- 1University of Applied Sciences of Western Switzerland, HES-SO, HEPIA-Agronomy, Geneva, Switzerland
- 2University of Lausanne, FGSE-IDYST, Lausanne, Switzerland
- 3Research Institute of Organic Agriculture FiBL, Lausanne, Switzerland
Both soil quality degradation and climate change mitigation issues emphasize the need to increase, or at least stabilize, the topsoil organic carbon content (wt%) in arable land. This on-farm study aimed at measuring the impact of agricultural practices on changes in soil organic carbon (SOC) content over 10 years. A total of 120 fields belonging to 120 farms representative of the cropping systems and soil properties in Western Switzerland (Lake Geneva region) was randomly selected. The field 0–20 cm topsoil was sampled at a 10-years interval, and the corresponding cropping practices were gathered using farmer’s interviews and the mandatory records of yearly practices at field level in Swiss-farms. Only 1) organic matter inputs and 2) cover-crop intensity were significantly correlated to SOC increase while 3) the soil tillage intensity and 4) the soil saturation in carbon expressed as a SOC to clay content ratio were correlated to SOC decrease. Among others, temporary meadows were not correlated to changes in SOC content mainly due to increased tillage and decreased cover-crops between meadows. Organic farming did not correlate either with SOC changes due to the large tillage intensity applied for weed control. The observed SOC content changes ranged from −56‰ to +74‰ and were well explained by a linear regression model with additive effect of the four identified SOC change factors. The additivity of these factors means that farmers can emphasize the methods of their choice when regenerating their soils. This study advocates that strict no-till is not required at low carbon saturation level (small SOC:Clay ratio). However, as carbon saturation increases, conservation tillage and then no-till practices become necessary to further increase SOC contents. These findings are in accordance with previous studies showing that since 2015 SOC is increasing at more than +4‰ on average in the region and provide practical insights to further manage the transition of farming systems towards soil regeneration.
1 Introduction
According to Olson et al., 2014, carbon sequestration is “the process of transferring CO2 from the atmosphere into the soil of a land unit, through plants, plant residues and other organic solids which are stored or retained in the unit as part of the soil organic matter (humus).” A sequestration for at least 20 years is often chosen as a criterion (IPCC, 2006). The 4-per-mille initiative (Minasny et al., 2017) has raised high hopes on the potential of this Negative Emission Technology (NET) to contribute to ecological transition by mitigating the increase in atmospheric CO2 due to fossil energy consumption. Briefly, it suggests that increasing by a factor of 1.004 every year the soil organic carbon (SOC) stocks in the soil upper layer would significantly reduce the CO2 concentration in the atmosphere. The feasibility of this initiative has been highly disputed (Powlson et al., 2011; Baveye et al., 2018; De Vries, 2018; Minasny et al., 2018; White et al., 2018; Chenu et al., 2019; Rumpel et al., 2020). The potential of this NET in agricultural soils, however, was underlined by two assessment reports of European Academies Science Advisory Council (EASAC, 2018, 2019). Consequently, much of the literature has been devoted to seeking methods achieving this objective (Dignac et al., 2017; Zomer et al., 2017; Chenu et al., 2019; Rumpel et al., 2020). These studies have mainly highlighted the potential of agricultural practices belonging to the pillars of Conservation Agriculture (Hobbs et al., 2008), i.e., continuous soil cover by plants and residues, limited tillage and diversification of crop rotation.
Regardless of climate issues, SOC is a major factor of topsoil fertility (Bünemann et al., 2018; King et al., 2020). In the second half of the 20th century, intensified agriculture caused a sharp decrease in topsoil SOC content (wt%) (McLauchlan, 2006), estimated at 50–70% of initial topsoil SOC content (Kucharik et al., 2001; Lal, 2004; Sanderman et al., 2017). This decrease was attributed to intense soil disturbance, shortening of the rotation lengths and export of the crop residues (West and Post, 2002; Reicosky, 2003; McLauchlan, 2006; Smith et al., 2012). Therefore, considerations including decline of soil quality and provision of ecosystem services are put forward to call for restoration of the SOC content in arable land (Swinton et al., 2007; Power, 2010; Blanco-Canqui et al., 2013), rather than focusing on carbon sequestration alone which may lead to hazardous practices for soil quality (Baveye et al., 2020). Moreover, the desirable SOC content in cropland was often related to the concept of clay complexation and saturation with organic carbon. This concept is expressed as a SOC:Clay ratio (Dexter et al., 2008; Johannes et al., 2017; Prout et al., 2020) that can be used to calculate carbon sequestration potential (Merante et al., 2017; Chen et al., 2019; Dupla et al., 2021).
Whether increasing the SOC content and SOC stock of the topsoil in cropland is an achievable objective is still highly disputed (e.g., Chambers et al., 2016; Baveye et al., 2018; White et al., 2018; Chenu et al., 2019; Paustian et al., 2019; Amelung et al., 2020; Giller et al., 2021). Highly contrasting conclusions are reported, particularly with respect to the potential of no-till practices and their influence on SOC loss (Dimassi et al., 2014; Powlson et al., 2014; Haddaway et al., 2017). However, most of these results were obtained on long term experiments (LTE).
A recent study performed on the Swiss long-term experiments reported negative annual change rates in SOC content of the 0–20 cm topsoil for all the LTEs regardless of the cropping system (Keel et al., 2019). Conversely, in another large scale on-farm study conducted in the Lake Geneva region, Dupla et al. (2021) showed that the SOC content of the cropland 0–20 cm topsoil was increasing on average, with annual SOC change rates ranging from less than −30‰ to more than +30‰. With a cropland mean SOC annual change rate increasing from −5‰ at the end of the 20th century to more than +5‰ at present, this study contradicted Keel et al. (2019) findings, though performed under the same climate and soil conditions. Consequently Dupla et al. (2021) concluded that LTE results cannot be extrapolated to farm fields, as already underlined by previous studies (Govaerts et al., 2009; Cook et al., 2013).
Based on the findings of Dupla et al. (2021), this study was performed in the same geographical area, namely in the Vaud and Geneva cantons—Switzerland. Here, we explored the relationships between the observed SOC content annual change rates in the 0–20 cm topsoil and the cropping practices, over the past 10 years, for 120 fields and farms representative of the soils and cropping systems of this region. Special attention was given to the practices commonly recognized as possible SOC content change factors such as cover crops and green manure, organic manure application, soil tillage intensity and temporary meadow.
2 Materials and Methods
2.1 Study Area
This study was conducted on 120 farms from Western Switzerland (Lake Geneva region), namely Geneva and Vaud cantons, western Switzerland (Figure 1). The climate is oceanic (Cfb) in the plains according to Köppen-Geiger climate types (Peel et al., 2007). The dominant soil type is Cambi-Luvisol (IUSS Working Group WRB, 2014), developed on calcareous Pleistocene moraines mixed with some Tertiary molasse fragments (local parent rock). Other soil types were not considered, and only farms with annual crops in the rotation were included (livestock farms relying exclusively on permanent meadows were not considered).
To receive ecological subsidies, Swiss farmers are required to analyze a topsoil (0–20 cm) composite sample from each of their field in a certified laboratory at least every 10 years. This sampling method was assessed as an unbiased and reliable method to determine SOC annual change rates at farm scale (Deluz et al., 2020). The corresponding database contains more than 35′000 soil analyses from 1993 to present and was used by Dupla et al. (2021) to determine the regional SOC annual change rate distribution and its change with time. The corresponding parameters are summarized in Table 1.
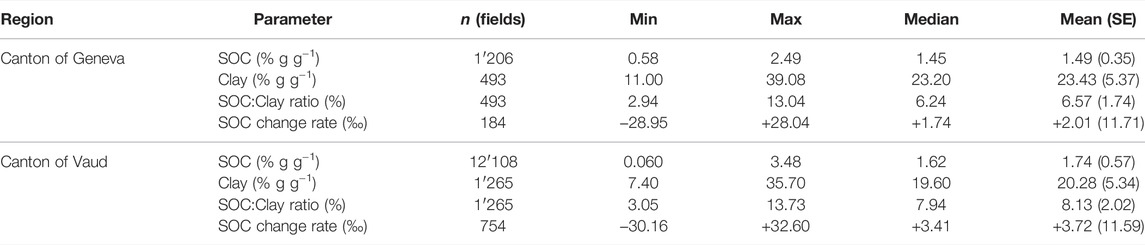
TABLE 1. Distribution of soil organic carbon (SOC) (%), clay (%), SOC to clay ratio (%), SOC:Clay ratio (%) and annual SOC change rates (‰) for Geneva and Vaud croplands.
2.2 Farm Selection and Data Collection
The 120 farms were randomly selected using the regional farming directory. One field per farm was randomly selected and the mandatory information recorded by farmers in their logbooks over the past 10 years was used to describe the farming practices conducted on the field. These include the different operations conducted on each field (soil preparation, fertilization, treatments, yield, cover-crops, and rotation) with the date and quantity of each product used or harvested. One-to-one in-depth interviews were conducted with the 120 farmers to validate their practices and complete them in case of missing information in the logbook. The survey included the different farming types in the region, such as organic, conventional, presence or absence of livestock.
We focused on the cropping practices potentially impacting SOC content changes, namely temporary meadow duration, cover-crop properties, organic matter application, soil tillage intensity and rotation diversity. These factors are described below and summarized in Table 2.
2.2.1 Cover-Crops
The numbered cover-crops only included green-manure, i.e., cover-crops whose residues are left on the field. Harvested or grazed cover-crops were considered as standard crops. The cover-crop biomass was not recorded in the past-ten years; however, we could calculate 1) the number and duration of long-lasting cover-crops (between summer harvest and a spring crop seeding); 2) the number and duration of short cover-crops (between a summer harvest and an autumn-crop seeding); and 3) the number of species in the cover-crops.
However, there is an obvious relationship between the number of cover-crops and the temporary meadow duration. Therefore, to better account for the efforts of the farmer to cover the soil independently from the proportion of temporary meadow in the rotation, an uncovered soil score was created to quantify cover-cropping missed opportunities. Over the rotation, the number of fallow periods longer than 6 weeks and not covered with a cover-crop was divided by the number of annual crops and then normalized to a 10-year period. For instance, in a 6-years rotation made of 4 annual crops and 2 years of temporary meadows, a farmer who has missed 2 opportunities to use cover crops would get a score of 2*10/4 = 5 equivalent to 5 cover-cropping missed opportunities over a 10-years rotation made only of annual crops.
2.2.2 Organic Matter Application
Organic amendments were standardized as humified organic matter inputs in t.ha−1 over the 10-year period. The amount of organic matter application (manure, slurry, digestate, and compost) was transformed to humified organic matter inputs depending on the organic amendment nature and form using the coefficients of specific stability index of organic matter (ISMO) (Bouthier et al., 2014) used in the AMG model (Andriulo et al., 1999; Levavasseur et al., 2020).
2.2.3 Soil Tillage Intensity Rating
The index of Soil Tillage Intensity Rating (STIR) (USDA, 2003) was used to estimate overall soil disturbance based on specific standardized scores attached to soil management practices. A single tillage operation to 23 cm depth gives for instance a score of 74, while direct seeding would give a score of 4. For each investigated field, all soil management practices over the last 10 years were considered to calculate STIR values. The cumulated values were then divided by ten to obtain the variable subsequently referred to as STIR.
Like cover-crops, the average tillage intensity over 10 years is highly influenced by the share of temporary meadow. To take into account the soil tillage intensity applied on annual crops regardless of temporary meadow duration in the rotation, a second index denoted STIRAC was also calculated over the annual crops duration in the rotation and then normalized to 10 years.
2.2.4 Temporary Meadow
Temporary meadow duration was expressed as percentage over the 10-year period.
2.3 Soil Sampling and Analysis
The selected fields were sampled at the beginning and the end of the 10-years interval by collecting 15 to 20 aliquots along the field diagonals at 0–20 cm depth to obtain a composite sample (Deluz et al., 2020). SOC and clay contents (wt%) were analyzed using Walkley-Black (Nelson and Sommers, 1983) and pipette methods (Jennings et al., 1922; Robinson, 1922), respectively.
This allowed the SOC:Clay ratio of the field to be calculated. The 10-years mean SOC annual change rate (‰) of each field being the equivalent of the common ratio in a geometric sequence, it was calculated using Eq. 1 (see Supplementary Material for additional computational explanations).
with SOC1 and SOC2 being the SOC contents of the soil at the beginning (SOC1) and end (SOC2) of the period, and n the number of years between the two analyses (n = 10).
2.4 Statistical Analysis and Modelling
All statistical analyses and modelling were performed with R (v 4.0.3, R Development Core Team 2020). Variables of interest included the SOC annual change rate, the clay content, the initial SOC content, the initial SOC:Clay ratio and eight cropping practices averaged over the 10 years (Table 2). All variables were quantitative. For each cropping practice, we also created a class variable which was subsequently used in an analysis of covariance (see below).
For each quantitative variable, the mean and median were calculated to represent the central tendency and the minimum, maximum and standard error of the mean (SEM) to represent dispersion. Linear models (simple and multiple regressions, analyses of variance and analyses of covariance—see details below) were then used to investigate relationships between the SOC annual change rate, soil properties, cropping practices and cantons. The α level for model and effect significance was set at 0.05 unless stated otherwise. Approximate normality and homogeneous variance of model residuals were assessed on residual plots.
A one-way analysis of variance (ANOVA) was conducted to test for differences in the SOC annual change rate, soil properties and cropping practices between the two cantons. We investigated bivariate linear relationships between quantitative variables by calculating Pearson’s correlation coefficients (see Supplementary Table S1). Significant correlations between the SOC annual change rate and other variables were further described with simple linear regressions.
A multiple regression analysis was subsequently conducted to account for the cumulative effect of soil properties and cropping practices on the SOC annual change rate (McCullagh and Nelder, 1989). The dependent variable was the SOC annual change rate, and predictors were selected among variables representing cropping practices and the initial SOC:Clay ratio. To maximize validity, reliability and parsimony (Chatfield, 1995), the regression analysis was not performed on the maximum model (the model containing all the potential predictors). Unimportant variables were first discarded during a model selection process consisting of a stepwise selection using an α level of 0.15 for both variable entry and variable removal. A permissive alpha level of 0.15 was used to make sure that any predictor potentially influencing the response variable remained included in the model. The multiple regression analysis was then conducted on the resulting model; the significance of predictors was from here on assessed using the regular alpha level of 0.05. To reduce model instability arising from variable collinearity, we excluded moderately to highly correlated predictors, defined here as variables having absolute correlation coefficients greater than 0.3. Multi-collinearity was low, and partial least square or ridge regression approaches did not yield any improvement.
To check for the robustness of the multiple regression result, we repeated the analysis using a covariance model, with the SOC annual change rate as dependent variable, the SOC:Clay ratio as a covariate, and cropping practices as class effects. Models using class variables as predictors are generally more robust to potential collinearity effect. Results were similar to what was obtained using multiple regression approaches and are presented in the Supplementary Material (see Supplementary Table S2). Due to the relatively high number of predictors, interaction effects could not robustly be assessed.
We used a Principal Component Analysis (PCA) (Wold et al., 1987) based on the correlation matrix to illustrate the relationship between the significant agricultural practices related to SOC annual change rates.
3 Results
3.1 Properties of the Sampled Fields and Surveyed Cropping Practices
The selected farms have a mean cultivated surface area of 50 ha, which is slightly above the mean size observed in the region (36 ha) and 15% of the farms were under Swiss organic farming label, which is slightly above the regional mean (11%). 48% of the farms had no livestock, and the average livestock units in the other farms was 10 which lies in the mean regional range (5.8–20.5).
The properties of the studied fields and the related cropping practices averaged on the 10-year period are reported in Table 3. Due to differences in agriculture management history, the cropping systems in the two cantons showed some differences. Livestock was more developed in Vaud farms than in Geneva farms. Geneva canton subsidizes cover crops of short fallow periods (e.g., from barley to colza) contrary to Vaud, and the rotations show little variation in spring crop frequency in Geneva compared to Vaud, which explains the differences in the corresponding cover-crop properties. However, the canton effect was not significant on the SOC annual change rate and its relationships with other variables.
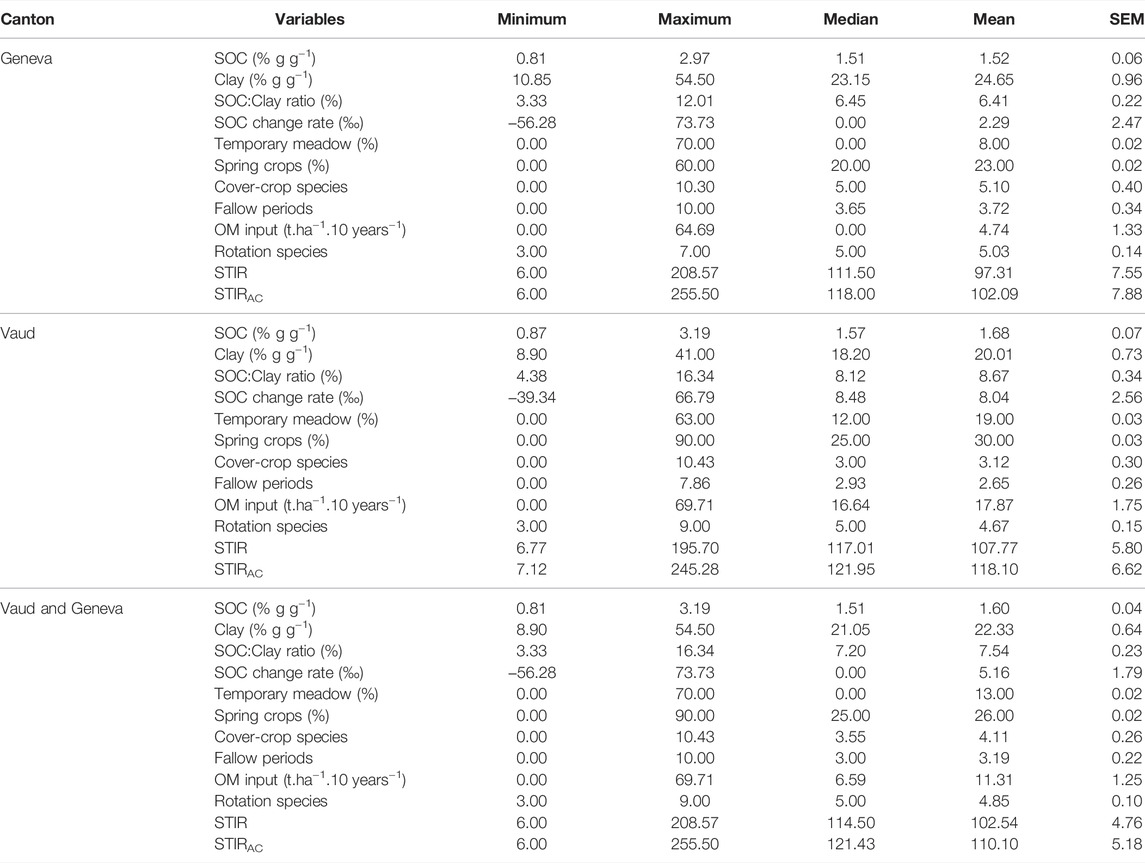
TABLE 3. Summary of the main soil properties and cropping practices. Soil organic carbon content (SOC) (%) and clay content (%) at the beginning of the 10-year period and their ratio (SOC:Clay ratio) (%). Rate of SOC annual change (‰). Share of temporary meadows in 10 years (%). Share of spring crops in 10 years (%). Mean number of species in the cover-crops. Number of fallow periods in 10 years. Cumulated organic matter amendments in humified organic matter in tons per 10 years. Number of species in the rotation. STIR: Mean Soil Tillage Intensity Rating per year over the 10-year period. STIRAC: Mean Soil Tillage Intensity Rating per year over the 10-year period.
The distributions of initial SOC and clay contents are presented in Figure 2, together with the values observed in the regional data base used in Dupla et al. (2021). As can be seen from their centroid and their distribution, the sampled fields from the 120 farms are representative of the regional SOC and clay contents, and SOC:Clay ratio distribution.
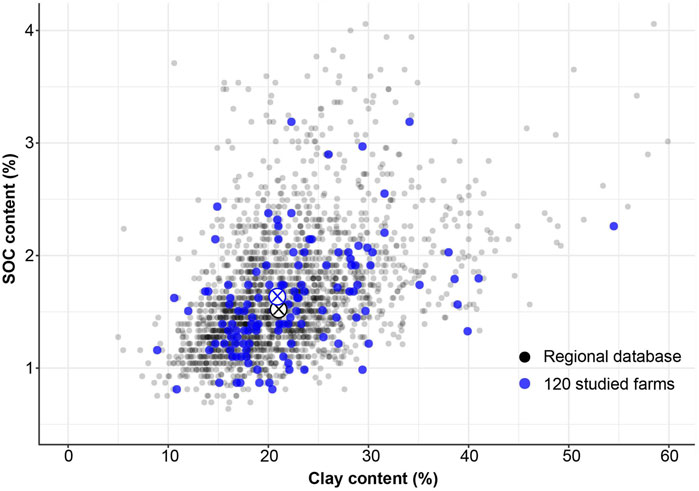
FIGURE 2. SOC vs. clay contents (circles) and respective centroids (circled X) of soil samples from the regional Geneva and Vaud database (grey) and from sampled fields of the 120 surveyed farms (blue).
The observed rates of SOC annual change show a median value of 0 and ranged from −56‰ to +74‰ These change rates display a similar pattern to the rates found at the regional scale by Dupla et al. (2021) (Table 1 and Table 3) despite a higher dispersion compared with the minimum and maximum rates (−30.12 and +32.47‰, respectively) in both cantons. According to ANOVA, the soils from Vaud had significantly less clay and a higher SOC:Clay ratio than in Geneva, but the SOC change rate was not significantly different between cantons, which is similar to the observations of the regional database study.
3.2 Pearson Correlations and Linear Regressions
Significant Pearson correlation coefficients between the observed rates of SOC annual change, the soil analyses, and the investigated cropping practices are presented in Table 4.
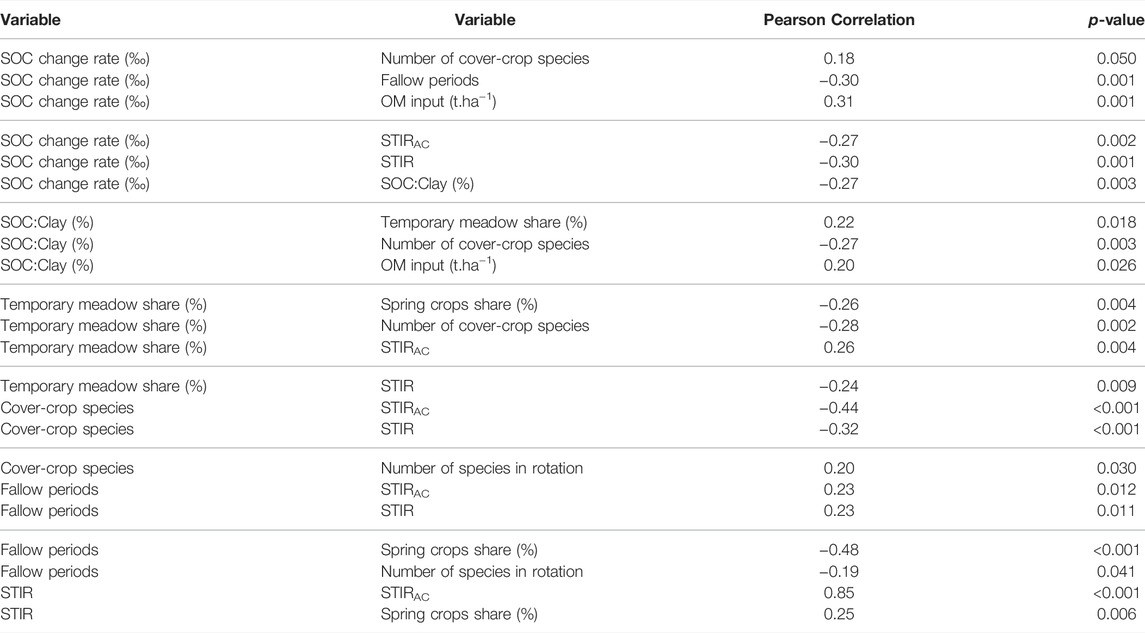
TABLE 4. Pearson correlation coefficients and p-value of the significant correlations between rates of SOC annual change, cropping practices, and soil properties. SOC refers to the soil organic carbon content at the beginning of the 10-year period.
Among the cropping practices, only the cover-crop related properties, STIR, and OM inputs showed significant correlation with the SOC annual change rate. Among the cover-crop properties, the number of fallow periods showed the largest and most significant correlation to SOC change rate (−0.3, p-value = 0.0009) and was, therefore, used in the multivariate analysis. The number of species in the cover-crops showed a positive effect on the SOC change rate (0.18, p-value = 0.0496). The STIR (−0.3, p-value = 0.001) and the STIRAC (−0.27, p-value = 0.002) were negatively correlated to the SOC change rate. Due to its stronger significance, the STIR rather than the STICAC was used in the multivariate analysis. The organic matter input was positively correlated to SOC change rates (0.26, p-value = 0.0042). Interestingly, neither the proportion of temporary meadow in the rotation nor the crop rotation diversity had a significant effect on the SOC annual change rate. The linear regressions between SOC annual change rates, SOC:Clay ratios and cropping practices are presented in Figure 3.
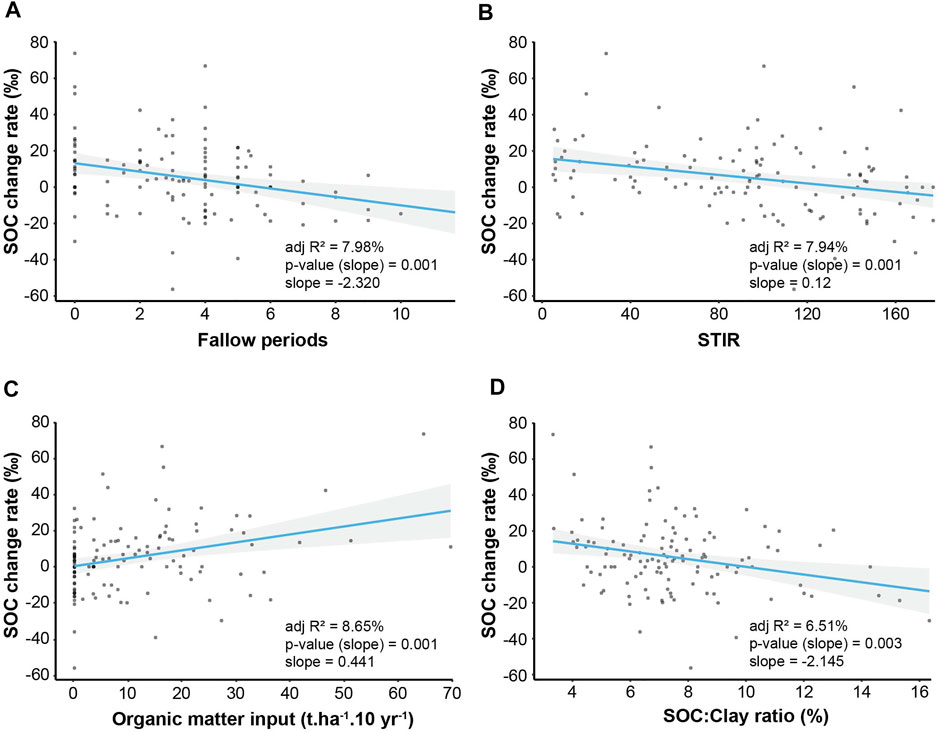
FIGURE 3. Linear regressions between SOC annual change rates (‰) and cropping practices ((A): fallow periods, (B): Soil Tillage Intensity (STIR) and (C): organic matter input) and between SOC annual change rates (‰) and initial SOC:Clay ratio (%) (D).
Among the soil properties, the SOC content and SOC:Clay ratio at the beginning of the 10-year period showed a negative correlation with the SOC annual change rate, namely −0.26 (p-value = 0.004) and −0.27 (p-value = 0.003), respectively. The correlations with the annual change rates and the other SOC change factors were more significant when using SOC:Clay ratio rather than with SOC alone (see Supplementary Table S1).
Some practices were significantly cross correlated. The STIR showed negative correlation to the cover-crops related indexes, such as number of species (−0.32, p-value < 0.001) and fallow periods (0.23, p-value = 0.01), as well as with temporary meadow duration (−0.24. p-value = 0.009) and spring crops share (0.25, p-value = 0.006). STIRAC showed similar correlations than STIR to all practices except spring crops (no correlation). Moreover, it showed a positive correlation to temporary meadow share (0.26, p-value = 0.004), contrary to STIR. Organic matter input was positively correlated to temporary meadow duration (0.21, p-value = 0.0236), and negatively correlated to the number of species in the cover-crops (−0.21, p-value = 0.0222) and fallow periods (−0.25, p-value = 0.006). This latter was negatively correlated to temporary meadow duration (−0.3, p-value = 0.0008).
3.3 Multivariate Analysis
According to stepwise regression, the independent variables that showed significant contribution to SOC change rates were the SOC:Clay ratio at the beginning of the period, fallow periods, STIR, and OM inputs. Since the covariance model with practices as class effects provided similar results to the linear regression model, we only present results from the latter (see Supplementary Table S2 for covariance results).
The results of the stepwise regression are presented in Table 5. All variables left in the model, namely the initial SOC:Clay ratio, fallow periods, STIR, and OM inputs, are significant at the 0.05 level. No other variable met this significance level for entry into the model. Introducing organic farming yielded no significant effect as well.

TABLE 5. General linear model of SOC annual change rates as a function of farming practices and soil properties.
According to stepwise regression analysis, OM input had a positive influence on SOC change rates while the initial SOC:Clay ratio, the proportion of fallow periods, and the tillage intensity had a negative influence. Other predictors such as meadow and spring crop share were not significant. The ranking of the relative effects, based on the partial R2, was not performed because of small effects and some collinearity among predictors. The corresponding model was additive and accounted for 33% variance of the annual SOC change rate.
The combined effect of the practices was illustrated by representing the linear relationships between SOC change rates and SOC:Clay ratios for different categories of OM input (Figure 4A) and STIR (Figure 4B). In both cases, the interaction between the categories and the slopes were not significant. Therefore, we kept a constant slope for the categories. Figure 4A shows that the larger the SOC:Clay ratio, the smaller the annual SOC change rate for a given OM input, with neutral SOC change rates on average at a SOC:Clay ratios of 7 and 12% for OM input categories of <5 t ha−1 y−1 and >5 t ha−1 y−1, respectively.
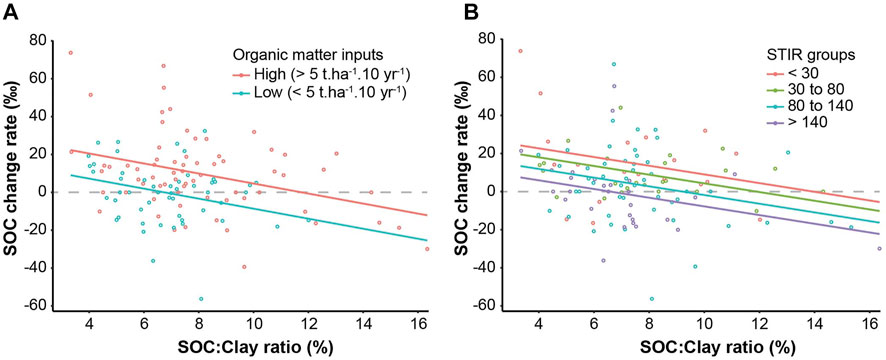
FIGURE 4. Panel (A) Linear relationships between annual rate of change in soil organic carbon content (SOC) and SOC:Clay ratio for “low” (<5 t ha−1 y−1) and “high” (>5 t ha−1 y−1) organic matter input categories with constant slope (−2.65). Panel (B) Linear relationships between annual rate of change in soil organic carbon content (SOC) and SOC:Clay ratio for soil tillage intensity (STIR) categories with constant slope (−2.28). Linear Intercepts, R2 and p-values (for slope and intercept) of each group can be found in Supplementary Table S3.
Figure 4B illustrates the role of the initial clay saturation status (SOC:Clay ratio) with respect to SOC increase and tillage intensity. Positive SOC change rates with large tillage intensities are mostly observed at small SOC:Clay ratios and more generally, the higher the SOC:Clay ratio, the lower the STIR needs to be to allow for a SOC increase. In other words, no-till becomes increasingly necessary at large SOC content, but is not required to increase SOC content at low clay saturation level.
4 Discussion
4.1 Significant Predictors Influencing Soil Organic Carbon Annual Change Rates
The correlations between the cropping practices depict different cropping systems that can be summarized as follows. The proportion of temporary meadow is largely varying between farms and between cantons. The larger this proportion, the higher the organic matter input, the initial SOC:Clay ratio, the STIRAC, the proportion of fallow periods corresponding to cover-cropping missed opportunities in the rotation, the spring crops share, and the lower the cover-crop diversity. Moreover, regardless of temporary meadow duration, STIR was highly variable, and the use of cover-crops (duration and diversity) was inversely proportional to the mechanical intensity (see Supplementary Figure S1). This means that 1) farmers having livestock and temporary meadow tend to apply high mechanical intensity and neglect cover-crops while applying more OM inputs; that 2) mechanical intensity in general jeopardizes cover-crop intensification; and that 3) farmers having no temporary meadows tend to reduce soil tillage intensity and to grow cover-crops to regenerate effective soil quality losses due to decades of intensive agriculture.
Data analysis shows that the only factors influencing significantly rates of SOC annual change are the cover-crops (intensity and diversity, positive effect), organic matter inputs (positive effect), and the mechanical intensity applied to the annual crops (negative effect). They represent, therefore, the pillars of SOC increase. Organic farming, temporary meadow duration as well as rotation diversity showed no significant effect, though often referred-to in the literature as soil regenerating and/or carbon sequestration factors (Fließbach et al., 2007; Senapati et al., 2014; Autret et al., 2016; Blanco-Canqui et al., 2017; Chenu et al., 2019; Colombi et al., 2019). Rotation diversity did not vary on a large range in this survey (Table 3). Moreover, longer rotations corresponded to contrasting strategies. In some cases, it corresponded to the addition of a “cash-crop,” namely potatoes or sugar-beet, while the longer rotation was also negatively correlated to fallow periods, thus corresponding to an increased use of the cover-crops and decreased tillage intensity.
Organic matter application and cover-crops intensity and diversity are highlighted in many studies as factors of SOC increase (Maltas et al., 2013; O’Connell et al., 2015; Ruis and Blanco-Canqui, 2017; Büchi et al., 2018; Wendling et al., 2019). Their significant effect is particularly remarkable in this survey because the cover-crop biomass was not available, though it is assumed to be a key factor (Blanco-Canqui et al., 2015; Poeplau and Don, 2015) and should be even more strongly correlated to SOC change rates than our available information. However, the decreasing number of fallow periods and the number of species in the cover-crops can be considered as indicators of farmers motivation, thus increasing the likelihood of higher biomass of cover-crops. This is a common observation made by the authors and the advising services in the region (Wendling et al., 2017).
4.2 Impact of Temporary Meadows and Organic Farming
The non-significant effect of the proportion of temporary meadow on SOC change rates (see Supplementary Figure S2), despite its large range (from 0 to 70% of the past 10-years rotations in this survey, Table 3), is particularly surprising with respect to the common acknowledgment that temporary meadow may be a guarantee of sustainable soil management in agriculture. However, temporary meadow duration was positively correlated to factors associated with SOC decrease (namely the STIRAC applied on the annual crops in the rotation, the number of fallow periods, and the SOC:Clay ratio), whereas it was negatively correlated to the number of species in the cover-crops which is considered as a key factor or SOC increase (e.g., Ranaldo et al., 2020). Moreover, there was no correlation between temporary meadow and OM inputs, which might be due to increased liquid manure and digestate application with increasing temporary meadow share, which is growingly observed. Temporary meadows, therefore, are not conducive to increasing the SOC content, especially when SOC:Clay ratio was already high. From this point of view 1) the larger SOC:Clay ratio observed with temporary meadows appears to be inherited from the past and is decreasing and 2) this should be mitigated by increasing OM inputs and/or decreasing tillage intensity and increasing cover-crop intensity on the annual crops.
Organic farming had no significant effect on SOC change rates as a predictor (see Supplementary Figure S3). It performed according to the intensity of application of the SOC increase factors. Organic farming is often associated with livestock and manure application but also to a high STIR for weed control, which is the case in this data set (see Supplementary Figure S4).
4.3 Soil Tillage is the Only Significant Factor Decreasing Soil Organic Carbon Annual Change Rates
The only practice accounting for SOC content decrease was the mechanical intensity. This may appear contradictory to some findings, such as Dimassi et al. (2014) or Powlson et al. (2014), who reported limited or no effect of tillage compared with no-till practices. However, these results were obtained in LTEs. They were observed under constant treatment over a long time, with site-specific SOC:Clay ratio, cropping practices and climate conditions, and with tillage as unique difference between the treatments. Such research is designed to quantify the potential SOC mineralization by tillage. Conversely, our observations cover a large range of soil conditions and practices interacting in the cropping systems. In particular, Table 4 shows that the Pearson correlation coefficient between STIR and fallow periods was positive (0.31, p-value = 0.0006). This can be related to a well-known observation that working the soil after a summer harvest not only delays the seeding of the cover-crop, but also dries out the soil down to the tillage limit, thus retarding seed emergence until significant rainfall events, usually occurring during fall in this region (Büchi et al., 2018). As a result, many farmers choose not to seed cover-crops in such conditions, or the seeded cover-crop shows a late development and reaches small biomass. Therefore, although the present on-farm results cannot adjudicate on the precise impact of soil tillage on SOC mineralization, they show that in a systemic perspective, soil tillage compromises the main SOC increase factor, namely cover-crops.
Additionally, threshold values of mechanical intensity can be derived from Figure 4B. Fields with conventional tillage (STIR > 140), conservation tillage (80 > STIR > 140) or no-till (STIR < 30) display positive SOC change rates up to SOC:Clay ratios of 7, 10 and 14% on average, respectively. These latter SOC:Clay ratios coincide with the soil structure vulnerability thresholds for poor, acceptable and optimal soil structures, respectively, (Johannes et al., 2017; Prout et al., 2020), thus confirming the relevance of these thresholds for SOC management.
4.4 Multivariate Analysis and Insights for Soil Organic Carbon Content Management
Stepwise regression analysis provides additional information to the Pearson correlations. The model accounts for 33% of the variance of the SOC annual change rate, which may be considered small. However, there are many reasons for this limited R2, because 1) the independent variables were collected from farmers and averaged over 10 years, which does not mean that they were constant during this period since cropping systems are continuously changing; 2) the climate conditions were different each year, with alternating wet and dry conditions in spring and autumn, thus sharply modifying the harvest dates and the cover-crops development conditions; and 3) the cover-crop biomass was not known though most likely representing the key information, which could be assessed by simulating the cover-crop biomass with the collected information and meteorological data. Figure 5 presents the distribution of the fields with respect to the two first principal components of the retained three agricultural practices accounting for SOC content change. The more intensely one practice was used, the more towards the practice’s arrowhead each field would be positioned. Conversely, if one practice was avoided (e.g., no-till for the STIR parameter) then the field would be located at the opposite end of the practice’s arrow in the PCA biplot. The SOC change rate categories display a gradual shift of concentration ellipses towards higher STIR, organic matter inputs and fallow period values as SOC change rates decrease. This gradual distribution casts light on the beneficial impact of low tillage intensity, organic amendments and intense cover-cropping on SOC content change.
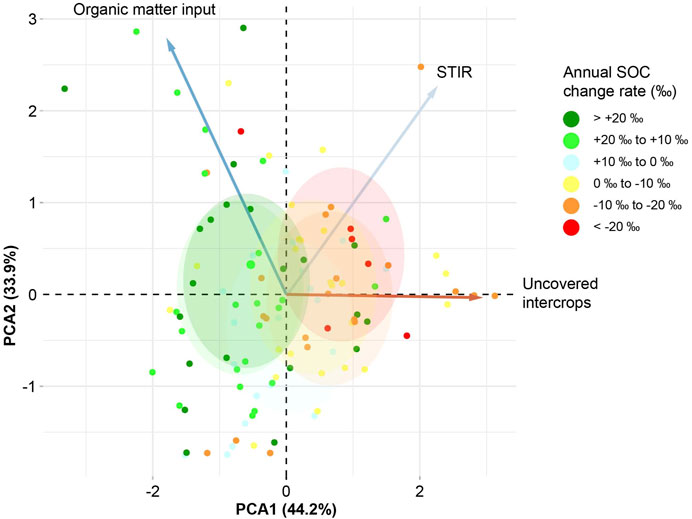
FIGURE 5. PCA biplot of the 120 studied fields together with the agricultural practices retained in the linear model projected on plane of the first two principal components (variance in %). Each field is colored according to its annual SOC change rate (‰). Euclidean concentration ellipses follow the same color scale.
The absence of clear statistical interactions implies that the roles of the different factors are additive. If so, farmers may select which SOC increase factors to emphasize when adapting their system. However, the higher the clay saturation by SOC, the more intense the use of the different factors should be, as illustrated for instance with tillage intensity in Figure 4B. Therefore, farmers could select the intensity to apply on the different SOC increase factors depending 1) on their cropping system, 2) whether they are in a regeneration strategy or not, and 3) their SOC:Clay ratio.
The observed SOC annual change rates are consistent with the regional study of Dupla et al. (2021). Contrary to findings in Swiss LTEs (Keel et al., 2019) many Swiss farms do increase their SOC content at a larger rate than 4‰ and the factors seem clearly identified. However, large negative SOC change rates are also observed, which could be corrected in the future by applying the corresponding changes in the cropping practices.
4.5 Implications for Soil Research
Together with the study of Dupla et al. (2021), these results may seem very contradictory with previous findings on SOC dynamics in arable land. The impact of tillage on SOC dynamics is considered negligible by Powlson et al. (2014) or Dimassi et al. (2014) while its strong negative effect appears in this on-farm study. In Swiss LTEs, all cropping practices, including conservation agriculture, induced a decrease in SOC according to the study by Keel et al. (2019) while high rates of SOC content increase are found on-farm. Experimental conditions in LTEs can deviate significantly from on-farm conditions, as illustrated in this study. On-farm practices are the result of systemic decisions considering different factors that co-evolve from year to year, whereas in LTEs we aim to quantify mechanistic processes by comparing the effects of single factors over the long term from a unique research perspective. For example, our results show that the higher the SOC:Clay ratio, the more detrimental the mechanical intensity can be to the SOC content, whereas in a field experiment dealing with this issue, only a narrow range of SOC:Clay ratios can be considered, thus excluding the observation of this effect. Furthermore, we have highlighted the negative interaction between tillage and cover crops, which is the result of systemic constraints and farmers’ choices, another effect that will not be revealed in the LTE.
These observations call for a thorough reflection when selecting research strategies, with a clear distinction between objectives. Experimentation under controlled conditions is of paramount importance to decipher mechanisms and quantify processes. In addition, field trials can provide useful demonstrations and stimulate farmer innovation. However, the transfer of these results to the farm may not be straightforward and may even lead to misinterpretation. On-farm soil quality management clearly requires systemic research, especially for key issues such as carbon sequestration and the preservation of soil functions.
5 Conclusion
In 120 farms representative of Western Switzerland (Lake Geneva region) agricultural sector, the most significant factors for SOC content increase or decrease corresponded to the mechanical intensity applied on annual crops (negative effect), the organic matter inputs (positive effect), and the frequency and diversity of non-harvested cover-crops (positive effect). The temporary meadow duration had no effect on the SOC content change, due to adverse practices on the annual crops of the corresponding rotations, namely larger mechanical intensity and lower cover crop frequency with increasing meadow duration. Moreover, organic matter application did not increase with temporary meadow. Therefore, the larger SOC to clay ratios observed with temporary meadow were interpreted as inherited from the past. In such a case, temporary meadows do not guarantee sustainable soil quality management.
The factors for SOC increase or decrease were additive. Clay saturation by SOC decreased the positive effects of organic matter inputs and cover-crops and increased the negative effects of tillage intensity. The cover-crop intensity and diversity were negatively correlated to tillage intensity, which may explain the significant negative effect of tillage intensity contrary to what was reported in some field experiments. This is consistent with a common on-farm observation that tilling the soil before seeding a cover-crop jeopardizes the chances to yield high biomass cover-crops, thus discouraging many farmers to seed expensive multi-species cover-crops especially during summer short fallow periods.
The observed SOC increases are consistent with the regional study of Dupla et al. (2021). In contrast with the results obtained in the Swiss LTEs (Keel et al., 2019), Swiss farms can increase their SOC content at a rate greater than 4‰, although large negative change rates can also be found, which can be corrected in the future by applying changes in cropping practices. These results define a roadmap for SOC-increasing cropping systems in the region. The roadmap is based on progressive changes in the intensity of the different SOC content levers, depending on the current SOC:Clay ratio of the fields.
Author Contributions
XD, TL and KG collected the data under the supervision of PB. SG performed the analytic calculations and statistical modelling. XD and PB wrote the manuscript with support from SG, EV and RC. All authors provided critical feedback and helped shape the content of this study.
Funding
Open access funding provided by University of Lausanne.
Conflict of Interest
The authors declare that the research was conducted in the absence of any commercial or financial relationships that could be construed as a potential conflict of interest.
Publisher’s Note
All claims expressed in this article are solely those of the authors and do not necessarily represent those of their affiliated organizations, or those of the publisher, the editors and the reviewers. Any product that may be evaluated in this article, or claim that may be made by its manufacturer, is not guaranteed or endorsed by the publisher.
Acknowledgments
The authors thank Sebastien Gassmann, Soil Protection Division, Geneva, and François Füllemann, Soil Protection Division, Vaud, Nicolas Courtois, Agrigenève agricultural advisory association, Geneva and Andy Gray, English editor, for facilitating this work.
Supplementary Material
The Supplementary Material for this article can be found online at: https://www.frontiersin.org/articles/10.3389/fenvs.2022.834055/full#supplementary-material
Abbreviations
AMG, model from Andriulo, Mary and Guerif on soil carbon dynamics (Andriulo et al., 1999); ANOVA, Analysis of Variance; ISMO, stability index of organic matter; LTE, Long Term Experiment; OM, Organic Matter; PCA, Principal Component Analysis; SEM, Standard Error of the Mean; SOC, Soil Organic Carbon; STIR, Soil Tillage Intensity Rating; STIRAC, Soil Tillage Intensity Rating calculated over annual crops’ duration.
References
Amelung, W., Bossio, D., de Vries, W., Kögel-Knabner, I., Lehmann, J., Amundson, R., et al. (2020). Towards a Global-Scale Soil Climate Mitigation Strategy. Nat. Commun. 11, 5427. doi:10.1038/s41467-020-18887-7
Andriulo, A., Mary, B., and Guerif, J. (1999). Modelling Soil Carbon Dynamics with Various Cropping Sequences on the Rolling Pampas. Agronomie 19, 365–377. doi:10.1051/agro:19990504
Autret, B., Mary, B., Chenu, C., Balabane, M., Girardin, C., Bertrand, M., et al. (2016). Alternative Arable Cropping Systems: A Key to Increase Soil Organic Carbon Storage? Results from a 16 Year Field experiment. Agric. Ecosyst. Environ. 232, 150–164. doi:10.1016/j.agee.2016.07.008
Baveye, P. C., Berthelin, J., Tessier, D., and Lemaire, G. (2018). The “4 Per 1000” Initiative: A Credibility Issue for the Soil Science Community? Geoderma 309, 118–123. doi:10.1016/j.geoderma.2017.05.005
Baveye, P. C., Schnee, L. S., Boivin, P., Laba, M., and Radulovich, R. (2020). Soil Organic Matter Research and Climate Change: Merely Re-storing Carbon versus Restoring Soil Functions. Front. Environ. Sci. 8. doi:10.3389/fenvs.2020.579904
Blanco-Canqui, H., Francis, C. A., and Galusha, T. D. (2017). Does Organic Farming Accumulate Carbon in Deeper Soil Profiles in the Long Term? Geoderma 288, 213–221. doi:10.1016/j.geoderma.2016.10.031
Blanco-Canqui, H., Shapiro, C. A., Wortmann, C. S., Drijber, R. A., Mamo, M., Shaver, T. M., et al. (2013). Soil Organic Carbon: The Value to Soil Properties. J. Soil Water Conservation 68, 129A–134A. doi:10.2489/jswc.68.5.129A
Blanco-Canqui, H., Shaver, T. M., Lindquist, J. L., Shapiro, C. A., Elmore, R. W., Francis, C. A., et al. (2015). Cover Crops and Ecosystem Services: Insights from Studies in Temperate Soils. Agron. J. 107, 2449–2474.
Bouthier, A., Duparque, A., Mary, B., Sagot, S., Trochard, R., Levert, M., et al. (2014). Adaptation et mise en øeuvre du modèle de calcul de bilan humique à long terme AMG dans une large gamme de systèmes de grandes cultures et de polyculture-élevage. Innov. Agronomiques 34, 125–139.
Büchi, L., Georges, F., Walder, F., Banerjee, S., Keller, T., Six, J., et al. (2019). Potential of Indicators to Unveil the Hidden Side of Cropping System Classification: Differences and Similarities in Cropping Practices between Conventional, No-Till and Organic Systems. Eur. J. Agron. 109, 125920. doi:10.1016/j.eja.2019.125920
Büchi, L., Wendling, M., Amossé, C., Necpalova, M., and Charles, R. (2018). Importance of Cover Crops in Alleviating Negative Effects of Reduced Soil Tillage and Promoting Soil Fertility in a winter Wheat Cropping System. Agric. Ecosyst. Environ. 256, 92–104. doi:10.1016/j.agee.2018.01.005
Bünemann, E. K., Bongiorno, G., Bai, Z., Creamer, R. E., De Deyn, G., de Goede, R., et al. (2018). Soil Quality - A Critical Review. Soil Biol. Biochem. 120, 105–125. doi:10.1016/j.soilbio.2018.01.030
Chambers, A., Lal, R., and Paustian, K. (2016). Soil Carbon Sequestration Potential of US Croplands and Grasslands: Implementing the 4 Per Thousand Initiative. J. Soil Water Conservation 71, 68A–74A. doi:10.2489/jswc.71.3.68A
Chatfield, C. (1995). Model Uncertainty, Data Mining and Statistical Inference. J. R. Stat. Soc. Ser. A (Statistics Society) 158, 419–466. doi:10.2307/2983440
Chen, S., Arrouays, D., Angers, D. A., Martin, M. P., and Walter, C. (2019). Soil Carbon Stocks under Different Land Uses and the Applicability of the Soil Carbon Saturation Concept. Soil Tillage Res. 188, 53–58. doi:10.1016/j.still.2018.11.001
Chenu, C., Angers, D. A., Barré, P., Derrien, D., Arrouays, D., and Balesdent, J. (2019). Increasing Organic Stocks in Agricultural Soils: Knowledge Gaps and Potential Innovations. Soil Tillage Res. 188, 41–52. doi:10.1016/j.still.2018.04.011
Colombi, T., Walder, F., Büchi, L., Sommer, M., Liu, K., Six, J., et al. (2019). On-farm Study Reveals Positive Relationship between Gas Transport Capacity and Organic Carbon Content in Arable Soil. SOIL 5, 91–105. doi:10.5194/soil-5-91-2019
Cook, S., Cock, J., Oberthür, Th., and Fisher, M. (2013). On-Farm Experimentation. Better Crops 97, 17–20.
De Vries, W. (2018). Soil Carbon 4 Per Mille: a Good Initiative but Let’s Manage Not Only the Soil but Also the Expectations. Geoderma 309, 124–129. doi:10.1016/j.geoderma.2017.05.026
Deluz, C., Nussbaum, M., Sauzet, O., Gondret, K., and Boivin, P. (2020). Evaluation of the Potential for Soil Organic Carbon Content Monitoring with Farmers. Front. Environ. Sci. 8, 113. doi:10.3389/fenvs.2020.00113
Dexter, A. R., Richard, G., Arrouays, D., Czyż, E. A., Jolivet, C., and Duval, O. (2008). Complexed Organic Matter Controls Soil Physical Properties. Geoderma 144, 620–627. doi:10.1016/j.geoderma.2008.01.022
Dignac, M.-F., Derrien, D., Barré, P., Barot, S., Cécillon, L., Chenu, C., et al. (2017). Increasing Soil Carbon Storage: Mechanisms, Effects of Agricultural Practices and Proxies. A Review. Agron. Sustain. Dev. 37, 14. doi:10.1007/s13593-017-0421-2
Dimassi, B., Mary, B., Wylleman, R., Labreuche, J., Couture, D., Piraux, F., et al. (2014). Long-term Effect of Contrasted Tillage and Crop Management on Soil Carbon Dynamics during 41 Years. Agric. Ecosyst. Environ. 188, 134–146. doi:10.1016/j.agee.2014.02.014
Dupla, X., Gondret, K., Sauzet, O., Verrecchia, E., and Boivin, P. (2021). Changes in Topsoil Organic Carbon Content in the Swiss Leman Region Cropland from 1993 to Present. Insights from Large Scale On-Farm Study. Geoderma 400, 115125. doi:10.1016/j.geoderma.2021.115125
EASAC (2019). Forest Bioenergy, Carbon Capture and Storage, and Carbon Dioxide Removal: An Update. Halle: German National Academy of Sciences Leopoldina.
EASAC (2018). Negative Emission Technologies: What Role in Meeting Paris Agreement Targets?. Halle: German National Academy of Sciences Leopoldina.
Fließbach, A., Oberholzer, H.-R., Gunst, L., and Mäder, P. (2007). Soil Organic Matter and Biological Soil Quality Indicators after 21 Years of Organic and Conventional Farming. Agric. Ecosyst. Environ. 118, 273–284. doi:10.1016/j.agee.2006.05.022
Giller, K. E., Hijbeek, R., Andersson, J. A., and Sumberg, J. (2021). Regenerative Agriculture: An Agronomic Perspective. Outlook Agric. 50, 13–25. doi:10.1177/0030727021998063
Govaerts, B., Verhulst, N., Castellanos-Navarrete, A., Sayre, K. D., Dixon, J., and Dendooven, L. (2009). Conservation Agriculture and Soil Carbon Sequestration: Between Myth and Farmer Reality. Crit. Rev. Plant Sci. 28, 97–122. doi:10.1080/07352680902776358
Haddaway, N. R., Hedlund, K., Jackson, L. E., Kätterer, T., Lugato, E., Thomsen, I. K., et al. (2017). How Does Tillage Intensity Affect Soil Organic Carbon? A Systematic Review. Environ. Evid. 6, 1–48. doi:10.1186/s13750-017-0108-9
Hobbs, P. R., Sayre, K., and Gupta, R. (2008). The Role of Conservation Agriculture in Sustainable Agriculture. Phil. Trans. R. Soc. B 363, 543–555. doi:10.1098/rstb.2007.2169
IPCC (2006). 2006 IPCC Guidelines for National Greenhouse Gas Inventories. Prepared by the National Greenhouse Gas Inventories Programme. Editor H. S. Eggleston, L. Buendia, K. Miwa, T. Ngara, and K. Tanabe (Japan: IGES).
IUSS Working Group WRB (2014). International Soil Classification System for Naming Soils and Creating Legends for Soil Maps. Update 2015 World Soil Resour. Rep. No. 106, 192.
Jennings, D. S., Gardner, W., and Gardner, W. (1922). A New Method of Mechanical Analysis of Soils. Soil Sci. 14, 485–499. doi:10.1097/00010694-192212000-00011
Johannes, A., Matter, A., Schulin, R., Weisskopf, P., Baveye, P., and Boivin, P. (2017). Optimal Organic Carbon Values for Soil Structure Quality of Arable Soils. Does clay Content Matter? Geoderma 302, 21. doi:10.1016/j.geoderma.2017.04.021
Keel, S. G., Anken, T., Büchi, L., Chervet, A., Fliessbach, A., Flisch, R., et al. (2019). Loss of Soil Organic Carbon in Swiss Long-Term Agricultural Experiments over a Wide Range of Management Practices. Agric. Ecosyst. Environ. 286, 106654. doi:10.1016/j.agee.2019.106654
King, A. E., Ali, G. A., Gillespie, A. W., and Wagner-Riddle, C. (2020). Soil Organic Matter as Catalyst of Crop Resource Capture. Front. Environ. Sci. 8, 50. doi:10.3389/fenvs.2020.00050
Kucharik, C. J., Brye, K. R., Norman, J. M., Foley, J. A., Gower, S. T., and Bundy, L. G. (2001). Measurements and Modeling of Carbon and Nitrogen Cycling in Agroecosystems of Southern Wisconsin: Potential for SOC Sequestration during the Next 50 Years. Ecosystems 4, 237–258. doi:10.1007/s10021-001-0007-2
Lal, R. (2004). Soil Carbon Sequestration Impacts on Global Climate Change and Food Security. Science 304, 1623–1627. doi:10.1126/science.1097396
Levavasseur, F., Mary, B., Christensen, B. T., Duparque, A., Ferchaud, F., Kätterer, T., et al. (2020). The Simple AMG Model Accurately Simulates Organic Carbon Storage in Soils after Repeated Application of Exogenous Organic Matter. Nutr. Cycl Agroecosyst 117, 215–229. doi:10.1007/s10705-020-10065-x
Maltas, A., Charles, R., Jeangros, B., and Sinaj, S. (2013). Effect of Organic Fertilizers and Reduced-Tillage on Soil Properties, Crop Nitrogen Response and Crop Yield: Results of a 12-year experiment in Changins, Switzerland. Soil Tillage Res. 126, 11–18. doi:10.1016/j.still.2012.07.012
McLauchlan, K. (2006). The Nature and Longevity of Agricultural Impacts on Soil Carbon and Nutrients: A Review. Ecosystems 9, 1364–1382. doi:10.1007/s10021-005-0135-1
Merante, P., Dibari, C., Ferrise, R., Sánchez, B., Iglesias, A., Lesschen, J. P., et al. (2017). Adopting Soil Organic Carbon Management Practices in Soils of Varying Quality: Implications and Perspectives in Europe. Soil Tillage Res. 165, 95–106. doi:10.1016/j.still.2016.08.001
Minasny, B., Arrouays, D., McBratney, A. B., Angers, D. A., Chambers, A., Chaplot, V., et al. (2018). Rejoinder to Comments on Minasny et al., 2017 Soil carbon 4 per mille Geoderma 292, 59-86. Geoderma 309, 124–129. doi:10.1016/j.geoderma.2017.05.026
Minasny, B., Malone, B. P., McBratney, A. B., Angers, D. A., Arrouays, D., Chambers, A., et al. (2017). Soil Carbon 4 Per Mille. Geoderma 292, 59–86. doi:10.1016/j.geoderma.2017.01.002
Nelson, D. W., and Sommers, L. (1983). Total Carbon, Organic Carbon, and Organic Matter. Methods Soil Analysis. Part. 2. Chem. Microbiol. properties 9, 539–579. doi:10.2134/agronmonogr9.2.2ed.c29
O'Connell, S., Grossman, J. M., Hoyt, G. D., Shi, W., Bowen, S., Marticorena, D. C., et al. (2015). A Survey of Cover Crop Practices and Perceptions of Sustainable Farmers in North Carolina and the Surrounding Region. Renew. Agric. Food Syst. 30, 550–562. doi:10.1017/S1742170514000398
Olson, K. R., Al-Kaisi, M. M., Lal, R., and Lowery, B. (2014). Experimental Consideration, Treatments, and Methods in Determining Soil Organic Carbon Sequestration Rates. Soil Sci. Soc. America J. 78, 348–360. doi:10.2136/sssaj2013.09.0412
Paustian, K., Larson, E., Kent, J., Marx, E., and Swan, A. (2019). Soil C Sequestration as a Biological Negative Emission Strategy. Front. Clim. 1. doi:10.3389/fclim.2019.00008
Peel, M. C., Finlayson, B. L., and McMahon, T. A. (2007). Updated World Map of the Köppen-Geiger Climate Classification. Hydrol. Earth Syst. Sci. 11, 1633–1644. doi:10.5194/hess-11-1633-2007
Poeplau, C., and Don, A. (2015). Carbon Sequestration in Agricultural Soils via Cultivation of Cover Crops - A Meta-Analysis. Agric. Ecosyst. Environ. 200, 33–41. doi:10.1016/j.agee.2014.10.024
Power, A. G. (2010). Ecosystem Services and Agriculture: Tradeoffs and Synergies. Phil. Trans. R. Soc. B 365, 2959–2971. doi:10.1098/rstb.2010.0143
Powlson, D. S., Stirling, C. M., Jat, M. L., Gerard, B. G., Palm, C. A., Sanchez, P. A., et al. (2014). Limited Potential of No-Till Agriculture for Climate Change Mitigation. Nat. Clim Change 4, 678–683. doi:10.1038/nclimate2292
Powlson, D. S., Whitmore, A. P., and Goulding, K. W. T. (2011). Soil Carbon Sequestration to Mitigate Climate Change: a Critical Re-examination to Identify the True and the False. Eur. J. Soil Sci. 62, 42–55. doi:10.1111/j.1365-2389.2010.01342.x
Prout, J. M., Shepherd, K. D., McGrath, S. P., Kirk, G. J. D., and Haefele, S. M. (2020). What Is a Good Level of Soil Organic Matter? an index Based on Organic Carbon to clay Ratio. Eur. J. Soil Sci. 72, 2493–2503. doi:10.1111/ejss.13012
Ranaldo, M., Carlesi, S., Costanzo, A., and Bàrberi, P. (2020). Functional Diversity of Cover Crop Mixtures Enhances Biomass Yield and weed Suppression in a Mediterranean Agroecosystem. Weed Res. 60, 96–108. doi:10.1111/wre.12388
Reicosky, D. C. (2003). “Tillage-Induced CO2 Emissions and Carbon Sequestration: Effect of Secondary Tillage and Compaction,” in Conservation Agriculture: Environment, Farmers Experiences, Innovations, Socio-Economy, Policy. Editors L. García-Torres, J. Benites, A. Martínez-Vilela, and A. Holgado-Cabrera (Dordrecht: Springer Netherlands), 291–300. doi:10.1007/978-94-017-1143-2_35
Robinson, G. W. (1922). A New Method for the Mechanical Analysis of Soils and Other Dispersions. J. Agric. Sci. 12, 306–321. doi:10.1017/s0021859600005360
Ruis, S. J., and Blanco‐Canqui, H. (2017). Cover Crops Could Offset Crop Residue Removal Effects on Soil Carbon and Other Properties: A Review. Agron.j. 109, 1785–1805. doi:10.2134/agronj2016.12.0735
Rumpel, C., Amiraslani, F., Chenu, C., Garcia Cardenas, M., Kaonga, M., Koutika, L.-S., et al. (2020). The 4p1000 Initiative: Opportunities, Limitations and Challenges for Implementing Soil Organic Carbon Sequestration as a Sustainable Development Strategy. Ambio 49, 350–360. doi:10.1007/s13280-019-01165-2
Sanderman, J., Hengl, T., and Fiske, G. J. (2017). Soil Carbon Debt of 12,000 Years of Human Land Use. Proc. Natl. Acad. Sci. U.S.A. 114, 9575–9580. doi:10.1073/pnas.1706103114
Senapati, N., Chabbi, A., Gastal, F., Smith, P., Mascher, N., Loubet, B., et al. (2014). Net Carbon Storage Measured in a Mowed and Grazed Temperate Sown Grassland Shows Potential for Carbon Sequestration under Grazed System. Carbon Manage. 5, 131–144. doi:10.1080/17583004.2014.912863
Smith, W. N., Grant, B. B., Campbell, C. A., McConkey, B. G., Desjardins, R. L., Kröbel, R., et al. (2012). Crop Residue Removal Effects on Soil Carbon: Measured and Inter-model Comparisons. Agric. Ecosyst. Environ. 161, 27–38. doi:10.1016/j.agee.2012.07.024
Swinton, S. M., Lupi, F., Robertson, G. P., and Hamilton, S. K. (2007). Ecosystem Services and Agriculture: Cultivating Agricultural Ecosystems for Diverse Benefits. Elsevier.
USDA (2003). Publication : USDA ARS. Available at: https://www.ars.usda.gov/research/publications/publication/?seqNo115=158750 (Accessed November 21, 2021).
Wendling, M., Büchi, L., Amossé, C., Jeangros, B., Walter, A., and Charles, R. (2017). Specific Interactions Leading to Transgressive Overyielding in Cover Crop Mixtures. Agric. Ecosyst. Environ. 241, 88–99. doi:10.1016/j.agee.2017.03.003
Wendling, M., Charles, R., Herrera, J., Amossé, C., Jeangros, B., Walter, A., et al. (2019). Effect of Species Identity and Diversity on Biomass Production and its Stability in Cover Crop Mixtures. Agric. Ecosyst. Environ. 281, 81–91. doi:10.1016/j.agee.2019.04.032
West, T. O., and Post, W. M. (2002). Soil Organic Carbon Sequestration Rates by Tillage and Crop Rotation. Soil Sci. Soc. Am. J. 66, 1930–1946. doi:10.2136/sssaj2002.1930
White, R. E., Davidson, B., Lam, S. K., and Chen, D. (2018). A critique of the paper 'Soil carbon 4 per mille' by Minasny et al. (2017). Geoderma 309, 115–117. doi:10.1016/j.geoderma.2017.05.025
Wold, S., Esbensen, K., and Geladi, P. (1987). Principal Component Analysis. Chemometrics Intell. Lab. Syst. 2, 37–52. doi:10.1016/0169-7439(87)80084-9
Keywords: soil organic carbon, soil organic matter, carbon content change, tillage, cover-crop, organic amendments, meadow, on-farm results
Citation: Dupla X, Lemaître T, Grand S, Gondret K, Charles R, Verrecchia E and Boivin P (2022) On-Farm Relationships Between Agricultural Practices and Annual Changes in Organic Carbon Content at a Regional Scale. Front. Environ. Sci. 10:834055. doi: 10.3389/fenvs.2022.834055
Received: 12 December 2021; Accepted: 11 March 2022;
Published: 24 March 2022.
Edited by:
Jörg Luster, Swiss Federal Institute for Forest, SwitzerlandReviewed by:
Marijn Van De Broek, ETH Zürich, SwitzerlandMirjam Helfrich, Thünen Institut of Climate-Smart Agriculture, Germany
Copyright © 2022 Dupla, Lemaître, Grand, Gondret, Charles, Verrecchia and Boivin. This is an open-access article distributed under the terms of the Creative Commons Attribution License (CC BY). The use, distribution or reproduction in other forums is permitted, provided the original author(s) and the copyright owner(s) are credited and that the original publication in this journal is cited, in accordance with accepted academic practice. No use, distribution or reproduction is permitted which does not comply with these terms.
*Correspondence: Xavier Dupla, eGF2aWVyLmR1cGxhQHVuaWwuY2g=