- Western Geographic Science Center, United States Geological Survey (USGS), Tucson, AZ, United States
by Gooden J., and Pritzlaff R. (2021). Front. Environ. Sci. 9:679189. doi: 10.3389/fenvs.2021.679189
Introduction
In their paper, the authors describe studies of rock detention structures (RDS) at four properties and create an argument to acknowledge RDS as nature-based solutions (NbS) based on their documented climate mitigation and adaption potential (Gooden and Pritzlaff, 2021). The adoption of national and international strategies to mitigate climate change and accompanying desertification and drought is dependent on decision-makers understanding the significance of impacts, acting on available anticipatory science, and assuring a commitment to financial investments (Bradford et al., 2018). As such in this invited commentary, I provide additional research describing RDS scalability throughout landscapes, perseverance over time, and contributions to a restoration stewardship economy that supports RDS as NbS.
Contemporary studies conducted on RDS span the United States-Mexico border in North America, describe multiple benefits they provide for people (ecosystem services), and are globally applicable to arid land environments (Norman, 2020). The installation of RDS sets biophysical cycles into motion that persist through centuries, providing anticipatory and restorative functions (Norman, 2020). Additionally, RDS allow capacity-building and risk mitigation that can alleviate climate change impacts in socio-environmentally vulnerable regions (Norman et al., 2021a; Norman et al. 2021a; Norman et al., 2021b). RDS provide sustainable NbS to address climate change and can be implemented to protect, sustainably manage, and restore ecosystems (International Union for Conservation of Nature, 2021).
Scalability in Space and Time
Over the past 1,000+ years, human populations in the arid North American Southwest have left archeological evidence of RDS across the landscape, but long-term ecohydrological, demographic and socio-economic drivers and impacts have yet to be explored in an integrative and iterative way (J. Dean, University of Arizona, written communication 11/12/2021; Fish and Fish, 1984; Hall et al., 2013; Norman, 2020). The scaling of RDS-NbS interventions and benefits from local to watershed scales and throughout time requires objective science. Researchers are working with land managers and restoration practitioners to establish experiments at multiple RDS installations throughout the Madrean Archipelago Ecoregion of North America to quantify the effects of RDS-NbS on hydrology, ecology, and soil productivity (Fandel et al., 2016; Norman et al., 2016; Norman et al., 2017; Norman et al., 2019; Norman, 2020; Callegary et al., 2021; Coy et al., 2021; Norman et al., 2021a; Wilson et al., 2021; Freimund et al., 2022).
Quantitative models and remotely sensed imagery expand site-based experiments at RDS into larger regional watersheds and allow forecasting and back-casting to extrapolate over time (Norman L. M. et al., 2010; Norman et al., 2014; Norman and Niraula, 2016; Norman et al., 2017; Wilson and Norman, 2018; Norman et al., 2019; Norman, 2020; Norman, 2021; Norman et al., 2021b; Petrakis et al., 2021; Lara-Valencia et al., 2022). RDS support vital ES that address societal problems, including flood regulation; water regulation, purification, and provisioning; habitat provisioning; erosion regulation, carbon sequestration and storage; social value and climate regulation (Norman, 2020; Norman et al., 2021b). These ES protect biodiversity and mitigate climate change with cumulative and multiplicative feedback effects that sustain their positive impacts in the long term (Norman L. et al., 2010; Norman et al., 2014; Norman et al., 2016; Norman and Niraula, 2016; Norman et al., 2017; Norman et al., 2019; Norman 2020; Norman et al., 2021a; Norman et al., 2021b).
Water and Climate Resilience Infrastructure
The focal paper (Gooden and Pritzlaff, 2021) translates the ecosystem services of rock detention structures (RDS; Norman, 2020) into Nature-based Solutions (NbS) and relates them to sustainable development goals. NbS is a term that is often used to re-frame ecosystem services and green infrastructure projects, to make them more politically palatable (O’Sullivan et al., 2020). Like green infrastructure, RDS rely on vegetation, soils, and natural processes to manage water and create healthier environments. However, unlike green infrastructure, which promotes passive rainwater harvesting to retain water in the built environment, RDS are established to detain water (not retain it) in more remote areas; allowing water to slowly pass through, infiltrate the soils and regenerate landscapes (Norman et al., 2014; Norman et al., 2016; Norman and Niraula, 2016; Norman et al., 2017; Wilson and Norman, 2018; Norman et al., 2019; Norman 2020; Norman et al., 2021a; Norman et al., 2021a; Norman et al., 2021b).
Example: Costs and Benefits
Costs: Check Dams
One of the disadvantages to using RDS is the associated costs and investment needed to secure them. It takes an average of 16 h of labor to install a 1-m-high RDS (check dam; Figure 1; J. T. Austin, former owner El Coronado Ranch, oral communication, 12/17/2021; Norman et al., 2022). Approximately 2000 RDS are installed on a remote, 769-ha forested watershed in southeastern Arizona (Norman and Niraula, 2016). Including current wages, equipment, and associated estimates, it costs approximately ∼$2,210 per ha to treat a watershed based on this established rate (∼2.6 check dams/ha; Callegary et al., 2021).
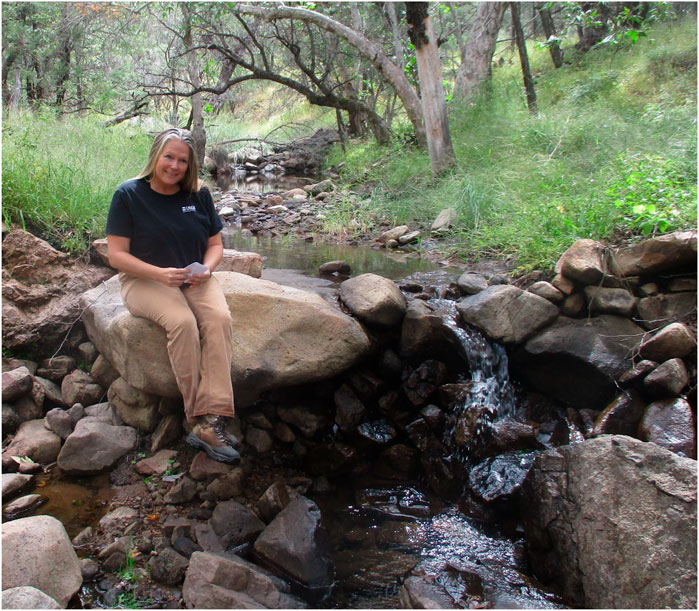
FIGURE 1. Author sitting on a 30-year-old check dam installed at the El Coronado Ranch study area, Chiricahua Mountains, southeastern Arizona, United States (photo by Gerry Norman, Oct. 2021).
In this example, I use back-of-the-envelope calculations to roughly extrapolate how much it would cost to preemptively treat all Federal and Tribal riparian areas in the state of Arizona (33,182 ha) with check dams. This ballpark figure entails installing 86,273 check dams with an estimated cost of $73M. For comparison, climate-related disasters in Arizona spurred Legislation of $100M for recovery and support efforts to help deal with damages related to post-fire flooding in 2021 (Office of the Governor, 2021).
Benefits: Ecosystem Services, Economics, and Equity
There are a lot of advantages to be realized from using RDS. Thomas et al. (2016) estimated the economic impacts of restoration associated with Federal lands, to be between 13–32 job-years and $2.2-$3.4M for every $1M spent.
In the example provided above for costs and using economic impacts of restoration documented by Thomas et al. (2016), I estimate the potential return on this ecological restoration investment scenario of installing check dams in Federal riparian areas ($73M) to produce the equivalent of >1,000 job-years and >$160M of economic output to local, regional, and national economies. And, given the durability of RDS, with some ongoing investment in repair and maintenance, the initial investment will provide long-term benefits (Norman L. M. et al., 2010; Norman 2020; Norman et al., 2021a; Norman et al., 2021b).
Based on targeted science research and results of the Aridland Water Harvesting Study (Norman, 2020; Norman et al., 2021a; Norman et al., 2021b), this example scenario (to install ∼86,273 check dams in Federal riparian areas of Arizona) could also:
✓ sequester ∼7.5 M tons [0.0075 Pg (7.5 Tg)] of atmospheric C in the soil storage (Norman and Niraula, 2016; Callegary et al., 2021);
✓ maintain or increase vegetation and biomass—with extended growing seasons and using stored soil moisture (Norman et al., 2014; Wilson and Norman, 2018; Wilson et al., 2021), further increasing C sequestration;
✓ extend ephemeral duration and surface-water availability (Norman et al., 2016; Norman and Niraula, 2016; Norman et al., 2017);
✓ mitigate floods and associated emergency response expenditures (Norman L. M. et al., 2010; Norman L. et al., 2010; Norman and Niraula, 2016; Norman et al., 2021b; Freimund et al., 2022);
✓ promote lateral flows and onsite storage of water (Fandel et al., 2016; Fandel, 2016; Norman et al., 2016; Norman et al., 2019);
✓ control erosion and nonpoint source pollution, improving water quality (Norman L. M. et al., 2010; Norman L. et al., 2010; Norman and Niraula, 2016; Norman et al., 2017; Norman et al., 2019); and
✓ reduce ambient temperatures (Norman et al., 2021).
Water-related ES in drylands also increases the quality of life for socio-environmentally vulnerable communities (Norman L. et al., 2010, Norman et al., 2012; Norman et al., 2013; Villarreal et al., 2013). A restoration economy, based on improved hydrology in degraded waterways and associated riparian areas, can also be created that improve lives and livelihoods in restored areas (Adams, 2016; Norman et al., 2021a; Norman et al., 2022).
Discussion
Over the past decade, a multi-disciplinary landscape-scale study has quantified anticipatory and restorative watershed functions of Rock Detention Structures (RDS) installed in the Madrean Archipelago Ecoregion of North America (Norman, 2020). In this commentary, I reference these larger temporal and spatial study extents to underpin RDS interventions as Nature-based Solutions (NbS) as presented in Gooden and Pritzlaff (2021), that restore dryland channels with impartial shares of social, environmental, and economic benefits.
Our planet needs solutions to mitigate impacts from our rapidly changing climate, and the ecosystem services of RDS justify the inclusion as NbS, useful to increase carbon storage and sequestration, increase water quality and quantity, buffer flood events, improve vegetation health and biodiversity, and help address global warming (Norman 2020; Norman et al., 2021a; Norman et al., 2021b). The focal paper proposes RDS as a feasible, cost-effective NbS that can contribute to climate mitigation (Gooden and Pritzlaff, 2021).
RDS-NbS are already being used to enhance preparedness, response, and resilience in vulnerable communities along the United States-Mexico border, where a restoration stewardship economy fosters hope, nourishes livelihoods, and establishes valuable ecosystem services via grassroots efforts (Norman et al., 2021a). Investments in RDS-NbS avert risk, damage, and cost associated with drought and flooding (Norman L. et al., 2010; Norman et al., 2014; Norman et al., 2016; Norman et al., 2019; Norman et al., 2021b). Moreover, capital appreciation, described herein, can double or triple restoration investments on Federal lands (Thomas et al., 2016), RDS-NbS provide a huge suite of benefits, and the structures can persist for millennium (Norman 2020).
Author Contributions
The author confirms being the sole contributor of this work and has approved it for publication.
Funding
Funding for this commentary was provided by the Land Change Science Program of the U.S. Geological Survey. Any use of trade, firm, or product names is for descriptive purposes only and does not imply endorsement by the U.S. Government.
Conflict of Interest
The author declares that she was an adviser of and has co-authored with Dr. Pritzlaff, who also contributed funding to some of the restoration work studied herein.
Publisher’s Note
All claims expressed in this article are solely those of the authors and do not necessarily represent those of their affiliated organizations, or those of the publisher, the editors, and the reviewers. Any product that may be evaluated in this article, or claim that may be made by its manufacturer, is not guaranteed or endorsed by the publisher.
Acknowledgments
The author thanks all the partners in the Sky Island Restoration Collaborative and appreciates reviews by Julio Betancourt and Michele Girard.
References
Adams, A. M. (2016). Restoration Economy” Strives to Protect Pollinators, Create Jobs. Reston, VA: Scientific American. Available at: https://www.scientificamerican.com/article/ldquo-restoration-economy-rdquo-strives-to-protect-pollinators-create-jobs/.
Bradford, J. B., Betancourt, J. L., Butterfield, B. J., Munson, S. M., and Wood, T. E. (2018). Anticipatory Natural Resource Science and Management for a Changing Future. Front. Ecol. Environ. 16, 295–303. doi:10.1002/fee.1806
Callegary, J. B., Norman, L. M., Eastoe, C. J., Sankey, J. B., and Youberg, A. (2021). Preliminary Assessment of Carbon and Nitrogen Sequestration Potential of Wildfire-Derived Sediments Stored by Erosion Control Structures in Forest Ecosystems, Southwest USA. Air Soil Water Res. 14, 117862212110017. doi:10.1177/11786221211001768
Coy, H. A., Wilson, N. R., Bennett, A. F., Hsieh, D., and Norman, L. M. (2021). Hydrologic Data Collected at Leaky Weirs, Cienega Ranch. Willcox, AZ: U.S. Geological Survey Data Release. March 2019 - October 2020).
Fandel, C. A. (2016). The Effect of Gabion Construction on Infiltration in Ephemeral Streams (Master of Science, Hydrology). Tucson, Ariz: The University of Arizona. Available at: https://repository.arizona.edu/handle/10150/622852.
Fandel, C., Callegary, J. B., Ferré, T. P. A., Norman, L. M., and Scott, C. A. (2016). Infiltration in Ephemeral Streams: Quantifying the Effect of Gabions on Vertical Water Flux Using Wildlife Cameras & Temperature Sensors, in Water Resources Research Center Annual Conference, “#AZwaterfuture: Tech, Talk, and Tradeoffs”, Tucson, Arizona.
Fish, P. R., and Fish, S. K. (1984). “Agricultural Maximization in the Sacred Mountain Basin, Central Arizona,”In Prehistoric Southwest. Agric. Strateg. Editors S. K. Fish, and P. R. Fish (Tempe, Arizona: Arizona State University Anthropological Research Papers No.), 33, 147–159.
Freimund, C. A., Garfin, G. M., Norman, L. M., Fisher, L. A., and Buizer, J. L. (2022). Flood resilience in paired US–Mexico border cities: a study of binational risk perceptions. Nat. Hazards. doi:10.1007/s11069-022-05225-x
Gooden, J., and Pritzlaff, R. (2021). Dryland Watershed Restoration With Rock Detention Structures: A Nature-Based Solution to Mitigate Drought, Erosion, Flooding, and Atmospheric Carbon. Front. Environ. Sci. 9, 679189. doi:10.3389/fenvs.2021.679189
Hall, S. J., Trujillo, J., Nakase, D., Strawhacker, C., Kruse-Peeples, M., Schaafsma, H., et al. (2013). Legacies of Prehistoric Agricultural Practices Within Plant and Soil Properties Across an Arid Ecosystem. Ecosystems 16, 1273–1293. doi:10.1007/s10021-013-9681-0n.org/review/853684/16/1304572
International Union for Conservation of Nature (2021). Nature-based Solutions. [WWW Document]. URL Available at: https://www.iucn.org/commissions/commission-ecosystem-management/our-work/nature-based-solutions.
Lara-Valencia, F., Garcia, M., Norman, L. M., Anides Morales, A., and Castellanos-Rubio, E. E. (2022). Integrating Urban Planning and Water Management Through Green Infrastructure in the United States-Mexico Border. Front. Water. 4, 782922. doi:10.3389/frwa.2022.782922
Norman, L., Callegary, J., Lacher, L., Wilson, N., Fandel, C., Forbes, B., et al. (2019). Modeling Riparian Restoration Impacts on the Hydrologic Cycle at the Babacomari Ranch, SE Arizona, USA. Water. 11, 381. doi:10.3390/w11020381
Norman, L. M., Girard, M. M., Pulliam, H. R., Villarreal, M. L., Clark, V., Flesch, A. D., et al. (2022). A Shared Vision for Enhancing Ecological Resilience in the U.S. - Mexico Borderlands: The Sky Island Restoration Collaborative. Society magazine SERNews.
Norman, L. M. (2021). International Watersheds Coping with Climate Hazards; Twin-City Solutions at Ambos Nogales and San Diego–Tijuana. Available at: https://arizona.app.box.com/s/597xsyavqspzgbp4t9sclgba8unpa9bz.
Norman, L. M., Brinkerhoff, F., Gwilliam, E., Guertin, D. P., Callegary, J., Goodrich, D. C., et al. (2016). Hydrologic Response of Streams Restored with Check Dams in the Chiricahua Mountains, Arizona. River Res. Applic. 32, 519–527. doi:10.1002/rra.2895
Norman, L. M., Caldeira, F., Callegary, J., Gray, F., O’ Rourke, M. K., Meranza, V., et al. (2012). Socio-Environmental Health Analysis in Nogales, Sonora, Mexico. Water Qual. Expo. Health. 4, 79–91. doi:10.1007/s12403-012-0067-x
Norman, L. M. (2020). Ecosystem Services of Riparian Restoration: A Review of Rock Detention Structures in the Madrean Archipelago Ecoregion. Air Soil Water Res. 13, 117862212094633. doi:10.1177/1178622120946337
Norman, L. M., Levick, L., Guertin, D. P., Callegary, J., Guadarrama, J. Q., Anaya, C. Z. G., et al. (2010). Nogales Flood Detention Study. U.S. Geol. Surv. Open-File Rep. 2010, 112. doi:10.3133/ofr20101262
Norman, L., Tallent-Halsell, N., Labiosa, W., Weber, M., McCoy, A., Hirschboeck, K., et al. (2010). Developing an Ecosystem Services Online Decision Support Tool to Assess the Impacts of Climate Change and Urban Growth in the Santa Cruz Watershed; Where We Live, Work, and Play. Sustainability 2, 2044–2069. doi:10.3390/su2072044
Norman, L. M., and Niraula, R. (2016). Model Analysis of Check Dam Impacts on Long-Term Sediment and Water Budgets in Southeast Arizona, USA. Ecohydrology & Hydrobiology. 16, 125–137. doi:10.1016/j.ecohyd.2015.12.001
Norman, L. M., Pulliam, H. R., Girard, M. M., Buckley, S. M., Misztal, L., Seibert, D., et al. (2021a). Editorial: Combining the Science and Practice of Restoration Ecology-Case Studies of a Grassroots Binational Restoration Collaborative in the Madrean Archipelago Ecoregion (2014-2019). Air Soil Water Res. 14, 117862212110094. doi:10.1177/11786221211009478
Norman, L. M., Ruddell, B. L., Tosline, D. J., Fell, M. K., Greimann, B. P., and Cederberg, J. R. (2021b). Developing Climate Resilience in Aridlands Using Rock Detention Structures as Green Infrastructure. Sustainability 13, 11268. doi:10.3390/su132011268
Norman, L. M., Sankey, J. B., Dean, D., Caster, J., DeLong, S., DeLong, W., et al. (2017). Quantifying Geomorphic Change at Ephemeral Stream Restoration Sites Using a Coupled-Model Approach. Geomorphology 283, 1–16. doi:10.1016/j.geomorph.2017.01.017
Norman, L., Villarreal, M., Niraula, R., Meixner, T., Frisvold, G., and Labiosa, W. (2013). Framing Scenarios of Binational Water Policy with a Tool to Visualize, Quantify and Valuate Changes in Ecosystem Services. Water 5, 852–874. doi:10.3390/w5030852
Norman, L., Villarreal, M., Pulliam, H. R., Minckley, R., Gass, L., Tolle, C., et al. (2014). Remote Sensing Analysis of Riparian Vegetation Response to Desert Marsh Restoration in the Mexican Highlands. Ecol. Eng. 70, 241–254. doi:10.1016/j.ecoleng.2014.05.012
O'Sullivan, F., Mell, I., and Clement, S. (2020). Novel Solutions or Rebranded Approaches: Evaluating the Use of Nature-Based Solutions (NBS) in Europe. Front. Sustain. Cities 2, 572527. doi:10.3389/frsc.2020.572527
Office of the Governor, D. D. (2021). PRIMER: Gila County Powers Through Wildfire. Flooding Recovery [WWW Document]. URL Available at: https://azgovernor.gov/governor/news/2021/09/primer-gila-county-powers-through-wildfire-flooding-recovery.
Petrakis, R. E., Norman, L. M., Vaughn, K., Pritzlaff, R., Weaver, C., Rader, A., et al. (2021). Hierarchical Clustering for Paired Watershed Experiments: Case Study in Southeastern Arizona, U.S.A. Water 13, 2955. doi:10.3390/w13212955
Thomas, C. C., Huber, C., Skrabis, K., and Sidon, J. (2016). Estimating the Economic Impacts of Ecosystem Restoration—Methods and Case Studies. U.S. Geol. Surv. Open-File Rep. 2016-1016 98. doi:10.3133/ofr20161016
Villarreal, M. L., Norman, L. M., Boykin, K. G., and Wallace, C. S. A. (2013). Biodiversity Losses and Conservation Trade-Offs: Assessing Future Urban Growth Scenarios for a North American Trade Corridor. Int. J. Biodiversity Sci. Ecosystem Serv. Management 9, 90–103. doi:10.1080/21513732.2013.770800
Wilson, N. R., Norman, L. M., Mount, A. C., Bennett, A., and Simpson, A. (2021). Short Term Vegetation Response Study at Watershed Restoration Structures in Southeastern Arizona, 2015 - 2019. U.S. Geological Survey Data Release.
Keywords: ecosystem services, natural climate solution, erosion control structures, riparian, restoration, climate adaptation and mitigation, resilience
Citation: Norman LM (2022) Commentary: Dryland Watershed Restoration With Rock Detention Structures: A Nature-Based Solution to Mitigate Drought, Erosion, Flooding, and Atmospheric Carbon. Front. Environ. Sci. 10:853684. doi: 10.3389/fenvs.2022.853684
Received: 12 January 2022; Accepted: 25 February 2022;
Published: 01 April 2022.
Edited by:
Borja Gonzalez Reguero, University of California, Santa Cruz, United StatesReviewed by:
George Nick Zaimes, International Hellenic University, GreeceThomas Sisk, Northern Arizona University, United States
Copyright © 2022 Norman. This is an open-access article distributed under the terms of the Creative Commons Attribution License (CC BY). The use, distribution or reproduction in other forums is permitted, provided the original author(s) and the copyright owner(s) are credited and that the original publication in this journal is cited, in accordance with accepted academic practice. No use, distribution or reproduction is permitted which does not comply with these terms.
*Correspondence: Laura M. Norman, bG5vcm1hbkB1c2dzLmdvdg==