- 1Univ. Littoral Côte d’Opale, CNRS, Univ. Lille, UMR 8187—LOG—Laboratoire d’Océanologie et de Géosciences, Wimereux, France
- 2Univ. Littoral Côte d’Opale, UMR 1158 BioEcoAgro, Institut Charles Viollette, USC Anses, Boulogne-sur-Mer, France
- 3ANSES—Laboratoire de Sécurité des Aliments, Boulevard du Bassin Napoléon, Boulogne-sur-Mer, France
Microplastics (MPs) (plastic particles < 5 mm in size) have become the most ubiquitous type of anthropogenic litter contaminating aquatic environments worldwide, and are capable of harming aquatic organisms and entering the food web. Microplastic research has rapidly evolved over several decades, with many studies sampling microplastic in surface waters. For sea surface sampling of MPs, different net devices have been used. But although there is an increasing number of studies using these devices to quantifying MPs in different aquatic environments, data comparison is difficult due to the lack of standardised, harmonised sampling methods and data reporting units. The Manta net device is actually the most commonly used method for sea surface sampling of MPs. The three main parts of this net are: the floating/stabilizing part, the opening mouth and the net bag, and each of them can have its own specifications. These specifications, along with the sampling methods, can be critical for the efficiency of the sampling accurate quantification of MPs in the aquatic environments. The use of different mesh sizes, inconsistency in trawling duration, speed and distance, and in the net opening dimensions, make it impossible to compare the studies between each other. This review analyzes the methodologies and characteristics of Manta nets used for MPs sampling, discussing factors that can impact the efficiency of the sampling and the quantification of MPs, and proposes recommendations in order to improve and standardize the sampling protocol.
Introduction
Microplastic (MPs) pollution represents a growing concern for contemporary society, due to the constant increase in plastic production and its disposal and accumulation in the aquatic environments. Annual plastic production has gone from 10 million tons in 1960 to 368 million tons in 2019 (Plastic Europe, 2020), and these data do not take into account PET (polyethylene terephthalate) fibers, PA (polyamide) and polyacyl. At such a scale, an obvious consequence of this production is the huge amount of plastic litter released into the environment, especially to aquatic ecosystems. The actual amount of plastic that is, released to the aquatic environment is hard to quantify but is estimated at between 5 and 20 million metric tons each year (Jambeck et al., 2015; Isobe et al., 2019). Plastic particles are now found worldwide, even in the remote areas like Antarctica and the Himalayas (González-Pleiter et al., 2020; Hamilton et al., 2021; Ajay et al., 2021).
The number of studies published on MPs pollution in aquatic environments has drastically increased in the last decade (Cowger et al., 2020). Such an increase has yielded a lot of knowledge on the scale of microplastic pollution and highlighted the need to employ the correct evaluation procedure. Analytical methods for identifying the polymer composition of MPs have achieved a broad consensus among scientists, with Raman and Fourier-transform infrared spectroscopy being the two most-used methods for identifying their nature. However, there is no standard sampling method used among studies and no recognised international protocol for surface water MPs sampling (Cowger et al., 2020; Ryan et al., 2020). The most commonly used field sampling methods for MPs in aquatic environment are nets, bottles, buckets and pumps (Hung et al., 2021).
Due to the high variability of MPs spatial distribution, the use of a pump or bulk sampler may not be representative since the covered sampling area is limited (Desforges et al., 2014; Song et al., 2014; GESAMP 2022). The sampled volumes with this method are also limited and overestimation of MPs abundance can be observed (Tamminga et al., 2019). However, these methods present some advantages, they are relatively easy to use and can usually be handled by one person and deployed in area where net trawling is impractical (harbour, shallow areas and near the shore … ). Unlike nets where the mesh size determines the minimum particle sampled, they also allow the very smallest MPs to be collected. An underestimation of the smallest particles can be a problem, especially when they are possibly the most dangerous for marine life.
Trawl nets have been widely used for sampling MPs in aquatic environment as they allow the sampling of large surfaces or volumes of water relatively quickly in order to obtain a representative sample of the studied area (Hidalgo-Ruz et al., 2012; Barrows et al., 2017; Gago et al., 2019). Among the different trawl nets the Neuston net has been after the Manta net the most used in the marine environment for sampling and monitoring MP contamination. However, due to its low reliability with respect to the sampling height within the water column, its design was upgraded to the Manta net design for more stability, buoyancy in the water and precision in the water layer being sampled (Brown and Cheng 1981). The principal difference between the Neuston nets consists in the height of the sampled water layer: Manta generally samples the first 15–25 cm while the Neuston net samples a larger water layer (generally slightly less than 50 cm). Currently, the Manta net is the most commonly used method for surface sampling MPs in saltwater as well as freshwater systems (Prata et al., 2019; Mai et al., 2018; Razeghi et al., 2021). There are five times more studies that have used Manta net than Neuston net in recent years (Figure 1).
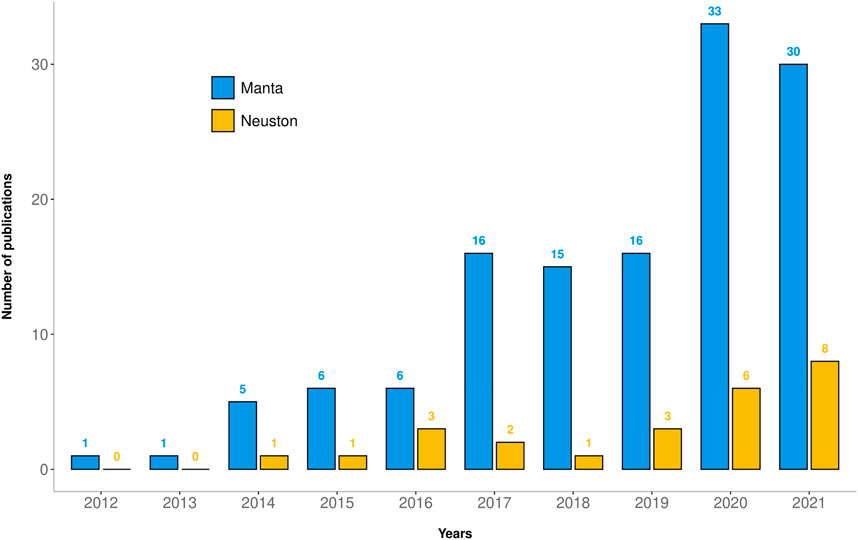
FIGURE 1. Number of publications per year using the Manta net (blue) or the Neuston net (yellow) as a sampling method for microplastics on the water surface during the last decade.
Many modifications on the early Manta net have been made and it has been used for collecting surface floating litter in oceans worldwide (Moore et al., 2001; Lattin et al., 2004) and more recently for sampling of MPs (e.g., Eriksen et al., 2013; de Lucia et al., 2014). Still, there is little consistency in the Manta net design with different sizes of the device and sampling criteria in aquatic environments (sampling duration, sampling speed, mesh size used … ).
This review aims to (i) analyze the methodologies and the characteristics of Manta nets used for MPs sampling, (ii) discuss factors that can impact the efficiency of the sampling and the MPs quantification, and (iii) propose recommendations in order to improve and standardize the sampling protocol.
In order to reach this objective an extensive literature review, using a scientific database (Scopus®) and a scientific article browser (Google Scholar®), was conducted to collect all articles published up to October 2021. No article including the Manta net for sampling MPs was identified before 2012. The employed research equations were “manta + microplastics” and “manta + microplastic.” Articles were conserved if the Manta net was directly used in the study for sampling MPs in aquatic environments. From Scopus®, 58 documents were collected, and the query on Google Scholar yielded 1340 documents, from which 71 new articles were included.
The reviewed studies covered all six continents with very different aquatic environments such as lakes, estuaries, rivers, coastal and open seas. The majority of them, 71%, covered seawater sampling in coastal areas Supplementary Table S1, whereas others studied freshwater areas like rivers (Ta et al., 2020; He et al., 2021; Frank et al., 2021 i.e.), lakes (Uurasjärvi et al., 2020; Bertoldi et al., 2021; Felismino et al., 2021, i.e.) or reservoirs (Ramadan and Sembiring, 2020) Supplementary Table S2.
Overview of the Characteristics of Manta Nets
The “Manta Net” was originally designed for collecting organisms and flotsam from the sea surface in the 1980s (Brown and Cheng, 1981). The Manta Net design and name derives from the surface-feeding Manta Ray (Manta sp.) because of its paired, anterior extensions flanking the mouth enables it to capture organisms at the ocean surface (Brown and Cheng, 1981). A Manta net consists of a frame part and the collecting net terminates in a collector Figure 2. The frame part is generally composed of stainless steel or aluminum, so that the aperture remains the same size during the sampling. These dimensions of the opening vary a lot according to the different studies. The width of the mouth can be from 30 cm up to 120 cm (Wang et al., 2020) and the height varies from 10 to 75 cm (Isobe et al., 2015) Figure 3. In the literature analyzed in this review, the most common dimension was 60 cm wide and 15 cm high (Figure 3), with half of the studies having apertures with at least one of these dimensions Supplementary Tables S1, S2. On both sides of the frame, the floating parts considered as “wings” or floaters, are able to keep the Manta net floating and stable during the whole sampling process. The floating part is usually the main difference between different models of Manta net outside of its dimensions. Wings can provide a better stability but the floating part can be adaptable towards the position of the Manta net in water.
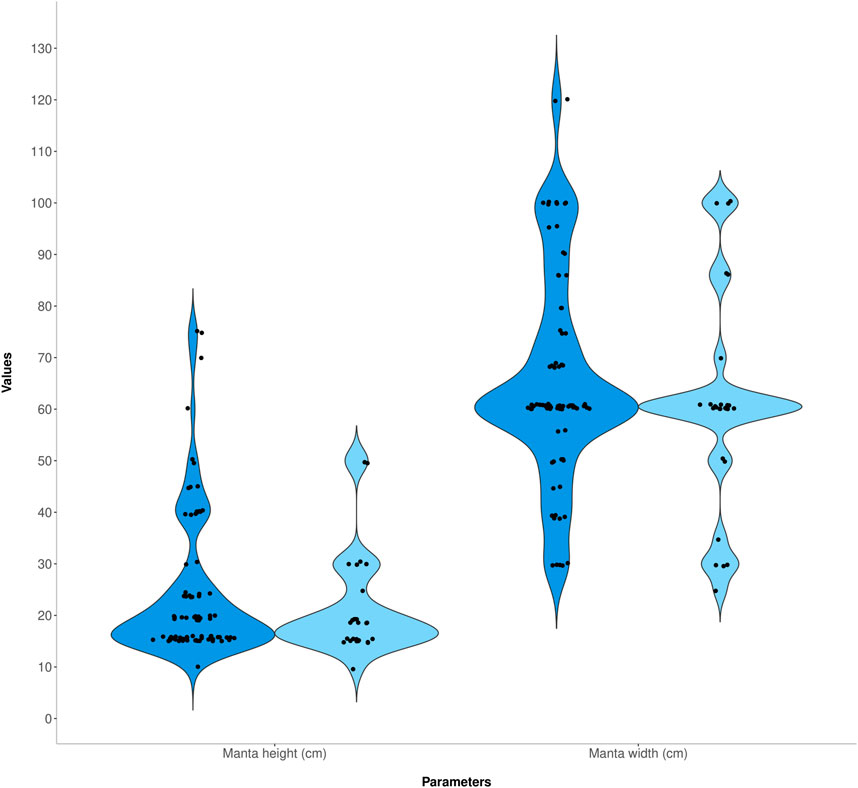
FIGURE 3. Distribution of Manta height and width in the reviewed literature, including studies of both saltwater (dark blue) and freshwater (light blue).
The dimensions of the net follow the dimensions of the open mouth for the opening area and the length of net is usually around 200–300 cm (Figure 4).
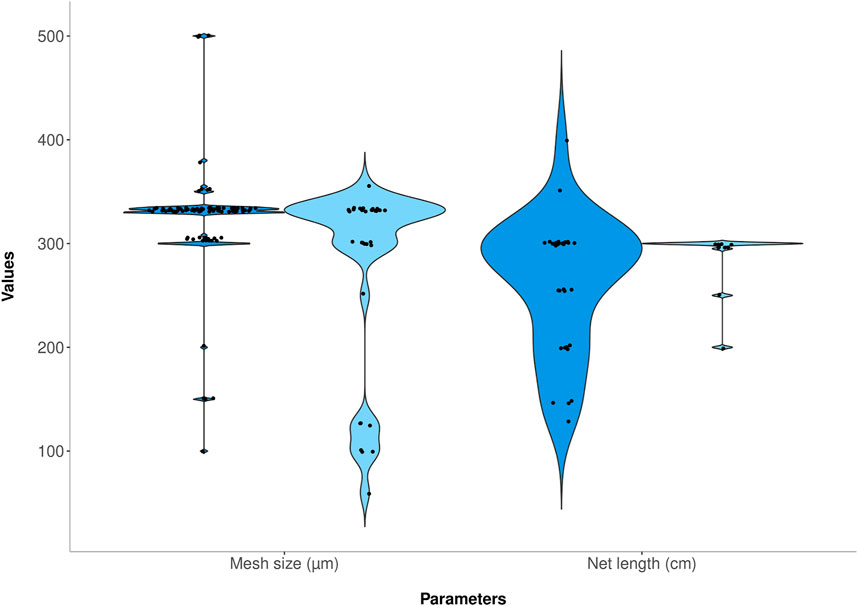
FIGURE 4. Distribution of Manta mesh size and net length in the reviewed literature, including studies of both saltwater (dark blue) and freshwater (light blue).
According to 90% of the studies, a mesh size of ca. 300–350 µm is the most widely used Figure 4. No particular reason is given for this mesh size except that Manta nets evolved from plankton sampling nets that often have a 330 µm mesh size. Besides 300–350 μm, other mesh sizes were also used in other studies e.g., 52, 60, 100, 125, 150, and 200 µm (Kazour et al., 2019; Herrera et al., 2020; Ramadan and Sembiring, 2020; Sembiring et al., 2020; Bertoldi et al., 2021; Zheng et al., 2021) Figure 4; Supplementary Table S2.
Sampling Procedures: Wake Zone, Speed, Time and Distance
According to the review analysis, Manta net sampling requires the use of a motorboat or similar vessel, or a sailing boat (Palatinus et al., 2019). The procedure consists of deploying the Manta net from the boat using different techniques to avoid turbulence and possible contamination from the boat and the sampling device. For example, poles and cables can be deployed from the side of the boat guaranteeing that the Manta net is kept far enough from the boat during sampling (Li et al., 2020). It is important to deploy the Manta net out of the wake zone (approx. 3–4 m distance from the boat) to prevent collecting water affected by the turbulence happening inside the wake zone. More than 80% of the studies reviewed followed this guideline in an attempt to avoid contamination caused by the boat.
The Manta trawl is towed horizontally and against the current. The hauling speed is an important parameter to take into consideration (Gago et al., 2019). Considering all the studies reviewed, 76% performed the MP sampling at a speed below 3 knots, but five studies (6%) sampled at a higher speed of around 4 to 5 knots (Green et al., 2018; Anderson et al., 2017; Ruiz-Orejón et al., 2018; Green et al., 2018; Teng et al., 2020) Figure 5. The AVANI trawl designed by Eriksen et al., 2018 can sample at higher speeds such as 8 knots. These high speeds always occurred on the open sea and while it reduces the time of sampling, it also results in the net becoming clogged more quickly. In contrast, six different studies employed a null speed sampling (Warrack et al., 2017; Deocaris et al., 2019; Constant et al., 2020; Mora-Teddy and Matthaei, 2020; Wong et al., 2020; Frank et al., 2021), which is only possible in rivers or streams where the current allows the MPs to be drawn into the net. The remaining studies did not mention any speed for the sampling step.
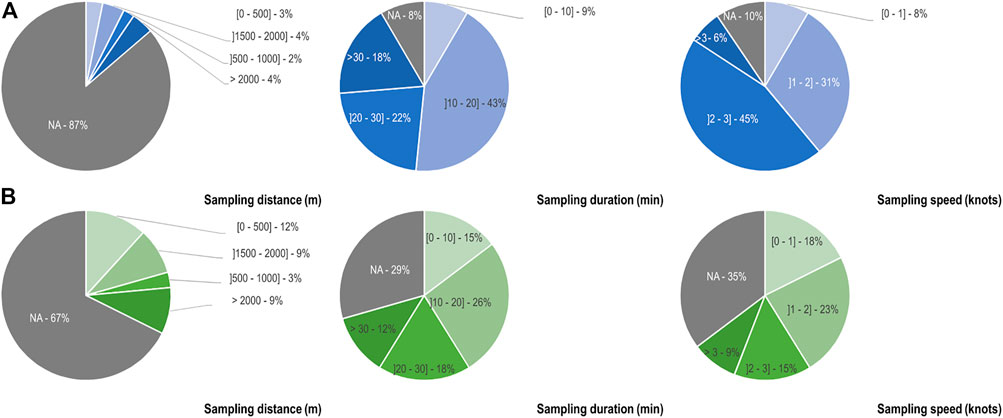
FIGURE 5. Dispersion of sampling distance, sampling duration and sampling speed of the reviewed literature including studies made in seawater (A) and freshwater (B).
The sampling speed influences the duration of the sampling that was also as a variable factor among the different studies. The sampling time ranged from 5 to 90 min with an average of 20 ± 5 min observed in 54% of studies Supplementary Tables S1, S2; Figure 5. The sampling time parameter has been studied by Michida et al. (2019), the authors recommended a sampling time of 20 min when the conditions allow it. The authors indicate that a 20 min sampling time is a good compromise between volume sampled and potential clogging problems. However, if the density of objects at the surface is too dense i.e., plankton, algae, fish eggs, the sampling time should be reduced. Some studies specified that if the particles of the water are too dense, a sampling time lower than 30 min is recommended (Viršek et al., 2016). A longer sampling time, higher than 90 min, can also lead to complications such as clogging of the net.
The distance covered during sampling is only reported in 16% of all the studies reviewed, Supplementary Tables S1, S2; Figure 5, mainly due to the fact that it can be deduced only with the sampling speed and duration or the number of rotations given by the flowmeter if one is used.
A Forgotten Parameter: Replicates
Another point exhibited in this review was that only 21% of the studies performed replicate sampling Supplementary Tables S1, S2. Replicate MP sampling is usually complicated by, for example, an inability to sample back and forth over the same sector. Moreover, it is also time consuming and the weather conditions can complicate the sampling. However, the results of replicate sampling at a location could better represent the real level of MPs in the studied environment. Three replicates of 10 min each instead of one sampling of 30 min are a good compromise between feasibility and a sufficiently representative sampling of the environment. Indeed, in this case, the risk of the net clogging or suffering another incident while sampling would be reduced. Moreover, comparison between these 3 replicates could be reported to gain an enhanced picture of variability in MP pollution at a given site.
Volume Sampled, Requirement of Precise Tools
In the studies reviewed here, the sampling area was calculated by multiplying the towing distance by the width of the trawl Supplementary Tables S1, S2. The volume of water was estimated using a flowmeter, or else by calculations based on the distance travelled by boat using a GPS at a constant speed, and then multiplying by the width of the Manta net frame (Stock et al., 2019). The sampling volume was clearly mentioned in only 11% of the reviewed studies. The sampled water volume was generally calculated using the following formula: sampled height underwater x width of the trawl x sampled distance. The sampled distance was calculated as “number of rotor rotations” x “flowmeter constant” when a flowmeter was present during the sampling. However, because the net’s immersion depth changes constantly with waves, wind and boat movement, it is difficult to accurately calculate the volume of water being filtered (Karlsson et al., 2020; Felismino et al., 2021). Most of the studies defined the water height approximately by considering “half of the Manta was submerged” (Felismino et al., 2021; He et al., 2021; Schmidt et al., 2021).
A study that has been reviewed here focused on developing an accurate method to measure the height of the water in order to obtain an accurate sample volume (Karlsson et al., 2020). The authors used a camera placed in front of the mouth opening of the Manta net which can follow and therefore estimate the height of the water during the sampling process. The study shows that a 30 min sampling with a few centimeters of height’s difference can lead to a huge difference in the sampling volumes. For example, an error of 48% was observed according to where the height is measured, between the lowest or the highest point reached by water (Karlsson et al., 2020). According to (Michida et al., 2019), the measurement of the water’s height remains an issue when using a Manta net and can lead to under- or over-estimating the volume sampled and the resulting concentration of MPs.
Attention should always be paid to the method used for measuring the volume sampled. Flowmeters and GPS methods for computing volume sampled can give very different results and can result in a halving or doubling of the MPs abundance (Gago et al., 2019). Using only the GPS could lead to misinterpretation of the distance travelled, for example, while the clogging effect and the currents also affect the real sampled volumes.
Survey of Microplastics in the Water Surface Affected by the Opening Mouth and Environmental Factors
Most of the reviewed studies referred to the Manta method for sampling surface and subsurface water without actually indicating the sampled depth Supplementary Tables S1, S2. The highest reported sampled depth using the Manta net was around 50 cm (De Lucia et al., 2014; Pini et al., 2018). However, the average sampled depth in all studies corresponds to 25 cm, which could be defined as a standard depth in upcoming standardized sampling methods. Naturally, and as previously discussed, the sampled depth is also largely dependent on the aperture characteristics.
Sampling with a Manta trawl requires relatively calm conditions to function properly (Anderson et al., 2017). Particles on the surface and the subsurface water are usually very dependent on meteorological and hydrodynamic factors such as wind, waves, currents and temperature (Drivdal et al., 2014; Kukulka et al., 2012). These phenomena can lead to the transport and the mixing of particles between surface and the subsurface water layers, and can be computed using wind speed and wave heights analysis (Kukulka et al., 2012). Collecting metadata related to those factors (meteorological and hydrodynamic) is thus very important during MPs sampling using a Manta net in order to better interpret the results. Studies showed that the wind’s speed and direction can interact with the presence of MPs in the environment by moving them from one environment to another (Collignon et al., 2012; Frias et al., 2020), and their concentration can also increase with rainfall and storm water runoff (Moore et al., 2001; Free et al., 2014; Sutton et al., 2016). The boat’s speed during sampling might also cause water turbulence that modifies the particles’ movement in the water column.
Along with meteorological and hydrodynamic factors, the physical properties of water, i.e., its density (mainly impacted by the temperature and the salinity), also impact the position of the MPs in the water column (Prata et al., 2019). This latter could also be modified by biofouling mechanisms that increases the density of particles (Kooi et al., 2017). Hence, in the same area, the type of polymers found at a depth of 40 cm can be different from those found at 15 cm depth (Lenaker et al., 2019). According to the reviewed studies, most of the polymers found onto the water surface and subsurface were PS (polystyrene), PE (polyethylene) and PP (polypropylene) (Frère et al., 2017; So et al., 2018; Aigars et al., 2021) Supplementary Tables S1, S2. These polymers have a density lower than 1 g/cm3, while PVC (polyvinyl chloride) or PET are more likely to be located deeper in the water column and in the bottom sediment due to their higher densities (Lenaker et al., 2019). But the opposite phenomenon is also observed when plastic particles with higher density than seawater are found at the surface and low-density polymers in benthic sediment (Suaria et al., 2016; Kaiser et al., 2017; Teng et al., 2020). Biofouling can be one explanation for these observations, when microorganisms that have high density develop on low density particles and chaotic behaviors of the water column transport high-density particles to the surface (Kooi et al., 2017). High-density particles were identified in 9% of the publications whereas lower density PE was characterized in 92% of the publications (Supplementary Tables S1, S2). Overall, all sampling methods considered, few studies have reported the distribution of polymers in the water column, and the impact of environmental phenomena like biofouling or weathering on the distribution of MPs in the water column are still poorly understood and need further development (Kooi et al., 2017).
Characteristics of the Net Impacting the Sampling
As previously mentioned, different sizes of the Manta’s net opening structure are reported in the literature, and no standard net size was used. The Manta nets used in freshwater and seawater are of similar sizes (opening and length) in terms of dimensions Figure 3, except for one device used in saltwater that was bigger than usual with a width of 100 cm (Pan et al., 2021). Smith et al. (1968) conducted an extensive series of instrumented net tows using nets of different shapes (cone and cylinder) and found that the clogging rate of the net depended on four factors:
1) The composition and the density of suspended materials in the water. Coastal waters with generally higher particle loading than oceanic waters will cause clogging to occur more rapidly.
2) The mesh size—the smaller the mesh size, the faster the net was clogged.
3) The ratio of filtering area to mouth area—the smaller the ratio the faster the clogging. For plankton net, the recommended ratio between the mesh-filtering area and the mouth area is a minimum of 6:1 (Tranter et al., 1968) for a mesh size of 0.3 mm and a ratio of 9:1 when using a net with smaller mesh openings (Saito, 2018).
4) The shape of the net—a cone net resisted clogging the best, closely followed by the cylinder-shaped net.
The clogging problem being mostly not reported in the reviewed publications, no precise information can be deduced. For example, few studies measure and adapt their sampling according to water turbidity or the density of suspended materials in the water (mesh size use, trawling duration).
The mesh net size seems to be the main factor affecting the sampling of MPs using a Manta net. Viršek et al. (2016) gave guidelines to sample MPs on the surface water with a Manta net and invoked the risk of nets getting clogged during sampling. The authors recommended 300 µm as the best mesh size to avoid any clogging problems, and Berov and Klayn 2020 offered the same advice. Excepting these two papers, no publication specifically focused on the clogging problem and how to solve it. Nevertheless, almost a third of the reviewed studies mentioned that clogging was problematic, and that they overcame this problem by using a mesh size of at least 300 µm and lowering both sampling time (around 20 min) and speed (less than 3 knots).
The reviewed studies highlighted that 90% of the authors used a mesh size around 300 µm Figure 4, but this size of mesh has been proven to underestimate the amount of MPs in both surface and subsurface water (Lindeque et al., 2020), especially when the concentration of MPs increases as the size distribution decreases (Kooi & Koelmans 2019). For example, the risk of underestimating microplastic pollution using higher mesh sizes investigated by Lindeque et al. (2020) showed that a 100 µm mesh sampled 2.5 times more MPs than to a 300 µm mesh and 10 times more than a 500 µm mesh.
The mesh size may directly influence the quantity of MPs sampled, but also has an impact upon the shapes of MPs collected, with beads only being sampled by a mesh size of 100 µm (Lindeque et al., 2020). The microplastic contamination while sampling with a Manta net mainly comes in the form of textile fibers according to (Gwinnett and Miller, 2021), but the Manta net sampling method is known to underestimate the fibers sampled in the environment as mentioned before, and fibers with a diameter <20 µm may not be retained (Ryan et al., 2020; Gwinnett and Miller, 2021). Hence, estimations of plastic fiber pollution levels derived from Manta net sampling should be made with this caveat.
According to the reviewed publications, the smallest ratio of filtering area to mouth area was around 8.6:1, a ratio which stills follow the instructions given by Tranter et al. (1968) and all the publications report the use of a cone shape net.
There are important sampling implications resulting from progressive net clogging, since the water ceases to be uniformly sampled as water will be pushed out of the net resulting in an underestimation of the collected MPs. As the pressure difference between the inside and the outside of the net increases, more particles are also extruded through the mesh. Once again, the use of a flowmeter could correct these effects due to the fact that it will give the exact amount of volume sampled by the Manta net (Gago J. et al., 2019).
Procedures to Avoid Contamination on the Field
Contamination during the sampling procedure is a point that has been reported in 25% of the reviewed studies, although it is crucial. Indeed, during field sampling most of the researchers had not really addressed ways to avoid environmental contamination, which is far more difficult than evaluating the contamination during laboratory analyses. Blank analyses can still be carried out by putting filtered Mill-Q water in an open stainless steel or glass container in order to analyze the atmospheric fallouts during the Manta net trawling. Systematically, the use of plastic tools must be avoided during the sampling and samples treatment, except if no stainless steel/glass tools alternative is available, in order to avoid contamination as much possible. A blank sample should also always be analyzed, when possible, at every step during the treatment and conditioning of the sample to check for possible contamination during the various stages of the process. Moreover, some parts of the Manta net device are often made of plastic polymers, such as the net that is, usually made of nylon, or the cod end collector that is, made of PVC. These plastic parts can be collected, analyzed and integrated in identification databases, in order to avoid overestimation of MP loads due to contamination by sampling devices (Scopetani et al., 2020).
Expression of Results
There is no standardized way of expressing the results among the studies. Slightly more than half of the reviewed publications present a number of particles per volume, usually a number of particles per cubic meter Supplementary Tables S1, S2. This percentage goes up to 78% of the reviewed studies located in streams or rivers are expressed with this unit, Supplementary Table S2. Indeed, this unit permitted the quantification of the MPs transported over a period amount of time, because the average water flow is usually known for a river or a stream. Another way of expressing the results is to give a number of particles per surface, as for example, 34% of the publications which expressed results as particles per square kilometer, Supplementary Tables S1, S2. This unit is more likely to be concern vast, open-water areas of seawater, or else very large freshwater areas such as the Great Lakes (Cable et al., 2017). However, this expression of results could lead to under or over estimation as the height of the water sampled is not considered and, therefore, it is hard to know which height of the water column has been really sampled. In addition, if the trawling speed is not constant, the volume of the water sampled will be affected. A third unit, reported in only 4% of the reviewed publications, was the expression of MPs mass per surface area, usually in milligrams per square kilometer (Hendrickson et al., 2018; Li et al., 2021). Finally, the remaining studies (7%) presented their results as a number of particles per transect of sampling, which reported the number of MPs found at every transect. Hence, there remains a lack of homogeneity in the expression of results among the studies of MPs’ abundance obtained with Manta net sampling Supplementary Tables S1, S2. These different units to express results make it impossible to compare the results among studies, even if the authors used the same settings during the sampling. The normalization of a harmonized system for expression of results would allow comparisons of the various studies and contribute to a better estimation of the extent and impact of MP pollution.
Proposition of Recommendation for Manta Net Sampling in the Frame of Harmonization
The future for sampling MPs with a Manta net would require first a harmonization and then a standardization of all the parameters in order to compare all data produced worldwide. Some characteristics should be described in order to obtain a more precise version of the protocols proposed, for example, by Viršek et al., 2016; Michida, Y., et al., 2019 and Cowger et al., 2020.
First, we recommend that sampling be always carried out under calm conditions, to limit the effects of turbulence on the water column. Sampling should not be carried out when timing and conditions are unsuitable, such as during high densities of natural particles or organisms, i.e., algae and plankton blooms (Michida, Y., et al., 2019). The environmental conditions, i.e., important metadata, should also always be recorded while a sample is collected, and include measurements of wind speed and direction, wave height, tide coefficient, sea temperature and weather conditions such as precipitation to allow comparisons with other survey results (Michida, Y., et al., 2019). Observation of the environmental conditions of the preceding few days is also very important, and can give good leads to explain the concentration of MPs in the water (Free et al., 2014; Sutton et al., 2016). It is recommended that the water turbidity be measured before each sampling campaign in order to adjust the Manta trawling time or distance and avoid clogging problems.
Secondly, the Manta net should be deployed horizontally in a counter current to optimize the sampling. Ideally, the device should be deployed from the side of the vessel (approx. 3–4 m distance from the boat) to avoid the disturbance from the ship’s wake, as well contamination from the ship itself.
The boat used for sampling with a Manta net should sail under 3 knots to properly stabilize the sampling net and to avoid tearing it by accident and to avoid turbulent inflow at the net mouth (Michida et al., 2019) Table 1.

TABLE 1. Recommended parameters while sampling with a Manta net according to the publications reviewed. W: width, H: height, L: net length.
The opening of the Manta net should always be just above the water surface by ca. 2 cm, in order to avoid excessive variation of the water height and limit atmospheric contamination.
A standardized size of the opening of the Manta should be defined: here we recommend that 25*60 cm is retained as a standardized size for the opening, not least because it is one of the most common dimensions used and hence will permit greater exploitation of previously gathered data Table 1.
The length of the sampling net should always be greater than 150 cm, if the above recommendations for the dimensions of the opening are followed, in order to avoid potential clogging problems. The recommended ratio between the mesh-filtering area and the mouth area of at least 6:1 should be respected.
The most widely used mesh size of 300 µm should be retained in order to preserve all the data background on the studies sites (Michida et al., 2019; Cowger et al., 2020) Table 1.
Replicates should always be carried out to improve the representativeness of the obtained results. To avoid clogging and other incidents while sampling, three replicates of a relatively short amount of time (between 10 and 20 min depending on the environmental conditions) seem to offer the best compromise between feasibility and representativeness of the sampled environment when compared with a long transect of 30 min or more Table 1.
Tracking the volume sampled is a major challenge while sampling with a Manta net: we recommend that a flowmeter should be used for every transect and placed in the middle of the opening during the whole sampling process. In this way, the volume sampled should be as precise as possible and uncertainty due to changes of water currents or any clogging effect can be reduced.
When possible, we recommend recording the net immersion depth during each sampling run for accurate calculation of the filtered water volume (Michida, Y., et al., 2019).
Contamination should be avoided as much as possible and attention should be paid in particular to contamination from fibers, as it has been proven that fiber abundance is underestimated while sampling with nets methods (Gwinnett and Miller, 2021). An environmental blank should be performed for every replicate during the sampling run, by placing filtered Milli-Q water in an open, stainless steel or glass container in order to analyze the atmospheric fallouts during the Manta net trawling. The results obtained with the blank should always be reported and subtracted from the water sampling results in order to correct obtained data and potential future data.
After each sampling event, the Manta net should be rinsed from the outside with water pumped from the environment (Viršek et al., 2016), the full area of the net has to be rinsed carefully in order to collect all the particles trapped in the cod end. The collector should then be removed from the main part and rinsed with filtered Milli-Q water directly into a glass container that has been cleaned with filtered ultra-pure water.
The results should also be expressed in all the units that are available in order to facilitate comparisons with other studies. We recommend that the results are expressed in particles per volume and area, as well as in mass per volume and area whenever possible.
The development of a sampling method which meets all of these characteristics will improve precision of the results and the comparability of levels of MPs contamination between studies and sampling sites. An interesting avenue for the future of MPs sampling would be to develop a new sampling device that combines the advantages of the Manta net with a large volume of water sampled but with greater stability to sample a constant height of water and presenting ease of use without having to use a boat and thus avoiding the sampling problems associated with the boat effect and contamination as described in this review. Such a device can be inspired by the use of an adapted and standardized aquatic drone that facilitates sampling in different aquatic environments regardless of depth or distance from the shore. It will also allow to explore more easily the contamination of aquatic environments by MPs according to a standardized protocol and will also enable sampling replicates to be carried out more easily.
Author Contributions
GP: conception of the article, collected and analyzed the data, developed the initial text and updating the article as per the suggestions; PD: conception of the article, revised the text and make substantial contributions towards genesis and design of the article; MK: revised the text and make substantial contributions towards genesis and design of the article; AD: designed the figures for descriptive statistics and revised the text and make substantial contributions towards genesis and design of the article; GD: revised the text and make substantial contributions towards genesis and design of the article; MD: contribution towards genesis and design of the article; RA: Conceptualization and methodological development of the study, revised the text and make substantial contributions towards genesis and design of the article.
Funding
This review is part of a PhD project named “Microplastics: Assessment of the contamination of organisms and aquatic environments in connection with the human consumption of aquatic product” funded by Université du Littoral Côte d'Opale, France as part of the IFSEA Graduate School. This work has been partially financially supported by the European Union (ERDF), the French Government, the Région Hauts-de-France and IFREMER, in the framework of the project CPER IDEAL 2021-2027.
Conflict of Interest
The authors declare that the research was conducted in the absence of any commercial or financial relationships that could be construed as a potential conflict of interest.
Publisher’s Note
All claims expressed in this article are solely those of the authors and do not necessarily represent those of their affiliated organizations, or those of the publisher, the editors and the reviewers. Any product that may be evaluated in this article, or claim that may be made by its manufacturer, is not guaranteed or endorsed by the publisher.
Acknowledgments
The authors are grateful to Dr. Mike Howsam for help with English proofreading. The authors would like to thank the Structure Fédérative de Recherche (SFR) Campus de la mer (France) for their support of Open access publication cost.
Supplementary Material
The Supplementary Material for this article can be found online at: https://www.frontiersin.org/articles/10.3389/fenvs.2022.811112/full#supplementary-material
References
Aigars, J., Barone, M., Suhareva, N., Putna-Nimane, I., and Dimante-Deimantovica, I. (2021). Occurrence and Spatial Distribution of Microplastics in the Surface Waters of the Baltic Sea and the Gulf of Riga. Mar. Pollut. Bull. 172, 112860. doi:10.1016/j.marpolbul.2021.112860
Ajay, K., Behera, D., Bhattacharya, S., Mishra, P. K., Ankit, Y., and Anoop, A. (2021). Distribution and Characteristics of Microplastics and Phthalate Esters from a Freshwater lake System in Lesser Himalayas. Chemosphere 283, 131132. doi:10.1016/j.chemosphere.2021.131132
Anderson, P. J., Warrack, S., Langen, V., Challis, J. K., Hanson, M. L., and Rennie, M. D. (2017). Microplastic Contamination in Lake Winnipeg, Canada. Environ. Pollut. 225, 223–231. doi:10.1016/j.envpol.2017.02.072
Barrows, A., Neumann, C., Berger, M., and Shaw, S. (2017). Grab vs. Neuston Tow Net: a Microplastic Sampling Performance Comparison and Possible Advances in the Field. Anal. Methods 9, 1446–1453. doi:10.1039/c6ay02387h
Berov, D., and Klayn, S. (2020). Microplastics and Floating Litter Pollution in Bulgarian Black Sea Coastal Waters. Mar. Pollut. Bull. 156, 111225. doi:10.1016/j.marpolbul.2020.111225
Bertoldi, C., Lara, L. Z., Mizushima, F. A. D. L., Martins, F. C. G., Battisti, M. A., Hinrichs, R., et al. (2021). First Evidence of Microplastic Contamination in the Freshwater of Lake Guaíba. Porto Alegre, Brazil: Science of the Total Environment, 759. doi:10.1016/j.scitotenv.2020.143503
Brown, D., and Cheng, L. (1981). New Net for Sampling the Ocean Surface. Mar. Ecol. Prog. Ser. 5, 225–227. doi:10.3354/meps005225
Cable, R. N., Beletsky, D., Beletsky, R., Wigginton, K., Locke, B. W., and Duhaime, M. B. (2017). Distribution and Modeled Transport of Plastic Pollution in the Great Lakes, the World’s Largest Freshwater Resource. Front. Environ. Sci. 5. doi:10.3389/fenvs.2017.00045
Collignon, A., Hecq, J.-H., Glagani, F., Voisin, P., Collard, F., and Goffart, A. (2012). Neustonic Microplastic and Zooplankton in the North Western Mediterranean Sea. Mar. Pollut. Bull. 64, 861–864. doi:10.1016/j.marpolbul.2012.01.011
Constant, M., Ludwig, W., Kerhervé, P., Sola, J., Charrière, B., Sanchez-Vidal, A., et al. (2020). Microplastic Fluxes in a Large and a Small Mediterranean River Catchments: The Têt and the Rhône, Northwestern Mediterranean Sea. Sci. Total Environ. 716, 136984. doi:10.1016/j.scitotenv.2020.136984
Cowger, W., Booth, A., Hamilton, B., Thaysen, C., Primpke, S., Munno, K., et al. (2020). Reporting Guidelines to Increase the Reproducibility and Comparability of Research on Microplastics. Appl. Spectrosc. 74.1066–1077 .doi:10.1177/0003702820930292
de Lucia, G. A., Caliani, I., Marra, S., Camedda, A., Coppa, S., Alcaro, L., et al. (2014). Amount and Distribution of Neustonic Micro-plastic off the Western Sardinian Coast (Central-Western Mediterranean Sea). Mar. Environ. Res. 100, 10–16. doi:10.1016/j.marenvres.2014.03.017
Deocaris, C. C., Allosada, J. O., Ardiente, L. T., Bitang, L. G. G., Dulohan, C. L., Lapuz, J. K. I., et al. (2019). Occurrence of Microplastic Fragments in the Pasig River. H2Open J. 2, 92–100. doi:10.2166/h2oj.2019.001
Desforges, J. P., Galbraith, M., Dangerfield, N., and Ross, P. S. (2014). Widespread Distribution of Microplastics in Subsurface Seawater in the NE Pacific Ocean. Mar. Pollut. Bull. 79, 94–99. doi:10.1016/j.marpolbul.2013.12.035
Drivdal, M., Broström, G., and Christensen, K. H. (2014). Wave-induced Mixing and Transport of Buoyant Particles: Application to the Statfjord A Oil Spill. Ocean Sci. 10, 977–991. doi:10.5194/os-10-977-2014
Eriksen, M., Liboiron, M., Kiessling, T., Charron, L., Alling, A., Lebreton, L., et al. (2018). Microplastic Sampling with the AVANI Trawl Compared to Two Neuston Trawls in the Bay of Bengal and South Pacific. Environ. Pollut. 232, 430–439. doi:10.1016/j.envpol.2017.09.058
Eriksen, M., Mason, S., Wilson, S., Box, C., Zellers, A., Edwards, W., et al. (2013). Microplastic Pollution in the Surface Waters of the Laurentian Great Lakes. Mar. Pollut. Bull. 77, 177–182. doi:10.1016/j.marpolbul.2013.10.007
Felismino, M. E. L., Helm, P. A., and Rochman, C. M. (2021). Microplastic and Other Anthropogenic Microparticles in Water and Sediments of Lake Simcoe. J. Great Lakes Res. 47, 180–189. doi:10.1016/j.jglr.2020.10.007
Frank, Y. A., Vorobiev, E. D., Vorobiev, D. S., Trifonov, A. A., Antsiferov, D. V., Soliman Hunter, T., et al. (2021). Preliminary Screening for Microplastic Concentrations in the Surface Water of the Ob and Tom Rivers in Siberia, Russia. Sustainability 13, 80. doi:10.3390/w13223248
Free, C. M., Jensen, O. P., Mason, S. A., Eriksen, M., Williamson, N. J., and Boldgiv, B. (2014). High-levels of Microplastic Pollution in a Large, Remote, mountain lake. Mar. Pollut. Bull. 85, 156–163. doi:10.1016/j.marpolbul.2014.06.001
Frère, L., Paul-Pont, I., Rinnert, E., Petton, S., Jaffré, J., Bihannic, I., et al. (2017). Influence of Environmental and Anthropogenic Factors on the Composition, Concentration and Spatial Distribution of Microplastics: A Case Study of the Bay of Brest (Brittany, France). Environ. Pollut. 225, 211–222. doi:10.1016/j.envpol.2017.03.023
Frias, J. P. G. L., Lyashevska, O., Joyce, H., Pagter, E., and Nash, R. (2020). Floating Microplastics in a Coastal Embayment: A Multifaceted Issue. Mar. Pollut. Bull. 158, 111361. doi:10.1016/j.marpolbul.2020.111361
Gago, J., Filgueiras, A., Pedrotti, M. L., Caetano, M., and Frias, J. (2019). Standardised Protocol for Monitoring Microplastics in Seawater. Deliverable 4, 1. JPI-Oceans BASEMAN Project. doi:10.25607/OBP-605
GESAMP (2022). Guidelines for the Monitoring and Assessment of Plastic Litter in the Ocean. Available at: http://www.gesamp.org/publications/guidelines-for-the-monitoring-and-assessment-of-plastic-litter-in-the-ocean (Accessed January 24, 2022).
González-Pleiter, M., Edo, C., Velázquez, D., Casero-Chamorro, M. C., Leganés, F., Quesada, A., et al. (2020). First Detection of Microplastics in the Freshwater of an Antarctic Specially Protected Area. Mar. Pollut. Bull. 161, 111811. doi:10.1016/j.marpolbul.2020.111811
Green, D. S., Kregting, L., Boots, B., Blockley, D. J., Brickle, P., da Costa, M., et al. (2018). A Comparison of Sampling Methods for Seawater Microplastics and a First Report of the Microplastic Litter in Coastal Waters of Ascension and Falkland Islands. Mar. Pollut. Bull. 137, 695–701. doi:10.1016/j.marpolbul.2018.11.004
Gwinnett, C., and Miller, R. Z. (2021). Are We Contaminating Our Samples? A Preliminary Study to Investigate Procedural Contamination during Field Sampling and Processing for Microplastic and Anthropogenic Microparticles. Mar. Pollut. Bull. 173, 113095. doi:10.1016/j.marpolbul.2021.113095
Hamilton, B. M., Bourdages, M. P. T., Geoffroy, C., Vermaire, J. C., Mallory, M. L., Rochman, C. M., et al. (2021). Microplastics Around an Arctic Seabird colony: Particle Community Composition Varies across Environmental Matrices. Sci. Total Environ. 773, 145536. doi:10.1016/j.scitotenv.2021.145536
He, D., Chen, X., Zhao, W., Zhu, Z., Qi, X., Zhou, L., et al. (2021). Microplastics Contamination in the Surface Water of the Yangtze River from Upstream to Estuary Based on Different Sampling Methods. Environ. Res. 196, 110908. doi:10.1016/j.envres.2021.110908
Hendrickson, E., Minor, E. C., and Schreiner, K. (2018). Microplastic Abundance and Composition in Western Lake Superior as Determined via Microscopy, Pyr-GC/MS, and FTIR. Environ. Sci. Technol. 52, 1787–1796. doi:10.1021/acs.est.7b05829
Herrera, A., Raymond, E., Martínez, I., Álvarez, S., Canning-Clode, J., Gestoso, I., et al. (2020). First Evaluation of Neustonic Microplastics in the Macaronesian Region, NE Atlantic. Mar. Pollut. Bull. 153, 110999. doi:10.1016/j.marpolbul.2020.110999
Hidalgo-Ruz, V., Gutow, L., Thompson, R. C., and Thiel, M. (2012). Microplastics in the Marine Environment: A Review of the Methods Used for Identification and Quantification. Environ. Sci. Technol. 46, 3060–3075. doi:10.1021/es2031505
Hung, C., Klasios, N., Zhu, X., Sedlak, M., Sutton, R., and Rochman, C. M. (2021). Methods Matter: Methods for Sampling Microplastic and Other Anthropogenic Particles and Their Implications for Monitoring and Ecological Risk Assessment. Integr. Environ. Assess. Manag. 17, 282–291. doi:10.1002/ieam.4325
Isobe, A., Iwasaki, S., Uchida, K., and Tokai, T. (2019). Abundance of Non-conservative Microplastics in the Upper Ocean from 1957 to 2066. Nat. Commun. 10, 417. doi:10.1038/s41467-019-08316-9
Isobe, A., Uchida, K., Tokai, T., and Iwasaki, S. (2015). East Asian Seas: A Hot Spot of Pelagic Microplastics. Mar. Pollut. Bull. 101, 618–623. doi:10.1016/j.marpolbul.2015.10.042
Jambeck, J. R., Geyer, R., Wilcox, C., Siegler, T. R., Perryman, M., Andrady, A., et al. (2015). Plastic Waste Inputs from Land into the Ocean. Science 347, 768–771. doi:10.1126/science.1260352
Kaiser, D., Kowalski, N., and Waniek, J. (2017). Effects of Biofouling on the Sinking Behavior of Microplastics. Environ. Res. Lett. 12. doi:10.1088/1748-9326/aa8e8b
Karlsson, T. M., Kärrman, A., Rotander, A., and Hassellöv, M. (2020). Comparison between Manta Trawl and In Situ Pump Filtration Methods, and Guidance for Visual Identification of Microplastics in Surface Waters. Environ. Sci. Pollut. Res. 27, 5559–5571. doi:10.1007/s11356-019-07274-5
Kazour, M., Jemaa, S., Issa, C., Khalaf, G., and Amara, R. (2019). Microplastics Pollution along the Lebanese Coast (Eastern Mediterranean Basin): Occurrence in Surface Water, Sediments and Biota Samples. Sci. Total Environ. 696, 133933. doi:10.1016/j.scitotenv.2019.133933
Kooi, M., and Koelmans, A. (2019). Simplifying Microplastic via Continuous Probability Distributions for Size, Shape, and Density. Environ. Sci. Techn. Lett. 6, 551–557. doi:10.1021/acs.estlett.9b00379
Kooi, M., Nes, E. H. v., Scheffer, M., and Koelmans, A. A. (2017). Ups and Downs in the Ocean: Effects of Biofouling on Vertical Transport of Microplastics. Environ. Sci. Technol. 51, 7963–7971. doi:10.1021/acs.est.6b04702
Kukulka, T., Proskurowski, G., Morét-Ferguson, S., Meyer, D. W., and Law, K. L. (2012). The Effect of Wind Mixing on the Vertical Distribution of Buoyant Plastic Debris. Geophys. Res. Lett. 39, n/a. doi:10.1029/2012gl051116
Lattin, G. L., Moore, C. J., Zellers, A. F., Moore, S. L., and Weisberg, S. B. (2004). A Comparison of Neustonic Plastic and Zooplankton at Different Depths Near the Southern California Shore. Mar. Pollut. Bull. 49, 291–294. doi:10.1016/j.marpolbul.2004.01.020
Lenaker, P. L., Baldwin, A. K., Corsi, S. R., Mason, S. A., Reneau, P. C., and Scott, J. W. (2019). Vertical Distribution of Microplastics in the Water Column and Surficial Sediment from the Milwaukee River Basin to Lake Michigan. Environ. Sci. Technol. 53, 12227–12237. doi:10.1021/acs.est.9b03850
Li, C., Busquets, R., and Campos, L. C. (2020). Assessment of Microplastics in Freshwater Systems: A Review. Sci. Total Environ. 707, 135578. doi:10.1016/j.scitotenv.2019.135578
Li, C., Wang, X., Liu, K., Zhu, L., Wei, N., Zong, C., et al. (2021). Pelagic Microplastics in Surface Water of the Eastern Indian Ocean during Monsoon Transition Period: Abundance, Distribution, and Characteristics. Sci. Total Environ. 755, 142629. doi:10.1016/j.scitotenv.2020.142629
Lindeque, P. K., Cole, M., Coppock, R. L., Lewis, C. N., Miller, R. Z., Watts, A. J. R., et al. (2020). Are We Underestimating Microplastic Abundance in the marine Environment? A Comparison of Microplastic Capture with Nets of Different Mesh-Size. Environ. Pollut. 265, 114721. doi:10.1016/j.envpol.2020.114721
Mai, L., Bao, L.-J., Shi, L., Wong, C. S., and Zeng, E. Y. (2018). A Review of Methods for Measuring Microplastics in Aquatic Environments. Environ. Sci. Pollut. Res. 25, 11319–11332. doi:10.1007/s11356-018-1692-0
Michida, Y., Chavanich, S., Chiba, S., Cordova, M. R., Cozsar Cabanas, A., Glagani, F., et al. (2019). Guidelines for Harmonizing Ocean Surface Microplastic Monitoring Methods. Japan: Ministry of the Environment. Version 1.1. doi:10.25607/OBP-867
Moore, C. J., Moore, S. L., Leecaster, M. K., and Weisberg, S. B. (2001). A Comparison of Plastic and Plankton in the North Pacific Central Gyre. Mar. Pollut. Bull. 42, 1297–1300. doi:10.1016/s0025-326x(01)00114-x
Mora-Teddy, A. K., and Matthaei, C. D. (2020). Microplastic Pollution in Urban Streams across New Zealand: Concentrations, Composition and Implications. New Zealand J. Mar. Freshw. Res. 54, 233–250. doi:10.1080/00288330.2019.1703015
Palatinus, A., Kovač Viršek, M., Robič, U., Grego, M., Bajt, O., Šiljić, J., et al. (2019). Marine Litter in the Croatian Part of the Middle Adriatic Sea: Simultaneous Assessment of Floating and Seabed Macro and Micro Litter Abundance and Composition. Mar. Pollut. Bull. 139, 427–439. doi:10.1016/j.marpolbul.2018.12.038
Pan, Z., Liu, Q., Jiang, R., Li, W., Sun, X., Lin, H., et al. (2021). Microplastic Pollution and Ecological Risk Assessment in an Estuarine Environment: The Dongshan Bay of China. Chemosphere 262, 127876. doi:10.1016/j.chemosphere.2020.127876
Pini, A., Tomassetti, P., Matiddi, M., de Lucia, G. A., Camedda, A., Zampetti, G., et al. (2018). “Microplastic Samplings and Inverse Trajectory Recognition in the Mediterranean Sea,” in 2018 IEEE International Workshop on Metrology for the Sea; Learning to Measure Sea Health Parameters (MetroSea). Bari, Italy: IEEE, 115–119. doi:10.1109/metrosea.2018.8657848
Plastics Europe (2020). Plastics - the Facts 2020 • Plastics Europe. Available at: https://plasticseurope.org/knowledge-hub/plastics-the-facts-2020/.
Prata, J. C., da Costa, J. P., Duarte, A. C., and Rocha-Santos, T. (2019). Methods for Sampling and Detection of Microplastics in Water and Sediment: A Critical Review. Trac Trends Anal. Chem. 110, 150–159. doi:10.1016/j.trac.2018.10.029
Ramadan, A. H., and Sembiring, E. (2020). “Occurrence of Microplastic in Surface Water of Jatiluhur Reservoir,” in E3S Web of Conferences. Bandung, Indonsesia: edp sciences. doi:10.1051/e3sconf/202014807004
Razeghi, N., Hamidian, A. H., Wu, C., Zhang, Y., and Yang, M. (2021). Scientific Studies on Microplastics Pollution in Iran: An In-Depth Review of the Published Articles. Mar. Pollut. Bull. 162, 111901. doi:10.1016/j.marpolbul.2020.111901
Ruiz-Orejón, L. F., Sardá, R., and Ramis-Pujol, J. (2018). Now, You See me: High Concentrations of Floating Plastic Debris in the Coastal Waters of the Balearic Islands (Spain). Marine Pollution Bulletin 133, 636–646. doi:10.1016/j.marpolbul.2018.06.010
Ryan, P. G., Suaria, G., Perold, V., Pierucci, A., Bornman, T. G., and Aliani, S. (2020). Sampling Microfibres at the Sea Surface: The Effects of Mesh Size, Sample Volume and Water Depth. Environ. Pollut. 258, 113413. doi:10.1016/j.envpol.2019.113413
Saito, H. (2018). “Plankton Net,” in Guidelines of Ocean Observations (Tokyo, Japan: The Oceanographic Society of Japan), 6, 601EN1–9. Plankton and Benthos.
Schmidt, N., Castro-Jiménez, J., Oursel, B., and Sempéré, R. (2021). Phthalates and Organophosphate Esters in Surface Water, Sediments and Zooplankton of the NW Mediterranean Sea: Exploring Links with Microplastic Abundance and Accumulation in the marine Food Web. Environ. Pollut. 272, 115970. doi:10.1016/j.envpol.2020.115970
Scopetani, C., Esterhuizen-Londt, M., Chelazzi, D., Cincinelli, A., Setälä, H., and Pflugmacher, S. (2020). Self-contamination from Clothing in Microplastics Research. Ecotoxicology Environ. Saf. 189, 110036. doi:10.1016/j.ecoenv.2019.110036
Sembiring, E., Fareza, A. A., Suendo, V., and Reza, M. (2020). “The Presence of Microplastics in Water, Sediment, and Milkfish (Chanos Chanos) at the Downstream Area of Citarum River, Indonesia,” in Water, Air, and Soil Pollution. Springer, 231. doi:10.1007/s11270-020-04710-y
Smith, P. E., Counts, R. C., and Clutter, R. I. (1968). Changes in Filtering Efficiency of Plankton Nets Due to Clogging under Tow. ICES J. Mar. Sci. 32, 232–248. doi:10.1093/icesjms/32.2.232
So, W. K., Chan, K., and Not, C. (2018). Abundance of Plastic Microbeads in Hong Kong Coastal Water. Mar. Pollut. Bull. 133, 500–505. doi:10.1016/j.marpolbul.2018.05.066
Song, Y. K., Hong, S. H., Jang, M., Kang, J.-H., Kwon, O. Y., Han, G. M., et al. (2014). Large Accumulation of Micro-sized Synthetic Polymer Particles in the Sea Surface Microlayer. Environ. Sci. Technol. 48, 9014–9021. doi:10.1021/es501757s
Stock, F., Kochleus, C., Bänsch-Baltruschat, B., Brennholt, N., and Reifferscheid, G. (2019). Sampling Techniques and Preparation Methods for Microplastic Analyses in the Aquatic Environment - A Review. Trac Trends Anal. Chem. 113, 84–92. doi:10.1016/j.trac.2019.01.014
Suaria, G., Avio, C. G., Mineo, A., Lattin, G. L., Magaldi, M. G., Belmonte, G., et al. (2016). The Mediterranean Plastic Soup: Synthetic Polymers in Mediterranean Surface Waters. Sci. Rep. 6, 37551. doi:10.1038/srep37551
Sutton, R., Mason, S. A., Stanek, S. K., Willis-Norton, E., Wren, I. F., and Box, C. (2016). Microplastic Contamination in the San Francisco Bay, California, USA. Mar. Pollut. Bull. 109, 230–235. doi:10.1016/j.marpolbul.2016.05.077
Ta, A. T., Babel, S., and Haarstick, A. (2020). Microplastics Contamination in a High Population Density Area of the Chao Phraya River, Bangkok. J. Eng. Technol. Sci. 52, 534–545. doi:10.5614/j.eng.technol.sci.2020.52.4.6
Tamminga, M., Stoewer, S.-C., and Fischer, E. K. (2019). On the Representativeness of Pump Water Samples versus Manta Sampling in Microplastic Analysis. Environ. Pollut. 254, 112970. doi:10.1016/j.envpol.2019.112970
Teng, J., Zhao, J., Zhang, C., Cheng, B., Koelmans, A. A., Wu, D., et al. (2020). A Systems Analysis of Microplastic Pollution in Laizhou Bay, China. Sci. Total Environ. 745, 140815. doi:10.1016/j.scitotenv.2020.140815
Tranter, D., Kerr, J., and Heron, A. (1968). Effects of Hauling Speed on Zooplankton Catches. Mar. Freshw. Res. 19, 65–76. doi:10.1071/mf9680065
Uurasjärvi, E., Hartikainen, S., Setälä, O., Lehtiniemi, M., and Koistinen, A. (2020). Microplastic Concentrations, Size Distribution, and Polymer Types in the Surface Waters of a Northern European lake. Water Environ. Res. 92, 149–156. doi:10.1002/wer.1229
Viršek, M., Palatinus, A., Koren, Š., Peterlin, M., Horvat, P., and Kržan, A. (2016). Protocol for Microplastics Sampling on the Sea Surface and Sample Analysis. J. Visualized Experiments 16 (118), 55161. doi:10.3791/55161
Wang, S., Chen, H., Zhou, X., Tian, Y., Lin, C., Wang, W., et al. (2020). Microplastic Abundance, Distribution and Composition in the Mid-west Pacific Ocean. Environ. Pollut. 264, 114125. doi:10.1016/j.envpol.2020.114125
Warrack, S., Challis, J. K., Hanson, M. L., and Rennie, M. D. (2017). Microplastics Flowing into Lake Winnipeg: Densities, Sources, Flux, and Fish Exposures. PMUSER 3, 5–15. doi:10.5203/pmuser.201730578
Wong, G., Löwemark, L., and Kunz, A. (2020). Microplastic Pollution of the Tamsui River and its Tributaries in Northern Taiwan: Spatial Heterogeneity and Correlation with Precipitation. Environ. Pollut. 260, 113935. doi:10.1016/j.envpol.2020.113935
Keywords: microplastics, sampling, manta, net, review, methods
Citation: Pasquier G, Doyen P, Kazour M, Dehaut A, Diop M, Duflos G and Amara R (2022) Manta Net: The Golden Method for Sampling Surface Water Microplastics in Aquatic Environments. Front. Environ. Sci. 10:811112. doi: 10.3389/fenvs.2022.811112
Received: 08 November 2021; Accepted: 21 February 2022;
Published: 01 April 2022.
Edited by:
Wojciech Mrozik, Newcastle University, United KingdomReviewed by:
Giuseppe Suaria, National Research Council (CNR), ItalySilvia Helena Lima Schwamborn, Federal University of Pernambuco, Brazil
Copyright © 2022 Pasquier, Doyen, Kazour, Dehaut, Diop, Duflos and Amara. This is an open-access article distributed under the terms of the Creative Commons Attribution License (CC BY). The use, distribution or reproduction in other forums is permitted, provided the original author(s) and the copyright owner(s) are credited and that the original publication in this journal is cited, in accordance with accepted academic practice. No use, distribution or reproduction is permitted which does not comply with these terms.
*Correspondence: Gabriel Pasquier, gab.pasquier@gmail.com