- 1Pesticides Chemistry and Toxicology Department, Faculty of Agriculture, Kafr-El-Sheikh University, Kafr El Sheikh, Egypt
- 2Nanotechnology Center, Chemistry Department, Faculty of Science, Kafr-El-Sheikh University, Kafr El Sheikh, Egypt
- 3Department of Zoonoses, Faculty of Veterinary Medicine, Sohag University, Sohag, Egypt
- 4Department of Clinical Laboratory Sciences, College of Applied Medical Sciences, Taif University, Taif, Saudi Arabia
- 5Department of Pathology, Faculty of Veterinary Medicine, Kafrelsheikh University, Kafr El sheikh, Egypt
Pesticides are among the major organic pollutants, and their random extensive applications threaten human health and ecosystems. Clearly, detoxification of toxic insecticides from the aquatic system remains a global priority. In the present study, a zinc oxide nanocatalyst was synthesized with suitable properties to achieve complete degradation of some insecticides (dimethoate and methomyl) from aqueous media. The ZnO catalyst was used in normal and in nano-size as a part of an advanced oxidation process in the presence of H2O2 and UV rays. The complete detoxification of the tested pesticides after treatment with the most effective process (ZnO(s)/H2O2/UV) was then examined by exploring the biochemical and histopathological changes in the liver and kidneys of treated rats compared to the control. The effect of water treatment by ZnO (nano)/H2O2/UV on the water quality parameters of treated water was also investigated. Interestingly, the present study reported that the degradation rates of the investigated insecticides were faster using the nano-sized ZnO catalyst than the regular ZnO catalyst. In this respect, complete decomposition of the tested insecticides (100%) under the ZnO(s)/H2O2/UV system was achieved after 320 min of irradiation. The half-lives of the tested insecticides under ZnO(c)/H2O2/UV were 43.86 and 36.28 for dimethoate and methomyl, respectively, while under the ZnO(c)/H2O2/UV system, the half-live values were 27.72 and 19.52 min for dimethoate and methomyl, respectively. On the other hand, there were no significant changes in the biochemical and histological parameters of rats treated with remediated water when compared to the control group. The treatment of water by zinc oxide nanocatalyst improved the quality of water parameters. Collectively, advanced oxidation processes using ZnO nanocatalyst can be considered as a promising treatment technology for the complete detoxification of methomyl and dimethoate in water. However, further research is warranted for the identification of the potential breakdown products.
Introduction
Nowadays, Egypt is facing and suffering from two huge problems. The first one is the Renaissance Dam in Ethiopia which might cause a shortage in water supply and reduce Egypt’s share of the Nile water by 14.5 billion cubic meters, which can irrigate three million Fadden (Chronicles, 2013). The second problem is environmental pollution in general, and particularly, agrochemical pollutants in surface water through the intensive use of pesticides and fertilizers (Derbalah and Ismail, 2013; Derbalah et al., 2014; Dahshan et al., 2016; Abbassy, 2018; Abdelrazek, 2019; Eissa et al., 2020; Eissa et al., 2021). The pollution of surface water and wastewater has increased sharply, and it constitutes a major problem due to the extensive use of these substances (Evgenidou et al., 2007). Monitoring of pesticides in surface water showed the presence of organochlorines, and organophosphorus and carbamate insecticides in water resources in the Kafr El Sheikh Governorate (Massoud et al., 2011; Ismail et al., 2015). Dimethoate is considered to be a priority pollutant in water; first, due to the wide range of usage of this insecticide against different pests, and second, its high toxicity (Lasram et al., 2009). Insecticide residues have been detected in drinking water sources, and the development of viable methods for their removal is a global priority (Derbalah et al., 2014; Ismail et al., 2015). Methomyl is a widely used carbamate pesticide characterized by its high toxicity and can cause great damage when polluted to the environment due to its high solubility in water (57.9 g/L at 25°C) (Tomlin, 2003). Methomyl can easily contaminate both ground and surface water as a result of its low affinity for absorption by surface soil (Strathmann and Stone, 2002). It should be stressed that conventional physicochemical techniques, such as adsorption, flocculation, reverse osmosis, and extraction, merely transfer the organic contaminants from one phase to another without destroying them (Pasieczna-Patkowska et al., 2010; Zhang et al., 2012). Also, the classical biological treatment has proven to be inefficient for decomposing ROPs because of their complex molecular structure, high toxicity, and poor biodegradability (van der Werf, 1996; Kümmerer et al., 2000; de Lima et al., 2007). Therefore, alternative advanced technologies such as advanced oxidation processes are required for the effective removal of pesticides from water. Taken into account, heterogeneous photocatalysis with a semiconducting photocatalyst has recently emerged as an advanced oxidation process in the environmental decontamination method suitable for the treatment of water, aqueous wastes, and wastewater (Mills and Le Hunte, 1997; Andreozzi et al., 1999; Hyung and Kim, 2009). Nanostructured semiconductors such as zinc oxide (ZnO) and titanium dioxide (TiO2) are potential candidates for the mineralization of toxic organic compounds and hazardous inorganic constituent (Curri et al., 2003), owing to their strong oxidizing ability, that is, the hydroxyl radical (OH). Zinc oxide is a semi-conductive material that can be fabricated with various morphological forms in normal and nanoscale sizes (Kumari et al., 2010). Furthermore, zinc oxide is environmentally friendly and does not have harmful effects on living organisms (Ismail et al., 2015). Fabrication of ZnO in nanostructures can change its properties including the surface area and light absorbance (Derbalah et al., 2019). Therefore, zinc oxide nanocatalyst can be used very efficiently to eliminate pesticide residues from water compared to commercial ones (Baruah et al., 2012; Derbalah et al., 2014; Derbalah et al., 2016; Derbalah et al., 2019). However, after the remediation of pesticide residues in water, a toxicity assessment is needed to directly assess the potential hazard of original pollutants and their metabolites (Derbalah, 2009). Treating pesticide residues in water using advanced oxidation methods to break them down remains inaccurate because sometimes the decomposition products of pesticides are more toxic than the pesticides themselves. Interestingly, toxicity testing is widely used to determine the potential toxicity of a particular compound or a sample of water on biological organisms along with measuring its prevalence. In contrast to a chemical analysis, toxicity testing can detect toxic compounds on the basis of their biological activity without requiring prior knowledge about the toxic substance itself. Clearly, toxicity testing offers many advantages over a chemical analysis which requires prior knowledge of toxic substances (Leusch and Chapman, 2011).
The use of an acetylcholinesterase (AChE) biosensor seems to be the most appropriate technique for unambiguous determination of toxicity of water samples and wastewater at different stages of organophosphorus and carbamate degradation processes. The main advantages of AChE bioassays include their selective sensitivity to organophosphates toxic photoproducts and prompt response, which enable online monitoring and control of photodegradation processes (Simonian et al., 2001; Kralj et al., 2007). Fundamental, physical, and chemical water quality parameters reflect the operational status of water/wastewater treatment processes (Van Vooren et al., 2001), and therefore are important for any remediation technology, not only for the complete removal of pollutants from water but also to determine the effect of the method on the water quality parameters.
The present study attempts to synthesize ZnO (nano-size) with good properties combined with evaluating its efficiency, compared to its commercial size (natural size) apart from the advanced oxidation processes, in the presence of H2O2 and light for the total decomposition of dimethoate and methomyl in water. The present study also aimed to confirm the complete detoxification of insecticide-contaminated water after treating it with the most effective treatment (ZnO(s)/H2O2/UV) with respect to altering several biochemical parameters and reporting histopathological changes in the treated liver and kidneys of rats compared to untreated control. The effect of water treatment by ZnO (nano)/H2O2/UV on the water quality parameters of treated water was also investigated.
Materials and Methods
Chemicals
Dimethoate and methomyl (99.5% purity) were obtained from the Central Laboratory for Pesticides, Agriculture Research Centre, Giza, Egypt. Meanwhile, zinc oxide (ZnO, 99% purity) was obtained from the Oxford Laboratories Pvt. Ltd., Mumbai, India. Hydrogen peroxide solution (H2O2) [50% (w/v)] was obtained from Piochem, Cairo, Egypt. Zinc acetate dihydrate [Zn(CH3COO)2·2H2O, 99.5% purity] was obtained from Loba Chemie Pvt. Ltd., Mumbai, India. Triethylamine (TEA) [(C2H5)3N, 99% purity] was obtained from Alpha Chemika, Mumbai, India. Ethyl alcohol (ethanol, C2H5OH, 95% purity) was obtained from Piochem, Cairo, Egypt. Zinc nitrate hexahydrate [Zn(NO3)2·6H2O, 99% purity] was obtained from Winlab, India.
Synthesis of ZnO Nonacatalyst
Zinc acetate dihydrate (ZnAc2.2H2O) and a capping agent (triethanolamine TEA) were mixed at a molar ratio of 5:3 in ethanol, typically with 3.2926 g of zinc acetate dihydrate and 1.255 ml of triethylamine. The mixed solution was stirred for 60 min at 50–60 °C in a condensation system until gaining a cloudy white appearance, indicating the growth of ZnO nanoparticles. The solution was then centrifuged for 10 min to separate the precipitate from the ethanol layer. The ethanol portion was removed by decantation, and the precipitate was washed with ethanol and dried under vacuum (El-Kemary et al., 2010). Then the precipitate was dried at 50°C and calcined at 400°C for 4 h to obtain a white powder of ZnO nanoparticles. Physical characterizations of the synthesized and commercial ZnO were performed using scanning electron microscopy (SEM; JEOL Ltd., Tokyo, Japan; JSM 6510) and transmission electron microscopy (TEM; JEOL; JEM-2100). A Rigaku-D/max 2500 diffractometer (Rigaku, Tokyo, Japan) with Cu-Kα radiation (λ = 0.15418 nm) was used to measure X-ray diffraction (XRD) for crystallization identification solid diffuse reflectance. The UV-Vis spectroscopy technique was employed to characterize the electronic structure of ZnO nanoparticles.
Photocatalytic Degradation of Tested Insecticides in Water
This experiment was designed to evaluate the efficiency of the advanced oxidation processes for the removal of dimethoate and methomyl from water using zinc oxide in either normal (commercial) or nano (synthesized) size mixed with H2O2 in the presence of UV light. The test solution was made by pouring 50 ml distilled water into a 100 ml flask, and then adding the required amount of dimethoate or methomyl that achieves a final concentration of 5 mg/L in a final volume of 100 ml while stirring the solution. Then the amount of zinc oxide required to prepare a concentration of 300 mg/L in a final volume of 100 ml was carefully added to the flask and shaken (Derbalah et al., 2014; Ismail et al., 2015; Derbalah et al., 2016). After that, the amount of hydrogen peroxide required to achieve a concentration of 20 mg/L in a final volume of 100 ml is then added (Derbalah and Ismail, 2013; Derbalah et al., 2014; Ismail et al., 2015; Derbalah et al., 2016). Using distilled water, the final volume of the solution in the flask was set at 100 ml. The pH of the solution was then adjusted to 7 using a Jenway pH/mV/temperature meter, Model 3510, which is considered the optimum pH for the zinc oxide catalyst (Ahmad et al., 2022). The solution was left in the dark for 30 min to ensure maximum pesticide adsorption on the ZnO surface. The dimethoate or methomyl solution was then exposed to UV light from 19 cm away (Laoufi NA, 2009). The solutions of the irradiated samples were removed at regular intervals (0, 10, 20, 40, 60, 80, 160, 320, and 360 min) for an HPLC analysis after the irradiation started (Derbalah, 2009). A blank experiment was carried out in the absence of light to assess abiotic loss of dimethoate or methomyl, as previously mentioned (Hansson et al., 2012). All of the experiments were carried out three times, with the average results being reported.
HPLC Analysis
The irradiated samples were analyzed directly by an HPLC system. For dimethoate, a mixture of acetonitrile and distilled water (60:40) was used as the mobile phase under the isocratic elution mode. The flow rate of the mobile phase was maintained at 1 ml min-1 and the column type used was (i.d. 4.6 mm; length 250 mm) filled with Wakosil-II 5 C18–100 (Wako). The used detector was UV at a wavelength of 210 nm, and the retention time was 3.30 min (Evgenidou et al., 2006). For the methomyl analysis, a mixture of acetonitrile and distilled water (20:80) was used as the mobile phase in the isocratic elution mode. The flow rate of the mobile phase was then maintained at 0.7 ml min−1. The HPLC system used a Zorbax C8 column (250 mm × 4.6 mm × 5 µm) with a UV detector at a wavelength of 231 nm and a retention time of 3.84 min (Tamimi et al., 2008). The degradation efficiency (%) was calculated as follows (Eq. 1):
where C0 is the initial concentration of insecticide, and C is the concentration of insecticide after photoirradiation (Rahmayeni et al., 2015).
To determine the degradation rate, plots of the natural log of the concentration over time were made. The degradation rate constant (slope), k, was calculated from the first-order rate equation (Eq. 2), as follows:
where Ct represents the concentration of the insecticide at time t, C0 represents the initial concentration, and k is the degradation rate constant. When the concentration falls to 50% of its initial concentration, the half-life (t1/2) was estimated as shown in Eq. 3 (Monkiedje and Spiteller, 2005).
Toxicity Test
Ethical Statement
The ethical approval of the present study was performed according to the ethical standards of the Faculty of Veterinary Medicine, Kafrelsheikh University, Egypt, which complies with all relevant Egyptian legislations. The ethical approval code number of the study is KFS-2019/4.
Treatment of Rats
Adult Wister male rats (Sprague Dawley) 8 weeks of age and 80–100 g in weight obtained from the Faculty of Veterinary Medicine, Kafrelsheikh University were used. Rats were housed in polypropylene cages under standard conditions with free access to drinking water and food. The rats were kept in a temperature-controlled room for 12 h (Korsrud et al., 1972) with 12-h light and dark cycles. The rats were given a standard diet, as described (Korsrud et al., 1972). Before treatment, rats were left for 2 weeks during feeding for adaptation. The toxicity protocol used in this study states the following: 1 ml water containing dimethoate or methomyl after being treated with ZnO(s)/H2O2/UV) for six hours was injected as oral dose to the rats once, and then they were kept under observation for 28 days before carrying out biochemical and histopathological tests. All water samples after remediation with ZnO(s)/H2O2/UV were stored for 1 week in a clean brown glass in refrigerator until administration to rats. The dose of the used insecticides given to the treated rats if no degradation was induced was equal to 500 μg/kg body weight. Rats were administered with the dose by using an injection syringe with a ball in the front to prevent the occurrence of bleeding or injury to the animal. The animals were divided into four groups (six rats of each). Two groups were orally administered one time with 1 ml of water contaminated with methomyl and dimethoate after remediation (6 h) with ZnO(s)/H2O2/UV. The third group was orally administered one time with 1 ml water containing ZnO(s)/H2O2 without insecticides. The fourth group of rats was treated with pure water only and considered as the control. After 28 days of oral administration, biochemical and histopathological tests were carried out to confirm the complete detoxification of insecticides in the treated water and to assess the toxicity of ZnO(s)/H2O2 without the tested insecticides relative to control. The animals were observed for clinical signs of toxicity (macroscopic investigation) once a day throughout the entire observation period, and the effects were observed in all six animals. All animal studies were approved by our Institutional Animal Ethics Committee.
Biochemical Assays
Blood samples were centrifuged at 4,500 rounds per minute (rpm) for 20 min, and the serum was collected for determination of the enzymatic activity. The colorimetric methods of Waber and Dtsch (1966), Schirmeister (1964), Reitman (1957), and Habig (1974) were used for determining the level of acetylcholinesterase, glutamic-pyruvic transaminase (GPT), glutamic-oxaloacetic transaminase (GOT), and glutathione-s-transferase (GST), respectively, using the kits.
Histopathological Examination
All rats were anesthetized and sacrificed before performing the postmortem examination, and all lesions were recorded. Specimens from the liver and kidneys were collected and maintained in neutral buffered formalin (10%) for histopathological examination (Derbalah and Ismail, 2013; Ismail et al., 2015). The specimens were then dehydrated in ascending grades of alcohols, cleared in xylene, embedded in paraffin wax, sectioned at 4 μm, stained with hematoxylin and eosin (HE) stains, and examined by a light microscope (Bancroft et al., 2013).
Water Quality Parameters Assessment
An experiment was carried out to assess the effect of ZnO (nano)/H2O2/UV treatment on the water quality parameters of treated fresh and drainage waters. Water samples were collected in pre-cleaned polyethylene bottles from the Al-Gharbiya main drain (drainage water) and the River Nile at Fowa (fresh water). After collection, the samples were immediately placed in dark boxes and processed within 6 h of collection. The samples were divided into two parts after filtration. The first was kept without treatment, and the second was treated with the ZnO (nano)/H2O2/UV system, as mentioned before. The treated and untreated water samples underwent a water quality analysis to evaluate the effects of ZnO (nano)/H2O2/UV on the water quality. The water samples were analyzed for their major physicochemical water quality parameters, such as pH, electrical conductivity (EC), total dissolved solids (TDS), chemical oxygen demand (COD), sulfate (SO42-), and biological oxygen demand (BOD), according to the method described (AMERICAN PUBLIC HEALTH ASSOCIATION 2005), at the Water Pollution Control Department, National Research Centre, Dokki, Giza, Egypt. All experiments were repeated three times, and the average values are reported.
Results and Discussion
Characterization of Commercial and Fabricated ZnO
Figure 1 shows typical scanning electron images of synthesized and commercial ZnO. Results indicate that the shape of the synthesized ZnO is spherical with a diameter in the range of 30–49 nm (Figure 1A). The commercial ZnO (Figure 1B) appears as randomly oriented hexagonal prisms with the diameter ranging from 34 to 119 nm and with length ranging from 169 to 399 nm. Additional studies on the structures of synthesized and commercial ZnO were conducted using transmission electron microscopy.
Figure 2A shows transmission electron microscopy (TEM) images of the synthesized ZnO(s). This image shows that the shape of most of these particles is spherical with small amount of hexagonal diameters. The diameter of the synthesized ZnO(s) lies in the range of 12.48–26.12 nm. Figure 2B reflects the shape of the commercial ZnO which appears as nanorods (nanowire) with hexagonal diameters in the range of 30–83.87 nm with length in the range of 70.96–406.45 nm. Figure 3A shows the XRD diffraction pattern of ZnO nanoparticles. The peaks are indexed as 31.4° (100), 34.54° (002), 35.90° (101), 56.27° (110), 62.54° (103), and 67.64° (112), respectively. All diffraction peaks of the sample correspond to the characteristic hexagonal wurtzite structure of ZnO nanoparticles (Joint Committee on Powder Diffraction Standards (2000); diffraction data file). Similar X-ray diffraction patterns were reported by Pung et al. (2012) and Chena et al. (Chena). The average particle size of ZnO nanoparticles was found to be in the range of 34–39.7 nm using the Scherer equation (Culity, 1987). The diffraction patterns corresponding to impurities were found to be absent. This proves that pure ZnO nanoparticles were as-synthesized. However, the XRD patterns of the nanoparticles are considerably broadened due to the very small size of these particles. The strong and narrow diffraction peaks indicated that the product has good crystallinity (Samuel, 2009). The X-ray diffraction pattern of the dried commercial ZnO is shown in Figure 2B. The peaks are centered at 31.82° (100), 34.54° (002), 36.42° (101), 47.22° (102), 56.29° (110), 62.57° (103), and 67.66° (112), respectively. Calculation of the particle size by the Debye–Sheerer equation revealed that the average of the commercial ZnO ranges from 61.66 to 75.8 nm. Figures 4A,B show FTIR spectra of the as-synthesized ZnO (NPs). Infrared studies were carried out in order to ascertain the purity and nature of the metal nanoparticles. Metal oxides generally give absorption bands in the fingerprint region, that is, below 1,000 cm−1, which arises from inter-atomic vibrations. The peaks observed in Figure 4A at 3455 and 1,083 cm−1 are may be due to O-H stretching and deformation, respectively, assigned to the water adsorption on the metal surface. The peaks at 1634 and 537 cm−1 correspond with Zn-O stretching and deformation vibration, respectively. The metal–oxygen frequencies observed for the respective metal oxides are in accordance with literature values (Markova-Deneva, 2010). Parthasarathi and Thilagavathi (2011) reported that similar FTIR spectra were observed in ZnO nanoparticles in their investigation. Figure 4B shows the observed peaks of the as-received commercial ZnO (nanoparticles); two peaks at 3441 and 1,089 cm−1 could be due to O-H stretching and deformation, respectively, assigned to the adsorbed water on the metal surface. The peaks at 1631 and 537 cm−1 correspond with Zn-O stretching and deformation vibration, respectively.
The room temperature and UV-Vis absorption spectra of an aqueous solution semiconductor ZnO for as-synthesized and as-received were measured and are shown in Figures 5A,B. ZnO particles were dispersed in deionized water with a suitable concentration, and then the solution was used to perform the UV-Vis measurement. The spectrum shows an absorption peak of the as-synthesized ZnO at a wavelength of 368.5 nm, Figure 5A, and at 376.2 nm, Figure 5B, of the as-received ZnO which can be assigned to the intrinsic band gap absorption of ZnO due to transition of electrons from the valence band to the conduction band (Yu et al., 2006). The band gap energy (E) was calculated as per the literature report using the following equation (Hoffmann et al., 1995):
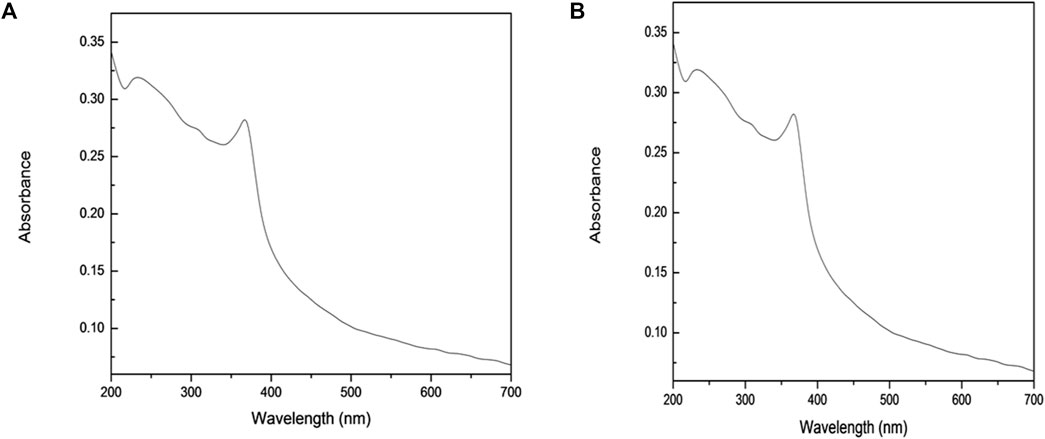
FIGURE 5. UV-Vis absorption spectra of an aqueous solution of (A) synthesized and (B) commercial ZnO.
where h is Plank’s constant (6.625 × 10–34 Js), c is the speed of light (3.0 × 108 m/s), and λ is the wavelength (m). According to this equation, the band gap of the synthesized ZnO is 3.36 eV, while the band gap of the as-received ZnO is 3.29 eV. The wide band gap energy of semiconductor nanoparticles is more reactive in a photocatalytic degradation of the organic pollutants (Dhal et al., 2015).
Photocatalytic Degradation of Dimethoate
The ZnO(s)/H2O2/UV system afforded the complete (100%) degradation of the tested insecticides (dimethoate and methomyl), followed by the ZnO(c)/H2O2/UV system, after 320 min of irradiation (Figure 6). The half-lives of methomyl were 35.36 and 19.52 under the ZnO(c)/H2O2/UV and ZnO(s)/H2O2/UV systems, respectively (Table 1). The half-lives of dimethoate were 44.14 and 26.25 min under the ZnO(c)/H2O2/UV and ZnO(s)/H2O2/UV systems, respectively (Table 1). The degradation of the tested insecticides under dark conditions using different systems was negligible than degradation under light conditions (data not published). Photocatalytic degradation by a semiconducting nanocatalyst is mainly considered as a potential and effective technology for the mineralization of pesticides into environmentally friendly compounds. In addition, nanoparticles have emerged as a sustainable alternative to conventional bulk materials, as robust, high surface area, heterogeneous photocatalysts and catalyst supports. In this study, the degradation rate of dimethoate and methomyl was greatly enhanced by irradiation under ZnO(s)/H2O2/UV relative to ZnO(c)/H2O2/UV. This is may be due to the fact that stabilized nanoparticles offer a much greater surface area in a small particle size and reactivity, which leads to a higher generation rate of hydroxyl radicals relative to the normal particles (Dhal et al., 2015) and subsequently a higher degradation rate of organic pollutants. Our present results of photodegradation of dimethoate are in harmony with the finding of many researchers (Tamimi et al., 2008; Massoud et al., 2011; Juang and Chen, 2014), who reported that the total disappearance of the dimethoate parent compound and other organophosphorus insecticides degraded was more than 90% by using the advanced oxidation processes system (photocatalysts in normal and nano-size) under UV radiation.
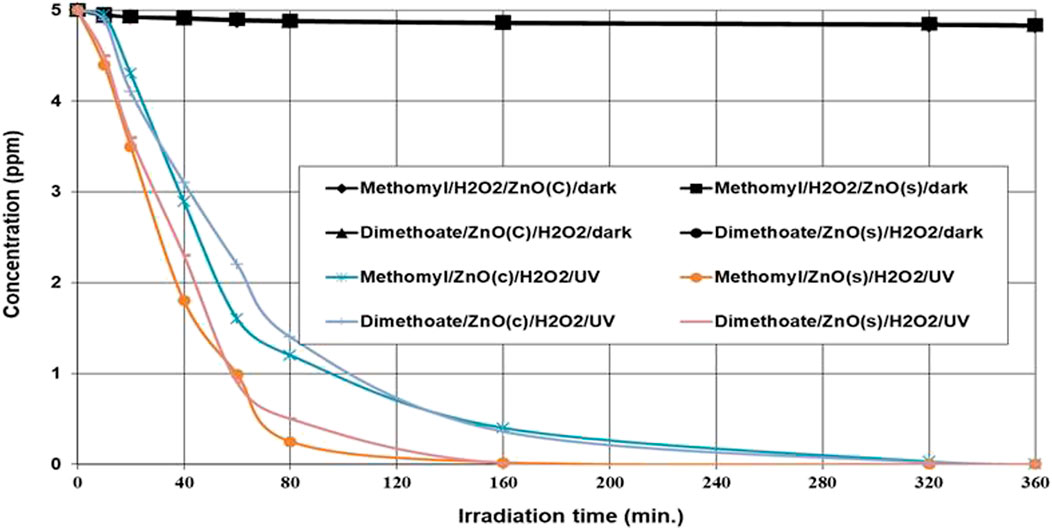
FIGURE 6. Photocatalytic degradation of dimethoate and methomyl at an initial concentration of 5 ppm under ZnO(s)/H2O2/UV and ZnO(c)/H2O2/UV systems.

TABLE 1. Calculated degradation rate constant and half-life values of dimethoate and methomyl under ZnO(c)/H2O2/UV and ZnO(s)/H2O2/UV systems.
In this study, dimethoate and methomyl were highly degraded by ZnO, possibly because zinc oxide is very reactive, the surface active sites are easily accessible to the substrate molecules, and diffusional resistance, which lead to the high generation of hydroxyl radicals by this system. The high hydroxyl radical generation rate is due to many reasons. The first reason is the ability of the ZnO semiconductor to act as a sensitizer in the presence of light and consequently enhance the photodegradation of methomyl or dimethoate. When the energy irradiating the conduction band is a greater than the band gap, valence band electrons are promoted to the conduction band, leaving a hole behind (Eq. 5). These electron (e-cb)–hole (h + vb) pairs can either recombine (Eq. 6) or interact separately with other molecules. Holes in the ZnO valence band can oxidize adsorbed water or hydroxide ions to produce •OH radicals (Eq. 7). Electrons in the conduction band on the catalyst surface can reduce molecular oxygen to the superoxide anion (Eq. 8). This radical may form hydrogen peroxide or hydroperoxy radicals (•OOH) (Eqs 9, 10). The second reason involves the addition of a hydrogen oxidant, which can enhance the degradation of the tested insecticides by acting as an electron acceptor, consequently promoting change separation and producing •OH radicals, according to Eqs 11, 12 (El-Kemary et al., 2010). After that, the hydroxyl radicals, which have a high degradation ability, attack methomyl or dimethoate to form intermediate products. These intermediates react with more hydroxyl radicals until methomyl or dimethoate is mineralized to CO2 and H2O (Eq. 13) (Hoffmann et al., 1995; Kaneco et al., 2007).
Advanced oxidation processes, especially those with nanocatalysts (Massoud et al., 2021), are promising remediation technologies for removing dimethoate or methomyl from water resources and are suitable for highly contaminated water, such as drainage or wastewater, due to the faster degradation rate via its great degradation power relative to bioremediation, which takes a long time, requires special environmental conditions, and is limited to biodegradable compounds (Derbalah et al., 2014). Although nanomaterials present seemingly limitless possibilities, they bring new challenges to understanding, predicting, and managing potential safety and health risks to workers. However, more research is needed to determine the key physical and chemical characteristics of nanoparticles that determine their hazard potential (Dhal et al., 2015).
Toxicity Assessment
Biochemical Parameters
The complete detoxification of the tested insecticides in water samples treated with the most effective treatments ZnO(s)/H2O2/UV was confirmed by measuring the effect of these treated samples on some biochemical parameters (AChE, GPT, GOT, and GST) relative to control in treated rats. The results showed that there were no significant differences in cholinesterase, GPT, GOT, and GST levels in the rats treated with water samples after remediation when compared to control (Table 2). Moreover, the effect of ZnO(s)/H2O2 itself without the tested insecticides on the same biochemical parameters to reflect its safety showed no significant differences in all biochemical parameters when compared to control.

TABLE 2. Effect of ZnO(nano)/H2O2/UV with and without dimethoate and methomyl on the activity of some biochemical parameters in rats.
The Histopathological Changes
The complete detoxification of dimethoate and methomyl in water treated with ZnO(s)/H2O2/UV was confirmed with respect to histopathological changes in the liver and kidneys of treated rats compared to untreated control ones. Liver of control rats showed basic features of hepatic lobule with centrally located central vein, and the hepatocytes were arranged in cords separated from each other by small blood spaces called hepatic sinusoids and the portal area containing the bile duct, hepatic artery, and portal vein (Figure 7A). The liver of rats treated with water contaminated with dimethoate after remediation with ZnO(s)/H2O2/UV showed focal accumulation of mononuclear inflammatory cell infiltration in the portal area accompanied with moderate hepatocellular cytoplasmic vacuolation (Figure 7B). Meanwhile, liver of rats treated with water contaminated with methomyl after remediation with ZnO(s)/H2O2/UV showed slight hepatocellular degeneration and sinusoidal congestion (Figure 7C). Liver of rats treated with water containing ZnO(s)/H2O2/UV without any insecticides showed mild vacuolation in the cytoplasm of the hepatocytes (Figure 7D). The kidneys of control rats showed normal cortex and medulla. The cortex is composed of glomerular tuft of capillaries surrounded by Bowman’s capsule forming glomeruli which are dispersed throughout, along with the proximal and distal convoluted tubules. The medulla is composed mainly of collecting ducts and loop of Henle (Figure 8A). Kidneys of rats treated with water contaminated with dimethoate after remediation with ZnO(s)/H2O2/UV showed slight to moderate changes in the form of focal interstitial nephritis with focal accumulation of mononuclear inflammatory cells in between and round the degenerated renal tubules with slight tubular dilatation. Tubular basophilia, with basophilic tubules accompanied with few single-cell necrosis, was also observed in the abovementioned lesion (Figure 8B). Kidneys of rats treated with water contaminated with methomyl after remediation with ZnO(s)/H2O2/UV showed mild interstitial mononuclear cell infiltration and slight congestion of the intertubular blood vessels as well as slight tubular dilatation (Figure 8C). Kidneys of rats treated with water containing ZnO(s)/H2O2/UV alone without dimethoate or methomyl showed almost the same histologic structure like that of the control group, except the observed slight renal tubule dilatation (Figure 8D).
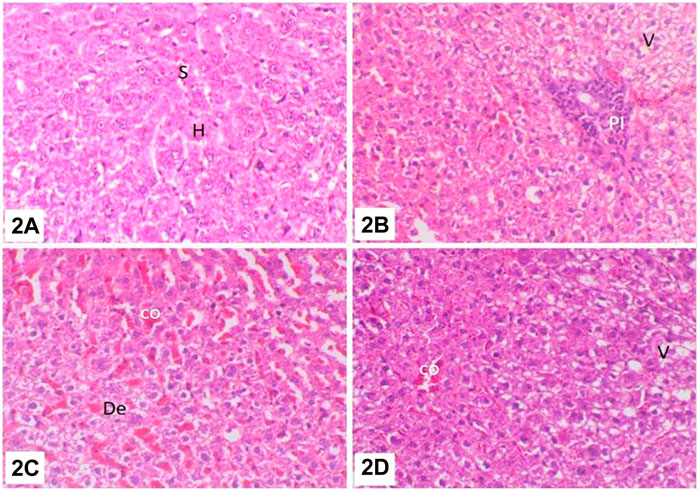
FIGURE 7. (A) Liver of control untreated rats: hepatic sinusoid (S) and hepatocytes (H); (B) liver of rats treated with water contaminated with dimethoate after remediation with ZnO(s)/H2O2/UV: hepatocellular vacuolar degeneration (V) and portal mononuclear cell infiltration (PI); (C) liver of rats treated with water contaminated with methomyl after remediation with ZnO(s)/H2O2/UV: sinusoidal congestion (co) and hepatocellular degeneration (De); (D) liver of rats treated with water containing ZnO (s)/H2O2 without any insecticides: hepatocellular vacuolar degeneration (V) and sinusoidal congestion (co).
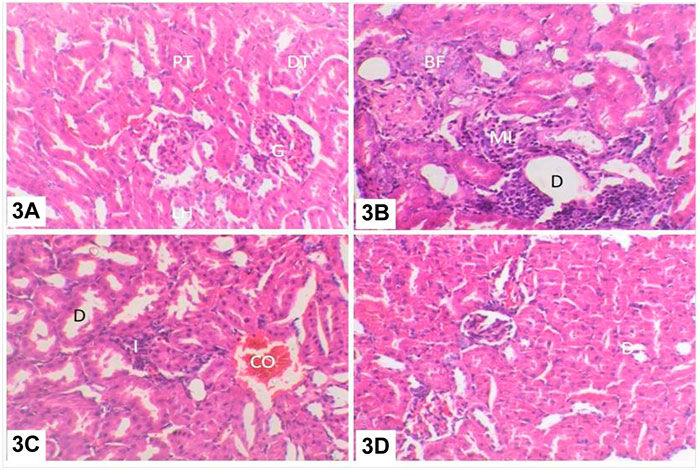
FIGURE 8. (A) Kidneys of control rats: glomerulus (G), proximal convoluted tubules (PT), and distal convoluted tubules (DT); (B) kidneys of rats treated with water contaminated with dimethoate after remediation with ZnO(s)/H2O2/UV: intertubular mononuclear cell infiltration (MI), renal casts (RC), tubular dilatation (D), and tubular basophilia (BF); (C) kidneys of rats treated with water contaminated with methomyl after remediation with ZnO(s)/H2O2/UV: tubular dilatation (D) and venous congestion (CO); (D) kidneys of rats treated with water containing ZnO (s)/H2O2 without any insecticides: tubular dilatation (D).
The total detoxification of pesticide-contaminated water after remediation is considered a limiting factor in the evaluation of the efficacy of any remediation technologies. Treated water samples after remediation had no significant effect on the activity of the measured biochemical parameters and histology in the kidney or liver of treated rats when compared to the control which implies that dimethoate or methomyl and their toxic metabolites were completely detoxified (Kralj et al., 2007). Also, the results confirmed that the ZnO nanocatalyst chemical was safe for human health (Derbalah and Ismail, 2013; Ismail et al., 2015). The results showed that the toxicity of treated water, including organic pollutants and advanced oxidation intermediates, was negative, and it can be considered as the key factor to evaluate the applicability of AOPs to improve water quality parameters (Abd El-Gawad, 2008). Advanced oxidation processes, especially using nanocatalysts, are promising remediation technologies for removing dimethoate or methomyl from water sources. Advanced oxidation processes, especially using nanocatalysts, can be a more suitable remediation technology for water contaminated with high concentration, such as drainage or wastewater, due to its faster degradation rate via its great degradation power than bioremediation that takes a long time, needs special environmental conditions, and is limited to those compounds that are biodegradable (Borm and Müller-Schulte, 2006).
It is well known that histopathological alterations are considered a rapid method to estimate the effects of irritants and chemicals in various tissues and organs, especially the in the liver and kidneys (Dutta et al., 1993; Bernet et al., 1999). To evaluate the efficacy of different tested remediation techniques in removing dimethoate and methomyl from fresh water, a toxicity assessment was carried out with respect to the biochemical parameters and the histopathological changes in rats. Exposure to dimethoate and methomyl can cause different histopathological changes in the liver and kidneys (Wafa et al., 2011). The changes in the liver and kidneys associated with dimethoate and methomyl have been attributed to their induced oxidative stress, lipid peroxidation, and the resultant free radical accumulation (El-Demerdash et al., 2013). In the present study, there were no significant but only mild alterations in the liver and kidneys of rats treated with fabricated nanoparticles. Treated rats with dimethoate or methomyl after remediation showed moderate hepatocellular cytoplasmic vacuolation and a focal inflammatory reaction in the portal area in the liver; focal interstitial nephritis, degenerated renal tubules, and tubular basophilia of renal tubules lining the epithelium which are considered as mild retrogressive degenerative changes indicate non-harmful effects of dimethoate or methomyl after remediation in comparison with the toxic effect of the exposure to dimethoate and methomyl on the liver and kidneys without remediation as described by Sharma et al. (2005), and it can be considered as an adaptive physiological response attempting to limit cell damage that could occur by toxic insecticides or its metabolites. The inflammatory reaction observed in the liver and kidneys of rats treated with water contaminated with methomyl after remediation with ZnO(s)/H2O2/UV is considered a defensive response of the tissues against the oxidative stress-induced injury imposed by methomyl (Rahm et al., 2017). Tubular basophilia is considered a feature of renal regenerative changes, and tubules lined by cells with basophilic cytoplasm exhibit increased replication rate than tubules lined by epithelial cells with normal eosinophilic appearance (Peter et al., 1986). This condition may be considered as renal tubule cell regeneration after various forms of tubular epithelial cell injury, as seen in coagulate necrosis induced by nephrotoxic chemicals such as mercuric chloride or hexachlorobutadine (Konishi and Ward, 1989) or secondary to the vascular damage, probably through ischemia and/or ischemia/reperfusion (Tochitani et al., 2016), or it can be considered as a sign of compensatory tubular hyperplasia (Descotes et al., 1996). The obtained results indicated low toxicity of ZnO nanocatalyst on the liver and kidneys, as reported (Mebert et al., 2017), and therefore, this verify the safety in the use of ZnO(s)/H2O2/UV in remediation of water for human health. It could be concluded that nanocatalyst itself did not induce any significant toxicity on treated rats with respect to biochemical and histological alterations in the treated rats. It is very important to note that toxicity testing in this study is insufficient to assess the safety of treated water for humans because exposure time was limited, exposure conditions did not take into account variability between species, and the measured end points were limited. In addition, this study focused on the disappearance and crash of parent compounds but not mineralization. It is also clear that complete detoxification of the compounds under study and their breakdown products has been confirmed, but the breakdown products have not been identified. Therefore, further studies are needed to determine the potential breakdown and to identify the main physical and chemical properties of nanoparticles that determine their potential hazards.
Effect of ZnO (nano)/H2O2/UV on Water Quality Parameters
In the present study, the effect of the photocatalytic oxidation of the tested insecticides in water using ZnO (nano)/H2O2/UV on the quality parameters of different treated water resources (fresh and drainage waters) was investigated. The data revealed that water examined from different sources after treatment showed considerable variations with respect to their physical characteristics. Al-Gharbiya main drain water was characterized by an increase in pH from 6.3 to 7.2, while the pH changed in the River Nile water at Fowa from 7.5 to 7.9 after treatment with the ZnO (nano)/H2O2/UV system, as shown in Table 3. The EC of the Al-Gharbiya main drain water increased from 738 to 863 μs, while that of River Nile water at Fowa increased from 450 to 460 μs after treatment with the ZnO (nano)/H2O2/UV system. The TDS increased from 431 to 520 mg/L and from 270 to 253 mg/L in the Al-Gharbiya main drain water and River Nile water at Fowa, respectively, after treatment with the ZnO (nano)/H2O2/UV system. The EC and TDS were found to be higher in the Al-Gharbiya main drain water and the River Nile water at Fowa after treatment. However, the TDS and EC after treatment were still low compared with the tolerance limit of drinking water (1,200 ppm) and drainage water (2000 ppm). The measured chemical water quality parameters varied in the examined water from different sources (Table 3). The COD of the Al-Gharbiya main drain water greatly decreased from 4530 to 231 mg/L, while that of the River Nile water at Fowa decreased from 839 to 80.0 mg/L after treatment, as shown in. The BOD of River Nile water at Fowa and Al-Gharbiya main drain water was reduced from 320 to 11 and from 97.2 to 18.31, respectively, after treatment, as shown in . The SO4 level of Al-Gharbiya main drain water slightly increased from 20 to 25 mg/L, while that of River Nile water at Fowa increased from 7.10 to 8.7 mg/L after treatment, as shown in .

TABLE 3. Physiochemical parameters of the drainage and fresh water before and after treatment with ZnO(nano)/H2O2/UV.
Emerging contaminants in wastewater effluents and drinking water influents have become a cause for concern in terms of potential impacts on human health. The application of advanced oxidation processes to improve water quality has been effectively demonstrated, with ever-increasing applications worldwide (Gilmour, 2012). Depending on the characteristics of the wastewater, many contamination criteria or standards, such as COD, BOD, total organic carbon (TOC), and TSS, are defined by the quality of the wastewater (Gupta et al., 2009). The ability of any method to treat wastewater is usually quantified by the decrease in the level of one or more of the water quality parameters mentioned before.
In this study, the measured water chemical quality parameters in both types of water were reduced after treatment with ZnO (nano)/H2O2/UV to levels lower than the tolerance limit of drinking and drainage water (wastewater), according to Egyptian Government Law 48 (Egyptian Association Of Environmental Affair Law 48, 1982). Additionally, the physical water quality parameters after remediation with ZnO (thin film)/H2O2/UV showed that the pH, EC, and TDS of the water samples from all sources varied within the permissible limits (Gupta et al., 2009). Therefore, the used photochemical method [ZnO (nano thin film)/H2O2/UV] is effective for pollutant removal and improving the water quality parameters. The reduction of the COD resulted from the reduction of the TOC in water, which may be due to the treatment of water with the ZnO (thin film)/H2O2/UV system leading to the degradation and mineralization of organic compounds and subsequently reducing the COD (Hansson et al., 2012). Finally, we conclude that the measured water quality parameters (pH, EC, TDS, BOD, and COD) were improved in both types of water after treatment with ZnO (nano thin film)/H2O2/UV. The SO42− levels were increased, possibly due to the efficiency of water treatment with ZnO (thin film)/H2O2/UV, which led to the mineralization of organic compounds (such as dimethoate) to their inorganic salts (Tomašević et al., 2007). An analysis of the physical water quality parameters showed that the pH, EC, and TDS varied within the permissible limits in all sources (Gupta et al., 2009) and were in compliance with Egyptian Government Law 48 (Egyptian Association of Environmental Affair Law 48). Taken into account, TDS and EC are considered to be indicators of the total amount of mobile charged ions, including minerals, salts, or metals, dissolved in a given volume of water; therefore, the increase in their levels after treatment may be due to mineralization of the organic compounds of the tested insecticide to its inorganic salts by ZnO (nano)/H2O2/UV.
Conclusion
Given the abovementioned information, AOPs could be a promising strategy for complete detoxification of the tested insecticides in contaminated water, especially using ZnO nanocatalysts. Detoxification testing (biochemical and histological tests), by exposing the treated water to a sensitive target, is an expressive procedure for the complete removal of pesticide toxicity from the treated water compared to relying on fully degraded pesticides. With this in mind, a pesticide can be completely destroyed, but its decomposition products can be more toxic than the compound itself, which illustrates the importance of fully evaluating detoxification. The water quality analysis confirmed that the used remediation method besides its efficacy in insecticides removal has also improved water quality parameters.
Data Availability Statement
The original contributions presented in the study are included in the article/Supplementary Material, further inquiries can be directed to the corresponding author.
Ethics Statement
The animal study was reviewed and approved by the ethical standards of Ethics Committee of the Faculty of Veterinary Medicine, Kafrelsheikh University, Egypt, which complies with all relevant Egyptian legislations. The ethical approval code number of the study is KFS-2019/4.
Author Contributions
Conceptualization: AM, IE-M, MUS, MHS, KA, EE, and AD. Data curation: MUS and MHS. Formal analysis: IE-M, MUS, AD, and MHS. Funding acquisition: MUS and MHS. Investigation: AM, AD, IE-M, MUS, and EE. Methodology: AM, IE-M, MUS, and AD. Project administration: AM and IE-M. Resources: MHS. Software: EE. Supervision: AM, AD, KA, and EE. Validation: AM, AD, and EE. Visualization: AM, EE, and AD. Writing—original draft: IE-M, MUS, MHS, KA, EE, and AD. Writing—review and editing: AM, MHS, EE, and AD. All authors have read and agreed to the published version of the manuscript.
Funding
This work was supported in a part by the Taif University Researchers Supporting Program (project number: TURSP-2020/153), Taif University, Saudi Arabia.
Conflict of Interest
The authors declare that the research was conducted in the absence of any commercial or financial relationships that could be construed as a potential conflict of interest.
Publisher’s Note
All claims expressed in this article are solely those of the authors and do not necessarily represent those of their affiliated organizations, or those of the publisher, the editors, and the reviewers. Any product that may be evaluated in this article, or claim that may be made by its manufacturer, is not guaranteed or endorsed by the publisher.
Acknowledgments
The authors would like to thank all who contributed to conduct this study and supported it.
References
Abbassy, M. M. S. (2018). Distribution Pattern of Persistent Organic Pollutants in Aquatic Ecosystem at the Rosetta Nile branch Estuary into the Mediterranean Sea, North of Delta, Egypt. Mar. Pollut. Bull. 131, 115–121. doi:10.1016/j.marpolbul.2018.03.049
Abd El-Gawad, H. A. (2008). Destruction of Toxic Organic (Phenol) Compounds Using Advanced Oxidation Process. Sci. Bull. Fac. Engin. Ain Shams Univ. 43 (3), 1–10. doi:10.21608/fjard.2008.197483
Abdelrazek, S. (2019). Monitoring Irrigation Water Pollution of Nile Delta of Egypt with Heavy Metals. Alexandria Sci. Exchange J. 40 (July-September), 441–450. doi:10.21608/asejaiqjsae.2019.50350
Ahmad, M., Abbas, G., Tanveer, M., and Zubair, M. (2022). ZnO and TiO2 Assisted Photocatalytic Degradation of Butachlor in Aqueous Solution under Visible Light. Eng. Proc. 12 (1), 77. doi:10.3390/engproc2021012077
Alves de Lima, R. O., Bazo, A. P., Salvadori, D. M., Rech, C. M., de Palma Oliveira, D., and de Aragão Umbuzeiro, G. (2007). Mutagenic and Carcinogenic Potential of a Textile Azo Dye Processing Plant Effluent that Impacts a Drinking Water Source. Mutat. Res. 626 (1-2), 53–60. doi:10.1016/j.mrgentox.2006.08.002
American Public Health Association (APHA) (2005). Standard Methods for the Examination of Water and Wastewater (21st ed.). Washington, DC: American Public Health Association.
Andreozzi, R., Caprio, V., Insola, A., and Marotta, R. (1999). Advanced Oxidation Processes (AOP) for Water Purification and Recovery. Catal. Today 53 (1), 51–59. doi:10.1016/s0920-5861(99)00102-9
Bancroft, J. D., Layton, C., and Suvarna, S. K. (2013). Bancroft’s Theory and Practice of Histological Techniques. 7th Ed. Churchill Livingstone, Elsevier, 151.
Baruah, S., K. Pal, S., and Dutta, J. (2012). Nanostructured Zinc Oxide for Water Treatment. Nanoasia 2 (2), 90–102. doi:10.2174/2210681211202020090
Bernet, D., Schmidt, H., Meier, W., Burkhardt-Holm, P., and Wahli, T. (1999). Histopathology in Fish: Proposal for a Protocol to Assess Aquatic Pollution. J. Fish. Dis. 22 (1), 25–34. doi:10.1046/j.1365-2761.1999.00134.x
Borm, P. J., and Müller-Schulte, D. (2006). Nanoparticles in Drug Delivery and Environmental Exposure: Same Size, Same Risks?
Chronicles, E. (2013). Group of the Nile Basin: Cairo UniversityVs Report on EthiopiaVs Great Renaissance Dam (Retrieved April 2, 2016).
Curri, M., Comparelli, R., Cozzoli, P., Mascolo, G., and Agostiano, A. (2003). Colloidal Oxide Nanoparticles for the Photocatalytic Degradation of Organic Dye. Mater. Sci. Eng. C 23 (1-2), 285–289. doi:10.1016/s0928-4931(02)00250-3
Dahshan, H., Megahed, A. M., Abd-Elall, A. M., Abd-El-Kader, M. A., Nabawy, E., and Elbana, M. H. (2016). Monitoring of Pesticides Water Pollution-The Egyptian River Nile. J. Environ. Health Sci. Eng. 14 (1), 15–19. doi:10.1186/s40201-016-0259-6
Derbalah, A. (2009). Chemical Remediation of Carbofuran Insecticide in Aquatic System by Advanced Oxidation Processes. J. Agric. Res. Kafr Elsheikh Univ. 35 (1), 308–327.
Derbalah, A., Ismail, A., Hamza, A., and Shaheen, S. (2014). Monitoring and Remediation of Organochlorine Residues in Water. Water Environ. Res. 86 (7), 584–593. doi:10.2175/106143014x13975035525221
Derbalah, A., and Ismail, A. (2013). Remediation Technologies of Diazinon and Malathion Residues in Aquatic System. Environ. Prot. Eng. 39 (3). doi:10.37190/epe130310
Derbalah, A., Ismail, A., and Shaheen, S. (2016). The Presence of Organophosphorus Pesticides in Wastewater and its Remediation Technologies. Environ. Eng. Manag. J. (Eemj) 15 (8). doi:10.30638/eemj.2016.190
Derbalah, A., Sunday, M., Chidya, R., Jadoon, W., and Sakugawa, H. (2019). Kinetics of Photocatalytic Removal of Imidacloprid from Water by Advanced Oxidation Processes with Respect to Nanotechnology. J. Water Health 17 (2), 254–265. doi:10.2166/wh.2019.259
Descotes, G., Pinard, D., Gallas, J.-F., Penacchio, E., Blot, C., and Moreau, C. (1996). Extension of the 4-week Safety Study for Detecting Immune System Impairment Appears Not Necessary: Example of Cyclosporin A in Rats. Toxicology 112 (3), 245–256. doi:10.1016/0300-483x(96)03407-5
Dhal, J. P., Mishra, B. G., and Hota, G. (2015). Hydrothermal Synthesis and Enhanced Photocatalytic Activity of Ternary Fe2O3/ZnFe2O4/ZnO Nanocomposite through cascade Electron Transfer. RSC Adv. 5 (71), 58072–58083. doi:10.1039/c5ra05894e
Dutta, H. M., Adhikari, S., Singh, N. K., Roy, P. K., and Munshi, J. S. (1993). Histopathological Changes Induced by Malathion in the Liver of a Freshwater Catfish, Heteropneustes Fossilis (Bloch). Bull. Environ. Contam. Toxicol. 51 (6), 895–900. doi:10.1007/BF00198287
Egyptian Association Of Environmental Affair Law 48 (1982). N.-., Permissible Values for Wastes in River Nile and Law 4. Cairo: Law of the Environmental Protection.
Eissa, F., Al-Sisi, M., and Ghanem, K. (2021). Occurrence, Human Health, and Ecotoxicological Risk Assessment of Pesticides in Surface Waters of the River Nile's Rosetta Branch, Egypt. Environ. Sci. Pollut. Res. 28 (39), 55511–55525. doi:10.1007/s11356-021-14911-5
Eissa, F., Ghanem, K., and Al-Sisi, M. (2020). Occurrence and Human Health Risks of Pesticides and Antibiotics in Nile tilapia along the Rosetta Nile branch, Egypt. Toxicol. Rep. 7, 1640–1646. doi:10.1016/j.toxrep.2020.03.004
El-Demerdash, F., Dewer, Y., ElMazoudy, R. H., and Attia, A. A. (2013). Kidney Antioxidant Status, Biochemical Parameters and Histopathological Changes Induced by Methomyl in CD-1 Mice. Exp. Toxicologic Pathol. 65 (6), 897–901. doi:10.1016/j.etp.2013.01.002
El-Kemary, M., El-Shamy, H., and El-Mehasseb, I. (2010). Photocatalytic Degradation of Ciprofloxacin Drug in Water Using ZnO Nanoparticles. J. Lumin. 130 (12), 2327–2331. doi:10.1016/j.jlumin.2010.07.013
Evgenidou, E., Konstantinou, I., Fytianos, K., and Albanis, T. (2006). Study of the Removal of Dichlorvos and Dimethoate in a Titanium Dioxide Mediated Photocatalytic Process through the Examination of Intermediates and the Reaction Mechanism. J. Hazard. Mater. 137 (2), 1056–1064. doi:10.1016/j.jhazmat.2006.03.042
Evgenidou, E., Konstantinou, I., Fytianos, K., and Poulios, I. (2007). Oxidation of Two Organophosphorous Insecticides by the Photo-Assisted Fenton Reaction. Water Res. 41 (9), 2015–2027. doi:10.1016/j.watres.2007.01.027
Gilmour, C. R. (2012). Water Treatment Using Advanced Oxidation Processes: Application Perspectives.
Gupta, D., Sunita, S. J., and Saharan, J. (2009). Physiochemical Analysis of Ground Water of Selected Area of Kaithal City (Haryana) India. Researcher 1 (2), 1–5.
Habig, W. H. (1974). Glutathione-S-Transferase UV Method. J. Biol. Chem. 249, 7130–7139. doi:10.1016/s0021-9258(19)42083-8
Hansson, H., Kaczala, F., Marques, M., and Hogland, W. (20122012). Photo-Fenton and Fenton Oxidation of Recalcitrant Industrial Wastewater Using Nanoscale Zero-Valent Iron. Int. J. Photoenergy. doi:10.1155/2012/531076
Hoffmann, M. R., Martin, S. T., Choi, W., and Bahnemann, D. W. (1995). Environmental Applications of Semiconductor Photocatalysis. Chem. Rev. 95 (1), 69–96. doi:10.1021/cr00033a004
Hyung, H., and Kim, J.-H. (2009). Dispersion of C60 in Natural Water and Removal by Conventional Drinking Water Treatment Processes. Water Res. 43 (9), 2463–2470. doi:10.1016/j.watres.2009.03.011
Ismail, A., Derbalah, A., and Shaheen, S. (2015). Monitoring and Remediation Technologies of Organochlorine Pesticides in Drainage Water. Polish J. Chem. Technol. 17 (1), 115–122. doi:10.1515/pjct-2015-0017
Juang, R.-S., and Chen, C.-H. (2014). Comparative Study on Photocatalytic Degradation of Methomyl and Parathion over UV-Irradiated TiO2 Particles in Aqueous Solutions. J. Taiwan Inst. Chem. Eng. 45 (3), 989–995. doi:10.1016/j.jtice.2013.09.025
Kaneco, S., Katsumata, H., Suzuki, T., Funasaka, T., and Kiyohisa, K. (2007). Solar Photocatalytic Degradation of Endocrine Disruptor Di-n-butyl Phthalate in Aqueous Solution Using Zinc Oxide. Bull. Catal. Soc. India 6 (3), 22–33.
Konishi, N., and Ward, J. (1989). Increased Levels of DNA Synthesis in Hyperplastic Renal Tubules of Aging Nephropathy in Female F344/NCr Rats. Vet. Pathol. 26 (1), 6–10. doi:10.1177/030098588902600102
Korsrud, G. O., Grice, H. C., and McLaughlan, J. M. (1972). Sensitivity of Several Serum Enzymes in Detecting Carbon Tetrachloride-Induced Liver Damage in Rats. Toxicol. Appl. Pharmacol. 22 (3), 474–483. doi:10.1016/0041-008x(72)90255-4
Kralj, M. B., Franko, M., and Trebše, P. (2007). Photodegradation of Organophosphorus Insecticides–Investigations of Products and Their Toxicity Using Gas Chromatography–Mass Spectrometry and AChE-thermal Lens Spectrometric Bioassay. Chemosphere 67 (1), 99–107. doi:10.1016/j.chemosphere.2006.09.039
Kumari, L., Li, W., Vannoy, C. H., Leblanc, R. M., and Wang, D. (2010). Zinc Oxide Micro-and Nanoparticles: Synthesis, Structure and Optical Properties. Mater. Res. Bull. 45 (2), 190–196. doi:10.1016/j.materresbull.2009.09.021
Kümmerer, K., Al-Ahmad, A., and Mersch-Sundermann, V. (2000). Biodegradability of Some Antibiotics, Elimination of the Genotoxicity and Affection of Wastewater Bacteria in a Simple Test. Chemosphere 40 (7), 701–710. doi:10.1016/s0045-6535(99)00439-7
Laoufi Na, B. F. (2009). “Photocatalytic Oxidation of Methomyl on an Immobilized Particle TiO2 Layer,” in The 11th International Conference on Environmental Science and Technology (China, Crete, Greece, 671–687.
Lasram, M. M., Annabi, A. B., El Elj, N., Selmi, S., Kamoun, A., El-Fazaa, S., et al. (2009). Metabolic Disorders of Acute Exposure to Malathion in Adult Wistar Rats. J. Hazard. Mater. 163 (2-3), 1052–1055. doi:10.1016/j.jhazmat.2008.07.059
Leusch, F., and Chapman, H. (2011). The Role of Toxicity Testing in Identifying Toxic Substances: A Framework for Identification of Suspected Toxic Compounds in Water. Canberra, Australia: Griffith University.
Markova-Deneva, I. (2010). Infrared Spectroscopy Investigation of Metallic Nanoparticles Based on Copper, Cobalt, and Nickel Synthesized through Borohydride Reduction Method. J. Univ. Chem. Tech. Metall. 45 (4), 351–378.
Massoud, A., Derbalah, A., El-Mehasseb, I., Allah, M. S., Ahmed, M. S., Albrakati, A., et al. (2021). Photocatalytic Detoxification of Some Insecticides in Aqueous Media Using TiO2 Nanocatalyst. Int. J. Environ. Res. Public Health 18 (17), 9278. doi:10.3390/ijerph18179278
Massoud, A. S. D. A. H., El-Fakhrany, I. I., and Allah, M. S. S. (2011). Toxicological Effects of Organosphorus Insecticides and Remediation Technologies of its Residues in Aquatic System B. Dimethoate. J. Agric. Res. Kafer El-sheikh Univ. 37, 516–533.
Mebert, A. M., Baglole, C. J., Desimone, M. F., and Maysinger, D. (2017). Nanoengineered Silica: Properties, Applications and Toxicity. Food Chem. Toxicol. 109, 753–770. doi:10.1016/j.fct.2017.05.054
Mills, A., and Le Hunte, S. (1997). An Overview of Semiconductor Photocatalysis. J. Photochem. Photobiol. A: Chem. 108 (1), 1–35. doi:10.1016/s1010-6030(97)00118-4
Monkiedje, A., and Spiteller, M. (2005). Degradation of Metalaxyl and Mefenoxam and Effects on the Microbiological Properties of Tropical and Temperate Soils. Int. J. Environ. Res. Public Health 2 (2), 272–285. doi:10.3390/ijerph2005020011
Parthasarathi, V., and Thilagavathi, G. (2011). Synthesis and Characterization of Zinc Oxide Nanopartilce and its Application on Fabrics for Microbe Resistant Defence Clothing. Int. J. Pharm. Pharm. Sci. 3 (4), 392–398.
Pasieczna-Patkowska, S., Czech, B., Ryczkowski, J., and Patkowski, J. (2010). Removal of Recalcitrant Pollutants from Wastewater. Appl. Surf. Sci. 256 (17), 5434–5438. doi:10.1016/j.apsusc.2009.12.132
Peter, C., Burk, J., and Vanzwieten, M. (1986). Spontaneous Nephropatheis in Rats. Toxicol. Pathol. 14, 91–100. doi:10.1177/019262338601400111
Pung, S.-Y., Lee, W.-P., and Aziz, A. (2012). Kinetic Study of Organic Dye Degradation Using ZnO Particles with Different Morphologies as a Photocatalyst. Int. J. Inorg. Chem. 2012, 1–9. doi:10.1155/2012/608183
Rahm, M., Atty, Y. A., Rahman, M. A., and Sabry, M. (2017). Structural Changes Induced by Gibberellic Acid in the Renal Cortex of Adult Male Albino Rats. MOJ Anat. Physiol. 3, 00080. doi:10.15406/mojap.2017.03.00080
Rahmayeni, D. A., Stiadi, Y., Jamarun, N., and Emriadi, A. S. (2015). Preparation, Characterization of ZnO/CoFe 2 O 4 Magnetic Nanocomposites and Activity Evaluation under Solar Light Irradiation. J. Chem. Pharm. Res. 7 (9S), 139–146.
Reitman, S. F. (1957). Glutamic – Pyruvate Transaminase Assay by Colorimetric Method. Am. J. Clin. Path 28, 56. doi:10.1093/ajcp/28.1.56
Samuel, M. S. B. L. a. K. C. G. (2009). Optical Properties of ZnO Nanoparticles. Acad. Rev. XVI (No. 1 & 2 SB), 57–65pp.
Schirmeister, J. (1964). Determination of Creatinine Level. Deutsche Medizinische Wochenschrift 89, 1940–1947. doi:10.1055/s-0028-1111251
Sharma, Y., Bashir, S., Irshad, M., Nag, T., and Dogra, T. (2005). Dimethoate-induced Effects on Antioxidant Status of Liver and Brain of Rats Following Subchronic Exposure. Toxicology 215 (3), 173–181. doi:10.1016/j.tox.2005.06.029
Simonian, A., Efremenko, E., and Wild, J. (2001). Discriminative Detection of Neurotoxins in Multi-Component Samples. Analytica Chim. Acta 444 (2), 179–186. doi:10.1016/s0003-2670(01)01099-6
Strathmann, T. J., and Stone, A. T. (2002). Reduction of the Pesticides Oxamyl and Methomyl by FeII: Effect of pH and Inorganic Ligands. Environ. Sci. Technol. 36 (4), 653–661. doi:10.1021/es011029l
Tamimi, M., Qourzal, S., Barka, N., Assabbane, A., and Ait-Ichou, Y. (2008). Methomyl Degradation in Aqueous Solutions by Fenton's Reagent and the Photo-Fenton System. Sep. Purif. Tech. 61 (1), 103–108. doi:10.1016/j.seppur.2007.09.017
Tochitani, T., Mori, M., Matsuda, K., Kouchi, M., Fujii, Y., and Matsumoto, I. (2016). Histopathological Characteristics of Renal Changes in Humanrenin-Angiotensinogen Double Transgenic Rats. J. toxicologic Pathol. 29 (2), 125–129. doi:10.1293/tox.2015-0055
Tomašević, A., Bošković, G., Mijin, D., and Kiss, E. E. (2007). Decomposition of Methomyl over Supported Iron Catalysts. React. Kinetics Catal. Lett. 91 (1), 53–59. doi:10.1007/s11144-007-5094-4
van der Werf, H. M. (1996). Assessing the Impact of Pesticides on the Environment. Agric. Ecosyst. Environ. 60 (2-3), 81–96. doi:10.1016/s0167-8809(96)01096-1
Van Vooren, L., Van De Steene, M., Ottoy, J.-P., and Vanrolleghem, P. (2001). Automatic Buffer Capacity Model Building for the Purpose of Water Quality Monitoring. Water Sci. Technol. 43 (7), 105–113. doi:10.2166/wst.2001.0400
Waber, H., and Dtsch, M. (1966). Cholinestrase Kinetic Colorimetric Method. Dtsch. Med. Wschr 91, 1927.
Wafa, T., Amel, N., Issam, C., Imed, C., Abdelhedi, M., and Mohamed, H. (2011). Subacute Effects of 2, 4-dichlorophenoxyacetic Herbicide on Antioxidant Defense System and Lipid Peroxidation in Rat Erythrocytes. Pestic. Biochem. Physiol. 99 (3), 256–264. doi:10.1016/j.pestbp.2011.01.004
Yu, H., Yu, J., Cheng, B., and Zhou, M. (2006). Effects of Hydrothermal post-treatment on Microstructures and Morphology of Titanate Nanoribbons. J. Solid State. Chem. 179 (2), 349–354. doi:10.1016/j.jssc.2005.10.024
Keywords: degradation, insecticides, toxicity, light, nanocatalyst, water
Citation: Massoud A, El-Mehasseb I, Saad Allah M, Elmahallawy EK, Alsharif KF, S. Ahmed M and Derbalah AS (2022) Advanced Oxidation Processes Using Zinc Oxide Nanocatalyst for Detoxification of Some Highly Toxic Insecticides in an Aquatic System Combined With Improving Water Quality Parameters. Front. Environ. Sci. 10:807290. doi: 10.3389/fenvs.2022.807290
Received: 02 November 2021; Accepted: 22 February 2022;
Published: 23 March 2022.
Edited by:
Mazhar Iqbal Zafar, Quaid-i-Azam University, PakistanReviewed by:
Mohamed Hassaan, National Institute of Oceanography and Fisheries (NIOF), EgyptMuhammad Bilal Khan Niazi, National University of Sciences and Technology (NUST), Pakistan
Copyright © 2022 Massoud, El-Mehasseb, Saad Allah, Elmahallawy, Alsharif, S. Ahmed and Derbalah. This is an open-access article distributed under the terms of the Creative Commons Attribution License (CC BY). The use, distribution or reproduction in other forums is permitted, provided the original author(s) and the copyright owner(s) are credited and that the original publication in this journal is cited, in accordance with accepted academic practice. No use, distribution or reproduction is permitted which does not comply with these terms.
*Correspondence: Ehab Kotb Elmahallawy, eehaa@unileon.es