- 1Sustainable Agroecosystems, Department of Environmental Systems Science, ETH Zurich, Zurich, Switzerland
- 2GreenGrass Ltd., Budapest, Hungary
- 3Institute of Geography and Earth Sciences, University of Pécs, Pécs, Hungary
Diversified farming systems are promoted to improve ecosystem services in agriculture while maintaining productivity. Intercropping could improve soil quality, the stability of yields and climate resilience. Whether direct emissions of greenhouse gases from soil are reduced as well, depends on the specific measures of diversification. Here, we determined the greenhouse gas emissions from soils of two diversification experiments in the Pannonian climate of Hungary. Firstly, in an asparagus field, oat and field pea was introduced as intercrop between the asparagus berms. Secondly, grass and aromatic herbs were intercropped in a vineyard between the grape rows. The results show that especially for nitrous oxide, average treatment emissions can increase with additional legumes (+252% with intercropped field peas) but decrease with aromatic herbs (−66%). No significant changes were found for methane exchange. This shows that, while other ecosystem services can be increased by intercropping, changes in soil greenhouse gas emissions by intercropping are highly context dependent.
1 Introduction
The intensification of agricultural practices has resulted in soil degradation, reduced biodiversity and increased economic risk for European farmers (Griffiths et al., 2016). Intense mechanization, excessive use of fertilizer (Zhang et al., 2021) and pesticides are the dominant features of modern monocultures; both in time and space the same species of plant is grown on arable fields to improve labor efficiency. In view of the socioeconomic and environmental problems arising from high-input systems, there is now a growing emphasis on crop diversification for an optimized use of resources (Wezel et al., 2014; Beillouin et al., 2021). A number of EU Horizon 2020 projects are addressing the challenge to reverse the dominance of monocultures by reintroducing diversity to agricultural systems: the crop diversity cluster (https://www.cropdiversification.eu/). One of these projects, DiverFarming, is focusing on stakeholder engagement to promote rural development while scientifically assessing measures taken by farmers. Practical diversification strategies are tested on their effects on productivity and ecosystem services such as carbon sequestration, reduced greenhouse gas (GHG) emissions, soil quality and fertility, erosion and contamination prevention. Diversification aims for an improvement of all ecosystem services, but there can be trade-offs that may hamper the provision of a certain service for the benefit of others (Tamburini et al., 2020). Especially changes in nitrous oxide (N2O) and methane (CH4) emissions from soil can be indirect effects of diversified management regimes. These effects are difficult to anticipate in advance and its measurement efforts are very high (Decock et al., 2015). N2O production in soil can be changed by reduced nitrogen availability in soil when intercropping increases the plant covered soil surface (Davidson et al., 2000). Increased plant cover in time or space could also lead to changes in CH4 oxidation due to root gas transport (Watson et al., 1997) and/or root exudates (Waldo et al., 2019). Additional plant growth is also expected to decrease soil moisture, increase soil organic carbon and increase water holding capacity, all of which have the potential to enhance methanotrophs in soils (Tiwari et al., 2018). An added value of diversification can be an increase of legumes in the field by inter-, cover- or mixed cropping that increases natural nitrogen fixation and soil fertility in general (Stagnari et al., 2017). This additional nitrogen, on the other hand, could increase losses in the form of N2O at certain points in time (Pappa et al., 2011). In addition, increased plant-derived dissolved organic matter can increase N2O emissions from soils (Qiu et al., 2015). However, there is evidence that the addition of legumes can mitigate N2O emission from permanent grasslands (Fuchs et al., 2018). Process based models seem to disagree on the magnitude of the effect (Fuchs et al., 2020). Especially in complex diversification treatments, there is a need to measure the emissions in the field that can be used to verify model output.
In this paper, we report on the greenhouse gas emissions from two case studies in Hungary, where innovative diversification treatments are applied on an asparagus field and a vineyard. Hungarian agriculture is especially in need of adapting diversified farming practices. In our first case study, the asparagus field is challenged by high erosion losses of its sandy soil. Diversification is a key strategy to reduce the loss of soil fertility and organic carbon through wind erosion (Elliott and Chevalier, 1996). Such soil losses are especially pronounced with crops that need an intensive soil tillage treatment, like when asparagus ridges are created. The second case study (CS11) tackles water erosion on vineyards (Rodrigo Comino et al., 2016) by increaseing soil cover (Dittrich et al., 2021). Alternatively to bare soil between grape rows, the winegrower in Villany grew an aromatic herb (Achillea millefolium) and mixed grass vegetation. These two distinct examples of diversification will add to the general discussion of how the effects on GHG emissions from soil can be assessed and what mechanisms lead to positive or negative leaking that adds to the overall environmental impact of crop diversification.
2 Materials and Methods
2.1 Field Sites
Diverfarming cooperates with farmers to implement diversification on farm experiments to measure the effects on ecosystem services including productivity and greenhouse gas emissions. In the two case studies within the Pannonian climate zone test diversifications that have been designed by the farmer and researchers together. We measured greenhouse gas emissions, mainly N2O and CH4, to better understand the consequences of the changed management for diversification. The pannonian case studies consist of two different cropping systems that were diversified according to the goals of Diverfarming and the needs of the local farmer. At both sites, data on meteorological parameters, yields, soil and greenhouse gases were collected for three cropping seasons.
2.1.1 Case Study 10-Jakabszállás
The Diverfarming experimental site CS10 (coordinates: 46°44′52.6″N; 19°34′25.7″E) lies in Central Hungary, in the vicinity of the village Jakabszállás. The soil is a Humic Arenosol on a Pleistocene alluvial fan of the Danube formed by wind blown sand, which has a uniform particle size distribution (median at 198 μm) (Figure 1) and is poor in nutrients (Ntotal: 0.3 g kg−1, Pavailable: 110 mg kg−1). The climate is continental, close to semiarid with mean annual temperature of 10.2°C and average annual precipitation of about 550 mm with frequent extremes. Annual potential evaporation amounts to 848 mm (meteo data from the Hungarian Meteorological Service, OMSz).
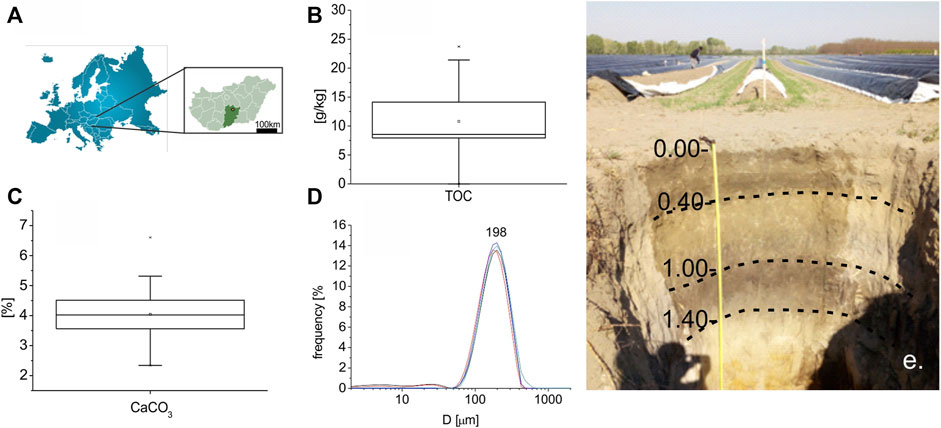
FIGURE 1. Asparagus field with soil profile and topsoil properties. (A), location; (B), total organic carbon; (C), carbonate content; (D), particle size distribution of the top layer; (E), soil profile (0–0.40 m: cultivated, 0.4–1.00 arenic, 1.00–1.40 buried organic, > 1.40 plinthic).
The asparagus field is used for intercropping in the 1.8 m wide space between the asparagus ridges (Figure 2). Pea and oat are chosen as intercrops to contrast the conventional bare soil area. Both intercrops are primarily used for green manure and partially as catch crops for animal feed. These species were chosen because they are widely grown in this pedoclimatic region and they have distinct agricultural features; field pea is a leguminous plant and has a high P and K content, whereas oat is also nutrient-rich but in contrast very tolerant to bad weather conditions and low soil fertility. The experimental design includes three replicates for each diversification treatment and the control in three blocks (alternating block design, Figure 2). Two chambers for GHG sampling were placed between the asparagus ridges on each replicate at the distance of 50 and 100 m from the field border. More information and results on the experiment including first soil quality results are provided in Rezsek et al. (2019).
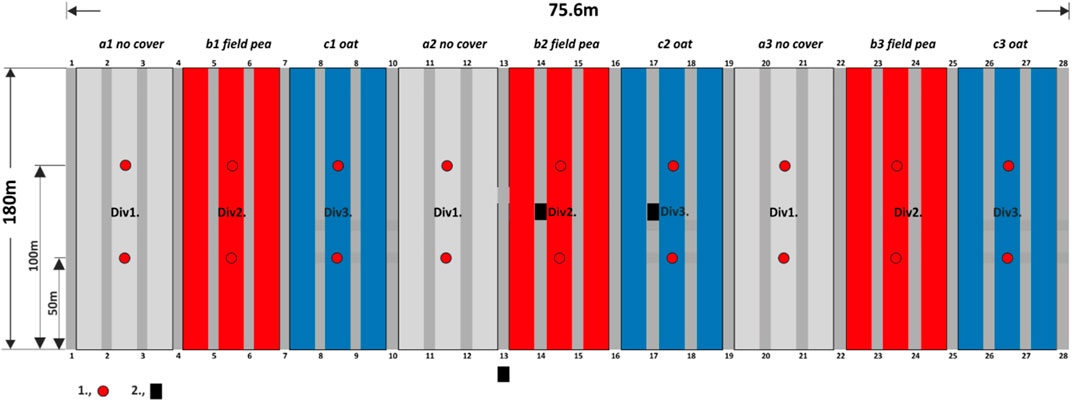
FIGURE 2. Experimental plot design of CS10 1: ,chambers, 2, in situ loggers for environmental parameters.
The field was intensively managed with several chemical plant protection substances (i.e., herbicide metribuzin and fungicides: difenokonasol, azoxistobin, tebukonazol and metriram). Fertilization was done (kg-N/ha in brackets) in 2018 on June 7th (69) and August 31st (72), 2019 on June 13th (63), October 7th (63) and in 2020 on June 15th (72), September 17th (5) and 24th (33). In total this was 340 kg-N/ha during the three growing seasons and the time of GHG measurements. Also the soil management was intensive, forming the ridges for the asparagus, covering them with plastic foil or disking for weed management.
2.1.2 Case Study 11—Villány
Case study number 11 lies in the Villány Wine District, Baranya County, Southwest-Hungary (coordinates: 45°51′47.8″N, 18°26′39.6″E, Figure 3). Mean annual temperature is 10.7°C (1981–2010) and average annual precipitation is 680 mm. The plantation has been cultivated for several centuries on southern slopes of 15–20° inclination with loamy, slightly calcareous and compacted Ramann’s brown forest soil (WRB: Chromic Cambisol). At the experimental plot, soil depth is 1.7–2.0 m. The average soil carbon content is 3.36% (SD 0.36), total carbon content is 25.96 g kg−1 and C/N ratio is 19 (Figure 4). The site has a high susceptibility for water erosion due to the hill slopes.
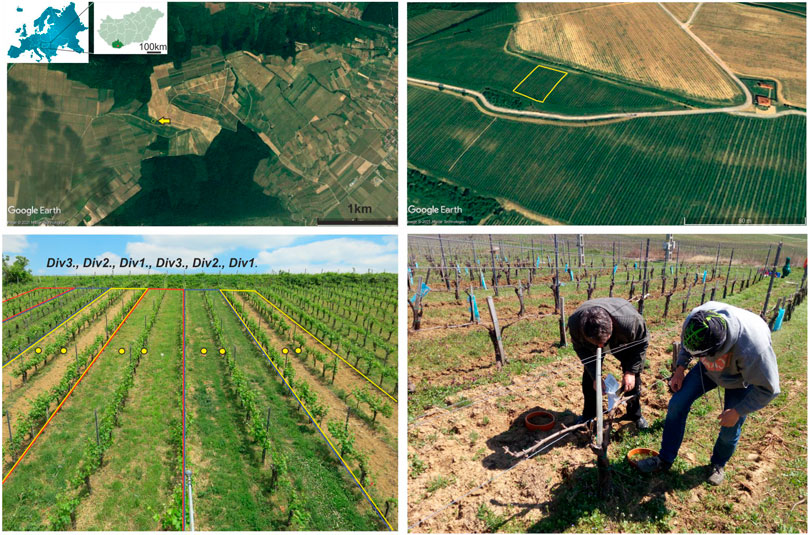
FIGURE 3. Villány, location of CS11 area (A,B), a part of treatments and place of the chambers, (C) setting chambers (D).
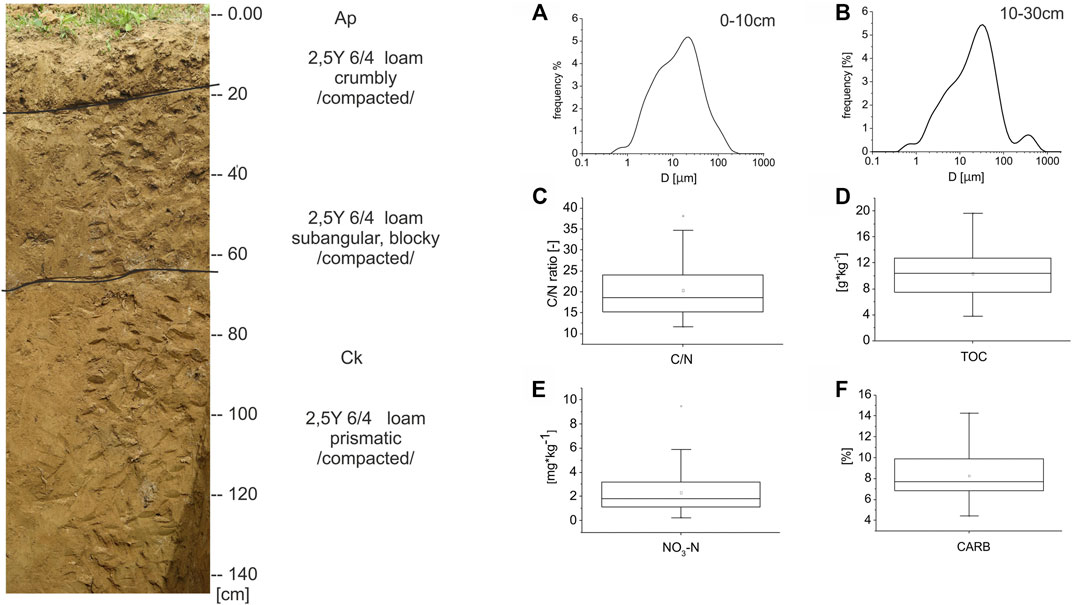
FIGURE 4. Villány, general soil profile and main important properties of the soil (data from last experimental year (2020) (A). characteristic PSD distribution of 0–10 cm and (B) 10–30 cm layer; (C):, C/N ratio; d: TOC (g kg−1); (E): NO3-N (mg kg−1); (F): carbonate content (%).
The alleys between the rows of grapes were diversified with firstly the aromatic herb Achillea millefolium (Yarrow) and the originally growing mixture of grasses. The control treatment without soil cover was treated with disks and cultivators twice in the beginning of each year, when stems were also mulched. The diversified treatments were mowed and left on the field in the alley 2–4 times during the year depending on plant growth. Figure 5 shows the experimental design of the vineyard with its two alleys between the grapes of 40 m length (north-south) per replicate where the diversification was applied. Hence, each replicate consists of a grape row that has the same diversification on both sides with a row of grapes in between the different treatments. Each treatment was replicated three times including 18 alleys of total width of 44 m (east-west).The three alternating replicates of each treatment form one block, that will also be used to analyse block effects in the field (aligned from left to right). Two chambers for GHG sampling per replicate were installed, one in the grape row between the plants and one in the alley between the grape rows (i.e., vines vs. tractor row). These two positions were analysed as additional fixed factor in the statistical model.
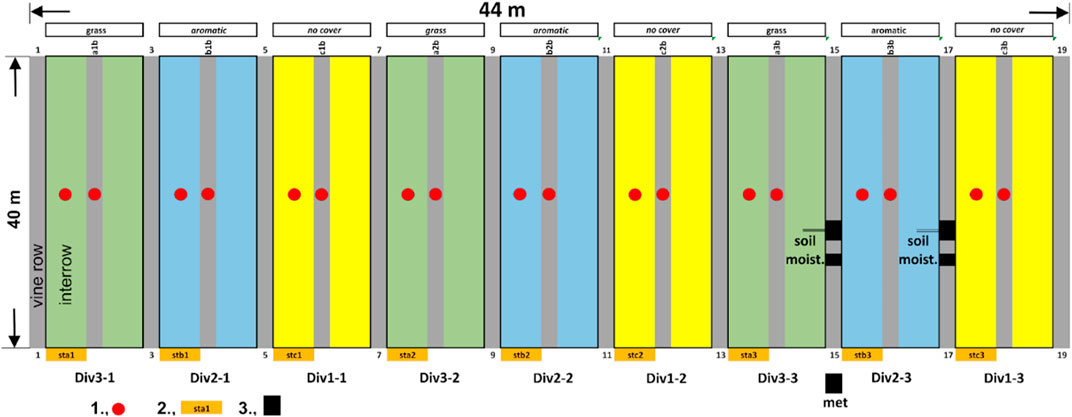
FIGURE 5. Villány CS11 diversifications: green: grass; blue: aromatic herb (Achillea millefolium); yellow: uncovered; 1: chambers; 2 sediment traps; 3: stations for environmental parameters (d).
Numerous organic farming approved substances are used to protect the grapes from diseases: Copernico, Nectaflor P, Wetcit, Kendal TE, Fitokondi, Mélius, Kumulus S, Sergomil-L 60, Organit, Polyversum, Vitisan, and FlavoPlant. There was no specific fertilizer applied but the mulching of the stems and plant material from the alleys can be considered as recycling input of nutrients.
2.2 Greenhouse Gas Sampling and Analysis
The three greenhouse gases carbon dioxide (CO2), nitrous oxide (N2O) and methane (CH4) were collected from static dark chambers of 25 cm diameter and height of 5 cm. The rims were permanently inserted into the soil to a depth that the headspace of the chamber is 5 cm high, leading to a chamber volume of 2.45 L. For the sampling, a lid with a vent tube and a sampling port was put on top. Four gas samples of 20 ml were taken at time 0, 10, 20, and 30 min into a pre-evacuated 12 ml Labco vial capped by a rubber septa. The glass vials were shipped to Zurich and measured on an Scion 456-GC (Bruker, Germany) gas chromatograph equipped with a thermal conductivity detector for CO2, an electron capture detector for N2O and a flame ionization detector for CH4. Gas fluxes were calculated by the (Fuß, 2017) using a combination of linear and non-linear flux estimates based on the measurement uncertainty and the flux size (Hüppi et al., 2018). From the total 3′363 N2O fluxes, 2′467 were evaluated by the robust-linear regression, 17 by standard linear regression (only 3 valid concentration measurements per chamber) and in 878 cases the non-linear HMR regression was applied. This shows that the chamber volume was appropriate since saturation effects were rarely observed from the low ratio of non-linear vs. linear cases (in roughly 1/4 of cases). By allowing for non-linear flux estimates, the average flux of the whole dataset was increased by 14.5%. Respecting the precision of the gas chromatograph and the performance of the chamber system the minimum detectable flux results in 2.49 μg N2O m−2h−1 and 8.16 μg CH4 m−2h−1(shiny app “minflxlim” 2020).
The sampling interval on the field was adjusted to the expected emissions during the growing seasons. In winter, the sampling frequency was reduced (winter break 1–2 months) from the normal bi-weekly sampling, whereas after fertilisation and soil management, the sampling was increased up to daily measurements. Aggregated emissions were calculated using linear interpolation of the daily fluxes (Fuß, 2017).
2.3 Statistical Analysis
For the analysis of N2O flux data on the significance of the treatment effect, the data was first log transformed to improve normal distribution. A linear mixed effect model was used to test the effect on N2O emissions and CH4 exchange from diversification treatment and block as fixed effect and the chamber number as random effect. The chamber number as random effect reflects the repeated measures during the sampling period at the same site (chamber) in the field. For CS11 position was an additional fixed effect controlling for within or between grape rows. The model was controled to have a variance inflation factor below three. The aggregated emission boxplot compare the treatments in a pairwise t-test and indicate significance at * (p < 0.05), ** (p < 0.01) or N.S (not significant, p > 0.05). The pairwise t-test was used in the figures on aggregated emissions per treatment.
3 Results
3.1 Case Study 10
3.1.1 Nitrous Oxide
The major N2O emission peaks each year in June are well resolved (Figure 6) due to a higher sampling frequency whereas outside the growing season the sampling was reduced. The large peaks from the rows in between the asparagus berm, coincide with fertilisation of 70 kg N ha−1 mid May in 2020, early June in 2018 and mid June in 2019. Smaller peaks could be observed in May and September, which could be due to soil management and fertilization respectively.
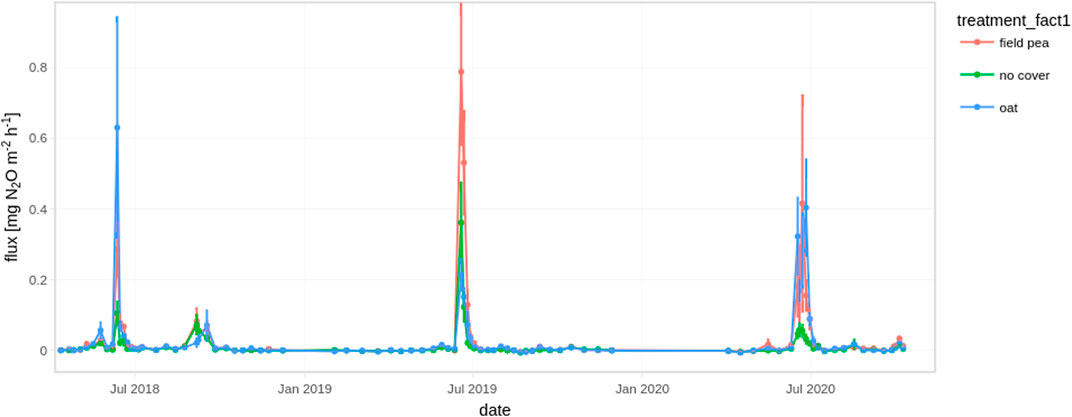
FIGURE 6. N2O emissions of CS10 during the three growing seasons (2018–2020) averaged by the diversification treatment (field pea, oat and no cover as control) with error bars indicating 1 standard error.
The control plots show the lowest N2O emissions in the asparagus field (Figure 7). There is a significant reduction of cumulated N2O emissions in the bare soil treatment, especially in contrast to field pea in the asparagus field. The linear mixed effect model indicates a significant effect of the diversification treatment (p = 0.007). Hence, field pea increased N2O emissions in comparison to bare soil to 252% over the 3 years of data. The oat treatment shows higher emissions than the control as well (211%) but without statistical significance. The average emission factor of the applied 340 kg N ha−1 is 0.54% (hence 1.8 kg N2O − N ha−1).
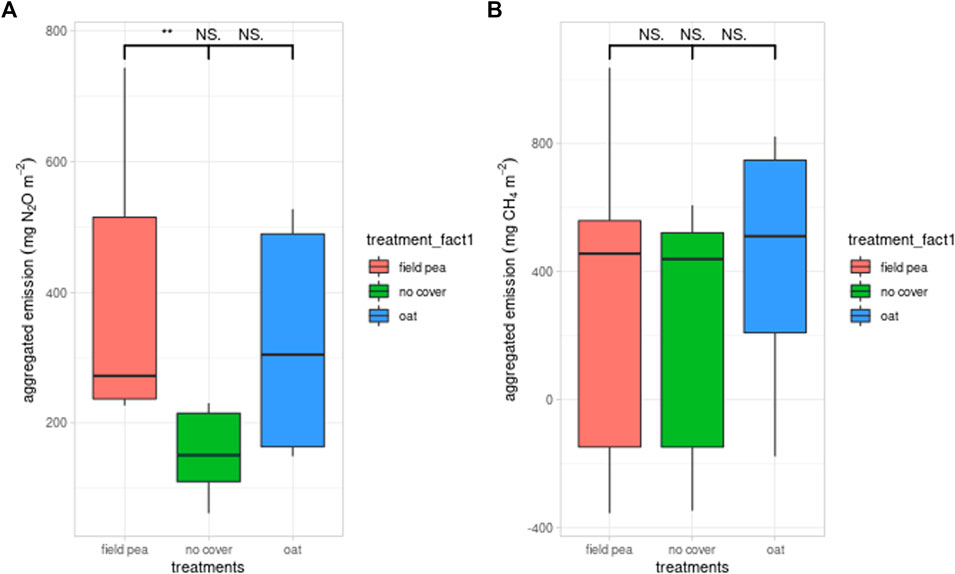
FIGURE 7. Boxplots of the aggregated N2O (A) and CH4 (B) exchange from CS10 by the diversification treatment; field pea, no cover(control) and oats.
3.1.2 Methane
Most methane measurements show an uptake by the soil (Figure 8). Only on September 27 in 2020 there was a large emission event in all treatments that dominated the aggregated methane balance over the whole measurement period. The average emission per treatment on this day was 6.2, 5.0, and 4.3 mg CH4 m−2h−1 in oat, field pea and no cover respectively (Figure 7). Including this special event in the aggregated emissions, turns them into an overall emission of about 500 mg CH4 m−2 without significant difference between the treatments. If this extraordinary event is omitted from the cumulative flux calculation, there is an uptake of roughly 360 mg CH4 m−2 with a significant effect of the diversification treatment (p = 0.007). In this case the oats had an increased CH4 uptake by roughly 9%, however the high emission event then masked this effect in the overall dataset.
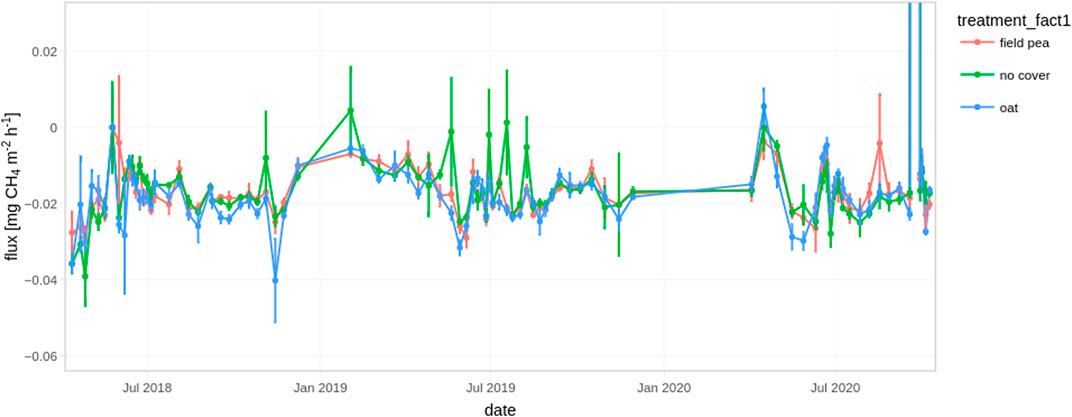
FIGURE 8. CH4 emissions of CS10 during the three growing seasons (2018–2020) averaged by the diversification treatment (field pea, oat and no cover as control) with error bars indicating 1 standard error.
3.2 Case Study 11
3.2.1 Nitrous Oxide
N2O measurements in CS11 show rather small fluxes, mostly below 0.1 mg N2O m−2h−1, with larger emission events only in the beginning until July 2018 (2 events 0.1 mg N2O m−2h−1) followed by culivator activity and mulching of the stems in early spring (Figure 9). The diversification treatment shows a significant difference in the linear mixed model (p = 0.0022) over the whole measurement period, but also after the initial large emissions in 2018 (p = 0.065). Aggregated emissions show a significant reduction by aromatic herbs compared to bare soil of 66% over the 3 years of data (Figure 11). In addition to the diversification treatment also the block (p = 0.009) and the position (p = 0.0012) of the chamber within the replicate (i.e., vines vs. tractor row) had a significant effect on N2O emissions.
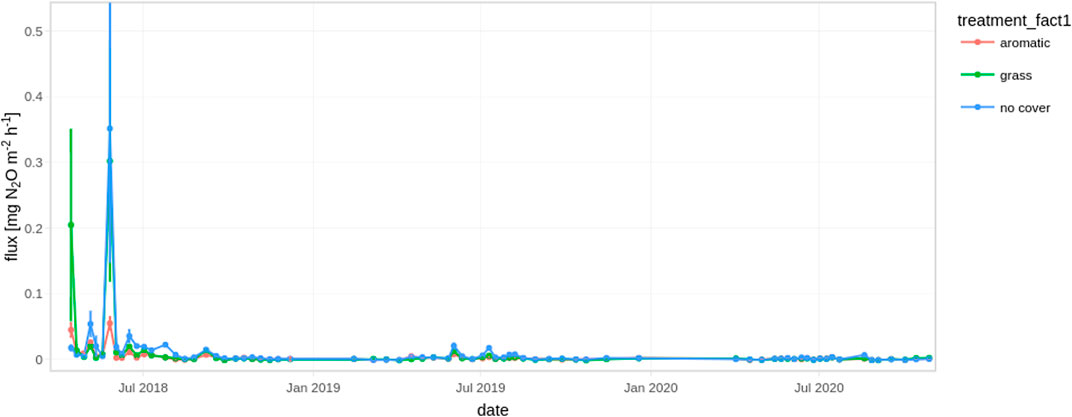
FIGURE 9. N2O emissions of CS11 during the three growing seasons (2018–2020) averaged by the diversification treatment (aromatic herbs, grass and no cover as control) with error bars indicating 1 standard error.
3.2.2 Methane
In general, methane was taken up by the soil with minor seasonal variations (Figure 10). There are no differences between the treatments but just a possible minor tendency for reduced methane oxidation by the uncovered treatment (Figure 11).
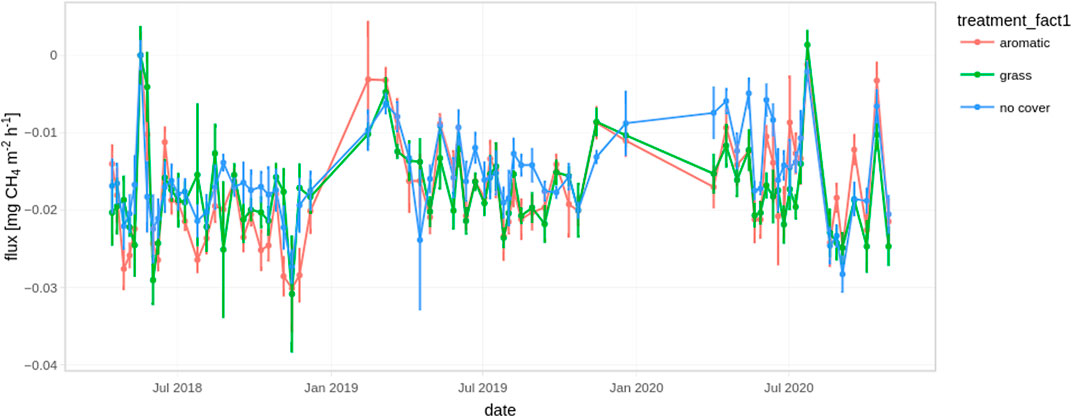
FIGURE 10. CH4 emissions of CS11 during the three growing seasons (2018–2020) averaged by the diversification treatment (aromatic herbs, grass and no cover as control) with error bars indicating 1 standard error.
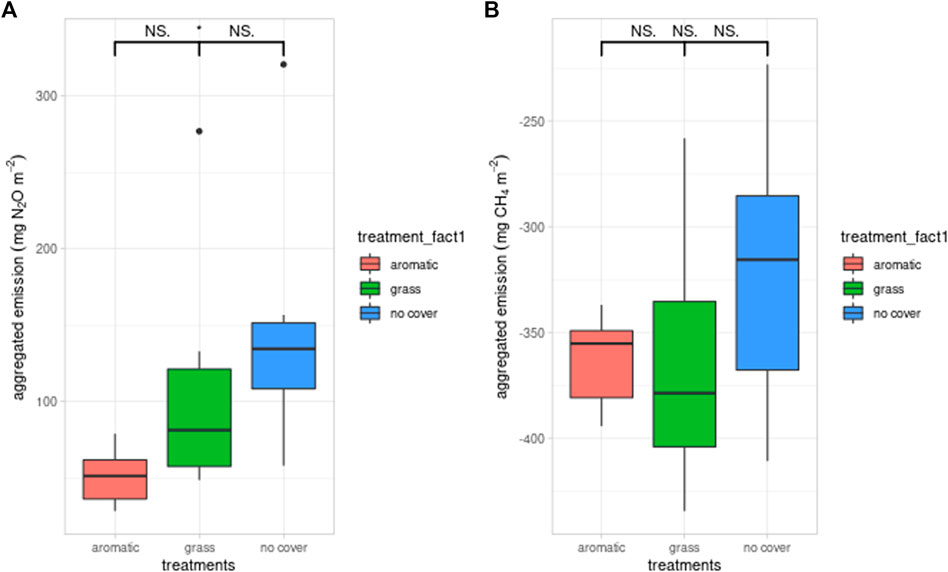
FIGURE 11. Boxplots of the aggregated N2O (A) and CH4 (B) exchange from CS11 by the diversification treatment; aromatic herbs, grass and no cover (control).
3.3 Soil Moisture and Temperature Data
Soil moisture and temperature in the soil show differences between the 3 years of data (Figures 12, 13). Whereas the summer in 2018 and 2020 was rather dry, 2019 had lots of precipitation. The sensor setup did not allow for differentiation between the treatments.
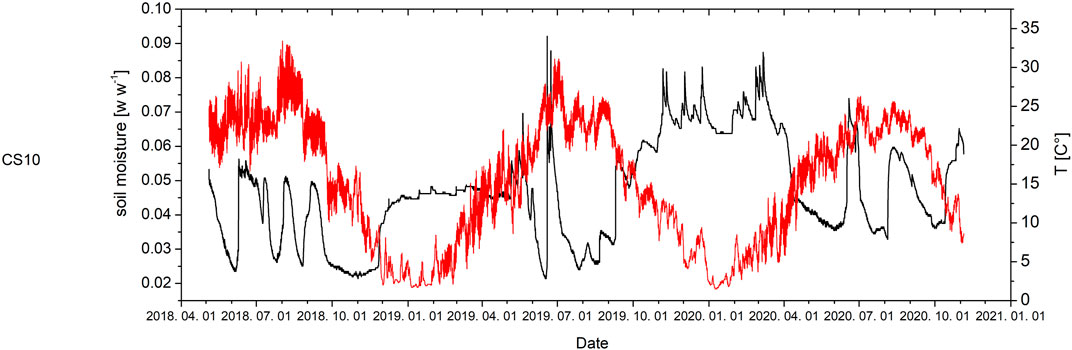
FIGURE 12. Temperature (red line, right axis in °C) and soil moisture (black line, left axis in VWC m3/m3) of CS 10 during the three growing seasons (%).
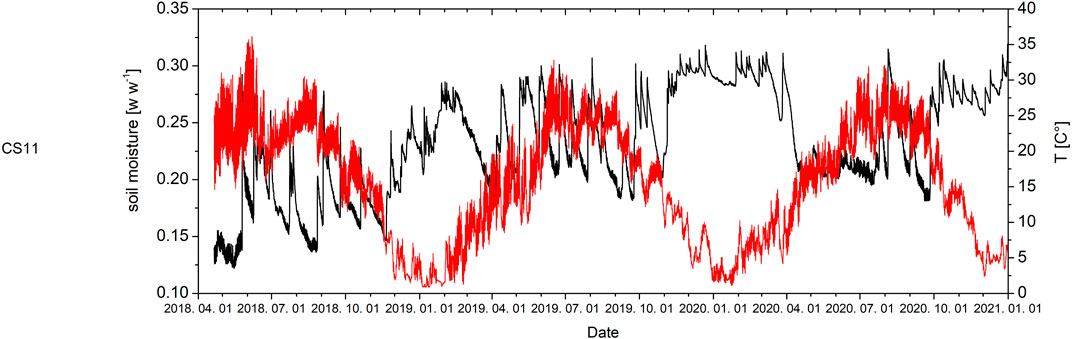
FIGURE 13. Temperature (red line, right axis in °C) and soil moisture (black line, left axis in VWC m3/m3) of CS 11 during the three growing seasons (%).
4 Discussion
With the large variety of diversifications applicable in European agriculture, the impact on GHG emissions is as diverse. There is no general hypothesis that with increased diversity of plants on the field, GHG emissions are lower. But there are opportunities for reduced N2O emissions especially, with the appropriate rate and timing of organic input management (Saha et al., 2021). However, a meta analysis about the effects of crop diversification on ecosystem services, showed that there is a trend for increased GHG emissions (Beillouin et al., 2021). They state that climate impacts of crop diversification are variable and context-dependent.
With respect to N2O emissions, decreased emissions can be expected due to reduced availability of mineral nitrogen from the increased plant cover (Davidson et al., 2000) and more balanced nitrogen supply with added legumes especially in grasslands (Fuchs et al., 2020). Cover cropping is a more specific example that according to a recent review publication decreases nitrogen leaching and increases carbon sequestration, but does not have a significant effect on N2O emissions over the whole reviewed data set (Abdalla et al., 2019). Our data supports reduced emissions from increased soil cover in CS11, where no additional fertilizer was applied in the inter row section of the vineyard. Although there is no significant difference in the pairwise comparison, the grass reduced N2O emissions less than herbs, possibly because in the grass mixture also some leguminous clovers were estabilshed. Alternatively, aromatic plants are known to produce substances that inhibit nitrification in soil, hence this could improve nitrogen efficiency and reduce N2O emissions (Kiran and Patra, 2003). Another study supports our finding by showing a 60% decrease in N2O emissions between cover cropping and tillage in a mediterranean vineyard (Marques et al., 2018). A similar vineyard field experiment also within Diverfarming showed that nitrate content did not change with aromatic herbs but ammonium was reduced, indicating that these plants impact nitrogen transformation in soil (Dittrich et al., 2021). Garland et al. (2011) looked into the effect of different management practices on N2O emissions from a Mediterranean vineyard and found fertilization and cover cropping had a larger impact than till or no-till soil management. They also highlighted the importance of measuring GHG emissions at different functional locations within a vineyard (i.e., vines and tractor row) because these different sites have distinct emission patterns. In CS11 we also differentiated between below vine row and interrows, but although significant, the effect of the position did not contrast the diversification effect.
In contrast, CS10 shows significantly increased emissions from leguminous field peas and just a tendency for increased emissions with oat compared to the uncovered soil. Although an increase in N2O emissions can be expected from increased nitrogen inputs by legumes, our results are not in agreement with studies that actually showed decreased N2O emissions from leguminous intercrops (Pappa et al., 2011; Senbayram et al., 2016). Carter and Ambus (2006) showed that biologically fixed N2 is a minor source for N2O in a grass-clover mixture by 15N tracers. Compared to the mulched annual field pea in our case, the perennial clover may not release as much nitrogen since the resulting N input from avoided root decomposition is less. Still our results of increased emissions in contrast to bare soil are not fully explained by just increased N content from field peas. Especially since oats also have a tendency for increased emissions. In our case an increase in plant-derived dissolved organic matter by the additional plants growing may have increased N2O emissions from soil (Qiu et al., 2015). Nevertheless, another study did show increased N2O emissions from non-legume cover crops in the absence of the growing season (Thomas et al., 2017). Although our measurements are increased mainly during the growing season and especially at the leguminous treatment, the suggested increase in N2O fluxes may still be related to increased substrate concentration for denitrification due to higher amounts of root-associated soil with cover crops. Nevertheless, the main emissions occur after the fertilization events in CS10. Mulching of the intercrops could lead to high N2O emissions and create a contrast to the bare soil situation where no plant material is decomposed (Garland et al., 2011; Saha et al., 2021). A meta analysis on the effect of cover crops on N2O emissions supports the finding that leguminous cover crops and incorporation thereof into soil tend to increase emissions (Basche et al., 2014).
Methane emissions did hardly change in diversification treatments. While there is a lot of research on how vegetation affects timing and location of methane emissions from wetlands, there is very little known on plant interactions with oxic, mineral soils. An increase in plant cover and root exudation would however rather increase methane emissions from soils (Waldo et al., 2019). In contrast we found that in the asparagus field (CS10), the plants tend to increase methane oxidation, especially oats significantly increased methane uptake compared to the bare soil. A reason for this might be the increased oxidation by methanotrophic bacteria in the upper aerated soil layer, leading to higher uptake of methane by the sandy soil. Nitrogen limitation is known to reduce the growth of methanotrophic bacteria in soils (Bodelier and Laanbroek, 2004). The intercrops could probably help retain nitrogen in soil that was then still available for methane oxidation in CS10. Methanotroph bacteria abundance is also enhanced with increased soil moisture, organic carbon content and water holding capacity (Tiwari et al., 2018). When considering intercropping, especially in contrast to bare soil, these soil properties are improved, which would lead to an increased methane oxidation potential. Such a positive side effect of increasing plant cover in diversified cropping systems can lead to considerable improvements of the GHG balance.
5 Conclusion
This study shows the importance of monitoring GHG emissions in complex diversification experiments. While mostly ecosystem services are improved by increasing diversity in farming systems, non-CO2 GHG emissions from soil can be both increased and decreased. However the extent of change is not necessarily high and it can be very cost and time consuming to monitor. There are general mechanisms that help to understand the impact of diversification via change in plant cover and consequtive effets on nutrient availability or soil moisture. The addition of leguminous intercrops can increase nitrous oxide emissions whereas the addition of herbs to uncovered interrows significantly decrease N2O emissions. Methane on the other hand is less affected by the diversification measures but its oxidation is influenced by an increased plant cover due to increased soil aeration and change in nitrogen availability.
Data Availability Statement
The datasets presented in this study can be found in online repositories. The names of the repository/repositories and accession number(s) can be found below: https://zenodo.org/record/6369860
Author Contributions
RH, JD, and JS contributed to conception and design of the study. RH and JS organized the data evaluation. RH performed the statistical analysis. RH wrote the first draft of the manuscript. RH and JS wrote sections of the manuscript. All authors contributed to manuscript revision, read, and approved the submitted version.
Funding
This research was funded by the European Commission Horizon 2020 project Diverfarming (Grant agreement 728003). The funders had no role in the design of the study; in the collection, analyses, or interpretation of data; in the writing of the manuscript; or in the decision to publish the results.
Conflict of Interest
LH was employed by the company GreenGrass Ltd.
The remaining authors declare that the research was conducted in the absence of any commercial or financial relationships that could be construed as a potential conflict of interest.
Publisher’s Note
All claims expressed in this article are solely those of the authors and do not necessarily represent those of their affiliated organizations, or those of the publisher, the editors and the reviewers. Any product that may be evaluated in this article, or claim that may be made by its manufacturer, is not guaranteed or endorsed by the publisher.
References
Abdalla, M., Hastings, A., Cheng, K., Yue, Q., Chadwick, D., Espenberg, M., et al. (2019). A Critical Review of the Impacts of Cover Crops on Nitrogen Leaching, Net Greenhouse Gas Balance and Crop Productivity. Glob. Change Biol. 25, 2530–2543. Publisher: Wiley Online Library. doi:10.1111/gcb.14644
Basche, A. D., Miguez, F. E., Kaspar, T. C., and Castellano, M. J. (2014). Do cover Crops Increase or Decrease Nitrous Oxide Emissions? A Meta-Analysis. J. Soil Water Conservation 69, 471–482. Publisher: Soil and Water Conservation Society. doi:10.2489/jswc.69.6.471
Beillouin, D., Ben-Ari, T., Malezieux, E., Seufert, V., and Makowski, D. (2021). Positive but Variable Effects of Crop Diversification on Biodiversity and Ecosystem Services. Glob. Change Biol. 27 (19), 4697–4710. Publisher: Wiley Online Library. doi:10.1111/gcb.15747
Bodelier, P. L. E., and Laanbroek, H. J. (2004). Nitrogen as a Regulatory Factor of Methane Oxidation in Soils and Sediments. FEMS Microbiol. Ecol. 47, 265–277. doi:10.1016/S0168-6496(03)00304-0
Carter, M. S., and Ambus, P. (2006). Biologically Fixed N2 as a Source for N2O Production in a Grass-clover Mixture, Measured by 15N2. Nutr. Cycl Agroecosyst 74, 13–26. doi:10.1007/s10705-005-4111-0
Davidson, E. A., Keller, M., Erickson, H. E., Verchot, L. V., and Veldkamp, E. (2000). Testing a Conceptual Model of Soil Emissions of Nitrous and Nitric Oxides. Bioscience 50, 667–680. Publisher: American Institute of Biological Sciences. doi:10.1641/0006-3568(2000)050[0667:tacmos]2.0.co;2
Decock, C., Lee, J., Necpalova, M., Pereira, E. I. P., Tendall, D. M., and Six, J. (2015). Mitigating N2O Emissions from Soil: from Patching Leaks to Transformative Action. SOIL 1, 687–694. Publisher: Copernicus GmbH. doi:10.5194/soil-1-687-2015
Dittrich, F., Iserloh, T., Treseler, C.-H., Hüppi, R., Ogan, S., Seeger, M., et al. (2021). Crop Diversification in Viticulture with Aromatic Plants: Effects of Intercropping on Grapevine Productivity in a Steep-Slope Vineyard in the Mosel Area, Germany. Agriculture 11, 95. doi:10.3390/agriculture11020095
Elliott, L. F., and Chevalier, P. (1996). Diversification for New Management Systems Opportunities in the Pacific Northwest. Am. J Alt. Ag 11, 77–82. Publisher: Cambridge University Press. doi:10.1017/S0889189300006822
Fuchs, K., Hörtnagl, L., Buchmann, N., Eugster, W., Snow, V., and Merbold, L. (2018). Management Matters: Testing a Mitigation Strategy for Nitrous Oxide Emissions Using Legumes on Intensively Managed Grassland. Biogeosciences 15, 5519–5543. Publisher: Copernicus GmbH. doi:10.5194/bg-15-5519-2018
Fuchs, K., Merbold, L., Buchmann, N., Bellocchi, G., Bindi, M., Brilli, L., et al. (2020). Evaluating the Potential of Legumes to Mitigate N 2 O Emissions from Permanent Grassland Using Process‐Based Models. Glob. Biogeochem. Cycles 34, e2020GB006561. eprint: https://agupubs.onlinelibrary.wiley.com/doi/pdf/10.1029/2020GB006561. doi:10.1029/2020GB006561
Fuß, R. (2017). Function (version 0.4) Gasfluxes: Greenhouse Gas Flux Calculation from Chamber Measurements. Available at: https://cran.r-project.org/web/packages/gasfluxes/index.html
Garland, G. M., Suddick, E., Burger, M., Horwath, W. R., and Six, J. (2011). Direct N2O Emissions Following Transition from Conventional till to No-Till in a Cover Cropped Mediterranean Vineyard (Vitis vinifera). Agric. Ecosyst. Environ. 141, 234–239. doi:10.1016/j.agee.2011.02.017
Griffiths, B. S., Römbke, J., Schmelz, R. M., Scheffczyk, A., Faber, J. H., Bloem, J., et al. (2016). Selecting Cost Effective and Policy-Relevant Biological Indicators for European Monitoring of Soil Biodiversity and Ecosystem Function. Ecol. Indicators 69, 213–223. doi:10.1016/j.ecolind.2016.04.023
Hüppi, R., Felber, R., Krauss, M., Six, J., Leifeld, J., and Fuß, R. (2018). Restricting the Nonlinearity Parameter in Soil Greenhouse Gas Flux Calculation for More Reliable Flux Estimates. PLOS ONE 13, e0200876–17. doi:10.1371/journal.pone.0200876
Kiran, U., and Patra, D. D. (2003). Medicinal and Aromatic Plant Materials as Nitrification Inhibitors for Augmenting Yield and Nitrogen Uptake of Japanese Mint (Mentha Arvensis L. Var. Piperascens). Bioresour. Techn. 86, 267–276. doi:10.1016/S0960-8524(02)00143-8
Marques, F. J. M., Pedroso, V., Trindade, H., and Pereira, J. L. S. (2018). Impact of Vineyard Cover Cropping on Carbon Dioxide and Nitrous Oxide Emissions in Portugal. Atmos. Pollut. Res. 9, 105–111. doi:10.1016/j.apr.2017.07.006
Pappa, V. A., Rees, R. M., Walker, R. L., Baddeley, J. A., and Watson, C. A. (2011). Nitrous Oxide Emissions and Nitrate Leaching in an Arable Rotation Resulting from the Presence of an Intercrop. Agric. Ecosyst. Environ. 141, 153–161. doi:10.1016/j.agee.2011.02.025
Qiu, Q., Wu, L., Ouyang, Z., Li, B., Xu, Y., Wu, S., et al. (2015). Effects of Plant-Derived Dissolved Organic Matter (DOM) on Soil CO2 and N2O Emissions and Soil Carbon and Nitrogen Sequestrations. Appl. Soil Ecol. 96, 122–130. doi:10.1016/j.apsoil.2015.07.016
Rezsek, M., Dezso, J., and Loczy, D. (2019). Crop Diversification for Asparagus in the Pannonian Pedoclimatic Region: Opportunities and Constraints. Agric. Res. Technol. Open Access J. 23, 250–252. Publisher: Juniper Publishers Inc. doi:10.19080/ARTOAJ.2019.22.556221
Rodrigo Comino, J., Iserloh, T., Morvan, X., Malam Issa, O., Naisse, C., Keesstra, S., et al. (2016). Soil Erosion Processes in European Vineyards: A Qualitative Comparison of Rainfall Simulation Measurements in Germany, Spain and France. Hydrology 3, 6. doi:10.3390/hydrology3010006
Saha, D., Kaye, J. P., Bhowmik, A., Bruns, M. A., Wallace, J. M., and Kemanian, A. R. (2021). Organic Fertility Inputs Synergistically Increase Denitrification-Derived Nitrous Oxide Emissions in Agroecosystems. Ecol. Appl. 31, e02403. Publisher: Wiley Online Library. doi:10.1002/eap.2403
Senbayram, M., Wenthe, C., Lingner, A., Isselstein, J., Steinmann, H., Kaya, C., et al. (2016). Legume-based Mixed Intercropping Systems May Lower Agricultural Born N2O Emissions. Energ Sustain. Soc. 6, 2. doi:10.1186/s13705-015-0067-3
Stagnari, F., Maggio, A., Galieni, A., and Pisante, M. (2017). Multiple Benefits of Legumes for Agriculture Sustainability: an Overview. Chem. Biol. Technol. Agric. 4, 2. doi:10.1186/s40538-016-0085-1
Tamburini, G., Bommarco, R., Wanger, T. C., Kremen, C., van der Heijden, M. G. A., Liebman, M., et al. (2020). Agricultural Diversification Promotes Multiple Ecosystem Services without Compromising Yield. Sci. Adv. 6, eaba1715. Publisher: American Association for the Advancement of Science. doi:10.1126/sciadv.aba1715
Thomas, B. W., Hao, X., Larney, F. J., Goyer, C., Chantigny, M. H., and Charles, A. (2017). Non-legume Cover Crops Can Increase Non-growing Season Nitrous Oxide Emissions. Soil Sci. Soc. America J. 81, 189–199. Publisher: Wiley Online Library. doi:10.2136/sssaj2016.08.0269
Tiwari, S., Singh, C., and Singh, J. S. (2018). Land Use Changes: a Key Ecological Driver Regulating Methanotrophs Abundance in upland Soils. Energ. Ecol. Environ. 3, 355–371. doi:10.1007/s40974-018-0103-1
Waldo, N. B., Hunt, B. K., Fadely, E. C., Moran, J. J., and Neumann, R. B. (2019). Plant Root Exudates Increase Methane Emissions through Direct and Indirect Pathways. Biogeochemistry 145, 213–234. Publisher: Springer. doi:10.1007/s10533-019-00600-6
Watson, A., Stephen, K. D., Nedwell, D. B., and Arah, J. R. M. (1997). Oxidation of Methane in Peat: Kinetics of CH4 and O2 Removal and the Role of Plant Roots. Soil Biol. Biochem. 29, 1257–1267. doi:10.1016/S0038-0717(97)00016-3
Wezel, A., Casagrande, M., Celette, F., Vian, J.-F., Ferrer, A., and Peigné, J. (2014). Agroecological Practices for Sustainable Agriculture. A Review. Agron. Sustain. Dev. 34, 1–20. Publisher: Springer. doi:10.1007/s13593-013-0180-7
Keywords: diversification in farming systems, intercropping, nitrous oxide emissions, non-linear gas fluxes, vineyard, asparagus field, soil
Citation: Hüppi R, Horváth L, Dezső J, Puhl-Rezsek M and Six J (2022) Soil Nitrous Oxide Emission and Methane Exchange From Diversified Cropping Systems in Pannonian Region. Front. Environ. Sci. 10:857625. doi: 10.3389/fenvs.2022.857625
Received: 18 January 2022; Accepted: 03 March 2022;
Published: 04 April 2022.
Edited by:
Marco Contin, University of Udine, ItalyReviewed by:
Debasish Saha, The University of Tennessee, United StatesTim J. Clough, Lincoln University, New Zealand
Copyright © 2022 Hüppi, Horváth, Dezső, Puhl-Rezsek and Six. This is an open-access article distributed under the terms of the Creative Commons Attribution License (CC BY). The use, distribution or reproduction in other forums is permitted, provided the original author(s) and the copyright owner(s) are credited and that the original publication in this journal is cited, in accordance with accepted academic practice. No use, distribution or reproduction is permitted which does not comply with these terms.
*Correspondence: Roman Hüppi, roman.hueppi@pm.me