- 1Department of Fish and Wildlife Conservation, Virginia Tech, Blacksburg, Blacksburg, VA, United States
- 2Interdisciplinary Program of EcoCreative, Ewha Womans University, Seoul, South Korea
- 3Laboratory of Animal Behaviour and Conservation, College of Biology and the Environment, Nanjing Forestry University, Nanjing, China
Biodiversity is declining worldwide with habitat loss and climate change being among the main threats. While it is easy to quantify habitat loss, the impacts of climate change are less obvious. It is therefore important to understand species habitat use and breeding phenology before a significant shift results in the loss of knowledge. Here, we determined the habitat use and breeding phenology for all Korean amphibian species based on citizen science (8,763 observations), collected between 1997–2020. We found the breeding seasons as we defined them to be generally shorter than described in the literature despite large variations between species. Species were further dichotomised into early and late breeders with breeding periods peaking in mid-March and mid-June respectively. We found early breeding species to have a shortened hibernating period with only six days being consistently devoid of observations over the 23 years of the period studied for the species with the shortest inactive season. Habitat use was significantly different between all species, with pair-wise comparisons highlighting greater differences among rather than within genera, highlighting the threats to species across all genera. In addition, our results set a baseline for future analyses about climate change and habitat use.
Introduction
The annual life cycle of amphibians is regulated by a large number of both biotic and abiotic variables (e.g., Wells, 2010). The breeding phenology is one of the most important regulators in the life cycle of amphibians and it is closely linked to environmental conditions such as temperature, photoperiod, lunar cycle, precipitation and landscapes (Salvador and Carrascal, 1990; Llorente et al., 2006; Grant, 2012; Green, 2017; Plenderleith et al., 2018; Lannoo and Stiles, 2020). The fluctuations and alterations in the interactions of these variables can cause small shifts on a year-to-year basis, resulting in large variations over longer periods (e.g., Lunghi et al., 2018). In addition, species have shown significant phenological shifts due to global warming (Carroll et al., 2009; Green, 2017). These differences in phenological shifts in combination with climate change play a role in global amphibian declines (Stuart et al., 2004). Furthermore, abiotic factors and biotic factors also directly influence the life cycle of amphibians. For instance, habitat alteration, fragmentation, and destruction have a significant impact on the phenological shifts of amphibians (Borzée et al., 2018a; Polat and Başkale, 2018).
Studies focusing on the breeding phenology of Korean amphibians usually refer to single breeding seasons, populations or areas (e.g., Borzée et al., 2017; Groffen, Borzée & Jang, 2018; Kim et al., 2020), which often do not represent the full extent of a species’ population. As a result, these studies provide a shorter or biased representation of the natural history of a species breeding across its full range and for multiple years (e.g., Yoo & Jang, 2012; Do et al., 2020; 2021). This shortcoming is due to logistics, time, and funding. Therefore, an easier way to collect long-term breeding phenology data is needed.
An alternative way to obtain this type of long-term phenology data is through citizens science. There are different citizens science platforms where anyone can upload observations of organisms and those get reviewed by peers. Acquiring data from citizen science platforms is not new, and the practice has incredibly expanded over the years (Gray et al., 2017; McKinley et al., 2017). It provides considerable benefits such as lower costs and simultaneous data collection in numerous geographic areas, and it enables citizens to be involved in the scientific process. Most importantly, citizen science generally results in much larger datasets in terms of species records (Lintott et al., 2010) which are of high quality when conducted under specific guidelines (Shirk et al., 2012; Gray et al., 2017). Drawbacks can be generalized by the risk of biased, incomplete, or unreliable data (Cohn, 2008; Dickinson et al., 2010; Conrad and Hilchey, 2011), and thus they should be manually corrected prior to analyses, either through the organized scrutiny of validators, or through general overview by other members of the citizen science community.
The purpose of the current study was to determine the breeding seasons and landscape requirements of all amphibian species from the Republic of Korea based on citizen science data and complemented by data from surveys conducted at the national scale by governmental organisations (Kim et al., 2021). With the accelerating changes in climate and environment it is critical to understand their impact on the breeding activity and phenology of amphibians (Blaustein et al., 2010; Walls et al., 2013; Cohen et al., 2018). This multi-year phenology data provides an important baseline for future studies on phenology shifts due to changing climate and landscapes.
Material and methods
Species
There are currently 22 native and one invasive amphibian species described in the Republic of Korea (although results for the newly described Onychodactylus species are not presented in this study due to the recent description; Borzée et al., 2020), accounting for 14 anurans and nine caudata (Table 1). All anurans are generally widespread, with the exception of Dryophytes suweonensis and D. flaviventris, which are restricted to agricultural wetlands on the western coast of the country (Kim et al., 2019; Borzée et al., 2020a), the newly described Onychodactylus species, and some of the southern Hynobius species, which are microendemics (Borzée et al., 2020; Borzée and Min, 2021). Other species such as Pelophylax chosenicus and Bufo stejnegeri are restricted to specific landscapes, but occur over a generally large area and locally abundant in some populations (Matsui, 2004; IUCN SSC Amphibian Specialist Group, 2020). Here, the extent of distribution is important as it directly correlates with the probability of observation by citizen scientists.
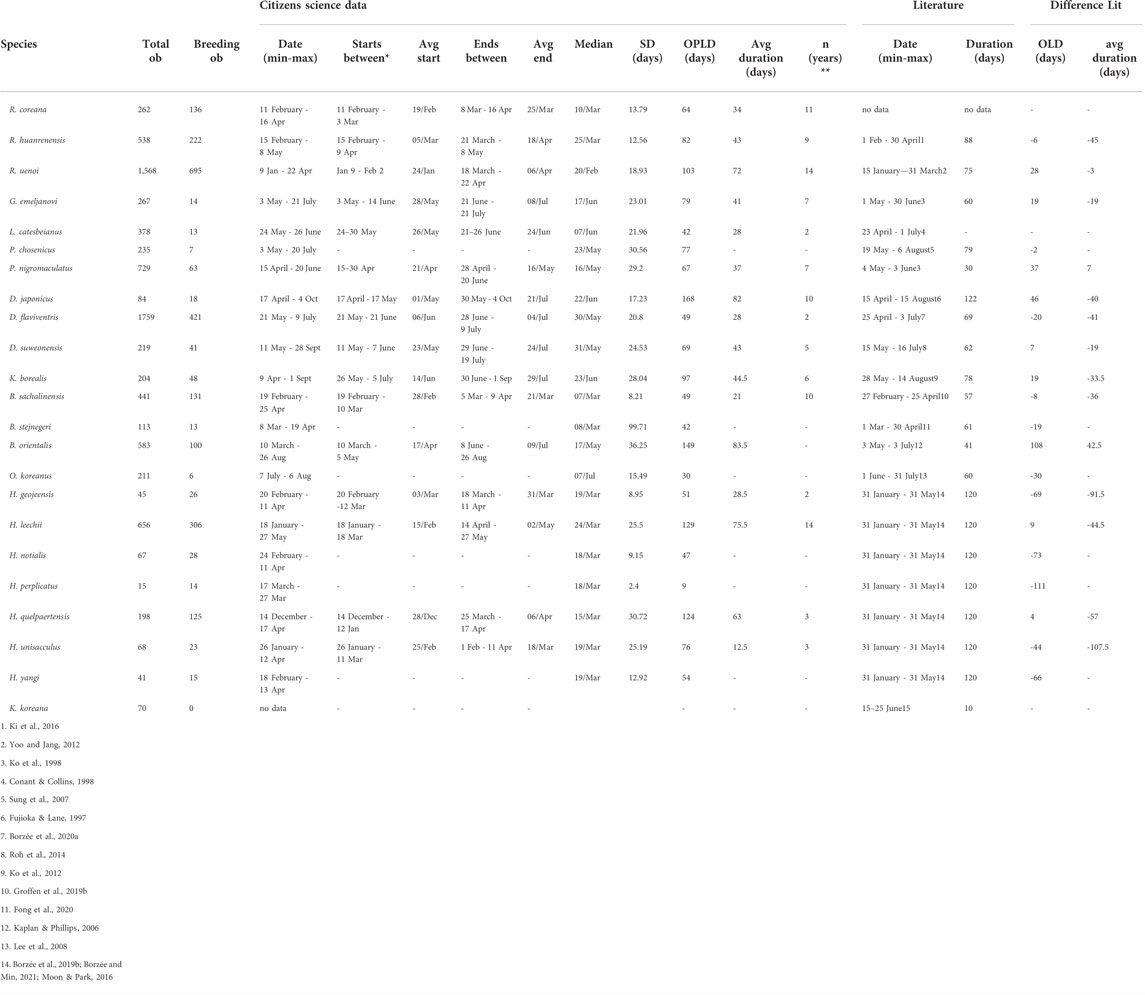
TABLE 1. Breeding phenology start/end dates, min and maximum duration, overall longest duration (OLD), and average durations based on citizens science data and the widest duration in literature. Also, the days difference in duration of the OLD and average breeding phenology between citizens science and literature (minus is days less in citizens science data compared to literature). Lithobates catesbeianus is invasive in Korea and breeding data from literature is from native range, and the data for Bufo stejnegeri is for the oviposition period only. The analysis is based on 8,763 citizen science observations collected between 02 March 1997 and 01 December 2020 downloaded from the platform iNaturalist. *as the first breeding date changes from year to year, this represents the range when the species start breeding. ** Years with more than three datapoints and used to calculate the starts/end periods and average duration.
Most Korean anuran species are generally abundant, with the exception of D. suweonensis and D. flaviventris that are likely to be critically endangered in the near future (Borzée, 2020), and B. stejnegeri for which decline is not documented due to the occurrence of the species at high elevation, and only in undisturbed areas. Lithobates catesbeianus (American bullfrog) is an invasive species present in most regions (Groffen et al., 2019a) but more abundant in the south west of the country (Kang et al., 2019). Among the caudata, Hynobius leechii is the only widespread species (Borzée and Min, 2021), with the relatively abundant Karsenia koreana restricted to mid elevations (Borzée et al., 2019a) and the widespread Onychodactylus koreanus restricted to high elevations (Poyarkov et al., 2014; Shin et al., 2021). All other Hynobius species are distributed in ranges <5,000 km2 (Borzée and Min, 2021) and have a strong connection to agricultural wetlands as the species mostly breed in fallow rice paddies (Do et al., 2020), with the exception of H. perplicatus which is restricted to a geographic area containing no rice paddies.
Data collection
The data for the breeding behavioural analysis was extracted from the citizen science database iNaturalist (https://www.inaturalist.org), a platform where anyone is able to upload observations of organisms. As of 1 December 2020, there were 10,213 amphibian observations on iNaturalist in the Republic of Korea, first observation 02 March 1997. The data is curated by users, with observations reaching a ‘research grade’ when more than two-thirds of the identifiers agree on a taxon, which generally prevents the misidentification of species, and can be updated at any time. Accordingly, our analyses required observations to have reached the ‘research grade’ level. We therefore curated the database for quality prior to data download, adding and correcting the species identification when needed. However, because of the platform’s rule mentioning that 2/3rd of voters must agree on the taxa before an observation is listed as ‘research grade’, the species identifications by multiple users for some observations brought the observation to the genus level, and these observations were therefore not used for the analyses.
To download the data, a query for observations was created with the filters for all amphibians, ‘South Korea’, and only ‘research grade’. The citizen science datapoints were then downloaded through iNaturalist (www.inaturalist.org/observations/export), filtered for observations dated up to 01 December 2020. Each observation was then binary qualified (presence/absence) for the categories ‘calling’, ‘amplexus’, ‘eggs’, ‘tadpoles’, ‘metamorph’, ‘adult’, and ‘death’. Only observations of ‘amplexus’, ‘eggs’, and ‘calling’ were used to describe the breeding season. On iNaturalist, it is possible to upload multiple media within a single observation, and every picture and sound file was checked for the categories described above. As a result, one observation can be coded positive for multiple categories. When there was a sound file uploaded with calls for an observation, both ‘adult’ and ‘calling’ were marked as present. When on a photo, an individual had a full vocal sac and was calling, both ‘adult’ and ‘calling’ were marked as present.
Observations of Bufo stejnegeri in amplexus (n = 28) could not be used in this study to determine their breeding season, because of their unique breeding behaviour, where males and females enter the stream between September and November and immediately amplex and stay in amplexus for 3–6 months in frozen-over streams until spring spawning (Lee and Park, 2009; Fong et al., 2020). In addition, there were not enough observations of eggs (n = 3) to determine the breeding season of B. stejnegeri. Furthermore, we could not determine the breeding season for Karsenia koreana (n = 62) due to a lack of breeding data points (n = 0). We also removed two data points for Rana uenoi as the dates of the observations and photographs conflicted.
Literature comparison
To compare citizen science data and previous breeding knowledge, we reviewed the literature for the breeding seasons of amphibians in the Republic of Korea. We used the widest published range in dates for the breeding season of a given species from the literature for comparison (Table 1). Detailed description of the breeding seasons is uncommon in the literature and nonspecific descriptions such as early or late in the month are used, along with unclear boundaries such as ‘from May until June’. For parametric analysis purposes, using the month of May as an example here, we transformed ‘early May’ to ‘May 3’, ‘mid-May’ to ‘May 15’, ‘late May’ to ‘May 23’, and ‘between May and June’ to ‘May 1—June 30’.
Data encoding
While we acknowledge the presence of latitudinal variations in breeding phenology and ecological requirements of species within the Korean peninsula (Andersen et al., 2022), we did not consider the variation large enough to impact the results of this study. In addition, we do not expect a significant gradient due to the small size of the country and creating bins would result in too small of a dataset to be meaningful for some species at some of the elevation bins. To use parametric statistical analyses, we converted the calendar date of each observation into the Julian date. In terms of activity patterns and hibernation, the conversion did not create any difficulty as day 1 is conveniently located during the hibernation period for most species. As a result, cold resistant species such as O. koreanus were active close to day 365. This encoding however resulted in difficulties for the analysis of species breeding before 01 January, such as Rana uenoi breeding in December at low latitudes, or B. stejnegeri amplexing before winter, and thus artificially resulting in a binomial distribution of the data. To solve this problem, we negatively encoded dates matching with breeding activity before January 01, in continuity with the ‘positive’ Julian dates given from 01 January. All Julian dates were attributed following the non-bissextile calendar.
Analyses of the breeding phenology
We tested the normal distribution of the breeding dataset for each species independently through a Shapiro-Wilk test. The data almost reached significance for a normal distribution for all species (0.29 ≤ D(6,695) ≤ 0.98, p > 0.049), and we decided to ignore the minor deviation due to the robustness of the analyses downstream, and the absence of violation for the other assumptions tested. To test for difference in breeding season between species, we adapted the analyses of duration from Chazard et al. (2017) to our dataset using a linear regression with the Julian breeding date as independent variable and species as dependent variable. The assumptions were fulfilled for this analysis: we detected one outlier for the following species: Dryophytes suweonensis, H. leechii, Kaloula borealis, Pelophylax nigromaculatus and Rana uenoi but decided to ignore them as they did not influence the results of the model. In addition, there was a linear relationship between the continuous independent variables and the logit transformation of the dependent variable, tested through the Box-Tidwell (Box and Tidwell, 1962) procedure with Bonferroni corrections (Tabachnick and Fidell, 2014).
Analyses of the breeding types
Classifying species into breeding types provides a framework for understanding how species respond to environmental conditions (Plenderleith et al., 2018). We adjusted the four breeding strategies defined by Plenderleith et al. (2018) to our climate: explosive, prolonged, and seasonal (winter and summer) breeders. We also adjusted the setting so that a species can fall into several categories, for instance prolonged and winter breeder. To determine the presence of explosive breeders, we restricted our dataset to species with at least 100 breeding observations, an arbitrary threshold ensuring that the short breeding period is related to the biology of the species and not the absence of data. We defined species as explosive breeders if their breeding season was short (Plenderleith et al., 2018), here defined if the totality of the breeding behaviour was confined to 50 days, also an arbitrary threshold to ensure an adequate representation of the breeding phenology. For instance, even for species where mating is restricted to a few days, males can be found waiting for females for up to 2 weeks and stay at the water body for about the same amount of time after breeding.
Seasonal breeders were defined as present at the water body in either winter or summer for a minimum of 30 and a maximum of 120 days, so that several waves of breeding individuals could be included within the breeding period, generally as a function of environmental variables (Llusia et al., 2013; Borzée et al., 2020b). These thresholds were selected since a shorter period than 30 days would be representative of an incomplete dataset and 120 days is the length of a meteorological season. We defined winter breeders as species with their breeding season including sections of the 2 months before or after the winter solstice (21 December, day 355 in the Julian calendar), and summer breeders as species with their breeding season including sections of the 2 months before or after the summer solstice (21 June, day 172 in the Julian calendar). Finally, the prolonged breeders were species with a breeding period longer than 120 days, excluding outliers based on the fact that abiotic variables are likely to display a significant change over the 3 months of a meteorological season, highlighting the disconnect between breeding behaviour and the environment. To determine the average breeding phenology of the species, we used only the years with at least three breeding datapoints. The longest possible duration is the number of days between the earliest breeding observation and latest breeding observation in any given year of a species. We analysed the breeding behaviour through descriptive statistics for each of the species independently, and for each of the breeding behaviours defined above.
Analyses of the inactive period
Here we define the inactive period, related to the putative hibernation period, as the period when individuals were not observed. For a few observations abnormally early or late in the year, we directly verified on the iNaturalist platform and removed the datapoints where individuals were obviously disturbed during their inactive period (n = 6). We removed observations from 1 to 6 November for D. suweonensis as the individuals were clearly buried and in their hibernaculum (Borzée et al., 2019c). This resulted in the removal of six datapoints and a 9-day shift in the latest date at which the species was active. The onset of the inactive period could not be determined for H. geojeensis, H. yangi and H. perplicatus due to the absence of datapoints in summer and fall, likely related to the fossorial behaviour of the species.
Analyses of habitat use
For the landscape analysis, we relied on the same datapoints extracted from iNaturalist with their geocoordinates, as well as the datapoints from the National Institute of Ecology (Kim et al., 2021), and loaded them into ArcMap 10.6.1 (ESRI, Redlands, California, United States). We extracted land cover data from the World Land Cover 30 m BaseVue 2013 (MDAUS) layer within a 50 m buffer centred on each observation using the Tabulate Area tool in ArcGIS Pro 2.8.0 (ESRI, Redlands, CA, United States). We obtained 22 land categories as the raster layer contains 22 landcover classes, and we re-assigned each category into one of the five categories selected for our study (Table 2). Finally, we converted the area values for each landcover class into percentages of total area.
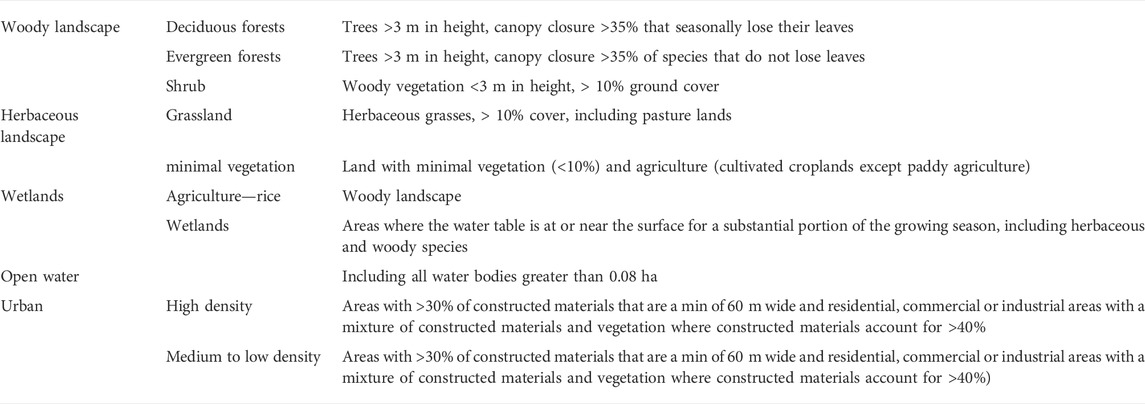
TABLE 2. The different habitat (sub)categories and their descriptions used to differentiate the landscape use between all amphibian species in the Republic of Korea.
All the variables (n = 55,222) were significantly distributed (Kolmogorov-Smirnov test, 0.24 < K < 0.53; df = 55,222; p < 0.001). To determine differences in habitat use between the species we analysed the dataset using a one-way ANOVA with the five categories as dependent variable and species as independent variable. We then used a pair-wise comparison post-hoc test to determine differences between species. All biostatistics analyses were ran in SPSS 21.0 (SPSS, Inc., Chicago, IL, United States) and graphs were plotted in R version 3.0.1 (RStudio Team, 2020) using the package gghalves (Tiedemann, 2020).
Results
Breeding phenology
We found evidence of breeding related behaviours in 28% (n = 2,465) of the 8,763 observations downloaded from iNaturalist for use in this study. Within anurans, we identified two main breeding seasons, the first one starting in January ₋ February (Rana spp.), and the second one in May (Figure 1 and Tables 1, 3). A different pattern was visible for caudata, with all species for which data was available breeding between February and May (Figure 1). The model for the linear regression used to determine differences between species was significant (t = −31.73, p < 0.001) and the breeding season was significantly different between each species pair (χ2 = 53,836.63, df = 1, F = 1,006.96, p < 0.001).
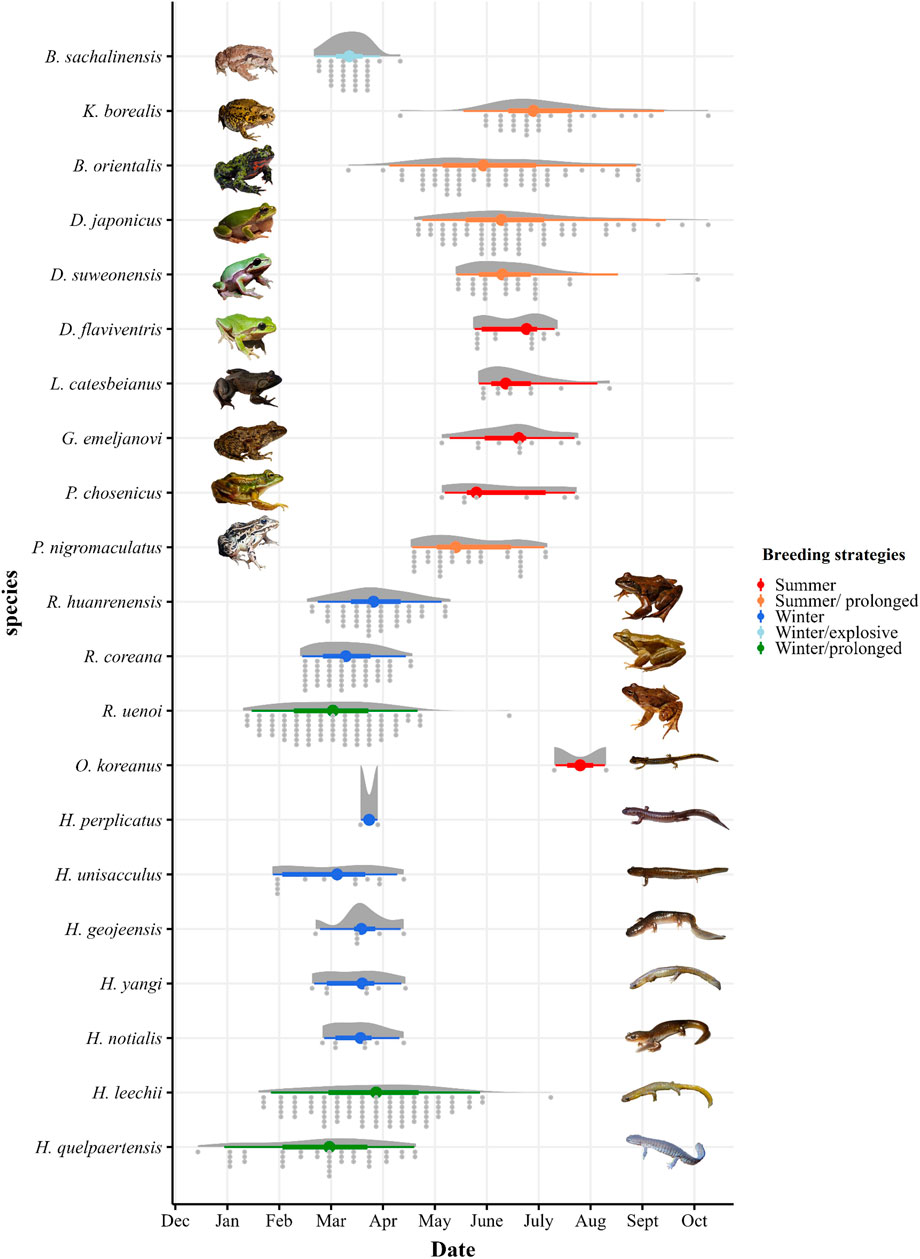
FIGURE 1. Distribution of the proportion of the observed breeding behaviour (amplexus, eggs, and/or calling), including outliers, based on 8,763 citizen science observations downloaded from iNaturalist for D. flaviventris (n = 18), D. suweonensis (n = 41), D. japonicus (n = 421), B. sachalinensis (n = 131), B. orientalis (n = 100), K. borealis (n = 48), R. coreana (n = 136), R. uenoi (n = 695), R. huanrenensis (n = 222), G. emeljanovi (n = 14), P. nigromaculatus (n = 63), P. chosenicus (n = 7), and L. catesbeianus (n = 13), H. geojeensis (n = 26), H. perplicatus (n = 14), H. notialis (n = 14), H. quelpaertensis (n = 125), H. unisacculus (n = 23), H. yangi (n = 15), H. leechii (n = 306), and O. koreanus (n = 6) in the Republic of Korea. The data was collected between 02 March 1997 and 01 December 2020. Boxplots indicate the median, interquartile range (IQR), and 1.5*IQR, boxplot colours indicate the different breeding strategies (summer, summer/prolonged, winter, winter/prolonged, winter/explosive, winter/prolonged).
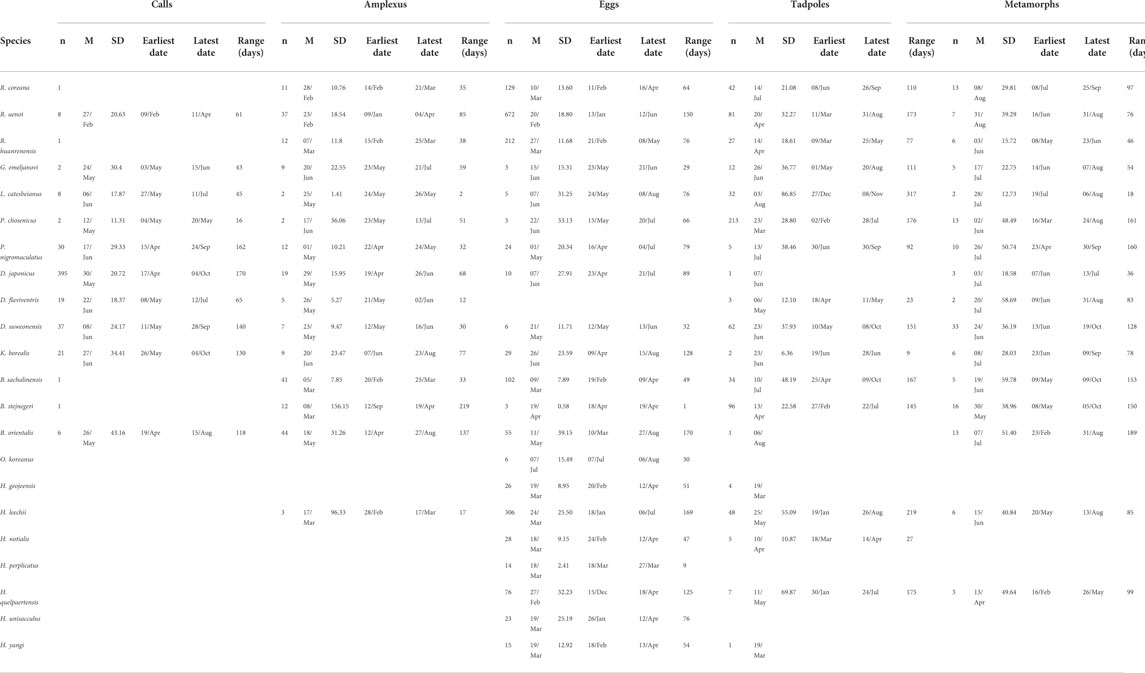
TABLE 3. Details (n = total observation, M is median, SD = standard deviation) on the phenology of calls, amplexus, eggs, tadpoles and metamorphs for Korean amphibians. The analysis is based on 8,763 citizen science observations collected between 02 March 1997 and 01 December 2020 downloaded from the platform iNaturalist.
Comparing literature and citizen science data
The peak breeding season from the citizen science data fell within the breeding season recorded in the literature for all species. However, the widest breeding seasons based on citizen science data for all species combined was on average 6.1 days shorter than reported in the literature (Standard deviation (SD) 47.9 days). Compared to citizen science data for the overall possible longest duration of breeding seasons, 11 were shorter (average 40.7 ± 33.3 days) and 10 were longer (average 31.9 ± 29 days) than described in the literature (Table 1). Six of the eight Hynobiid salamanders had a shorter breeding season based on the citizen science data than recorded in the literature (average 65.5 ± 27.79 days shorter, see Table 1). While only five out of 13 anuran species had a shorter breeding season (average 11 ± 7.2 days) than described in the literature (Table 1). The largest differences were recorded for H. perplicatus, for which breeding season was 111 days longer in the literature than from the citizen science data; and B. orientalis, for which breeding season was 108 days longer based on the citizen science data compared to the literature (see Table 1). We did not use the literature data for L. catesbeianus in these calculations due to the lack of data available for the Republic of Korea or northeast Asia.
Breeding modes
With a 49-day breeding period, based on 131 observations across 17 years, Bufo sachalinensis (previously Bufo gargarizans Othman et al., 2022) was the only amphibian in the Republic of Korea that fit the definition applied to explosive breeder. Consequently, the other species were categorised as non-explosive breeding species. Based on our data, six anuran species, B. orientalis (170 days), Kaloula borealis (178 days), Pelophylax nigromaculatus (162 days), Rana uenoi (154 days), Dryophytes japonicus (170 days), D. suweonensis (140 days), and two salamander species, Hynobius leechii (169 days), and H. quelpaertensis (125 days), were categorized as prolonged breeders (Figure 1; Table 4). While the breeding season of B. stejnegeri was longer than 120 days, it includes the hibernating period and consequently we did not qualify the species as a prolonged breeder.
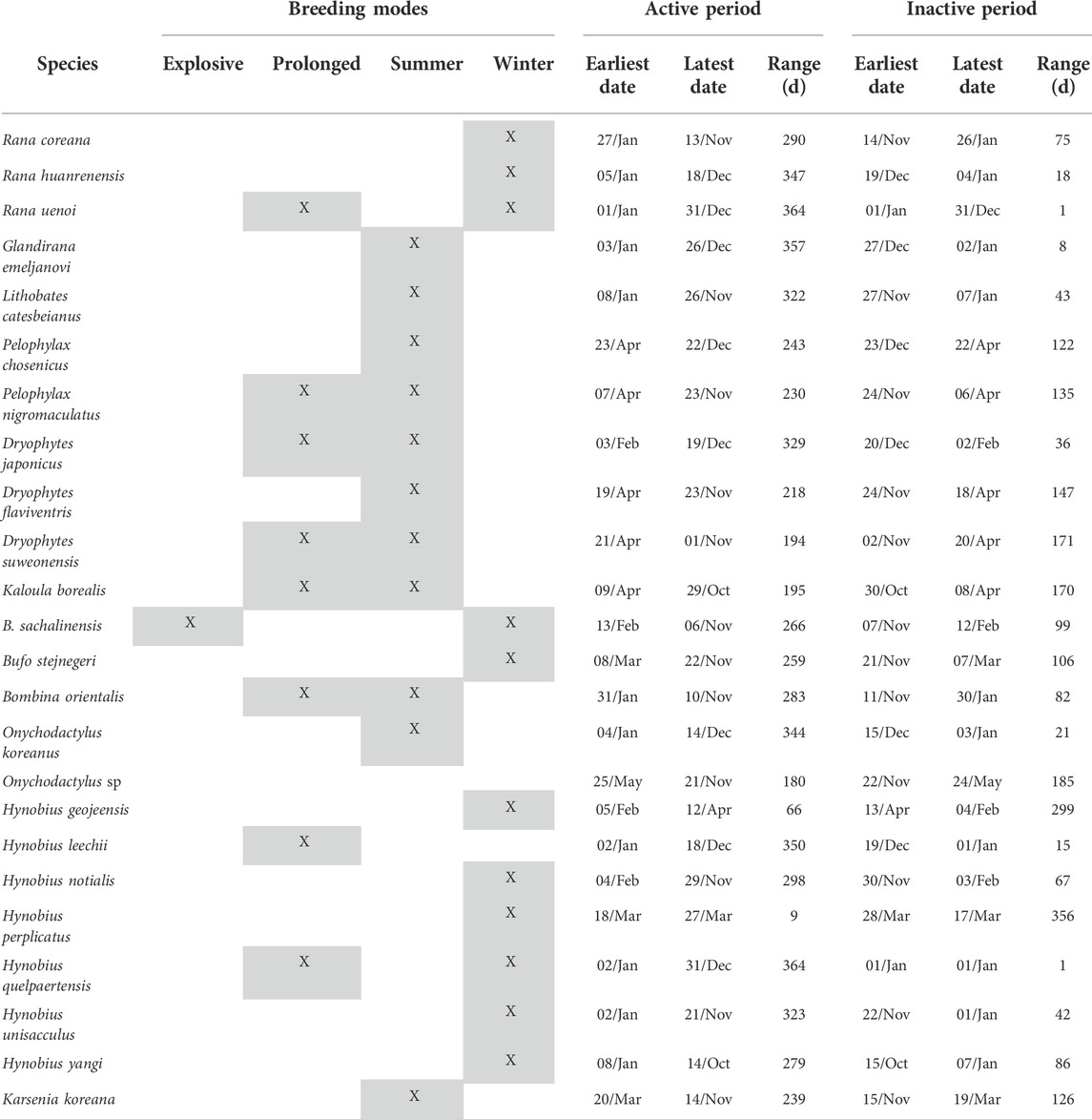
TABLE 4. Details on inactive period and breeding modes for all amphibian species of the Republic of Korea, based on 8,763 citizen science observations collected between 02 March 1997 and 01 December 2020 downloaded from the platform iNaturalist. Due to the lack of data (n = 12) Onychodactylus sp. was taken out of the table.
Following our definition, only H. yangi (54 days, starting 18 February), H. unisacculus (76 days, starting 26 January) H. geojeensis (51 days, starting 20 February), R. huanrenensis (82 days, starting 15 February) and R. coreana (64 days, starting February 11) qualified as winter breeders and were not explosive or prolonged breeders. When including prolonged and explosive breeding species, B. sachalinensis (49 days, starting 19 February), R. uenoi (154 days, starting 9 January), H. quelpaertensis (125 days, starting 14 December) and H. leechii (169 days, starting 18 January) were also defined as winter breeders. Hynobius notialis (47 days, starting 24 February) and H. perplicatus (9 days, starting18 March) were also likely to be linked to the winter breeding season despite the small sample size. Finally, B. stejnegeri also matches with the definition of winter breeder, despite the unusual amplexing behavior temporarily overlapping with hibernation (Table 4).
Excluding explosive and prolonged breeding species, summer breeders were D. flaviventris (49 days, starting 21 May), P. chosenicus (77 days, starting 3 May), Glandirana emeljanovi (79 days, starting 2 May), Lithobates catesbeianus (79 days, starting 24 May) and O. koreanus (30 days, starting 7 July). When including explosive and prolonged breeders, B. orientalis (170 days, starting 10 March), D. japonicus (170 days, starting 17 April), D. suweonensis (140 days, starting 11 May), K. borealis (178 days, starting 9 April), and P. nigromaculatus (162 days, starting April 15) also qualified as summer breeders (see Figure 1. and Table 4). Karsenia koreana may also qualify as a summer breeder, based on limited literature information (Moon & Park, 2016; see Table 1).
Inactive period
The definition of the inactive period relied on a total of n = 2,459 datapoints. The inactive period saw species being split into two contrasting groups, although not matching with summer or winter breeding nor prolonged or explosive breeders. The onset of the inactive period was around mid-November for the first set of species and late December for the other set (Table 4 and Figure 2). The significant divergence was also visible the start of their active period, with winter breeders starting significantly earlier, in early January, and summer breeders starting between February and March (Figure 1 and Table 4).
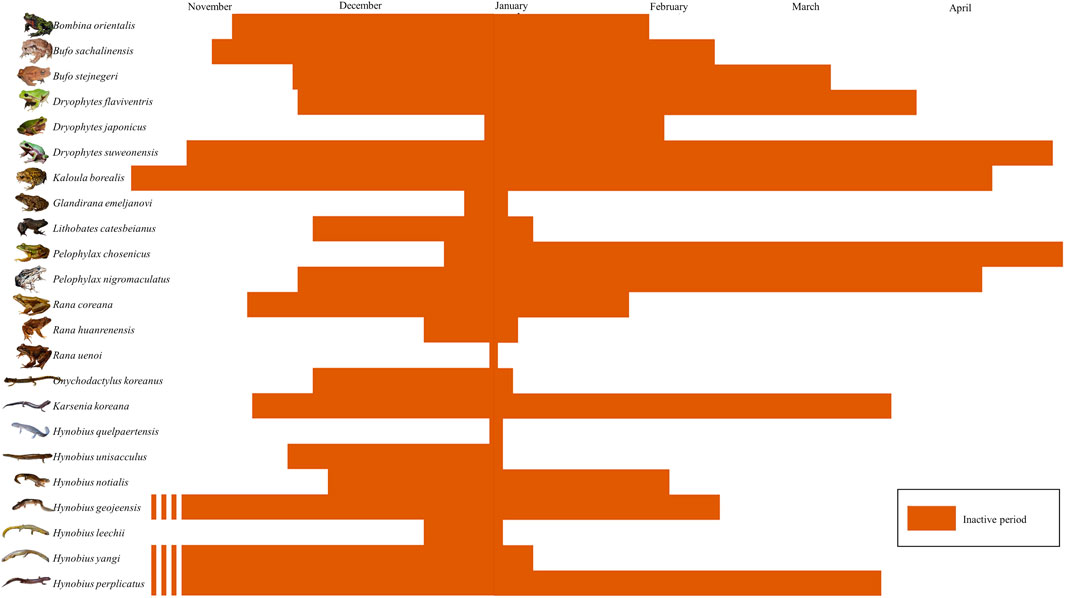
FIGURE 2. Inactive period for all Korean amphibians based on citizen science data. The onset of inactive period is missing for three Hynobius species because of the lack of data. The analysis is based on 8,763 citizen science observations collected between 02 March 1997 and 01 December 2020 downloaded from the platform iNaturalist.
Habitat use
The ANOVA test showed that all habitat types were significantly different among species, meaning that species occupy landscapes differently: woody (χ2 = 113,716,621; F (22,55,220) = 288.14, p < 0.001), herbaceous (χ2 = 110,383,889; F (22,55,220) = 118.74, p < 0.001), wetland (χ2 = 21,912,649; F (22,55,220) = 235.94, p < 0.001), water (χ2 = 5,814,936; F (22,55,220) = 44.27, p < 0.001) and urban (χ2 = 5,553,598; F (22,55,220) = 22.04, p < 0.001). The post-hoc tests highlighted numerous significant pair-wise comparisons for the woody, herbaceous and wetland landscapes (Figure 3), but fewer for the open water and urban landscapes (Annex 1; Figure 3).
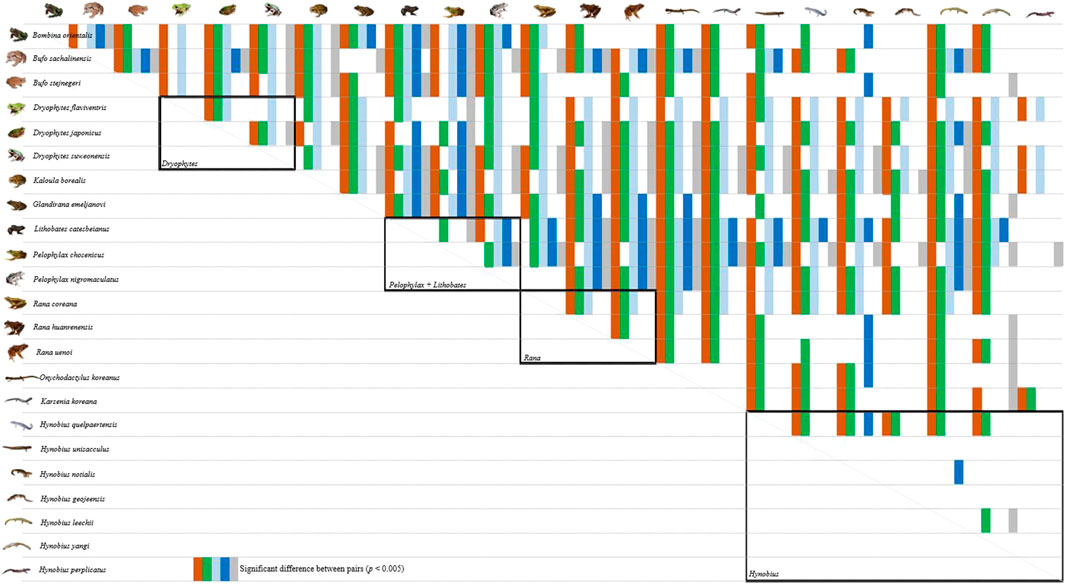
FIGURE 3. Result of the post-hoc Tuckey test to compare the habitat use between all species pair. A ‘coloured cell’ represents a p < 0.005 (exact results in Annex 1), and thus a different use in habitat between the species. The colour coding is such as brown is woody, green is herbaceous, light blue wetland, dark blue is water, and grey is urban. Intra-generic comparisons are highlighted by black boxes. The analysis is based on 8,763 citizen science observations collected between 02 March 1997 and 01 December 2020 downloaded from the platform iNaturalist.
In addition, the amount of significant pair-wise comparisons was higher for anurans than for caudata (Figure 3). In the woody landscape, most pair-wise comparisons were significant for anurans, and thus highlighting a different use of the landscape, at the exception of the comparison of D. flaviventris with L. catesbeianus, Pelophylax sp. and two Rana sp., and the comparison of D. japonicus with Pelophylax sp. and the same two Rana species. In opposition, the pairwise relationships between species in caudata were not significant species, with the exception of H. quelpaertensis in relation to the other Hynobius species. No other clear pattern emerged, except for K. koreana, O. koreanus and H. leechii being significantly different from all anurans (Figure 3). The number of significantly different pairs decreased in the herbaceous landscape (Figure 3), but the pattern was consistent with that of the woody landscape. These two landscapes are representative of breeding habitats and the result shows a segregation between species outside of the breeding season.
The wetland landscape was the most represented landscape for the breeding habitat of all species combined (Figure 3), and the pattern for anurans was similar to that of woody and herbaceous landscapes, with most of the pair-wise comparisons between anurans species being significant, as well as most of the anuran-caudata pairs. A clear segregation was visible between species when grouped by breeding season; anurans starting to breed early in the year (B. stejnegeri, B. orientalis, G. emeljanovi, R. uenoi and R. huanrenensis) were not significantly different from caudata (Figure 3).
Discussion
In this study we described the breeding phenology and habitat use for all described amphibian species from the Republic of Korea based on citizen science data collected over 23 years. Our results thus create a baseline that can be used for later comparisons. The peak of the breeding season of all species fell within the breeding season described in the literature when available. The breeding season of species with fewer observation data points deviated more often from the seasons described in the literature. For example, three out of eight species of Hynobius were described in the past year (Borzée and Min, 2021) while a fourth was described only 5 years ago (H. unisacculus; Min et al., 2016). These salamanders have a very cryptic life history and a relatively small distribution compared to the other Hynobiidae species in Korea, making observation through citizen science difficult. The recent description and small distribution have therefore resulted in fewer observations (average n = 19), for instance observations in fall are missing for three of these species, and more observations are likely to extend their known breeding season and make it match more with the literature.
We found that while only a single toad species qualified as an explosive breeding species (B. sachalinensis, 49 days in line with the literature: Cheong, Sung & Park, 2008), numerous species had extended breeding seasons. Plenderleith et al. (2018) suggested that the advantage of explosive breeding is the decreased predation risks by aquatic species such as fish, however, in the Republic of Korea the only explosive breeder (Bufo sachalinensis) is one of the two species with toxins to avoid predation (Bókony et al., 2016). This fact, along with the fact that other species have long breeding periods, highlights that predation by aquatic species does not seem to shape the breeding phenology of Korean amphibians. Landscape modifications have, however, resulted in an increase in predation on Hynobius (Bae et al., 2019) and Dryophytes (Borzée et al., 2017) and we can therefore conclude that Korean species are not properly adapted to the changes brought to the environment by human activities.
A limitation to this conclusion is that the definition of breeding type is based on the totality of the country, not along latitudinal bins because of sample size. An analysis using bins when data becomes available may shorten the duration of the breeding period at a site, with other species possibly changing to fit the definition of explosive breeder. Breeding observations for B. stejnegeri were recorded well over 120 days, from fall to late winter. The species should therefore be categorized as prolonged and winter breeder. We however did not include B. stejnegeri in either of these categories as the species is in amplexus for 3–6 months but spawning over a restricted time frame in spring (March-April; Lee & Park, 2009; Fong et al., 2020). Based on the number of days when breeding excluding amplexus were observed, the species is close to being an explosive breeder, although it does not display the typical breeding behaviour of explosive breeders.
While our results were generally matching with the literature, we found some points of discrepancy. The literature categorised R. huanrensis as an explosive breeder because it spawns over a short period in early spring (Yoo and Jang, 2012), however, based on 222 breeding behaviour observations over 13 years we determined the breeding season to be between 15 February and 8 May (range = 82 days). While the species is not an explosive breeder at the national scale over 13 years, the yearly average breeding season was 43 days, qualifying it as explosive breeder from year to year. Our classification categorises R. huanrenensis as a winter breeder, and thus the breeding season of the species is probably importantly influenced by the weather, a year-dependant variable also subjected to climate change, and thus the likely explanation for the longer overall breeding season over multiple years (Blaustein et al., 2001). Furthermore, to the best of our knowledge there is no described breeding season in the literature for L. catesbeianus and R. coreana in the Republic of Korea.
In their natural range L. catesbeianus is a prolonged breeder, however according to our data (76 days) L. catesbeianus does not have a prolonged breeding season in the Republic of Korea. This has probably to do with the heavy influence of weather on the life history of amphibians (Plenderleith et al., 2018), and species with a smaller distribution will encounter a lower variety of weather condition compared to species with a larger distribution. When the weather is not matching with the ecological requirements of the species for breeding (e.g., Llusia et al., 2013), the mismatch will result in a relatively shorter breeding seasons for species with a small range as they do not experience the wider range of weather patterns impacting species with wider ranges. Besides L. catesbeianus, treefrogs in the Republic of Korea are another example illustrating this point: D. japonicus has the largest distribution, followed by D. suweonensis and then D. flaviventris, a ranking by range size in line with the duration of the breeding season: 168, 69 and 49 days respectively.
In this study we used a citizen science database, the advantage is that the data is collected over several decades and is therefore relevant to establish a strong baseline, and the data originates from most geographic areas from the focal country: the Republic of Korea. A clear drawback is that most data comes from generally easily accessible areas and non-work days, a common trend for citizen science data (Bird et al., 2014). However, by supplementing the dataset with surveys conducted by the leading national ecological organization we contravene both the limitation of accessibility by citizen science, and the working-hours restrictions linked to governmental organisations. For instance, citizen science data from the Republic of Korea showed a high accuracy when describing the range of Hynobius salamanders, comparable to standard molecular tools, with the exception of areas under taxonomic uncertainty (Borzée et al., 2019b), where trained observers are likely to better-assess species’ identity. By combining the two types of data, we also resolve the bias arising from a larger number of observations for charismatic and easily observable species.
We were not able to determine the boundaries of the hibernation period for any of the species due to the nature of the data. We had to qualify this period as inactive as the species hide before hibernation and are therefore unlikely to be observed. This is for instance the case of D. japonicus, where individuals move up the trees in fall, before it becomes too cold (Borzée et al., 2018b). The absence of latitudinal bins and year by year observation also meant that the inactive period in winter was greatly shorter than what would be expected for all of the species (Lee and Park, 2016). For instance, some species were determined to be inactive for as little as 6 days. This is not representative of the length of the hibernation, but the number of days when the species were not observed for any of the 23 years used for this analysis. In addition, some secretive species such as salamanders are unlikely to be detected in winter, when citizen science records are not as numerous. Therefore, species with a low number of observations, such as H. notialis and H. perplicatus, are also likely to be winter breeders, but due to the lack of data they are a couple of days shy of being categorized as such. In addition, due to global warming, amphibians have shown significant phenological shifts, generally starting to breed earlier in the season (Carroll et al., 2009; Green, 2017), and preliminary results for R. uenoi and B. sachalinensis in the Republic of Korea follow the same pattern (Kwan et al., 2020).
Anthropogenic changes in landscapes, through degradation or loss, generally result in biodiversity losses (Pyron, 2018). It is therefore important to understand the position and role of species in all landscapes to also establish a baseline before a too important shift blurs the relationship and knowledge on the historical presence and use of landscapes (Haberle, 2007). This is especially important in the landscape studied here as we found a greater difference in habitat use among genera than within genera, likely as a result of niche conservatism within closely related species (Losos, 2008; Prinzing et al., 2017) and thus highlighting more prevalent threats to specific clades.
The fact that fewer pairs were significantly different in the herbaceous than in other landscapes, but more pairs were significantly different in the woody landscape highlights that pressure for niche differentiation is greater in the landscape where the species breed. For instance, the convergence of numerous species to similar breeding habitats in late winter results in increased competition for breeding resources, and thus diversification of habitat use. The absence of many significantly different pairs within and between anuran and caudata in both the water and the urban landscapes is representative of a similar pattern for all species, here, the avoidance of the habitat.
The importance of establishing a baseline is demonstrated in this study, as closely related species are more likely to be extirpated faster. Some exceptions do need to be noted, for instance the divergence of threat level between the threatened D. suweonensis and D. flaviventris in comparison with the abundant D. japonicus. Here, this dichotomy is likely explained by the deep divergence between the two clades within the same genus (Borzée et al., 2020a). Another exception is that of Hynobius salamanders, with H. quelpaertensis being the only species significantly different from the others in the genus. This divergence is likely explained by the fact that H. quelpaertensis is the only species occurring on a basaltic island, which has been shown to have an impact on the behaviour and morphology of amphibians and reptiles due to the predation pressure (Kang et al., 2017; Shin and Borzée, 2020).
Data availability statement
Publicly available datasets were analyzed in this study. This data can be found here: The data was extracted from the citizen science database iNaturalist (https://www.inaturalist.org) for all amphibian observation uploaded between 02 March 1997 and 5 November 2020 in the Republic of Korea.
Ethics statement
Ethical review and approval was not required for the animal study because While focused on vertebrates, the data was collected online.
Author contributions
JG and AB designed the experiment, collected data, analysed data, wrote the first draft, and revised the manuscript. DA collected data, analysed data and revised the manuscript. AB provided financial resources.
Funding
This work was supported by the Foreign Youth Talent Program (QN2021014013L) from the Ministry of Science and Technology to AB.
Acknowledgments
We are grateful to iNaturalist for providing such a wealth of information.
Conflict of interest
The authors declare that the research was conducted in the absence of any commercial or financial relationships that could be construed as a potential conflict of interest.
Publisher’s note
All claims expressed in this article are solely those of the authors and do not necessarily represent those of their affiliated organizations, or those of the publisher, the editors and the reviewers. Any product that may be evaluated in this article, or claim that may be made by its manufacturer, is not guaranteed or endorsed by the publisher.
References
Andersen, D., Litvinchuk, S. N., Jang, H. J., Jiang, J., Koo, K. S., Maslova, I., et al. (2022). Incorporation of latitude-adjusted bioclimatic variables increases accuracy in species distribution models. Ecol. Modell. 469, 109986. doi:10.1016/j.ecolmodel.2022.109986
Bae, Y., Kong, S., Yi, Y., Jang, Y., and Borzée, A. (2019). Additional threat to Hynobius salamander eggs: Predation by loaches (Misgurnus sp.) in agricultural wetlands. Anim. Biol. Leiden. Neth. 69, 451–461. doi:10.1163/15707563-20191070
Bird, T. J., Bates, A. E., Lefcheck, J. S., Hill, N. A., Thomson, R. J., Edgar, G. J., et al. (2014). Statistical solutions for error and bias in global citizen science datasets. Biol. Conserv. 173, 144–154. doi:10.1016/j.biocon.2013.07.037
Blaustein, A. R., Belden, L. K., Olson, D. H., Green, D. M., Root, T. L., and Kiesecker, J. M. (2001). Amphibian breeding and climate change. Conserv. Biol. 15, 1804–1809. doi:10.1046/j.1523-1739.2001.00307.x
Blaustein, A. R., Walls, S. C., Bancroft, B. A., Lawler, J. J., Searle, C. L., and Gervasi, S. S. (2010). Direct and indirect effects of climate change on amphibian populations. Diversity 2, 281–313. doi:10.3390/d2020281
Bókony, V., Móricz, Á., Tóth, Z., Gál, Z., Kurali, A., Mikó, Z., et al. (2016). Variation in chemical defense among natural populations of common toad, Bufo bufo, tadpoles: The role of environmental factors. J. Chem. Ecol. 42, 329–338. doi:10.1007/s10886-016-0690-2
Borzée, A., Andersen, D., Groffen, J., Kim, H.-T., Bae, Y., and Jang, Y. (2019a). Climate change-based models predict range shifts in the distribution of the only asian plethodontid salamander: Karsenia koreana. Sci. Rep. 9, 11838. doi:10.1038/s41598-019-48310-1
Borzée, A., Baek, H. J., Lee, C. H., Kim, D. Y., Song, J.-Y., Suh, J.-H., et al. (2019b). Scientific publication of georeferenced molecular data as an adequate guide to delimit the range of Korean Hynobius salamanders through citizen science. Acta Herpetol. 14, 27–33. doi:10.13128/Acta_Herpetol-24102
Borzée, A., Choi, Y., Kim, Y. E., Jablonski, P., and Jang, Y. (2019c). Interspecific variation in seasonal migration and brumation behavior in two closely related species of treefrogs. Front. Ecol. Evol. 7, 55. doi:10.3389/fevo.2019.00055
Borzée, A., Heo, K., and Jang, Y. (2018a). Relationship between agro-environmental variables and breeding Hylids in rice paddies. Sci. Rep. 8, 8049. doi:10.1038/s41598-018-26222-w
Borzée, A., Kim, K., Heo, K., Jablonski, P. G., and Jang, Y. (2017). Impact of land reclamation and agricultural water regime on the distribution and conservation status of the endangered Dryophytes suweonensis. PeerJ 5, e3872. doi:10.7717/peerj.3872
Borzée, A., Kim, M., Kim, J. Y., Kim, T., and Jang, Y. (2018b). Microhabitat use during brumation in the Japanese treefrog, Dryophytes japonicus. Amphib. Reptil. 39, 163–175. doi:10.1163/15685381-17000036
Borzée, A., Messenger, K. R., Chae, S., Andersen, D., Groffen, J., Kim, Y. I., et al. (2020a). Yellow sea mediated segregation between north east Asian dryophytes species. PLoS One 15, e0234299. doi:10.1371/journal.pone.0234299
Borzée, A., and Min, M.-S. (2021). Disentangling the impacts of speciation, sympatry and the island effect on the morphology of seven Hynobius sp. salamanders. Animals 11, 187. doi:10.3390/ani11010187
Borzée, A. (2020). Recommendations for IUCN red list conservation status of the “dryophytes immaculatus group” in north east Asia. Diversity 12, 336. doi:10.3390/d12090336
Borzée, A., Shin, Y., Poyarkov, N. A., Jeon, J. Y., Baek, H. J., Lee, C. H., et al. (2020). Dwindling in the mountains: Description of a critically endangered and microendemic Onychodactylus species (Amphibia, Hynobiidae) from the Korean peninsula. China: Zoological Research. In press.
Borzée, A., Sin, E., Oh, S., and Jang, Y. (2020b). Spring voices in Korean rice fields: The effect of abiotic variables and syntopic calls on the calling activity of the treefrog dryophytes suweonensis. Asian herpetological research. Asian Herpetol. Res. 11, 335–341. doi:10.16373/j.cnki.ahr.190046
Box, G. E. P., and Tidwell, P. W. (1962). Transformation of the independent variables. Technometrics 4, 531–550. doi:10.1080/00401706.1962.10490038
Carroll, E. A., Sparks, T. H., Collinson, N., and Beebee, T. J. C. (2009). Influence of temperature on the spatial distribution of first spawning dates of the common frog (Rana temporaria) in the UK. Glob. Chang. Biol. 15, 467–473. doi:10.1111/j.1365-2486.2008.01726.x
Chazard, E., Ficheur, G., Beuscart, J. B., and Preda, C. (2017). How to compare the length of stay of two samples of inpatients? A simulation study to compare type I and type II errors of 12 statistical tests. Value Health 20, 992–998. doi:10.1016/j.jval.2017.02.009
Cheong, S., Sung, H., and Park, S. (2008). Inability of mate and species recognition by male Asian toads, Bufo gargarizans. Anim. Cells Syst. Seoul. 12, 93–96. doi:10.1080/19768354.2008.9647160
Cohen, J. M., Lajeunesse, M. J., and Rohr, J. R. (2018). A global synthesis of animal phenological responses to climate change. Nat. Clim. Chang. 8, 224–228. doi:10.1038/s41558-018-0067-3
Cohn, J. P. (2008). Citizen science: Can volunteers do real research? BioScience 58, 192–197. doi:10.1641/b580303
Conant, R., and Collins, J. T. (1998). Peterson field guide to eastern & central north American reptiles and Amphibians. Boston: Houghton Mifflin.
Conrad, C. C., and Hilchey, K. G. (2011). A review of citizen science and community-based environmental monitoring: Issues and opportunities. Environ. Monit. Assess. 176, 273–291. doi:10.1007/s10661-010-1582-5
Dickinson, J. L., Zuckerberg, B., and Bonter, D. N. (2010). Citizen science as an ecological research tool: Challenges and benefits. Annu. Rev. Ecol. Evol. Syst. 41, 149–172. doi:10.1146/annurev-ecolsys-102209-144636
Do, M. S., Son, S. J., Choi, G., Yoo, N., Koo, K. S., and Nam, H. K. (2021). Anuran Community Patterns in the rice fields of the mid-western region of the Republic of Korea. Glob. Ecol. Conserv. 26, e01448. doi:10.1016/j.gecco.2020.e01448
Do, M. S., Suh, J.-H., Son, S.-J., Choi, G., Yoo, N., Jung, J.-H., et al. (2020). Breeding area characteristics of wonsan salamander (Hynobius leechii) inhabiting rice field around the mid-west in region of South Korea. Korean J. Herpetol. 11, 21–29. doi:10.1016/j.gecco.2020.e01448
Fong, J. J., Yang, B. T., Li, P. P., Waldman, B., and Min, M. S. (2020). Phylogenetic systematics of the water toad (Bufo stejnegeri) elucidates the evolution of semi-aquatic toad Ecology and pleistocene glacial refugia. Front. Ecol. Evol. 7, 1–10. doi:10.3389/fevo.2019.00523
Fujioka, M., and Lane, S. J. (1997). The impact of changing irrigation practices in rice fields on frog populations of the Kanto Plain, central Japan. Ecol. Res. 12, 101–108. doi:10.1007/BF02523615
Grant, R. A. (2012). The effect of environmental variables on amphibian breeding phenology. The Open University PhD thesis. doi:10.21954/ou.ro.0000ee36
Gray, S., Jordan, R., Crall, A., Newman, G., Hmelo-Silver, C., Huang, J., et al. (2017). Combining participatory modelling and citizen science to support volunteer conservation action. Biol. Conserv. 208, 76–86. doi:10.1016/j.biocon.2016.07.037
Green, D. M. (2017). Amphibian breeding phenology trends under climate change: Predicting the past to forecast the future. Glob. Chang. Biol. 23, 646–656. doi:10.1111/gcb.13390
Groffen, J., Borzée, A., and Jang, Y. (2018). Preference for natural borders in rice paddies by two treefrog species. Anim. Cells Syst. Seoul. 22, 205–211. doi:10.1080/19768354.2018.1475301
Groffen, J., Kong, S., Jang, Y., and Borzée, A. (2019a). The invasive American bullfrog (Lithobates catesbeianus) in the Republic of Korea: History and recommendations for population control. Manag. Biol. Invasion. 10, 517–535. doi:10.3391/mbi.2019.10.3.08
Groffen, J., Oh, S. Y., Kwon, S., Jang, Y., and Borzee, A. (2019b). High mortality in Bufo gargarizans eggs associated with an undescribed Saprolegnia ferax strain in the Republic of Korea. Dis. Aquat. Organ. 137, 89–99. doi:10.3354/dao03434
Haberle, S. G. (2007). Prehistoric human impact on rainforest biodiversity in highland New Guinea. Phil. Trans. R. Soc. B 362, 219–228. doi:10.1098/rstb.2006.1981
IUCN SSC Amphibian Specialist Group (2020). Bufo stejnegeri. The IUCN Red List of Threatened Species e.T54766A63862727. RLTS.T54766A63862727.en (Accessed February 20, 2020). doi:10.2305/IUCN.UK.2020-1
Kang, C., Sherratt, T. N., Kim, Y. E., Shin, Y., Moon, J., Song, U., et al. (2017). Differential predation drives the geographical divergence in multiple traits in aposematic frogs. Behav. Ecol. 28, 1122–1130. doi:10.1093/beheco/arx076
Kang, H.-J., Koo, K., and Sung, H.-C. (2019). Current distribution of American bullfrog Rana catesbeiana shaw, 1802 in the republic of Korea. Bioinvasions Rec. 8, 942–946. doi:10.3391/bir.2019.8.4.23
Kaplan, R. H., and Phillips, P. C. (2006). Ecological and developmental context of natural selection: Maternal effects and thermally induced plasticity in the frog Bombina orientalis. Evol. 60, 142. doi:10.1554/05-327r.1
Ki, K.-S., Gim, J.-Y., and Lee, J.-Y. (2016). Water temperature and sound environment characteristics of Huanren Brown frog oviposition sites. Korean J. Environ. Ecol. 30, 344–352. doi:10.13047/kjee.2016.30.3.344
Kim, H.-T., Kim, H., Jeon, G., and Kim, D. (2019). ´Arrow guide of amphibians and reptiles´. Seoul, Republic of Korea. Seoul: Econature.
Kim, H. W., Yoon, S., Kim, M., Shin, M. S., Yoon, H., and Kim, K. (2021). EcoBank: A flexible database platform for sharing ecological data. Biodivers. Data J. 9, e61866. doi:10.3897/bdj.9.e61866
Kim, K., Macias, D., Borzée, A., and Jang, Y. (2020). Ueno’s Brown frog Rana uenoi indiscriminately ceases calling in the presence of daytime birds. Ethol. Ecol. Evol. 32, 251–263. doi:10.1080/03949370.2020.1717638
Ko, S.-B., Chang, M.-H., Song, J.-Y., and Oh, H.-S. (2012). Meteorological factors influencing breeding biology of Kaloula borealis. Korean J. Environ. Ecol. 26, 876–883. https://koreascience.kr/article/JAKO201207339092522.page
Ko, S. kun, Mook Kang, H., Im, W. Bin, and Bang Kwon, H. (1998). Testicular cycles in three species of Korean frogs: Rana nigromaculata, Rana rugosa, and Rana dybowskii. Gen. Comp. Endocrinol. 111, 347–358. doi:10.1006/gcen.1998.7118
Kwan, S., Borzée, A., Koo, K. S., and Jang, Y. (2020). Yearly and regional difference on the spawning season of three Korean amphibian species. Onlin: Conference of the Korean Society of Ecology.
Lannoo, M. J., and Stiles, R. M. (2020). Uncovering shifting Amphibian ecological relationships in a World of environmental Change1. Herpetologica 76, 144–152. doi:10.1655/0018-0831-76.2.144
Lee, J.-H., and Park, D. (2009). Effects of body size, operational sex ratio, and age on pairing by the asian toad, Bufo stejnegeri. Zool. Stud. 48, 334–342. Available at: http://zoolstud.sinica.edu.tw/Journals/48.3/334.pdf (Accessed June 17, 2020).
Lee, J.-H., Ra, N.-Y., Eom, J., and Park, D. (2008). Population dynamics of the long-tailed clawed salamander larva, Onychodactylus fischeri, and its age structure in Korea. J. Ecol. Environ. 31, 31–36. doi:10.5141/jefb.2008.31.1.031
Lee, J., and Park, D. (2016). Nature and ecology. Seoul, Republic of Korea.The encyclopedia of Korean amphibians
Lintott, C., Schawinski, K., Bamford, S., Slosar, A., Land, K., Thomas, D., et al. (2010). Galaxy zoo 1: Data release of morphological classifications for nearly 900 000 galaxies. Mon. Not. R. Astron. Soc. 410, 166–178. doi:10.1111/j.1365-2966.2010.17432.x
Llorente, G., Montori, A., and Richter-Boix, A. (2006). Breeding phenology of an amphibian community in a Mediterranean area. Amphib. Reptil. 27, 549–559. doi:10.1163/156853806778877149
Llusia, D., Marquez, R., Beltran, J. F., Moreira, C., and do Amaral, J. P. (2013). Environmental and social determinants of anuran lekking behavior: Intraspecific variation in populations at thermal extremes. Behav. Ecol. Sociobiol. 67, 493–511. doi:10.1007/s00265-012-1469-2
Losos, J. B. (2008). Phylogenetic niche conservatism, phylogenetic signal and the relationship between phylogenetic relatedness and ecological similarity among species. Ecol. Lett. 11, 995–1003. doi:10.1111/j.1461-0248.2008.01229.x
Lunghi, E., Corti, C., Manenti, R., Barzaghi, B., Buschettu, S., Canedoli, C., et al. (2018). Comparative reproductive biology of European cave salamanders (Genus hydromantes): Nesting selection and multiple annual breeding. Salamandra 54, 101–108. ISSN 0036‐3375
Matsui, M. (2004). IUCN SSC Amphibian Specialist Group. 2021. Pelophylax chosenicus. The IUCN Red List of Threatened Species. 2021 e.T58577A110101963 (Accessed November 8, 2011). doi:10.2305/IUCN.UK.2004.RLTS.T58577A11806007.en
McKinley, D. C., Miller-Rushing, A. J., Ballard, H. L., Bonney, R., Brown, H., Cook-Patton, S. C., et al. (2017). Citizen science can improve conservation science, natural resource management, and environmental protection. Biol. Conserv. 208, 15–28. doi:10.1016/j.biocon.2016.05.015
Min, M. S., Baek, H. J., Song, J. Y., Chang, M. H., and Poyarkov, N. A. (2016). A new species of salamander of the genus Hynobius (Amphibia, Caudata, Hynobiidae) from South Korea. Zootaxa 4169, 475–503. doi:10.11646/zootaxa.4169.3.4
Moon, K., and Park, D. (2016). Report of Karsenia koreana eggs oviposited within a semi-natural terrarium constructed at natural habitat. Korean J. Herpetol. 7, 1–5.
Othman, S. N., Litvinchuk, S. N., Maslova, I., Dahn, H., Messenger, K. R., Andersen, D., et al. (2022). From Gondwana to the Yellow Sea, evolutionary diversifications of true toads Bufo sp. in the Eastern Palearctic and a revisit of species boundaries for Asian lineages. Elife 11, e70494–42. doi:10.7554/eLife.70494
Plenderleith, T. L., Stratford, D., Lollback, G. W., Chapple, D. G., Reina, R. D., and Hero, J. M. (2018). Calling phenology of a diverse amphibian assemblage in response to meteorological conditions. Int. J. Biometeorol. 62, 873–882. doi:10.1007/s00484-017-1490-2
Polat, F., and Başkale, E. (2018). Phenology and factors influencing the abundance of Lyciasalamandra fazilae (Amphibia: Salamandridae) in Turkey. Salamandra 54, 123–131. ISSN 0036-3375
Poyarkov, N. A. J., Che, J., Min, M.-S., Kuro-o, M., Yan, F., Li, C., et al. (2014). Review of the systematics, morphology and distribution of Asian Clawed Salamanders, genus Onychodactylus (Amphibia, Caudata: Hynobiidae), with the description of four new species. Zootaxa 3465, 1. doi:10.11646/zootaxa.3465.1.1
Prinzing, A., Ozinga, W. A., Brändle, M., Courty, P. E., Hennion, F., Labandeira, C., et al. (2017). Benefits from living together? Clades whose species use similar habitats may persist as a result of eco‐evolutionary feedbacks. New Phytol. 213, 66–82. doi:10.1111/nph.14341
Pyron, R. A. (2018). Global amphibian declines have winners and losers. Proc. Natl. Acad. Sci. U. S. A. 115, 3739–3741. doi:10.1073/pnas.1803477115
Roh, G., Borzée, A., and Jang, Y. (2014). Spatiotemporal distributions and habitat characteristics of the endangered treefrog, Hyla suweonensis, in relation to sympatric H. japonica. Ecol. Inf. 24, 78–84. doi:10.1016/j.ecoinf.2014.07.009
RStudio Team (2020). RStudio: Integrated development for R. Available at: http://www.rstudio.com/.
Salvador, A., and Carrascal, L. M. (1990). Reproductive phenology and temporal patterns of mate access in Mediterranean anurans. J. Herpetol. 24, 438–441. doi:10.2307/1565070
Shin, Y., and Borzée, A. (2020). Melanism in the Ussuri pitviper (Gloydius ussuriensis) from the Republic of Korea, with remarks on color variations in the species. Jordan J. Nat. Hist. 7, 60–63.
Shin, Y., Min, M. S., and Borzée, A. (2021). Driven to the edge: Species distribution modeling of a clawed salamander (Hynobiidae: Onychodactylus koreanus) predicts range shifts and drastic decrease of suitable habitats in response to climate change. Ecol. Evol. 11, 14669–14688. doi:10.1002/ece3.8155
Shirk, J., Ballard, H., Wilderman, C., Phillips, T., Wiggins, A., Jordan, R., et al. (2012). Public participation in scientific research: A framework for deliberate design. Ecol. Soc. 17, 29–48. doi:10.5751/es-04705-170229
Stuart, S. N., Chanson, J. S., Cox, N. A., Young, B. E., Rodrigues, A. S. L., Fischman, D. L., et al. (2004). Status and trends of amphibian declines and extinctions worldwide. Science 306, 1783–1786. doi:10.1126/science.1103538
Sung, H.-C., Cha, S.-M., Cheong, S.-W., Park, D.-S., and Park, S.-R. (2007). Monitoring local populations and breeding migration patterns of the gold-spotted pond frog, Rana chosenica. J. Ecol. Environ. 30, 121–126. doi:10.5141/jefb.2007.30.2.121
Tabachnick, B., and Fidell, L. (2014). Using multivariate statistics. 6th Edition. Boston: Pearson. 0205849571
Tiedemann, F. (2020). gghalves. Available at: https://erocoar.github.io/gghalves/.
Walls, S. C., Barichivich, W. J., and Brown, M. E. (2013). Drought, deluge and declines: The impact of precipitation extremes on amphibians in a changing climate. Biol. (Basel) 2, 399–418. doi:10.3390/biology2010399
Wells, K. D. (2010). The ecology and behavior of amphibians. Chicago: University of Chicago Press. Available at: https://press.uchicago.edu/ucp/books/book/chicago/E/bo5298950.html.
Keywords: breeding, wetland, forests, anura, caudata, Korean peninsula
Citation: Groffen J, Andersen D and Borzée A (2022) Breeding phenology and landscape use in all amphibian species from the Republic of Korea based on open-source data. Front. Environ. Sci. 10:846481. doi: 10.3389/fenvs.2022.846481
Received: 08 February 2022; Accepted: 22 August 2022;
Published: 31 October 2022.
Edited by:
Hideyuki Doi, University of Hyogo, JapanReviewed by:
Jose Valdez, German Centre for Integrative Biodiversity Research (iDiv), GermanyTatjana Dujsebayeva, Institute of Zoology of Republic of Kazakhstan, Kazakhstan
Copyright © 2022 Groffen, Andersen and Borzée. This is an open-access article distributed under the terms of the Creative Commons Attribution License (CC BY). The use, distribution or reproduction in other forums is permitted, provided the original author(s) and the copyright owner(s) are credited and that the original publication in this journal is cited, in accordance with accepted academic practice. No use, distribution or reproduction is permitted which does not comply with these terms.
*Correspondence: Amaël Borzée, YW1hZWxib3J6ZWVAZ21haWwuY29t