- 1Department of Agricultural and Forestry Science and Technology, Chongqing Three Gorges Vocation College, Chongqing, China
- 2Key Laboratory of Water Environment Evolution and Pollution Control in Three Gorges Reservoir, Chongqing Three Gorges University, Wanzhou, China
- 3School of Hydrology and Water Resources, Nanjing University of Information Science and Technology, Nanjing, China
The riparian zone is a transitional zone of terrestrial ecosystems and aquatic ecosystems, which is one of the main sources of reservoir sediment and pollutants. The sediment loss has an increasing trend in the riparian zone of the Three Gorges Reservoir (TGR), which was closely related to sediment particle size composition change in the riparian zone, but its distribution and factors are still unclear. Hydrological conditions, sediment particle size composition and vegetation properties in the riparian zone were investigated in 3 mainstream and 8 tributary sections along the Yangtze River within the TGR across 450 km. On the one hand, both sediment sand content and median particle size (D50) showed a horizontal raising trend from the upstream to the dam region of the TGR at the mainstream and tributary sites. On the other hand, compared to the upper-middle mainstream and the tributaries of Longxi, Wu, Longxi, Pengxi, Daning, Tongzi rivers, the D50 was much higher in the lower altitudes of the riparian zone in the tributaries of Wubu, Ruxi, and Xiangxi and the mainstream in the lower reaches of the Yangtze River. With the raising of the riparian zone slope, D50 decreased in the mainstream riparian zone sites, while increased in the tributary riparian zone sites (p < 0.05). Besides, D50 was 2.3 times higher in the south aspect relative to the north aspect of the riparian zone in the TGR. No significant relationship was found between root biomass of a local dominate species-Cynodon Dactylon (Linn.) and D50. Hydrological and geographical variables could predict 64%–67% of the D50 variance, and thus could be regarded as the main predictor of D50 in the riparian zone of the TGR.
Introduction
The Three Gorges Dam (TGD) is a critical hydro-electric project in China, which completed the 175 m trial impoundment since 2010, forming the 660 km Three Gorges Reservoir (TGR) strip from Zigui County to the central district of Chongqing (Su et al., 2017). The drainage area is about 1 × 106 km2 in the TGR, which intercepts the outlet of the upper reaches and the main channel of the Yangtze River (Tang et al., 2016; Pei et al., 2018). Based on the flow regulation, the water-level in the TGR gradually rises to 175 m in September and kept constant until the following January for power generation and irrigation. Thereafter, it recedes to the lowest level (145 m) in late June for flood control (Ye et al., 2011), which disordered the natural balance of sediment transport in the TGR (Bing et al., 2016).
The reverse seasonal hydrological process has led to the formation of a riparian zone in the TGR with an area of ∼349 km2 and a vertical drop of 30 m (Wu et al., 2004; Zhang et al., 2020). It represents a critical transitional zone between an aquatic and terrestrial environment. Thus, it could alternate between being sediment “source” or “sink” based on the periodic water-level fluctuations in the TGR (Fei Ye et al., 2019). The sediment in the riparian zone of the TGR mainly originates from water impoundment and bank erosion (Panwar et al., 2016; Wang et al., 2016a). The sediment particle size composition largely depends on the responses of sediment stability to hydrological stresses (Ran et al., 2020). The finer particles in sediment may be easily moved by fluid flow, forming a layer of solid particles in the riparian zone’s surface layer (Knighton 1980; Rhoads 1989). Further, the finer particles in sediments can be released to the water-column via hydrodynamic disturbance under variable sedimentary conditions, causing potential risk to aquatic biota or the TGD operation (Tang et al., 2016). In the torrent streams of the Mediterranean environment, investigating the effects of check dams on riparian vegetation, relationships were found between synthetic vegetation parameters (such as, global canopy cover and weighted canopy height) and the number of species (Bombino et al., 2008).
Fundamentals of sediment transport pointed out that the sediment transport regime is mainly controlled by the chemical composition of the fluid, the physicochemical properties of cohesive sediments, and the hydrodynamics, which can effectively affect the processes of deposition, settling, erosion, advection, dispersion, and flocculation, etc (Hayter and Gailani, 2014). The periodic hydrologic fluctuation is one of the crucial driving factors for sediment transportation (Lin et al., 2018). First, the impoundment turned the natural river into a lake by reducing flow velocity and increasing the flow displacement. Second, the enlarged width of the river channel can increase the dam storage (Yang et al., 2015). The sediment in riparian zone may be relocated between the mainstream and tributaries, the upstream and downstream areas, or among riparian zone altitudes (Lu and Higgitt, 2001). However, the sediment particle size distribution in the riparian zone of the Yangtze River on the basin-scale under periodic flood has not received adequate attention and empirical investigation. Therefore, it is critical to clarify the distribution and its influencing factors of sediment particle size composition in the riparian zone of the TGR for the security operation of the Three Gorges Dam.
This study aimed to 1) investigate the distribution of sediment particle size composition in the riparian zone in the TGR and 2) clarify the factors that regulate sediment particle size composition in the riparian zone of the TGR. We hypothesize that 1) sediment median particle size (D50) in the riparian zone horizontally increases from the upstream of the Yangtze River to the TGD; 2) D50 are primarily driven by hydrological and geographic variables.
Materials and Methods
Study Area
The TGR is located in the upper reaches of the Yangtze River (N28o 10′–N31o 13′ and E105 o 11′–E110 o 38′), which represents the inundated and migratory area affected by the TGR backwater after the completion of the TGD (Figure 1 and Table 1). The administrative area extends from Yichang in Hubei Province in the East to Jiangjin in Chongqing in the west, including 4 counties in Hubei Province and 22 districts (counties) in Chongqing City, with an area of 5.8 × 104 km2 (Ming-feng Wang et al., 2020). The region is characterized by rolling hills and valleys in the transition zone (from the second to the third step) of the terrain in China (Tang et al., 2016). It has subtropical monsoon and humid climate, with an average annual precipitation of 1120 mm, an average annual temperature of 16.3–18.2°C, numerous high-temperature days, a frost-free period of 300–340 days, and an annual sunlight period of 1200–1600 h in most areas (Lin et al., 2019).
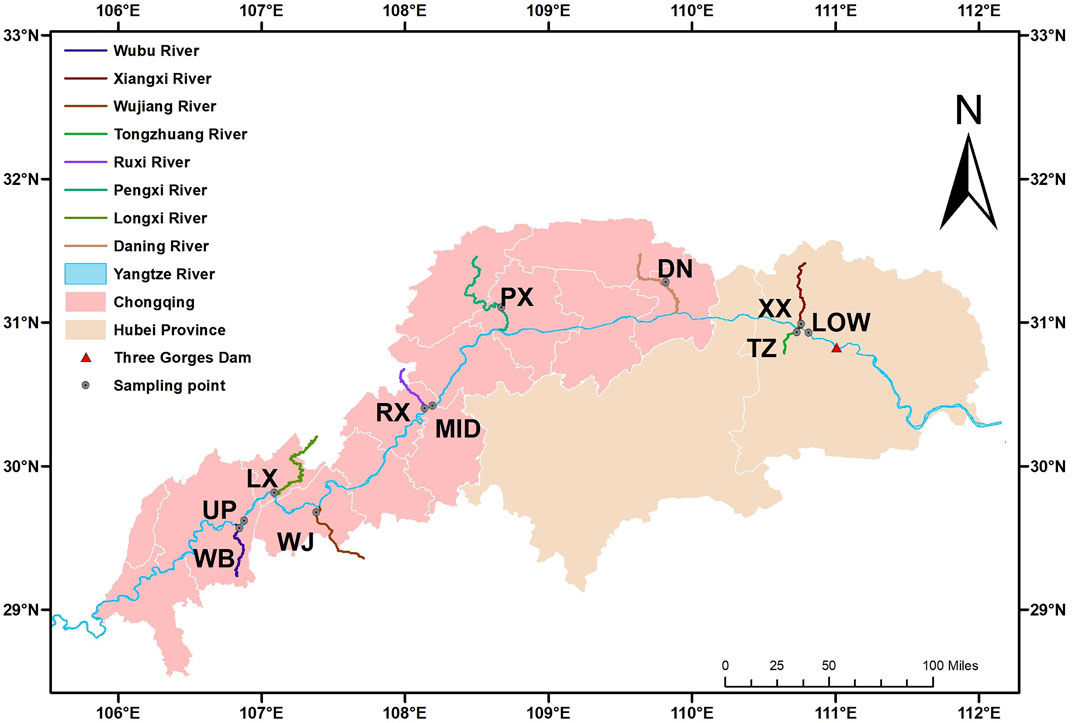
FIGURE 1. Sampling sites in the Three Gorges Reservoir. Wubu (WB), Longxi (LX), Wujiang (WJ), Ruxi (RX), Pengxi (PX), Daning (DN), Tongzhuang (TZ), and Xiangxi (XX) rivers; upstream (UP), middle (MID), and lower (LOW) sections of the Three Gorges Reservoir.
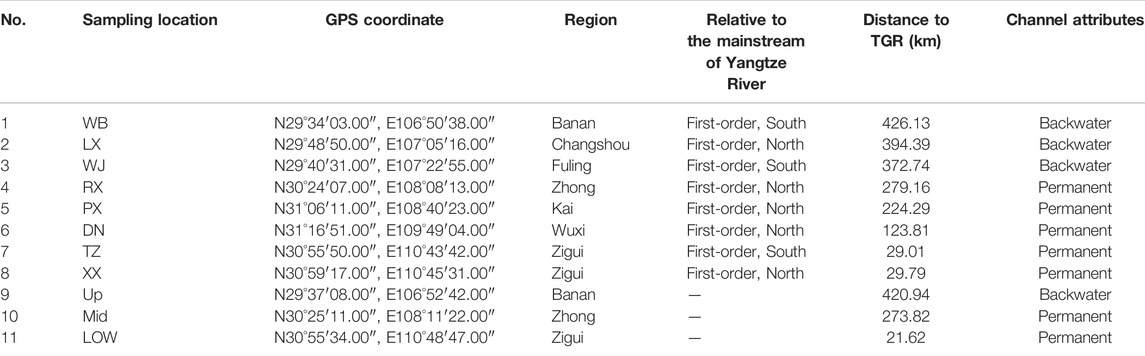
TABLE 1. Characteristics of sampling transects in the mainstream and tributaries of the Yangtze River. Wubu (WB), Longxi (LX), Wu (WJ), Ruxi (RX), Pengxi (PX), Daning (DN), Tongzhuang (TZ), and Xiangxi (XX) rivers; upper (Up), middle (Mid), and lower (LOW) reaches of the mainstream of the Yangtze River.
Sampling
Surface sediment (0–20 cm) was collected from 3 mainstream sites and 8 tributary sites in the riparian zone (145–155, 155–165, and 165–175 m altitudes) and non-flooding area (175–185 m altitude) of the Yangtze River in July 2019 (Figures 1, 2). Each sample was consisted of three random sampling plots (1 × 1 m) at each altitude. Thus, there were 132 samples in total, including 11 transects × 4 altitudes × 3 replicates. All soil samples were immediately stored in a 5°C incubator and then brought to a laboratory within 24 h. All of them were air-dried and passed through a 2-mm sieve after removing plant residues. In each plot, the shoot of dominant species of Cynodon Dactylon (Linn.) was removed and the root part as belowground biomass was carefully collected by shaking, and then brought back into the laboratory immediately. Root samples were washed and 60oC oven-dried and weighed.
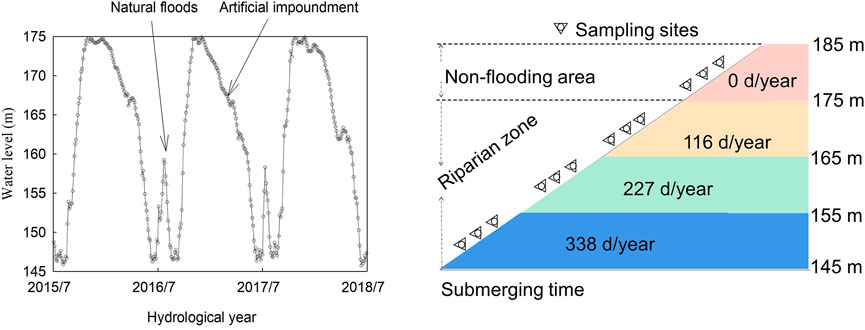
FIGURE 2. Water-level fluctuation in the Three Gorges Reservoir and plot distribution in the riparian zone of the Three Gorges Reservoir (TGR).
Laboratory Analysis
Particle size composition was measured using the hydrometer method (Gee and Bauder, 1979). Bulk samples were grouped into clay (<0.002 mm), silt (0.002–0.02 mm) and sandy (0.02–2 mm) fractions. Median diameter size (D50) was quantified by the particle-size distribution curves (Gee and Or, 2002). Soil moisture and bulk density were measured by 100 cm3 soil core (McKenzie et al., 2002).
Data Acquisition and Processing
The daily water-level in the TGR was obtained from www.ctgpc.com.cn, reported by the China Three Gorges Group Limited Company. The flooding duration was obtained from the hydrological data (2015–2018) using GetData (V2.20) with a 3 m interval. Structure equation modelling (SEM) was used to assess potential causal relationships of D50 with hydrological and geographic variables. The overall goodness of fitting for the model was tested by chi-square (χ2). The model is satisfying when χ2/df within 0–2, non-significant χ2 test (p > 0.05) and low values of root mean square error of approximation, χ2, and akaike information criterion (Schermelleh-Engel et al., 2003), and indicate that the difference between the observed and modelled values is acceptable.
Statistical Analysis
One-way ANOVA was used to examine variations in particle-size composition and D50 between altitudes. All statistical analyses and plots were obtained using SPSS 20.0 for Windows and SigmaPlot 12.5, respectively.
Results
Sediment Moisture, Bulk Density and Particle Size Composition
The sediment moisture, bulk density, and proportions of clay, silt, and sand varied from 3.00 to 59.00%, 0.59–1.60 g cm−3, 7.00–43.00%, 9.00–65.00%, and 10.00–81.00%, respectively (Table 2). The coefficient of variation (C.V.) of sand content was the highest (43.25%), while that of bulk density was the lowest (15.23%). The C.V. of moisture, silt, and sand were >30%, thus showing a strong variation (Table 2). The proportion of clay decreased (r = 0.41 p < 0.05, Figure 3A), and that of sand (Figure 3C) and D50 increased from upstream of the Yangtze River to the TGD at both mainstream (Figure 3D) and tributary (Figure 3E) sites across 450 km, while no significant trend was observed for the proportion of silt content in the riparian zone of the TGR (Figure 3B).
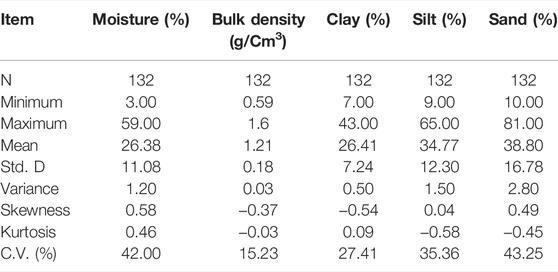
TABLE 2. Descriptive statistics of sediment particle size composition, moisture, and bulk density in the riparian zone of the TGR.
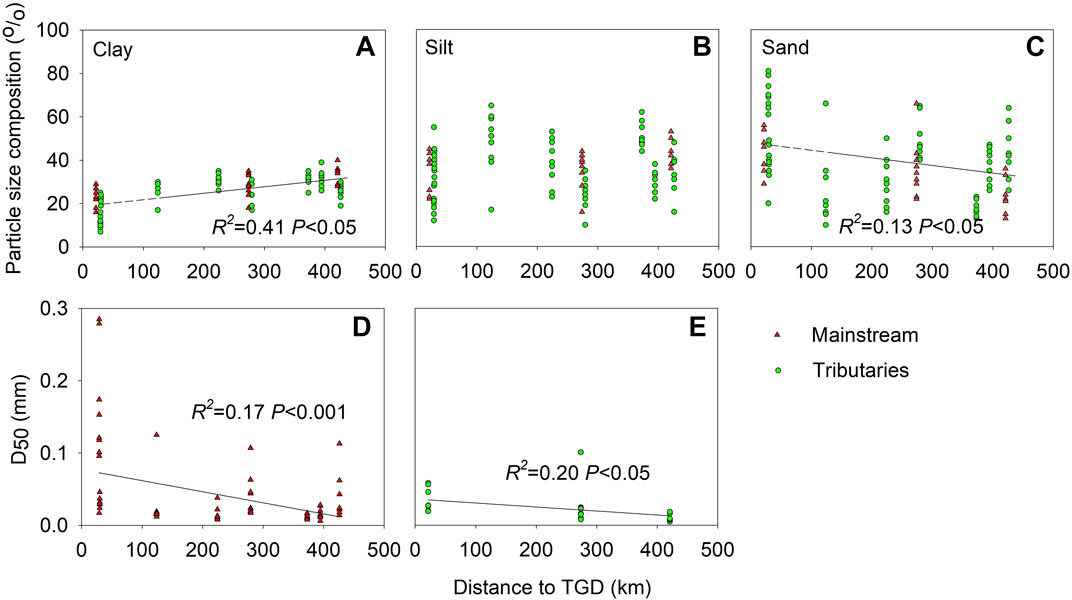
FIGURE 3. The distribution of particle size composition [Clay, (A); Silt, (B); Sand, (C)], and D50 in riparian zone sediment [Mainstream, (D); Tributary, (E)] with the distance to the Three Gorges Dam (TGD).
Sediment Particle-Size Distribution Along the Riparian Zone Altitudes
D50 was totally the highest for the TZ River (Figure 4J) and the lowest for the Wu River (Figure 4F). The D50 and the percentage of sand (coarser particle) decreased in the upper (Figure 4A) and middle (Figure 4B) sites and increased in the lower mainstream site (Figure 4C) with the decline of the riparian zone altitude. Besides, the proportion of sand and D50 decreased in the LX (Figure 4E), WU (Figure 4F), PX (Figure 4H), DN (Figure 4I), and TZ (Figure 4J) rivers, while it increased in the WB (Figure 4D), RX (Figure 4G), and XX (Figure 4K) rivers with the decline of the riparian zone altitudes.
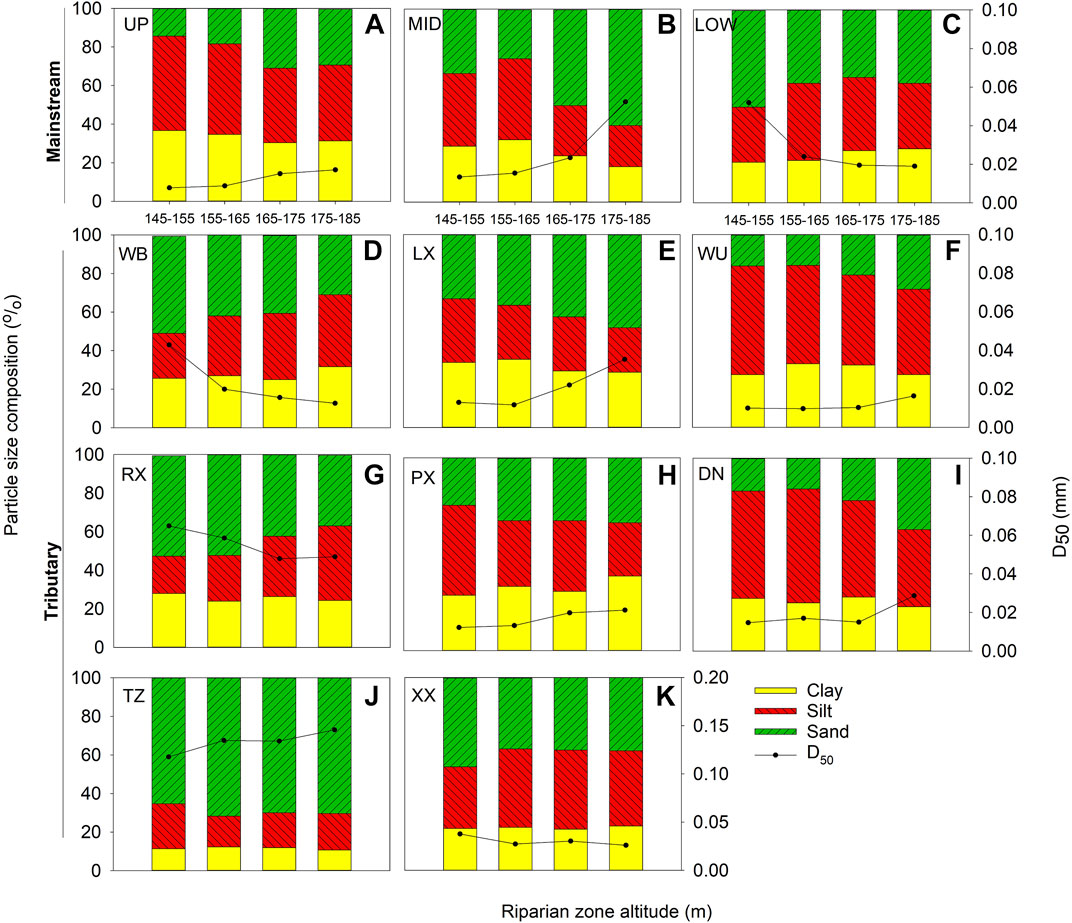
FIGURE 4. Particle-size and D50 distribution along the altitude of the riparian zone in the mainstream and tributaries of the TGR. Mainstream: upstream sections [UP, (A)], middle sections [MID, (B)], and lower sections [LOW, (C)]. Tributary: Wubu river [WB, (D)], Longxi river [LX, (E)], Wujiang river [WJ, (F)], Ruxi river [RX, (G)], Pengxi river [PX, (H)], Daning river [DN, (I)], Tongzhuang river [TZ, (J)], and Xiangxi river [XX, (K)].
Linking D50 to the Slope, Aspect, and Root Biomass of the Riparian Zone
The D50 was negatively correlated with the riparian zone’s slope in the mainstream (Figure 5A, p < 0.05) and positively correlated with the slope in the tributaries (Figure 5B, p < 0.05). D50 of the riparian zones located in the southern aspect of the Yangtze River was 2.3 times higher than that in the northern aspect (Figure 6). No significant relationship was found between D50 and root biomass of local dominant species-Cynodon Dactylon (Linn.) (Figure 7).
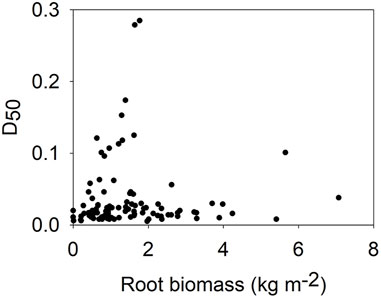
FIGURE 7. D50 distribution with root biomass of Cynodon Dactylon (Linn.) in the riparian zone of the TGR.
Modelling Drivers of D50
The SEM analysis showed that the submerging time had a direct positive effect on sediment bulk density and moisture. Further, the sediment bulk density negatively affected sediment clay content, while clay content had a direct negative effect on the D50 variation (Figure 8A). Besides, the geographical location of the riparian zone (the distance to the Three Gorges Dam) had a direct positive effect on sediment clay and the slope of riparian zone, while both of them had a direct negative impact on D50 variation (Figure 8B). The hydrological condition (submerging time) and geographical location could explain 64%–67% of D50 variation (Figure 8). Considering the total effect of direct and indirect effects, riparian zone slope and sediment bulk density could be regarded as the most critical predictors shaping the D50 variation (Figure 9).
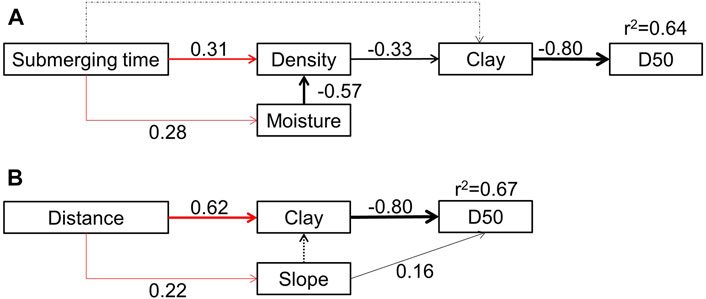
FIGURE 8. SEM with variables (boxes) and potential causal relationships (arrows) for D50. The black-headed arrows indicate that the hypothesized direction of causation is a negative relationship; contrarily, the red-headed arrows represent a positive relationship. The arrow-width is proportional to the value of path coefficients. The grey dashed-lines represent relationships that could exist between variables, but which are insignificant. Standardized path coefficients (numbers) could reflect the importance of the variables within the model (Colman and Schimel, 2013). The model for D50 (A) had χ2 = 4.652, df = 4, p = 0.053, RMSEA = 0.034, and AIC = 36.652; that for D50 (B) had χ2 = 1.4, df = 1, p = 0.231, RMSEA = 0.055, and AIC = 27.434. Clay: sediment clay content; Density: sediment bulk density; Distance: distance to the TGD.
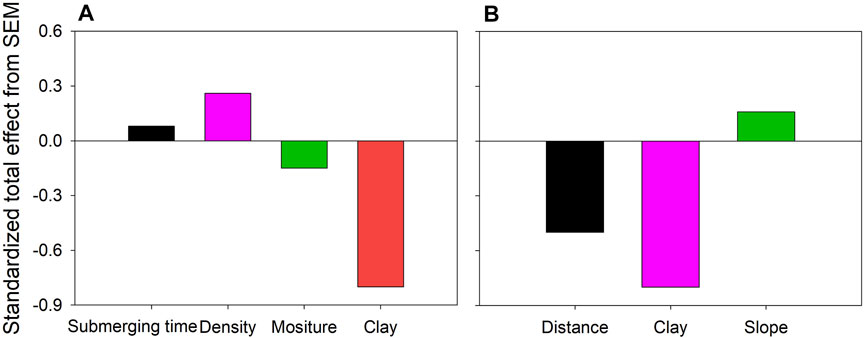
FIGURE 9. Standardized total effect (direct plus indirect) on D50 derived from the structural equation modeling (SEM). Clay: sediment clay content; Density: sediment bulk density; Distance: distance to the TGD. A: corresponding to SEM (Figure 8A); B: corresponding to SEM (Figure 8B).
Discussion
Horizontal Trend of Sediment Particle Size Composition
A horizontal coarsening trend of sediment particle size in the riparian zone from upstream of the Yangtze River to the TGD was observed in the TGR across 450 km (Figure 3). The sediment in the riparian zone mainly originates from suspended aquatic sediment deposition and surrounding basin erosion (Tang et al., 2016; Tang et al., 2018). Sediment particle-size composition is a crucial factor in determining the sediment transport capacity under the water level fluctuation condition. Besides, suspended sediment is one of the primary causes of sedimentary landform’s evolution in the riparian zone (Tang et al., 2014). Further, the sink sediment with finer particles in the riparian zone can quickly enter the reservoir under drastic hydrological fluctuations (Chen Ye et al., 2019). When sediment enters the reservoir, a horizontal variation is usually seen in the sedimentation processes due to a change in hydrodynamic conditions (Xinchen Wang et al., 2020). Moreover, sediment’s emptying effect due to flood discharge can result in a more severe riparian zone erosion towards the TGD (Zhang et al., 2012). Conversely, the increasing trend of finer particles (clay and silt) was found in the riparian zone towards the TGD (Bing et al., 2016), but the research only focused on the mainstream without considering the riparian zone altitudes and the tributaries. The emptying effect might be responsible for the coarsening trend in riparian zone sediment (Rice and Church, 1998; Zhang et al., 2012). Moreover, the blocking effect is relatively higher in the backwater area of the distal upstream (Huang et al., 2018). Thus, the finer sediment particle tends to deposit in the tail-end riparian zone of the reservoir rather than the surrounding of the TGD.
Hydrological Regime for Particle Size Composition Distribution
Periodic water-level fluctuations might be a potential driver for the distinct distribution of sediment particles in the riparian zone of the TGR. The SEM results suggested that submerging time was the primary driver of D50 (Figure 7A), which supported our second hypothesis. The more clay loss occurred in the riparian zone of the lower mainstream and the WB, RX, and XX tributaries, while more finer particles were enriched in the LX, WU, PX, DN, and TZ rivers (Figure 4). It can be explained as follows: first, the complex dynamic action of slope-runoff and current/wave/water-level fluctuations caused a distinct variation in the surface-sediment with different particle sizes in the riparian zone (Pan et al., 2017; Shmilovitz et al., 2020). Second, the flow velocity decreased with the TGR impoundment (Chen et al., 2008; Wei et al., 2016). This occurred particularly in the case of the tributaries as a result of the backwater blocking effect, which has caused numerous water quality issues associated with sediment input into the tributaries of the TGR (Li et al., 2018). Finally, the local impact of sediment load caused a temporal variability in sediment supply from different parts of the TGR (Lu and Higgitt, 2001; Bing et al., 2016).
Influence of Land-Use on Sediment Erosion-Deposition
Land use in the riverside basin has significantly influenced sediment particle size distribution in the riparian zone. The riparian zone erosion increased with vegetation’s destruction and succession (Corenblit et al., 2009). The annual erosion of forest land, shrub, grassland, and farmland accounts for 6.19%, 10.76%, 23.05%, and 60% of the total erosion of the TGR area, respectively, while the corresponding sediment export was accounted for 5.95%, 12.42%, 35.46%, and 46.16% of the total sediment export, respectively (Shi et al., 1991). Severe sediment erosion occurs due to cultivation along steep slopes (Lu and Higgitt, 2001). Besides, vegetation density could determine the sediment deposition rate by influencing the surface roughness of the riparian zone (Nagy et al., 2018). However, the vegetation cover indicated by the root-biomass weakly impacted D50 distribution (Figure 7). It might be explained by the fact that biodiversity drastically decreases under the anti-seasonal flow regulation, and only a few perennial herbs survive in the riparian zone from long-term winter flooding (Lin et al., 2020).
Morphology on Particle Size Composition Distribution
The channel morphology primarily influenced sediment particle size distribution in the riparian zone. About 40% of the riparian zone is distributed along the mainstream, while 60% is located along the Yangtze River’s tributaries. However, the riparian zone located in the Yangtze River’s southern aspect showed more severe erosion with the higher D50 (Figure 6). It was primarily related to the local terrain that comprises agricultural platforms (paddy fields and dry land) or steep slopes between platforms.
The slope also determines the distribution of sediment particle size in the riparian zone (Wang et al., 2016a). Sediment with more finer particle size composition tends to deposit in the riparian zone with a gentle slope, which tends to be lost in the riparian zone with a steep slope. With the slope becoming more gentle, finer sediment decreased in the riparian zone of the mainstream, and increased in the riparian zone of the tributaries (Figure 5). The riparian zones with slopes of <15o and 15–25o accounted for 60.94% and 18.56% of the total areas of riparian zone, respectively (Wang et al., 2016b). Moreover, the riparian zone slope in the mid-upper reaches of the TGR was gentle, thus benefitting finer sediment storage. Contrarily, the riparian zone slope in the lower reaches was relatively steep and composed of hillside bedrock (Bing et al., 2016), thus, it is hard to maintain relatively finer particles in the riparian zone under variable water levels (Bao et al., 2015). Thus, the slope plays a crucial role in distribution of particle size composition in the riparian zone sediment of the TGR. In addition, some studies have pointed out that lithology and climate factors play a role in determining sediment particle size (Khan and Chakrapani 2016; Daityari and Khan 2017), but they are not involved in this study.
Conclusion
Our investigation demonstrates that the finer sediment in the riparian zone has a decreased trend from the upstream of the Yangtze River to the TGD across 450 km. The trend was inconsistent between the mainstream and tributaries among the riparian zone’s altitudes due to the differences in land-use, morphology, and hydrological condition. Hydrological and geographical variables could predict 64%–67% of the D50 variance, and thus could be regarded as the main predictor of D50 in the riparian zone of the TGR. Thus, to reduce the finer sediment input, the conservation and stabilization of riparian zone in the TGR, especially in the mainstream and the surrounding areas near the TGD, should be highly concerned for the safe operation of the TGR.
Data Availability Statement
The original contributions presented in the study are included in the article/Supplementary Material, further inquiries can be directed to the corresponding author.
Author Contributions
JL designed the study, drew the figures and tables, and drafted the manuscript; L. Zhang, C. Yan, YL, X. Xiang, and SZ attended the field investigation and laboratory measurement; ZY, BL, AK, and DL instructed and coordinated the project, edited and smoothed the manuscript.
Funding
This work was supported by the Science and Technology Research Program of Chongqing Municipal Education Commission (KJZD-K202001203 and KJZD-K202003501); the Program of Chongqing Science and Technology Commission (cstc2020jcyj-msxmX0095); the Innovative Research Group of Universities in Chongqing (CXQT P19037).
Conflict of Interest
The authors declare that the research was conducted in the absence of any commercial or financial relationships that could be construed as a potential conflict of interest.
Publisher’s Note
All claims expressed in this article are solely those of the authors and do not necessarily represent those of their affiliated organizations, or those of the publisher, the editors and the reviewers. Any product that may be evaluated in this article, or claim that may be made by its manufacturer, is not guaranteed or endorsed by the publisher.
Acknowledgments
We thank L. Zhang, C. Yan, X. Xiang for field investigation. We also sincerely thank two anonymous reviewers for their necessary suggestions which vastly improved the manuscript.
References
Bao, Y., Gao, P., and He, X. (2015). The Water-Level Fluctuation Zone of Three Gorges Reservoir - A Unique Geomorphological Unit. Earth-Science Rev. 150, 14–24. doi:10.1016/j.earscirev.2015.07.005
Bing, H., Zhou, J., Wu, Y., Wang, X., Sun, H., and Li, R. (2016). Current State, Sources, and Potential Risk of Heavy Metals in Sediments of Three Gorges Reservoir, China. Environ. Pollut. 214, 485–496. doi:10.1016/j.envpol.2016.04.062
Bombino, G., Gurnell, A. M., Tamburino, V., Zema, D. A., and Zimbone, S. M. (2008). Sediment Size Variation in Torrents with Check Dams: Effects on Riparian Vegetation. Ecol. Eng. 32, 166–177. doi:10.1016/j.ecoleng.2007.10.011
Chen, X., Yan, Y., Fu, R., Dou, X., and Zhang, E. (2008). Sediment Transport from the Yangtze River, China, into the Sea over the Post-Three Gorge Dam Period: A Discussion. Quat. Int. 186, 55–64. doi:10.1016/j.quaint.2007.10.003
Chen Ye, C., Chen, C., Butler, O. M., Rashti, M. R., Esfandbod, M., Du, M., et al. (2019). Spatial and Temporal Dynamics of Nutrients in Riparian Soils after Nine Years of Operation of the Three Gorges Reservoir, China. Sci. Total Environ. 664, 841–850. doi:10.1016/j.scitotenv.2019.02.036
Colman, B. P., and Schimel, J. P. (2013). Drivers of Microbial Respiration and Net N Mineralization at the Continental Scale. Soil Biol. Biochem. 60, 65–76. doi:10.1016/j.soilbio.2013.01.003
Corenblit, D., Steiger, J., Gurnell, A. M., Tabacchi, E., and Roques, L. (2009). Control of Sediment Dynamics by Vegetation as a Key Function Driving Biogeomorphic Succession within Fluvial Corridors. Earth Surf. Process. Landforms 34, 1790–1810. doi:10.1002/esp.1876
Daityari, S., and Khan, M. Y. A. (2017). Temporal and Spatial Variations in the Engineering Properties of the Sediments in Ramganga River, Ganga Basin, India. Arabian J. Geosciences 10, 1–13. doi:10.1007/s12517-017-2915-2
Fei Ye, F., Ma, M. H., Wu, S. J., Jiang, Y., Zhu, G. B., Zhang, H., et al. (2019). Soil Properties and Distribution in the Riparian Zone: the Effects of Fluctuations in Water and Anthropogenic Disturbances. Eur. J. Soil Sci. 70, 664–673. doi:10.1111/ejss.12756
Gee, G. W., and Or, D. (2002). 2.4 Particle‐size Analysis. Methods Soil Anal. Part 4 Phys. Methods 5, 255–293. doi:10.2136/sssabookser5.4.c12
Gee, G. W., and Bauder, J. W. (1979). Particle Size Analysis by Hydrometer: a Simplified Method for Routine Textural Analysis and a Sensitivity Test of Measurement Parameters. Soil Sci. Soc. Am. J. 43, 1004–1007. doi:10.2136/sssaj1979.03615995004300050038x
Hayter, E. J., and Gailani, J. Z. (2014). “Fundamentals of Sediment Transport,” in Processes, Assessment and Remediation of Contaminated Sediments. Editor D. D. Reible (New York, NY: Springer New York), 25–79. doi:10.1007/978-1-4614-6726-7_3
Huang, F., Wu, Y., Qian, B., Guo, L., Zhao, D., Ren, L., et al. (2018). An Environmental Flow Assessment of a River's Blocking Effect on a lake in a River-lake System: Application in the Yangtze-Poyang System. Environ. Monit. Assess. 190, 453. doi:10.1007/s10661-018-6825-x
Khan, M. Y. A., and Chakrapani, G. J. (2016). Geostatistical and Geospatial Approaches for the Characterization of Natural Resources in the Environment. Cham: Springer International Publishing, 307–312. doi:10.1007/978-3-319-18663-4_47Particle Size Characteristics of Ramganga Catchment Area of Ganga River
Knighton, A. D. (1980). Longitudinal Changes in Size and Sorting of Stream-Bed Material in Four English Rivers. Geol. Soc. America Bull. 91, 55–62. doi:10.1130/0016-7606(1980)91<55:lcisas>2.0.co;2
Li, J., Yang, W., Li, W., Mu, L., and Jin, Z. (2018). Coupled Hydrodynamic and Water Quality Simulation of Algal Bloom in the Three Gorges Reservoir, China. Ecol. Eng. 119, 97–108. doi:10.1016/j.ecoleng.2018.05.018
Lin, J., Zhang, S., Liu, D., Yu, Z., Zhang, L., Cui, J., et al. (2018). Mobility and Potential Risk of Sediment-Associated Heavy Metal Fractions under Continuous Drought-Rewetting Cycles. Sci. Total Environ. 625, 79–86. doi:10.1016/j.scitotenv.2017.12.167
Lin, J., Tang, Y., Liu, D., Zhang, S., Lan, B., He, L., et al. (2019). Characteristics of Organic Nitrogen Fractions in Sediments of the Water Level Fluctuation Zone in the Tributary of the Yangtze River. Sci. Total Environ. 653, 327–333. doi:10.1016/j.scitotenv.2018.10.394
Lin, J., Zhou, S., Liu, D., Zhang, S., Yu, Z., and Yang, X. (2020). Relative Contribution of Environmental and Nutritional Variables to Net Primary Production of Cynodon Dactylon (Linn.) Pers in the Riparian Zone of a Three Gorges Tributary. Ecol. Evol. 10, 7073–7081. doi:10.1002/ece3.6409
Lu, X. X., and Higgitt, D. L. (2001). Sediment Delivery to the Three Gorges. Geomorphology 41, 157–169. doi:10.1016/s0169-555x(01)00113-1
McKenzie, N., Coughlan, K., and Cresswell, H. (2002). Soil Physical Measurement and Interpretation for Land Evaluation, 5 (Clayton South, VIC: Csiro Publishing).
Ming-feng Wang, M.-f., Tian, F.-x., He, X.-b., Anjum, R., Bao, Y.-h., and Collins, A. L. (2020). Dry Cropland Changes in China's Three Gorges Reservoir Region during the Period 1990 to 2015. J. Mt. Sci. 17, 516–527. doi:10.1007/s11629-019-5598-1
Nagy, J., Kiss, T., Fehérváry, I., and Vaszkó, C. (2018). Changes in Floodplain Vegetation Density and the Impact of Invasive Amorpha Fruticosa on Flood Conveyance. J. Environ. Geogr. 11, 3–12. doi:10.2478/jengeo-2018-0008
Pan, D., Gao, X., Dyck, M., Song, Y., Wu, P., and Zhao, X. (2017). Dynamics of Runoff and Sediment Trapping Performance of Vegetative Filter Strips: Run-On Experiments and Modeling. Sci. Total Environ. 593-594, 54–64. doi:10.1016/j.scitotenv.2017.03.158
Panwar, S., Khan, M. Y. A., and Chakrapani, G. J. (2016). Grain Size Characteristics and Provenance Determination of Sediment and Dissolved Load of Alaknanda River, Garhwal Himalaya, India. Environ. Earth Sci. 75, 91. doi:10.1007/s12665-015-4785-9
Pei, S., Jian, Z., Guo, Q., Ma, F., Qin, A., Zhao, Y., et al. (2018). Temporal and Spatial Variation and Risk Assessment of Soil Heavy Metal Concentrations for Water-Level-Fluctuating Zones of the Three Gorges Reservoir. J. Soils Sediments 18, 2924–2934. doi:10.1007/s11368-018-1966-7
Ran, Y., Wu, S., Zhu, K., Li, W., Liu, Z., and Huang, P. (2020). Soil Types Differentiated Their Responses of Aggregate Stability to Hydrological Stresses at the Riparian Zones of the Three Gorges Reservoir. J. Soils Sediments 20, 951–962. doi:10.1007/s11368-019-02410-7
Rhoads, B. (1989). Longitudinal Variations in the Size and Sorting of Bed Material along Six Arid-Region Mountain Streams. Catena Suppl. 14, 87–105.
Rice, S., and Church, M. (1998). Grain Size along Two Gravel-Bed Rivers: Statistical Variation, Spatial Pattern and Sedimentary Links. Earth Surf. Process. Landforms 23, 345–363. doi:10.1002/(sici)1096-9837(199804)23:4<345::aid-esp850>3.0.co;2-b
Schermelleh-Engel, K., Moosbrugger, H., and Müller, H. (2003). Evaluating the Fit of Structural Equation Models: Tests of Significance and Descriptive Goodness-Of-Fit Measures. Methods Psychol. Res. Online 8, 23–74.
Shi, D., Yang, Y., Xixi, L., and Liang, Y. (1991). An Analysis of Soil Erosion Rules and Sediment Producing Sources in the Region of Three-Gorge of the Yangtze River [J] [in chinese]. J. Soil Water Conservation 3, 9–21. doi:10.13870/j.cnki.stbcxb.1991.03.002
Shmilovitz, Y., Morin, E., Rinat, Y., Haviv, I., Carmi, G., Mushkin, A., et al. (2020). Linking Frequency of Rainstorms, Runoff Generation and Sediment Transport across Hyperarid Talus‐pediment Slopes. Earth Surf. Process. Landforms 45, 1644–1659. doi:10.1002/esp.4836
Su, X., Nilsson, C., Pilotto, F., Liu, S., Shi, S., and Zeng, B. (2017). Soil Erosion and Deposition in the New Shorelines of the Three Gorges Reservoir. Sci. Total Environ. 599-600, 1485–1492. doi:10.1016/j.scitotenv.2017.05.001
Tang, Q., Bao, Y., He, X., Fu, B., Collins, A. L., and Zhang, X. (2016). Flow Regulation Manipulates Contemporary Seasonal Sedimentary Dynamics in the Reservoir Fluctuation Zone of the Three Gorges Reservoir, China. Sci. Total Environ. 548-549, 410–420. doi:10.1016/j.scitotenv.2015.12.158
Tang, Q., Bao, Y., He, X., Zhou, H., Cao, Z., Gao, P., et al. (2014). Sedimentation and Associated Trace Metal Enrichment in the Riparian Zone of the Three Gorges Reservoir, China. Sci. Total Environ. 479-480, 258–266. doi:10.1016/j.scitotenv.2014.01.122
Tang, Q., Collins, A. L., Wen, A., He, X., Bao, Y., Yan, D., et al. (2018). Particle Size Differentiation Explains Flow Regulation Controls on Sediment Sorting in the Water-Level Fluctuation Zone of the Three Gorges Reservoir, China. Sci. Total Environ. 633, 1114–1125. doi:10.1016/j.scitotenv.2018.03.258
Wang, B.-y., Yan, D.-c., Wen, A.-b., and Chen, J.-c. (2016a). Influencing Factors of Sediment Deposition and Their Spatial Variability in Riparian Zone of the Three Gorges Reservoir, China. J. Mt. Sci. 13, 1387–1396. doi:10.1007/s11629-015-3806-1
Wang, B., Yan, D., Wen, A., and Chen, J. (2016b). Sediment Particle Size in Riparian Zone of the Three Gorges Reservoir and its Implication on Sources (In Chinese). Resour. Environ. Yangtze Basin 25, 1421–1429. doi:10.11870/cjlyzyyhj201609013
Wei, X., Han, L., Gao, B., Zhou, H., Lu, J., and Wan, X. (2016). Distribution, Bioavailability, and Potential Risk Assessment of the Metals in Tributary Sediments of Three Gorges Reservoir: the Impact of Water Impoundment. Ecol. Indicators 61, 667–675. doi:10.1016/j.ecolind.2015.10.018
Wu, J., Huang, J., Han, X., Gao, X., He, F., Jiang, M., et al. (2004). The Three Gorges Dam: an Ecological Perspective. Front. Ecol. Environ. 2, 241–248. doi:10.1890/1540-9295(2004)002[0241:ttgdae]2.0.co;2
Xinchen Wang, X., Zhang, H., Bertone, E., Stewart, R. A., and O’Halloran, K. (2020). Numerical Study of the Hydrodynamic and Sediment Transport Process in a Subtropical Water Reservoir: The Impacts of Storms and Winds. Environmental Modeling & Assessment, 1–18. doi:10.1007/s10666-020-09719-5
Yang, S. L., Xu, K. H., Milliman, J. D., Yang, H. F., and Wu, C. S. (2015). Decline of Yangtze River Water and Sediment Discharge: Impact from Natural and Anthropogenic Changes. Sci. Rep. 5, 12581. doi:10.1038/srep12581
Ye, C., Li, S., Zhang, Y., and Zhang, Q. (2011). Assessing Soil Heavy Metal Pollution in the Water-Level-Fluctuation Zone of the Three Gorges Reservoir, China. J. Hazard. Mat. 191 (1–3), 366–372. doi:10.1016/j.jhazmat.2011.04.090
Zhang, Q., Li, L., Wang, Y-G., Werner, A. D., Xin, P., Jiang, T., et al. (2012). Has the Three-Gorges Dam Made the Poyang Lake Wetlands Wetter and Drier? Geophys. Res. Lett. 39. doi:10.1029/2012gl053431
Keywords: particle size composition, water-level fluctuation, Three Gorges Dam, Yangtze River, sediment
Citation: Liu D, Nie Q, Xiong C, Yu Z, Lv Y, Zhang S, Cheng S, Lan B, Kumar A, Yu Z and Lin J (2022) Sediment Particle Size Composition in the Riparian Zone of the Three Gorges Reservoir. Front. Environ. Sci. 10:820700. doi: 10.3389/fenvs.2022.820700
Received: 23 November 2021; Accepted: 14 March 2022;
Published: 30 March 2022.
Edited by:
Carlo Camporeale, Politecnico di Torino, ItalyReviewed by:
Giuseppe Bombino, Mediterranea University of Reggio Calabria, ItalyMohd Yawar Ali Khan, King Abdulaziz University, Saudi Arabia
Copyright © 2022 Liu, Nie, Xiong, Yu, Lv, Zhang, Cheng, Lan, Kumar, Yu and Lin. This is an open-access article distributed under the terms of the Creative Commons Attribution License (CC BY). The use, distribution or reproduction in other forums is permitted, provided the original author(s) and the copyright owner(s) are credited and that the original publication in this journal is cited, in accordance with accepted academic practice. No use, distribution or reproduction is permitted which does not comply with these terms.
*Correspondence: Junjie Lin, anVuamllbGluQHNhbnhpYXUuZWR1LmNu