enzymes that rendered to subchronic liver damage. These later findings are consistent with those reported previously (Sayim, 2007; Kim et al., 2008; Singh et al., 2013).
Organophosphorus pesticides induce various histopathological changes in the liver and kidneys (Zidan, 2015). These induced histopathological changes include bleeding, infiltration of inflammatory cells, and necrosis (Kalender et al., 2006) and were consistent with several previous reports (Kerem et al., 2007; Afshar et al., 2008). In this work, the kidneys of rats which received malathion for 24 h did not show any microscopic abnormalities. However, marked renal changes were observed in rats sacrificed after 21 days of treatment. It seems that the long time exposure of kidneys to malathion resulted in subchronic toxicity and a series of degenerative changes in the glomerular tufts and peritubular renal capillaries, chronic pyelonephritis with infiltration of numerous mononuclear cells, and squamous metaplasia of the lining epithelium of the renal pelvis (Yun et al., 2015; Iavicoli et al., 2016). The proximal tubules are the main site for xenobiotic biotransformation, making them more susceptible to chemical insult, and therefore, all reported lesions were confined mainly to the renal cortex (Lock and Reed, 1998).
The brain is a highly sensitive organ to toxicity and highly susceptible to oxidative processes (Savolainen, 1978). As observed in this work, the brain showed slight histopathological changes in rats treated with malathion for 24 h, but these histopathological changes became more severe after treatment for 21 days, indicating the stress condition of these rats, which was reported in previous studies (Cheng et al., 2020). As mentioned previously, some pesticides, such as organophosphorus compounds, are neurotoxic (Ahmed et al., 2011). Malathion is considered one of the compounds that interfere with the chemical neurotransmission or ion channels and cause reversible neurotoxic effects such as neuritis and gliosis, consistent with previous research (Eddleston et al., 2002; Gunnell et al., 2007).
Regarding the lungs, as shown, malathion was not toxic to the lungs after 24 h. However, after a long period (21 days) of exposure to malathion, the lungs showed more severe pulmonary changes. The resulting pulmonary lesions might be attributed to the breakdown of the alveolar epithelial/endothelial barrier and the release of exudative inflammatory infiltrate into the lungs (Hussain and Sultan, 2005). However, a previous study (Adamis et al., 1999) mentioned that the pathophysiological processes leading to these inflammatory reactions are unclear, but pulmonary toxicity can induce acute inflammatory reactions with different features.
Examination of the male reproductive organs after exposure to malathion is of great importance (Bustos-Obregon et al., 2001). In this study, rats treated with malathion for 24 h showed the same histological structure as the control group. This result is in agreement with that reported previously (Recena et al., 2006). Meanwhile, malathion-treated rats showed evident testicular changes after 21 days. Previous reports have shown that malathion affects the testes’ germinal and somatic cells, decreases testosterone activity, and consequently inhibits spermatogenesis through damage and vacuolation of Sertoli’s cells and germ cells (Steger et al., 1999; Farag et al., 2000; Mahgoub and El-Medany, 2001). In this work, necrosis and edema in the seminiferous tubules and interstitial tissue were similar to those noticed in several previous studies (Farag et al., 2000; Khan et al., 2001; Uzunhisarcikli et al., 2007). Taken together, the study concluded that low or sub-lethal doses of malathion have toxic effects on the treated rats, particularly after 21 days of treatment reflected in a series of biochemical and histopathological changes. This shows that exposure to malathion residues in food or water can have serious long-term toxic effects on human and animal health, particularly at low doses.
Conclusion
According to the present findings, malathion pesticide induced several biochemical alterations combined with a series of histopathological changes and damage in the liver, kidneys, brain, lung, and testes after a short time of exposure to a dose of 5 mg/kg. Considering this, the toxic effects of malathion on human health cannot be overlooked, especially when long-term exposure and low doses of the pesticide are considered. Our study suggests future research to investigate the toxicological effects of malathion at low concentrations close to the environmental level and over longer periods of time.
Data Availability Statement
The original contributions presented in the study are included in the article/Supplementary Material; further inquiries can be directed to the corresponding author.
Ethics Statement
Animal care and experimental protocols used in the present study followed relevant guidelines and regulations of the ethical standards of Veterinary Medicine, Kafrelsheikh University, Egypt. The ethical approval code number of the study is KFS-2019/3.
Author Contributions
Conceptualization: AM, MS, IE-F, MA, KA, EE, and AD; data curation: MS and MA; formal analysis: IE-F, MS, AA, and MA; funding acquisition: MS and MA; investigation: AM, AD, IE-F, MS, and EE; methodology: AM, MS, and AD; project administration: AM and IE-F; resources: MS and MA; software: EE; supervision: AM, AD, NN, ND, KA, and EE; validation: AM, AD, and EE; visualization: AM, EE, and AD; writing—original draft: NN, MS, MA, ND, KA, EE, and AD; and writing—review and editing: AM, MS, MA, EE, and AD. All authors have read and approved of the published version of the manuscript.
Funding
This work was supported in part by the Taif University Researchers Supporting Program (Project Number TURSP-2020/153), Taif University, Saudi Arabia.
Conflict of Interest
The authors declare that the research was conducted in the absence of any commercial or financial relationships that could be construed as a potential conflict of interest.
Publisher’s Note
All claims expressed in this article are solely those of the authors and do not necessarily represent those of their affiliated organizations, or those of the publisher, the editors, and the reviewers. Any product that may be evaluated in this article, or claim that may be made by its manufacturer, is not guaranteed or endorsed by the publisher.
References
Abdel-Rahman, M. S., Lechner, D. W., and Klein, K. M. (1985). Combination Effect of Carbaryl and Malathion in Rats. Arch. Environ. Contam. Toxicol. 14 (4), 459–464. doi:10.1007/bf01055532
Adamis, Z., Tátrai, E., Honma, K., and Ungváry, G. (1999). Effects of Lead(II) Nitrate and a Dithiocarbamate Fungicide on the Rat Lung. J. Appl. Toxicol. 19 (5), 347–350. doi:10.1002/(sici)1099-1263(199909/10)19:5<347::aid-jat587>3.0.co;2-3
Adeel, M., Shakoor, N., Hussain, T., Azeem, I., Zhou, P., Zhang, P., et al. (2021). Bio-interaction of Nano and Bulk Lanthanum and Ytterbium Oxides in Soil System: Biochemical, Genetic, and Histopathological Effects on Eisenia fetida. J. Hazard. Mater. 415, 125574. doi:10.1016/j.jhazmat.2021.125574
Adeel, M., Tingting, J., Hussain, T., He, X., Ahmad, M. A., Irshad, M. K., et al. (2020). Bioaccumulation of Ytterbium Oxide Nanoparticles Insinuate Oxidative Stress, Inflammatory, and Pathological Lesions in ICR Mice. Environ. Sci. Pollut. Res. 27 (26), 32944–32953. doi:10.1007/s11356-020-09565-8
Afshar, S., Farshid, A., Heidari, R., and Ilkhanipour, M. (2008). Histopathological Changes in the Liver and Kidney Tissues of Wistar Albino Rat Exposed to Fenitrothion. Toxicol. Ind. Health 24 (9), 581–586. doi:10.1177/0748233708100090
Agrahari, S., Pandey, K. C., and Gopal, K. (2007). Biochemical Alteration Induced by Monocrotophos in the Blood Plasma of Fish, Channa Punctatus (Bloch). Pesticide Biochem. Physiology 88 (3), 268–272. doi:10.1016/j.pestbp.2007.01.001
Ahmad, S., Zia-Ul-Haq, M., Imran, M., Iqbal, S., Iqbal, J., and Ahmad, M. (2008). Determination of Residual Contents of Pesticides in Rice (Oryza Sativa L) Crop from Different Regions of Pakistan. Pak. J. Bot. 40 (3), 1253–1257.
Ahmed, M. S., Massoud, A. H., Derbalah, A. S., Al-Brakati, A., Al-Abdawani, M. A., Eltahir, H. A., et al. (2020). Biochemical and Histopathological Alterations in Different Tissues of Rats Due to Repeated Oral Dose Toxicity of Cymoxanil. Anim. (Basel) 10 (12), E2205. doi:10.3390/ani10122205
Ahmed, M. S., Massoud, A. H., Derbalah, A. S., and Ismail, A. A. (2011). Pathological and Biochemical Assesment of the Fungicide (Metalaxyl) on Rats. Egypt. J. Comp. Pathol. Clin. Pathol. 24, 136–154.
Badr, A. M. (2020). Organophosphate Toxicity: Updates of Malathion Potential Toxic Effects in Mammals and Potential Treatments. Environ. Sci. Pollut. Res. 27 (21), 26036–26057. [pii]. doi:10.1007/s11356-020-08937-410.1007/s11356-020-08937-4
Banaee, M., Sureda, A., Mirvaghefi, A. R., and Ahmadi, K. (2011). Effects of Diazinon on Biochemical Parameters of Blood in Rainbow Trout (Oncorhynchus mykiss). Pesticide Biochem. physiology 99 (1), 1–6. doi:10.1016/j.pestbp.2010.09.001
Bancroft, J., and Stevens, A. (1996). Theory and Practice of Histological Techniques. 4th ed. New York/London/San Francisco/Tokyo: Churchill Livingstone.
Barlas, N. E. (1996). Toxicological Assessment of Biodegraded Malathion in Albino Mice. Bull. Environ. Contam. Toxicol. 57 (5), 705–712. doi:10.1007/s001289900247
Belfield, A., and Goldberg, D. M. (1971). Revised Assay for Serum Phenyl Phosphatase Activity Using 4- Amino - Antipyrine. Enzyme 12, 561–573. doi:10.1159/000459586
Bhardwaj, J. K., and Saraf, P. (2014). Malathion-Induced Granulosa Cell Apoptosis in Caprine Antral Follicles: An Ultrastructural and Flow Cytometric Analysis. Microsc. Microanal. 20, 1861–1868. doi:10.1017/s1431927614013452
Bogusz, M. (1968). Influence of Insecticides on the Activity of Some Enzymes Contained in Human Serum. Clin. Chim. Acta 19 (3), 367–369. doi:10.1016/0009-8981(68)90260-x
Budischak, S. A., Belden, L. K., and Hopkins, W. A. (2009). Relative Toxicity of Malathion to Trematode-Infected and Noninfected Rana palustris Tadpoles. Arch. Environ. Contam. Toxicol. 56 (1), 123–128. doi:10.1007/s00244-008-9167-9
Bustos-Obregón, E., Díaz, O., and Sobarzo, C. (2001). Parathion Induces Mouse Germ Cells Apoptosis. Ital. J. Anat. Embryol. 106 (2 Suppl. 2), 199–204.
Celik, I., Yilmaz, Z., and Turkoglu, V. (2009). Hematotoxic and Hepatotoxic Effects of Dichlorvos at Sublethal Dosages in Rats. Environ. Toxicol. 24 (2), 128–132. doi:10.1002/tox.20390
Chamber, H. (1992). Organophosphorus Compounds: an Overview. Organophosphates Chem. fate Eff. 3–17. doi:10.1016/b978-0-08-091726-9.50005-7
Cheng, B., Zhang, H., Hu, J., Peng, Y., Yang, J., Liao, X., et al. (2020). The Immunotoxicity and Neurobehavioral Toxicity of Zebrafish Induced by Famoxadone-Cymoxanil. Chemosphere 247, 125870. doi:10.1016/j.chemosphere.2020.125870
Chowdhury, M. A. Z., Banik, S., Uddin, B., Moniruzzaman, M., Karim, N., Gan, S. H., et al. (2012). Organophosphorus and Carbamate Pesticide Residues Detected in Water Samples Collected from Paddy and Vegetable Fields of the Savar and Dhamrai Upazilas in Bangladesh. Ijerph 9 (9), 3318–3329. doi:10.3390/ijerph9093318
Cornelius, C. E., Bishop, J., Switzer, J., and Rhode, E. A. (1959). Serum and Tissue Transaminase Activities in Domestic Animals. Cornell Vet. 49 (1), 116–126.
Derbalah, A., and Ismail, A. (2013). Remediation Technologies of Diazinon and Malathion Residues in Aquatic System. Environ. Prot. Eng. 39 (3). doi:10.37190/epe130310
Derbalah, A., Massoud, A., El-Mehasseb, I., Allah, M. S., Ahmed, M. S., Al-Brakati, A., et al. (2021). Microbial Detoxification of Dimethoate and Methomyl Residues in Aqueous Media. Water 13 (8), 1117. doi:10.3390/w13081117
Dumschat, C., Müller, H., Stein, K., and Schwedt, G. (1991). Pesticide-sensitive ISFET Based on Enzyme Inhibition. Anal. Chim. acta 252 (1-2), 7–9. doi:10.1016/0003-2670(91)87189-e
Duncan, D. B. (1955). Multiple Range and Multiple F Tests. Biometrics 11 (1), 1–42. doi:10.2307/3001478
Eddleston, M., Szinicz, L., Eyer, P., and Buckley, N. (2002). Oximes in Acute Organophosphorus Pesticide Poisoning: a Systematic Review of Clinical Trials. Qjm 95 (5), 275–283. doi:10.1093/qjmed/95.5.275
Enan, E. E., El‐Sebae, A. H., Enan, O. H., and El‐Fiki, S. (1982). In‐vivo Interaction of Some Organophosphorus Insecticides with Different Biochemical Targets in White Rats. J. Environ. Sci. Health, Part B 17 (5), 549–570. doi:10.1080/03601238209372341
Esen, M., and Uysal, M. (2018). Protective Effects of Intravenous Lipid Emulsion on Malathion-Induced Hepatotoxicity. Bratisl. Lek. Listy 119 (6), 373–378. doi:10.4149/BLL_2018_069
Farag, A., Eweidah, M., and El-Okazy, A. (2000). Reproductive Toxicology of Acephate in Male Mice. Reprod. Toxicol. 14 (5), 457–462. doi:10.1016/s0890-6238(00)00094-0
Ghanem, N. F., Hassan, N. A., and Ismail, A. A. (2006). Biochemical and Histopathological Changes Induced in Rats Fed on Diets and Byproducts of Fumigated Wheat Grains with Phostoxin. Pro. 4th Int. Con. Biol.Sc. (zool), 259–268.
Gokcimen, A., Gulle, K., Demirin, H., Bayram, D., Kocak, A., and Altuntas, I. (2007). Effects of Diazinon at Different Doses on Rat Liver and Pancreas Tissues. Pesticide Biochem. physiology 87 (2), 103–108. doi:10.1016/j.pestbp.2006.06.011
Gornal Ac, B. C., and David, M. M. (1949). Protein–Biuret Colorimetric Method. J. Biol. Chem. 177, 751.
Gunnell, D., Fernando, R., Hewagama, M., Priyangika, W. D. D., Konradsen, F., and Eddleston, M. (2007). The Impact of Pesticide Regulations on Suicide in Sri Lanka. Int. J. Epidemiol. 36 (6), 1235–1242. doi:10.1093/ije/dym164
Gurushankara, H. P., Krishnamurthy, S. V., and Vasudev, V. (2007). Effect of Malathion on Survival, Growth, and Food Consumption of Indian Cricket Frog (Limnonectus Limnocharis) Tadpoles. Arch. Environ. Contam. Toxicol. 52 (2), 251–256. doi:10.1007/s00244-006-0015-5
Hamid, A., Yaqub, G., Ayub, M., and Naeem, M. (2020). Determination of Malathion, Chlorpyrifos, λ-cyhalothrin and Arsenic in Rice. Food Sci. Technol. 41, 461–466. doi:10.1590/fst.01020
Hazarika, A., Sarkar, S. N., Hajare, S., Kataria, M., and Malik, J. K. (2003). Influence of Malathion Pretreatment on the Toxicity of Anilofos in Male Rats: a Biochemical Interaction Study. Toxicology 185 (1-2), 1–8. doi:10.1016/s0300-483x(02)00574-7
He, B., Ni, Y., Jin, Y., and Fu, Z. (2020). Pesticides-induced Energy Metabolic Disorders. Sci. Total Environ. 729, 139033. doi:10.1016/j.scitotenv.2020.139033
Hemingway, J., Hawkes, N., Prapanthadara, L., Jayawardenal, K. G. I., and Ranson, H. (1998). The Role of Gene Splicing, Gene Amplification and Regulation in Mosquito Insecticide Resistance. Phil. Trans. R. Soc. Lond. B 353 (1376), 1695–1699. doi:10.1098/rstb.1998.0320
Hernández, A. F., Gil, F., Lacasaña, M., Rodríguez-Barranco, M., Gómez-Martin, A., Lozano, D., et al. (2013). Modulation of the Endogenous Antioxidants Paraoxonase-1 and Urate by Pesticide Exposure and Genetic Variants of Xenobiotic-Metabolizing Enzymes. Food Chem. Toxicol. 61, 164–170. doi:10.1016/j.fct.2013.05.039
Hussain, A. M., and Sultan, S. T. (2005). Organophosphorus Insecticide Poisoning: Management in Surgical Intensive Care Unit. J. Coll. Physicians Surg. Pak 15 (2), 100–102. doi:10.2005/JCPSP.100102
Iavicoli, I., Fontana, L., and Nordberg, G. (2016). The Effects of Nanoparticles on the Renal System. Crit. Rev. Toxicol. 46 (6), 490–560. doi:10.1080/10408444.2016.1181047
International Agency for Research on Cancer, I (2015). Evaluation of Fve Organophosphate Insecticides and Herbicides (WHO), 20 March. Monographs 112. Lyon, France: IARC
Kalender, Y., Uzunhisarcikli, M., Ogutcu, A., Acikgoz, F., and Kalender, S. (2006). Effects of Diazinon on Pseudocholinesterase Activity and Haematological Indices in Rats: the Protective Role of Vitamin E. Environ. Toxicol. Pharmacol. 22 (1), 46–51. doi:10.1016/j.etap.2005.11.007
Kerem, M., Bedirli, N., Guerbuez, N., Ekinci, O., Bedirli, A., Akkaya, T., et al. (2007). Effects of Acute Fenthion Toxicity on Liver and Kidney Function and Histology in Rats. Turkish J. Med. Sci. 37 (5), 281–288.
Khan, I. A., Reddy, B. V., Mahboob, M., Rahman, M. F., and Jamil, K. (2001). Effects of Phosphorothionate on the Reproductive System of Male Rats. J. Environ. Sci. Health, Part B 36 (4), 445–456. doi:10.1081/pfc-100104188
Khan, S. M., Sobti, R. C., and Kataria, L. (2005). Pesticide-induced Alteration in Mice Hepato-Oxidative Status and Protective Effects of Black Tea Extract. Clin. Chim. Acta 358 (1-2), 131–138. doi:10.1016/j.cccn.2005.02.015
Kim, Y. S., Kim, J. S., Cho, H. S., Rha, D. S., Kim, J. M., Park, J. D., et al. (2008). Twenty-eight-day Oral Toxicity, Genotoxicity, and Gender-Related Tissue Distribution of Silver Nanoparticles in Sprague-Dawley Rats. Inhal. Toxicol. 20 (6), 575–583. doi:10.1080/08958370701874663
Korsrud, G. O., Grice, H. C., and McLaughlan, J. M. (1972). Sensitivity of Several Serum Enzymes in Detecting Carbon Tetrachloride-Induced Liver Damage in Rats. Toxicol. Appl. Pharmacol. 22 (3), 474–483. doi:10.1016/0041-008x(72)90255-4
Kostaropoulos, I., Papadopoulos, A. I., Metaxakis, A., Boukouvala, E., and Papadopoulou-Mourkidou, E. (2001). The Role of glutathioneS-Transferases in the Detoxification of Some Organophosphorus Insecticides in Larvae and Pupae of the Yellow Mealworm,Tenebrio molitor (Coleoptera: Tenebrionidae). Pest. Manag. Sci. 57 (6), 501–508. doi:10.1002/ps.323
Kumar, R., Nagpure, N. S., Kushwaha, B., Srivastava, S. K., and Lakra, W. S. (2010). Investigation of the Genotoxicity of Malathion to Freshwater Teleost Fish Channa Punctatus (Bloch) Using the Micronucleus Test and Comet Assay. Arch. Environ. Contam. Toxicol. 58 (1), 123–130. doi:10.1007/s00244-009-9354-3
Lasram, M. M., Dhouib, I. B., Bouzid, K., Lamine, A. J., Annabi, A., Belhadjhmida, N., et al. (2014). Association of Inflammatory Response and Oxidative Injury in the Pathogenesis of Liver Steatosis and Insulin Resistance Following Subchronic Exposure to Malathion in Rats. Environ. Toxicol. Pharmacol. 38 (2), 542–553. doi:10.1016/j.etap.2014.08.007
LaVerda, N. L., Goldsmith, D. F., Alavanja, M. C. R., and Hunting, K. L. (2015). Pesticide Exposures and Body Mass Index (BMI) of Pesticide Applicators from the Agricultural Health Study. J. Toxicol. Environ. Health, Part A 78 (20), 1255–1276. doi:10.1080/15287394.2015.1074844
Lock, E. A., and Reed, C. J. (1998). Xenobiotic Metabolizing Enzymes of the Kidney. Toxicol. Pathol. 26 (1), 18–25. doi:10.1177/019262339802600102
Mahgoub, A. A., and El-Medany, A. H. (2001). Evaluation of Chronic Exposure of the Male Rat Reproductive System to the Insecticide Methomyl. Pharmacol. Res. 44 (2), 73–80. doi:10.1006/phrs.2001.0816
Manole, A., Herea, D., Chiriac, H., and Melnig, V. (2008). Laccase Activity Determination. Scientific Annals of Alexandru Ioan Cuza din Iaşi University, 17–24.
Massoud, A., Derbalah, A., El-Mehasseb, I., Allah, M. S., Ahmed, M. S., Albrakati, A., et al. (2021). Photocatalytic Detoxification of Some Insecticides in Aqueous Media Using TiO2 Nanocatalyst. Ijerph 18 (17), 9278. doi:10.3390/ijerph18179278
Massoud, A., Derbalah, A. S., Iman, A., Abd-Elaziz, I., and Ahmed, M. (2010). Oral Toxicity of Malathion at Low Doses in Sprague-Dawley Rats: A Biochemical and Histopathological Study. Monofyia Vet. J. 7 (1), 183–196.
Massoud, A., El-Mehasseb, I., Saad Allah, M., Elmahallawy, E. K., Alsharif, K. F., S. Ahmed, M. M., et al. (2022). Advanced Oxidation Processes Using Zinc Oxide Nanocatalyst for Detoxification of Some Highly Toxic Insecticides in an Aquatic System Combined with Improving Water Quality Parameters. Front. Environ. Sci. 10, 807290. doi:10.3389/fenvs.2022.807290
Menrath, R. L. E., Gray, K. W., and Cameron, C. W. (1973). Toxicity of Bunamidine. N. Z. Veterinary J. 21, 212–215. doi:10.1080/00480169.1973.34109
Mortensen, S. R., Hooper, M. J., and Padilla, S. (1998). Rat Brain Acetylcholinesterase Activity: Developmental Profile and Maturational Sensitivity to Carbamate and Organophosphorus Inhibitors. Toxicology 125 (1), 13–19. doi:10.1016/s0300-483x(97)00157-1
Navarrete-Meneses, M. P., Salas-Labadía, C., Sanabrais-Jiménez, M., Santana-Hernández, J., Serrano-Cuevas, A., Juárez-Velázquez, R., et al. (2017). "Exposure to the Insecticides Permethrin and Malathion Induces Leukemia and Lymphoma-Associated Gene Aberrations In Vitro ". Toxicol. Vitro 44, 17–26. doi:10.1016/j.tiv.2017.06.013
Ncibi, S., Ben Othman, M., Akacha, A., Krifi, M. N., and Zourgui, L. (2008). Opuntia ficus Indica Extract Protects against Chlorpyrifos-Induced Damage on Mice Liver. Food Chem. Toxicol. 46 (2), 797–802. doi:10.1016/j.fct.2007.08.047
Neal, R. A. (1972). A Comparison of the In Vitro Metabolism of Parathion in the Lung and Liver of the Rabbit. Toxicol. Appl. Pharmacol. 23 (1), 123–130. doi:10.1016/0041-008x(72)90211-6
Ogutcu, A., Suludere, Z., and Kalender, Y. (2008). Dichlorvos-induced Hepatotoxicity in Rats and the Protective Effects of Vitamins C and E. Environ. Toxicol. Pharmacol. 26 (3), 355–361. doi:10.1016/j.etap.2008.07.005
Peter Guengerich, F., and Avadhani, N. G. (2018). Roles of Cytochrome P450 in Metabolism of Ethanol and Carcinogens. Alcohol Cancer, 15–35. doi:10.1007/978-3-319-98788-0_2
Recena, M. C., Pires, D. X., and Caldas, E. D. (2006). Acute Poisoning with Pesticides in the State of Mato Grosso Do Sul, Brazil. Sci. Total Environ. 357 (1-3), 88–95. doi:10.1016/j.scitotenv.2005.04.029
Reitman, S., and Frankel, S. (1957). A Colorimetric Method for the Determination of Serum Glutamic Oxalacetic and Glutamic Pyruvic Transaminases. Am. J. Clin. Pathol. 28 (1), 56–63. doi:10.1093/ajcp/28.1.56
Rettich, F. (1980). Residual Toxicity of Wall-Sprayed Organophosphates, Carbamates and Pyrethroids to Mosquito Culex pipiens Molestus Forskal. J. Hyg. Epidemiol. Microbiol. Immunol. 24 (1), 110–117.
Rezg, R., Mornagui, B., Kamoun, A., El-Fazaa, S., and Gharbi, N. (2007). Effect of Subchronic Exposure to Malathion on Metabolic Parameters in the Rat. Comptes rendus Biol. 330 (2), 143–147. doi:10.1016/j.crvi.2006.11.002
Rose, H. A., and Wallbank, B. E. (1986). Mixed-function Oxidase and Glutathione S-Transferase Activity in a Susceptible and a Fenitrothion-Resistant Strain of Oryzaephilus Surinamensis (Coleoptera: Cucujidae). J. Econ. entomology 79 (4), 896–899. doi:10.1093/jee/79.4.896
Savolainen, H. (1978). Superoxide Dismutase and Glutathione Peroxidase Activities in Rat Brain. Res. Commun. Chem. Pathol. Pharmacol. 21 (1), 173–176.
Sayim, F. (2007). Dimethoate-induced Biochemical and Histopathological Changes in the Liver of Rats. Exp. Toxicol. Pathol. 59 (3-4), 237–243. doi:10.1016/j.etp.2007.05.008
Selmi, S., El-Fazaa, S., and Gharbi, N. (2015). Oxidative Stress and Alteration of Biochemical Markers in Liver and Kidney by Malathion in Rat Pups. Toxicol. industrial health 31 (9), 783–788. doi:10.1177/0748233713475507
Shackelford, C., Long, G., Wolf, J., Okerberg, C., and Herbert, R. (2002). Qualitative and Quantitative Analysis of Nonneoplastic Lesions in Toxicology Studies. Toxicol. Pathol. 30 (1), 93–96. doi:10.1080/01926230252824761
Singh, S. P., Kumari, M., Kumari, S. I., Rahman, M. F., Mahboob, M., and Grover, P. (2013). Toxicity Assessment of Manganese Oxide Micro and Nanoparticles in Wistar Rats after 28 Days of Repeated Oral Exposure. J. Appl. Toxicol. 33 (10), 1165–1179. doi:10.1002/jat.2887
Steger, K., Rey, R., Louis, F., Kliesch, S., Behre, H., Nieschlag, E., et al. (1999). Reversion of the Differentiated Phenotype and Maturation Block in Sertoli Cells in Pathological Human Testis. Hum. Reprod. 14 (1), 136–143. doi:10.1093/humrep/14.1.136
Tchounwou, P. B., Patlolla, A. K., Yedjou, C. G., and Moore, P. D. (2015). Environmental Exposure and Health Effects Associated with Malathion Toxicity. Toxic. Hazard Agrochem. 51, 2145–2149. doi:10.5772/60911
Timur, S., Önal, S., Karabay, N. Ü., Sayim, F., and Zi̇hni̇oğlu, F. (2003). In Vivo effects of Malathion on Glutathione-S-Transferase and Acetylcholinesterase Activities in Various Tissues of Neonatal Rats. Turkish J. Zoology 27 (3), 247–252. doi:10.3906/zoo-0212-1
Toś-Luty, S., Obuchowska-Przebirowska, D., Latuszyńska, J., Tokarska-Rodak, M., and Haratym-Maj, A. (2003). Dermal and Oral Toxicity of Malathion in Rats. Ann. Agric. Environ. Med. 10, 101–106.
US Department of Health and Human Services (1999). Agency for Toxic Substances and Disease RegistryToxicological Profle for Asbestos (Update). Atlanta: Agency for Toxic Substances and Disease Registry.
Uzunhisarcikli, M., Kalender, Y., Dirican, K., Kalender, S., Ogutcu, A., and Buyukkomurcu, F. (2007). Acute, Subacute and Subchronic Administration of Methyl Parathion-Induced Testicular Damage in Male Rats and Protective Role of Vitamins C and E. Pesticide Biochem. physiology 87 (2), 115–122. doi:10.1016/j.pestbp.2006.06.010
Waber, H., and Dtsch, M. (1966). Cholinestrase Kinetic Colorimetric Method. Dtsch. Med. Wschr 91, 1927.
Yang, M. C., McLean, A. J., Rivory, L. P., and Le Couteur, D. G. (2000). Hepatic Disposition of Neurotoxins and Pesticides. Pharmacol. Toxicol. 87 (6), 286–291. doi:10.1034/j.1600-0773.2000.pto870608.x
Yehia, M. A., El-Banna, S. G., and Okab, A. B. (2007). Diazinon Toxicity Affects Histophysiological and Biochemical Parameters in Rabbits. Exp. Toxicol. Pathol. 59 (3-4), 215–225. doi:10.1016/j.etp.2007.09.003
Yun, J.-W., Kim, S.-H., You, J.-R., Kim, W. H., Jang, J.-J., Min, S.-K., et al. (2015). Comparative Toxicity of Silicon Dioxide, Silver and Iron Oxide Nanoparticles after Repeated Oral Administration to Rats. J. Appl. Toxicol. 35 (6), 681–693. doi:10.1002/jat.3125
Zheng, Y., and Hwang, H.-M. (2006). Effects of Temperature and Microorganisms on Malathion Transformation in River Water. Bull. Environ. Contam. Toxicol. 76 (4), 712–719. doi:10.1007/s00128-006-0978-y
Zhou, R., Yu, Y., Zhang, W., Wang, D., Bai, Y., Wang, Y., et al. (2022). Sensory Disturbance by Six Insecticides in the Range of μg/L in Caenorhabditis elegans. Front. Environ. Sci. 10, 859356. doi:10.3389/fenvs.2022.859356
Keywords: malathion, biochemical parameters, toxicity, histopathology, rats
Citation: Massoud A, SaadAllah M, Dahran NA, Nasr NE, El-Fkharany I, Ahmed MS, Alsharif KF, Elmahallawy EK and Derbalah A (2022) Toxicological Effects of Malathion at Low Dose on Wister Male Rats With Respect to Biochemical and Histopathological Alterations. Front. Environ. Sci. 10:860359. doi: 10.3389/fenvs.2022.860359
Received: 22 January 2022; Accepted: 13 April 2022;
Published: 11 May 2022.
Edited by:
Jiehong Guo, University of Minnesota Twin Cities, United StatesReviewed by:
Muhammad Adeel, Beijing Normal University, ChinaWu Dong, Inner Mongolia University for Nationalities, China
Copyright © 2022 Massoud, SaadAllah, Dahran, Nasr, El-Fkharany, Ahmed, Alsharif, Elmahallawy and Derbalah. This is an open-access article distributed under the terms of the Creative Commons Attribution License (CC BY). The use, distribution or reproduction in other forums is permitted, provided the original author(s) and the copyright owner(s) are credited and that the original publication in this journal is cited, in accordance with accepted academic practice. No use, distribution or reproduction is permitted which does not comply with these terms.
*Correspondence: Ehab Kotb Elmahallawy, ZWVoYWFAdW5pbGVvbi5lcw==