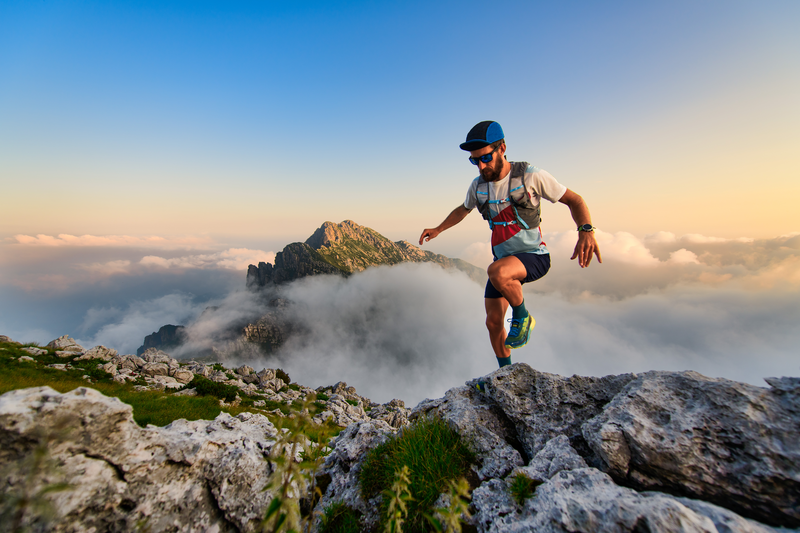
95% of researchers rate our articles as excellent or good
Learn more about the work of our research integrity team to safeguard the quality of each article we publish.
Find out more
REVIEW article
Front. Environ. Sci. , 11 April 2022
Sec. Toxicology, Pollution and the Environment
Volume 10 - 2022 | https://doi.org/10.3389/fenvs.2022.814590
This article is part of the Research Topic Analysis, Fate and Transformation of Emerging Contaminants in Soil and Sediment View all 7 articles
Sewage sludge (SS) is a biosolid that includes nutrients, organic matter, and a mixture of micropollutants and pathogens. Regarding its final disposal, several criteria should be met to avoid the dissemination of the included micropollutants in the environment. Hence, an adequate treatment prior to SS disposal is highly required. Solar drying is being acknowledged as a sustainable process of SS treatment, yet it is still unclear to what extent this technique is efficient. This review aimed to assess the impact of solar drying on the composition of SS from environmental and agronomic standpoints. Herein, we present the state-of-the-art with regard to solar drying efficiency in terms of water content reduction, DM increase, agronomic parameters evolvement, and micropollutant stabilization including pathogens, heavy metals (HMs), and organic micropollutants. The reviewed literature is mostly focused on two drying cycles: summer and winter, thus addressing the extreme conditions met within a year with respect to temperature. Under different climatic conditions, more than 80% of dry matter is reached during summer. In winter, the efficiency decreases to an average of 50% of DM. Negatively correlated to DM content, pathogen concentration in SS significantly decreased, while DM increased. Thus, more efficiency in terms of pathogen abatement is reported in summer than in winter (e.g., 96% against 60% during summer and winter, respectively, under semi-arid climate). The high reliance of solar drying efficiency on weather has been deduced in terms of DM content increase and pathogen removal. Where climatic conditions are not favorable for solar drying, hybrid design and liming are the highly recommended methods to remove pathogens from SS. A few studies on the fate of HMs in SS during solar drying concluded that solar drying does not involve any removal mechanisms. Changes in HM speciation in solar-dried sludge were reported highlighting a decrease in their mobility. As for organic micropollutants (PAHs and antibiotics), only their occurrence in SS is reported in the literature, and their behavior during the solar drying process is still not addressed. This review allowed concluding the following: 1) solar drying is a sustainable, relevant process for SS handing in terms of volume reduction and pathogen removal, particularly in semi-arid regions; 2) solar drying does not lower the SS agronomic value and does not remove HMs, but under semi-arid climate, it changes HM speciation and reduces their mobility. The gap in research regarding organic micropollutant and heavy metal behavior during SS solar drying has been emphasized as a way forward for research within this topic. Hence, more research is required to help stakeholders decide on the feasibility of an agricultural disposal of solar-dried sludge.
1) Sewage sludge presents not only an important agronomic value but also a high content of pathogens, heavy metals, and organic micropollutants;
2) solar drying of sludge is involved in SS management for water removal, pathogen abatement, and organic and inorganic micropollutant stabilization;
3) impact of solar drying on water and pathogen removal is the most addressed aspect in the literature;
4) the total content of metals in sludge and the changes in their speciation are explored in this review;
5) the fate of organic micropollutants in SS during solar drying is still not addressed in the literature.
Human activities lead to increase in water consumption worldwide. Hence, an increase in the wastewater streams evacuated toward the treatment units is mainly observed. Wastewater treatment plants (WWTPs) produce treated wastewater as a valuable resource that can be reused in agriculture and also as industrial processing water. Nevertheless, it also generates different by-products that could affect the environment in case of an inadequate management. Sewage sludge (SS) is one of the most important by-products generated by wastewater treatment processes. Also known as a biosolid, it can be defined as the solid or semi-solid leftover after the treatment of wastewater (Metcalf and Eddy, 1991). It is a complicated and heterogeneous substrate containing organic and inorganic materials as well as microorganisms. China, the European Union, and the United States are the largest producers of SS, with 9.18 million tons of sludge (dry solid) in 2009, 11.7 million tons in 2010, and more than 8 million tons of dry solids in 2010 (Bennamoun et al., 2013). In Morocco, SS production of 300,000 tons is expected by 2025 (Phénixa, 2010). SS treatment represents almost 50% of the whole cost dedicated for WWTP construction and operation (LeBlanc et al., 2008). Despite the adopted treatments at the WWTP level, final disposal of SS remains a problem to be addressed and an opportunity of valorization to be seized.
In a global extent, the methods for final disposal are landfilling, incineration, and agricultural recycling. Nowadays, many restrictions are present regarding SS landfilling, and thus, the amount of landfilled sludge is decreasing. Indeed, from 2010 to 2020, the percentage of landfilled sludge decreased from 11 to 4% in the European Union (Raheem et al., 2018) and it is expected to continue to encourage phasing out the landfilling of all organic residuals including SS. As for incineration, despite it reduces 90% of SS volume with the removal of pathogenic organisms (Wu et al., 2016), it becomes an implausible alternative since it ends with several issues such as greenhouse gas emissions and other hazardous materials (dioxins, furans, and HMs) (Fytili and Zabaniotou, 2008). However, in the United States, 15% of the produced SS is still incinerated, despite the high costs involved, especially within the huge restrictive standards set in the climate change framework (Peccia and Westerho, 2015). On the other hand, the agricultural use of SS is gaining interest worldwide. Indeed, awareness is increasing about SS not only as an organic amendment and nutrient source for plants but also for improving soil health. In the European context, the application of SS for arable land increased from 43% in 2010 to 45% in 2020 (Samolada and Zabaniotou, 2014). In the United States, beneficial application of SS on agricultural land including cropland, rangeland, and pastures is the major ultimate use. According to Kalogo (2008) and Peccia and Westerho (2015), 55% of the produced SS is applied to arable land with N and P contents of 3.4% and 2.3%, respectively (Raheem et al., 2018).
In Morocco, despite the existing treatment of SS at the WWTP scale, recycling of SS into agricultural soils remains limited, and only a few large-scale experiments have been tested. Some pilot trials involving composted SS with green waste demonstrated a significant added value of SS in terms of crop yield enhancement, while the quality of the obtained compost was in accordance with the European standard (Soudi, 2001). However, SS landfilling is still common practice in Morocco, thus presenting olfactory nuisances, overproduction of lixiviate, and many other environmental issues. Despite being the most environmentally friendly alternative for the final disposal of SS, recycling in agricultural soils is still a controversial issue since it contains, besides organic matter and plant nutrients, pathogens, organic, and inorganic micropollutants that could affect the soil–plant system and then the food chain.
Within all the previous alternatives of SS final disposal, drying is commonly used. This step is generally aimed at reducing the volume of sludge (due to its high water content) and sanitizing. The different ways of drying are natural drying, mechanical drying, thermal drying, and other hybrid drying installations. Solar drying, a process that operates under greenhouse plants, is widely used to accelerate the water evaporation rate exploiting the artificial greenhouse effect and avoiding the equilibrium of vapor pressure between SS and air by controlled indoor air ventilation (Mathioudakis et al., 2013). This process is more involved in SS management fields when compared to other more expensive alternatives such as thermal drying that exhibits strong energy needs mostly provided by fossil fuels (Collard et al., 2017). In addition to the high costs involved in thermal drying, it weakens SS organic matter which is observed by an increase in lipids with a decrease in humus (Collard et al., 2017). Contrariwise, with a low energy demand, solar drying ends with a strong increase in humic-like substances parallel to a decrease in lipids, which corresponds to a significant complexification of the OM. Thus, solar drying tends to stabilize SS and produces suitable amendment which is favorable to organic carbon sequestration in soils, while thermal drying leads to produce a sludge-based fertilizer (mineral) rather than an organic amendment (Soriano-Disla et al., 2010; Collard et al., 2017). Solar dryers are implemented worldwide for large- and medium-sized WWTPs (Brison et al., 2012; Bennamoun et al., 2017). In fact, more than 70 solar drying installations were built in the United States, the European Union, and Australia in 2006 (Shanahan et al., 2010). In Morocco, where solar radiation is characterized by an average of 3,000 h/year of sunshine, the solar drying process is increasingly adopted in WWTPs. For instance, 28 solar dryers were built on an extended surface (40.320 m2) in order to treat 75.000 tons/year of SS produced by the WWTP in Marrakech city. Such a solar drying platform is considered as the largest one in the world (Thermo-system, 2018). Research activity in the solar drying process of SS has been triggered in 2000. However, there is a slow positive evolution of scientific publications in this field (Figure 1A), and the main contributing countries are the United States and China (Figure 1B). The number of publications increased from six in 2006 to only 25 publications in 2020 with a focus on drying kinetics.
FIGURE 1. (A) Evolution pattern of scientific publication on solar drying of SS from 2000 to 2021. (B) Main countries contributing in scientific production related to solar drying of SS. The keywords used in Web of Science are solar drying, sludge, pathogens, and micropollutants.
Based on temperature as a key parameter, the solar drying process has been reported for a significant efficiency in terms of water removal and thus SS volume reduction (Lima et al., 2012; Bennamoun et al., 2013; Collard et al., 2017; An-nori et al., 2020b). For instance, An-nori et al. (2020a) reported daily maxima ranging between 45 and 68°C during a summer drying cycle with semi-arid climate inside the greenhouse plant dedicated to SS drying. SS subjected to such operating conditions may reach an average dry matter content of 90%. This water removal technique may be very detrimental for pathogens and act as a removal factor, as reported by Manga et al. (2016). In addition to this indirect impact on pathogens, the high temperatures inside the solar plants have been demonstrated to be deleterious for fecal contamination indicators (Shanahan et al., 2010; Paluszak et al., 2012; An-nori et al., 2020b). Moreover, these high temperatures have been reported to directly act on parasites in SS by increasing their sensitivity to many stressors (eg., high pH), which comes out with inactive helminth eggs (Barrett, 1976; Pecson et al., 2007; Hafidi et al., 2018). Over all the SS physicochemical characteristics, only a decrease in moisture content and a slight decrease in pH and electrical conductivity are highlighted in the literature, while the organic matter content and nutrients are not significantly affected. This leads to assume that the agronomic value of solar-dried sludge remains significant, despite a long resting period inside the greenhouse plants. However, the changes in physicochemical properties of SS lead to raise a research hypothesis which says that an additional impact of solar drying on organic micropollutants and heavy metals is plausible.
This review is engaged to examine this research hypothesis throughout the recent literature (last 20 years). Thus, it aimed to assess the physicochemical and microbiological quality of SS and the organic and inorganic micropollutant occurrence. Then an emphasis is placed on reviewing the impact of the solar drying process on water content, agronomic parameters, and the fate of micropollutants, mainly heavy metals, HAPs, and antibiotics, within different operating conditions. Thus, this review will be an important contribution to establish a state-of-the-art regarding solar drying process and its usefulness in SS management fields mainly before its recycling in agriculture. Henceforth, the findings of this review will guide readers to assess the efficiency of such a process as a sanitizing and stabilizing treatment of SS and to point out the gaps in the literature to be filled through further research studies in the field.
Sewage sludge is characterized by a high content of organic and inorganic substances, including microbial biomass, pathogens, nutrients N and P, and metals. Depending mainly on the raw wastewater quality and SS processing, SS is known for its very heterogeneous physicochemical composition and high water content. In this review, the composition of SS is reviewed focusing on parameters that are likely to be affected by the solar drying process.
SS is known for its high water content that can exceed more than 90% (Gao et al., 2020). Before using SS, for either soil disposal or energy recovery, this moisture content has to be reduced to minimum levels using several available drying techniques. In fact, the separation of water from sludge is still a major problem in wastewater treatment plants, and it depends on water distribution in SS. Thus, this latter constitutes a decision tool for SS dewatering and drying techniques. The method of the drying test originally proposed by Vesilind (1994) allowed assessing sludge drying behavior, which led to identify water distribution in SS. By this method, a typical drying curve (Figure 2) can be divided into a constant rate period, first falling rate period, second falling rate period, and the equilibrium stage. The moisture removed during the constant rate period, the first falling rate period, and the second falling rate period are regarded as free water, interstitial water, and surface water, respectively, while the residual moisture content in the equilibrium stage refers to water of hydration. Figure 2 indicates the three transition zones of physical characteristics taking place when removing each water fraction (Lowe, 1995). The first zone is the wet zone wherein sludge still contains free water; sludge in this zone flows freely and spreads easily. In the second zone (sticky zone), interstitial water is removed, and the sludge is pasty and hardly flows, while in the third zone where bound water (vicinal and water of hydration) is removed, SS is crumbly and mixes easily. For more illustration of the aforementioned information, the distribution of water around and within sludge particles is concretely modeled in Figure 3. Free water is not associated with and not influenced by the suspended solid particles. Vicinal water represents the water molecules immediately adjacent to the solid surface of SS. Its properties differ from bulk water due to structural differences induced by the proximity to the surface of SS particles (Drost-Hansen, 1981). Thus, SS with a large specific surface exhibits a high content of vicinal water, as it is the case of activated SS (Heukelekian et al., 1956). Water of hydration is defined as chemically bound water to the particles and removable only by the expenditure of thermal energy. Table 1 illustrates the content of water in SS at different treatment stages in WWTP. Raw sludge constitutes all the previously mentioned water fractions (95–99%). Settling removes free water from SS, which comes out as thickened sludge with 90–95% water. Using mechanical dewatering techniques is necessary to remove interstitial water (Taylor et al., 2017), and this results in a mechanically dewatered sludge with 68–80% water. The abundance of vicinal water in activated SS explains the fact that dewatering techniques such as belt filters and centrifugation involved at the WWTP level lead to SS with considerable moisture content, whereas treatments involving high temperatures such as solar and thermal drying can bring moisture below 6% (Mathioudakis et al., 2009). Hence, using drying techniques is necessary to enhance water removal and help better manage the transport and final disposal of SS. In this review, water removal is assessed as a response of the solar drying technique, and the associated chemical and microbiological parameters are reviewed.
FIGURE 2. Drying behavior of sewage sludge obtained from drying experiments (adapted from Lowe (1995).
FIGURE 3. Water distribution in SS according to Vesilind (1994).
TABLE 1. ontent of water in sewage sludge at different treatment stages according to different authors.
The physicochemical composition of SS depends on many factors, including wastewater origin, weather conditions, adopted process for water treatment at the WWTP level, and applicable conditioning process on residual sludge (Werther and Ogada 1999; Jarde et al., 2003). The concentration of organic matter varies from 30% to 88% (Metcalf and Eddy, 1991). Organic matter consists of particulate matter removed by gravity in primary sludge, lipids (6–19% of organic matter), polysaccharides, proteins, amino acids (up to 33% of organic matter), lignin, metabolism products, and microbial bodies from biological treatments (Inoue et al., 1996; Jarde et al., 2003). Plant nutrients are often present in sludge including nitrogen, potassium, and phosphorus. The concentration of such fertilizing elements varies highly, as reported in Table 2. According to the sludge type, OM can vary from 30% to 88% (Metcalf and Eddy, 1991). For instance, primary sludge, resulting from simple settling at the beginning of SS processing, contains mostly mineral particles rather than organic materials, thus explaining an average of 30% of OM. Nevertheless, secondary SS resulting after the biological treatment of wastewater is mostly composed of extracellular polymeric substance (EPS) (Mininni et al., 2004); consequently, more than 80% of OM is often measured in secondary SS (Metcalf and Eddy, 1995; Mininni et al., 2004). Nitrogen is also reported in the literature to be highly variable (from 0.8% to 11%), and this is attributed to the presence or absence of the nitrifying and denitrifying process at the biological treatment stage in the WWTP. Both the existing inorganic nitrogen and the one resulting from mineralization of the organic part can contribute to satisfy plant’s nitrogen requirement (Zebarth et al., 2009). The content of SS in terms of OM and other nutrients shown in Table 2 allows assuming the suitability of SS as an organic amendment. It is worth mentioning that secondary sludge, if treated separately from primary sludge, is more relevant for agricultural recycling since it has high content of OM, nitrogen, and phosphorus and less hazardous substances compared to primary SS (Khan and Ongerth, 2001; Mininni et al., 2004).
TABLE 2. Physicochemical properties of SS (Pedersen, 1981; Metcalf and Eddy, 1995; Rulkens, 2008).
In addition to feeding the plants, Soriano-Disla et al. (2010) reported that SS recycling into agricultural soils contributes to carbon sequestration and enhances soil’s resilience. An-nori et al. (2020b) reported that applying one ton of SDS allows recycling at least 350 kg of carbon into soil. The included organic matter in sludge would enhance the clay–humus complex formulation and improve the soil structure, which would increase water retention and decrease vulnerability to erosion. This seems very relevant for the exhausted soils within the African context. For instance, in Morocco, the soil structure is being continuously degraded because of erosion and mining (Mrabet et al., 2000). The mean value of OM content is below 2%, and the total annual loss of the agricultural soil is estimated to be 100 million tons (FAO, 2015). Henceforth, using SS as an alternative in agriculture could be an appealing solution to boost soil’s health. Despite these agronomic virtues, SS may include a mixture of micropollutants such as pathogens, HMs, and some other organic pollutants. Therefore, the direct application of sludge in the soil must be controlled to avoid the potential dissemination of those hazardous materials in the environment.
HMs are defined as metallic elements that have a relatively high density compared to water (Kim and Fergusson, 1993). They are transition metals which have atomic masses more than 0.002 kg, weight about 5 N/m3, and density more than 5 g/cm3 (Singh et al., 2009). HMs are naturally occurring elements that include essential (e.g., Cu, Fe, Ni, Cr, Se, As, Co, and Zn) and non-essential metals (Cd, Hg, and Pb). Essential HMs are part of essential micronutrients for plants and play vital roles in several cellular reactions, including electron transfer, enzyme activation, redox reactions, and pigments synthesis (Babula et al., 2009; Fageria et al., 2009; Chaffai and Koyama, 2011). However, non-essential metals do not intervene in any biological reaction and cause toxic impacts even at low concentrations (Torres et al., 2008). When sewage sludge is applied to agricultural soils, HMs may pose a serious problem. For instance, cadmium (Cd) released from SS after long-term disposal can accumulate in plant tissues such as roots and leaves and probably transmit to humans via the food chain, resulting in a potential decline of the human immune system. Elemental mercury (Hg) has been found to vaporize from amended soil with SS under the effect of solar radiation. If consumed, Hg is known to be harmful to the human central nervous system. Many activities exacerbate the presence of HMs at the WWTP level, especially in the sludge. For instance, in addition to its geochemical source, tanning industry is a major contributor in terms of the chromium content in SS generated in the WWTP of Marrakech (Table 3). Indeed, all the used chromium salts in the tanning industry (e.g., chromium sulfate) are discharged into WWTP and they end up in sludge (EL Fels et al., 2015; Shahid et al., 2017; An-nori et al., 2020a). The presence of HMs in SS, rather than treated wastewater, is due to their adsorption potential and their affinity toward solid fraction. It is worth mentioning that primary sludge is more polluted than secondary sludge regarding heavy metals. In fact, due to their low water solubility and lipophilic properties, these compounds are associated with the particulate sewage fraction and are therefore principally removed in the primary settling tank (Angelidaki and Ahring, 1999). Depending on the wastewater origin, HMs could be present in SS in different concentrations. The occurrence of HMs in SS in the Moroccan context is rarely addressed. Some studies were engaged to assess the total content of metals in raw, composted, and solar-dried sludge (Table 3).
TABLE 3. Concentration (mg/Kg) of heavy metals in different types of sludge. S1 and S2: raw biological sludge. S3: composted sludge and S4: solar-dried sludge.
When intended for recycling in the agricultural soils, the dissemination of the included HMs in the environment becomes a major concern. In fact, it has been widely demonstrated that speciation, rather than the total content of HMs, is a reliable indicator to assess their availability for living organisms (Lund and Chang, 1982; Jamali et al., 2009; He et al., 2013). Correspondingly, speciation assessment of HMs can be performed by fractioning that consists of determining the amount of metal ions bound by the individual fractions using sequential extraction (Hanay et al., 2008; He et al., 2013). As a result of HM’s speciation analysis, Lasheen and Ammar (2009) showed that Mn, Ni, and Zn were present in exchangeable carbonate and Fe/Mn oxides (the most mobile fractions), while Cd, Cu, Cr, and Fe showed more affinity toward organic and sulfide (exhibiting some degree of mobility) and the residual form (stable and inert phases). Furthermore, HM’s toxicity relies also on the aqueous speciation, which refers to the soluble and insoluble forms. For instance, chromium occurs in different valence states. The trivalent chromite [Cr (III)] and hexavalent chromate [Cr (VI)] are more stable and predominant states in the natural environment (Elzinga and Cirmo, 2010). In addition, Cr (VI) is known to be highly mobile in soil and more toxic than Cr (III) to living organisms including plants (Shahid et al., 2017).
Several investigations demonstrated that HM-stressed plant shows some abnormalities in terms of development and growth. Alterations in cellular regulating mechanisms and gene regulation are widely highlighted in the literature (Hussain et al., 2004; Chaffai and Koyama, 2011; Choppala et al., 2014). HMs, especially the non-essential ones, slow down the physiological reactions through the alteration of biomolecules and regulatory proteins or sometimes through substitution of crucial elements (Sarwar et al., 2010). Moreover, HMs may affect the antioxidant defense system of plants by generating reactive oxygen species (ROS) (Sarwar et al., 2010; Chaffai and Koyama, 2011; Choppala et al., 2014). Also, It has been reported that HMs generate free radicals in cells which induce plant toxicity (Singh et al., 2009). Metals may also induce water stress by affecting stomatal and transpiration activities and the water content in leaves while decreasing the size and the quantity of xylem tissue, chloroplast, and cell (Singh et al., 2009). Table 4 summarizes the toxicity of some studied HMs toward some crops. Being more vigilant in terms of limiting the dissemination of HMs in the agricultural soils, the European commission has set three types of metal limits: 1) the maximum HM content in sludge for agriculture use; 2) the maximum HM content in treated sludge for soil application; and 3) the 10-year average rate of metals accumulated during successive application of sludge (Table 5).
TABLE 5. Maximum values of metal content in SS used in agriculture, their rate of application, and concentration in sludge-treated soils in the Commission of the European Communities directive (Commission of the European Communities et al., 2009).
To limit the dissemination of toxic metals in soil from SS, many countries have established standards for sludge recycling in agriculture (Figure 4). The standards were set based on the concentration of metals in sludge, the total content of metal that can be added and often how frequently this can be applied, and finally the concentration of metals in soil which can be exacerbated after sludge application.
FIGURE 4. Maximum metal concentrations in SS for an agricultural use (mglKg) [Data are derived from Agence Nationale pour la Rkcupkration et 1’∼limination des Dkchets, 1988; UK Statutory Instruments (1989); Berg et al. (1993); U.S. Environmental Protection Agency (1993)]; (Page, 1994).
Sewage sludge contains both saprophytes and pathogens microorganisms. Some of them are essential to ensure the secondary treatment in the activated sludge process and other biological treatment methods of wastewater. The main groups of pathogenic organisms existing in SS are the enteric bacteria, parasites, viruses, protozoa, and fungi (Fijalkowski et al., 2017). These microorganisms end up in sludge via adsorption on particles and then settling during wastewater treatment. Table 6 summarizes the most abundant species in SS. The occurrence of these species is closely related to wastewater characteristics, operational parameters of sludge treatment at the WWTP, and geographic location (Goberna et al., 2018). For instance, industrial wastewater, characterized by severe abiotic conditions (mainly pH, T, and HM content), has lower diversity of microorganisms in sludge than domestic wastewater (Ju et al., 2014). However, the processing of SS at the WWTP level intervenes in its microbial composition (Lloret et al., 2016). For instance, anaerobic digestion is reported in the literature to lower down the pathogenic microorganism load, although some of them can survive or regrow in the digested product during subsequent treatment (Bagge et al., 2005; Pepper et al., 2006; Holm-Nielsen et al., 2009).
Due to the limitations related to identifying and quantifying bacteria exhaustively through culture-based methods, regulations and monitoring schemes typically focus on bacterial indicators. In fact, while investigating the efficiency of a process to stabilize pathogenic microorganisms in SS, some indicators are commonly used throughout the literature. Indeed, fecal coliform bacteria and Enterococci are common indicators of enteric bacterial pathogens, whereas F+ coliphage has been used as an indicator of enteric virus. It is worth mentioning that due to the omnipresence of antibiotics in the environment in general and in SS in particular (Ezzariai et al., 2018), sludge may contain some resistant bacterial strains such as multiresistant Escherichia coli (Reinthaler et al., 2013). The fecal coliforms are widely known to induce adverse damage regarding public health. For instance, Escherichia coli produces verotoxins, which have been associated with disease outbreaks of diarrhea, hemorrhagic colitis, and hemolytic uremic syndrome in humans (Pradell et al., 2000).
For an exhaustive screening of pathogens in SS, metagenome analysis constitutes a qualitative approach that enables pathogen identification and provides a potential solution to the challenges associated with the traditional culture-based methods mainly for viruses’ identification. Based on such a novel approach, a wide occurrence of newly emerging respiratory viruses (e.g., coronaviruses) and the relative minor abundance of Enteroviruses in SS have been reported (Bibby and Peccia, 2013). Interestingly, several updated research in the circumstances of the latest outbreak of COVID-19, declared as a pandemic by the World Health Organization (WHO), reported the presence of SARS-CoV-2 in SS in many countries (Amoah et al., 2020; Yang et al., 2020; Balboa et al., 2021), starting in the Netherlands (Medema et al., 2020). This probably means that handling raw SS may lead to the dissemination of the virus.
Parasites are also among the most abundant pathogenic microorganisms in SS. In fact, they are distinguished from the rest of pathogens by their recalcitrance in the environment (Capizzi-Banas et al., 2004). Three groups of helminth eggs in both raw wastewater and SS including nematodes (e.g., Ascaris lumbricoides, Trichuris trichiura, and Toxocara spp.), cestodes (e.g., Taenia solium and Taenia saginata), and trematodes (e.g., Schistosoma spp.) were found with which high sanitary risks are associated (Scott, 2013). Initially existing in wastewater, helminth eggs are usually converted to sludge during wastewater processing, mainly while settling, since their density exceeds that of water (1.056–1.237) (Gantzer et al., 2001; Koné et al., 2007). Several authors reported that SS has important loads of parasites belonging to diverse taxa (Jiménez, 2007; Koné et al., 2007; Navarro and Jiménez, 2011; Rocha et al., 2016; El Fels et al., 2019). However, Ascaris’ eggs remain the most prevalent compared with other identified parasites.
In Morocco, the occurrence of such pathogens is addressed in wastewater rather than SS mainly when treated wastewater is intended for agriculture recycling. Shaoua et al. (2018) demonstrated the effectiveness of the activated sludge process to remove helminths from treated wastewater under semi-arid climate. Idrissi et al. (2020) reported that the lagooning process does not ensure a total purification of wastewater in terms of helminth eggs concentration. Regardless the effectiveness of wastewater treatment in terms of parasites removal, the conversion of helminth eggs in wastewater to SS is often reported (Hajjami et al., 2013; Chaoua et al., 2018, Idrissi et al., 2020). Several studies investigated the occurrence of helminth eggs in SS belonging to different processes of WWTPs such as lagooning, activated sludge, and waste stabilization ponds (Table 7). El Hayany et al. (2018) and Hafidi et al. (2018) reported the prevalence of Ascaris’ eggs, which is the most resistant species among nematodes, in SS sampled from an activated sludge process-based WWTP. The load of Ascaris’ eggs in raw fresh sludge is significantly higher than the load recorded in dehydrated sludge (from 6 to 8 eggs/g and from of 0–3 eggs/g, respectively). Regarding the cestodes group, Khadra et al. (2019a) recorded 7.6 eggs/g of Hymenolepis nana in fresh SS, while only 0.91 eggs/g was revealed in thickened SS. The prevalence of trematodes is lower than those of nematodes and cestodes. For instance, El Hayany et al. (2018) reported a concentration less than 1 eggs/g of Schistosoma spp. in both fresh and dehydrated SS sampled from a lagooning-based WWTP in a semi-arid climate. Overall, nematodes are the most prevailing species in SS. This is likely attributed to Moroccan’s culinary habits, mainly the consumption of well-cooked meat, which does not favor the transmission of cestodes and trematodes (Mrabet and Agoumi 1991). Overall, Ascaris’s eggs are most prevalent, among the nematodes, in SS generated by a different process of wastewater treatment. Indeed, they have been frequently reported as the most common helminth eggs in domestic wastewater in Morocco, Mexico, Brazil, and Colombia (Jiménez, 2007). The abundance of Ascaris’ eggs in the environment has been related to its high egg production and its important survival capacity even under severe environmental conditions. According to Feachem et al. (1983), female Ascaris spp. lays about 200,000 eggs per day compared to Trichuris spp., which produces between 2000 and 10,000 eggs per day. Data in Table 7 also emphasize that sludge belonging to natural lagoons are more concentrated in helminth eggs than that in the activated sludge process. This is likely due to the residence time of the sludge which is shorter in the activated sludge process (2–5 days) than in the lagooning (7 years). This is substantiating that settling for a long period in the lagoons leads to an enrichment of sludge in terms of helminth eggs content (El Hayany et al., 2018). The existing literature on helminth eggs’ occurrence in sludge also demonstrated that dewatering techniques lead to a decrease in the load of helminth eggs in SS. For instance, dewatering of SS using drying beds allowed a decrease of almost 40% of the total helminth eggs, but the final load still did not meet the standards for agricultural recycling (El Hayany et al., 2018). Based on the epidemiological risks, the WHO (2006) recommended a threshold of 1 helminth egg/g (DM). The French legislation (French official Journal, 1998) considers sludge treatment as efficient if the final product contains less than 3 viable helminth eggs/10 g (DM). The U.S. Environmental Protection Agency (US Epa Protocol, 1999) defines treated sludge as “Class A biosolids” when it contains 1 helminth egg/4 g (DM). In the Moroccan context, there is a lack of legislation regarding helminths in SS for agricultural application; a situation that underlines the necessity to attribute more interest to parasites in SS and national standards development.
Overall, despite the existing studies on helminth eggs occurrence in sludge in the Moroccan context, more investigations are required in order to cover several geographical areas to address the prevalence of parasites in sludge. More research studies should be conducted to fill the gap in terms of helminth eggs diagnosis in SS. In addition, investigations on solar-dried sludge in terms of its content in helminth eggs should be triggered involving different climate and geographic conditions, especially when the sludge is intended to be recycled in agriculture.
Due to human and industrial activities, SS may contain persistent organic micropollutants such as polycyclic aromatic hydrocarbons (PAHs), polychlorobiphenyls, and nonylphenols (Planas et al., 2005; Amir et al., 2005). Dubey et al. (2021) reviewed the occurrence of a cocktail of organic micropollutants including the recently emerging ones. According to the U.S. Geological Survey (USGS, 2008), emerging contaminants are defined as “any synthetic or naturally occurring chemical or any microorganism that is not commonly monitored or regulated in the environment with potentially known or suspected adverse ecological and human health effects”. Emerging contaminants such as antibiotics, personal care products, drugs, and veterinary products are quantified in SS, and their concentrations vary from ng/kg to mg/kg in the stabilized SS (Tavazzi et al., 2012; Ezzariai et al., 2018). It is widely demonstrated in the literature that such hazardous organic compounds are commonly removed from wastewater via biodegradation and sorption mechanisms, resulting in sewage sludge (Aemig et al., 2019). Indeed, sorption mechanisms of hydrophobic organic micropollutants are due to the surface area of both live and dead biomass (0.8–1.7 m2/g) (Wang et al., 1993; Rogers, 1996; Tavazzi et al., 2012).
Among organic micropollutants, PAHs are the most often addressed in the literature due to their mutagenic and carcinogenic behavior. Their omnipresence in the environment is due to their multiple sources. Not intentionally produced by human activities, the PAHs are by-products of incomplete combustions of organic matter (Rogers, 1996; Abad et al., 2005). Additionally, some natural phenomena could exacerbate their spread in the environment (wildfire, eruptions, etc.). PAHs are hydrophobic in nature and can easily become unavailable for degradation in sewage systems by adsorption onto solid particles during the biological treatment of wastewaters explaining their biological recalcitrance (Wild et al., 1990; Amir et al., 2008). Sorption of PAHs increases with an increase in molecular weight, hydrophobicity, phenolic, and aromatic compound content in the dissolved/colloidal matter (Barret et al., 2010). Several investigations demonstrated that 95% of PAHs can be removed from wastewater and then converted to SS (Fijalkowski et al., 2017; Dubey et al., 2021). Indeed, the range of total PAHs detected in wastewater and SS is from 0.002 to 20 ppm (Gawlik 2012; Fijalkowski et al., 2017). In the context of Morocco, PAHs occurrence in SS is rarely addressed. El Hammoudani and Dimane (2021) have monitored the occurrence of PAHs during sludge treatment including thickening, mechanical dewatering, and chemical stabilization (lime addition) in an activated sludge-based WWTP. The studied PAHs ranged between the quantification limit and 0.25 mg/kg in thickened sludge, while a maximum of 0.10 mg/kg has been recorded in chemically stabilized dewatered sludge. The highest observed concentrations were recorded for naphthalene, pyrene, and benzo (g, h, i) perylene. In the Moroccan context where recycling SS in agriculture is for an important relevance, a lack of a database gathering such organic micropollutants in sludge and their behavior under sludge treatments is observed. This gap incites to trigger more research in this field. In addition to the PAHs, the prevalence of antibiotics constitutes a major concern when the application of raw/treated sludge on agricultural soils is intended. Over the past decades, the increasing use of antibiotics in infection therapies and livestock production has resulted in a significant accumulation of these drugs and their metabolites in SS (Chee-Sanford et al., 2009; Yang et al., 2018). These compounds end up in SS with concentrations varying between few nanograms and 100 mg kg−1 (dry matter). The four main families (tetracyclines, sulfonamides, macrolides, and fluoroquinolones) have been quantified in raw sludge. Ezzariai et al. (2018) reported concentrations superior to 1,000 μg kg−1 dry matter of ciprofloxacin, norfloxacin, and tylosine, more than 10 μg kg−1 dry matter of tetracyclines and oxytetracycline, and lower concentrations of macrolides sulfonamides (more than 10 μg kg−1 dry matter). It is worth noticing that antibiotics are widely investigated in raw sludge and composted sludge when intended to be recycled in agriculture but rarely in solar-dried sludge. In addition, antibiotics in raw and composted sludge have been also addressed in terms of dissemination of antimicrobial resistance which lowers the antibiotics efficiency and then threatens public health (Chen et al., 2007). Antibiotics in sludge, when applied to agricultural soils, may induce toxicity to plants. In fact, antibiotics (e.g., quinolones and fluoroquinolones) in composted sludge demonstrated a high genotoxic effect on Vicia faba root tip even at low concentrations (Khadra et al., 2019b; Khadra et al., 2019c).
Solar dryers with a greenhouse structure are increasingly used. In Europe, many solar dryers, mainly in Germany, France, Austria, Turkey, and Poland, were constructed (Bennamoun 2012). The drying process can be carried out in open, semi-open, or covered tunnel greenhouses. Open greenhouses are much criticized in terms of water removal efficiency, uncontrolled odors, and greenhouse gases emission. To avoid these shortcomings, covered greenhouses are introduced as an efficient option for sludge drying (Bennamoun, 2012).
The general design of solar dryers consists of a greenhouse made with a transparent material and a floor where sludge is disposed in thick layers. To avoid fermentation problems, the thickness of the layer should not exceed 40 cm. Furthermore, fans and ventilations are generally used in order to regenerate the air inside the greenhouse, thus evacuating the humidified air. In some systems, a robot is used to mix and aerate the sludge, thus renewing the surface exchange and avoiding fermentation and crust formation (Kamil Salihoglu et al., 2007; Bennamoun, 2012; Bennamoun et al., 2013). Sensors are placed in the greenhouse in order to ensure a real time control of the key parameters involved in the drying process, mainly temperature and relative humidity (Figure 5).
FIGURE 5. Structure of a covered solar dryer (Kamil Salihoglu et al., 2007).
The effectiveness of solar dryers in terms of water removal from SS has been widely investigated using mechanically dewatered SS. This is to say that this paragraph deals with the impact of solar drying on vicinal and chemically bound water that persisted after dewatering. As described in Table 8, water removal depends wholly on the climatic conditions and more particularly solar radiations and temperature. Due to the greenhouse effect, an average of 80% of dry matter content is reached in summer in different geographical contexts under different climates. For instance, under semi-arid climate, SS sampled from the WWTP of Marrakech reached at least 90% DM content in summer after 2 weeks of drying (An-nori et al., 2020a). A study conducted in Poland (continental climate) stated that SS reached 86% of DM in summer (Paluszak et al., 2012). Autumn and spring are also favorable for solar drying of SS (Table 8). Indeed, the reached DM content during these two drying cycles is comparable well to summer season in terms of water removal. On the other hand, lower efficiency in terms of water removal has been reported in winter in some European countries and on average less than 50% DM content is reached (Bux et al., 2001; Paluszak et al., 2012). However, in the case of semi-arid climate, the solar drying process is efficient in both winter and summer (Belloulid et al., 2017).
The existing data regarding solar drying efficiency in terms of water removal demonstrated a significant removal of vicinal water and the chemically bound water, especially where climate is favorable (e.g., semi-arid climate). This is substantiating the usefulness of such a process in SS management after the mechanical dewatering techniques. The shortcomings met during winter could be fixed by involving further treatments such as liming that is known to increase temperature and then boost water removal.
The solar drying process decreased pathogen content, while high temperatures are recorded. In fact, when the water activity index decreases, the microbiological growth is inhibited (Paluszak et al., 2012). The fate of pathogens during solar drying has been rarely addressed. Throughout the literature, only seven studies were conducted since 2001 and only one study treated the removal of helminth eggs from SS during the solar drying process (Table 8). The revised literature used in this review includes both technical and pilot-scale solar dryers in order to assess the impact of solar drying on pathogen content in SS. Most of the existing studies involved two drying cycles including summer and winter, as these two seasons refer to the extreme temperatures involved in the solar drying process. The experiments conducted by Paluszak et al. (2012) in the north of Poland involving a hybrid system, using the solar effect and the heating floor system, allowed to assess its capacity to deal with the limitations of solar drying during winter. Mechanically dewatered sludge (using belt filters and centrifugation) was mostly used to conduct the solar drying process. Only one reported study investigated the impact of the solar drying process on limed dewatered SS. The dry matter content at the beginning of solar drying varies between 15 and 30% according to the process involved in mechanical dewatering. Regardless of the initial moisture content, the DM by the end of solar drying reached an average of 80% in summer and almost 40% in winter. As it is difficult to exhaustively assess the concentration of pathogenic microorganisms in SS after the solar drying process, total coliform and fecal coliform are mostly studied in the literature as indicators of contamination in sludge and among the criteria to assess the sanitizing effect of sludge treatment. Indeed, the pathogens content before and after solar drying is expressed in CFU and MPM for fecal contamination indicators and in egg/g (FM) for helminth eggs. The abatement rates have been calculated in the present review.
The reviewed studies compared well in terms of the abatement rate of pathogens, which is reported to be more important in summer than in other seasons. Shanahan et al., 2010 conducted a large scale solar drying of SS and assessed the efficiency of such a covered structure (plastic film roof) in terms of SS sanitization using indicators of fecal contamination. The assessment was based on absence–presence aspect after 12 days of drying. Solar-dried biosolids were analyzed for selected pathogenic microbial including fecal coliforms, total coliforms, and bacteriophages as indicators of viral survival throughout the drying process. At the end of the solar drying process, although fecal coliforms were found to be present, Enteroviruses, parasites, E. coli, and Salmonella spp. were removed by 12 days of drying.
Under semi-arid climate, Belloulid et al. (2017) demonstrated, through a pilot-scale covered greenhouse tunnel made of polycarbonate, that the high temperatures recorded in summer allowed a significant abatement of fecal coliform and total coliform (almost 96%). However, the dying cycle in winter was less efficient in removing pathogens (almost 60%). Paluszak et al. (2012) demonstrated that using other sources of heat boosts the effect of solar drying to remove pathogenic microorganisms during winter. In fact, an abatement rate of 91.7% is reached, compared to only 60% recorded by Belloulid et al. (2017) when involving only solar radiation. Liming before mechanical dewatering and solar drying processes leads to a high abatement rate of fecal and total coliform (more than 99%). Thus, the combination of lime and greenhouse effect where, climatic conditions are not favorable, is efficient for pathogen removal. Regarding the fate of helminth eggs in SS when subjected to the solar drying process, the single reviewed study demonstrated significant removal of helminth eggs in summer under semi-arid climate. A complete removal of some species (Shistosoma sp., Capillaria sp., Trichuris sp., Toxocara sp., and Taenia sp.) is reported, while an incomplete abatement of Ascaris eggs was noticed, and the final load in SDS exceeds the threshold established by the WHO of an agricultural application of SDS. However, to not pronounce restrictive decisions on the efficiency of the solar drying process in terms of helminth eggs removal, more studies involving different operating conditions should be conducted.
Overall, the aforementioned findings rely on the pathogen removal in SS to high temperatures inside the solar dryer. These findings, if compared to those of the previous section, which reported the highly significant increase in the DM content after solar drying, it can be possible to assume some mechanisms behind pathogen removal. In fact, the temperatures inside the solar dryers may affect directly and indirectly the fate of pathogens in SS. On the first hand, the direct impact is likely to be attributed to the damages that would occur at the cellular level. For instance, helminth eggs when exposed to high temperatures, including those commonly recorded inside the solar dryers, become sensitive to many stressors as the membrane lipids of their shell is often affected (Barrett, 1976; Pecson et al., 2007; Hafidi et al., 2018). On the other hand, solar drying induces indirect pathogens abatement through DM increase. This results in a decrease in the water activity index in SS which induces an osmotic stress on pathogens. As a result, pathogens cells cannot take up water and become inactive.
What should be criticized in the few reviewed studies regarding pathogen removal after solar drying of SS is the fact that only the presence–absence aspect has been considered for many indicators of fecal contamination. However, the spore analysis did not appear in focus in all the reported studies. While assessing the sanitizing effect of solar drying of SS for an agricultural valorization, the absence of microorganisms is not sufficient to guarantee a safe use. In other words, if spores are still present in SDS, when incorporated to agricultural soils, they may regrow under favorable conditions. Moreover, when assessing the removal of helminth eggs using the solar drying process, viability aspect should also be considered to avoid any underestimation of their associated risks.
More information is needed in the literature regarding the behavior of organic micropollutants and HMs in sludge during the solar drying process. In fact, only two studies have addressed the variation of total HM content, and the behavior of organic contaminants is still an unsettled issue. As indicated in Table 9, no significant removal of HMs from SS has been recorded. Therefore, it has been concluded that the solar drying process does not involve any mechanisms to remove HMs from SS. However, results reported by An-nori et al. (2020a) demonstrated changes in HM speciation under semi-arid climate. Cr, Cu, and Pb, initially bound to the oxidizable fraction (80, 70, and 80%, respectively), were found to be abundant in the residual form after 45 days of sludge solar drying (78, 73, and 71%, respectively). Ni, mostly associated to the reducible fraction (43.7%) in the mechanically dewatered sludge, was found mostly associated to the residual fraction by the end of the solar drying process (55%). The changes occurred during solar drying of SS in terms of HM speciation were referred to the significant decrease in moisture content and organic matter changes. In fact, when dry matter increases, amorphous minerals could be converted to crystalline and stable form. This explains the increase in the residual fraction and the decrease in the reducible form of Ni. The conversion of the oxidizable fraction to the residual one in the case of Cr, Cu, and Pb is linked to the organic matter change, as confirmed by Milinovic et al. (2017), indicating that drying at 40°C changed the nature of organic compounds.
The reviewed results of the single existing study regarding heavy metals behavior in SS during the solar drying process focused only on Cu, Ni, Cr, and Pb as they were the most abundant. Moreover, it was conducted in summer season under semi-arid climate, which constitutes the most favorable climatic conditions for such a process. Thus, the above-discussed results in terms of speciation changes are not enough to decide on the efficiency of the solar drying process in terms of HM stabilization. To do so, further investigations on a large HM spectrum are necessary to be conducted covering different operating conditions.
Advances in wastewater treatment technologies in the last decades have led to an increase in SS production worldwide. Sludge recycling in agriculture became a solution to restore exhausted soils, namely, in the African context, as it includes plant nutrients and important organic matter content.
Solar drying is involved at the wastewater treatment plant scale as an extended dewatering technique. When solar-dried sludge is intended for an agricultural application, multiple questions are raised regarding the efficiency of such a process in terms of pathogen removal and HMs and other organic micropollutant behavior. Moreover, a question regarding agronomic characteristics of SDS is raised. This review focused on the impact of the solar drying process on the water content in sludge (hence DM), pathogen removal, and heavy metals and organic micropollutant behavior. The few existing data in the literature confirm that solar drying increases very significantly increases the DM content in sludge; starting with 20%–30%, a range of 80%–90% is reached in summer within different geographical contexts under different climates. However, lower efficiency in the increase in the DM content can be noticed in winter in continental climate. In the case of semi-arid climate, the solar drying process is efficient in both winter and summer in terms of water removal. Solar drying has more significant impact on pathogens in sludge in summer than in winter. Indeed, the high temperatures in summer allow a very significant abatement of fecal coliform and total coliform (almost 96%). Contrariwise, the drying cycle in winter showed a lower removal rate (an average of 60%). The reviewed data regarding solar drying efficiency in terms of water removal, hence sludge volume reduction, confirm the usefulness of such a process especially where climate conditions are favorable (e.g., semi-arid climate).
To overcome the reliance of solar drying on weather where climatic conditions are not favorable in winter, hybrid solar dryers are used with particular emphasis as they lead to a complete pathogen removal. Liming sludge before undergoing the solar drying process is also an efficient option to boost the effect of solar drying while sanitizing SS.
Regarding HM behavior, it has been concluded in light of the reviewed data that the solar drying process does not involve any removal mechanisms. However, changes in terms of speciation have been demonstrated showing a reduction in heavy metals availability.
Since 2001, interest has been devoted to the application of the solar drying process on SS as a stabilizing and sanitizing treatment. However, research is still in progress, and more investigations are needed to decide on the effectiveness of the solar drying process in terms of pathogen removal and HM stabilization. In fact, the existing data, reviewed in this study regarding HM speciation changes, are still not enough to decide on HM mobility reduction due to solar drying process, as long as more drying cycles are not conducted (only summer cycle has been reported). Organic micropollutants in solar-dried SS are still an unsettled issue throughout the literature.
In light of the reviewed literature and from an agricultural and environmental standpoint, the following points are to be considered as a way forward for research linked to solar drying of SS and as a contribution to help SS management stakeholders to decide on the feasibility of an agricultural application of SDS:
1) investigations on the solar drying process should cover different operating conditions including mainly the less favorable and the most favorable ones and taking into account the intrinsic quality of SS, which is known to be highly variable;
2) more investigations are needed to assess the removal of pathogens during the solar drying process, and this should also include the spores to avoid any underestimation of pathogens related risks;
3) viability aspect should be considered in future investigations on parasites removal in order to assess the sanitizing effect of solar drying of SS more accurately;
4) investigation of PAHs, antibiotics, and other emerging contaminants in sludge while undergoing the solar drying process should be triggered;
5) the further research, that is highly required in the present context, is supposed to help stakeholders to develop standards regarding organic micropollutants in SS.
AA-n: formulation of the hypothesis, development of review objectives, defining the methodology, data analysis, interpretation and synthesis, original draft writing, substantial correction; AE: review outline formulation, substantial correction; KEM: language editing, substantial correction; LEF: co-supervisor, substantial correction; MEG: co-supervisor, substantial correction; MH: supervisor, substantial correction, final approval of the submitted version.
The authors declare that the research was conducted in the absence of any commercial or financial relationships that could be construed as a potential conflict of interest.
All claims expressed in this article are solely those of the authors and do not necessarily represent those of their affiliated organizations, or those of the publisher, the editors, and the reviewers. Any product that may be evaluated in this article, or claim that may be made by its manufacturer, is not guaranteed or endorsed by the publisher.
The Supplementary Material for this article can be found online at: https://www.frontiersin.org/articles/10.3389/fenvs.2022.814590/full#supplementary-material
Aadraoui, M., Elbaghdadi, M., Rais, J., Barakat, A., Ennaji, W., Karroum, L. A., et al. (2018). Characteristics of Sewage Sludge Produced from Wastewater Treatment Plant in the Moroccan City Khouribga. Dwt 112, 179–185. doi:10.5004/dwt.2018.22069
Abad, E., Martínez, K., Planas, C., Palacios, O., Caixach, J., and Rivera, J. (2005). Priority Organic Pollutant Assessment of Sludges for Agricultural Purposes. Chemosphere 61, 1358–1369. doi:10.1016/j.chemosphere.2005.03.018
Adamski, J. M., Danieloski, R., Deuner, S., Braga, E. J. B., de Castro, L. A. S., and Peters, J. A. (2012). Responses to Excess Iron in Sweet Potato: Impacts on Growth, Enzyme Activities, mineral Concentrations, and Anatomy. Acta Physiol. Plant 34 (5), 1827–1836. doi:10.1007/s11738-012-0981-3
Agence Nationale pour la Rkcupkration et 1'∼limination des Dkchets (1988). La volorisation agricole des boues des stations d'epuration urbaines. Cahiers techniques de la direction de l'eau et de la prevention des pollutions et des risques, 23. Angers, France. Publication. Agence Nationale pour la Rkcuperation et 1'élimination des Dkchets.
Aemig, Q., Doussiet, N., Danel, A., Delgenès, N., Jimenez, J., and Houot, S. (2019). Organic micropollutants’ distribution within sludge organic matter fractions explains their dynamic during sewage sludge anaerobic digestion followed by composting. Environmental Science and Pollution Research. doi:10.1007/s11356-018-4014-7
Amir, S., Hafidi, M., Merlina, G., Hamdi, H., and Revel, J. C. (2005). Fate of Polycyclic Aromatic Hydrocarbons during Composting of Lagooning Sewage Sludge. Chemosphere 58, 449–458. doi:10.1016/j.chemosphere.2004.09.039
Amir, S., Merlina, G., Pinelli, E., Winterton, P., Revel, J. C., and Hafidi, M. (2008). Microbial community dynamics during composting of sewage sludge and straw studied through phospholipid and neutral lipid analysis. Journal of Hazardous Materials 159, 593–601.
Amoah, I. D., Singh, G., Stenström, T. A., and Reddy, P. (2017). Detection and Quantification of Soil-Transmitted Helminths in Environmental Samples: A Review of Current State-Of-The-Art and Future Perspectives. Acta Tropica 169, 187–201. doi:10.1016/j.actatropica.2017.02.014
Amoah, I. D., Kumari, S., and Bux, F. (2020). Coronaviruses in Wastewater Processes: Source, Fate and Potential Risks. Environ. Int. 143, 105962. doi:10.1016/j.envint.2020.105962
Angelidaki, I., and Ahring, B. K. (1999). “Anaerobic Digestion of Sludge: Focusing on Degradation of the Contained Organic Contaminants. in: Problems Around Sludge,” in ‘Proceedings of the Workshop jointly organised by the Directorate-General for the Environment and the Ispra Joint Research Centre of the European Commission’, Stresa, 18–19 November. Editors Langenkamp, and Marmo, 38–47.
An-nori, A., El Fels, L., Ezzariai, A., El Gharous, M., El Mejahed, K., and Hafidi, M. (2020a). Effects of Solar Drying on Heavy Metals Availability and Phytotoxicity in Municipal Sewage Sludge under Semi-arid Climate. Environ. Tech. Innovation 19, 101039. doi:10.1016/j.eti.2020.101039
An-nori, A., Fels, L. El., Ezzariai, A., Hayani, B., Mejahed, K. El., Gharous, M. El., et al. (2020b). Effectiveness of Helminth Egg Reduction by Solar Drying and Liming of Sewage Sludge. Environmental Science and Pollution Research. doi:10.1007/s11356-020-11619-w
Babula, P., Adam, V., Opatrilova, R., Zehnalek, J., Havel, L., and Kizek, R. (2009). “Uncommon Heavy Metals, Metalloids and Their Plant Toxicity: a Review,” in Organic Farming, Pest Control and Remediation of Soil Pollutants. Dordrecht: Springer, 275–317. doi:10.1007/978-1-4020-9654-9_14
Bagge, E., Sahlström, L., and Albihn, A. (2005). The Effect of Hygienic Treatment on the Microbial flora of Biowaste at Biogas Plants. Water Res. 39, 4879–4886. doi:10.1016/j.watres.2005.03.016
Balboa, S., Mauricio-iglesias, M., Rodriguez, S., Martínez-Lamas, L., Vasallo, F. J., Regueiro, B., et al. (2021). The Fate of SARS-COV-2 in WWTPS Points Out the Sludge Line as a Suitable Spot for Detection of COVID-19. Sci. Total Environ. 772, 145268. doi:10.1016/j.scitotenv.2021.145268
Barceló, J., Poschenrieder, C., Andreu, I., and Gunsé, B. (1986). Cadmium-Induced Decrease of Water Stress Resistance in Bush Bean Plants (Phaseolus vulgaris L. Cv. Contender) I. Effects of Cd on Water Potential, Relative Water Content, and Cell Wall Elasticity. J. Plant Physiol. 125, 17–25. doi:10.1016/S0176-1617(86)80239-5
Barret, M., Carrère, H., Latrille, E., Wisniewski, C., and Patureau, D. (2010). Micropollutant and Sludge Characterization for Modeling Sorption Equilibria. Environ. Sci. Technol. 44, 1100–1106. doi:10.1021/es902575d
Barrett, J. (1976). Studies on the Induction of Permeability in Ascaris lumbricoides Eggs. Parasitology 73 (1), 109–121. doi:10.1017/S0031182000051374
Belloulid, M. O., Hamdi, H., Mandi, L., and Ouazzani, N. (2019). Solar Drying of Wastewater Sludge: a Case Study in Marrakesh, Morocco. Environ. Tech. 40, 1316–1322. doi:10.1080/09593330.2017.1421713
Bennamoun, L., Arlabosse, P., and Léonard, A. (2013). Review on Fundamental Aspect of Application of Drying Process to Wastewater Sludge. Renew. Sust. Energ. Rev. 28, 29–43. doi:10.1016/j.rser.2013.07.043
Bennamoun, L., Arlabosse, P., Leonard, A., Bennamoun, L., Arlabosse, P., and Leonard, A. (2017). Review on Fundamental Aspect of Application of Drying Process to Wastewater Sludge to Cite This Version : HAL Id : Hal-01643397 28. Renewable and Sustainable Energy Reviews, 28–43. doi:10.1016/j.rser.2013.07.043
Bennamoun, L. (2012). Solar Drying of Wastewater Sludge: A Review. Renew. Sust. Energ. Rev. 16, 1061–1073. doi:10.1016/j.rser.2011.10.005
Berg, R., Denneman, C. A. J., and Roels, J. M. (1993). “Risk Assessment of Contaminated Soil: Proposals for Adjusted, Toxicologically Based Dutch Soil Clean-Up Criteria,” in Contaminated Soil '93. Editors F. Arendt, G.J. Annokkke, R. Bosman, and W.J. van den Brink (Dordrecht, The Netherlands: Kluwer Academic Publishers), 349–364. Weftec 2001. doi:10.1007/978-94-011-2018-0_52
Bibby, K., and Peccia, J. (2013). Identi Fi Cation of Viral Pathogen Diversity in Sewage Sludge by Metagenome Analysis. Environmental science and technology 47, 1945–1951. doi:10.1021/es305181x
Bien, J. B., Bien, J. D., and Matysiak, B. (1999). Gospodarka Odpadami Woczyszczalniach Scieków; Wydawnictwa Politechniki Cz‘estochowskiej: Cz‘estochowa. Poland.
Bouazizi, H., Jouili, H., Geitmann, A., and El Ferjani, E. (2010). Copper Toxicity in Expanding Leaves of Phaseolus vulgaris L.: Antioxidant Enzyme Response and Nutrient Element Uptake. Ecotoxicol. Environ. Saf. 73 (6), 1304–1308. doi:10.1016/j.ecoenv.2010.05.014
Bouhoum, K., Amahmid, O., and Asmama, S. (2018). Occurrence and Removal of Protozoan Cysts and Helminth Eggs in Waste Stabilization Ponds in Marrakech. Water Science and Technology, D 42 (10–11), 159–164.
Brison, C., Perret, J. M., and Canler, J. P. (2010). Le séchage solaire des boues : Etat actuel de l’art et retours d’expérience. [Rapport Technique] irstea 80 hal-02593906. Available at: https://hal.inrae.fr/view/index/identifiant/hal-02593906
Bux, M., Baumann, R., Philipp, W., Conrad, T., and Mühlbauer, W. (2001). Class-a by solar drying recent experiences in Europe, in Proceedings of the Water Environment Federation (WEFTE’01), Atlanta. GA, 301–317.
Capizzi-Banas, S., Deloge, M., Remy, M., and Schwartzbrod, J. (2004). Liming as an Advanced Treatment for Sludge Sanitisation: Helminth Eggs Elimination-Ascaris Eggs as Model. Water Res. 38, 3251–3258. doi:10.1016/j.watres.2004.04.015
Chaffai, R., and Koyama, H. (2011). Heavy Metal Tolerance in Arabidopsis thaliana. Adv. Bot. Res. 60, 149. Academic Press. doi:10.1016/b978-0-12-385851-1.00001-9
Chang, Z., Long, G., Zhou, J. L., and Ma, C. (2020). Valorization of Sewage Sludge in the Fabrication of Construction and Building Materials: A Review. Resour. Conservation Recycling 154, 104606. doi:10.1016/j.resconrec.2019.104606
Chaoua, S., Boussaa, S., Khadra, A., and Boumezzough, A. (2018). Efficiency of Two Sewage Treatment Systems (Activated Sludge and Natural Lagoons) for Helminth Egg Removal in Morocco. J. Infect. Public Health 11, 197–202. doi:10.1016/j.jiph.2017.07.026
Chen, G., Lock Yue, P., and Mujumdar, A. S. (2002). Sludge Dewatering and Drying. Drying Tech. 20, 883–916. doi:10.1081/drt-120003768
Chee-Sanford, R. I., Koike, I. G., Krapac, H. D., Oliver, A. C., Yannarell, J. C., and Chee-Sanford, R. I. (2009). Monitoring and source tracking of tetracycline resistance genes in lagoons and groundwater adjacent to swine production facilities over a 3-year period. Appl. Environ. Microbiol 73, 4813–4823. doi:10.1128/AEM.00665-07
Chen, J., Yu, Z., Michel, F. C., Wittum, T., Morrison, M., Al, C. E. T., et al. (2007). Development and Application of Real-Time PCR Assays for Quantification of Erm Genes Conferring Resistance to Macrolides-Lincosamides-Streptogramin B in Livestock Manure and Manure Management Systems. Appl. Environ. Microbiol. 73, 4407–4416. doi:10.1128/AEM.02799-06
Choppala, G., SaifullahBolan, N., Bolan, N., Bibi, S., Iqbal, M., Rengel, Z., et al. (2014). Cellular Mechanisms in Higher Plants Governing Tolerance to Cadmium Toxicity. Crit. Rev. Plant Sci. 33 (5), 374–391. doi:10.1080/07352689.2014.903747
Collard, M., Teychené, B., and Lemée, L. (2017). Comparison of Three Different Wastewater Sludge and Their Respective Drying Processes: Solar, thermal and Reed Beds - Impact on Organic Matter Characteristics. J. Environ. Manage. 203, 760–767. doi:10.1016/j.jenvman.2016.05.070
Commission of the European Communities (1986). Council Directive 86/278/EEC of 12 June 1986 on the protection of the environment, and in particular of the soil, when sewage sludge is used in agriculture OJ L 181, 6–12.
Delmail, D., Labrousse, P., Hourdin, P., Larcher, L., Moesch, C., and Botineau, M. (2011). Differential Responses of Myriophyllum Alterniflorum DC (Haloragaceae) Organs to Copper: Physiological and Developmental Approaches. Hydrobiologia 664 (1), 95–105. doi:10.1007/s10750-010-0589-9
Drost-Hansen, W. (1981). “The Occurrence and Extent of Vicinal Water,” in Biophysics of Water. Editor F. Franks (New York: John Wiley & Sons).
Dubey, M., Mohapatra, S., Tyagi, V. K., Suthar, S., and Kazmi, A. A. (2021). Occurrence, fate, and persistence of emerging micropollutants in sewage sludge treatment. Environmental Pollution 273, 116515.
El Fels, L., Hafidi, M., Silvestre, J., Kallerhoff, J., and Pinelli, E. (2015). Efficiency of co-composting process to remove genotoxicity from sewage sludge contaminated with hexavalent chromium. Ecological Engineering 82, 355–360.
El Fels, L., El Hayany, B., El Faiz, A., Saadani, M., Houari, M., and Hafidi, M. (2019). Sludge Nematodes, Cestodes, and Trematodes Eggs Variation from Lagooning, Activated Sludge and Infiltration-Percolation Wastewater Treatment System under Semi-arid Climate. Environ. Sci. Pollut. Res. 26, 570–576. doi:10.1007/s11356-018-3618-2
El Hammoudani, Y., and Dimane, F. (2021). Occurrence and Fate of Micropollutants during Sludge Treatment: Case of Al-Hoceima WWTP, Morocco. Environ. Challenges 5, 100321. doi:10.1016/j.envc.2021.100321
El Hammoudani, Y., Dimane, F., and El Ouarghi, H. (2019). Characterization of sewage sludge generated from wastewater treatment plant in relation to agricul- tural use. Environ. Water Sci. Res. Public Health Territ. Intellig. 3, 47–52. doi:10.48421/IMIST.PRSM/ewash-ti-v3i1.15085
El Hayany, B., El Glaoui, G. E. M., Rihanni, M., Ezzariai, A., El Faiz, A., El Gharous, M., et al. (2018). Effect of Dewatering and Composting on Helminth Eggs Removal from Lagooning Sludge under Semi-arid Climate. Environ. Sci. Pollut. Res. 25, 10988–10996. doi:10.1007/s11356-017-1066-z
Elzinga, E. J., and Cirmo, A. (2010). Application of Sequential Extractions and X-ray ab- Sorption Spectroscopy to Determine the Speciation of Chromium in Northern New Jersey Marsh Soils Developed in Chromite Ore Processing Residue (COPR). J. Hazard. Mater. 183, 145e154.
Ezzariai, A., Hafidi, M., Khadra, A., Aemig, Q., El Fels, L., Barret, M., et al. (2018). Human and Veterinary Antibiotics during Composting of Sludge or Manure: Global Perspectives on Persistence, Degradation, and Resistance Genes. J. Hazard. Mater. 359, 465–481. doi:10.1016/j.jhazmat.2018.07.092
Fageria, N. K., Filho, M. B., Moreira, A., and Guimara˜es, C. M. (2009). Foliar Fertilization of Crop Plants. J. Plant Nutr. 32 (6), 10441064. doi:10.1080/01904160902872826
FAO (2015). Boosting Africa’s Soils;From the Abuja Declaration on Fertilizers to a Sustainable Soil Management Framework for Food and Nutrition Security in Africa by 2030, 29th Session of the FAO Regional Conference for Africa.
Fars, S., Oufdou, K., Nejmeddine, A., Hassani, L., Melloul, A. A., Bousselhaj, K., et al. (2005). Antibiotic Resistance and Survival of Faecal Coliforms in Activated Sludge System in a Semi-arid Region (Beni Mellal, Morocco). World J. Microbiol. Biotechnol. 21, 493–500. doi:10.1007/s11274-004-2613-6
Feachem, R., Bradley, D. J., Garelick, H., and Mara, D. (1983). Sanitation and Disease: Health Aspects of Excreta and Wastewater Management. Chiche- ster: John Wiley & Sons.
Feitz, A. J., Guan, J., and Waite, T. D. (2001). Size and Structure Effects on Centrifugal Dewatering of Digested Sewage Sludge. Water Sci. Technol. 44, 427–435. doi:10.2166/wst.2001.0798
Fijalkowski, K., Rorat, A., Grobelak, A., and Kacprzak, M. J. (2017). The Presence of Contaminations in Sewage Sludge - the Current Situation. J. Environ. Manage. 203, 1126–1136. doi:10.1016/j.jenvman.2017.05.068
Fytili, D., and Zabaniotou, A. (2008). Utilization of Sewage Sludge in EU Application of Old and New Methods-A Review. Renew. Sust. Energ. Rev. 12, 116–140. doi:10.1016/j.rser.2006.05.014
Gantzer, C., Gaspard, P., Galvez, L., Huyard, A., Dumouthier, N., and Schwartzbrod, J. (2001). Monitoring of Bacterial and Parasitological Contamination during Various Treatment of Sludge. Water Res. 35, 3763–3770. doi:10.1016/S0043-1354(01)00105-1
Gao, N., Kamran, K., Quan, C., and Williams, P. T. (2020). Thermochemical Conversion of Sewage Sludge: A Critical Review. Prog. Energ. Combustion Sci. 79, 100843. doi:10.1016/j.pecs.2020.100843
Gawlik, B. (2012). “FATE SEES Results of a Pan-European Snapshot of Randomly Taken Sewage Sludge Sample,” in Presented at the Proceedings of Workshop DG ENV and DG JRC, Brussels-Belgium.
Gerardi, M. H., and Zimmerman, M. C. (2005). “Wastewater Pathogens,” in Global Atlas of Excreta, Wastewater Sludge, and Biosolids Management (Hoboken, NJ, USA: John Wiley & Sons). doi:10.1002/0471710431
Goberna, M., Simón, P., Hernández, M. T., and García, C. (2018). Prokaryotic Communities and Potential Pathogens in Sewage Sludge: Response to Wastewaster Origin, Loading Rate and Treatment Technology. Sci. Total Environ. 615, 360–368. doi:10.1016/j.scitotenv.2017.09.240
Hafidi, M., El Fels, L., El Asli, A., and Ouhdouch, Y. (2018). Effect of Co-composting on Helminth Eggs Removal. Environ. Eng. Manag. J. 17, 459–465. doi:10.30638/eemj.2018.047
Hajjami, K., Ennaji, M. M., Fouad, S., Oubrim, N., and Cohen, N. (2013). Wastewater Reuse for Irrigation in Morocco: Helminth Eggs Contamination’s Level of Irrigated Crops and Sanitary Risk (A Case Study of Settat and Soualem Regions). J. Bacteriol. Parasitol. 4, 163. doi:10.4172/2155-9597.1000163
Hanay, Ö., Hasar, H., Kocer, N. N., and Aslan, S. (2008). Evaluation for Agricultural Usage with Speciation of Heavy Metals in a Municipal Sewage Sludge. Bull. Environ. Contam. Toxicol. 81, 42–46. doi:10.1007/s00128-008-9451-4
He, Q., Ren, Y., Mohamed, I., Ali, M., Hassan, W., and Zeng, F. (2013). Assessment of Trace and Heavy Metal Distribution by Four Sequential Extraction Procedures in a Contaminated Soil. Soil Water Res. 8, 71–76. doi:10.17221/20/2012-swr
Heidrich, M., and Witkowski, C. (2005). Urz ˛adzenia Do Oczyszczania ´Scieków. Warszawa, Poland: Wydawnictwo Seidel-Przywecki Sp. z o.o., 201–234. Soziale Arbeit und Häusliche Gewalt. doi:10.2307/j.ctvbkk043.12
Hernandez, L. E., Carpena-Ruiz, R., and Garate, A. (1996). Alterations in the mineral Nutrition of Pea Seedlings Exposed to Cadmium. J. Plant Nutr. 19 (12), 15811598. doi:10.1080/01904169609365223
Heukelekian, H., and Weinberg, E. (1956). Bound Water and Activated Sludge Bulking. Sewage Ind. Wastes 28, 558. Israelachvili.
Holm-Nielsen, J. B., Al Seadi, T., and Oleskowicz-Popiel, P. (2009). The Future of Anaerobic Diges- Tion and Biogas Utilization. Bioresour. Technol. 100, 5478–5484. doi:10.1016/j.biortech.2008.12.046
Hussain, D., Haydon, M. J., Wang, Y., Wong, E., Sherson, S. M., Young, J., et al. (2004). P-type ATPase Heavy Metal Transporters with Roles in Essential Zinc Homeostasis in Arabidopsis. Plant Cell 16 (5), 1327–1339. doi:10.1105/tpc.020487
Idrissi, Y. A., Mansouri, D., El Yemli, A., Kherrati, I., Berrid, N., Ghachoui, E. H., et al. (2020). Parasitological Assessment of the Purifying Performance of the Wastewater Station by Natural Lagoon of Azilal, Morocco. Egypt. J. Aquat. Biolo. Fish. 24 (3), 299–309. doi:10.21608/ejabf.2020.92401
Inoue, S., Sawayama, S., Ogi, T., and Yokoyama, S.-y. (1996). Organic Composition of Liquidized Sewage Sludge. Biomass and Bioenergy 10 (1), 37–40. doi:10.1016/0961-9534(95)00056-9
Islam, E., Yang, X., Li, T., Liu, D., Jin, X., and Meng, F. (2007). Effect of Pb Toxicity on Root Morphology, Physiology and Ultrastructure in the Two Ecotypes of Elsholtzia Argyi. J. Hazard. Mater. 147 (3), 806–816. doi:10.1016/j.jhazmat.2007.01.117
Jamali, M. K., Kazi, T. G., Arain, M. B., Afridi, H. I., Jalbani, N., Kandhro, G. A., et al. (2009). Speciation of Heavy Metals in Untreated Sewage Sludge by Using Microwave Assisted Sequential Extraction Procedure. J. Hazard. Mater. 163, 1157–1164. doi:10.1016/j.jhazmat.2008.07.071
Jarde, E., Mansuy, L., and Faure, P. (2003). “Characterization of the Macromolecular Organic Content of Sewage Sludges by Thermally Assisted Hydrolysis and Methylation- Gas Chromatographyemass Spectrometer (THM-GC/MS),” in Pyrolysis 2002 Conference, Japan. Issue 68e69, 331e350. doi:10.1016/S0165-2370(03)00053-6
Jiménez, B. (2007). Helminth Ova Control in Sludge: A Review. Water Sci. Technol. 56, 147–155. doi:10.2166/wst.2007.713
Ju, F., Xia, Y., Guo, F., Wang, Z., and Zhang, T. (2014). Taxonomic Relatedness Shapes Bacterial Assembly in Activated Sludge of Globally Distributed Wastewater Treatment Plants. Environ. Microbiol. 16, 2421–2432. doi:10.1111/1462-2920.12355
Kalogo, Y. (2008). Global Water Research Coalition, State of Science Report: Energy and Resource Recovery from Sludge. Global Water Research Coalition. Alexandria, VA: Water Environment Research Foundation, 238.
Kamil Salihoglu, N., Pinarli, V., and Salihoglu, G. (2007). Solar Drying in Sludge Management in Turkey. Renew. Energ. 32, 1661–1675. doi:10.1016/j.renene.2006.08.001
Khadra, A., Ezzariai, A., Kouisni, L., and Hafidi, M. (2019a). Helminth Eggs Inactivation Efficiency by Sludge Co-composting under Arid Climates. Int. J. Environ. Health Res. 31, 530–537. doi:10.1080/09603123.2019.1671960
Khadra, A., Ezzariai, A., Merlina, G., Capdeville, M.-J., Budzinski, H., Hamdi, H., et al. (2019b). Fate of Antibiotics Present in a Primary Sludge of WWTP during Their Co-composting with palm Wastes. Waste Manage. 84, 13–19. doi:10.1016/j.wasman.2018.11.009
Khadra, A., Pinelli, E., Ezzariai, A., Mohamed, O., Merlina, G., Lyamlouli, K., et al. (2019c). Assessment of the Genotoxicity of Antibiotics and Chromium in Primary Sludge and Compost Using Vicia faba Micronucleus Test. Ecotoxicology Environ. Saf. 185, 109693. doi:10.1016/j.ecoenv.2019.109693
Khan, S. J., and Ongerth, J. E. (2001). Estimation of Pharmaceutical Residues in Primary and Secondary Sewage Sludge Based on Quantities of Use and Fugacity Modelling. Water Sci. Tech. 46, 105–114. doi:10.2166/wst.2002.0065
Kim, N., and Fergusson, J. (1993). Concentrations and Sources of Cadmium, Copper, lead and Zinc in House Dust in Christchurch, New Zealand. Sci. Total Environ. 138, 1–21. doi:10.1016/0048-9697(93)90400-z
Koné, D., Cofie, O., Zurbrügg, C., Gallizzi, K., Moser, D., Drescher, S., et al. (2007). Helminth Eggs Inactivation Efficiency by Faecal Sludge Dewatering and Co-composting in Tropical Climates. Water Res. 41, 4397–4402. doi:10.1016/j.watres.2007.06.024
Lasheen, M. R., and Ammar, N. S. (2009). Assessment of Metals Speciation in Sewage Sludge and Stabilized Sludge from Different Wastewater Treatment Plants, Greater Cairo, Egypt. J. Hazard. Mater. 164, 740–749. doi:10.1016/j.jhazmat.2008.08.068
LeBlanc, R. J., Matthews, P., and Richard, R. P. (2009). Global Atlas of Excreta, Wastewater Sludge, and Biosolids Management: Moving Forward the Sustainable and welcome Uses of a Global Resource. Choice Rev. Online 47, 47–1767. doi:10.5860/choice.47-1767
Lima, M. R. P., Zandonade, E., and Sobrinho, P. A. (2012). Characteristics of WWTP Sludge after Drying in Greenhouse for Agricultural Purposes. Water Sci. Technol. 66, 1460–1466. doi:10.2166/wst.2012.326
Lloret, E., Pascual, J. A., Brodie, E. L., Bouskill, N. J., Insam, H., Juárez, M. F.-D., et al. (2016). Sewage Sludge Addition Modifies Soil Microbial Communities and Plant Performance Depending on the Sludge Stabilization Process. Appl. Soil Ecol. 101, 37–46. doi:10.1016/j.apsoil.2016.01.002
López-Luna, J., González-Chávez, M. C., Esparza-García, F. J., and Rodríguez-Vázquez, R. (2009). Toxicity Assessment of Soil Amended with Tannery Sludge, Trivalent Chromium and Hexavalent Chromium, Using Wheat, Oat and Sorghum Plants. J. Hazard. Mater. 163, 829–834. doi:10.1016/j.jhazmat.2008.07.034
Lowe, P. (1995). Developments in the thermal Drying of Sewage Sludge. Water Environ. J. 9 (3), 306e316. doi:10.1111/j.1747-6593.1995.tb00944.x
Sposito, G., Lund, L. J., and Chang, A. C. (1982). Trace Metal Chemistry in Arid-Zone Field Soils Amended with Sewage Sludge: I. Fractionation of Ni, Cu, Zn, Cd, and Pb in Solid Phases. Soil Sci. Soc. Am. J. 46. 260–264.
Manga, M., Evans, B., Camargo-Valero, A., and Horan, N. (2016). The Fate of Helminth eggs during the Co-composting of Faecal Sludge with Chicken Feathers and Market waste. Proceedings of the 13th IWA Specialized Conference on Small Water and Wastewater Systems (SWWS) and 5th IWA Specialized Conference on Resources-Oriented Sanitation (ROS). Athens, Greece, September 14-16, 2016.
Mathioudakis, V. L., Kapagiannidis, A. G., Athanasoulia, E., Diamantis, V. I., Melidis, P., and Aivasidis, A. (2009). Extended Dewatering of Sewage Sludge in Solar Drying Plants. Desalination 248, 733–739. doi:10.1016/j.desal.2009.01.011
Mathioudakis, V. L., Kapagiannidis, A. G., Athanasoulia, E., Paltzoglou, A. D., Melidis, P., and Aivasidis, A. (2013). Sewage Sludge Solar Drying: Experiences from the First Pilot-Scale Application in Greece. Dry. Technol. 31 (5), 519e526.
Medema, G., Heijnen, L., Elsinga, G., Italiaander, R., and Brouwer, A. (2020). Presence of SARS-Coronavirus-2 RNA in Sewage and Correlation with Reported COVID-19 Prevalence in the Early Stage of the Epidemic in The Netherlands. Environ. Sci. Technol. Lett. 7, 511–516. doi:10.1021/acs.estlett.0c00357
Metcalf , , and Eddy, (1991). Wastewater Engineering: Treatment, Disposal and Reuse. McGraw-Hill Book Co.
Milinovic, J., Vidal, M., Lacorte, S., and Rigol, A. (2014). Leaching of Heavy Metals and Alkylphenolic Compounds from Fresh and Dried Sewage Sludge. Environ. Sci. Pollut. Res. 21, 2009–2017. doi:10.1007/s11356-013-2100-4
Mininni, G., Braguglia, C. M., Ramadori, R., and Tomei, M. C. (2004). An Innovative Sludge Management System Based on Separation of Primary and Secondary Sludge Treatment. Water Science and Technology 50 (9), 145–154.
Ministry of Environment Morocco (2010). Phénixa : étude pour l’Elaboration de la Stratégie Nationale de Gestion des Boues des Stations d’Epuration des Eaux au Maroc. rapport phase 1.
Mrabet, T. K., and Agoumi, A. (1991). OEufs d’Helminthes parasites dans les eaux usées de Nador. Acte de la VII ème Journée de la Société Marocaine de Parasitologie. Marrakech, 15–16.
Mrabet, R., Saber, N., El-Brahli, A., Lahlou, S., and Bessam, F. (2000). Total, Particulate Organic Matter and Structural Stability of a Calcixeroll Soil under Different Wheat Rotations and Tillage Systems in a Semiarid Area of Morocco. Soil Tillage Res. 57, 225–235. doi:10.1016/S0167-1987(00)00180-X
Navarro, I., and Jiménez, B. (2011). Evaluation of the WHO Helminth Eggs Criteria Using a QMRA Approach for the Safe Reuse of Wastewater and Sludge in Developing Countries. Water Sci. Tech. 63, 1499–1505. doi:10.2166/wst.2011.394
Mcgrath, S. P., Chang, A. C., Page, A. L., and Witter, E. (1994). Land Application of Sewage Sludge: Scientific Perspectives of Heavy Metal Loading Limits in Europe and the United States. Environ. Rev. 2, 108–118.
Paluszak, Z., Skowron, K., Sypuła, M., and Skowron, K. J. (2012). Microbiological Evaluation of the Effectiveness of Sewage Sludge Sanitization with Solar Drying Technology. Int. J. Photoenergy 2012, 1–11. doi:10.1155/2012/341592
Peccia, J., and Westerhoff, P. (2015). We Should Expect More Out of Our Sewage Sludge. Environ. Sci. Technol. 49, 8271–8276. doi:10.1021/acs.est.5b01931
Pecson, B. M., Barrios, J. A., Jiménez, B. E., and Nelson, K. L. (2007). The Effects of Temperature, pH, and Ammonia Concentration on the Inactivation of Ascaris Eggs in Sewage Sludge. Water Res. 41, 2893–2902. doi:10.1016/j.watres.2007.03.040
Pepper, I. L., Brooks, J. P., and Gerba, C. P. (2006). Pathogens in Biosolids. Adv. Agron. 90, 1–41. doi:10.1016/s0065-2113(06)90001-7
Planas, C., Abad, E., Martínez, K., Palacios, O., Caixach, J., and Rivera, J. (2005). Priority organic pollutant assessment of sludges for agricultural purposes. Chemosphere 61, 1358–1369.
Pradell, N., Pbrjiatr, A., Champs, C. De., Palcoux, J. B., Sirot, T., and Forestierl, C. (2000). Les infections B Escherichia coli producteurs de verotoxines: étude de la prévalence chez l’enfant dans la région Auvergne.
Raheem, A., Sikarwar, V. S., He, J., Dastyar, W., Dionysiou, D. D., Wang, W., et al. (2018). Opportunities and Challenges in Sustainable Treatment and Resource Reuse of Sewage Sludge: A Review. Chem. Eng. J. 337, 616–641. doi:10.1016/j.cej.2017.12.149
Reinthaler, F. F., Galler, H., Feierl, G., Haas, D., Leitner, E., Mascher, F., et al. (2013). Resistance patterns of Escherichia coli isolated from sewage sludge in comparison with those isolated from human patients in 2000 and 2009. J. Water Health 11 (1), 13e20. doi:10.2166/wh.2012.207
Rocha, M. C. V. d., Barés, M. E., and Braga, M. C. B. (2016). Quantification of Viable Helminth Eggs in Samples of Sewage Sludge. Water Res. 103, 245–255. doi:10.1016/j.watres.2016.07.039
Rogers, H. R. (1996). Sources, Behaviour and Fate of Organic Contaminants during Sewage Treatment and in Sewage Sludges. Sci. Total Environ. 185, 3–26. doi:10.1016/0048-9697(96)05039-5
Romanowska, E., Wróblewska, B., Drożak, A., Zienkiewicz, M., and Siedlecka, M. (2008). Effect of Pb Ions on Superoxide Dismutase and Catalase Activities in Leaves of Pea Plants Grown in High and Low Irradiance. Biol. Plant. 52 (1), 80–86. doi:10.1007/s10535-008-0012-9
Romdhana, M. H., Lecomte, D., Ladevie, B., and Sablayrolles, C. (2009). Monitoring of Pathogenic Microorganisms Contamination during Heat Drying Process of Sewage Sludge. Process Saf. Environ. Prot. 87 (6), 377–386. doi:10.1016/j.psep.2009.08.003
Rulkens, W. (2008). Sewage Sludge as a Biomass Resource for the Production of Energy: Overview and Assessment of the Various Options. Energy Fuels 22, 9–15. doi:10.1021/ef700267m
Samolada, M. C., and Zabaniotou, A. A. (2014). Comparative Assessment of Municipal Sewage Sludge Incineration, Gasification and Pyrolysis for a Sustainable Sludge-To-Energy Management in Greece. Waste Manage. 34, 411–420. doi:10.1016/j.wasman.2013.11.003
Sarwar, N., Saifullah, S. S., Malhi, S. S., Zia, M. H., Naeem, A., Bibi, S., et al. (2010). Role of mineral Nutrition in Minimizing Cadmium Accumulation by Plants. J. Sci. Food Agric. 90 (6), 925–937. doi:10.1002/jsfa.3916
Scott, R. (2013). Fate and Behaviour of Parasites in Wastewater Treatment Systems, Handbook of Water and Wastewater Microbiology. Portsmouth PO1 3HF: University of Portsmouth. doi:10.1016/B978-0-12-470100-7.50032-7
Shahid, M., Shamshad, S., Rafiq, M., Khalid, S., Bibi, I., Niazi, N. K., et al. (2017). Chromium Speciation, Bioavailability, Uptake, Toxicity and Detoxification in Soil-Plant System: A Review. Chemosphere 178, 513–533. doi:10.1016/j.chemosphere.2017.03.074
Shanahan, E. F., Roiko, A., Tindale, N. W., Thomas, M. P., Walpole, R., and Kurtböke, D. İ. (2010). Evaluation of Pathogen Removal in a Solar Sludge Drying Facility Using Microbial Indicators. Int. J. Environ. Res. Public Health 7, 565–582. doi:10.3390/ijerph7020565
Sharma, D. C., Sharma, C. P., and Tripathi, R. D. (2003). Phytotoxic Lesions of Chromium in maize. Chemosphere 51, 63–68. doi:10.1016/s0045-6535(01)00325-3
Singh, S., Yadav, V., Arif, N., Singh, V. P., Dubey, N. K., Ramawat, N., et al. (2009). Uptake Mechanisms , Toxicity , and Alleviation, Plant Life Under Changing Environment. INC. doi:10.1016/B978-0-12-818204-8.00001-1
Siqueira-Silva, A. I., Silva, L. C. d., Azevedo, A. A., and Oliva, M. A. (2012). Iron Plaque Formation and Morphoanatomy of Roots from Species of Restinga Subjected to Excess Iron. Ecotoxicology Environ. Saf. 78, 265–275. doi:10.1016/j.ecoenv.2011.11.030
Soriano-Disla, J. M., Navarro-Pedreño, J., and Gómez, I. (2010). Contribution of a Sewage Sludge Application to the Short-Term Carbon Sequestration across a Wide Range of Agricultural Soils. Environ. Earth Sci. 61, 1613–1619. doi:10.1007/s12665-010-0474-x
Soudi, B. (2001). Compostage des déchets ménagers et valorisation du compost. Cas des petites et moyennes communes au Maroc. Actes édition Revue RT.E. N° 121-Décember, 102.
Tavazzi, S., Locoro, G., Comero, S., Sobiecka, E., Loos, R., Gans, O., et al. (2012)Occurrence and Levels of Selected Compounds in European Sewage Sludge Samples. Institute for Environment and Sustainability Ispra, en Italie, FATE SEES. doi:10.2788/67153
Taylor, P., Novak, J. T., and Novak, J. T. (2017). Drying Technology : An International Journal Dewatering of Sewage Sludge. Dewatering. Sewage Sludge, 37–41. doi:10.1080/07373930600840419
Thermo-system (2018). la plus grande installation du séchage solaire au monde à Marrakech entre en service. Available at: http:// www.thermo-system.com/fr/entreprise/annonces-actuelles/annoncesactuelles-accessed at Jully 2021.
Tripathi, D. K., Singh, V. P., and Kumar, D. (2012). Impact of exogenous silicon addition on chromium uptake, growth, mineral elements, oxidative stress, antioxidant capacity, and leaf and root structures in rice seedlings exposed to hexavalent chromium. Acta Physiol. Plant. 34 (1), 279–289.
Tomulescu, I. M., Radoviciu, E. M., Merca, V. V., and Tuduce, A. D. (2004). Effect of Copper, Zinc and lead and Their Combinations on the Germination Capacity of Two Cereals. Acta Agraria Debreceniensis 15, 39–42. doi:10.34101/actaagrar/15/3355
Torres, M. A., Barros, M. P., Campos, S. C. G., Pinto, E., Rajamani, S., Sayre, R. T., et al. (2008). Biochemical Biomarkers in Algae and marine Pollution: a Review. Ecotoxicology Environ. Saf. 71 (1), 1–15. doi:10.1016/j.ecoenv.2008.05.009
U.S. Environmental Protection Agency (1993). Standards for the Use or Disposal of Sewage Sludge. Fed. Regist. 58, 9248–9415.
UK Statutory Instruments (1989). The Sludge (Use in Agriculture) Regulations. SI 1263. London: Her Majesty's Stationery Office.
US Epa Protocol (1999). Control of Pathogens and Vector Attraction in Sewage Sludge. Washington, DC: USEPA’s Pathogen Equivalency Committee (PEC), USEPA Environmental Regulations and Technology, Office of Research and Development EP A/625/R-92/013, 177.
USGS (2008). Mineral Commodity Summaries 2008. Washington, USA: US Geological Survey (USGS), United States Government Printing Office.
Vesilind, P. A. (1994). The Role of Water in Sludge Dewatering. Water Environ. Res. 66 (1), 4–11. doi:10.2175/wer.66.1.2
Wang, L., Govind, R., and Dobbs, R. A. (1993). Sorption of Toxic Organic Compounds on Wastewater Solids: mechanism and modeling environ. Sci. Technol 27, 152.
Werther, J., and Ogada, T. (1999). Sewage Sludge Combustion. Prog. Energ. Combustion Sci. 25 (162), 55–116. doi:10.1016/s0360-1285(98)00020-3
Wild, S. R., McGrath, S. P., and Jones, K. C. (1990). The polynuclear aromatic hydrocarbon (PAH) content of archived sewage sludges. Chemosphere 20 (6), 703–771.
WHO-World Health Organization (2006). Excreta and Greywater Use in Agriculture. Guidelines for the Safe Use of Wastewater, Excreta and Greywater 4. Geneva: WHO
Wu, M.-H., Lin, C.-L., Huang, W.-C., and Chen, J.-W. (2016). Characteristics of Pervious concrete Using Incineration Bottom Ash in Place of sandstone Graded Material. Construction Building Mater. 111, 618–624. doi:10.1016/j.conbuildmat.2016.02.146
Yang, B., Meng, L., and Xue, N. (2018). Removal of five fluoroquinolone antibiotics during broiler manure composting. Environ. Technol. 39, 373e381. doi:10.1080/09593330.2017.1301568
Yang, W., Cai, C., and Dai, X. (2020). The Potential Exposure and Transmission Risk of SARS-CoV-2 through Sludge Treatment and Disposal. Resour. Conservation Recycling 162, 105043. doi:10.1016/j.resconrec.2020.105043
Zebarth, B. J., Drury, C. F., Tremblay, N., and Cambouris, A. N. (2009). Opportunities for Improved Fertilizer Nitrogen Management in Production of Arable Crops in Eastern Canada: A Review. Can. J. Soil Sci. 89, 113–132. doi:10.4141/CJSS07102
Keywords: Heavy metals, micropollutants, stabilization, agricultural use, organic amendment
Citation: An-nori A, Ezzariai A, El Mejahed K, El Fels L, El Gharous M and Hafidi M (2022) Solar Drying as an Eco-Friendly Technology for Sewage Sludge Stabilization: Assessment of Micropollutant Behavior, Pathogen Removal, and Agronomic Value. Front. Environ. Sci. 10:814590. doi: 10.3389/fenvs.2022.814590
Received: 13 November 2021; Accepted: 24 February 2022;
Published: 11 April 2022.
Edited by:
Haiyan Wang, Zhejiang University, ChinaReviewed by:
Sartaj Ahmad Bhat, Gifu University, JapanCopyright © 2022 An-nori, Ezzariai, El Mejahed, El Fels, El Gharous and Hafidi. This is an open-access article distributed under the terms of the Creative Commons Attribution License (CC BY). The use, distribution or reproduction in other forums is permitted, provided the original author(s) and the copyright owner(s) are credited and that the original publication in this journal is cited, in accordance with accepted academic practice. No use, distribution or reproduction is permitted which does not comply with these terms.
*Correspondence: Mohamed Hafidi, SGFmaWRpQHVjYS5hYy5tYQ==
Disclaimer: All claims expressed in this article are solely those of the authors and do not necessarily represent those of their affiliated organizations, or those of the publisher, the editors and the reviewers. Any product that may be evaluated in this article or claim that may be made by its manufacturer is not guaranteed or endorsed by the publisher.
Research integrity at Frontiers
Learn more about the work of our research integrity team to safeguard the quality of each article we publish.