- 1Key Laboratory of State Forestry and Grassland Administration on Soil and Water Conservation, Beijing Forestry University, Beijing, China
- 2Yanchi Research Station, School of Soil and Water Conservation, Beijing Forestry University, Beijing, China
- 3Engineering Research Center of Forestry Ecological Engineering, Ministry of Education, Beijing Forestry University, Beijing, China
- 4China Institute of Water Resource and Hydropower Research, Beijing, China
- 5College of Resources and Environmental Economics, Inner Mongolia University of Finance and Economics, Hohhot, China
Pinus sylvestris var. mongolica (P. sylvestris) is a pioneer afforestation species for wind and sand fixation in northern China. However, most plantations have undergone unprecedented degradation since the early 1990s. Ectomycorrhizal (EcM) fungi are expected to be substantially related to the degradation of P. sylvestris plantations; however, the direct evidence for this remains unclear. For this purpose, we investigated variation among EcM fungal communities associated with P. sylvestris root samples of three age groups (half-mature, near-mature, and mature) in Mu Us Desert. Our results found a total of 72 EcM fungal operational taxonomic unit (OTU) belonging to Basidiomycota (63) and Ascomycota (9). The EcM fungal community is highly enriched in Rhizopogon, Tuber, Tomentella, and Inocybe, and the variations of their abundance mainly contribute to community differences. The diversity index and fungal species richness showed increasing trends toward the mature forest but did not reach a significant level (p > 0.05). The main factors that affected the dominant EcM fungal community were URE, INV, and SOC, but for the whole EcM fungal community, the effects of soil environments with plantation aging are not significant (p > 0.05). Understanding the effects of environmental factors on the structuring of the EcM fungal communities is critical to sustainable forest management in the future.
1 Introduction
Ectomycorrhizal (EcM) fungi are well known to form symbiotic associations with many dominant trees in temperate and boreal forest ecosystems (Kolarikova et al., 2017; Koizumi et al., 2018; Sasse et al., 2018). The long-standing coevolution process leads to the mutually beneficial interaction between plants and microorganisms (Lemanceau et al., 2017). EcM fungi obtain carbohydrates from the host plant to meet the needs of their own growth (Zak et al., 2019). As root mutualistic symbionts, EcM fungi assist their host plant by enhancing mineral nutrients and moisture access as well as protecting against pathogens and environmental stress (Tedersoo et al., 2020). Consequently, EcM fungi play vital roles in host health and stability maintenance of forest systems (Courty et al., 2010; Sebastiana et al., 2018), and various life processes of ecosystem are sustained by them. Soil environments and host features have proven to strongly control soil fungal communities at a local scale (Wang et al., 2021). Various studies have reported that EcM fungal communities are significantly affected by biotic (e.g., plant community) and abiotic (e.g., climate and soil physico-chemical property) factors (Tedersoo et al., 2012; Bachelot et al., 2016; Yang et al., 2019). Climates are crucial in shaping EcM fungal communities, for example, a too high or too low temperature is not suitable for the habit of many EcM fungi (van der linde et al., 2018). Arnolds (1991) has confirmed that conifers are negatively affected by increasing nitrogen. Further, stand age as a basis feature of the forest ecosystem has a strong impact on the establishment and development of the EcM fungi communities (Spake et al., 2016).
Pinus sylvestris var. mongolica (P. sylvestris) is the most important tree species for combating desertification in reforestation, Northern China. So far, it was introduced to more than 13 provinces and covered an area of more than 7.0 × 105 ha in semi-arid regions (Song et al., 2019). Unfortunately, P. sylvestris plantation has been experiencing dieback since 1990s (Song et al., 2018). To get around this, many hypotheses have been put forward and confirmed (Zhu et al., 2006). The two factors most commonly mentioned in these hypotheses are soil water and nutrients (Liu et al., 2009; Zhao et al., 2020). The water shortage and depletion of nutrients are important factors affecting plant growth in desertification areas in the desertified regions. Water is the major limiting factor for the survival and growth of P. sylvestris due to the large water consumption for transpiration and the limitations of natural rainfall conditions in arid and semi-arid ecosystems (Hu et al., 2015; Liu et al., 2021a). In addition, soil nutrient is an essential constraining factor affecting plantation development (Zhang et al., 2021). With the development of forests, soil nutrient depletion is serious, so the nutrients used for the EcM fungal growth are limited. The declined groundwater and erratic precipitation upset the water balance of plants and restrict the growth and development of plants by affecting nutrient absorption (Geml et al., 2017). However, considering these two primary causal factors alone cannot fully explain the phenomenon of plantation degradation. Therefore, it remains a major challenge to reveal why P. sylvestris plantations were degraded on such a large scale.
Temperate forest ecosystems of semi-arid deserts are changing as a result of the increased drought (Garcia et al., 2016). Ectomycorrhizal symbionts enhance plant resistance to drought stress and damage compensation and maintain the dynamic balance and structural stability of forest ecosystems. In consequence, the variations of the EcM fungal communities directly affect forest growth and health (Soudzilovskaia et al., 2015). Furthermore, soil microorganisms are sensitive to climate changes; hence, EcM fungi also affect the response of the whole forest ecosystem to environmental change (Tedersoo et al., 2012; van der Linde et al., 2018). P. sylvestris is a typical ectomycorrhizal-dependent species, and the existence of it is almost entirely dependent on the presence of symycotic fungi (Dean et al., 2015; Guo M.-s. et al., 2020). Consequently, it is becoming increasingly clear that the potential of the EcM fungi in degraded plantations cannot be ignored.
P. sylvestris has been introduced into Mu Us Desert for decades and suffered serious degradation. Here, we hypothesized that the changes in the EcM fungal community are largely a consequence of the variations in the soil system caused by stand aging and lead to the degradation of P. sylvestris plantation. For this purpose, we collected the root of P. sylvestris plantations with various stand ages in the Mu Us Desert, and the EcM fungi community composition was examined by high-throughput sequencing technology. The objectives of this study were (1) to identify the EcM fungal community composition of P. sylvestris plantation among different stand ages and (2) to determine the major drivers of the EcM fungal community compositions of P. sylvestris plantation in the Mu Us Desert. This improved information can provide a deep-going implication on efficiently restoring degraded plantations.
2 Materials and Methods
2.1 Study Site
The study site was located in the Hongshixia Sandy Botanical Garden of Yulin City, Shaanxi Province (38°26′N, 109°12′E, 1080 m a.s.l.), which is on the southern edge of the Mu Us Desert (Supplementary Figure S1). It is characterized by the temperate semi-arid continental monsoon climate with an annual temperature, precipitation, and evaporation of 9.1°C, 385 mm, and 2914 mm, respectively. The frost-free period ranges from 160 to 170 days. The soil is mainly composed of aeolian sandy soil, which is loose and very susceptible to flow and erosion. The dominated arbor plants are P. sylvestris and P. tabuliformis. The shrub and herb include Caragana korshinskii, Lespedeza bicolor, Cares duriuscula, Rhamnus parvifolia, Salsola collina, and Potentilla anserine.
2.2 Sample Collection and Treatment
Field investigation and sample collection were conducted in August 2017, which is the peak of plant growth season and microbial activity period. We randomly set a 50 m × 50 m plot in P. sylvestris plantations across 26, 32, and 43 a, which represent half-mature (MUh), near-mature (MUn), and mature (MUm) forests, respectively (Table 1). There were no tending measures and visible disturbance by anthropogenic activities since the establishment of plantations.
At each plot, five healthy and widely spaced standard trees were selected as sampling objects. Loose litters, herbs, and the undergrowth humus layer were gently removed prior to digging out the fine root samples carefully along the base of the trunk. After that, three repeated fine samples were taken from each standard tree; then, the three samples were fully mixed. A total of 15 mixed root samples (3 plots × 5 mixed samples = 15 composite samples) were used for DNA extraction and molecular identification. The ectomycorrhizal infected roots were accompanied with soil placed into plastic bags to keep the root tips fresh. Root samples were kept cool after sampling and stored at 4°C. Soil samples were collected around the root, with three repeated soil samples collected in each point, and fully mixed into one (3 plots × 5 mixed samples = 15 composite samples). Soil samples from 0 to 20 cm depth were obtained to determine soil physico-chemical properties and enzyme activities. After passing through a 2 mm sieve, the soil samples were divided into two parts; one was stored at 4°C in a refrigerator to determine enzyme activities and the content of available nutrients in soils, and the other was taken straight back to determine soil physical properties, soil organic matter (SOM), and soil total nutrient.
2.3 Soil Property Analysis
Soil water content (SWC) was determined by the gravimetric method after drying the soil sample in an oven at 105°C for 12 h. Soil pH was measured using a pH meter (PHS-3E, INESA, Shanghai, China); the mixture of soil and water is 1:2.5. Soil organic matter (SOM) content was measured by the K2Cr2O7–H2SO4 oxidation technique (Walkley and Black, 1934). Soil total nitrogen (TN), total phosphorus (TP), nitrate nitrogen (NO3−-N), and ammonium nitrogen (NH4+-N) were determined using an automated element analyzer (Smartchem 200, Italy). TN used the indophenol-blue spectrophotometric method, and TP used the Mo-Sh anti-colorimetric analysis method (John and Matt, 1970; Mason et al., 1999). Soil invertase (INV) activity, urease (URE) activity, and phosphatase (PHO) activity were assayed as described by Tabatabai (1994).
2.4 Molecular Identification of EcM Fungi
DNA was extracted and purified using the Powersoil® DNA Isolation Kit (Mo Bio Laboratories, Carlsbad, CA, United States), according to the manufacturer's protocol. The genomic DNA concentrations were checked on 1% agarose gels electrophoresis and quantified on a NanoDrop NC2000 spectrophotometer (Thermo Fisher Scientific, Waltham, MA, United States). The fungal internal transcribed spacer (ITS) region was amplified on Eppendorf Mastercycler Gradient Thermocycler (Germany); for the ITS1 regions of fungi, the primers were ITS1F (5′-GGAAGTAAAAGTCGTAACAAGG-3′) and ITS2 (5′-TCCTCCGCTTATTGATATGC-3′) (Amend et al., 2010). For each sample, an eight-digit barcode sequence was added to the 5′ end of the forward and reverse primers (provided by Allwegene Company, Beijing). The polymerase chain reaction (PCR) mixtures were analyzed on a Mastercycler Gradient (Eppendorf, Germany) using 25 μL reaction volumes, containing 2.5 μL 2 × Taq PCR MasterMix, 3 μL BSA (2 ng/μL), 1 μL Forward Primer (5 μM), 1 μL Reverse Primer (5 μM), 2 μL template DNA, and 5.5 μL ddH2O. The cycling parameters were 95°C for 5 min, followed by 28 cycles of 95°C for 45 s, 55°C for 50 s, and 72°C for 45 s with a final extension at 72°C for 10 min. The PCR products were purified using an Agencourt AMPure XP Kit. Deep sequencing was performed on an Illumina Miseq platform at Allwegene Company (China, Beijing). After the run, image analysis, base calling, and error estimation were performed using Illumina Analysis Pipeline Version 2.6.
The raw data were first screened, and then the sequences shorter than 230 bp and with low-quality scores (≤20) were removed. After removing non-fungal sequence reads, the fungal sequences were clustered into operational taxonomic units (OTUs) (Blaxter et al., 2005) at a 97% similarity level using the Uparse algorithm of Vsearch (Version 2.7.1) (Edgar, 2010). The remaining high-quality sequences were queried against the GenBank (https://academic.oup.com/nar/article/50/D1/D161/6447240) non-redundant nucleotide database in NCBI using local BLASTn (https://blast.ncbi.nlm.nih.gov/). The MEGAN (Version 6.0) program was used to assign BLAST hits to taxa of the NCBI taxonomy. Non-EcM fungal OTUs were filtered out by FUNGuild (Version 1.0, http://www.stbates.org/guilds/app.php) (Zhang et al., 2015). The key EcM fungal species were analyzed by using the analysis of modular topological roles (within-module connectivity (Zi) ≥ and among-module connectivity (Pi) ≥ 0.62). All the identified sequences were submitted to the GenBank with the accession numbers of SUB7166485 (from MT229482 to MT229553) (Table 3).
2.5 Data Analysis
Define “dominant species” as the EcM fungi detected in 80% of all samples, “frequent species” as the EcM fungi detected in 30% of all samples, and “rare species” as the EcM fungi detected in less than 30% of all samples. The important value of OTUs is represented by the sum of its relative abundance and relative abundance. α-diversity indices were calculated based on the OTU dates, including the number of identified genera (S), Shannon index (H′), Simpson’s index (d), and evenness index of species richness [Pielou (J)]. The differences in community structure of the EcM fungi were analyzed by non-metric multidimensional scaling (NMDS) analysis. Bray–Curtis dissimilarity index was calculated to examine the dissimilarity of the OTU community in different sites. Dissimilarity matrices of environmental data sets were calculated based on Euclidean distance. A redundancy analysis (RDA) was used to determine the correlation between the EcM fungal community (genus level) and soil properties. Subsequently, Spearman rank correlation was used to explain the relationship between the EcM fungal community (genus level) and soil properties, also based on Bray–Curtis distance. The correction heatmap was a visual correlation between all soil properties and the five most frequently recovered genera. After converting soil properties to a matrix, we performed the Mantel test to determine the significance of each environmental variable. The EcM fungal network was constructed based on random matrix theory using the OTU data, which present in at least eight (>50%) samples (Deng et al., 2012). All network analyses were performed using the MENA platform (http://ieg4.rccc.ou.edu/mena/main.cgi).
One-way analysis of variance (ANOVA) with the least-significant difference (LSD) and the Tukey test were performed to determine the significant difference among soil variables and EcM fungal diversity. All statistical analyses were performed in SPSS (Version 20.0), and p < 0.05 was considered statistically significant. The α-diversity indices, Bray–Curtis dissimilarity index, network analysis, correction heatmap, and Mantel test were implemented using the R (Version 3.5.1) program. RDA was performed using CANOCO (Version 4.5). The network diagram was visualized by Cytoscape (Version 3.9.0).
3 Results
3.1 Soil Properties
In P. sylvestris plantation, all the soil properties varied by stand age (p < 0.05, Table 3). Soil TN, SOM, NO3−, INV, and URE were significantly different with stand aging (p < 0.05). In general, soil in the Mu Us Desert was alkali to neutral. The maximum values of soil nutrient contents (SOM, TN, and TP) were found in half-mature forests. Soil URE and PHO enzymes were the most active in mature forests.
3.2 Diversity and Composition of the EcM Fungal Community
A total of 361,548 high-quality sequences were obtained from roots associated with P. sylvestris, assigned to 807 fungal OTUs. A total of 72 of these had an EcM lifestyle and were identified to the genus level and below (Table 2). With stand aging, the numbers of OTUs and rhiness of genus increased first and then decreased; the values of the remaining α-diversity indices gradually decreased. The impact of stand age on the EcM fungal community has not reached a significant level (p > 0.05, Figure 1).
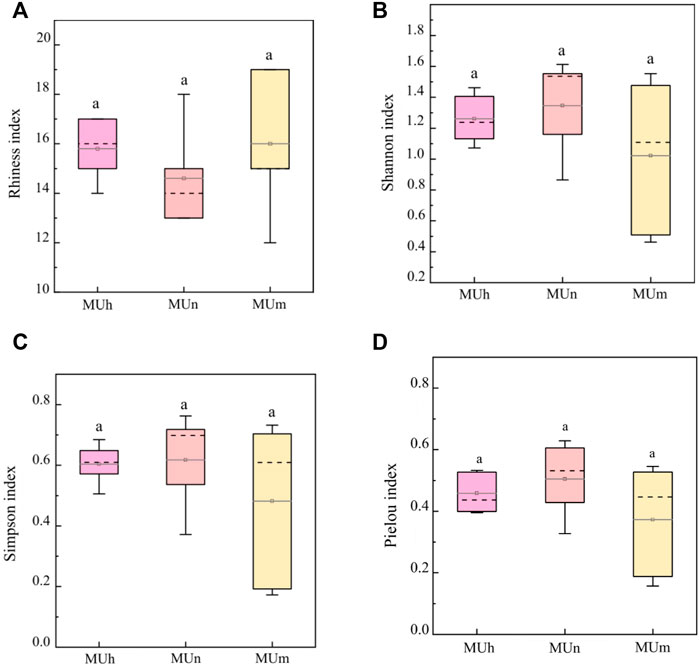
FIGURE 1. Diversity of the EcM fungi associated with P. sylvestris at different age groups. Letters indicate significant differences for each parameter (p < 0.05; one-way ANOVA, Tukey test) based on the values determined for each site (5 replicates/site). (A) Rhiness index, (B) Shannon index, (C) Simpson index, (D) Pielou index.
Our studies found that the EcM fungal OTUs of P. sylvestris plantations in the Mu Us Desert were assigned to two phyla, four classes, 12 orders, 18 families, and 23 genera (Figure 2; Supplementary Table S1). Of all 72 EcM OTUs, Basidiomycota was the most abundant phylum (83%) with 63 OTUs, following by Ascomycota (17%) with nine OTUs. Among them, 13 and 14 species were detected for the dominant and common species, respectively. The rare species was the largest pool (45 species) accounting for 62.50% of the total species. At the genus level, Rhizopogon was the most dominant EcM fungus; it has the highest relative abundance in all age groups, especially in MUn and MUm (more than 50%, Figure 2). Besides, the genera of Tuber, Tomentella, and Inocybe were also widely distributed in all plantations. With the aging of stand, Rhizopogon increased first and then decreased, while Tomentella and Inocybe were just the opposite. Tuber was accumulated with stand age.
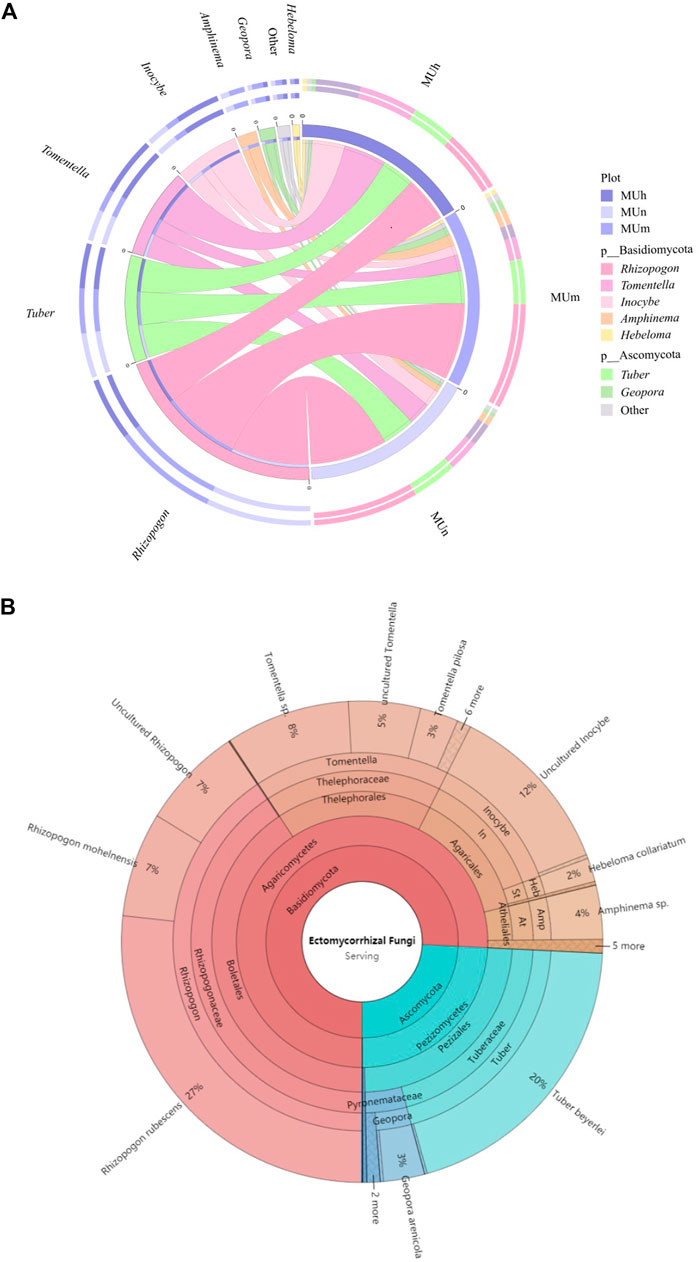
FIGURE 2. Distribution and relative abundance of the EcM fungal community at the genus level (A) and a Krona chart of taxonomic affiliation of EcM fungi (B). Inner circles represent higher taxonomic ranks, and more detailed taxonomic ranks (up to the species level) are presented in outer circles. In: Inocybeceaea, St: Strophariaceae, Heb: Hebloma, Amp: Amphinema of P. sylvestris plantation in three age groups. “Other” represents genera with relative abundance <1%, which are Hygrocybe, Suillus, Cortinarius, Clitopilus, Agaricus, Ramaria, Lactarius, Hydnum, Geastrum, Tulostoma, Peziza, Wilcoxina, Genabea, Sebacina, and Lachnum. The left half of the figure shows the relative abundance of EcM fungal genera in different plots, and the right half shows the relative abundance of different EcM fungal genera in the sample plot.
The Bray–Curtis distances of the EcM fungal community composition in three stand ages are shown and ordered by NMDS. The stress value is 0.1679 (<0.2), so the Bray–Curtis distance can better reflect the difference of community composition (Table 3). The coordinates of scatter points indicated that the EcM fungal composition varied in different stand ages (Figure 3). ANOSIM results confirmed that the difference of fungal community structure was obvious with stand age (R = 0.88, p > 0.05). Based on the area of the confidence ellipse and Bray–Curtis distances (Figure 3; Table 3), the EcM fungal community structures of the near-mature and mature forests were the most similar.
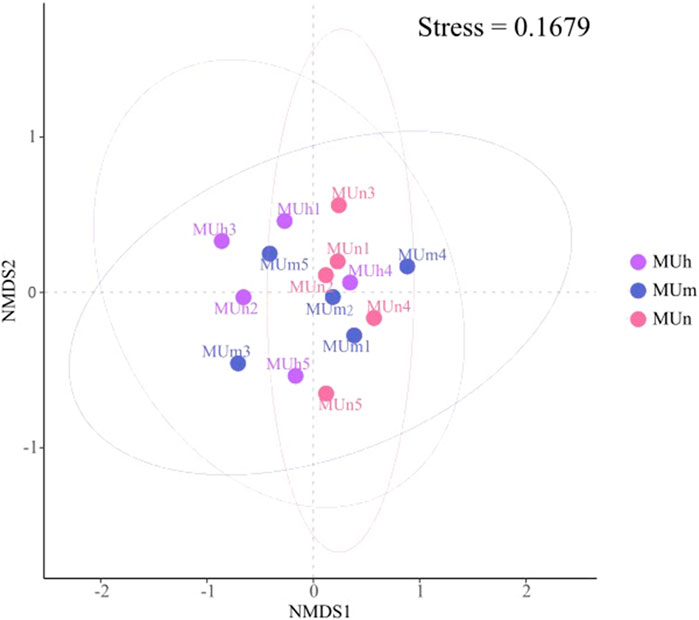
FIGURE 3. NMDS ordination of the EcM fungal community associated with P. sylvestris at different age groups, Stress = 0.1679. Each point represents a different sample (five samples from each age group). The confidence ellipse represents the confidence region in different age plots (α = 0.05).
3.3 Corrections Between EcM Fungal Communities
The molecular ecological network of the EcM fungi reflects the coexistence relationship of species in the environment (Figure 4). After removing the OTUs with average relative abundances less than 0.01%, a network that included 57 nodes and 435 edges was constructed. Of all these edges, 206 (47.36%) had shown positive corrections and 229 (52.64%) had shown negative correlations. In the EcM fungal network, the average degree, average clustering coefficient, and average path distance were 15.263, 0.571, and 1.875, respectively. The modularity calculation of the EcM network divides the whole network into four individual models (Supplementary Figure S2). OTU306 (classified as Rhizopogon mohelnensis), OTU43 (uncultured Peziza, Peziza sp.), OTU40 (classified as Clitopilus sp.), and OTU14 (classified as Clitopilus sp.) of Basidiomycota and OTU114 (classified as Rhizopogon mohelnensis), OTU56 (classified as Wilcoxina mikolae), and OTU36 (classified as Geopora arenicola) of Ascomycota had weak relationship with other species. We identified key EcM fungal species by using the analysis of modular topological roles. According to within-module connectivity (Zi) and among-module connectivity (Pi), OTU60 (Zi = 2.60 > 2.5, Pi = 0.625 > 0.6, classified as Hebeloma collariatum) was the core species of the model (Supplementary Figure S3).
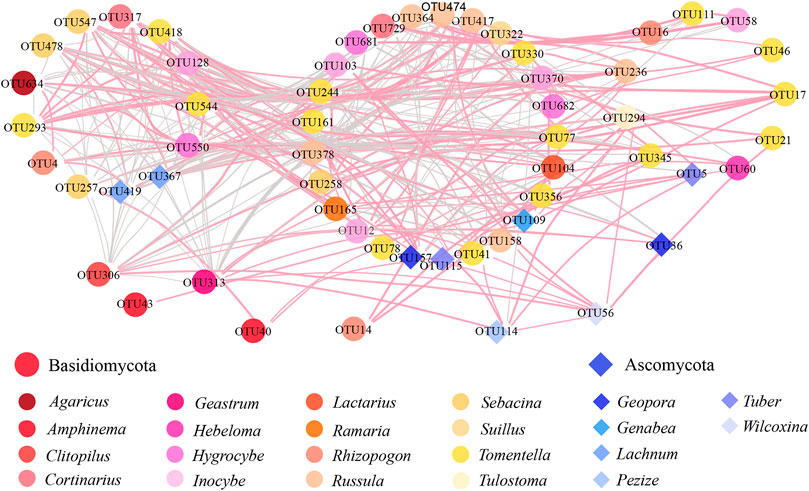
FIGURE 4. EcM fungal co-occurrence networks of P. sylvestris plantation. The dots and triangles in the figure represent different EcM OTUs, and different colors of shapes represent different fungal genera. The thickness of links indicates the edge weight of genera. The pink line indicates a positive correlation, and the gray line indicates a negative correlation.
3.4 Relationship Between EcM Fungal Communities and Soil Properties
The EcM fungal community composition through stand ages was driven by soil properties (Figures 5, 6; Supplementary Table S3). The redundancy (RDA) analysis revealed the impact of soil characteristics on the EcM fungal community, and the contribution rates of principal components 1 and 2 were 25.78 and 18.49%, respectively. All four axes in total contributed 67.3% of the explanation (Figure 5). Among all soil properties, NO4+ content had the highest contribution rate (15.32%). The contributions of SOC, TN, INV, and stand age all exceeded 11%.
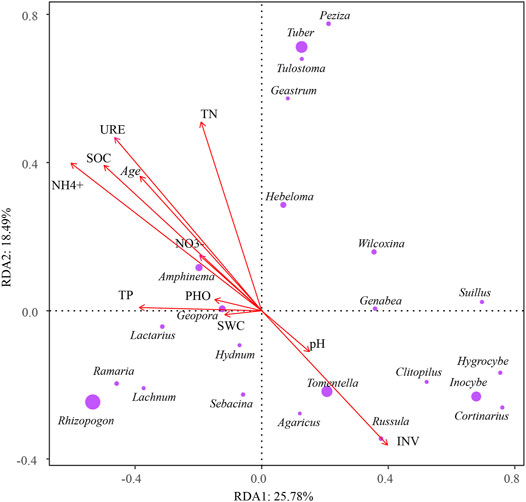
FIGURE 5. RDA illustrating the effects of soil characteristics on the EcM fungal community. The red arrow represents soil characteristics. The purple circle represents EcM fungi, and the size of the circle represents the relative abundance. The arrow direction and length indicate correlation to EcM fungal composition and effect size of the variables.
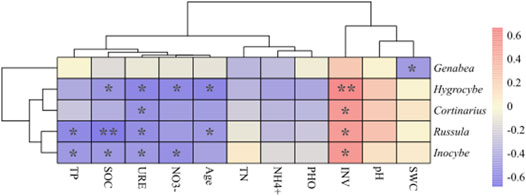
FIGURE 6. Correction heatmap between soil factors and main EcM fungi. The x-axis and y-axis are environmental factors and the top five genera. The R values and p value of Spearman’s rank correlation are obtained by calculation. R values are shown in different colors; the legend on the right is the color range of different R values. *: p < 0.05, **: p < 0.01.
The correction heatmap and Mantel test between soil factors and major EcM genera were created by Spearman’s rank correlation (Figure 6; Supplementary Tables S2, S3). The INV had a significant positive effect on the EcM fungal community, while SOC, TP, and stand age have a significant negative effect on the major EcM fungi (p < 0.05 or p < 0.01). URE and NO3− had an excellent negative correlation to the EcM fungal community (p < 0.05), although their contribution rates were low.
4 Discussion
4.1 Variations in EcM Fungal Diversity and Community Composition
EcM fungi are abundant and diverse in temperate and boreal forests (Steidinger et al., 2020), and the succession and variation of the underground EcM fungal community was a complex ecological process (Bardgett and van der Putten, 2014; Franklin et al., 2014). The diversity indices and community structure of EcM fungi changed with stand aging in the Mu Us Desert, but the effect of forest age was not significant. This was consistent with previous studies that the diversity of the fungal community was not correlated with the aging stand (Wang et al., 2020). As expected, the EcM fungal community was dominated by Basidiomycota rather than Ascomycetes, which is consistent with previous results (Geml et al., 2017). It is known that most of the known EcM fungi are Basidiomycota, which are diverse and adaptive to various environments (Boeraeve et al., 2018). Therefore, Basidiomycota considerably contributes to the ecological restoration in deserts.
The endemic genera were found across different stands, and the EcM fungal community structure was significantly different between three age stands. The distribution pattern of the EcM fungi indicates the succession of EcM fungi during forest development and the influence of stand age on it. The variations in plant community and soil characteristics caused by stand age altered the establishment and distribution of the EcM fungal community (Hayward et al., 2015). Rhizopogon was the dominant EcM fungal genera of P. sylvestris across all stand ages in the Mu Us Desert. Besides, Tomentella, Inocybe, and Tuber were also widely distributed in P. sylvestris plantations. These OTU-richest genera are also commonly detected in boreal coniferous forests (van Dorp et al., 2016). They are easily dispersed and widely adapted to the harsh environment (Ryberg et al., 2008; Bahram et al., 2011). Obviously, they are “multistage” fungi with an excellent competitive ability (Taylor and Bruns, 1999).
As the largest genus of hypogenous Basidiomycota, Rhizopogon were widely distributed and associated with pines; it is also the most common EcM fungi of P. sylvestris plantations in the Mu Us Desert. Especially, Rhizopogon proliferated in the high-carbon near-mature forest due to the high demand for carbohydrates (Mujic et al., 2014). Further, R. rubescens and R. mohelnensis contribute to the stress resistance; therefore, they conduce to the improvement in survival of conifer seedlings in a desert environment (Bahram et al., 2011). As saprotrophic EcM fungi, Tomentella prospers the nutrient cycling and is widely distributed throughout the Northern hemisphere forests (Han et al., 2017). Besides, Tomentella is also of great resistance to environmental stresses, supported by the presence in the degraded oak forest (Mosca et al., 2007). Inocybe is ecologically important in the forest succession by providing water and minerals for the host plants (Mason et al., 1983). In the Mu Us Desert, Inocybe were clearly decreasing from the half-mature forest to a mature forest. Soil moisture consumption is greatly responsible for this instance due to their preference of an arid environment, which has obvious significance in the desertification region. Furthermore, Cortinarius, Amphinema, and Russula were widely distributed in the mature forest. They are typical “late-stage” fungi, which are usually found associated with older forests and have strong competitive abilities (Hayward et al., 2015; Guo M. et al., 2020). The core fungi of the EcM fungal network are classified as Hebeloma, which is a frequent EcM genus, and its species is usually associated with a wide range of Pinaceae trees (Gryta et al., 2010).
Suillus is regarded as the most generic EcM fungi associated with pine, but it was diminished in the Mu Us Desert; this result is inconsistent with previous studies (Policelli et al., 2018). Geopora is considered to be an important mutual partner for host plants resisting the stress conditions. Notably, Russula and Peziza are also detected in the P. sylvestris plantations, which usually rely on fertile soil and prefer high pH, respectively. Lactarius, Hebeloma, and Lachnum accounted for a very small percentage; this was supported by the previous studies that they were not easily established in desertification regions (Desai et al., 2016). Curiously, some common drought-tolerant and barren-resistant EM fungal species were missed, such as Lycoperdon and Cenococcum (Jany et al., 2003).
4.2 The Drivers of the EcM Fungal Community
The primary drivers of the EcM fungi community at different scales are not necessarily the same (Peay and Matheny, 2016). EcM fungi were strongly determined by the soil environment, and the edaphic factors are approved to be the major drivers of the EcM fungal community at a local scale (Miyamoto et al., 2015). Forest development is usually accompanied by variations in the soil system (Zhao et al., 2020); EcM fungi are highly sensitive to the environmental changes. Therefore, host-related variables strongly influence the EcM fungal community. The variations of soil properties with stand aging are of great importance to shape fungal communities (Truong et al., 2019). We found that most of the factors we measured in this study were proved to sufficiently affect the community structure of the EcM fungi. Among them, the soil enzyme activities of invertase and urease were the most major abiotic factors causing EcM fungal communities in cation regions. They were the most important enzymes for soil fertility and nitrogen metabolism. ECM fungi have different preferences and demands for nutrient strategies, and the nutrient competition in fungal communities alters the assembly of the EcM fungal communities (Yang et al., 2021). EcM fungi-induced soil nutrient cycling substantially contributes to the growth and health of host plants. Nitrogen is the foremost limiting nutrient in conifer forest systems (Sebastiana et al., 2018). In our study, N shift was clearly observed, that is, NH4+ and NO3− concentrations significantly increased with aging stand. Meanwhile, EcM fungi (e.g., Peziza and Russlua) were negatively correlated with soil N availability. This finding confirms that EM fungal absorption capacity varies in response to the various forms of N, so N availability determines the community composition of EcM fungi (Kjøller et al., 2012). Furthermore, EcM fungi are good decomposers of soil organic matter and play a major role in the turnover and stabilization process of soil nutrients. The sufficient soil nutrients provide a suitable microenvironment for fungi, which is the basis of expanding and prospering the EcM fungal community.
Water shortage was considered to be an important abiotic factor; it was also the strongest abiotic candidate filter (Liu et al., 2021b). This result is consistent with previous studies that soil moisture is a key factor to alter the EcM fungi community composition in arid environments (Dean et al., 2015). Meanwhile, with the specificity of EcM fungi to drought stress, in return, variations in the EcM fungal community have direct impacts on the growth and development of host plants (Kennedy and Peay, 2007). Besides, considerable research studies have been devoted to come to the conclusion that soil pH is another key driver of the EcM fungal community (Kutszegi et al., 2015). The EcM fungal community changes significantly even with a very small variation of pH values. On one hand, soil pH affects the growth of the EcM fungi and mycelial metabolism. On the other hand, soil pH indirectly alters the EcM fungal community by changing nutrient availability (Bennett et al., 2017). Generally, EcM fungi prefer slightly acidic to neutral soils, and the growth of the EcM fungi is inhibited at a higher-pH environment. In the Mu Us Desert, the soil pH ranged from 7.27 to 7.58. The weakly alkaline soil leads to potential variations in the EcM fungal community.
EcM fungi can secrete nutrient-related enzymes to regulate the nutrient cycling. The active soil enzyme contributes to the N and P absorption and slows the decomposition of soil organic matter (Lindahl and Tunlid, 2014). Therefore, the interaction between EcM fungi and the soil system is affected by soil enzyme activity. Generally speaking, urease and phosphatase benefit the mineralization of nitrogen and phosphorus, thus providing available nitrogen and phosphorus for the hosts (Bidartondo et al., 2001). In this study, the urease activity is increasing with stand aging; it is beneficial to available nitrogen supply for host plants. This finding was also confirmed by the soil nitrogen in suit investigation. Invertase is related to the transformation of soil organic carbon, but with the increase in soil organic matter, invertase activities were significantly reduced. This reduction was also reported that only a few EcM fungi have saprophytic functions and are active in the decomposition of soil organic matter (Jonard et al., 2014).
4.3 Implications of EcM Fungi for P. sylvestris Plantation Degradation
EcM fungi and host plants are a community of shared common interests, and they get feedback from each other. The growth of P. sylvestris plantation is inseparable from the assistance of EcM fungi, and EcM fungi also benefit from the host plant (Zhu et al., 2006). Therefore, the interaction between EcM fungi and host plants is a potential but crucial indicator for the degradation of P. sylvestris plantation. Natural forests with diverse ages and hierarchical structures usually have abundant, diverse, and complex EcM fungal community compositions and structures (Grebenc et al., 2009). Compared to the original forest, artificial plantation is simple in structure and has poor stability, resulting in EcM fungi with low heterogeneity and diversity (Guo M. et al., 2020). Therefore, this simple EcM fungal community composition may be one of the reasons for the degradation of plantation. There is overwhelming evidence corroborating the notion that structure and diversity determine ecosystem function and stability (Huang et al., 2021).
As the most typical EcM fungi, Suillus has extreme host specificity of Pinaceae, especially P. sylvestris. Suillus forms symbionts with conifer species and substantially contributes to P. sylvestris invasion and seedling establishment by improving soil nutrient absorption (Policelli et al., 2018). Previous studies have demonstrated that Suillus is the dominant EcM fungal genus of P. sylvestris in nature forests (Truong et al., 2019). However, only one species of Suillus associated with P. sylvestris was found in the Mu Us Desert. Hence, we deduced that functional EcM fungi Suillus (e.g., Lycoperdon, Cenococcum) commonly found in nature forests contribute to the P. sylvestris plantation degradation. This assignment has explained the central importance of EcM fungi in P. sylvestris plantation degradation. In a desert environment, there are intensely interspecific and intraspecific competitive interactions for the limiting resources. Therefore, the simplification community structure and the loss of EcM fungal diversity and function eventually lead to the degradation of P. sylvestris plantation.
5 Conclusion
In Mu Us Desert, the obtained 72 ECM fungal OTUs were identified into two phyla and 23 genera associated with P. sylvestris plantation, and the EcM fungal composition was dominated by Rhizopogon, Inocybe, Tomentella, and Tuber. Diversity indices and community structures of the EcM fungi had no significant effect by stand age. The major abiotic drivers of the dominant EcM fungal genera were NO4+, SOC, TN, INV, and stand age; thus, the combination of soil physico-chemical properties and soil enzyme activities jointly drives the variations of the major EcM fungal community to a certain extent. We highly suppose that the soil-induced community simplification and assembly variations of the EcM fungi and the loss of some fungal groups (e.g., Suillus) with specific functions substantially contribute to the degradation of P. sylvestris plantation. In the future, sustainable forest management requires unremitting efforts on EM fungal community structures and functions at the spatial and temporal multiscale toward a changing world.
Data Availability Statement
The datasets presented in this study can be found in online repositories. The names of the repository/repositories and accession number(s) can be found below: NCBI [accession: MT229482-MT229553].
Author Contributions
YR and MG performed the experiments. YR conceived the pager, analyzed the data, and wrote paper. YR and GD led the writing of the manuscript. All authors performed supervision and finalized the manuscript and agree to be accountable for all aspects of the work.
Funding
This work was founded by the Fundamental Research Funds for the Central Universities, the National Key Research and Development Program of China, and the Natural Science Foundation of Inner Mongolia [Grant numbers (2021ZY47), (2018YFC0507100), and (2020BS03001)].
Conflict of Interest
The authors declare that the research was conducted in the absence of any commercial or financial relationships that could be construed as a potential conflict of interest.
Publisher’s Note
All claims expressed in this article are solely those of the authors and do not necessarily represent those of their affiliated organizations or those of the publisher, the editors, and the reviewers. Any product that may be evaluated in this article or claim that may be made by its manufacturer is not guaranteed or endorsed by the publisher.
Supplementary Material
The Supplementary Material for this article can be found online at https://www.frontiersin.org/articles/10.3389/fenvs.2022.858452/full#supplementary-material
References
Amend, A. S., Seifert, K. A., Samson, R., and Bruns, T. D. (2010). Indoor Fungal Composition Is Geographically Patterned and More Diverse in Temperate Zones Than in the Tropics. Proc. Natl. Acad. Sci. U.S.A. 107 (31), 13748–13753. doi:10.1073/pnas.1000454107
Arnolds, E. (1991). Decline of Ectomycorrhizal Fungi in Europe. Agric. Ecosyst. Environ. 35, 209–244. doi:10.1016/0167-8809(91)90052-Y
Bachelot, B., Uriarte, M., Zimmerman, J. K., Thompson, J., Leff, J. W., Asiaii, A., et al. (2016). Long-lasting Effects of Land Use History on Soil Fungal Communities in Second-Growth Tropical Rain Forests. Ecol. Appl. 26, 1881–1895. doi:10.1890/15-1397.1
Bahram, M., Põlme, S., Kõljalg, U., Zarre, S., and Tedersoo, L. (2011). Regional and Local Patterns of Ectomycorrhizal Fungal Diversity and Community Structure along an Altitudinal Gradient in the Hyrcanian Forests of Northern Iran. New Phytol. 193, 465–473. doi:10.1111/j.1469-8137.2011.03927.x
Bardgett, R. D., and van der Putten, W. H. (2014). Belowground Biodiversity and Ecosystem Functioning. Nature 515 (7528), 505–511. doi:10.1038/nature13855
Bennett, J. A., Maherali, H., Reinhart, K. O., Lekberg, Y., Hart, M. M., and Klironomos, J. (2017). Plant-soil Feedbacks and Mycorrhizal Type Influence Temperate forest Population Dynamics. Science 355, 181–184. doi:10.1126/science.aai8212
Bidartondo, M. I., Ek, H., Wallander, H., and Söderström, B. (2001). Do nutrient Additions Alter Carbon Sink Strength of Ectomycorrhizal Fungi? New Phytol. 151, 543–550. doi:10.1046/j.1469-8137.2001.00180.x
Blaxter, M., Mann, J., Chapman, T., Thomas, F., Whitton, C., Floyd, R., et al. (2005). Defining Operational Taxonomic Units Using Dna Barcode Data. Phil. Trans. R. Soc. B 360 (1462), 1935–1943. doi:10.1098/rstb.2005.1725
Boeraeve, M., Honnay, O., and Jacquemyn, H. (2018). Effects of Host Species, Environmental Filtering and forest Age on Community Assembly of Ectomycorrhizal Fungi in Fragmented Forests. Fungal Ecol. 36, 89–98. doi:10.1016/j.funeco.2018.08.003
Courty, P.-E., Buée, M., Diedhiou, A. G., Frey-Klett, P., Le Tacon, F., Rineau, F., et al. (2010). The Role of Ectomycorrhizal Communities in forest Ecosystem Processes: New Perspectives and Emerging Concepts. Soil Biol. Biochem. 42, 679–698. doi:10.1016/j.soilbio.2009.12.006
Dean, S. L., Warnock, D. D., Litvak, M. E., Porras-Alfaro, A., and Sinsabaugh, R. (2015). Root-associated Fungal Community Response to Drought-Associated Changes in Vegetation Community. Mycologia 107, 1089–1104. doi:10.3852/14-240
Deng, Y., Jiang, Y.-H., Yang, Y., He, Z., Luo, F., and Zhou, J. (2012). Molecular Ecological Network Analyses. BMC Bioinformatics 13, 113. doi:10.1186/1471-2105-13-113
Desai, N. S., Wilson, A. W., Powers, J. S., Mueller, G. M., Egerton-Warburton, L. M., and Egerton-Warburton, L. M. (2016). Ectomycorrhizal Diversity and Community Structure in Stands of Quercus Oleoides in the Seasonally Dry Tropical Forests of Costa Rica. Environ. Res. Lett. 11, 125007. doi:10.1088/1748-9326/11/12/125007
Edgar, R. C. (2010). Search and Clustering Orders of Magnitude Faster Than BLAST. Bioinformatics 26 (19), 2460–2461. doi:10.1093/bioinformatics/btq461
Franklin, O., Näsholm, T., Högberg, P., Högberg, M. N., and Högberg, M. N. (2014). Forests Trapped in Nitrogen Limitation - an Ecological Market Perspective on Ectomycorrhizal Symbiosis. New Phytol. 203, 657–666. doi:10.1111/nph.12840
Geml, J., Morgado, L. N., Semenova‐Nelsen, T. A., and Schilthuizen, M. (2017). Changes in Richness and Community Composition of Ectomycorrhizal Fungi Among Altitudinal Vegetation Types on Mount Kinabalu in Borneo. New Phytol. 215, 454–468. doi:10.1111/nph.145610.1111/nph.14566
Gryta, H., Debaud, J. C., Effosse, A., Gay, G., and Marmeisse, R. (1997). Fine‐scale Structure of Populations of the Ectomycorrhizal Fungus Hebeloma Cylindrosporum in Coastal Sand Dune forest Ecosystems. Mol. Ecol. 6 (4), 353–364. doi:10.1046/j.1365-294X.1997.00200.x
Guo, M.-s., Ding, G.-d., Gao, G.-l., Zhang, Y., Cao, H.-y., and Ren, Y. (2020a). Community Composition of Ectomycorrhizal Fungi Associated with Pinus Sylvestris Var. Mongolica Plantations of Various Ages in the Horqin Sandy Land. Ecol. Indicators 110, 105860. doi:10.1016/j.ecolind.2019.105860
Guo, M., Gao, G., Ding, G., and Zhang, Y. (2020b). Drivers of Ectomycorrhizal Fungal Community Structure Associated with Pinus Sylvestris Var. Mongolica Differ at Regional vs. Local Spatial Scales in Northern China. Forests 11 (3), 323. doi:10.3390/f11030323
Han, Q., Huang, J., Long, D., Wang, X., and Liu, J. (2017). Diversity and Community Structure of Ectomycorrhizal Fungi Associated with Larix Chinensis across the alpine Treeline Ecotone of Taibai Mountain. Mycorrhiza 27, 487–497. doi:10.1007/s00572-017-0766-z
Hayward, J., Horton, T. R., and Nuñez, M. A. (2015). Ectomycorrhizal fungal communities coinvading with P inaceae host plants in A rgentina: G ringos bajo el bosque. New Phytol. 208, 497–506. doi:10.1111/nph.13453
Hu, Y.-L., Niu, Z.-X., Zeng, D.-H., and Wang, C.-Y. (2015). Soil Amendment Improves Tree Growth and Soil Carbon and Nitrogen Pools in Mongolian pine Plantations on post-mining Land in Northeast China. Land Degrad. Develop. 26, 807–812. doi:10.1002/ldr.2386
Jany, J.-L., Martin, F., and Garbaye, J. (2003). Respiration Activity of Ectomycorrhizas from Cenococcum Geophilum and Lactarius Sp. In Relation to Soil Water Potential in Five Beech Forests. Plant Soil 255, 487–494. doi:10.1023/A:1026092714340
John, M. K., and Matt, K. J. S. S. (1970). Colorimetric Determination of Phosphorus in Soil and Plant Materials with Ascorbic Acid. Soil Sci. 109, 214–220. doi:10.1097/00010694-197004000-00002
Jonard, M., Fürst, A., Verstraeten, A., Thimonier, A., Timmermann, V., Potočić, N., et al. (2014). Tree mineral Nutrition Is Deteriorating in Europe. Glob. Change Biol. 21, 418–430. doi:10.1111/gcb.12657
Kennedy, P. G., and Peay, K. G. (2007). Different Soil Moisture Conditions Change the Outcome of the Ectomycorrhizal Symbiosis between Rhizopogon Species and Pinus Muricata. Plant Soil 291, 155–165. doi:10.1007/s11104-006-9183-3
Kjøller, R., Nilsson, L. O., Hansen, K., Schmidt, I. K., Vesterdal, L., and Gundersen, P. (2012). Dramatic Changes in Ectomycorrhizal Community Composition, Root Tip Abundance and Mycelial Production along a Stand‐scale Nitrogen Deposition Gradient. New Phytol. 194, 278–286. doi:10.1111/j.1469-8137.2011.04041.x
Koizumi, T., Hattori, M., and Nara, K. (2018). Ectomycorrhizal Fungal Communities in alpine Relict Forests of Pinus Pumila on Mt. Norikura, Japan. Mycorrhiza 28, 129–145. doi:10.1007/s00572-017-0817-5
Kolaříková, Z., Kohout, P., Krüger, C., Janoušková, M., Mrnka, L., and Rydlová, J. (2017). Root-associated Fungal Communities along a Primary Succession on a Mine Spoil: Distinct Ecological Guilds Assemble Differently. Soil Biol. Biochem. 113, 143–152. doi:10.1016/j.soilbio.2017.06.004
Kutszegi, G., Siller, I., Dima, B., Takács, K., Merényi, Z., Varga, T., et al. (2015). Drivers of Macrofungal Species Composition in Temperate Forests, West Hungary: Functional Groups Compared. Fungal Ecol. 17, 69–83. doi:10.1016/j.funeco.2015.05.009
Lindahl, B. D., and Tunlid, A. (2014). Ectomycorrhizal Fungi - Potential Organic Matter Decomposers, yet Not Saprotrophs. New Phytol. 205, 1443–1447. doi:10.1111/nph.13201
Liu, Y., Bao, G., Song, H., Cai, Q., and Sun, J. (2009). Precipitation Reconstruction from Hailar pine (Pinus Sylvestris Var. Mongolica) Tree Rings in the Hailar Region, Inner Mongolia, China Back to 1865 AD. Palaeogeogr. Palaeoclimatol. Palaeoecol. 282 (1-4), 81–87. doi:10.1016/j.palaeo.2009.08.012
Liu, Z., Liu, Q., Wei, Z., Yu, X., Jia, G., and Jiang, J. (2021b). Partitioning Tree Water Usage into Storage and Transpiration in a Mixed forest. For. Ecosyst. 8, 72. doi:10.1186/s40663-021-00353-5
Liu, Z., Zhang, H., Yu, X., Jia, G., and Jiang, J. (2021a). Evidence of Foliar Water Uptake in a conifer Species. Agric. Water Manage. 255 (2), 106993. doi:10.1016/j.agwat.2021.106993
Mason, C. J., Edwards, M., Riby, P. G., and Coe, G. (1999). The Use of Microwaves in the Acceleration of Digestion and Colour Development in the Determination of Total Kjeldahl Nitrogen in Soil. Analyst 124 (11), 1719–1726. doi:10.1039/a903623g
Mason, P. A., Wilson, J., Last, F. T., and Walker, C. (1983). The Concept of Succession in Relation to the Spread of Sheathing Mycorrhizal Fungi on Inoculated Tree Seedlings Growing in Unsterile Soils. Plant Soil 71, 247–256. doi:10.1007/BF02182659
Miyamoto, Y., Sakai, A., Hattori, M., and Nara, K. (2015). Strong Effect of Climate on Ectomycorrhizal Fungal Composition: Evidence from Range Overlap between Two Mountains. ISME J. 9, 1870–1879. doi:10.1038/ismej.2015.8
Mosca, E., Montecchio, L., Sella, L., and Garbaye, J. (2007). Short-term Effect of Removing Tree Competition on the Ectomycorrhizal Status of a Declining Pedunculate oak forest (Quercus Robur L.). For. Ecol. Manage. 244 (1-3), 129–140. doi:10.1016/j.foreco.2007.04.019
Mujic, A. B., Hosaka, K., and Spatafora, J. W. (2014). Rhizopogon Togasawariana Sp. nov., the First Report of Rhizopogon Associated with an Asian Species of Pseudotsuga. Mycologia 106, 105–112. doi:10.3852/13-055
Peay, K. G., and Matheny, P. B. (2016). The Biogeography of Ectomycorrhizal Fungi - A History of Life in the Subterranean. New York, NY: John Wiley & Sons Ltd, 341–361. doi:10.1002/9781118951446.ch19
Policelli, N., Bruns, T. D., Vilgalys, R., and Nuñez, M. A. (2019). Suilloid Fungi as Global Drivers of pine Invasions. New Phytol. 222, 714–725. doi:10.1111/nph.15660
Ryberg, M., Nilsson, R. H., Kristiansson, E., Töpel, M., Jacobsson, S., and Larsson, E. (2008). Mining Metadata from Unidentified ITS Sequences in Genbank: a Case Study in Inocybe (Basidiomycota). BMC Evol. Biol. 8, 50. doi:10.1186/1471-2148-8-50
Sasse, J., Martinoia, E., and Northen, T. (2018). Feed Your Friends: Do Plant Exudates Shape the Root Microbiome? Trends Plant Sci. 23, 25–41. doi:10.1016/j.tplants.2017.09.003
Sebastiana, M., daSilva, A. B., Matos, A. R., Alcântara, A., Silvestre, S., and Malhó, R. (2018). Ectomycorrhizal Inoculation with Pisolithus Tinctorius Reduces Stress Induced by Drought in Cork Oak. Mycorrhiza 28, 247–258. doi:10.1007/s00572-018-0823-2
Song, H.-H., Yan, T., and Zeng, D.-H. (2019). Establishment of Mixed Plantations of Pinus Sylvestris Var. Mongolica and Populus × Xiaozhuanica May Not Be Appropriate: Evidence from Litter Decomposition. J. For. Res-Jap. 12, 857–870. doi:10.1093/jpe/rtz020
Song, L., Zhu, J., Li, M., Zhang, J., Zheng, X., and Wang, K. (2018). Canopy Transpiration of Pinus Sylvestris Var. Mongolica in a Sparse wood Grassland in the Semiarid sandy Region of Northeast China. Agric. For. Meteorology 250, 192–201. doi:10.1016/j.agrformet.2017.12.260
Soudzilovskaia, N. A., Douma, J. C., Akhmetzhanova, A. A., van Bodegom, P. M., Cornwell, W. K., Moens, E. J., et al. (2015). Global Patterns of Plant Root Colonization Intensity by Mycorrhizal Fungi Explained by Climate and Soil Chemistry. Glob. Ecol. Biogeogr. 24, 371–382. doi:10.1111/geb.12272
Spake, R., van der Linde, S., Newton, A. C., Suz, L. M., Bidartondo, M. I., and Doncaster, C. P. (2016). Similar Biodiversity of Ectomycorrhizal Fungi in Set-Aside Plantations and Ancient Old-Growth Broadleaved Forests. Biol. Conservation 194, 71–79. doi:10.1016/j.biocon.2015.12.003
Steidinger, B. S., Bhatnagar, J. M., Vilgalys, R., Taylor, J. W., Qin, C., Zhu, K., et al. (2020). Ectomycorrhizal Fungal Diversity Predicted to Substantially Decline Due to Climate Changes in north American Pinaceae Forests. J. Biogeogr. 47, 772–782. doi:10.1111/jbi.13802
Taylor, D. L., and Bruns, T. D. (1999). Community Structure of Ectomycorrhizal Fungi in a Pinus Muricata forest: Minimal Overlap between the Mature forest and Resistant Propagule Communities. Mol. Ecol. 8, 1837–1850. doi:10.1046/j.1365-294x.1999.00773.x
Tedersoo, L., Bahram, M., Toots, M., Diédhiou, A. G., Henkel, T. W., Kjøller, R., et al. (2012). Towards Global Patterns in the Diversity and Community Structure of Ectomycorrhizal Fungi. Mol. Ecol. 21, 4160–4170. doi:10.1111/j.1365-294X.2012.05602.x
Tedersoo, L., Bahram, M., and Zobel, M. (2020). How Mycorrhizal Associations Drive Plant Population and Community Biology. Science 367 (6480), eaba1223. doi:10.1126/science.aba1223
Truong, C., Gabbarini, L. A., Corrales, A., Mujic, A. B., Escobar, J. M., Moretto, A., et al. (2019). Ectomycorrhizal Fungi and Soil Enzymes Exhibit Contrasting Patterns along Elevation Gradients in Southern Patagonia. New Phytol. 222, 1936–1950. doi:10.1111/nph.15714
van der Linde, S., Suz, L. M., Orme, C. D. L., Cox, F., Andreae, H., Asi, E., et al. (2018). Environment and Host as Large-Scale Controls of Ectomycorrhizal Fungi. Nature 558, 243–248. doi:10.1038/s41586-018-0189-9
van Dorp, C. H., Beiler, K. J., and Durall, D. M. (2016). Dominance of a Rhizopogon Sister Species Corresponds to forest Age Structure. Mycorrhiza 26, 169–175. doi:10.1007/s00572-015-0660-5
Walkley, A., and Black, I. A. (1934). An Examination of the Degtjareff Method for Determining Soil Organic Matter, and a Proposed Modification of the Chromic Acid Titration Method. Soil Sci. 37 (1), 29–38. doi:10.1097/00010694-193401000-00003
Wang, D. D., Zhao, W., Reyila, M., Huang, K. C., Liu, S., and Cui, B. K. (2020). Diversity of Microbial Communities of Pinus Sylvestris Var. Mongolica at Spatial Scale. Microorganisms 10, 371. doi:10.3390/microorganisms10020371
Wang, Y.-L., Zhang, X., Xu, Y., Babalola, B. J., Xiang, S.-M., Zhao, Y.-L., et al. (2021). Fungal Diversity and Community Assembly of Ectomycorrhizal Fungi Associated with Five pine Species in Inner mongolia, china. Front. Microbiol. 12, 646821. doi:10.3389/fmicb.2021.646821
Yang, W., Zhang, D., Cai, X., Xia, L., Luo, Y., Cheng, X., et al. (2019). Significant Alterations in Soil Fungal Communities along a Chronosequence of Spartina Alterniflora Invasion in a Chinese Yellow Sea Coastal Wetland. Sci. Total Environ. 693, 133548. doi:10.1016/j.scitotenv.2019.07.354
Yang, Y., Zhang, X., Hartley, I. P., Dungait, J. A. J., Wen, X., Li, D., et al. (2021). Contrasting Rhizosphere Soil Nutrient Economy of Plants Associated with Arbuscular Mycorrhizal and Ectomycorrhizal Fungi in Karst Forests. Plant Soil 470, 81–93. doi:10.1007/s11104-021-04950-9
Zak, D. R., Pellitier, P. T., Argiroff, W., Castillo, B., James, T. Y., Nave, L. E., et al. (2019). Exploring the Role of Ectomycorrhizal Fungi in Soil Carbon Dynamics. New Phytol. 223, 33–39. doi:10.1111/nph.15679
Zhang, W., Yuan, Y., Yang, S., Huang, J., and Huang, L. (2015). ITS2 Secondary Structure Improves Discrimination between Medicinal “Mu Tong” Species when Using DNA Barcoding. PLoS ONE 10, e0131185. doi:10.1371/journal.pone.0131185
Zhang, Y., Cao, H., Zhao, P., Wei, X., Ding, G., Gao, G., et al. (2021). Vegetation Restoration Alters Fungal Community Composition and Functional Groups in a Desert Ecosystem. Front. Environ. Sci. 9, 589068. doi:10.3389/fenvs.2021.589068
Zhao, P. s., Guo, M. s., Gao, G. l., Zhang, Y., Ding, G. d., Ren, Y., et al. (2020). Community Structure and Functional Group of Root‐associated Fungi of Pinus Sylvestris Var. Mongolica across Stand Ages in the Mu Us Desert. Ecol. Evol. 10 (6), 3032–3042. doi:10.1002/ece3.6119
Keywords: ectomycorrhizal fungi, Pinus sylvestris var. mongolica, stand age, edaphic factor, diversity and community composition
Citation: Ren Y, Guo M, Ding G and Wang Y (2022) Ectomycorrhizal Fungi Associated With Pinus sylvestris var. mongolica Were Altered by Soil Environments With Aging Plantation in a Semi-arid Desert. Front. Environ. Sci. 10:858452. doi: 10.3389/fenvs.2022.858452
Received: 20 January 2022; Accepted: 17 March 2022;
Published: 27 April 2022.
Edited by:
Shengbo Xie, Northwest Institute of Eco-Environment and Resources (CAS), ChinaReviewed by:
Yuling Li, Agricultural University of Hebei, ChinaDang Xiaohong, Inner Mongolia Agricultural University, China
Copyright © 2022 Ren, Guo, Ding and Wang. This is an open-access article distributed under the terms of the Creative Commons Attribution License (CC BY). The use, distribution or reproduction in other forums is permitted, provided the original author(s) and the copyright owner(s) are credited and that the original publication in this journal is cited, in accordance with accepted academic practice. No use, distribution or reproduction is permitted which does not comply with these terms.
*Correspondence: Guodong Ding, dingguodong@bjfu.edu.cn