- 1College of Agronomy, Shandong Agricultural University, Tai’an, China
- 2State Key Laboratory of Crop Biology, Shandong Agricultural University, Tai’an, China
Peanut (Arachis hypogaea L.) is a significant oil and protein crop. Its yields greatly depend on the availability of phosphorus (P). Root morphology and P absorption efficiency are important factors affecting the P uptake capacity, but their relationships in peanuts are rarely reported. Here, we report the effect of root morphology and P absorption efficiency on the P uptake capacity in peanuts using 235 germplasms. In this work, we use the P uptake rate per plant to reflect the P uptake capacity. The P uptake capacity was significantly increased after low-P treatment and showed great differences among the germplasms. The germplasms with higher P absorption efficiency and a well-developed root system have higher P uptake capacity. Under both P conditions, the P absorption efficiency plays more important roles than root morphology in P uptake capacity, and the P uptake rate per unit root dry weight and the P uptake rate per unit root surface area contributed the most. Root morphology contributes more to the P uptake capacity under low-P treatment than under sufficient-P conditions, and root surface area contributed the most. Forty-eight germplasms with higher P uptake capacity were screened, and they had three different uptake strategies under low-P treatment. These findings indicated that low-P stress induces root growth and improves the P absorption efficiency of peanuts to ensure the plant gets enough P; provides new insights into the relationship between the P uptake capacity, P absorption efficiency, and root morphology; and furnishes important evaluation indexes for high P-efficient germplasm selection.
Introduction
Phosphorus (P) is an essential macroelement for crop growth and development, which participates in multiple important biological processes (Lorenzo-Orts et al., 2020). Although P is abundant in soil, little available P can be directly absorbed and utilized by plants due to fixation of the soil (Li et al., 2011; Manschadi et al., 2014). Under low-P conditions, plants improve the P uptake and utilization efficiency by a series of morphological, physiological, and biochemical adaptations; however, these functions were significantly different among crops or genotypes (Yuan et al., 2017; Kale et al., 2020; Soumya et al., 2021). Previous research found that P uptake is more important than P utilization when P supply is limited, while P utilization has a greater impact on P efficiency for a sufficient P supply (Manske et al., 2001; Wang et al., 2010a). In coffee, the contribution of P uptake efficiency to yield formation increased significantly under low-P (LP) conditions (Neto et al., 2016). Therefore, studying the P-efficient absorption characteristics is of great significance to enhance the adaptability of crops in the LP environment.
Plants have evolved a variety of beneficial root traits and physiological traits to obtain more amounts of P under the LP environment (López-Arredondo et al., 2014; Nestler et al., 2016; Liu, 2021; Zhang et al., 2021). On the one hand, the increase in the P absorption area is conducive to higher P uptake capacity. Under P deficiency, the plants distribute more photosynthetic products to the root to improve their development and growth. Excellent root morphology and root configuration were promoted to upgrade the P absorption area (Wang et al., 2010b; Falk et al., 2020; Bello, 2021). On the other hand, when available P diffuses to the root surface, a higher P absorption efficiency per unit area is important for P acquisition. The root acquires P mainly in the form of inorganic phosphate (Pi, orthophosphate) by Pi transporters in plasma membranes, and the plasma membrane-localized Pi transporter activity affects P acquisition and allocation (Liao et al., 2019). Under LP conditions, cells express predominantly high-affinity Pi transporters, which could enhance P uptake ability by promoting the Pi transport from the root surface to the cytoplasm (Lapis-Gaza et al., 2014; Sudhakar et al., 2018; Wang et al., 2018).
Plant P acquisition is generally driven by cooperations of morphological and physiological responses (Lynch and Ho, 2005; Honvault et al., 2020). Because these traits require carbon costs, plants tend to rely primarily on a few of these favorable traits (Pearse et al., 2006; Raven et al., 2018; Wen et al., 2019). In maize and wheat, the root morphology plays more important roles than the physiological responses under LP conditions (Lyu et al., 2016; Wen et al., 2017), whereas root exudates increased more significantly in chickpeas (Lyu et al., 2016). Trade-offs and coordination between morphological and physiological traits resulted in multiple P uptake strategies, resulting in similar total P uptake levels (Lyu et al., 2016; Honvault et al., 2020). Due to the diversity and complexity of the P uptake strategies among germplasms, it is unilateral to evaluate P uptake efficiency by a few germplasms. Therefore, it is necessary to evaluate and identify P uptake efficiency, as well as its influencing factors, in a wide range of populations.
The peanut (Arachis hypogaea L.) is an important oil and protein crop. P deficiency is one of the factors limiting its production (Singh et al., 2015; Naga Madhuri et al., 2018). The P uptake capacity reflects the adaptability to certain P conditions. The P uptake rate per plant is defined as the P absorption per plant per unit time and can be used to reflect the P uptake capacity. At present, few studies are available regarding P uptake capacity in peanuts, and related studies were only based on recombinant inbred line (RIL) populations or dozens of germplasms to evaluate the changes of few root morphology traits (Krishna, 1997; Jadhav and Gowda, 2012; Kumar et al., 2015). For example, Jadhav and Gowda (2012) studied the root morphology in a recombinant inbred line (RIL) population and found significant differences in root traits between germplasms under different P treatments. Krishna (1997) and Kumar et al. (2015) found that increased root biomass, root length, and P accumulation per unit root length are beneficial to P absorption, but different varieties rely on different uptake strategies. The P uptake capacity under P deficiency was affected by the expression of root traits associated with soil foraging and P transporter activity associated with P absorption efficiency. But limited information is available on the contribution of the root morphology and P absorption efficiency. Moreover, the selection and evaluation of P-efficient uptake peanut cultivars and germplasms were rarely reported, which restricted the extension of P-efficient cultivars and the development and use of P-efficient germplasms. In our research, the total root length, root surface area, root volume, average root diameter, root tip number, specific root length, and root dry weight per plant were measured to reflect the root morphology; the P uptake rate per unit root dry weight/root length/root surface area/root volume was calculated to represent the P absorption efficiency. A diverse panel including 235 peanut germplasms with extensive phenotypic variations in P efficiency was grown in LP and sufficient-P (SP) conditions. The objectives of this study were to (i) evaluate the effects of LP stress on the P uptake capacity, P absorption efficiency, and root morphology; (ii) evaluate the contribution of the P absorption efficiency and root morphology to P uptake capacity under LP and SP conditions; and (iii) screen P-efficient germplasms and explore P-efficient uptake strategies. These analyses clarify the key factors affecting the P uptake capacity and the P uptake strategies of different P-efficient germplasms, providing the experimental method and theoretical basis for the investigation of the P efficiency in peanuts.
Materials and Methods
Plant Materials
A diverse panel consisting of 235 peanut germplasms was used in this study (Supplementary Table S1). These lines covered 87 (37.02%) lines of ssp. fastigiate (62 var. vulgaris, 22 var. fastigiata, and 3 var. aequatoriana), 85 (36.17%) lines of ssp. hypogaea (65 var. hypogaea and 20 var. hirsuta), and 63 (26.81%) lines of irregular types (Zhang et al., 2017). These germplasms included main cultivars, native varieties, a peanut core collection, important breeding parents, and advanced breeding lines. The lines also showed a broad geographic distribution: 169 (71.91%) lines were from different areas of China, 55 (23.40%) were from 17 countries, and 11 (4.68%) were unknown (Supplementary Table S1). There was a significant phenotypic variation within the population, and key agronomic traits showed normal distributions (Zhang et al., 2017). All the germplasms were stored by our group.
Experiments Design
Hydroponic experiments were carried out in a greenhouse. Intact plump seeds with similar sizes were surface-sterilized with 0.1% H2O2 for 6 h and thoroughly rinsed by sterilizing deionized water. The seeds were then placed on wet degreasing cotton in germination disks and grown in the dark for about 5 days at 26°C. The seeds should stay moist during germination. After unfolding, 10 seedlings similar in size were transplanted to a hydroponic box with deionized water for 3 days and then cultured in 1/5 Hoagland’s nutrient solution (1.4 L) with sufficient (0.6 mmol/L) and low (0.01 mmol/L) KH2PO4. Sixteen days after P treatment, plants were cultured in deionized water for 24 h, and then transferred to 1/5 Hoagland’s nutrient (1.4 L) with 0.2 mmol/L KH2PO4 for 8 h after LP treatment (then transferred to deionized water for 16 h) and 24 h after the SP condition (Supplementary Figure S1). Three biological repeats were performed for each treatment, and 10 seedlings were tested in each replication. The composition of Hoagland’s solution was as follows: 1 mmol/L KNO3, 1 mmol/L Ca (NO3)2·4H2O, 0.4 mmol/L MgSO4·7H2O, 1 mmol/L KH2PO4, 0.01 mmol/L EDTA·Fe, 0.1 × 10−3 μmol/L H3BO3, 0.15 × 10−3 μmol/L MnSO4, 0.03 × 10−3 μmol/L, ZnSO4·7H2O, 1.0 × 10−6 μmol/L Na2MoO4·2H2O, 0.16 × 10−6 μmol/L CuSO4·5H2O, and 0.21 × 10−6 μmol/L CoCl2·6H2O (Hoagland and Arnon, 1950). The nutrient solution was changed every 4 days, and pH was maintained at 6.0 using HCl or NaOH. The LP nutrient solution was supplemented with KCl to ensure that the K concentration was consistent with that of the SP nutrient solution. The seedlings were grown in a greenhouse under 16-h light/8-h dark photoperiod (11.4K LuX light intensity) at 24°C.
P Concentration and Root Morphology Measurement
The P uptake rate was measured by using the depletion method after 16 days of P treatment (Claassen and Barber, 1974; Bhadoria et al., 2004). To maximize the P uptake rate, plants were cultured in deionized water for 24 h and then transferred to 1/5 Hoagland’s nutrient (1.4 L) with 0.2 mmol/L KH2PO4. To minimize P consumption in the solution under the condition of different P uptake rates after high- and low-P treatments, the plants were treated for 8 h after LP treatment (then transferred to deionized water for 16 h) and 24 h after the SP condition. Then the nutrient solution was replenished to 1.4 L, and 3 ml nutrient solution was taken from each hydroponic box after 8-h LP treatment and 24-h SP condition. The P concentration in nutrient solution was determined by using the vanadium molybdate blue colorimetric method (Murphy and Riley, 1962). The P uptake rate per plant (PUR, μmol h−1 plant−1) is the P absorption per plant per unit time and reflects the P uptake capacity of the peanut. Considering the diversity of root morphology of different germplasms, the P uptake rate per unit root dry weight (PUR_RDW), P uptake rate per unit root length (PUR_RL), P uptake rate per unit root surface area (PUR_RSA), and P uptake rate per unit root volume (PUR_RV) were calculated to reflect the P absorption efficiency.
where C1 is the P concentration of nutrient solution before absorption, V1 is the volume of nutrient solution before absorption, C2 is the P concentration of nutrient solution after absorption, V2 is the volume of nutrient solution after absorption, h is the absorption time, and N is the number of plants in each box.
At harvest (18 days after P treatment), the roots from 6 plants per treatment were harvested and rinsed with deionized water. The total root length (TRL), root surface area (RSA), root volume (RV), average root diameter (ARD), and root tip number (RTN) were measured using the WinRHIZO LA-S image analysis system (WinRHizo LA-S, Re´gent Instr. Inc., Quebec, Canada). Then the root samples were dried in an oven at 80°C until the dry weight was constant, and then root dry weight (RDW) was measured. The specific root length (SRL) (m g−1) was determined by dividing the RDW by TRL (Table 1). These seven indices were used to reflect the root morphology. We also measured the shoot dry weight (SDW) and calculated the total dry weight (TDW) and root/shoot ratio (RSR). Three biological repeats were carried out for each treatment.
To better understand the responses of LP treatment, the relative values of all traits were calculated by dividing the values of LP treatment by the values of the SP condition (Yuan et al., 2017).
Data Analysis
Descriptive statistics, analyses of variance (ANOVA), and correlation were performed using SPSS 21.0 (SPSS Inc., Chicago, IL, United States), and then we calculated the broad-sense heritability (hB2) by hB2 = σg2/(σg2+σe2), where σg2 means genetic variance and σe2 means environmental variance (Knapp et al., 1985). Clustering based on Ward’s method was executed using a squared Euclidean distance matrix of the PUR after LP treatment, germplasms were divided into low P uptake capacity, medium P uptake capacity, and high P uptake capacity. Principal component analysis (PCA) was carried out using the “FactomineR” package in R version 4.1.0 (R Foundation for Statistical Computing, Vienna, 2005). Hierarchical classification on principal components (HCPC) was then performed using the first two principal components (80% of the total variability) to define groups with similar patterns of P absorption efficiency and root morphology. To underline the relative ability of the P absorption efficiency and root morphology for P uptake capacity, partial square path modeling (PLS PM) was performed using the “pls pm” package in R version 3.6.0 (Sanchez, 2013). Two clusters of variables, or “latent variables,” were defined, respectively: the “root morphology” variables encompassing TRL, RSA, RV, ARD, RTN, SRL, and RDW, and the “P absorption efficiency” variables encompassing PUR_RDW, PUR_RL, PUR_RSA, and PUR_RV. Overall model quality was evaluated with the goodness of fit (GoF) index, and significance was determined with 95% confidence. Graphs were plotted using the “ggplot2” package in R version 4.1.0.
Results
Effects of LP Stress on the P Uptake Capacity, P Absorption Efficiency, and Root Morphology
P deficiency enhanced root production and induced root morphological remodeling in peanuts. Some germplasms produced more and longer lateral roots and increased the root volume and root surface area for P exploration (Jadhav and Gowda, 2012; Kumar et al., 2015). To investigate the root morphology differences among peanut germplasms under LP treatment, we performed scanning and analysis of roots using 235 germplasms. Scanning of root systems revealed variable changes among germplasms when exposed to LP (Figure 1). After quantifying the TRL, RSA, RV, ARD, and RTN of all germplasms, we found that these indexes display normal distribution through the population (Figure 2).
To clarify the effects of LP treatment on the P uptake capacity and root morphology, we performed comprehensive analysis of 12 traits (Table 2). Significant (p < 0.001) differences were detected among the populations under SP and LP conditions (Table 2; Figure 2). Compared to the SP condition, a significant increase (335%) was noted in PUR after LP treatment in all germplasms (Table 2). However, the range of relative value showed that the root morphology varied differently among germplasms after LP treatment. For example, fenghua6, Sichuanhongbaili, PI274193, and Toalson significantly increased (Figure 1, Supplementary Figure S3); Baisha1016, 03F032, and 07H226 decreased; and 98D080-2, Silicai (1), and Ji0608-228 significantly decreased (Supplementary Figure S2). Similar results were also found in the P uptake capacities among germplasms (Table 2). The mean value of RDW, TRL, RV, RSA, RTN, and SRL of the population all increased after LP treatment in comparison to SP condition, their relative values ranged from 1.17 to 1.46, and the increasing range was 28.57, 41.30, 27.44, 36.39, 21.88, and 13.19%, respectively. The ARD showed a relatively small change and decreased by 1.45%. The PUR_RDW, PUR_RL, PUR_RSA, and PUR_RV of the populations all increased under LP treatment. Their relative values were all greater than 3.4, and they increased significantly by 243, 201, 206, and 221%, respectively. The coefficients of variation (CVs) of all traits, except ARD, were close to or above 20% (Table 2). The hB2 of all traits ranged from 57.42 to 78.14%.
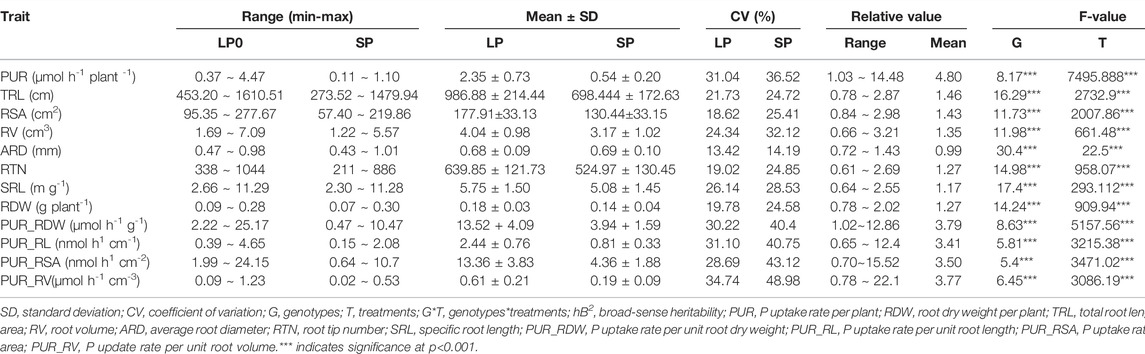
TABLE 2. Phenotypic variation and analysis of variance (ANOVA) of investigated traits among peanut germplasms under low-P (LP) and sufficient-P (SP) conditions.
These findings indicated that LP stress induces the root growth and improves the P absorption efficiency of peanuts to ensure the plant gets enough P for the shoot growth (Figure 2). This regular pattern is universally valid throughout the peanut population.
Comparison of P Uptake Capacity Among Germplasms
Based on the average PUR of 235 germplasms after LP treatment, these germplasms were divided into three clusters: high P uptake capacity, medium P uptake capacity, and low P uptake capacity (Supplementary Figure S4, Figure 3A), which make up 20.4% (48), 46.4% (109), and 33.2% (78) of the population, respectively. Under SP conditions, the P uptake capacity of the high, medium, and low clusters was 0.63, 0.57, and 0.44 μmol h−1 plant −1, while under LP treatment, it was 3.42, 2.43, and 1.58 μmol h−1 plant −1, respectively.
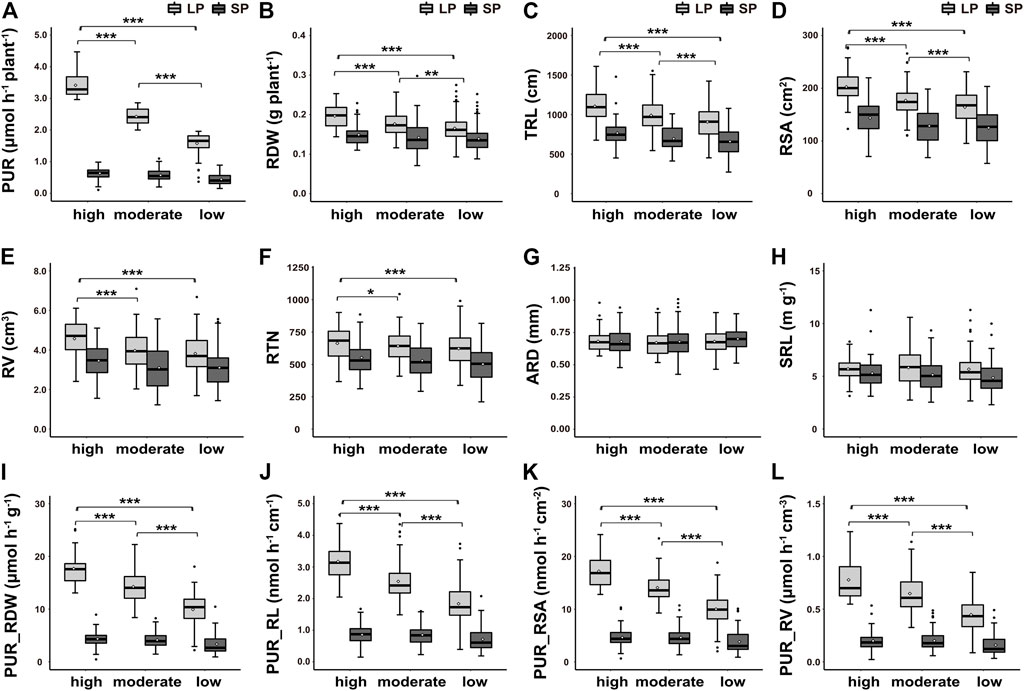
FIGURE 3. Box and whisker plot showing differences in 12 traits among different P uptake capacity of peanut germplasms under low-P (LP) and sufficient-P (SP) treatments. (A) P uptake rate per plant (PUR), (B) root dry weight per plant (RDW), (C) total toot length (TRL), (D) root surface area (RSA), (E) root volume (RV), (F) root tip number (RTN), (G) average root diameter (ARD), (H) specific root length (SRL), (I) P uptake rate per unit root dry weight (PUR_RDW), (J) P uptake rate per unit root length (PUR_RL), (K) P uptake rate per unit root surface area (PUR_RSA), and (L) P uptake rate per unit root volume (PUR_RV). High, moderate, and low represent high, moderate, and low P uptake rates per plant, respectively. Horizontal lines inside boxes, median; white dot, mean; box hinges, first and third quartiles; whiskers, full range of the data; black dots represent outliers. *, **, and *** indicate significance at p < 0.05, p < 0.01, and p < 0.001, respectively.
The root morphology of germplasms belonging to each cluster was analyzed, respectively, to explore their relationship with P uptake capacity. As shown in Figure 3, under SP conditions, none of the 12 indexes showed significant differences among the three clusters. Under LP treatment, RDW, TRL, RSA, PUR_RDW, PUR_RL, PUR_RSA, and PUR_RV represented the extremely significant difference between the three clusters (Figures 3B–D,I–L), while the difference was not detected in RV and RTN of moderate and low clusters (Figures 3E,F). The ARD and SRL showed little variation between the three clusters (Figures 3G,H). These findings indicated that germplasms whose RDW, TRL, and RSA respond strongly to LP stress usually showed higher P uptake capacity.
Contributions of P Absorption Efficiency and Root Morphology to the P Uptake Capacity
To investigate the relationships between the root morphology and P uptake capacity, correlation analysis was conducted. As shown in Table 3, the PUR was significantly and positively correlated with the PUR_RDW, PUR_RL, PUR_RSA, and PUR_RV, with correlation coefficients greater than 0.600 under two P conditions. Significantly positive correlations were also found between the PUR and the RDW, TRL, RV, RSA, and RTN with correlation coefficients between 0.143 and 0.430 after LP treatment. In comparison with LP treatment, the PUR showed a weaker positive correlation with RDW, TRL, RV, and RSA, with correlation coefficients between 0.163 and 0.240 under SP conditions. These results show that the P absorption efficiency and root morphology were significantly correlated with P uptake capacity, and the correlation of P absorption efficiency was greater than that of root morphology. The PCA also underlined the importance of P absorption efficiency and root morphology under LP treatment, and the contribution of P absorption efficiency on the P uptake capacity was larger than that of root morphology (Figure 4).
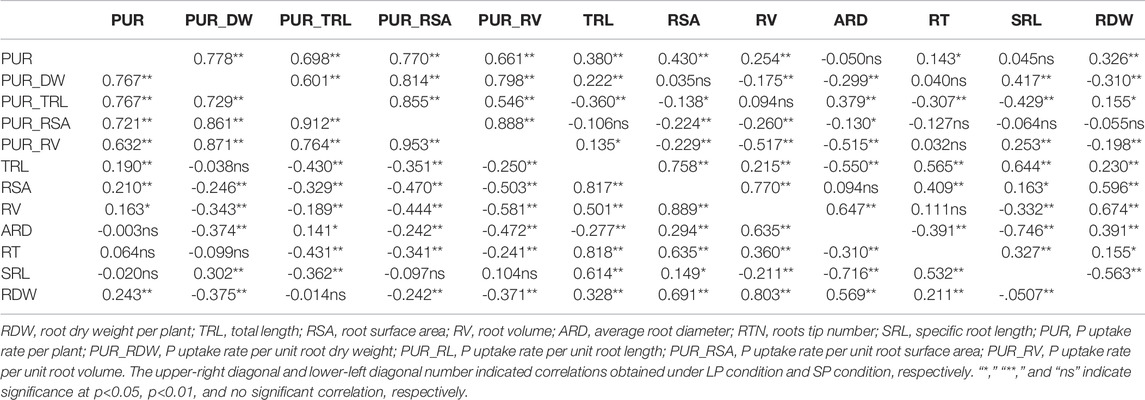
TABLE 3. Relationships between PUR and root morphology/P absorption efficiency traits under low-P (LP) and sufficient-P (SP) conditions.
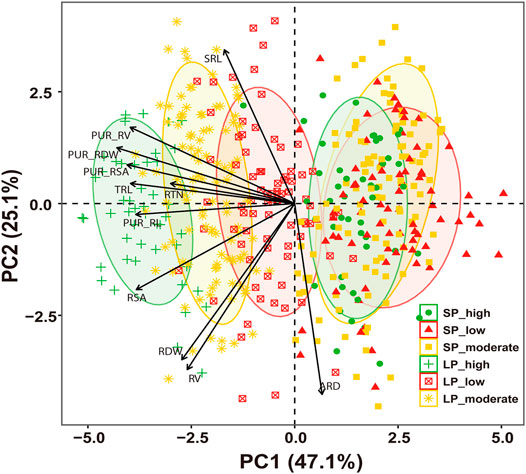
FIGURE 4. Principal component analysis (PCA) of genotype and genotype × trait interaction. SP_high, high P uptake rate per plant under sufficient-P treatment; SP_low, low P uptake rate per plant under sufficient-P treatment; SP_moderate, moderate P uptake rate per plant under sufficient-P treatment; LP_high, high P uptake rate per plant under low-P treatment; LP_low, low P uptake rate per plant under low-P treatment; LP_moderate, moderate P uptake rate per plant under low-P treatment. RDW, root dry weight per plant; TRL, total toot length; RSA, root surface area; RV, root volume; ARD, average root diameter; RTN, root tip number; SRL, specific root length; PUR_RDW, P uptake rate per unit root dry weight; PUR_RL, P uptake rate per unit root length; PUR_RSA, P uptake rate per unit root surface area; PUR_RV, P uptake rate per unit root volume.
To further explore the effects of P absorption efficiency and root morphology on the P uptake capacity, the PLS PM was also used. As shown in Figure 5, PLS PM (R2 = 0.960 under LP treatment, R2 = 0.875 under SP condition) showed that the effects of P absorption efficiency (coef = 0.868 under LP treatment, coef = 0.911 under SP condition) on P uptake capacity were larger than that on root morphology (coef = 0.520 under LP treatment and coef = 0.385 under SP condition) under the two P conditions. The effects of root morphology on P uptake capacity after LP treatment were larger than that under the SP condition, while the opposite result occurred in P absorption efficiency. The most impactful contributor to the root morphology was RSA > TRL > RDW under LP treatment and RDW > RSA under the SP condition. Under the LP treatment, PUR_RDW and PUR_RSA were the better single predictor in the P absorption efficiency component, followed by PUR_RL and PUR_RV. Under SP conditions, PUR_RDW and PUR_RL were both the best single predictor in the P absorption efficiency component, followed by PUR_RSA. These findings indicate that the P absorption efficiency, especially PUR_RDW and PUR_RSA, has a larger contribution to the P uptake capacity than root morphology under two P conditions; and the contribution of root morphology (mainly RSA) to P uptake capacity increased under LP treatment compared to the SP condition.
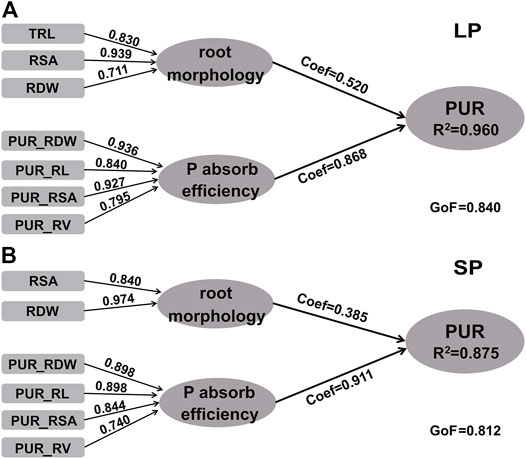
FIGURE 5. Partial least square-path models predicting PUR. (A) Low-P (LP) condition and (B) sufficient-P (SP) condition. Coef is the path coefficient. GoF, goodness of fit of the model; R2, coefficients of determination of the endogenous latent variables; RDW, root dry weight per plant; TRL, total toot length; RSA, root surface area; RV, root volume; ARD, average root diameter; RTN, root tip number; SRL, specific root length; PUR, P uptake rate per plant; PUR_RDW, P uptake rate per unit root dry weight; PUR_RL, P uptake rate per unit root length; PUR_RSA, P uptake rate per unit root surface area; PUR_RV, P uptake rate per unit root volume. Coef are significant at 95% confidence intervals (p value < 0.05).
Screening and Evaluation of Germplasms With High P Uptake Capacity
To compare the P uptake differences among germplasms, PCA and HCPC were performed on the P absorption efficiency and root morphology of 48 germplasms with high P uptake capacity after LP treatment. As shown in Figure 6A, the first two PCA components represented 74.4% of the total variability. Based on the PCA scores, the germplasms with high P uptake capacity were divided into four groups via HCPC (Figures 6B, 7). Group 1 (G1), encompassing 11 germplasms (such as EC7746 and Yangfanhuasheng), showed the higher PUR_RDW, PUR_RL, PUR_RSA, and PUR_RV after LP treatment but poor root morphology compared with other high P uptake capacity germplasms; group 2 (G2), encompassing 18 germplasms (such as Putiangoubi and Miandianhuasheng), showed medium to strong P absorption efficiency and well-developed root system after LP treatment; group 3 (G3), encompassing 7 germplasms (such as Huayu22 and Haiyangdalidun), presented the greatest PUR_RL, RV, ARD, and RDW, while the rest showed average behavior; and group 4 (G4), encompassing 12 germplasms (such as Fenghua4 and Sichuanhongbaili), showed higher RDW, TRL, RV, RSA, RTN, and SRL after LP treatment, but P absorption efficiency was lower than that in other high P uptake capacity germplasms. Under SP conditions, similar behaviors were found in the root morphology between four groups with LP treatment, but there was no significant difference in P absorption efficiency. These results suggest that the trade-off and coordination between P absorption efficiency and root morphology could result in similar levels of total P uptake under LP stress.
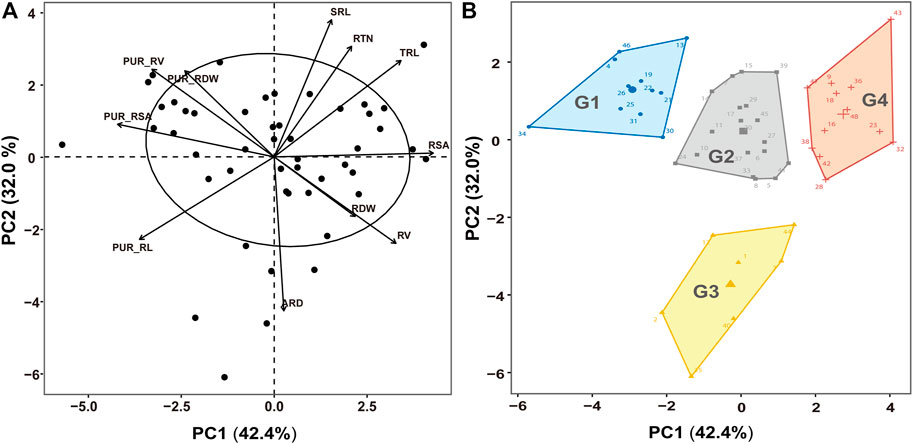
FIGURE 6. Principal component analysis (PCA) of P absorption efficiency traits and root traits under low-P (LP) treatment. (A) Variable covariance along with the first two components and (B) clusters formed with hierarchical classification on principal components (HCPC). RDW, root dry weight per plant; TRL, total toot length; RSA, root surface area; RV, root volume; ARD, average root diameter; RTN, root tip number; SRL, specific root length; PUR_RDW, P uptake rate per unit root dry weight; PUR_RL, P uptake rate per unit root length; PUR_RSA, P uptake rate per unit root surface area; PUR_RV, P uptake rate per unit root volume.
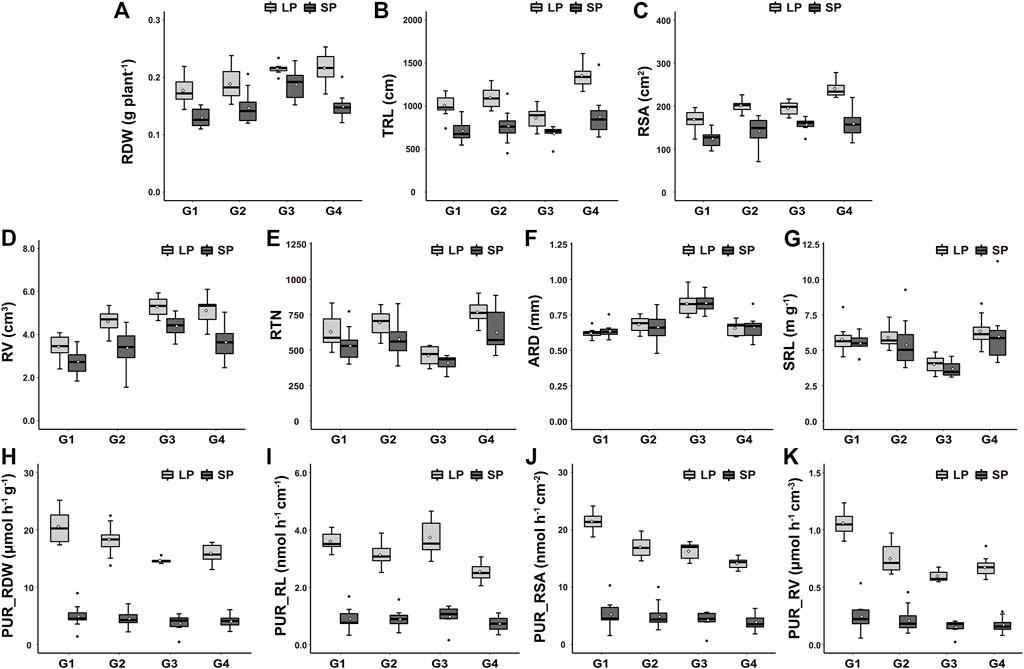
FIGURE 7. Box and whisker plot showing differences in 11 traits among high-P uptake capacity peanut germplasms under low-P (LP) and sufficient-P (SP) treatments. (A) Root dry weight per plant (RDW), (B) total toot length (TRL), (C) root surface area (RSA), (D) root volume (RV), (E) root tip number (RTN), (F) average root diameter (ARD), (G) specific root length (SRL), (H) P uptake rate per unit root dry weight (PUR_RDW), (I) P uptake rate per unit root length (PUR_RL), (J) P uptake rate per unit root surface area (PUR_RSA), and (K) P uptake rate per unit root volume (PUR_RV). High, moderate, and low represent high, moderate, and low P uptake rates per plant, respectively. Horizontal lines inside boxes, median; white dot, mean; box hinges, first and third quartiles; whiskers, full range of the data; black dots represent outliers.
Discussion
P Uptake Strategies of Peanut Germplasm Under LP Stress
With the decrease in available P in soil, it is more and more important to select varieties with high P uptake efficiency and P utilization efficiency. Identification and evaluation of the P-efficient germplasms have been studied in many crops, including wheat (Soumya et al., 2021), rice (Kale et al., 2020), and soybean (Wang et al., 2010b). P-efficient germplasms are suitable for growth under LP conditions, which is conducive to decreasing the P fertilizer utilization and protecting the environment. In our study, 48 germplasms with higher P uptake capacity, possessing the stronger P absorption efficiency and well-developed root system under LP stress (Figure 3), were selected. These excellent varieties include both main cultivars (Zhonghua4 and Fenghua5), which are dominant in certain areas of cultivation, and some selected germplasms (02P175, etc.). This provides a basis for the selection and utilization of P-efficient germplasms in peanuts.
Although both P absorption efficiency and root morphology are essential for P acquisition, their costs and benefits in a specific context resulted in multiple coordination and trade-offs among traits (Lynch and Ho, 2005; Raven et al., 2018). Different P-uptake strategies were formed among germplasms (Lyu et al., 2016; Wen et al., 2019; Honvault et al., 2020). In this study, three P uptake strategies were identified among germplasms with high P uptake capacity according to their P absorption efficiency and root morphology (Figure 7). The first strategy presented a well-developed root system (with the largest TRL and RSA) but lower P absorption efficiency, encompassing Sichuanhongbaili, 51015-2, etc. This strategy was probably oriented toward available P uptake area, a well-developed root system could increase soil foraging, and lager TRL and RSA denote an important exploration of a given soil volume (Liu, 2021). The other strategy presented stronger P absorption efficiency, possessing the highest PUR_RDW, PUR_RSA, and PUR_RV, but poor root morphology compared with other high-P uptake capacity germplasms, encompassing Funongbaipi and Yinyou5. The high P absorption efficiency of these germplasms is probably due to the higher activity of P transporters in the root (Sun et al., 2012; Liu C. et al., 2018). The third strategy focused on the interaction of the root morphology and P absorption efficiency, including two situations: one is that all traits showed good performance; for example, Zhonghua4 had both stronger P absorption efficiency and a well-developed root system; the other presented high expressions of partly traits; for example, Huayu22 showed outstanding performance in PUR_RL, RV, and ARD, with other traits showing poor performance. This phenomenon also indicates that the regulation and interaction between traits are very complex (McCormack et al., 2015; Honvault et al., 2020). In practical application, the selection of germplasms with optimal P uptake strategies according to the change of environment not only improves the application value of germplasms but also the environmental P utilization efficiency.
The Variation of Root Morphology and P Absorption Efficiency After LP Treatment and Their Effects on P Uptake Capacity
The P uptake efficiency varied widely among genotypes and environments (Wissuwa and Ae, 2001; Patricio, 2016). The improvement of P uptake capacity is the key to coping with P-deficiency stress. Our results also demonstrated this view (Table 2, Figure 1). The higher P uptake efficiency is generally achieved by modifying root growth, such as superficial root architecture, exudation of organic acid anions (Wang et al., 2015; Falk et al., 2020; Bello, 2021; Liu, 2021), or enhancing the ability to transport P from the root surface into the plant body (such as the expression of high-affinity Pi transporters) (Sun et al., 2012; Lapis-Gaza et al., 2014). In this study, the P absorption efficiency and root morphology were significantly correlated with the P uptake capacity after LP treatment (Table 3), which showed that a higher P uptake capacity may be related to a stronger P absorption efficiency and well-developed root system.
The influx of P in soil is mainly by diffusion, but poor diffusion rates and mobility result in insufficient effectiveness of soil rhizosphere P, so modifying root growth plays an important role in P uptake (Havlin, 1999; Liu, 2021). It has been reported in rice (Vejchasarn et al., 2016), wheat (Deng et al., 2018), maize (Liu Z. et al., 2018), and cotton (Mai et al., 2018) that root trait response to P nutrition is complex and shows different variations among genotypes. For peanuts, the study of the changes in the root morphology under LP conditions is limited; only root length, root dry weight, secondary root number, and root volume have been studied (Krishna, 1997; Jadhav and Gowda, 2012; Kumar et al., 2015); and only dozens of peanut varieties were used in some studies (Krishna, 1997; Jadhav and Gowda, 2012). In this study, we evaluated 7 root morphology traits of 235 peanut germplasms with a broad geographic distribution and significant phenotypic variation in P efficiency. Extensive and significant differences in roots were found among germplasms after LP treatment (Table 2; Figure 1). For example, PI274193 significantly increased root dry matter accumulation, Baisha2-3 and Sichuanhongbaili display longer main roots and more lateral roots, Meiyinxuan41165 and Lainong10 exhibit longer lateral roots, Grif 12545 and Toalson showed more and longer lateral roots (Figure 1, Supplementary Figure S3), while Silicai (1), Ji0608-228, and 98D080-2 showed shorter root length and less lateral roots, and Baisha1016 and 03F032 were less affected (Supplementary Figure S2). This comprehensively reflected the diversity of root changes among peanut germplasms under LP conditions. Previous studies have found that the differences in root morphology traits such as root configuration and root length resulted in the change in P uptake efficiency among crops and varieties (Bello, 2021; Liu, 2021). In this study, significantly positive correlations were found between the PUR and the RDW, TRL RV, RSA, and RT after LP treatment (Table 3). Similar results were also found in maize (Gu et al., 2016) and wheat (Soumya et al., 2021). On this basis, we further underlined the RSA was the most impactful contributor to the P uptake capacity, followed by the TRL and RDW under LP treatment through PLS PM (Figure 5). This is mainly because lager RSA and TRL lead to a lager P uptake area, which is conducive to higher P uptake capacity (Liu, 2021). The aforementioned results were rarely reported in peanuts. These findings revealed the changes of root morphology traits among peanut germplasms under LP stress and proposed that root traits mainly influence P uptake capacity.
The plasma membrane-localized Pi transporter in the root regulates the P uptake (Sudhakar et al., 2018; Wang et al., 2018). The high-affinity transporter activity was enhanced after LP treatment, and a low concentration of extracellular P could be transported across the membrane into the cell, which was the main way to absorb P under LP conditions (Sudhakar et al., 2018; Wang et al., 2018; Liao et al., 2019). The transmembrane transfer activity can be improved by increasing the amount of transporter proteins while the unit transporter activity is unchanged. For example, the overexpression of ZmPT7 in maize (Wang et al., 2020), OsPHT1;3 and OsPht1;8 in rice (Jia et al., 2011; Chang et al., 2019), and TaPht1;4 in wheat (Liu et al., 2013) significantly enhanced the P uptake capacity, while the knockout lines represented reduced P uptake capacity. These may be the underlying mechanisms of high P absorption efficiency in some germplasms. Interestingly, we found that P absorption efficiency was positively correlated with P uptake capacity (Table 3; Figure 5). The contributor for the P absorption efficiency component was PUR_RDW > PUR_RSA > PUR_RL > PUR_RV, and the path coefficient of PUR_RDW and PUR_RSA was greater than 0.9. An increase in transporting activity of each transporter protein also increased P uptake capacity. In this study, the P absorption efficiency showed extensive variations among germplasms after LP treatment, but most germplasms significantly increased and showed different increasing ranges (Table 2; Figure 2). We hypothesized that the expression level of Pi transporter-encoding genes or transporting activity of Pi transporter was induced under LP stress in these germplasms. This will be helpful in the identification of high-affinity Pi transporters, selection, and breeding of high P-efficient cultivars.
While both higher P absorption efficiency and root morphology should benefit the P uptake, their contribution varied in different crops, varieties, and P conditions (Lyu et al., 2016; Wen et al., 2019; Honvault et al., 2020). Identification of P efficiency in the field requires heavy workload and a long working cycle and is susceptible to environmental factors. In this study, we found that P absorption efficiency was the critical factor affecting P uptake capacity for peanuts. After LP treatment, the correlation between P uptake capacity and P absorption efficiency (correlation coefficient >0.6) was larger than that between P uptake capacity and root morphology (correlation coefficient <0.5) (Table 3). PLS-PM also proved that the effect of P absorption efficiency on P uptake capacity (coef = 0.868) was greater than that of root morphology (coef = 0.520) (Figure 5). These findings provide new insights into the relationship between P uptake capacity and root morphology/P absorption efficiency and furnish important evaluation indexes for high P-efficient uptake germplasm selection. These results provide a reference for functional gene screening, mechanism studies, and further identification in the field.
Data Availability Statement
The original contributions presented in the study are included in the article/Supplementary Material, further inquiries can be directed to the corresponding authors.
Author Contributions
Conceptualization: YW, KZ and FL; methodology: SZ; software: SZ and LL; validation: SZ, LL and XZ; investigation: SZ, MZ, XW, JZ, XL, QW and LL; resources: YW and KZ; writing—original draft preparation: SZ; writing—review and editing: LL, YW and SZ; visualization: SZ and LL; project administration: YW, KZ and FL; and funding acquisition: KZ and FL. All authors have read and agreed to the published version of the manuscript.
Funding
This research was funded by the National Natural Science Foundation of China (NSFC, 31871561); the Peanut Seed Industry Project in Shandong Province, China (No. 2020LZGC001); the Earmarked Fund for China Agriculture Research System (CARS-13); and the Earmarked Fund for Agriculture Research System in Shandong province, China (SDAIT-04-03).
Conflict of Interest
The authors declare that the research was conducted in the absence of any commercial or financial relationships that could be construed as a potential conflict of interest.
Publisher’s Note
All claims expressed in this article are solely those of the authors and do not necessarily represent those of their affiliated organizations, or those of the publisher, the editors, and the reviewers. Any product that may be evaluated in this article, or claim that may be made by its manufacturer, is not guaranteed or endorsed by the publisher.
Supplementary Material
The Supplementary Material for this article can be found online at: https://www.frontiersin.org/articles/10.3389/fenvs.2022.855815/full#supplementary-material
References
Bello, S. K. (2021). An Overview of the Morphological, Genetic and Metabolic Mechanisms Regulating Phosphorus Efficiency via Root Traits in Soybean. J. Soil Sci. Plant Nutr. 21, 1013–1029. doi:10.1007/s42729-021-00418-y
Bhadoria, P. S., Dessougi, H. E., and Liebersbach, H. (2004). P Uptake Kinetics, Size of Root System and Growth of maize and Groundnut in Solution Culture. Plant Soil 262 (1-2), 327–336. doi:10.1023/b:plso.0000037051.16411.03
Chang, M. X., Gu, M., Xia, Y. W., Dai, X. L., Dai, C. R., Zhang, J., et al. (2019). OsPHT1;3 Mediates Uptake, Translocation, and Remobilization of Phosphate under Extremely Low Phosphate Regimes. Plant Physiol. 179, 656–670. doi:10.1104/pp.18.01097
Claassen, N., and Barber, S. A. (1974). A Method for Characterizing the Relation between Nutrient Concentration and Flux into Roots of Intact Plants. Plant Physiol. 54, 564–568. doi:10.1104/pp.54.4.564
Deng, Y., Teng, W., Tong, Y.-P., Chen, X.-P., and Zou, C.-Q. (2018). Phosphorus Efficiency Mechanisms of Two Wheat Cultivars as Affected by a Range of Phosphorus Levels in the Field. Front. Plant Sci. 9, 1614. doi:10.3389/fpls.2018.01614
Falk, K. G., Jubery, T. Z., O’Rourke, J. A., Singh, A., Sarkar, S., Ganapathysubramanian, B., et al. (2020). Soybean Root System Architecture Trait Study through Genotypic, Phenotypic, and Shape-Based Clusters. Plant Phenomics 2020, 1–23. doi:10.34133/2020/1925495
Gu, R., Chen, F., Long, L., Cai, H., Liu, Z., Yang, J., et al. (2016). Enhancing Phosphorus Uptake Efficiency through QTL-Based Selection for Root System Architecture in maize. J. Genet. Genomics 43, 663–672. doi:10.1016/j.jgg.2016.11.002
Havlin, J. (1999). Soil Fertility and Fertilizers: An Introduction to Nutrient Management. 6th edn. Upper Saddle River, N.J: Prentice-Hall.
Hoagland, D. R., and Arnon, D. J. (1950). The Water-Culture Method for Growing Plants without Soil. Circ. Calif. Agric. Exp. Stn. 347, 32. doi:10.1016/S0140-6736(00)73482-9
Honvault, N., Houben, D., Nobile, C., Firmin, S., Lambers, H., and Faucon, M.-P. (2020). Tradeoffs Among Phosphorus-Acquisition Root Traits of Crop Species for Agroecological Intensification. Plant Soil 461, 137–150. doi:10.1007/s11104-020-04584-3
Jadhav, S. S., and Gowda, M. V. C. (2012). Assessment of Genetic Variation and Selection of Most Responsive Lines for Root Traits in Relation to Phosphorous Nutrition in Groundnut (Arachis hypogaea L.). Indian J. Genet. Pl Br. 72 (4), 439–444. doi:10.1016/j.tree.2012.07.002
Jia, H., Ren, H., Gu, M., Zhao, J., Sun, S., Zhang, X., et al. (2011). The Phosphate Transporter Gene OsPht1;8 Is Involved in Phosphate Homeostasis in Rice. Plant Physiol. 156, 1164–1175. doi:10.1104/pp.111.175240
Kale, R. R., Anila, M., Mahadeva Swamy, H. K., Bhadana, V. P., Senguttuvel, P., Subrahmanyam, D., et al. (2020). Morphological and Molecular Screening of rice Germplasm Lines for Low Soil P Tolerance. J. Plant Biochem. Biotechnol. 30, 275–286. doi:10.1007/s13562-020-00586-5
Knapp, S. J., Stroup, W. W., and Ross, W. M. (1985). Exact Confidence Intervals for Heritability on a Progeny Mean Basis 1. Crop Sci. 25, 192–194. doi:10.2135/cropsci1985.0011183X002500010046x
Krishna, K. R. (1997). Phosphorus Uptake and Utilization Efficiency in Peanut1. Peanut Sci. 24, 1–6. doi:10.3146/i0095-3679-24-1-1
Kumar, A., Pandey, A., and Gowda, M. V. C. (2015). Evaluation of Groundnut Genotypes for Tolerance to Phosphorus Deficiency. J. Plant Nutr. 38, 687–699. doi:10.1080/01904167.2014.936613
Lapis-Gaza, H. R., Jost, R., and Finnegan, P. M. (2014). Arabidopsis Phosphate Transporter1 Genes Pht1;8 and Pht1;9 Are Involved in Root-To-Shoot Translocation of Orthophosphate. BMC Plant Biol. 14 (1), 1–19. doi:10.1186/s12870-014-0334-z
Li, H., Huang, G., Meng, Q., Ma, L., Yuan, L., Wang, F., et al. (2011). Integrated Soil and Plant Phosphorus Management for Crop and Environment in China. A Review. Plant Soil 349, 157–167. doi:10.1007/s11104-011-0909-5
Liao, Y.-Y., Li, J.-L., Pan, R.-L., and Chiou, T.-J. (2019). Structure-Function Analysis Reveals Amino Acid Residues of Arabidopsis Phosphate Transporter AtPHT1;1 Crucial for its Activity. Front. Plant Sci. 10. doi:10.3389/fpls.2019.01158
Liu, C., Su, J., Stephen, G. u. K., Wang, H., Song, A., Chen, F., et al. (2018a). Overexpression of Phosphate Transporter Gene CmPht1;2 Facilitated Pi Uptake and Alternated the Metabolic Profiles of Chrysanthemum under Phosphate Deficiency. Front. Plant Sci. 9, 686. doi:10.3389/fpls.2018.00686
Liu, D. (2021). Root Developmental Responses to Phosphorus Nutrition. J. Integr. Plant Biol.J. Integr. Plant Biol. 63 (6), 1065–1090. doi:10.1111/jipb.13090
Liu, X., Zhao, X., Zhang, L., Lu, W., Li, X., and Xiao, K. (2013). TaPht1;4, a High-Affinity Phosphate Transporter Gene in Wheat (Triticum aestivum), Plays an Important Role in Plant Phosphate Acquisition under Phosphorus Deprivation. Funct. Plant Biol. 40, 329–341. doi:10.1071/FP12242
Liu, Z., Liu, X., Craft, E. J., Yuan, L., Cheng, L., Mi, G., et al. (2018b). Physiological and Genetic Analysis for maize Root Characters and Yield in Response to Low Phosphorus Stress. Breed. Sci. 68, 268–277. doi:10.1270/jsbbs.17083
López-Arredondo, D. L., Leyva-González, M. A., González-Morales, S. I., López-Bucio, J., and Herrera-Estrella, L. (2014). Phosphate Nutrition: Improving Low-Phosphate Tolerance in Crops. Annu. Rev. Plant Biol. 65, 95–123. doi:10.1146/annurev-arplant-050213-035949
Lorenzo‐Orts, L., Couto, D., and Hothorn, M. (2020). Identity and Functions of Inorganic and Inositol Polyphosphates in Plants. New Phytol. 225, 637–652. doi:10.1111/nph.16129
Lynch, J. P., Ho, M. D., and phosphorus, L. (2005). Rhizoeconomics: Carbon Costs of Phosphorus Acquisition. Plant Soil 269 (1-2), 45–56. doi:10.1007/s11104-004-1096-4
Lyu, Y., Tang, H., Li, H., Zhang, F., Rengel, Z., Whalley, W. R., et al. (2016). Major Crop Species Show Differential Balance between Root Morphological and Physiological Responses to Variable Phosphorus Supply. Front. Plant Sci. 7, 1939. doi:10.3389/fpls.2016.01939
Madhuri, K. V. N., Latha, P., Vasanthi, R. P., John, K., Reddy, P. V. R. M., Murali, G., et al. (2018). Evaluation of Groundnut Genotypes for Phosphorus Efficiency through Leaf Acid Phosphatase Activity. Lr. doi:10.18805/lr-3927
Mai, W., Xue, X., Feng, G., Yang, R., and Tian, C. (2018). Can Optimization of Phosphorus Input lead to High Productivity and High Phosphorus Use Efficiency of Cotton through Maximization of Root/mycorrhizal Efficiency in Phosphorus Acquisition? Field Crops Res. 216, 100–108. doi:10.1016/j.fcr.2017.11.017
Manschadi, A. M., Kaul, H.-P., Vollmann, J., Eitzinger, J., and Wenzel, W. (2014). Reprint of "Developing Phosphorus-Efficient Crop Varieties-An Interdisciplinary Research Framework". Field Crops Res. 165, 49–60. doi:10.1016/j.fcr.2013.12.016
Manske, G. G. B., Ortiz-Monasterio, J. I., van Ginkel, M., González, R. M., Fischer, R. A., Rajaram, S., et al. (2001). Importance of P Uptake Efficiency versus P Utilization for Wheat Yield in Acid and Calcareous Soils in Mexico. Eur. J. Agron. 14, 261–274. doi:10.1016/S1161-0301(00)00099-X
McCormack, M. L., Dickie, I. A., Eissenstat, D. M., Fahey, T. J., Fernandez, C. W., Guo, D., et al. (2015). Redefining fine Roots Improves Understanding of Below‐ground Contributions to Terrestrial Biosphere Processes. New Phytol. 207, 505–518. doi:10.1111/nph.13363
Murphy, J., and Riley, J. P. (1962). A Modified Single Solution Method for the Determination of Phosphate in Natural Waters. Analytica Chim. Acta 27, 31–36. doi:10.1016/S0003-2670(00)88444-5
Nestler, J., Keyes, S. D., and Wissuwa, M. (2016). Root Hair Formation in rice (Oryza sativaL.) Differs between Root Types and Is Altered in Artificial Growth Conditions. Exbotj 67, 3699–3708. doi:10.1093/jxb/erw115
Neto, A. P., Favarin, J. L., Hammond, J. P., Tezotto, T., and Couto, H. T. Z. (2016). Analysis of Phosphorus Use Efficiency Traits in Coffea Genotypes Reveals Coffea Arabica and Coffea Canephora Have Contrasting Phosphorus Uptake and Utilization Efficiencies. Front. Plant Sci. 7. doi:10.3389/fpls.2016.00408
Pearse, S. J., Veneklaas, E. J., Cawthray, G. R., Bolland, M. D. A., and Lambers, H. (2006). Carboxylate Release of Wheat, Canola and 11 Grain Legume Species as Affected by Phosphorus Status. Plant Soil 288, 127–139. doi:10.1007/s11104-006-9099-y
Raven, J. A., Lambers, H., Smith, S. E., and Westoby, M. (2018). Costs of Acquiring Phosphorus by Vascular Land Plants: Patterns and Implications for Plant Coexistence. New Phytol. 217, 1420–1427. doi:10.1111/nph.14967
Sandaña, P. (2016). Phosphorus Uptake and Utilization Efficiency in Response to Potato Genotype and Phosphorus Availability. Eur. J. Agron. 76, 95–106. doi:10.1016/j.eja.2016.02.003
Singh, A. L., Chaudhari, V., and Ajay, B. C. (2015). Screening of Groundnut Genotypes for Phosphorus Efficiency under Field Conditions. Ind. Jrnl. Gen. Plnt. Bree. 75 (3), 363–371. doi:10.5958/0975-6906.2015.00057.7
Soumya, P. R., Singh, D., Sharma, S., Singh, A. M., and Pandey, R. (2021). Evaluation of Diverse Wheat (Triticum aestivum) and Triticale (× Triticosecale) Genotypes for Low Phosphorus Stress Tolerance in Soil and Hydroponic Conditions. J. Soil Sci. Plant Nutr. 21 (2), 1236–1251. doi:10.1007/s42729-021-00436-w
Srivastava, S., Upadhyay, M., Srivastava, A., Abdelrahman, M., Suprasanna, P., and Tran, L.-S. (2018). Cellular and Subcellular Phosphate Transport Machinery in Plants. Ijms 19 (7), 1914. doi:10.3390/ijms19071914
Sun, S., Gu, M., Cao, Y., Huang, X., Zhang, X., Ai, P., et al. (2012). A Constitutive Expressed Phosphate Transporter, OsPht1;1, Modulates Phosphate Uptake and Translocation in Phosphate-Replete Rice. Plant Physiol. 159, 1571–1581. doi:10.1104/pp.112.196345
Vejchasarn, P., Lynch, J. P., and Brown, K. M. (2016). Genetic Variability in Phosphorus Responses of rice Root Phenotypes. Rice 9, 29. doi:10.1186/s12284-016-0102-9
Wang, F., Cui, P. J., Tian, Y., Huang, Y., Wang, H. F., Liu, F., et al. (2020). Maize Zmpt7 Regulates Pi Uptake and Redistribution Which Is Modulated by Phosphorylation. Plant Biotechnol. J. 18, 2406–2419. doi:10.1111/pbi.13414
Wang, F., Deng, M., Xu, J., Zhu, X., and Mao, C. (2018). Molecular Mechanisms of Phosphate Transport and Signaling in Higher Plants. Semin. Cel Developmental Biol. 74, 114–122. doi:10.1016/j.semcdb.2017.06.013
Wang, X., Shen, J., and Liao, H. (2010a). Acquisition or Utilization, Which Is More Critical for Enhancing Phosphorus Efficiency in Modern Crops? Plant Sci. 179, 302–306. doi:10.1016/j.plantsci.2010.06.007
Wang, X., Yan, X., and Liao, H. (2010b). Genetic Improvement for Phosphorus Efficiency in Soybean: a Radical Approach. Ann. Bot. 106 (1), 215–222. doi:10.1093/aob/mcq029
Wang, Y.-L., Almvik, M., Clarke, N., Øgaard, A. F., Krogstad, T., Lambers, H., et al. (2015). Contrasting Responses of Root Morphology and Root-Exuded Organic Acids to Low Phosphorus Availability in Three Important Food Crops with Divergent Root Traits. AoB Plants 7 (7), plv097. doi:10.1093/aobpla/plv097
Wen, Z., Li, H., Shen, J., and Rengel, Z. (2017). Maize Responds to Low Shoot P Concentration by Altering Root Morphology rather Than Increasing Root Exudation. Plant Soil 416, 377–389. doi:10.1007/s11104-017-3214-0
Wen, Z., Li, H., Shen, Q., Tang, X., Xiong, C., Li, H., et al. (2019). Tradeoffs Among Root Morphology, Exudation and Mycorrhizal Symbioses for Phosphorus‐acquisition Strategies of 16 Crop Species. New Phytol. 223, 882–895. doi:10.1111/nph.15833
Wissuwa, M., and Ae, N. (2001). Genotypic Variation for Tolerance to Phosphorus Deficiency in rice and the Potential for its Exploitation in rice Improvement. Plant Breed. 120, 43–48. doi:10.1046/j.1439-0523.2001.00561.x
Yuan, Y., Gao, M., Zhang, M., Zheng, H., Zhou, X., Guo, Y., et al. (2017). QTL Mapping for Phosphorus Efficiency and Morphological Traits at Seedling and Maturity Stages in Wheat. Front. Plant Sci. 8, 614. doi:10.3389/fpls.2017.00614
Zhang, L., Chu, Q., Zhou, J., Rengel, Z., and Feng, G. (2021). Soil Phosphorus Availability Determines the Preference for Direct or Mycorrhizal Phosphorus Uptake Pathway in maize. Geoderma 403, 115261. doi:10.1016/j.geoderma.2021.115261
Keywords: peanut germplasms, phosphorus uptake rate, phosphorus uptake capacity, root morphology, phosphorus absorption efficiency
Citation: Zhu S, Luo L, Zhang X, Zhao M, Wang X, Zhang J, Wan Q, Li X, Wan Y, Zhang K and Liu F (2022) Study on the Relationship of Root Morphology and Phosphorus Absorption Efficiency With Phosphorus Uptake Capacity in 235 Peanut (Arachis hypogaea L.) Germplasms. Front. Environ. Sci. 10:855815. doi: 10.3389/fenvs.2022.855815
Received: 16 January 2022; Accepted: 17 March 2022;
Published: 14 April 2022.
Edited by:
Haigang Li, Inner Mongolia Agricultural University, ChinaReviewed by:
Zhihui Wen, China Agricultural University, ChinaYilin Li, Institute of Soil Science (CAS), China
Copyright © 2022 Zhu, Luo, Zhang, Zhao, Wang, Zhang, Wan, Li, Wan, Zhang and Liu. This is an open-access article distributed under the terms of the Creative Commons Attribution License (CC BY). The use, distribution or reproduction in other forums is permitted, provided the original author(s) and the copyright owner(s) are credited and that the original publication in this journal is cited, in accordance with accepted academic practice. No use, distribution or reproduction is permitted which does not comply with these terms.
*Correspondence: Yongshan Wan, eXN3YW5Ac2RhdS5lZHUuY24=; Kun Zhang, a3VuemhAc2RhdS5lZHUuY24=; Fengzhen Liu, bGl1ZnpAc2RhdS5lZHUuY24=
†These authors have contributed equally to this work