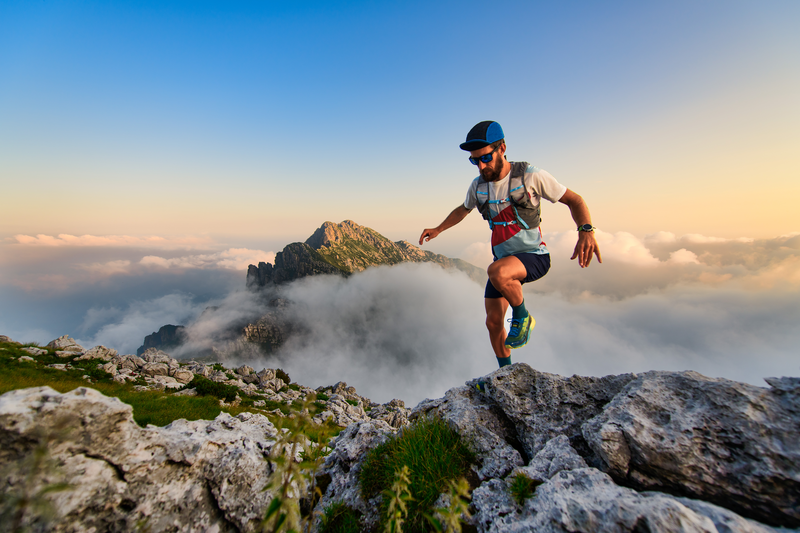
95% of researchers rate our articles as excellent or good
Learn more about the work of our research integrity team to safeguard the quality of each article we publish.
Find out more
ORIGINAL RESEARCH article
Front. Environ. Sci. , 01 June 2022
Sec. Toxicology, Pollution and the Environment
Volume 10 - 2022 | https://doi.org/10.3389/fenvs.2022.830589
This article is part of the Research Topic Environmental Impacts of Pesticides: Environmental Fate, Ecotoxicology, Risk Assessment, and Remediation View all 17 articles
Agriculture on sloping lands is prone to processes of overland flow and associated soil detachment, transportation, and deposition. The transport of pesticides to off-target areas related to runoff processes and soil erosion poses a threat of pollution to the downstream environment. This study aimed to quantify transport of pesticides both dissolved in water and in the particulate phase in transported sediments. Particulate phase transport of pesticides on short temporal time scales from agricultural fields is scarcely studied. During two growing seasons (2019 and 2020) rainfall—runoff events were monitored in a catchment of 38.5 ha. We selected 30 different pesticides and one metabolite based on interviews with the farmers on the application pattern. Concentrations for these 31 residues were analyzed in runoff water (dissolved phase–DP) and sediment (particulate phase–PP) and in soil samples taken in the agricultural fields. In all runoff events active substances (AS) were detected. There was a clear difference between DP and PP with 0–5 and 8–18 different AS detected in the events, respectively. Concentrations in PP were higher than in DP, with factors ranging from 12 to 3,700 times. DP transport mainly occurs in the first days after application (69% within 10 days), and PP transport occurs over the long term with 90% of transported mass within 100 days after application. Potato cultivation was the main source of runoff, erosion, and pesticide transport. Cereals and apples with grassed inter-rows both have a very low risk of pesticide transport during overland flow. We conclude that for arable farming on sloping lands overland transport of pesticide in the particulate phase is a substantial transport pathway, which can contribute to pollution over longer time periods compared to transport in water. This process needs to be considered in future assessments for pesticide fate and environmental risk.
The use of pesticides in agriculture is one of the key components in the rapid increase of food production in the past decades. In the coming decades, sustaining food security for the growing world population is one of the main global challenges (FAO, IFAD, UNICEF, WFP, WHO 2020). Aktar et al. (2009) stated that without pesticide use, the current quantity and quality of global food production cannot be sustained. However, the downside of the extensive use of pesticides is that it poses threats to human health and the environment (Bevan et al., 2017; Casado et al., 2019; Silva et al., 2019), including significant biodiversity loss (Geiger et al., 2010; Lanz et al., 2018). On average 3.14 kg ha−1 of pesticides was applied on cropland in the EU-28 in 2018 (FAO 2020). Beside reaching the target pest, these pesticides can be pollutants to other parts of on- and offsite ecosystems (Boivin and Poulsen 2017; Topping et al., 2020). Although many improvements in pesticide type, application efficiency and mitigation are made, still uncertainties and threats exist on off-target effects of pesticide use (Sattler et al., 2007; Lechenet et al., 2017), especially in cases of farming on sloping land.
Agriculture on sloping lands is prone to processes of overland flow and associated soil detachment, transportation, and deposition. For the EU the average soil loss on arable land, which covers 28% of the surface area, has been estimated at 2.46 t/ha per year (Panagos et al., 2015), but locally it can exceed 50 t/ha depending on rainfall erosivity, slope, erodibility of the soil, land use and land management. Soil erosion itself is a threat due to the loss of fertile topsoil which is needed for crop cultivation. However, the transport of pesticides to off-target areas related to runoff processes and soil erosion poses another threat of pollution to the downstream environment (Tang et al., 2012). Runoff and erosion events are often characterized by short high intensity peaks (Lefrancq et al., 2017) which can contain high concentrations of pesticides in both runoff water and transported sediment (Peruzzo, Porta, and Ronco 2008; Climent et al., 2019). Multiple studies investigating the pesticide pollution of headwaters and rivers show increased concentrations of pesticides in water after runoff events (Peruzzo et al., 2008; Lefrancq et al., 2017; Casado et al., 2019; Climent et al., 2019; Halbach et al., 2021), often exceeding the regulatory limits. In the current legal framework in the EU, the limits in water are 0.1 µg L−1 for single substances and 0.5 µg L−1 for combined mixtures (European directive 2013/39/EC). However, threshold values for soils and eroding sediments as well as sediment in surface water bodies are not set and are complex to derive due to the wide diversity in active substances, toxicity and possible degradation (Silva et al., 2019; Geissen et al., 2021).
In sloping areas, soil erosion occurs alongside runoff, and beside the dissolved phase (DP) the particulate phase (PP) is a transport pathway for pesticides. The contribution of particulate phase transport to total transport of pesticides, and the potential risk this could pose for off-site locations, is currently not well understood. Payraudeau and Gregoire (2012) concluded that dissolved and particulate transport of pesticides in rapid flow processes, mainly via overland flow, can function as a main transport pathway for pesticides to open water. Enrichment processes during rainfall events and erosion cause the transported sediment to contain higher amounts of pollutants than the surrounding soils (Ghadiri and Rose, 1991a) which increases off-site pollution risk (Ghadiri and Rose, 1991b). In a review of the transport characteristics of pesticides (Wauchope 1978), erosion related transport of pesticides was stated to be relevant only for strongly sorbing pesticides and main transport risk was by water. Less water-soluble pesticides with a higher sorption coefficient were introduced into the market to avoid leaching to groundwater and overland transport. Several widely used pesticides adsorb strongly to sediment and organic materials (Tang et al., 2012) and are thus classified as slightly mobile or non-mobile substances (McCall et al., 1980).
Synthesizing the results presented in multiple studies in the past decade, reveals that the contribution of pesticide transport in PP versus DP is unclear and various studies are contradicting. On the one hand, several studies on field scale conclude that PP transport is of minor importance (often <5% of the total load) (Maillard et al., 2011; Oliver et al., 2012; Todorovic et al., 2014; Napoli et al., 2016). However, other plot scale and flume experiments show potentially high transport of pesticides in PP (Yang, et al., 2015; Melland et al., 2016; Bento et al., 2018). Yang et al. (2015a) observed a natural rainfall event 2 days after pesticide application and detected DP concentrations of 0.7–1.3 µg L−1 and PP content of 40–60 µg L−1. The contribution of PP to total observed pesticide transport was 71%. At larger scales, concentrations of pesticides in total suspended solids (TSS) in rivers, and in river or stream sediments are often much higher than in water (Masiá et al., 2013; Cruzeiro et al., 2016; Climent et al., 2019), which is related to significant transport in the particulate phase.
Within the mentioned studies there is variation in design how the process of overland transport of pesticides is studied. On small plot scale, experiments are usually done within several days after pesticide applications. These experiments are on arable soils comparing tillage (Todorovic et al., 2014) or residue cover effects on transport (Melland et al., 2016; Bento et al., 2018). Field scale studies include vineyard or fruit tree orchards (Oliver et al., 2012; Maillard and Imfeld 2014; Napoli et al., 2016), but no results for arable land on field scale are known to the authors. Lastly several studies investigated pesticide transport dissolved in water and in TSS of river systems throughout the growing season (Masiá et al., 2013; Cruzeiro et al., 2016; Climent et al., 2019). However, runoff, erosion and related pesticide transport processes occur on short temporal scale and evaluation on annual basis is not sufficient to understand the exact scope of this transport (Tang et al., 2012; Lefrancq et al., 2017). On the other hand, the spatial scale of erosion processes demands to be analyzed on field and small catchment scale (Cerdan et al., 2004). Plot experiments can indicate effects but cannot include larger scale connectivity, heterogeneity and topography effects (Boardman and Evans 2020). To gain a further understanding of PP and DP transport of pesticides, field observations that include different land use types are needed.
In this study multiple season field scale observations were done to quantify the contribution of PP transport of pesticides in a small agricultural catchment. Observations were done for multiple land uses and active substances (AS). We hypothesize that PP pesticide transport will contribute substantially to total overland transport of pesticides from small agricultural catchments in sloping areas during erosive rainstorm events. To verify this hypothesis, this study quantified the contribution of particulate phase transport to the total off-site transport of pesticides during and after rainfall events. For this purpose, a measurement campaign was set up in which we sampled rainfall-runoff events at high temporal resolution at the catchment outlet and performed multiple field sampling surveys within the catchment during 2019 and 2020.
Sampling and field observations were done in a small agricultural catchment (38.6 ha) in the hilly area of South-Limburg, Netherlands (Figure 1). The catchment has a typical land use pattern for the loess belt covering parts of Belgium and the south of Limburg in the Netherlands. Arable agriculture was the main land use covering 25.2 ha, consisting of a crop rotation of winter cereals and intensive crops like potatoes, sugar beet and chicory. An apple orchard covered 5.3 and 5.0 ha was extensively managed grassland. The remaining area consisted of roads, urban area, and a small tree nursery. All agricultural fields were under conventional crop management, including pesticide applications, with apple and potato cultivation having the highest annual application rates.
FIGURE 1. Map of the study area in South-Limburg, the Netherlands indicating sampling points. The letters (A,B and C) indicate the three fields of main interest in this study.
The catchment has an elevation difference of 30 m (80–110 m. a.s.l.) and an average slope of 6.3% (sd ± 4.0%, range 0.1–30%). The catchment borders were calculated with QGIS 3.16 (QGIS 2021), based on the AHN3 DTM with a 0.5 m resolution (AHN 2019). The borders were adjusted if field observations showed different flow lines. The soil type is loess soil, a homogenous, fertile, but erosion sensitive Luvisol with a silt loam texture. Soil characteristics are (mean ± SE): sand 45.6 ± 2.2%, silt 51.0 ± 2.1%, clay 3.5 ± 0.2%, OM 3.6 ± 0.3%, pH 6.5 ± 0.10. The catchment is located in a temperate climate (Cfb) with a mean annual temperature of 10.7°C (±0.7°C) (KNMI 2021b) and mean annual precipitation of 757 mm (±108 mm) (KNMI 2021a), based on measurements from 1991–2020 at a weather station 2.5 km from the catchment. A dry ditch connects the fields to the catchment outlet, in which only for short periods after intensive rainfall events discharge occurs.
To relate measured pesticide concentrations at the outlet to application and management on the fields, samples were collected throughout the catchment after five runoff events (+ in Figure 1). A baseline soil survey (04 March 2020), consisting of 30 samples covering the whole catchment through a structured random design, was done before the growing season of 2020 (▲ in Figure 1). A total of 68 samples from the top 1 cm of the soil was collected. These samples were stored in a freezer and used for analysis of pesticides, texture, organic matter content, and pH. The concentrations of different pesticides in the upper layer of the fields indicates potential transport risk by runoff and erosion. In addition, pesticide application data (date, pesticide type, application concentration) were obtained from the landowners of the three main agricultural fields in the catchment (A, B and C in Figure 1).
Other data on climate, land use and soil conditions were monitored to investigate the dynamics and processes driving the pesticide transport during the rainfall events. Data was collected for rainfall, crop type, crop cover and soil moisture content of fields. Rainfall was measured at the outlet with a 0.2 mm tipping bucket (ARG100, Campbell Sci.) on a 1 min resolution. Crop height and cover was measured during surveys, soil moisture content was measured using a Time Domain Reflectometer (TDR) during surveys after events. Finally, radar precipitation data (5 min, 1 km2) from KNMI was used (KNMI 2021c) to obtain a spatial coverage of rainfall in the catchment and to fill gaps in the timeseries of the tipping bucket.
At the outlet of the catchment the water and sediment discharge was measured by a two feet Parshall flume, with an accurate measurement range between 12 and 650 L s−1 (See Supplementary Figure S1). Data was collected during runoff events and independent of pesticide application pattern. When free flow conditions in the channel were met, the accuracy of the Parshall flume is ±3% (Parshall 1926; Bos 1989). Water level readings were collected every minute at two locations in the flume. Automated sampling at the outlet, with an ISCO 3700 (Teledyne Isco, Inc. United States), was activated when the discharge would exceed 12 L s−1. Then, a runoff sample was pumped from the stream every 6 min. This was done using an inlet device, optimized for sampling of sediment and water samples, adapted from Gettel et al. (2011) and Eads and Thomas (1983). All details on the flume measurement set-up are given in Supplementary Material S1.1. A maximum of 12 samples could be taken by the sampler, resulting in 72 min of sampled event duration, which covered 80% of the runoff peaks occurring in this catchment in 2019 and 2020.
During the measurement years 2019 and 2020, based on the discharge data from the flume, 39 runoff events occurred. Due to temporarily malfunctioning of the equipment or because of insufficient sample coverage during some events, the analysis in this study is based on 14 events (see Supplementary Material S1.2 for further details). The analyzed events do not show significant (p < 0.05) differences with the not analyzed events, when comparing runoff and erosion characteristics. However, due to limitations in event duration for sample collection and minimal erosion for pesticide analysis, the analyzed subset shows a tendency for shorter events with higher runoff and erosion rates (see Supplementary Figure S2).
Each sample during a runoff event consisted of two bottles with identical content. One was used for analysis of pesticides in sediment and water, the other was used to derive total suspended solids (TSS) in water and other parameters of interest (OM, pH, and texture).
The soil and sediment analysis was done at the Soil Hydro Physics laboratory at Wageningen University. For TSS determination the samples were weighed and dried at 105°C for 24 h. For runoff TSS samples and soil samples in the catchment, dried sediment was collected and used to determine pH (suspension in 0.01 M CaCl), OM (loss on ignition) (Roper et al., 2019) and texture. For the determination of texture, 1 g of dried sediment was sieved on 500 µm, and analyzed with laser diffraction (Mastersizer S, Malvern Panalytical) in triplicate on wet samples by the department of Earth and Environmental Sciences, KU Leuven, Belgium.
To investigate pesticide concentrations for both components of the runoff (water and TSS), the samples were separated using a centrifuge (30 min at 3,600 rpm). The water part was transferred to a new tube and both samples were stored in a freezer until further analysis. During this stage samples were combined when the sediment content was too low for individual analysis; sediments from the 6 min timesteps were combined until a minimum of 7 g dry material was obtained for analysis.
The selection of pesticides for analysis was based on the application of pesticides in the growing season of 2019. The applications by the two landowners managing the 3 largest fields (A, B and C in Figure 1) of the area, where used as initial pesticide list. A selection of these compounds was analyzed, based on analysis possibilities within the limited mass of TSS samples (often <10 gr) and financial budget. Compounds were selected that could be analyzed with a multi-residue method (LC- MS/MS positive). Prior studies (Bento et al., 2018; Yang et al., 2015a, 2015b) indicated particulate phase (PP) transport for glyphosate and AMPA to be important, so these compounds were also selected for this research, and a single residue method was used for determination. These two analyses covered 31 compounds: 20 fungicides, five herbicides and five insecticides, and amino-methyl phosphonic acid (AMPA) as metabolite of glyphosate. The chemical characteristics of the selected AS range for adsorption (koc) from 1.6–4.2 106 ml g−1 and for degradation (DT50) from 0.8–419 days, according to data retrieved from the Public Pesticide Database (PPDB) (Lewis et al., 2016).
The list of chemicals and reagents used for the analysis is identical to Silva et al. (2019). The glyphosate and AMPA analysis was done several weeks earlier than the multi-residue analysis. To minimize sample disturbances, aliquots for both analyses were prepared at the same moment and stored in a freezer until further analysis. Because TSS sediment samples did not always provide enough material for the standard analysis, adapted masses (1/4 or 1/2 of the standard weight) were used if needed. Analysis for glyphosate and AMPA was done following the procedure described by Bento et al., 2016 and Bento et al., 2018. The multi residue analysis was done according the QuEChERS approach for soil and sediment samples (Anastassiades et al., 2003; Mol et al., 2008).
The quality control and chemical determination of the samples was done according to the guidance document for pesticide residues in food and feed (European Commission 2019). Calibration standards were prepared and injected at the start and end of each sampling sequence. The standards consisted of 1.25, 3.125, 6.25, 12.5 and 50 ng ml−1; 0.625 and 25 ng ml−1 were added during analyses of diluted samples. The glyphosate/AMPA standards were 5, 10, 20, 50, 100, 200, 500, 1,000 and ng ml−1. See Supplementary Material S2.1 for further details.
Since all analyzed soil and sediment samples contained soil moisture, and in varying amounts, the measured concentrations had to be adjusted for the volumetric water content (VWC). This was done by drying the sample material after the extraction to derive the dry weight of the soil or sediment. The concentration was corrected for VWC and the concentration of the compound in the relating water sample. All final concentrations were adjusted for the recovery of the compound. The recovery was detected based on 9 quality control (QC) samples, with a known concentration for each compound.
All data analysis, calculations and statistics were done using R software (R Core Team 2021), including the “drc” package for first order decay analysis (Ritz et al., 2015). To compare rainfall events with each other the event index (EVI) was calculated (Baartman et al., 2013).
The discharge was calculated based on the water height measured in the Parshall flume. Discharge was calculated based on the empirical formulas given by Parshall (1926). When submergence exceeded 50%, free flow conditions were not met and the discharge was corrected (Parshall 1926), for more details see Supplementary Material S1.1.
The calculations of the total pesticide loads in particulate (PP) and dissolved phase (DP) were based on variables that each introduce uncertainty towards the final quantification. Propagation of uncertainty was calculated with the standard deviation expressed as relative error for each variable (Taylor and Thompson 1998). The uncertainty for each variable is presented in more detail in Supplementary Material S3. While uncertainty of primary measurements (e.g., rainfall or water discharge) is still low, the propagation of the error during calculations results in uncertainty of 18% for pesticide loads in TSS.
Synthesizing the results obtained in this study, we found that transport of pesticides during erosive runoff events is determined by three main aspects. Both the quantity as well as the main mode of transport are influenced by 1) the precipitation events and related runoff, 2) the chemical characteristics of the transported active substances as well as by 3) the land use and managements on the fields. The findings and dynamics of each of these processes in relation to pesticide transport are discussed in detail below.
The 14 events included in this study cover a wide range in terms of rainfall intensity, total precipitation and related runoff and erosion (Figure 2). Two events (28 May 2019 and 16 August 2020) had high erosion rates, with mean total suspended solids (TSS) concentrations of 44 and 21 g L−1, respectively, while the mean TSS concentration for all events was 7.5 g L−1. The total water discharged from the catchment during the selected events was 5,800 (±290) m3 and the total soil loss was 79 (±5) ton dry soil, resulting in a ratio Qsed: Qwat of 1–73. This sediment loss equals 3.1 t ha−1 for the arable fields in the catchment. Although not all events that occurred in these two seasons (39) are included, the observed erosion rates are in line with the estimated average of 2.46 t ha−1 y−1 for arable lands in Europe (Panagos et al., 2015).
FIGURE 2. Overview of rainfall (A), runoff (B), related erosion (C) and total pesticides transported (g) by particulate (PP) and dissolved phase (DP) (D) for the 14 events measured in 2019 and 2020. Error bars indicate uncertainty of total value (±18% PP and ±17% DP, see Section 2.5).
There is no significant relation between rainfall characteristics and runoff or erosion. Linear regression was applied on the total discharge of water and sediment, with total rainfall and event duration as explanatory variables. This results in an r2 of 0.68 for total amount of runoff. No direct relationship between the event characteristics and the amount of erosion could be found. This can be related to other variables like crop cover and soil moisture which varied between events and influence erosion rates and runoff (Morgan et al., 1984; Zambon et al., 2021). Because of the event-based nature of overland flow, longer term monitoring is needed to understand the variability between rainfall events, cultivated crops and seasons within the year (Borrelli et al., 2021). The events included in this study, show the different mechanisms that affect transport and partitioning between phases of AS during overland flow. However, the high variability does not allow for generalizations, because adding or subtracting several events can influence general findings to a large extent.
The Glyphosate and AMPA results for the three events from 23-09-2020 are excluded from further analysis, due to occurrence of point source pollution. The glyphosate concentration on 23-09-2020, which is a small event in terms of runoff and erosion, is 550 mg kg−1 in PP and 2.2 mg L−1 in DP. These values are a factor 20–50 higher than all other detected levels in the discharge as well as on the fields in the catchment. Point source pollution which reaches the outlet at the first rainfall event can explain these extreme peaks (Reichenberger et al., 2007; Syafrudin et al., 2021). Because this study aims to investigate diffuse source transport, these results are not taken into account. A detailed description and further discussion is given in the Supplementary Material S2.2.
Within the analyzed events, six events occurred with substantial transport (>5 g) of pesticides in DP and PP combined, in the other events the transported load was small (see Figure 2D). The pesticide concentrations (PP and PD combined) during the 14 events ranged from 0.2 (±0.04) to 160 (±30) µg L−1. The contribution of PP transport to total overland transport shows high variability between events, ranging from 0.1% to 99%. When comparing the transport for each event with the characteristics of rainfall, runoff and erosion, the concentrations of pesticides were not related to the intensity of rainfall or runoff. Both the quantity of runoff as well as the concentrations of active substances transported could result in high total mass transported from the catchment. The event of 16-08-2020 had a high discharge of water and sediment (Figures 2B,C), but the concentrations of AS were not high (Figure 3). Opposingly, the event of 17-06-2020 was relatively small in terms of runoff, but had high concentrations, resulting in substantial amounts of transported pesticides.
FIGURE 3. Concentrations of active substances (AS) detected in runoff during each event in the particulate (PP) and dissolved (DP) phase. Superscript in legend denotes: F Fungicides, H Herbicides, and I Insecticides. All AS in a specific event with a content in the PP below 0.1 mg kg−1 are grouped for visualization. * For the events after 23-09-2020, Glyphosate and AMPA are excluded due to point source pollution.
The high concentrations of pesticides detected in the particulate phase (PP) of runoff, caused increased transport of pesticides in PP when total erosion during a runoff event increased (r2 = 0.60). The two events with TSS above 10 g L−1 had most transport in PP. In events with TSS concentrations between 3 and 10 g L−1 the dominant transport mode can vary, and with lower concentrations the PP share drops towards zero. The partitioning between DP and PP in terms of concentration varied through the events. In all events the PP concentration was higher than DP, with factors ranging from 12 to 3,700 for the 9 events in which DP was detected, for six events no DP concentration was measured. Other studies taking both PP and DP into account report factors ranging from 10–1,000 times higher PP concentrations compared to DP (Todorovic et al., 2014; Maillard and Imfeld 2014; Cruzeiro et al., 2016; Yang et al., 2015b).
The mean concentrations were always cocktails of multiple active substances (AS). Figure 3 shows the composition of AS for each event in both phases of the runoff. The number of detected AS in PP ranged from 8–18, compared to 0–5 different AS in the DP. During the whole observation period 8 different AS were measured in DP against 24 (including AMPA) in the PP. However the LOQ for water samples in this study was 2.5 µg L−1, which corresponds with analysis in other studies (Peruzzo et al., 2008; Melland et al., 2016). This limits the detection of lower concentrations of AS in water.
The difference in measured concentrations, and related evaluation of environmental risks, between short term local events and larger temporal scales was emphasized by Lefrancq et al. (2017). During peaks in runoff, pesticide concentrations up to 387 µg L−1 were detected in runoff water by Lefrancq et al. (2017); in the present study the maximal concentration during an event was 128 µg L−1. Concentrations, in both PP and DP, increased a lot during the main application period when rainfall events occurred shortly after pesticide applications (see Figure 3). In 9 of the 14 events observed during our study the single substance as well as combined concentrations exceeded the regulatory limits, 0.1 and 0.5 µg L−1, respectively, set by the EU (European directive 2013/39/EC). When the pesticides enter the headwater streams they are also easily transported over longer distances, and pesticides are detected in many smaller streams throughout Europe (Casado et al., 2019).
Increased PP transport related to higher erosion rates corresponds with other studies investigating the PP and DP transport of pesticides. Melland et al. (2016), found that the PP could be significant (up to 47% of total load) with TSS concentrations ranging from 1.4–10.3 g L−1. Yang et al. (2015a) also reports high (70–80%) PP transport in a study with erosion rates of 7–10 tons ha−1. Several studies that conclude PP transport is not substantial (Maillard et al., 2011; Oliver et al., 2012; Napoli et al., 2016) all observed low TSS concentrations. The erosion rates in the region of the catchment of this study were assessed to be between 1–2 t ha−1 y−1 (Panagos et al., 2015), while observed erosion rates during the seasons 2019 and 2020 were slightly higher in this study. However the estimated mean of 2.46 t ha−1 y−1 for erosion prone lands in Europe (Panagos et al., 2015), stresses the need to take particulate phase transport of pesticides into account in further assessments for pesticide fate and environmental risk.
Whether pesticides are transported to off-site areas also depends on the behavior of the specific active substance after application on the field. Biodegradability (DT50), adsorption (koc) to soil particles and solubility in water (Sw) are the main characteristics that prescribe this behavior (Leonard 1990). During the observations in this study, dissolved phase (DP) transport occurred mainly shortly after the applications of AS to the field: 62% of total DP transport occurred within 5 days after field application, 69% within 10 days and 98% within 50 days. In contrast, PP transport occurred over a much longer period, with 9% of the total transported mass within 5 days, 10% within 10 days, 31% in 50 days and 90% within 100 days after application. All AS which were transported in high loads in DP shortly after applications, were transported for longer periods in PP afterwards. The field plot studies on differences in DP and PP transport from arable fields (Todorovic et al., 2014; Melland et al., 2016), have analyzed transport rates for events occurring shortly (<3 days) after pesticide application, concluding that DP transport is dominant. The data from the current study also shows that shortly after application DP transport occurs in substantial amounts. However, for rainfall events longer after application, pesticides are still detected in PP and can be transported during events with runoff and erosion.
Table 1 compares the amount of applied AS for each chemical characteristic with the amount of transported AS. Statistical analysis, including regression, correlation and multivariate analysis did not show a relation between koc or Sw of AS and transport in either DP or PP. The transport in “high” as well as “low” solubility classes occurs more in PP than in DP, and no relation with transport can be found in this study. However, the mass transported for each class of adsorption does show a trend. The ratio of transport in DP to PP shifts from mainly DP for “mobile” AS to mainly PP for AS classified as “mobile.” This result corresponds with the expected behavior of pesticides, in relation to this adsorption coefficient (Wauchope et al., 2002; Leonard 1990). However, not all individual AS follow this pattern. The AS which were detected in DP have koc values ranging from 48 up to 1.6 104 ml g−1, according to the PPDB, which covers the classes “mobile” up to “non-mobile.” Moreover, nine AS were detected in PP but not in DP, which were applied within 10 days before an occurring rainfall event. Koc values for these AS range from 1.6 up to 1.6 104 ml g−1 (PPDB, Lewis et al., 2016). Based on the chemical classification at least Flonicamid (very mobile) and Cymoxanil (mobile) would be expected to be detected in DP.
These findings correspond with analysis in other studies, where some AS were found to follow the theoretical classification well, but other AS were transported more in an unexpected phase. Oliver et al. (2012) reported that the AS with highest solubility was transported proportionally less in DP compared to AS with lower solubility properties. In a field experiment with runoff 2 days after the application of pesticides, the AS with higher adsorption to soil particles were also transported most in PP (Melland et al., 2016). On larger scales, also no significant correlation was found between adsorption characteristics of AS and partitioning between DP and PP (Climent et al., 2019), although more different AS and in higher frequency where detected in PP (Cruzeiro et al., 2016). These results from other studies as well as the observations in the present study indicate that solubility and adsorption characteristics may not suffice to predict the dominant transport mode and related environmental fate of pesticides.
Mandipropamid, Glyphosate, Metobromuron and Prosulfocarb were the main transported AS in PP. Prosulfocarb has a high koc and low solubility, and it is only detected in PP. Glyphosate is detected in both phases although the majority of transport occurred in PP (97%). The other two AS have more affinity for dissolved transport and were also transported in DP, mandipropamid for 41% and metobromuron for 63% of total mass. During the growing seasons a total of 52 different AS were applied. Due to limitations in the analysis, several AS that might be interesting for analysis, had to be excluded. This were mancozeb, diquat and captan among others, of which some were applied in high frequency or had chemical characteristics that suggested high potential for PP transport (Chiovarou and Siewicki 2008). Therefor the transported loads in this study cannot be used as a mass balance, because including or excluding several AS from the analysis can influence the quantities that are reported.
The persistence of an AS is of major influence for the transport by runoff. Of the 30 analyzed AS, seven where not detected in any runoff event, these are characterized by a fast degradation (DT50 < 32 days). Two of these AS were applied during potato cultivation and 5 on the apple orchard. Table 1 shows that persistence influences the risk of transport. Of total applied AS mass, 50% is non-persistent, and 50% is moderately persistent or persistent. However, in the runoff, these ratios change, and only 13% of transported AS belong to the “non-persistent” class, compared to 87% of transported mass belonging to the moderately-persistent and persistent class.
In Figure 4, a comparison of the decrease of transported concentration over time since application is shown for six AS that were detected on a regular basis. Three of these were transported in both phases. The data shows that after 60 days no transport in DP is detected, while transport in PP lasts much longer. In addition, the concentrations transported in DP drop faster over time; even for metribuzin where the fitted 1st order decay curves have nearly the same slope for DP and PP. After 150 days significant concentrations were still detected in PP. The fitted curves show a reasonably good fit for DP with residual standard errors (RSE) between 0.06 and 0.12. This confirms the overall observation that in the 14 events of this dataset, 69% of all DP transport occurs within 10 days after application on the field. This finding corresponds with other studies, which report DP transport mainly close to application (Boithias et al., 2014; Cruzeiro et al., 2016; Melland et al., 2016). PP concentrations have a higher RSE, meaning that the time between application and event alone cannot explain concentration or transported load in PP. Differences in reported degradation rates (e.g., PPDB) with observed degradation in the field can be caused by the high variability in environmental degradation of AS (Arias-Estévez et al., 2008; Bento et al., 2016).
FIGURE 4. Decrease of the relative concentrations in DP and PP for six AS. The concentration range (min—max) of the AS detected in runoff is given, ppb in PP is µg kg−1, and in DP µg L−1, rse is the residual standard error.
The three AS shown in Figure 4, that were transported in both phases, were all applied frequently, which increases the chance for a runoff event to occur close to the application date. Epoxiconazole and Bixafen both show low or no decrease in transported concentration over time: both compounds have slow biodegradation (98 and 203 days DT50, respectively) and originate from applications of the previous growing season. Bixafen was applied one time in 2019 and once in 2018 but was detected on stable levels around 50 µg kg−1 on arable fields in this study. The concentrations in which these AS were transported are low compared to, e.g., Prosulfocarb, however these AS were transported with every erosive runoff event and in this way formed a constant source of pollution. No studies were found that relate the transported load of different AS to the varying biodegradation rate in the environment. However studies on concentrations and detection of AS in rivers, clearly show increased levels and numbers of different AS during periods with intensive pesticide use, often spring and summer (Peruzzo et al., 2008; Cruzeiro et al., 2016; Climent et al., 2019).
The three chemical characteristics of interest; biodegradation, water solubility and adsorption coefficient alone cannot explain the transported concentrations or the partitioning between dissolved and particulate phase. The transported mass during an event, both in DP and PP, depends not only on concentration, but also on runoff and erosion quantity and related variables, which are strongly event specific. The transport dynamics on field scale are also influenced by runoff generation processes and sediment connectivity (Heckmann et al., 2018; Bracken and Jacky. 2007), which takes into account factors like upstream area, spatial land use distribution and field specific land management (see Section 3.3.), distance to the outlet (Borselli et al., 2008; Cavalli et al., 2013) and rainfall amounts and intensity (López-Vicente and Ben-Salem 2019).
Based on the application data for each field and the concentrations of AS during field surveys in the soil, the source field and associated land use type can be derived for the AS detected at the catchment outlet during the events (a full table with AS, detection, field concentration and application data is supplied in Supplementary Table S9). Land use and management of agricultural fields affect runoff, erosion, and thus the potential transport of AS to off-site locations. The number of detected AS in runoff is the highest in the main cropping season (June—August), which corresponds with the frequency of applications on the fields.
On the apple orchard the highest total amount of pesticides was applied, with a mean of 21 kg ha−1 active ingredients (Supplementary Table S8), and the frequency of applications throughout the growing season is high. This does however not result in high discharges of pesticides at the catchment outlet. Six AS were detected in the runoff, of which four were fungicides, one herbicide and one insecticide. Four of these (Fluxapyroxad, Pyraclostrobin, Chlorantraniliprole and Difenoconazole) where only applied on apples and were not transported in DP, only in PP and contributed 1% to total PP load. Besides that, Boscalid and Glyphosate were applied, which are also used in potato cultivation, the exact source field could not be derived based on this dataset. These compounds contribute 1% and 23% tot total DP and PP transport, respectively. The borders and interrow area of the orchard were covered with grass, which has a reducing effect on soil erosion and a filtering and buffering effect on the transport of pesticides (Muñoz-Carpena et al., 2018; Reichenberger et al., 2019; Yu et al., 2019). During field observation, no signs of erosion were recorded from the apples. These findings correspond with studies on orchards and vineyards with grassed rows, also reporting low AS transport (Oliver et al., 2012; Napoli et al., 2016).
None of the transported AS can be directly associated with the cereal cultivation. This corresponds with the low erosion risk associated with cereals in summer (Prasuhn 2020) and the low total amount of applied pesticides (0.4 kg ha−1, Supplementary Table S8). However, during cereal cultivation persistent AS were applied (Epoxiconazole, Bixafen and Isopyrazam), which were still detected in the soil in the next growing season, when a different crop was grown on the field (i.e., the next crop in the rotation). These three AS were detected in 100%, 100% and 93% of all runoff events, respectively. They were transported from the field in the rotation after the cereals, when potatoes were cultivated, which caused the runoff and erosion. The transport of these AS 1 year after application contributes for 4% of the PP transported mass from the catchment. To the authors no other studies are known presenting results of delayed transport of pesticides in overland transport, however persistent AS were shown to be a risk for leaching to groundwater (Reichenberger et al., 2007; Schuhmann et al., 2019).
The main source of pesticide transport in this study was the field cultivated with potatoes. 21 of all detected AS (including AMPA) where related to this cultivation, this also included persistent AS which were applied in previous cropping seasons (see previous section). At least 72% of PP and 99% of DP transport originates from the potato cultivation. As discussed above, Glyphosate and Boscalid can originate from apples as well as potato cultivation. Based on the observations of major runoff and erosion the potato field is likely to also be the main source area for these AS. Potato cultivation on sloping lands is a combination of erosion prone land use (Olivier et al., 2014) with high amount of pesticide applications (11 kg ha−1, Supplementary Table S8). During field observations potato cultivation was identified as the main source of runoff and erosion (see Supplementary Figures S3–S5), which corresponds with the detected AS in the runoff and TSS. These results show that erosion prone and pesticide intensive cultivations form the main risk for both DP and PP transport of pesticides during runoff events. In the currently available literature of PP transport of pesticide only plot scale simulations on arable fields are available, comparing either tillage practices or crop and mulch cover (Todorovic et al., 2014; Yang et al., 2015a; Melland et al., 2016; Bento et al., 2018). These studies show the effect of increased transport with erosion prone land management types. However, the smaller scale and often short time between application and runoff event of these studies, did not suffice to understand the extent of potential PP transport from arable fields with an erosion prone cultivation.
The large difference in observed transport between the potato cultivation and the cereals or apple orchard, emphasize that preventing erosion and runoff will also reduce the overland transport of pesticide. Implementation of on-site measure like vegetated filter strips (Muñoz-Carpena, Ritter, and Fox 2019) or micro-dams between the potato ridges (Olivier et al., 2014) can reduce runoff and erosion from the specific fields. Moreover off-site erosion measures like riparian buffers and wetlands are shown to mitigate further transport of pesticides in to the environment (Imfeld et al., 2021).
This study confirmed our hypothesis that particulate phase transport contributes to total overland transport of pesticides on field scale on sloping land. Based on observations in two growing seasons (2019 and 2020) in which 14 rainfall-runoff events were sampled we can conclude that in a small agricultural catchment with intensive arable farming, PP transport contributed substantially to total overland transport of pesticides.
Event specific contributions of PP show high variability, which is the result of interacting factors, including hydrological and sediment dynamics during the event, chemical characteristics of the transported active substances and land management on the fields. This results in high variability in quantity, phase and type of the transported pesticides. Land use types which are pesticide intensive and erosion prone, pose a threat for high discharges of pesticides via runoff, both in dissolved and particulate phase.
Transport in DP occurred mainly shortly after application of the pesticide (69% within 10 days). Opposingly, the transport of pesticides in PP occurs over much longer time spans, where 90% of the total transport is reached within 100 days after application in this study.
Biodegradability (DT50), adsorption to soil (koc) and solubility in water (Sw) of AS alone cannot explain the transport mode and related fate of individual pesticides after application. However, the biodegradability (DT50) of an active substance determines how long it will be available for overland transport either in PP or DP. Most non-persistent AS were not transported or only shortly after application, where several persistent AS were transported year-round during erosive runoff events.
Considering the extent of erosion from arable land, significantly more transport occurs than is predicted when PP transport during runoff events is not taken into account. Our results imply that event-scale dynamics are important for pesticide transport and that interactions between management, event characteristics and applied active substances can cause events that contribute disproportionally high to total transport. Because most transport of pesticides occurred from erosion prone land use, this study emphasizes the need for on-site and off-site erosion mitigation to prevent off-site transport of pesticides in the environment.
For further generalization and understanding of the findings in the study, additional observational data will be valuable. Transport behavior in different pedoclimatic and geomorphic environments as well as under different land management practices, will further increase our understanding of these dynamics. The current study is limited to three land use types, on erosion prone loess soils. Moreover the observation period of 2 years, results in a variety of rainfall events, but longer term observations are needed to be able to derive generalized conclusions. Other research methods, such as modelling studies that investigate the relevant variables and that can explicitly simulate the dynamics within the catchment, might add to our ability to assess the pollution risk of currently used pesticides.
The datasets presented in this article are available at https://doi.org/10.4121/19690684. The code for analysis is available at https://doi.org/10.4121/19690840 or https://github.com/mcommelin/pesticide_transport_runoff_erosion
MC, JB, MR, and VG designed the field research, MC did the data collection. Together with PZ the chemical analysis and interpretation was performed. MC wrote the draft manuscript and JB, PZ, MR, and VG edited and reviewed for the final version.
This research has received funding from the European Union’s Horizon 2020 research and innovation program under grant agreement No. 727984.
The authors declare that the research was conducted in the absence of any commercial or financial relationships that could be construed as a potential conflict of interest.
All claims expressed in this article are solely those of the authors and do not necessarily represent those of their affiliated organizations, or those of the publisher, the editors and the reviewers. Any product that may be evaluated in this article, or claim that may be made by its manufacturer, is not guaranteed or endorsed by the publisher.
The authors thank Piet Peters for his help with the fieldwork, the waterboard of Limburg for the kind sharing of data and assistance during field observations, and the landowners for cooperation by sharing data and giving access to their fields. We would like to thank the reviewers for their helpful comments on an earlier draft of this paper.
The Supplementary Material for this article can be found online at: https://www.frontiersin.org/articles/10.3389/fenvs.2022.830589/full#supplementary-material
AHN, (2019). AHN3 DTM. Available at: https://www.ahn.nl/ahn-3.
Aktar, M. W., Sengupta, D., and Chowdhury, A. (2009). Interdisciplinary toxicology, and undefined 2009. “Impact of Pesticides Use in Agriculture: Their Benefits and Hazards”. Sciendo. Com. doi:10.2478/v10102-009-0001-7
Anastassiades, M., Lehotay, S. J., Štajnbaher, D., and Schenck, F. J. (2003). Fast and Easy Multiresidue Method Employing Acetonitrile Extraction/Partitioning and "Dispersive Solid-phase Extraction" for the Determination of Pesticide Residues in Produce. J. AOAC Int. 86 (2), 412–431. doi:10.1093/jaoac/86.2.412
Arias-Estévez, M., López-Periago, E., Martínez-Carballo, E., Simal-Gándara, J., Mejuto, J.-C., and García-Río, L. (2008). The Mobility and Degradation of Pesticides in Soils and the Pollution of Groundwater Resources. Agric. Ecosyst. Environ. 123, 247–260. doi:10.1016/j.agee.2007.07.011
Baartman, J. E. M., Temme, A. J. A. M., Veldkamp, T., Jetten, V. G., and Schoorl, J. M. (2013). Exploring the Role of Rainfall Variability and Extreme Events in Long-Term Landscape Development. Catena 109 (October), 25–38. doi:10.1016/j.catena.2013.05.003
Bento, C. P. M., CommelinCommelin, M. C., Baartman, J. E. M., Yang, X., Peters, P., Mol, H. G. J., et al. (2018). Spatial Glyphosate and AMPA Redistribution on the Soil Surface Driven by Sediment Transport Processes - A Flume Experiment. Environ. Pollut. 234 (March), 1011–1020. doi:10.1016/j.envpol.2017.12.003
Bento, C. P. M., Yang, X., Gort, G., Xue, S., van Dam, R., Zomer, P., et al. (2016). Persistence of Glyphosate and Aminomethylphosphonic Acid in Loess Soil under Different Combinations of Temperature, Soil Moisture and Light/Darkness. Sci. Total Environ. 572 (December), 301–311. doi:10.1016/j.scitotenv.2016.07.215
Bevan, R., Brown, T., Matthies, F., Sams, C., Jones, K., Hanlon, J., et al. (2017). Human Biomonitoring Data Collection from Occupational Exposure to Pesticides. EFSA Support. Publ. 14 (3), 1185E. doi:10.2903/SP.EFSA.2017.EN-1185
Boardman, J., and Evans, R. (2020). The Measurement, Estimation and Monitoring of Soil Erosion by Runoff at the Field Scale: Challenges and Possibilities with Particular Reference to Britain. Prog. Phys. Geogr. Earth Environ. 44 (1), 31–49. doi:10.1177/0309133319861833
Boithias, L., Sauvage, S., Srinivasan, R., Leccia, O., and Sánchez-Pérez, J.-M. (2014). Application Date as a Controlling Factor of Pesticide Transfers to Surface Water during Runoff Events. Catena 119 (August), 97–103. doi:10.1016/j.catena.2014.03.013
Boivin, A., and Poulsen, V. (2017). Environmental Risk Assessment of Pesticides: State of the Art and Prospective Improvement from Science. Environ. Sci. Pollut. Res. 24 (8), 6889–6894. doi:10.1007/s11356-016-8289-2
Borrelli, P., Alewell, C., Alvarez, P., Anache, J. A. A., Baartman, J., Ballabio, C., et al. (2021). Soil Erosion Modelling: A Global Review and Statistical Analysis. Sci. Total Environ. 780 (August), 146494. doi:10.1016/j.scitotenv.2021.146494
Borselli, L., Cassi, P., and Torri, D. (2008). Prolegomena to Sediment and Flow Connectivity in the Landscape: A GIS and Field Numerical Assessment. CATENA 75 (3), 268–277. doi:10.1016/J.CATENA.2008.07.006
Bos, M. G. G. (1989). Discharge Measurement Structures. 3rd ed. Delft, The Netherlands: Delft Hydraul. Lab. doi:10.1201/9781315141343-2
Bracken, L. J., and Croke, J. (2007). The Concept of Hydrological Connectivity and its Contribution to Understanding Runoff-Dominated Geomorphic Systems. Hydrol. Process. 21 (13), 1749–1763. doi:10.1002/HYP.6313
Casado, J., Brigden, K., Santillo, D., and Johnston, P. (2019). Screening of Pesticides and Veterinary Drugs in Small Streams in the European Union by Liquid Chromatography High Resolution Mass Spectrometry. Sci. Total Environ. 670 (June), 1204–1225. doi:10.1016/j.scitotenv.2019.03.207
Cavalli, M., Trevisani, S., Comiti, F., and Marchi, L. (2013). Geomorphometric Assessment of Spatial Sediment Connectivity in Small Alpine Catchments. Geomorphology 188 (April), 31–41. doi:10.1016/J.GEOMORPH.2012.05.007
Cerdan, O., Le Bissonnais, Y., Govers, G., Lecomte, V., Van Oost, K., Couturier, A., et al. (2004). Scale Effect on Runoff from Experimental Plots to Catchments in Agricultural Areas in Normandy. J. Hydrology 299 (1–2), 4–14. doi:10.1016/j.jhydrol.2004.02.017
Chiovarou, E. D., and Siewicki, T. C. (2008). Comparison of Storm Intensity and Application Timing on Modeled Transport and Fate of Six Contaminants. Sci. Total Environ. 389 (1), 87–100. doi:10.1016/J.SCITOTENV.2007.08.029
Climent, M. J., Herrero-Hernández, E., Sánchez-Martín, M. J., Rodríguez-Cruz, M. S., Pedreros, P., and Urrutia, R. (2019). Residues of Pesticides and Some Metabolites in Dissolved and Particulate Phase in Surface Stream Water of Cachapoal River Basin, Central Chile. Environ. Pollut. 251 (August), 90–101. doi:10.1016/j.envpol.2019.04.117
Cruzeiro, C., Pardal, M. Â., Rodrigues-Oliveira, N., Castro, L. F. C., Rocha, E., and Rocha, M. J. (2016). Multi-Matrix Quantification and Risk Assessment of Pesticides in the Longest River of the Iberian Peninsula. Sci. Total Environ. 572 (December), 263–272. doi:10.1016/j.scitotenv.2016.07.203
Eads, R. E., and Thomas, R. B. (1983). Evaluation of a Depth Proportional Intake Device for Automatic Pumping Samplers. J. Am. Water Resour. Assoc. 19 (2), 289–292. doi:10.1111/j.1752-1688.1983.tb05328.x
European Commission (2019). Analytical Quality Control and Method Validation for Pesticide Residues Analysis in Food and Feed(SANTE/12682/2019). Sante/12682/2019 Available at: https://www.eurl-pesticides.eu/docs/public/tmplt_article.asp?CntID=727.
FAO (2020). FaoStat. Available at: http://www.fao.org/faostat/en/#data/EPData.
FAO, IFAD, UNICEF, WFP, WHO (2020). The State of Food Security and Nutrition in the World 2020. doi:10.4060/ca9692en
Geiger, F., Bengtsson, J., Berendse, F., Weisser, W. W., Emmerson, M., Morales, M. B., et al. (2010). Persistent Negative Effects of Pesticides on Biodiversity and Biological Control Potential on European Farmland. Basic Appl. Ecol. 11 (2), 97–105. doi:10.1016/j.baae.2009.12.001
Geissen, V., Silva, V., Lwanga, E. H., Beriot, N., Oostindie, K., Bin, Z., et al. (2021). Cocktails of Pesticide Residues in Conventional and Organic Farming Systems in Europe - Legacy of the Past and Turning Point for the Future. Environ. Pollut. 278 (June), 116827. doi:10.1016/j.envpol.2021.116827
Gettel, M., Gulliver, J. S., Kayhanian, M., Degroot, G., Brand, J., Mohseni, O., et al. (2011). Improving Suspended Sediment Measurements by Automatic Samplers. J. Environ. Monit. 13 (10), 2703–2709. doi:10.1039/c1em10258c
Ghadiri, H., and Rose, C. W. (1991a). Sorbed Chemical Transport in Overland Flow: I. A Nutrient and Pesticide Enrichment Mechanism. J. Environ. Qual. 20 (3), 628–33. doi:10.2134/jeq1991.00472425002000030020x
Ghadiri, H., and Rose, C. W. (1991b). Sorbed Chemical Transport in Overland Flow: II. Enrichment Ratio Variation with Erosion Processes. J. Environ. Qual. 20 (3), 628–41. doi:10.2134/jeq1991.00472425002000030021x
Halbach, K., Möder, M., Schrader, S., Liebmann, L., Schäfer, R. B., Schneeweiss, A., et al. (2021). Small Streams-Large Concentrations? Pesticide Monitoring in Small Agricultural Streams in Germany during Dry Weather and Rainfall. Water Res. 203 (August), 117535. doi:10.1016/j.watres.2021.117535
Heckmann, T., Cavalli, M., Cerdan, O., Foerster, S., Javaux, M., Lode, E., et al. (2018). Indices of Sediment Connectivity: Opportunities, Challenges and Limitations. Earth-Science Rev. 187 (December), 77–108. doi:10.1016/J.EARSCIREV.2018.08.004
Imfeld, G., Payraudeau, S., Tournebize, J., Sauvage, S., Macary, F., Chaumont, C., et al. (2021). The Role of Ponds in Pesticide Dissipation at the Agricultural Catchment Scale: A Critical Review. Water 13, 1202. doi:10.3390/w13091202
KNMI (2021a). Monthly and Yearly Amount of Precipitation. Available at: https://cdn.knmi.nl/knmi/map/page/klimatologie/gegevens/maandgegevens/mndgeg_380_rh24.txt.
KNMI (2021b). Monthly and Yearly Mean Temperatures. Available at: https://cdn.knmi.nl/knmi/map/page/klimatologie/gegevens/maandgegevens/mndgeg_380_tg.txt.
KNMI (2021c). Precipitation - Radar/Gauge 5 minute Final Reanalysis Accumulations over the Netherlands. https://dataplatform.knmi.nl/dataset/nl-rdr-data-rfcor-5m-1-0.
Lanz, B., Dietz, S., and Swanson, T. (2018). The Expansion of Modern Agriculture and Global Biodiversity Decline: An Integrated Assessment. Ecol. Econ. 144 (February), 260–277. doi:10.1016/j.ecolecon.2017.07.018
Lechenet, M., Dessaint, F., Py, G., Makowski, D., and Munier-Jolain, N. (2017). Reducing Pesticide Use while Preserving Crop Productivity and Profitability on Arable Farms. Nat. Plants 3 (3), 2017. doi:10.1038/nplants.2017.8
Lefrancq, M., Jadas-Hécart, A., La Jeunesse, I., Landry, D., and Payraudeau, S. (2017). High Frequency Monitoring of Pesticides in Runoff Water to Improve Understanding of Their Transport and Environmental Impacts. Sci. Total Environ. 587-588 (June), 75–86. doi:10.1016/j.scitotenv.2017.02.022
Leonard, R. A. (1990). “Movement of Pesticides into Surface Waters,” in Pesticides in the Soil Environment: Processes, Impacts, and Modeling (John Wiley & Sons), 303–49, 303–349. doi:10.2136/sssabookser2.c9
Lewis, K. A., Tzilivakis, J., Warner, D. J., and Green., A. (2016). An International Database for Pesticide Risk Assessments and Management. Hum. Ecol. Risk Assess. Int. J. 22 (4), 1050–1064. doi:10.1080/10807039.2015.1133242
López-Vicente, M., and Ben-Salem, N. (2019). Computing Structural and Functional Flow and Sediment Connectivity with a New Aggregated Index: A Case Study in a Large Mediterranean Catchment. Sci. Total Environ. 651 (February), 179–191. doi:10.1016/J.SCITOTENV.2018.09.170
Maillard, E., and Imfeld, G. (2014). Pesticide Mass Budget in a Stormwater Wetland. Environ. Sci. Technol. 48 (15), 8603–8611. doi:10.1021/es500586x
Maillard, E., Payraudeau, S., Faivre, E., Grégoire, C., Gangloff, S., and Imfeld, G. (2011). Removal of Pesticide Mixtures in a Stormwater Wetland Collecting Runoff from a Vineyard Catchment. Sci. Total Environ. 409 (11), 2317–2324. doi:10.1016/j.scitotenv.2011.01.057
Masiá, A., Campo, J., Vázquez-Roig, P., Blasco, C., and Picó, Y. (2013). Screening of Currently Used Pesticides in Water, Sediments and Biota of the Guadalquivir River Basin (Spain). J. Hazard. Mater. 263 (December), 95–104. doi:10.1016/j.jhazmat.2013.09.035
McCall, P. J., Laskowski, D. A., Swann, R. L., and Dishburger, H. J. (1980). “Measurement of Sorption Coefficients of Organic Chemicals and Their Use in Environmental Fate Analysis Test Protocols for Environ.mental Fate and Movement of Toxicants,” in Proceedings of the 94th Annual Meeting of the American Association of Official Analytical Chemists (AOAC).
Melland, A. R., Silburn, D. M., McHugh, A. D., Fillols, E., Rojas-Ponce, S., Baillie, C., et al. (2016). Spot Spraying Reduces Herbicide Concentrations in Runoff. J. Agric. Food Chem. 64 (20), 4009–4020. doi:10.1021/acs.jafc.5b03688
Mol, H. G. J., Plaza-Bolaños, P., Zomer, P., De Rijk, T. C., Stolker, A. A. M., and Mulder, P. P. J. (2008). Toward a Generic Extraction Method for Simultaneous Determination of Pesticides, Mycotoxins, Plant Toxins, and Veterinary Drugs in Feed and Food Matrixes. Anal. Chem. 80 (24), 9450–9459. doi:10.1021/ac801557f
Morgan, R. P. C., Morgan, D. D. V., and Finney, H. J. (1984). A Predictive Model for the Assessment of Soil Erosion Risk. J. Agric. Eng. Res. 30 (C), 245–253. doi:10.1016/S0021-8634(84)80025-6
Muñoz-Carpena, R., Fox, G. A., Ritter, A., Perez-Ovilla, O., and Rodea-Palomares, I. (2018). Effect of Vegetative Filter Strip Pesticide Residue Degradation Assumptions for Environmental Exposure Assessments. Sci. Total Environ. 619-620, 977–987. doi:10.1016/j.scitotenv.2017.11.093
Muñoz-Carpena, R., Ritter, A., and Fox, G. A. (2019). Comparison of Empirical and Mechanistic Equations for Vegetative Filter Strip Pesticide Mitigation in Long-Term Environmental Exposure Assessments. Water Res. 165, 114983. doi:10.1016/j.watres.2019.114983
Napoli, M., Marta, A. D., Zanchi, C. A., Orlandini, S., Anna Dalla Ad Marta, Ad., et al. (2016). Transport of Glyphosate and Aminomethylphosphonic Acid under Two Soil Management Practices in an Italian Vineyard. J. Environ. Qual. 45 (5), 1713–1721. doi:10.2134/jeq2016.02.0061
Oliver, D. P., Kookana, R. S., Anderson, J. S., Cox, J. W., Waller, N., and Smith, L. H. (2012). Off-Site Transport of Pesticides in Dissolved and Particulate Forms from Two Land Uses in the Mt. Lofty Ranges, South Australia. Agric. Water Manag. 106 (April), 78–85. doi:10.1016/j.agwat.2011.11.001
Olivier, C., Goffart, J. P., Baets, D., Xanthoulis, D., Fonder, N., Lognay, G., et al. (2014). Use of Micro-dams in Potato Furrows to Reduce Erosion and Runoff and Minimise Surface Water Contamination through Pesticides. Commun. Agric. Appl. Biol. Sci. 79 (3), 513
Panagos, P., Borrelli, P., Poesen, J., Ballabio, C., Lugato, E., Meusburger, K., et al. (2015). The New Assessment of Soil Loss by Water Erosion in Europe. Environ. Sci. Policy 54 (December), 438–447. doi:10.1016/j.envsci.2015.08.012
Parshall, R. L. (1926). The Improved Venturi Flume. T. Am. Soc. Civ. Eng. 89 (1), 841–851. doi:10.1061/taceat.0003626
Peruzzo, P. J., Porta, A. A., and Ronco, A. E. (2008). Levels of Glyphosate in Surface Waters, Sediments and Soils Associated with Direct Sowing Soybean Cultivation in North Pampasic Region of Argentina. Environ. Pollut. 156 (1), 61–66. doi:10.1016/j.envpol.2008.01.015
Prasuhn, V. (2020). Twenty Years of Soil Erosion On‐farm Measurement: Annual Variation, Spatial Distribution and the Impact of Conservation Programmes for Soil Loss Rates in Switzerland. Earth Surf. Process. Landforms 45 (7), 1539–1554. doi:10.1002/ESP.4829
QGIS (2021). QGIS Geographic Information System, 3.16. Hannover QGIS Assosaction. http://www.qgis.org.
R Core Team (2021). R: A Language and Environment for Statistical Computing. Vienna: R Foundation for Statistical Computing. https://www.r-project.org/.
Reichenberger, S., Bach, M., Skitschak, A., and Frede, H.-G. (2007). Mitigation Strategies to Reduce Pesticide Inputs into Ground- and Surface Water and Their Effectiveness; A Review. Sci. Total Environ. 384 (1–3), 1–35. doi:10.1016/j.scitotenv.2007.04.046
Reichenberger, S., Sur, R., Kley, C., Sittig, S., and Multsch, S. (2019). Recalibration and Cross-Validation of Pesticide Trapping Equations for Vegetative Filter Strips (VFS) Using Additional Experimental Data. Sci. Total Environ. 647, 534–550. doi:10.1016/j.scitotenv.2018.07.429
Ritz, C., Baty, F., Streibig, J. C., and Gerhard, D. (2015). Dose-Response Analysis Using R. PLoS ONE 10 (12), e0146021. doi:10.1371/journal.pone.0146021
Roper, W. R., Robarge, W. P., Osmond, D. L., and Heitman, J. L. (2019). Comparing Four Methods of Measuring Soil Organic Matter in North Carolina Soils. Soil Sci. Soc. Am. J. 83 (2), 466–474. doi:10.2136/sssaj2018.03.0105
Sattler, C., Kächele, H., and Verch, G. (2007). Assessing the Intensity of Pesticide Use in Agriculture. Agric. Ecosyst. Environ. 119 (3–4), 299–304. doi:10.1016/j.agee.2006.07.017
Schuhmann, A., Klammler, G., Weiss, S., Gans, O., Fank, J., Haberhauer, G., et al. (2019). Degradation and Leaching of Bentazone, Terbuthylazine and S-Metolachlor and Some of Their Metabolites: A Long-Term Lysimeter Experiment. Plant Soil Environ. 65 (No. 5), 273–281. doi:10.17221/803/2018-PSE
Silva, V., Mol, H. G. J., Zomer, P., Tienstra, M., Ritsema, C. J., and Geissen, V. (2019). Pesticide Residues in European Agricultural Soils - A Hidden Reality Unfolded. Sci. Total Environ. 653 (February), 1532–1545. doi:10.1016/j.scitotenv.2018.10.441
Syafrudin, M., Kristanti, R. A., Yuniarto, A., Hadibarata, T., Rhee, J., Al-Onazi, W. A., et al. (2021). Pesticides in Drinking Water-A Review. Int. J. Environ. Res. Public. Health. 18, 468. doi:10.3390/IJERPH18020468
Tang, X., Zhu, B., and Katou, H. (2012). A Review of Rapid Transport of Pesticides from Sloping Farmland to Surface Waters: Processes and Mitigation Strategies. J. Environ. Sci. 24 (3), 351–361. doi:10.1016/S1001-0742(11)60753-5
Taylor, J. R., and Thompson., W. (1998). An Introduction to Error Analysis: The Study of Uncertainties in Physical Measurements. Phys. Today 51 (1), 57–58. doi:10.1063/1.882103
Todorovic, G. R., Rampazzo, N., Mentler, A., Blum, W. E. H., Eder, A., Strauss, P., et al. (2014). Influence of Soil Tillage and Erosion on the Dispersion of Glyphosate and Aminomethylphosphonic Acid in Agricultural Soils. Int. Agrophysics 28 (1), 93–100. doi:10.2478/intag-2013-0031
Topping, C. J., Aldrich, A., and Berny, P. (2020). Overhaul Environmental Risk Assessment for Pesticides. Science 367, 360–363. doi:10.1126/science.aay1144
Wauchope, R. D. (1978). The Pesticide Content of Surface Water Draining from Agricultural Fields-A Review. J. Environ. Qual. 7 (4), 459–472. doi:10.2134/jeq1978.00472425000700040001x
Wauchope, R. D., Yeh, S., Linders, J. B. H. J., Kloskowski, R., Tanaka, K., Rubin, B., et al. (2002). Pesticide Soil Sorption Parameters: Theory, Measurement, Uses, Limitations and Reliability. Pest. Manag. Sci. 58, 419–445. doi:10.1002/ps.489
Yang, X., Wang, F., Bento, C. P. M., Meng, L., van Dam, R., Mol, H., et al. (2015a). Decay Characteristics and Erosion-Related Transport of Glyphosate in Chinese Loess Soil under Field Conditions. Sci. Total Environ. 530-531 (October), 53087–53195. doi:10.1016/j.scitotenv.2015.05.082
Yang, X., Wang, F., Bento, C. P. M., Xue, S., Gai, L., van Dam, R., et al. (2015b). Short-Term Transport of Glyphosate with Erosion in Chinese Loess Soil - A Flume Experiment. Sci. Total Environ. 512-513513 (April), 406–414. doi:10.1016/j.scitotenv.2015.01.071
Yu, C., Duan, P., Yu, Z., and Gao, B. (2019). Experimental and Model Investigations of Vegetative Filter Strips for Contaminant Removal: A Review. Ecol. Eng. 126 (October 2018), 25–36. doi:10.1016/j.ecoleng.2018.10.020
Keywords: erosion, pesticide transport, particulate phase, event-based monitoring, runoff
Citation: Commelin MC, Baartman JEM, Zomer P, Riksen M and Geissen V (2022) Pesticides are Substantially Transported in Particulate Phase, Driven by Land use, Rainfall Event and Pesticide Characteristics—A Runoff and Erosion Study in a Small Agricultural Catchment. Front. Environ. Sci. 10:830589. doi: 10.3389/fenvs.2022.830589
Received: 07 December 2021; Accepted: 09 May 2022;
Published: 01 June 2022.
Edited by:
Jiehong Guo, University of Minnesota Twin Cities, United StatesReviewed by:
Cláudio Ernesto Taveira Parente, Federal University of Rio de Janeiro, BrazilCopyright © 2022 Commelin, Baartman, Zomer, Riksen and Geissen. This is an open-access article distributed under the terms of the Creative Commons Attribution License (CC BY). The use, distribution or reproduction in other forums is permitted, provided the original author(s) and the copyright owner(s) are credited and that the original publication in this journal is cited, in accordance with accepted academic practice. No use, distribution or reproduction is permitted which does not comply with these terms.
*Correspondence: Meindert C. Commelin, bWVpbmRlcnQuY29tbWVsaW5Ad3VyLm5s
Disclaimer: All claims expressed in this article are solely those of the authors and do not necessarily represent those of their affiliated organizations, or those of the publisher, the editors and the reviewers. Any product that may be evaluated in this article or claim that may be made by its manufacturer is not guaranteed or endorsed by the publisher.
Research integrity at Frontiers
Learn more about the work of our research integrity team to safeguard the quality of each article we publish.