- 1Finnish Environment Institute, Helsinki, Finland
- 2Department of Civil, Environmental and Natural Resources Engineering, Luleå University of Technology, Luleå, Sweden
- 3Department of Water, Energy and Environmental Engineering, University of Oulu, Oulu, Finland
Current practices in wastewater management lead to inefficient recovery and reuse of nutrients and can result in environmental problems. Source separation systems have been shown to be an efficient way of recovering nutrients and energy from wastewaters, both in rural and urban context. Studies on nutrient recovery potential and life cycle impacts of source separation systems are mainly limited to small systems (for example a few households) while the impacts of upscaling source separation to a regional level have hardly been studied, especially in sparsely populated areas where the cost of the connection to a main treatment plant is higher. This study examines the regional nutrient balance of two source separation scenarios—black water separation and urine diversion—and compares them to the existing conventional wastewater system. The analysis comprises three sparsely populated regions of northern Finland and Sweden, including rural, peri-urban and urban areas. In addition, climate impacts are assessed based on existing life cycle assessment (LCA) studies. According to the results, by source separation it is possible to achieve a significant increase in the recovery rate of phosphorus (41–81%) and nitrogen (689–864%) compared to the conventional system. Depending on the region up to 65% of the mineral phosphorus and 60% of mineral nitrogen fertilisers could be theoretically replaced. Furthermore, the climate and eutrophication impacts would decrease with the implementation of such systems, but an increase in acidification may occur. However, even if the benefits of source separation systems are undisputed in terms of nutrient recovery, the implementation of such systems would to a large extent require an entire system change of the wastewater treatment sector and a wide paradigm change towards a circular economy.
1 Introduction
Nutrient recovery is one of the key drivers promoting sustainability in wastewater management and the circular economy (Hoffmann et al., 2020; Larsen et al., 2021a). Wastewater contains, among other substances, phosphorus and nitrogen, which are vital nutrients for food production. Current practices in wastewater management lead to inefficient recovery and reuse of wastewater-based nutrients and can result in environmental problems such as eutrophication, contribute to climate change, and undermine global food security (Kjerstadius et al., 2017; Skambraks et al., 2017; Wielemaker et al., 2018; Hoffmann et al., 2020; Öberg et al., 2020). Instead of circulating nutrients in wastewater back to food production, the need for nutrients is met by introducing more mineral fertilizers to the system. The extensive mining of phosphate rocks has led to the significant depletion of known stocks, resulting in the inclusion of phosphate rock on the EU list of critical raw materials (European Commission, 2017). Moreover, the production of nitrogen fertilizers is responsible for about 0.8% of global greenhouse gas emissions due to its high energy consumption (Brentrup, 2009).
In Finland and Sweden, approximately 9,800 tonnes of phosphorus (P) and 78,000 tonnes of nitrogen (N) reach wastewater treatment plants annually (SYKE, 2019; SEI, 2020). After treatment, around 4 and 5% of phosphorus and 34 and 40% of nitrogen is left in the effluent and thus released to water bodies, in Finland and Sweden respectively (Naturvårdsverket, 2018; Dagerskog and Olsson, 2020; Lehtoranta et al., 2021a). Most of the phosphorus and nitrogen removed from the wastewater in physical, chemical and biological purification processes accumulate in the sludge. However, only a small fraction of the nutrients originally contained in the wastewater can be found in plant-available form in the produced sludge (Warman and Termeer, 2005). Currently, sludge-based products are mainly used in landscaping, in relatively small land areas but in large amounts, and only a small portion of the nutrients end up being utilized by plants, resulting in “hot spots” of nutrient and pollutant loads to waters (Valtanen et al., 2015).
There are also safety concerns regarding the direct land spread of sludge-based products, which decrease the willingness to recycle wastewater nutrients and organic matter back to agriculture (SEI, 2020). For example, there are uncertain risks of soil, crop and water course contamination by pathogens, heavy metals and micro-organic pollutants (e.g., pharmaceuticals and personal care products, etc.) (Seleiman et al., 2020). The current wastewater treatment methods are inadequate to remove them, and part of the harmful substances end up in discharge water and some are retained in the sludge (Magnusson and Norén, 2014; Vieno, 2014; Talvitie et al., 2017; Vieno et al., 2018; Ylivainio et al., 2020; Lehtoranta et al., 2021a).
However, the Finnish government aims at recycling wastewater sludge-based nutrients mainly as fertilizers by 2030 (Ministry of Environment, 2019). In Sweden, the quality assurance certification system REVAQ sets limits on key pollutants and encourages wastewater treatment plants to improve their sludge management approach in order to meet certain quality standards (Dagerskog and Olsson, 2020). About 45% of the sludge produced in Sweden is REVAQ-certified and both the Swedish Farmers Association and the Swedish Water and Wastewater Association recommend its application in farmland (Dagerskog and Olsson, 2020). Concerning nutrient recovery targets, no specific legislation has yet been implemented in Sweden, despite the existence of general goals and regulatory instruments regarding resource conservation, reuse and recycling (Swedish government, 1998). In general, to meet the targets, wastewater treatment systems need to be developed to achieve the safe recovery of nutrients and organic matter. The implementation of new technologies, such as RAVITA and NPHarvest (Rossi et al., 2018; Kaljunen et al., 2021), in centralized wastewater treatment plants (WWTPs), could result in even 1.3 times greater phosphorus recovery and three times greater nitrogen recovery when compared with current technologies, as well as decrease the accumulation of hazardous substances (Lehtoranta et al., 2021a).
Apart from end-of-pipe solutions, source separation has emerged as an efficient way of recovering wastewater-based nutrients in both rural (Lennartsson et al., 2009; Malila et al., 2019) and urban contexts (Nagy and Zseni, 2017; Turlan, 2019; Lehtoranta et al., 2022). Municipal wastewater contains a mixture of black water (containing faeces, urine and toilet paper) and grey water (from kitchen appliances, showers, etc.). If the nutrient-rich black water is not mixed with grey water and other nutrient-poor waters (e.g., industrial wastewater, stormwater or infiltration and inflow), the nutrients can be recovered more easily from highly concentrated flows without being mixed with harmful substances, originating from other sources than toilet water (Jönsson et al., 2005; Tidåker et al., 2006b; Saliu and Oladoja, 2021). For example, grey water contains a large portion of the heavy metals found in wastewater (Simha, 2021); and by separating the black water at source the metal fraction input from the grey water is excluded. Moreover, the concentrations of most metals in source-separated black water are generally much lower than in sewage sludge, and hence black water utilization is more effective in reducing metals in agriculture (Tervahauta et al., 2014). However, the black water fraction contains the majority of pathogens in wastewater and the risk of pathogenic presence in the end products will depend on the method used to treat the separated black water. Significant pathogen inactivation can be achieved, for example, with urea treatment (Nordin et al., 2009; Fidjeland et al., 2015) which has been proven as a robust option for safe recycling of plant nutrients, as well as thermophilic anaerobic digestion applied to blackwater (Moerland et al., 2020) but also sewage sludge (Zhao and Liu, 2019). In addition, urine contains the majority of pharmaceuticals and hormones found in wastewater (Udert et al., 2006), but for example, Viskari and others (2018) found that apart from progesterone the concentrations of all extractable pharmaceuticals and hormones in the soil fertilized with source-separated urine remained below the detection limit (Viskari et al., 2018). Nevertheless, the risk of harmful substances needs to be further investigated and country specific conditions considered.
Within conventional wastewater treatment, most of the nitrogen is removed in energy-intensive processes and part of the nitrogen is released into the atmosphere as nitrous oxide (a very potent greenhouse gas). In addition, the removal of phosphorus is based on chemical precipitation, and consequently the phosphorus is in a poorly soluble form and thus not easily available for plants (Tidåker et al., 2006b; Ylivainio et al., 2020). Black water comprises only 15% of the whole domestic wastewater volume (Motiva, 2020), but contains about 90% of the nitrogen and 80% of the phosphorus (Viskari et al., 2017; Saliu and Oladoja, 2021). Urine is even more concentrated than black water, as it comprises less than 2% of domestic wastewater volume and includes about 80% of the total nitrogen and about half of the total phosphorus and potassium (Jönsson et al., 2005). Thus, the separation of black water enables highest nutrient recovery, but urine diversion maximizes the amount of nutrients in the separated fractions and minimizes the additional burden from faecal pathogens at the same time (Viskari et al., 2021). Both black water and urine separation systems therefore have great potential to increase nutrient recovery and utilization as the nutrients are available in a more soluble and contaminant-free form; however, the unwanted occurrence of hormones and pharmaceuticals still needs to be considered.
Finland and Sweden are both sparsely populated countries, and their population density continues to decline further north. Due to long distances, expanding the municipal sewage networks is often not a technically and economically viable option. Currently, about 15 and 12% of Finnish and Swedish populations (respectively) are not connected to the sewage network (Statistics Sweden, 2021a; Lapinlampi, 2021). Furthermore, a significant part of the existing on-site wastewater treatment systems is made up of septic tanks only, thus failing to meet the legislated treatment requirements in terms of BOD and nutrient removal (Swedish EPA, 2018; Kallio, 2020). According to conservative estimates, some 114,000 properties in Finland (Kallio and Suikkanen, 2019) and 175,000 properties in Sweden (Olshammar et al., 2015) (figures include holiday homes) require immediate upgrades to their on-site systems due to insufficient treatment. In general, wastewater treated and discharged in non-sewered areas cause higher load to water bodies than those treated in centralized systems, thus they are a significant source of diffuse pollution (Vienonen, 2007). In fact, wastewater from sparsely populated areas and secondary residences is currently in Finland the second most significant source of phosphorus load in water systems after agriculture (Tattari et al., 2015). Despite the efforts to decrease diffuse load, Finland did not achieve its nutrient reduction targets set for 2021 (HELCOM, 2013; Räike et al., 2020). The introduction of source separation systems in rural areas could help reduce the load of organic matter, nutrients and pathogens on receiving waters, as only grey waters would be treated on-site. Another aspect is that small WWTPs report difficulties in treating sludge from septic tanks under their environmental permits due to the increased load on their systems, leading to sludge being transported longer distances to larger units (Tarkka and Leppänen 2019). Moreover, considerable debts are reported in both Finland (ROTI, 2021) and Sweden (Malm et al., 2013; Hedström et al., 2016) related to the renovation of sewage networks and WWTPs. Therefore, careful consideration is needed to evaluate which solutions are worth implementing in the future, i.e., expanding the network or implementing different technologies for on-site sanitation.
In sum, the replacement of conventional and ineffective treatment systems with source separation options in sparsely populated areas offers a great opportunity to promote nutrient recycling and thus sustainable sanitation. The aim of this work was to examine the advantages and drawbacks of implementing source-separating sanitation in northern Finland and Sweden. This study estimates the potential of recovering nitrogen and phosphorus from wastewater in rural and peri-urban areas. The evaluation was based on defined scenarios for the implementation of source separation systems where the characteristics of the settlements in the region were considered. In addition, the environmental impacts of source separation in the whole region were assessed based on data reported in previous life cycle assessment (LCA) studies. The impacts of black water and urine diversion systems were compared to those of conventional wastewater systems used in the regions studied. The findings of this work are expected to improve the general understanding regarding the potential and feasibility of source separation systems in cold and sparsely populated areas of Nordic countries.
2 Materials and Methods
2.1 Study Area
The study area includes three sparsely populated regions: Lapland and North Ostrobothnia in northern Finland as well as Norrbotten county in northern Sweden, which have a population density of 1.9, 11.2 and 2.6 persons/km2, respectively. By comparison, the population density in whole Finland is 18, in Sweden 25 and, e.g., in France 123 persons/km2 (World Bank 2020). The division of the population between urban and rural areas is quite similar (Table 1). Currently, the share of the population not connected to the sewage collection network is 22% in North Ostrobothnia, 24% in Lapland and 13% in Norrbotten. Different types of on-site wastewater treatment systems are used. Infiltration systems are generally used in permanent dwellings, including infiltration beds (drain fields) and sand filters. However, septic tanks without secondary treatment still constitute the most common treatment method in these areas. Urine or black water separation systems or dry toilets are rare and mainly used in holiday homes (McConville et al., 2017; Viskari et al., 2017; Kallio, 2020).
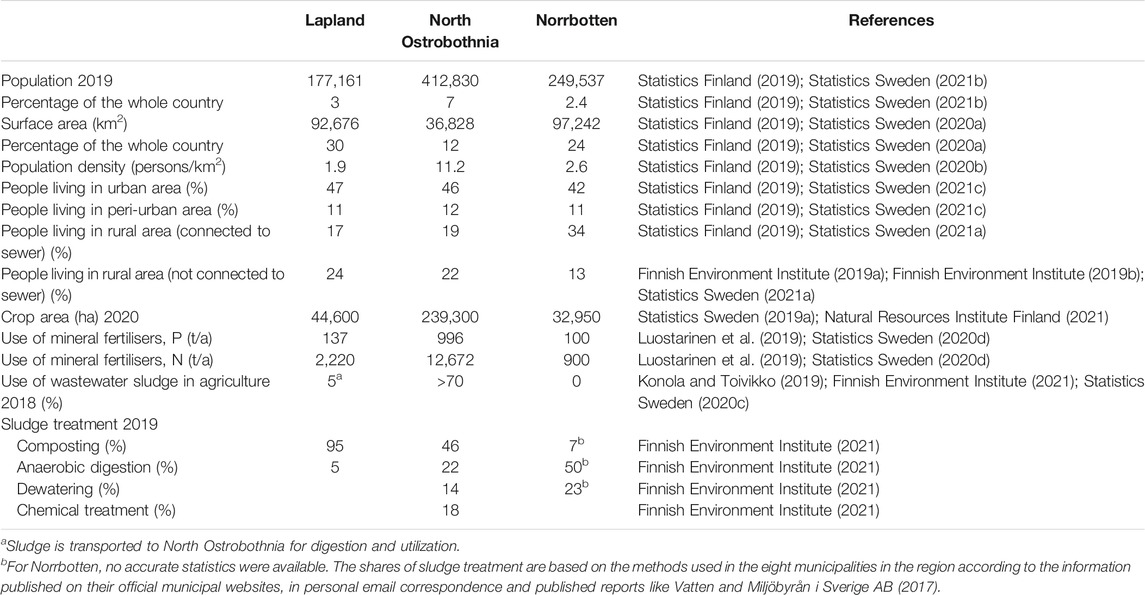
TABLE 1. Statistics for the study area including Lapland and North Ostrobothnia in Finland and Norrbotten in Sweden.
In Finland, about half of the sludge produced in centralized wastewater treatment plants is used in landscaping and about 40% in agriculture (Konola and Toivikko, 2019), while in Sweden, about 39% of the sludge produced is used in agriculture, and 42% is used for landscaping and covering mine tailings (Statistics Sweden, 2020c). However, sludge utilization rates vary significantly among the regions under investigation. In North Ostrobothnia more than 70% of the sludge produced is used in agriculture, whereas in Lapland and Norrbotten, agricultural use is non-existent (Konola and Toivikko, 2019; Statistics Sweden, 2020c). However, part of the sludge produced in Lapland is transported to North Ostrobothnia for further treatment and utilization.
In general, sludge produced in on-site treatment systems implemented in households outside the sewage network is treated in central wastewater treatment plants. Long transportation distances of relatively small amounts of sludge pose challenges for logistics and sludge treatment. Cold climate causes the sludge to freeze during transportation, leading to additional expense in terms of heating energy to allow treatment (Lauronen, 2017). Harsh winter conditions and low precipitation also limit agriculture in the study area, with activities mainly focusing on livestock farming. The thermal growing season and long-term average precipitation are slightly lower in Lapland and Norrbotten than in North Ostrobothnia (Swedish Meteorological and Hydrological Institute, 2017; Finnish Meteorological Institute, 2021; Swedish Meteorological and Hydrological Institute, 2021) which results in prominent differences in available crop areas (Table 1).
The study area was divided into urban, peri-urban and rural areas based on current population estimates (Table 1). The urban areas (42–47% of the population in the study area) are densely populated areas with at least 15,000 inhabitants and high building density, whereas peri-urban areas (11–12% of the population) have lower population density and mainly consist of detached or terraced houses. In urban and peri-urban areas, households are 100% connected to the sewage network. The rural areas consist of areas where houses are connected (17–34%) or not connected (13–24%) to sewage networks. Summer houses were not included in the study due to the lack of available data. Moreover, the implementation of source separation systems in urban areas was not assessed in this study because retrofitting of such systems in built-up areas is more difficult due to lack of space and costs, for example (McConville et al., 2017; Hoffmann et al., 2020). Retrofitting in semi-urban and rural areas is assumed to be a more feasible alternative.
2.2 Scenario Definitions and Assumptions
Two scenarios were defined and compared to a Reference System, which reflects the current wastewater management practices in northern Finland and Sweden (Figure 1). Due to the large size of the studied regions and the diversity of operating WWTPs, the defined Reference System and Scenarios have been streamlined.
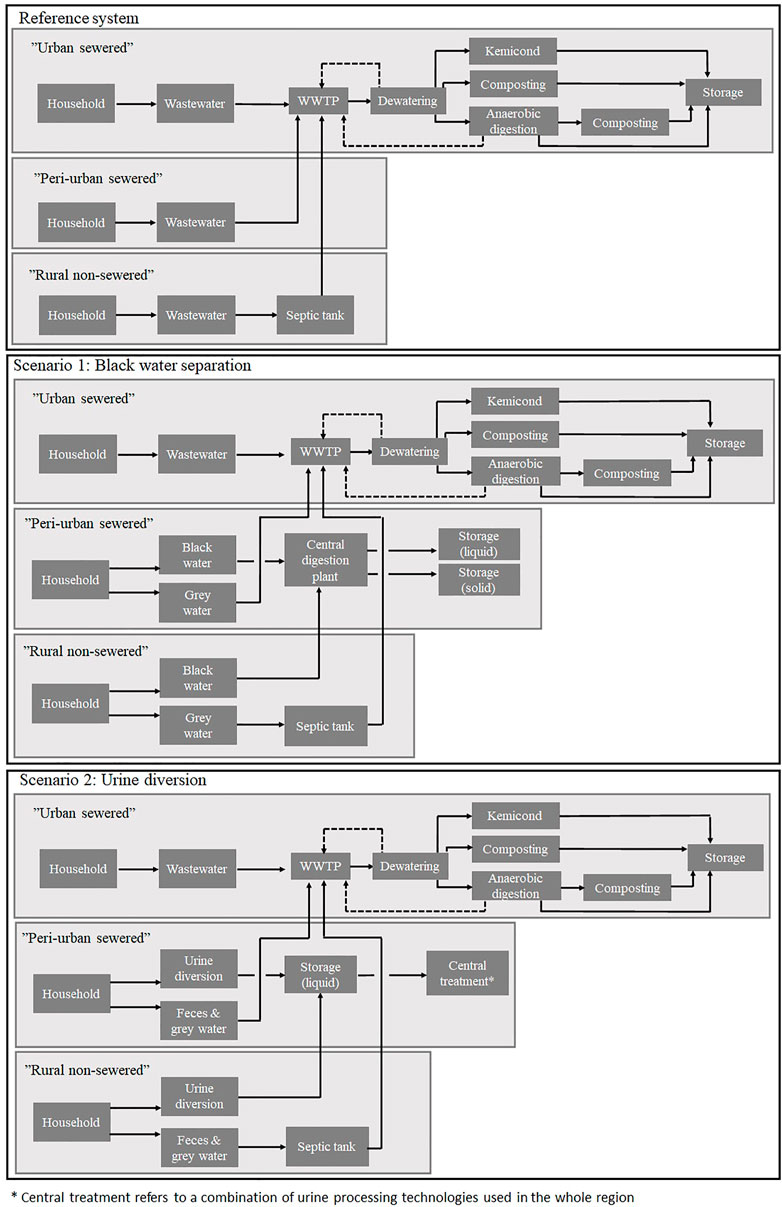
FIGURE 1. Flowcharts of reference system and the defined Scenarios 1 and 2. Solid lines represent the wastewater and wastewater-based nutrient flows in the system. Dashed lines represent reject waters. The distribution of WWTPs sludge treatment in the three regions studied is presented in Table 1.
Households were allocated into three groups based on population density and sewage collection coverage. Urban areas connected to the sewage network (“urban sewered”); rural and peri-urban areas connected to sewage network (“peri-urban sewered”); and rural areas not connected to the sewage network (“rural non-sewered”). The Reference System aims to describe the current wastewater management practices in the studied regions: the totality of wastewater produced in “urban sewered” and “peri-urban sewered” areas are treated in municipal WWTPs. In the “rural non-sewered” areas, wastewater is treated in on-site treatment systems (e.g., a sand filter) and the sludge from the septic tank is transported to WWTPs for treatment. The WWTPs were assumed to consist of the following treatment stages commonly used in Finland and Sweden: pre-treatment, primary sedimentation, activated sludge process (with simultaneous precipitation) and sludge dewatering. Regarding the sludge management practices, it was assumed that all the sludge formed in WWTPs is treated at anaerobic digestion plants and the solid fraction of the digestate is composted and utilised, while the liquid fraction is circulated back to a WWTP for treatment (a common procedure in Finland and Sweden). Details regarding sludge management activities in the study area are provided in Table 1.
Scenarios 1 and 2 (Figure 1) were formulated to describe the possible implementation of source-separating sanitation options in peri-urban and rural areas. In urban areas, the conventional system was assumed to remain (as a Reference System) unchanged.
- Scenario 1 (black water separation), black water from “peri-urban sewered” and “rural non-sewered” is source-separated and treated at centralized anaerobic digestion plants. It was assumed that both solid and liquid fractions resulting from solid-liquid separation of digestate are utilized. Grey water from “peri-urban sewered” households as well as sludge produced in grey water treatment systems in “rural non-sewered” households are treated at WWTPs instead of the centralized anaerobic digestion plant, aiming to reduce the amount of harmful substances in the nutrient-rich end products. Grey water from “rural non-sewered” is treated on-site with a septic tank followed by a sand filter.
- Scenario 2 (urine diversion), urine from “peri-urban sewered” and “rural non-sewered” areas is collected by using urine diverting toilets and treated centrally in local facilities. Brown water (faeces, toilet paper and flushing water) and grey water from “peri-urban sewered” communities are treated at WWTPs as well as the sludge produced in on-site systems used in “rural non-sewered” households.
In both defined scenarios, untreated black water and sludge produced in on-site treatment systems in “rural non-sewered” are transported to WWTPs or centralized anaerobic digestion plants.
2.3 Data Collection and Analysis Methodology
2.3.1 Nutrient Recovery Potential Calculations
The nutrient recovery potential of the Reference System and defined Scenarios 1 and 2 were evaluated based on substance flow analysis (SFA), which is a generic method to quantify flows and stocks of substances (Van der Voet, 2002). The method has been applied in several wastewater and nutrient recovery studies (Breen, 1990; Fan et al., 1996; Puig et al., 2008; Tervahauta et al., 2013; Khiewwijit et al., 2015; Venkatesean et al., 2016; Hong et al., 2019; Cai et al., 2020). In this study, the nutrient recovery potentials were calculated to describe the portion of all nutrients discharged with the wastewater from the households in the study area that have been recovered (Reference System) or could be recovered (Scenarios 1 and 2). The main principle used in the calculation is the mass balance, i.e., the inflow into the system is equal to the outflows. The recovery potential of nutrients (phosphorus and nitrogen), was calculated utilizing a bottom-up approach, starting with nutrient excretion, wastewater treatment and management, and finally storage of fractions. The steps included in each scenario are presented in Figure 1 and the factors used for nutrient recovery estimate in Table 3.
Calculations were made separately for the three groups: “urban sewered”, “peri-urban-sewered” and “rural non-sewered”. Furthermore, calculations regarding the three study areas were also performed separately due to differences in population densities and current sludge treatment and utilization practices (described in Table 1). Specifically, the fractions of volatile compounds and nutrients discharged from treatment, processing and storage were determined by using literature data on the nutrient recovery capacity of each function (Table 3). The estimated nutrient recovery capacity describes the amount of nutrients in the end products which could be utilized in agriculture.
The amount of nutrients produced per person in Finland and Sweden used in this study are presented in Table 2. Data regarding the composition of urine and faeces was taken from Jönsson et al. (2005), whose values are smaller than those in the Government Decree on Treating Domestic, 2011, to avoid the overestimation of available nutrients. However, data from the Government Decree (209/2011) was used for grey water, and therefore the reported values are more conservative than those reported by Jönsson et al. (2005). Nitrogen fractions contained in urine were assumed to be soluble, i.e., ammonium nitrogen. In order to simplify the calculations, it was assumed that all excreted nutrients end up in wastewater management systems, and no nutrient losses were assumed to occur. However, to reduce the risk of overestimating the nutrients produced, it was assumed that children and teenagers excrete 50% less urine and 30% less faeces than adults (Laak, 1974; Almeida et al., 1999; Karak and Bhattacharyya, 2011). The share of children and teenagers amongst the total population was defined as 15.8% in Finland (Statistics Finland, 2019) and 23.3% in Sweden (Statistics Sweden, 2021d).
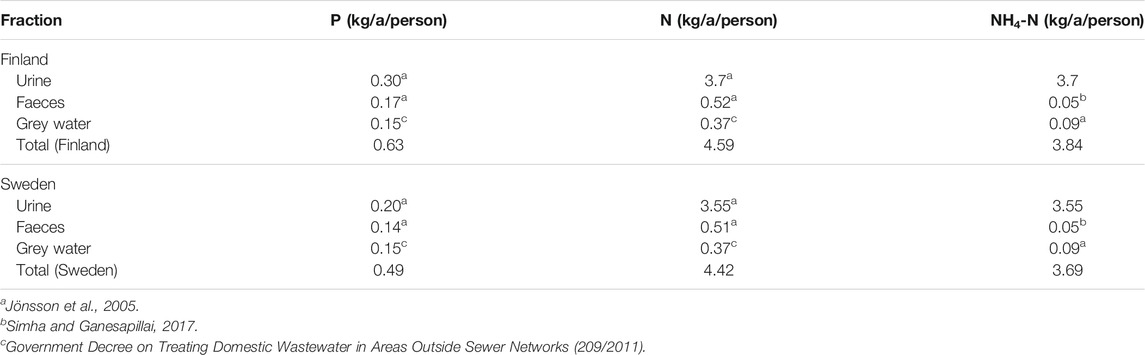
TABLE 2. Amount of nutrients produced annually by a person in Finland and Sweden, taking into consideration the contribution of children and teenagers in the general population.
Regarding management and processing practices of wastewater-based fractions, a literature review and findings from previous studies (e.g., Malila et al., 2019; Lehtoranta et al., 2021a; Lehtoranta et al., 2021b; Lehtoranta et al., 2022) were utilized to define the baseline of nutrient recovery (in percentages) of different treatment methods and technologies (Table 3) for the whole study region which comprises several facilities of different sizes. Therefore, the same nutrient recovery values were applied for Finnish and Swedish regions, as the wastewater characteristics and sludge treatment processes are similar in both countries. The baseline of nutrient recovery describes the typical values in northern Finland and Sweden. However, some treatment methods are not currently in use and the variation within the processes (such as solid-liquid separation) is high. This caused difficulties in defining the baseline. Therefore, variations in nutrient recovery efficiency were used in these processing practices to account for uncertainties in baseline values (shown in parentheses in Table 3). For example, several techniques are available for urine treatment, such as the Vuna process, NPHarvest, membrane technologies, Sanitation 360 and ammonia stripping (e.g., Etter et al., 2015; Mönkäre et al., 2016; Marttinen et al., 2017; Kaljunen et al., 2021; Simha et al., 2021). None of these technologies are currently implemented on a large scale in the regions studied, but if urine diversion were more common, it would be more likely that several parallel technologies would be applied. Thus, an average value was defined for the baseline, and the variation in recovery rates linked to these technologies were considered in the uncertainty analysis. In practice, the calculations were performed separately, first using the baseline values and then performing uncertainty analysis using the minimum and maximum values presented in Table 3 (recovery variations for different technologies).
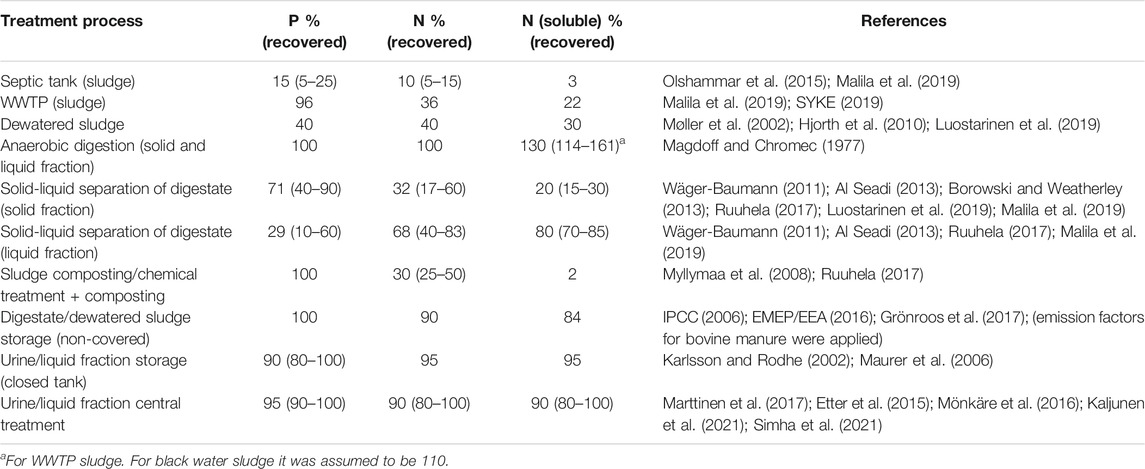
TABLE 3. Phosphorus, nitrogen and ammonium nitrogen (in percentages) recovered from the input material of different treatment processes. Variation range is shown in parentheses.
For black water, the nutrients in both the liquid and solid fractions of the digestate were taken into account in the calculations, but no further processing was considered. During anaerobic digestion, part of the organic nitrogen is mineralised to the soluble form (NH4-N) (Table 3) (Magdoff and Chromec, 1977). For sewage sludge digestion, a conversion value from the literature was used, but if the same value was applied for black water separation, the NH4-N would exceed the amount of total nitrogen (total-N) contained in the fraction because the ratio of NH4-N and total N is already high. Since no appropriate literature data was found, it was assumed that mineralization rises the NH4-N recovery potential to 110% (compared to 130% for digestion of sewage sludge). Therefore, 98% of the total-N in black water separation was assumed to be in soluble form after anaerobic digestion.
2.3.2 Other Environmental Indicator Calculations
Climate change, eutrophication and acidification impacts were estimated for the Reference System and scenarios based on emission factors determined and reported in previous life cycle assessment (LCA) studies of Malila et al. (2019) and Lehtoranta et al. (2022). These emission factors were used for urban, peri-urban and rural areas separately (Table 4). The reported values were used due to their relevance in terms of study design and regional assumptions. However, there are some differences within the system boundaries and selected technologies to the system boundaries defined in this study that need to be considered.
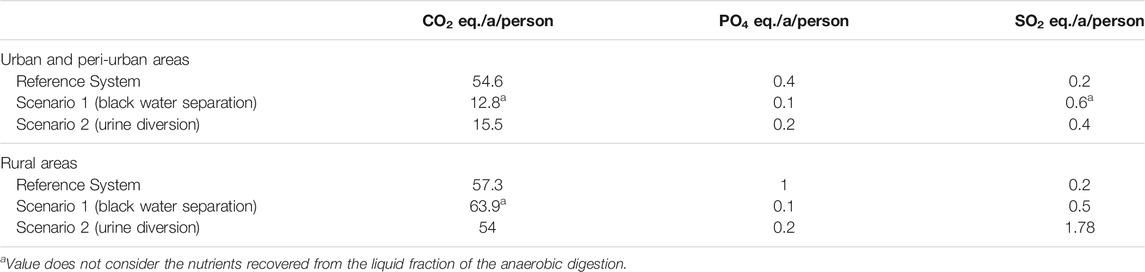
TABLE 4. Emission factors for climate change (CO2 eq.), eutrophication (PO4 eq.) and acidification (SO2 eq.) based on Lehtoranta et al. (2022) for peri-urban areas and Malila et al. (2019) for rural areas.
Lehtoranta et al. (2022) studied the environmental impacts of source separation in the new sustainable city district of Hiedanranta, Finland. The analysis covered the whole Hiedanranta city district (26,000 inhabitants and 6,510 jobs) and its wastewater management. In the conventional system (Reference System), the wastewater is treated in Sulkavuori WWTP, which applies the latest WWTP technologies without additional efforts to improved nutrient recycling. The sludge from the WWTP is digested, composted and utilized in agriculture replacing the use of mineral fertilizers. For source separating systems, grey water in the first scenario and grey water and faeces in the second scenario were treated at the Sulkavuori WWTP. Black water treatment was assumed to be carried out at a local AD plant at Hiedanranta and the reject water was assumed to circulated back to the AD unit in accordance with current practices in Finland. The surplus biogas produced in the black water scenario was assumed to be upgraded for transport fuel replacing the use of fossil fuels. The digestate from local AD plant and WWTP sludge treatment were assumed to be used as fertilizers, replacing mineral fertilizers. In urine diversion systems, urine was assumed to be hygienized, collected and transported for field application, where its use replaced the use mineral fertilizers. No sophisticated treatment technologies for urine or sludge processing were assumed.
Malila et al. (2019) investigated the environmental impacts of source separation in Finnish rural areas. In the study, the conventional system was defined as a system in which wastewater from all households was treated together in one on-site three-chamber septic tank followed by a sand filter. Sludge removed from the septic tank was transported to the WWTP for further treatment followed by digestion and composting. The compost was assumed to be utilized in landscaping and no avoided impacts were assumed. In the blackwater separation system (Scenario 1), urine and faeces were collected together with a vacuum toilet system to minimize the water consumption and the need for transportation. The blackwater was transported to a local anaerobic digestion plant from which the dry fraction of the digestate was used as fertilizer, replacing the use of mineral fertilizers. The liquid fraction was assumed to be circulated back to the process. Greywater was treated similarly as wastewater in the Reference System. In the urine separation system, urine and faeces were collected separately with a separating dry toilet. The collected urine was stored in a closed tank on the property and transported to the field once a year to be used as fertilizer. Faeces were composted in a composter and the compost used at the property. Nutrients from urine and faeces replaced the use of mineral fertilizers. Greywater was treated similarly as in the black water system.
The differences in system boundaries compared to the nutrient recovery potential system boundaries used in this study are as follows; Lehtoranta et al. (2022) did not take the nutrient recovery potential of reject water flows from blackwater treatment processes into account as it was assumed that the reject water was circulated back to the process. In the nutrient recovery potential calculations of this study, the reject water from blackwater digestion was assumed to be utilized as such. Therefore, the nutrient recovery obtained in this study is higher than in the case where nutrients of the reject flows are not recovered. This results in underestimation of avoided impacts from fertilizer use compared to system boundaries in this study. On the other hand, if the nutrients of the rejects are utilized, it might in practice require the introduction of a new type of technology (not considered in the LCA studies), which in turn often consumes more energy reducing climate benefits. Furthermore, Malila et al. (2019) considered dry toilets instead of separating water toilets in urine diversion system. Also, the study assumes that the separated urine is stored and used in agriculture, and no sophisticated nutrient recovery technologies (such as presented in this study, Table 3) are applied. Moreover, in blackwater separation, the treatment and nutrient potential of reject water in AD plants were excluded from the study. In both studies, the whole life cycle including built infrastructure (such as pipes, toilets, treatment plants), the use of the system (energy consumption, direct emissions), transportation of fractions, treatment and nutrient recovery, storage of fractions, field application of fractions and substitutions of energy and mineral fertilisers (total soluble nitrogen and 60% of total phosphorus according to Finnish environmental compensation system) was considered, but the end-of-life was excluded. The emission factors for climate change (CO2 eq.), eutrophication (PO4 eq.) and acidification (SO2 eq.) were presented in a per person per year unit. The LCA data used are presented in more detail in the respective papers (Malila et al., 2019; Lehtoranta et al., 2022).
3 Results and Discussion
3.1 Nutrient Recovery Potential
According to the results obtained, the implementation of source separation systems can lead to more efficient nutrient recovery in the studied regions. Both phosphorous and nitrogen recovery potentials increased significantly in Scenarios 1 and 2 in comparison to the Reference System (Figure 2). The total phosphorus (P) recovery potential of the Reference System was 164 tonnes P/year in total (Figure 2; Table 5). From these, only a relatively small fraction (3.9%) derived from the “rural non-sewered” areas while the “peri-urban sewered” contributed with ca 45%. In the Reference System, the potential in rural areas is low due to on-site treatment, where most of the nutrients are discharged and accumulate in the soil, as only 15% remains in the sludge transported to the WWTP (see Table 3). The phosphorus recovery potential of Scenarios 1 and 2 were 297 tonnes P/year and 233 tonnes P/year respectively mostly due to increased recovery in rural non-sewered areas. Therefore, the phosphorous recovery potential could increase significantly (41–81%) if source separation systems were to be implemented in the peri-urban and rural areas of the regions studied. In addition, the phosphorus recovered in the Reference System is chemically bonded (mostly to Fe and Al from the coagulation chemicals used in the study area) with low plant availability (Ylivainio et al., 2020). On the other hand, the phosphorus recovered in Scenarios 1 and 2 is of better quality for agricultural use with 44–62% present in plant-available form in northern Finland and 51–74% in northern Sweden. A fraction of the phosphorus in source separated urine and blackwater, will precipitate in plant available form either spontaneously or due to chemical additions used in treatment technologies (such as magnesium or calcium) (Harder et al., 2019; Larsen et al., 2021b). Moreover, the amount of harmful substances like metals in sludge recovered from source separation systems is lower than in sludge from centralised systems (Jönsson et al., 2005; Tidåker et al., 2006b; Viskari et al., 2018; Saliu and Oladoja, 2021). This can potentially increase the social acceptance of wastewater-based nutrients in agriculture, although the presence of pharmaceuticals and hormones are often regarded as limiting factors, even though it has been suggested that they are likely to be degraded during the growing season (Viskari et al., 2018).
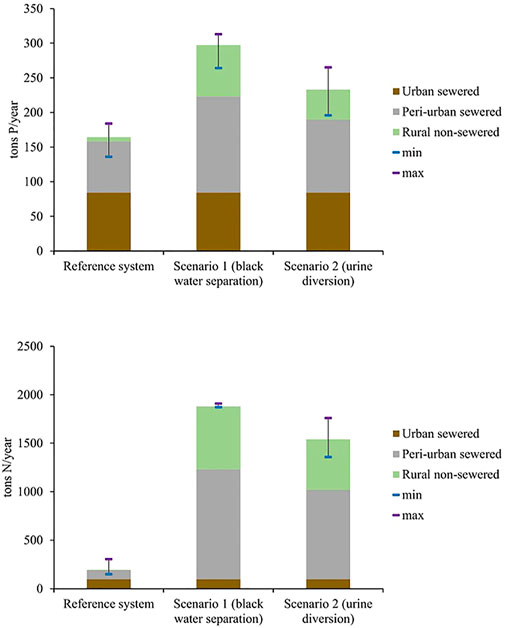
FIGURE 2. Recovery potential of phosphorus (A) and nitrogen (B) (P/N tonnes/year) including the three studied regions in northern Finland and Sweden.
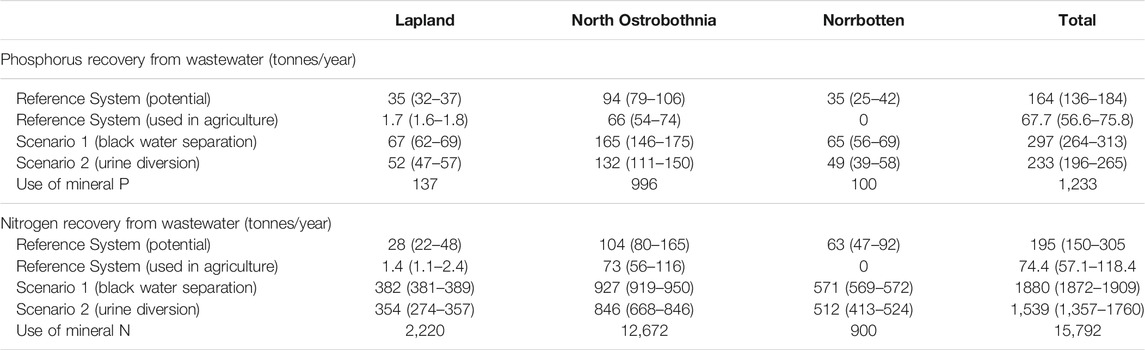
TABLE 5. Phosphorus and nitrogen recovery potential in the studied regions. The average followed by maximum and minimum values (in parentheses) regarding the recovery potential of different treatment processes and residual streams. Extracted from available studies (Table 3) and compared to the use of mineral fertilizers.
In the Reference System, the total nitrogen recovery potential in northern Finland and Sweden was 195 tonnes N/year (Figure 2; Table 5), from which 24% was in readily plant-available (soluble) form as most of the soluble nitrogen is lost in treatment processes. The nitrogen recovery potentials of Scenarios 1 and 2 were substantially higher (689–864% respectively) than the Reference System. This high increase in nitrogen recovery is a consequence of practices that aim to recover nitrogen from all wastewater fractions (i.e., reject waters from AD and urine), rather than losing it in gas emissions to the atmosphere (activated sludge process, composting) or circulating it back to the process with reject water. Moreover, the fraction of soluble nitrogen contained in the total N was also higher (average 93%), reflecting the substantial potential for recovery of easily plant-available nitrogen. In addition, it is important to highlight that the “urban sewered” areas contributed only 5–6% of the total N recovery potential in Scenarios 1 and 2 which once again reflects the significant potential of source separation in peri-urban and rural areas. In order to increase the recovery of (soluble) nitrogen in urban areas (Reference System), the nutrients contained in reject from anaerobic digestion plants could be recovered with new technologies. However, the application of recovered nutrients was not included in the results of nutrient recovery potential. It is worth noting that soluble nitrogen evaporates easily as ammonia, and to retain the high potential of soluble nitrogen in recovered end products, nutrients need to be stored and applied to fields in accordance with good practices to minimize evaporation.
There were no significant differences in nutrient recovery potential among the three regions studied, as populations are largely distributed in the same way between urban, peri-urban and rural areas. However, measurable differences were observed. In Norrbotten, the proportion of the population in peri-urban areas (45%) was higher than in Lapland (28%) and in North Ostrobothnia (31%) (Table 1). This resulted in higher nutrient recovery potentials from peri-urban areas in the Norrbotten region. However, the total nutrient recovery potential of Norrbotten and North Ostrothnia regions were very similar (Table 5).
Based on the estimated nutrient recovery potential (average) of Scenarios 1 and 2, wastewater-derived nutrients could replace 38–49% of mineral phosphorus fertilizers used in Lapland, 13–17% in North Ostrobothnia and 49–65% in Norrbotten (Table 5). In the case that soluble nitrogen would replace the use of mineral nitrogen fertilizers in agriculture, source separation could cover 13–16% of the need for mineral nitrogen in Lapland, 5–7% in North Ostrobothnia and 50–60% in Norrbotten. However, it is important to note that, despite the substantial phosphorus recovery potential from wastewater in the Reference System, in reality this potential is not realized as sewage sludge is not currently used in agriculture (mostly used in landscaping) in Lapland and Norrbotten. If nutrient recovery measures are implemented and recovery improves in the regions studied, policy measures will be essential to ensure nutrient recycling back to agriculture. The final products with a high fertilizer value could be used in or near production areas to reduce the environmental impacts of transportation. However, due to the short growing season in the studied regions, longer transportation could allow their application in other areas where the climate is not a limiting factor. The resulting benefits and trade-offs in that context would need to be studied further.
Regarding the reliability of estimated nutrient recovery potentials, the range of minimum and maximum rates of nutrient recovery potentials (percentages) from different treatment processes and residual streams were used to indicate the variance of technologies (Table 3, Table 5). The results showed that variation is largest among nitrogen recovery in the Reference System, where the total N recovery would result in 24% lower or 57% higher recovery compared to the average value used. The high variation is a consequence of the high variability observed in solid-liquid separation technologies and sludge composting (Table 3) and the fact that liquid fractions are circulated back to the processes in the Reference System. Instead, the variation is lower in the two proposed scenarios because the nutrients in liquid fraction are considered a resource: Scenario 1, min. −0.4%, maximum, +1.5%; and Scenario 2, min. −11%, maximum, +14%. For phosphorus recovery, the variation in the Reference System is min. −26%, max. +12%, for Scenario 1, min. −16, max +5% and for Scenario 2, min. −26, max. +14%.
Source separation systems can become a critical part of resource-efficient wastewater management as they offer an opportunity to improve the safety and reusability of recovered nutrients. Blackwater separation (Scenario 1) can potentially recover slightly more nutrients than urine diversion since it recovers nutrients both in urine and faeces (Table 5). In addition, black water separation also provides the opportunity to recover organic matter and the utilization of other feedstocks for anaerobic digestors (not considered in this study). For example, kitchen waste can be collected and treated anaerobically together with black water to produce energy in the form of biogas and digestate (residual) for fertilizer use, increasing the nutrient and energy recovery potential even further.
Urine diversion can be feasible in rural areas, especially if it is treated on-site to decrease the volume for collection and transportation (e.g., Malila et al., 2019; Turlan, 2019). In peri-urban areas, the collection of urine might require a separate sewage system or on-site treatment also aiming at reducing the need for transportation. Practical issues related to urine diversion also occur, e.g., urine forms struvite spontaneously, which might clog pipes and pose a risk to the functioning of collection and pre-treatment systems (Doyle and Parsons, 2002; Altinbas et al., 2009; von Bahr and Kärrman, 2019).
3.2 Environmental Indicators
The environmental impacts of the Reference System and defined scenarios in the regions studied was estimated based on values reported by Lehtoranta et al. (2022) for urban and peri-urban areas and by Malila et al. (2019) for rural areas (Table 4).
3.2.1 Climate Change Impacts
The estimated climate change impacts of the Reference System (Figure 3) were 9,700 tonnes of CO2 eq./year in Lapland, 22,800 tonnes of CO2 eq./a in North Ostrobothnia and 13,700 tonnes of CO2 eq./year in Norrbotten. The implementation of source separation Scenarios 1 and 2 would result in 23.8 and 25.6% lower generation of CO2 eq./year (respectively) than in the Reference System in the three regions (Figure 3).
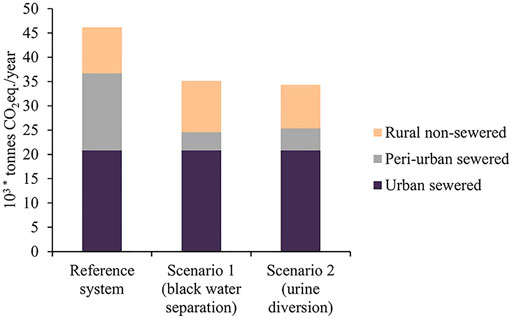
FIGURE 3. Climate change impact (tonnes CO2 eq./year) of Reference System and defined source separation Scenarios 1 and 2 as well as the contribution of urban, rural and peri-urban areas.
The effect of source separation on climate change impacts was strongest in the Norrbotten region. This is due to the fact that 45% of the population in Norrbotten live in peri-urban areas with lower emission factors than in rural areas (see Table 4). In the North Ostrobothnia and Lapland regions, the respective figure is 28–31%. Altogether, rural areas contributed 20% of the CO2 eq. emissions in the Reference System, 30% in Scenario 1 and 26% in Scenario 2. About 34% of the climate change impacts are derived from peri-urban areas in the Reference System, and 10–13% from Scenarios 1 and 2 respectively. The highest share of CO2 eq. emissions originated from households in urban areas (45–60%).
In total, the results of this study showed that the emissions of CO2-eq. could decrease by 18–32%, depending on the region, if source separation systems were implemented both in rural and peri-urban areas. However, the results indicate a risk of increased climate impacts in sparsely populated rural areas if source separation (both urine diversion and black water) systems are implemented, but substantially lower emissions for peri-urban areas. Moreover, the separation of black water caused higher climate impacts compared to the urine diversion. This is because in the areas with urine diversion (rural areas), using the dry toilet reduced the transportation and treatment of brown and grey water at WWTP.
The results obtained depend on the boundaries used and the assumptions made in the background data (Malila et al., 2019; Lehtoranta et al., 2022). For example, the recovered nutrients were assumed to decrease the need for mineral fertilizers. The production of mineral nitrogen fertilizers is energy-intensive and causes greenhouse gas emissions of about 3.67 kg CO2 eq./kg N (Brentrup et al., 2016). Replacing the use of mineral fertilizers with the recovered nitrogen would directly save 5.3–6.4 tonnes of greenhouse gas emissions annually. Source separation also enables energy production, which contributed significantly to the environmental performance of Scenario 1, particularly because it was assumed to replace the use of fossil fuels. The production of biogas could be even higher if local biogas plants would for example, enhance the collection and digestion of kitchen waste. Moreover, the background data for the urine diversion did not include any advanced treatment. If the processing of urine (or blackwater) would have been included, the climate change impacts would have been higher for Scenario 2 due to increased energy consumption.
The system boundaries of the LCA in both peri-urban and rural context did not include the nutrient potential of reject waters of black water digestion to replace mineral fertilizers. Thus, the avoided impacts are underestimated regarding the nutrient recovery potential. The potential to reduce climate impacts, as the utilization of the nutrients in the reject water replaces the use of mineral fertilizers has proven to be high (Lehtoranta et al., 2022). However, the potential benefits in terms of energy and substituted mineral fertilizers might not be achieved if the use of mineral fertilizers is not reduced in the same proportion (IPCC, 2014). Furthermore, the future development of an emission factor for mineral fertilizers will have a significant impact on avoided emissions.
One of the main contributors to climate change in source separation systems is on-site emissions, which are highly uncertain due to a lack of research data, especially on the cold climatic conditions in the Nordic regions. In sparsely populated areas, some studies indicate that long transport distances have a significant impact on climate emissions (Lehtoranta et al., 2014), but according to Turlan (2019), the results show the opposite. However, the impact of transporting black water, for example, can be minimized by using a low-flush or vacuum toilet in black water separation (Lehtoranta et al., 2014) or on-site treatment for urine to reduce volume. In conventional wastewater treatment, the largest contributions originate from purification processes aiming at nutrient and organic matter removal.
3.2.2 Eutrophication Impacts
The total eutrophication impact of the Reference System was estimated to be 435 tonnes of PO4 eq./year (Figure 4). By implementing source-separation systems in peri-urban and rural areas, eutrophication impacts can be decreased by 46–54% in Lapland, 45–54% in North Ostrobothnia and 41–52% in Norrbotten for Scenarios 1 and 2 respectively. The reduction in eutrophication results from the improvements in rural on-site wastewater systems and improved nutrient recovery and reuse. The total eutrophication impact of the three regions was 44% lower in Scenario 1 and 53% lower in Scenario 2 when compared to the Reference System. The highest reduction in the eutrophication impacts due to the implementation of source separation was observed in rural areas (80 and 87% Scenarios 1 and 2, respectively).
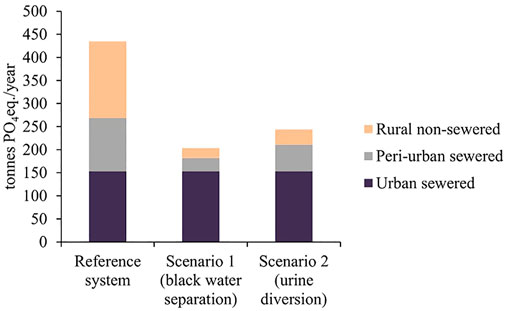
FIGURE 4. Eutrophication (tonnes PO4 eq./year) impacts of Reference System and defined source separation Scenarios 1 and 2 as well as the contribution of urban, rural and peri-urban areas.
The results obtained agree with most available reports stating that source separation systems have lower eutrophication impacts when compared to conventional systems (Tidåker et al., 2006a; Spånberg et al., 2014; Malila et al., 2019; Lehtoranta et al., 2022). On the other hand, Kjerstadius et al. (2016) estimated that freshwater eutrophication was higher for source separation systems (mainly due to nutrient extraction in the ammonia stripper) but lower than the conventional system for marine eutrophication. However, eutrophication impacts rely heavily in several agricultural pressures and the physical attributes of the catchments (Dupas et al., 2015), and therefore the LCA results should be viewed critically (Morelli et al., 2018). A scenario modeling of the catchment area would further contribute to the evaluation of the eutrophication impacts of source separation in regional level.
3.2.3 Acidification
The total acidification impact of the three regions was 168 tonnes of SO2 eq./year (Figure 5) for the Reference System. If implemented, the source separation scenarios would increase acidification impacts (101% for Scenario 1 and 190% for Scenario 2) in comparison to the Reference System, with the largest contributors being the ammonia emissions from storage and field application of sludge in black water and urine scenarios (Malila et al., 2019; Lehtoranta et al., 2022). Ammonia emissions from sewage sludge application and storage are low because there is little soluble nitrogen left in the composted dry fraction of sewage sludge.
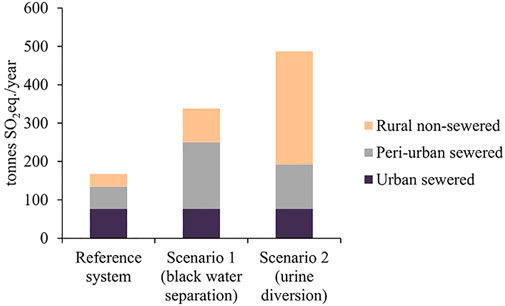
FIGURE 5. Acidification (tonnes SO2 eq./year) impacts of Reference System and defined source separation Scenarios 1 and 2 as well as the contribution of urban, rural and peri-urban areas.
The increased risk of acidification in source separation systems has also been recognized in other studies (Tidåker et al., 2006a; Spånberg et al., 2014). The results show that the urine diversion has the highest risk of acidification. However, the background data of LCA (Malila et al., 2019; Lehtoranta, et al., 2022) do not consider any sophisticated nutrient recovery or spreading technologies which could potentially decrease the impacts. Also, the black water scenario (Scenario 1) does not include the potential of nutrients in reject water (Lehtoranta et al., 2022), which results in lower acidification than Scenario 2. To ensure that ammonia evaporation is kept to a minimum, the urine and black water must be processed further and appropriate storage and spreading practices should be used (Webb et al., 2005).
3.3 Overall Considerations
It is important to highlight that LCA and LCA-based studies (such as the work reported here) are dependent on the study boundaries, assumptions and available data. Such studies often differ in the design of the scenarios, system boundaries and functional units. Region-specific data and assumptions, such as electricity mix used in the region, can also have significant effects. The results on emissions can differ greatly depending on the treatment techniques for the different fractions, or the inclusion of fertilizer production, field and land application of waste fractions and replacement of nutrients on farmland, for example.
In this study, previous studies were applied to create a streamlined analysis that highlights the potential of source separating systems in Nordic regions. Although the results show significant potential in decreasing the overall environmental impacts, it is clear, that many factors affect the benefits achieved and their realization. Moreover, Lehtoranta et al. (2022) stated that the environmental impacts of source separation are highly related to the planning and design of the systems as well as the policy measures supporting it. Literature reports from previous LCA studies on source separation systems reveal substantial variations in the estimated climate impacts. While some studies report that source separation systems have lower carbon footprints than conventional wastewater treatment (Spångberg et al., 2014; Kjerstadius et al., 2017; Lehtoranta et al., 2022), others show the opposite (Thibodeau et al., 2014) or no difference (Tidåker et al., 2006a). It is therefore evident that an LCA tailored specifically to the sparsely populated Nordic region, would bring added value.
Overall, the assessment of the climate impacts of regional source separation systems would require a systematic and more comprehensive analysis of life cycle impacts, especially the impacts on soil and land use, which are typically excluded from LCA studies due to incomplete methods and uncertain impact assessments (Brandão et al., 2013; Petersen et al., 2013; Arzoumanidis et al., 2014; Soimakallio et al., 2015; Celestina et al., 2019). In general, nutrient recycling leads to an improvement in environmental performance by decreasing the need for mineral fertilizers and helping to restore the organic matter content of soil, which improves soil structure and micro-organism activities and diminishes nutrient leaching (Liang et al., 2017; Wiesmeier et al., 2019). Furthermore, any changes in nutrient recovery and sludge production and processing affects the carbon balance of the region. The impacts of harmful substances on soil biota and carbon sequestering is not well known and requires more research so that their effects can be included in LCA studies. Overall, the role of soil microbes in the carbon cycle is still poorly understood (Liang et al., 2017; Chenu et al., 2019).
However, in order to exploit the nutrient recovery potential at a regional level, more research on environmental impacts is needed. Moreover, a feasibility study on the most relevant technologies for northern climatic conditions would be of great interest. Also, for example, the impact of transportation is significant in sparsely populated areas with long distances (Lehtoranta et al., 2014). In order to assess the transport distances required for separate collection and the need for new regional treatment facilities, the issue should be addressed through comprehensive regional logistics modeling. Thus, the question of sludge treatment in centralized versus decentralized plants is essential. A systemic change in wastewater treatment and nutrient recovery requires significant investments and readiness for legislative changes (McConville et al., 2017; Viskari et al., 2021). According to some studies, life cycle costs are lower in source separation than in a conventional centralized system (Wood et al., 2015; Schoen et al., 2017), especially if compensations from produced energy and fertilizers are acknowledged (Xue et al., 2016; Lehtoranta et al., 2022). Operating and maintenance costs are lower when compared with other on-site systems (Vidal et al., 2019), but in some urine diversion cases, investment costs are higher than in centralized wastewater treatment systems (Lennartsson et al., 2009; Lehtoranta et al., 2022). However, the life cycle cost of source separation should be studied and compared to the possible improvements and investments needed in municipal WWTPs. This would clarify the context and scale in which source separation would be an economically and environmentally viable alternative (McConville et al., 2017).
Although this study concentrated on rural and peri-urban areas, the urban solutions are necessary to consider when looking at the comprehensive picture of the circular economy in the water supply and sanitation sector. There are several development projects implementing source-separation in urban environment (Stowa, 2014; Lennartsson and Kvarnström, 2017; Skambraks et al., 2017; Lennartsson et al., 2019; Gomez et al., 2020). Experiments in small-scale source separation projects can enable a shift to large-scale design and implementation (Bisschops et al., 2019). However, the implementation of source separation systems is hindered by several problems, which are related to administrative issues, weakness of the interchange between knowledge development and entrepreneurial activity, and responsibilities (Lennartsson et al., 2017; Lennartsson and Kvarnström, 2017; McConville et al., 2017; Lehtoranta et al., 2021b).
This study focused on sparsely populated areas. It is important to note that source separation could reduce the diffuse pollution load of households’ on-site wastewater treatment systems. Due to long distances, the expansion of sewage networks are not economically feasible. Furthermore, the amount of septic tank sludge collected and treated in municipal WWTPs would be decreased. In cold winter conditions on-site wastewater treatment systems do not always work ideally due to reduced biological activity (Luostarinen et al., 2007; Kauppinen et al., 2014; Kinnunen et al., 2021; Vidal et al., 2021). Source separation has partially addressed this problem, as only grey waters are left for on-site treatment.
Source separation itself does not increase recycling it only allows for more efficient recycling of nutrients. Thus, to realize the potential, we need policy support for the agricultural use of wastewater-based nutrients, rather than wasting them on landscaping, whether they come from fractions separated from sewage sludge or source separation. The implementation of source separation systems would, to a large extent, require an entire system change of the wastewater treatment sector. Among water experts, nutrient recovery is acknowledged as a highly significant aspect of the circular economy, but the probability of realisation is perceived as low (Laitinen et al., 2019). Water services, as an essential communal infrastructure service, is rather conventional and rigid in terms of change (Heino, 2016). While rational planning and conventional habits prevail in the education and management of water services (Innes and Booher, 2010; Kurki, 2016), management problems have become increasingly interconnected with political and social domains (Teisman et al., 2013; Linton and Budds, 2014). Thus, in order to exploit the potential of source separation, technical and monetary investments are not sufficient, but there is a need for a wide paradigm change towards the circular economy in the water services sector.
4 Conclusion
This study showed that in sparsely populated areas such as northern Finland and Sweden, implementing source separation both in rural and peri-urban areas would significantly increase the potential for nutrient recovery from wastewaters and reduce the overall climate and eutrophication impact of wastewater management, but lead to increase in acidification. In addition, our findings show that the role of rural and peri-urban areas are important in promoting the circular economy of wastewater management. Moreover, the careful design and planning are essential to achieve environmental benefits. Based on the results obtained, the following can be stated:
- The implementation of source separation systems can lead to more efficient nutrient recovery in the studied regions.
- Both phosphorous and nitrogen recovery potentials increased significantly in Scenarios 1 (black water separation) and 2 (urine diversion) in comparison to the Reference System (centralized wastewater treatment).
- Phosphorus recovery potential could increase significantly (41–81%) if source separation systems were to be implemented in peri-urban and rural areas of the regions studied. In addition, the phosphorus recovered in Scenarios 1 and 2 would be of better quality for agricultural use, with a higher percentage in plant-available form.
- The nitrogen recovery potentials of Scenarios 1 and 2 were substantially higher (689–864%, respectively) than the Reference System. Moreover, the fraction of soluble nitrogen (easily plant available) contained in the total N was also higher.
- Black water separation (Scenario 1) can potentially recover slightly more nutrients than urine diversion (Scenario 2).
- Depending on the region, about 13–65% of the mineral phosphorus and 5–60% of mineral nitrogen fertilizers could be replaced with recovered nutrients.
- The implementation of source separation Scenarios 1 and 2 would result in 23.8 and 25.6% lower generation of CO2 eq./a (respectively) than the Reference System in the three regions.
- The total eutrophication impact of the three regions was 44% lower in Scenario 1 and 53% lower in Scenario 2 when compared to the Reference System.
- The total acidification impact in the study area was 101% higher in Scenario 1 and 190% higher in Scenario 2.
Although the benefits of a source separation system are clear, their implementation would, to a large extent, require a systemic change in the wastewater management sector and a wide paradigm change towards the circular economy.
Data Availability Statement
The original contributions presented in the study are included in the article, further inquiries can be directed to the corresponding authors.
Author Contributions
SL, VL, BV, HP, RN, and IH contributed to the conception and design of the study, the main responsibility being at corresponding author SL. BV and VL gathered the data regarding Finland and Sweden. SL was responsible on all the calculations made. SL and VL wrote the first draft of the manuscript. EH, BV, and IH commented and modified the paper. All authors contributed to manuscript by commenting, reading, and approving the submitted version.
Funding
This study was supported by the project “Small-scale wastewater treatment systems: governance, efficiency, resources recovery, environment contamination risks and innovative solutions for processes optimization (On-Site, 2019–2022)”, funded by the Interreg Nord 2014–2020 program.
Conflict of Interest
The authors declare that the research was conducted in the absence of any commercial or financial relationships that could be construed as a potential conflict of interest.
Publisher’s Note
All claims expressed in this article are solely those of the authors and do not necessarily represent those of their affiliated organizations, or those of the publisher, the editors and the reviewers. Any product that may be evaluated in this article, or claim that may be made by its manufacturer, is not guaranteed or endorsed by the publisher.
References
Al Seadi, T., Drosg, B., Fuchs, W., Rutz, D., and Janssen, R. 2013. Biogas Digestate Quality and Utilization. Published in: A. Wellinger, J. Murphy, and D. Baxter (edit.). The Biogas Handbook. Science Production and Applications. Woodhead Publishing Limited, Cambridge. S. 267–301.doi:10.1533/9780857097415.2.267
Almeida, M. C., Butler, D., and Friedler, E. (1999). At-source Domestic Wastewater Quality. Urban Water 1 (1), 49–55. doi:10.1016/s1462-0758(99)00008-4
Altinbas, M. (2009). “Nitrogen Recovery via Struvite Production,” in Environmental Technologies to Treat Nitrogen Pollution: Priciples and Engineering. Editor F. Cervantes (London: IWA Publishing), 239–268.
Arzoumanidis, I., Fullana-i-Palmer, P., Raggi, A., Gazulla, C., Raugei, M., Benveniste, G., et al. (2014). Unresolved Issues in the Accounting of Biogenic Carbon Exchanges in the Wine Sector. J. Clean. Prod. 82, 16–22. doi:10.1016/j.jclepro.2014.06.073
Bisschops, I., Kjerstadius, H., Meulman, B., and van Eekert, M. (2019). Integrated Nutrient Recovery from Source-Separated Domestic Wastewaters for Application as Fertilisers. Curr. Opin. Environ. Sustainability 40, 7–13. doi:10.1016/j.cosust.2019.06.010
Borowski, S., and Weatherley, L. (2013). Co-digestion of Solid Poultry Manure with Municipal Sewage Sludge. Bioresour. Tech. 142, 345–352. doi:10.1016/j.biortech.2013.05.047
Brandão, M., Levasseur, A., Kirschbaum, M. U. F., Weidema, B. P., Cowie, A. L., Jørgensen, S. V., et al. (2013). Key Issues and Options in Accounting for Carbon Sequestration and Temporary Storage in Life Cycle Assessment and Carbon Footprinting. Int. J. Life Cycle Assess. 18, 230–240. doi:10.1007/s11367-012-0451-6
Breen, P. F. (1990). A Mass Balance Method for Assessing the Potential of Artificial Wetlands for Wastewater Treatment. Water Res. 24 (6), 689–697. doi:10.1016/0043-1354(90)90024-z
Brentrup, F., Hoxha, A., and Christensen, B. (2016). “Carbon Footprint Analysis of mineral Fertilizer Production in Europe and Other World Regions,” in Conference paper. The 10th International Conference on Life Cycle Assessment of Food (LCA Food 2016) (College Dublin, Ireland: University).
Brentrup, F. (2009). “The Impact of mineral Fertilizers on the Carbon Footprint of Crop Production,” in Conference paper: International Plant Nutrition Colloquium XVI (Davis/US: University of California). Available at: https://www.researchgate.net/publication/312595960_The_impact_of_mineral_fertilizers_on_the_carbon_footprint_of_crop_production.
Cai, J., Liang, P., Qiu, Y., Cao, J., and Huang, X. (2020). A Mass Balance Framework to Evaluate the New Pathways of Biological Nutrient Removal in Wastewater Treatment. J. Water Process Eng. 37, 101523. doi:10.1016/j.jwpe.2020.101523
Celestina, C., Hunt, J. R., Sale, P. W. G., and Franks, A. E. (2019). Attribution of Crop Yield Responses to Application of Organic Amendments: A Critical Review. Soil Tillage Res. 186, 135–145. doi:10.1016/j.still.2018.10.002
Chenu, C., Angers, D. A., Barré, P., Derrien, D., Arrouays, D., and Balesdent, J. (2019). Increasing Organic Stocks in Agricultural Soils: Knowledge Gaps and Potential Innovations. Soil Tillage Res. 188, 41–52. doi:10.1016/j.still.2018.04.011
Dagerskog, L., and Olsson, O. (2020). Swedish Sludge Management at the Crossroads. Stockholm, Sweden: Stockholm Environment Institute.
Doyle, J. D., and Parsons, S. A. (2002). Struvite Formation, Control and Recovery. Water Res. 36, 3925–3940. doi:10.1016/s0043-1354(02)00126-4
Dupas, R., DelmasDorioz, M. J-M., DoriozMoatar, J.-M. F., Garnier, J., Moatar, F., and Gascuel-Odoux, C. (2015). Assessing the Impact of Agricultural Pressures on N and P Loads and Eutrophication Risk. Ecol. Indicators 48, 396–407. doi:10.1016/j.ecolind.2014.08.007
EMEP/EEA (2016). EMEP/EEA Air Pollutant Emission Inventory Guidebook 2016. 3.B Manure Management. European Environment Agency. Available at: https://www.eea.europa.eu/publications/emep-eea-guidebook-2016.
Etter, B., Udert, K. M., and Gounden, T. (2015). Valorisation of Urine Nutrients: Promoting Sanitation and Nutrient Recovery through Urine Separation. Switzerland: VUNA Final ReportEawag, Dübendorf.
European Commission (2017). Communication from the Commission to the European Parliament, the Council, the European Economic and Social Committee and the Committee of the Regions on the 2017 List of Critical Raw Materials for the EU. Brussels: COM/2017/0490 final, 13.9. Available at: https://eur-lex.europa.eu/legal-content/EN/TXT/?uri=CELEX:52017DC0490.
Fan, J-X., Urbain, V., and Manem, J. (1996). Nitrification and Mass Balance with a Membrane Bioreactor for Municipal Wastewater Treatment. Water Sci. Technol. 34 (1-2), 129–136.
Fidjeland, J., Svensson, S.-E., and Vinnerås, B. (2015). Ammonia Sanitization of blackwater for Safe Use as Fertilizer. Water Sci. Technol. 71, 795–800. doi:10.2166/wst.2015.033
Finnish Environment Institute (2021). Information Retrieval from the YLVA-Database 29th March 2021. Helsinki, Finland: Kirsi Merilehto.
Finnish Environment Institute (2019a). Vesihuolto -Lappi (Water Management -Lapland). Available at: https://www.ymparisto.fi/fi-FI/Vesi/Vesien_kaytto/Vesihuolto?f=Lapin_ELYkeskus (Retrieved July 13, 2020).
Finnish Environment Institute (2019b). Vesihuolto -Pohjois-Pohjanmaa (Water Management -North Ostrobothnia). Available at: https://www.ymparisto.fi/fi-FI/Vesi/Vesien_kaytto/Vesihuolto?f=PohjoisPohjanmaan_ELYkeskus (Retrieved July 13, 2020) [In Finnish].
Finnish Meteorological Institute (2021). Terminen Kasvukausi (Thermal Growing Season). Available at: https://www.ilmatieteenlaitos.fi/terminen-kasvukausi (Retrieved Jan 13, 2021) [In Finnish].
Gomez, C., Medina, B., Sabucedo, J. M., Smith, D., and Obed, J. (2020). Recovery and Utilization of Nutrients 4 Low Impact Fertilizer. European Union Horizon 2020. Run4Life. Available at: https://run4life-project.eu/wp-content/uploads/2020/06/Run4Life-Deliverable-6.1-Initial-Mapping-of-Stakeholder-and-Expectations.pdf.
Government Decree on Treating Domestic (2011). Government Decree on Treating Domestic Wastewater in Areas outside Sewer Networks 209/2011. Finland: Ministry of the Environment. Available at: https://www.finlex.fi/fi/laki/kaannokset/2011/en20110209.pdf.
Grönroos, J., Munther, J., and Luostarinen, S. (2017). Calculation of Atmospheric Nitrogen and NMVOC Emissions from Finnish Agriculture. Description of the Revised Model. Reports of the Finnish Environment Institute, 37.
Harder, R., Wielemaker, R., Larsen, T. A., Zeeman, G., and Öberg, G. (2019). Recycling Nutrients Contained in Human Excreta to Agriculture: Pathways, Processes, and Products. Crit. Rev. Environ. Sci. Tech. 49 (8), 695–743. doi:10.1080/10643389.2018.1558889
Hedström, A., Svensson, G., and Viklander, M. (2016). Challenges in Water and Wastewater Transport Systems in Cold Regions. Advances in Particle Science and Separation Meeting Tomorrows Challenges, 1–8.
Heino, O. (2016). Paradigman Jäljillä. Tutkimus Vesihuollon Ajattelumalleista. (Exploring the Paradigm. Research on Thought Patterns of Water Services)Doctoral Dissertation. Tampere University of Technology. Available at: https://trepo.tuni.fi/handle/10024/114521 [In Finnish].
HELCOM (2013). Nutrient Input Reduction Scheme. Available at: https://helcom.fi/baltic-sea-action-plan/nutrient-reduction-scheme/targets/.
Hjorth, M., Christensen, K. V., Christensen, M. L., and Sommer, S. G. (2010). Solid-liquid Separation of Animal Slurry in Theory and Practice. A Review. Agron. Sustain. Dev. 30, 153–180. doi:10.1051/agro/2009010
Hoffmann, S., Feldmann, U., Bach, P. M., Binz, C., Farrelly, M., Frantzeskaki, N., et al. (2020). A Research Agenda for the Future of Urban Water Management: Exploring the Potential of Nongrid, Small-Grid, and Hybrid Solutions. Environ. Sci. Technol. 54, 5312–5322. doi:10.1021/acs.est.9b05222
Hong, Y., Lee, I., Lee, W., and Kim, H. (2019). Mass-balance-model-based Evaluation of Sewage Treatment Plant Contribution to Residual Pharmaceuticals in Environmental Waters. Chemosphere 225, 378–387. doi:10.1016/j.chemosphere.2019.03.046
IPCC (2006). “2006 IPCC Guidelines for National Greenhouse Gas Inventories,” in Prepared by the National Greenhouse Gas Inven-Tories Programme. Editors H. S. Eggleston, L. Buendia, K. Miwa, T. Ngara, and K. Tanabe (Japan: IGES).
Innes, J., and Booher, D. (2010). Planning with Complexity. An Introduction to Collaborative Rationality for Public Policy. New York, NY: Routledge.
IPCC (20142014). Climate Change 2014. Mitigation of Climate Change. Intergovernmental Panel on Climate Change. WGIII. Work-Ing Group III Contribution to the Fifth Assessment Report of the Intergovernmental Panel on Climate Change. Cambridge University Press.
Jönsson, H., Baky, A., Jeppsson, U., Hellström, D., and Kärrman, E. (2005). Composition of Urine, Faeces, Greywater and Biowaste. Urban: Urban Water - Chalmers University of Technology, 6–49. Water Report.
Kallio, J., and Suikkanen, J. (2019). Muistio Haja-Asutusalueiden Jätevedenkäsittelyn Toimeenpanon Alueellisesta Tilanteesta 2019. (Memo of the Regional Situation in Implementing On-Site Sanitation in 2019). Finnish Environment Institute, 25.6. Available at: https://www.google.com/url?sa=t&rct=j&q=&esrc=s&source=web&cd=&ved=2ahUKEwjXutygg7LvAhXElYsKHTHsCkQQFjAAegQIARAD&url=https%3A%2F%2Fwww.ymparisto.fi%2Fdownload%2Fnoname%2F%257B4B2C6DDE-A31E-4F31-825D-FDFB6D6F0A0F%257D%2F147799&usg=AOvVaw2ytivZodq4KF_Pxzpf2ff0 [In Finnish].
Kallio, J. (2020). Jätevesineuvonta Haja-Asutusalueilla 2011-2019 – Loppuraportti. (On-Site Wastewater Treatment Guidance 2011-2019 – Final Report). Suomen ympäristökeskuksen raportteja 48 | 2020. Helsinki: Finnish Environment Institute. Available at: https://helda.helsinki.fi/bitstream/handle/10138/323554/SYKEra_48_2020_Jatevesineuvonta.pdf?sequence=1&isAllowed=y [In Finnish].
Karak, T., and Bhattacharyya, P. (2011). Human Urine as a Source of Alternative Natural Fertilizer in Agriculture: A Flight of Fancy or an Achievable Reality. Resour. Conservation Recycling 55 (4), 400–408. doi:10.1016/j.resconrec.2010.12.008
Karlsson, S., and Rodhe, L. (2002). Översyn Av Statistiska Centralbyråns Beräkning Av Ammoniakavgången I Jordbruket – Emissionsfaktorer För Ammoniak Vid Lagring Och Spridning Av Stallgödsel. Sweden: JTI Uppdragsrapport.
Kauppinen, A., Martikainen, K., Matikka, V., Veijalainen, A.-M., Pitkänen, T., Heinonen-Tanski, H., et al. (2014). Sand Filters for Removal of Microbes and Nutrients from Wastewater during a One-Year Pilot Study in a Cold Temperate Climate. J. Environ. Manage. 133, 206–213. doi:10.1016/j.jenvman.2013.12.008
Khiewwijit, R., Temmink, H., Rijnaarts, H., and Keesman, K. J. (2015). Energy and Nutrient Recovery for Municipal Wastewater Treatment: How to Design a Feasible Plant Layout? Environ. Model. Softw. 68, 156–165. doi:10.1016/j.envsoft.2015.02.011
Kinnunen, J., Rossi, P. M., Herrmann, I., Ronkanen, A-K., and Heiderscheidt, E. (2021). Factors Affecting Effluent Quality in On-Site Decentralized Wastewater Treatment Systems in Cold Climate Regions. Nordic Wastewater Conf. NORDIWA 2021, 28–0901. (ONLINE)./10/2021.
Kjerstadius, H., Bernstad Saraiva, A., Spångberg, J., and Davidsson, Å (2017). Carbon Footprint of Urban Source Separation for Nutrient Recovery. J. Environ. Manage. 197, 250–257. doi:10.1016/j.jenvman.2017.03.094
Kjerstadius, H., Saraiva, B. A., and Spångberg, J. (2016). Can Source Separation Increase Sustainability of Sanitation Management? Report Nr.5. Lund: VA-teknik Södra.
Konola, I., and Toivikko, S. (2019). Yhdyskuntalietteen Käsittelyn Ja Hyödyntämisen Nykytilannekatsaus. Suomen Vesilaitosyhdistys. Helsinki. Vesilaitosyhdistyksen monistesarja nro 57. Available at: https://www.vvy.fi/site/assets/files/3078/yhdyskuntaliet-teen_ka_sittelyn_ja_hyo_dynta_misen_nykytilannekatsaus_vvy_nr_57.pdf [In Finnish].
Kurki, V. (2016). Negotiating Groundwater Governance. Lessons from Contentious Aquifer Recharge projectsDoctoral Dissertation. Tampere University of Technology.
Laak, R. (1974). “Relative Pollution Strengths of Undiluted Waste Materials Discharged in Households and the Dilution Waters Used for Each,” in Manual of Grey Water Treatment Practice. Editor J. H. T. Winneberger (Michigan, USA: Ann Arbor, 68–78.
Laitinen, J., Antikainen, R., Hukka, J. J., and Katko, T. S. (2019). Water Supply and Sanitation in a green Economy Society: the Case of Finland. Public Works Manag. Pol. 25 (1), 33–50. doi:10.1177/1087724X19847211
Lam, L., Kurisu, K., and Hanaki, K. (2015). Comparative Environmental Impacts of Source-Separation Systems for Domestic Wastewater Management in Rural China. J. Clean. Prod. 104, 185–198. doi:10.1016/j.jclepro.2015.04.126
Lapinlampi, T. 2021. Vesihuoltolaitokset 2014. Viemärilaitokset. (Water Utilities 2014. Wastewater). Suomen Ympäristökeskuksen Raportteja 23 | 2021. Finnish Environment Institute, Helsinki. SYKEra_23_2021_Vesihuoltolaitokset-2014-Viemarilaitokset.pdf [In Finnish].
Larsen, T. A., Gruendl, H., and Binz, C. (2021a). The Potential Contribution of Urine Source Separation to the SDG Agenda - a Review of the Progress So Far and Future Development Options. Environ. Sci. Water Res. Technol. doi:10.1039/d0ew01064b
Larsen, T. A., Riechmann, M. E., and Udert, K. M. (2021b). State of the Art of Urine Treatment Technologies: A Critical Review. Water Res. X 13, 100114. doi:10.1016/j.wroa.2021.100114
Lauronen, M. (2017). Jätevesilietteen Eri Käsittelyvaihtoehtojen Kasvihuonekaasupäästöjen Vertailu Pohjoisissa Olosuhteissa. (Greenhouse Gas Emissions of Different Wastewater Sludge Treatment Alternatives in the Northern Periphery). Oulu: Master’s thesis. University of Oulu.
Lehtoranta, S., Malila, R., Fjäder, P., Laukka, V., Mustajoki, J., and Äystö, L. (2021a). Jätevesien ravinteet kiertoon turvallisesti ja tehokkaasti (Efficient and Safe Nurtient Recovery from Municipal Wastewaters). Suomen ympäristökeskuksen raportteja 18, 2021. Helsinki: Finnish Environment Institute [In Finnish].
Lehtoranta, S., Malila, R., Kämäri, T., Johansson, A., Särkilahti, M., and Viskari, E-L. (2021b). Vesihuollon kiertotalouden edistäminen kaupunkiympäristössä. Erottelevan sanitaation mahdollisuudet. (Promoting Circular Economy of Water Supply in Urban Environment). Suomen ympäristökeskuksen raportteja 6/2021. Helsinki: Finnish Environment Institute. [In Finnish].
Lehtoranta, S., Malila, R., Särkilahti, M., and Viskari, E-L. (2022). To Separate or Not? A Comparison of Wastewater Management Systems for the new city District of Hiedanranta. Finland. Environ. Res. 208, 112764. doi:10.1016/j.envres.2022.112764
Lehtoranta, S., Vilpas, R., and Mattila, T. J. (2014). Comparison of Carbon Footprints and Eutrophication Impacts of Rural On-Site Wastewater Treatment Plants in Finland. J. Clean. Prod. 65, 439–446. doi:10.1016/j.jclepro.2013.08.024
Lennartsson, M., Kvarnstöm, E., McConville, J., and Hagman, M. (2017). Planning and Implementation of Source-Separating Wastewater Systems. The Importance of Policies and People. MACRO- Mat I Cirkulära Robusta System. Available at: http://www.macrosystem.se/wp-content/uploads/2017/10/SRS_H_planningandimplement_liggande.pdf.
Lennartsson, M., and Kvarnström, E. (2017). Food and Energy in a Circular Economy, 2017. Final Report. Stockholm June 20, 2017Available at: https://carbonneutralcities.org/wp-content/uploads/2018/05/Food-and-Energy-in-the-Circular-Economy.pdf.
Lennartsson, M., Kvarnström, E., Lundbreg, T., Buenfil, J., and Sawyer, R. (2009). Comparing Sanitation Systems Using Sustainability Criteria. Stockholm, Sweden: Stockholm Environment InstituteEcoSanRes Series, 2009–2011.
Lennartsson, M., McConville, J., Kvarnström, E., Hagman, M., and Kjerstadius, H. (2019). Investments in Innovative, Urban Sanitation- Decision-Making Processes in Sweden. Water Alternatives 12 (2), 588–608.
Liang, C., Schimel, J. P., and Jastrow, J. D. (2017). The Importance of Anabolism in Microbial Control over Soil Carbon Storage. Nat. Microbiol. 2, 17105. doi:10.1038/nmicrobiol.2017.105
Linton, J., and Budds, J. (2014). The Hydrosocial Cycle: Defining and Mobilizing a Relational-Dialectical Approach to Water. Geoforum 57, 170–180. doi:10.1016/j.geoforum.2013.10.008
Luostarinen, S., Grönroos, J., Lemola, R., Lehtonen, E., Lehtoranta, S., Tampio, E., et al. (2019). “Ravinnelaskuri – Alueellisen ravinnekierron suunnittelun työkalu. (Nutrient calculator),” in Luonnonvara- ja biotalouden tutkimus (Finland: Natural resouces Institute Finland). Available at: https://www.luke.fi/projektit/ravinnelaskuri/ [In Finnish].
Luostarinen, S., Sanders, W., Kujawa-Roeleveld, K., and Zeeman, G. (2007). Effect of Temperature on Anaerobic Treatment of Black Water in UASB-Septic Tank Systems. Bioresour. Tech. 98 (5), 980–986. doi:10.1016/j.biortech.2006.04.018
Mönkäre, T., Kinnunen, V., Tampio, E., Ervasti, S., Lehtonen, E., Kettunen, R., et al. (2016). Ravinnevisio - Selvitys Pirkanmaan Puhdistamolietteiden Ja Biojätteiden Ravinteista Ja Niiden Potentiaalisesta Käytöstä. Pirkanmaan Elinkeino-, Liikenne- Ja Ympäristökeskus, Helsinki. Raportteja 74, 2016. Available at: https://www.doria.fi/bitstream/han-dle/10024/124381/Raportteja%2074%202016.pdf?sequence=2 [in Finnish].
Magdoff, F. R., and Chromec, F. W. (1977). Nitrogen Mineralization from Sewage Sludge. J. Environ. Sci. Health . A: Environ. Sci. Eng. 12, 191–201. doi:10.1080/10934527709374747
Magnusson, K., and Norén, F. (2014). Screening of Microplastic Particles in and Down-Stream a Wastewater Treatment Plant. Sweden: IVL Swedish Environmental Research Institute. Number C 55 August 2014 Report.
Malila, R., Lehtoranta, S., and Viskari, E.-L. (2019). The Role of Source Separation in Nutrient Recovery - Comparison of Alternative Wastewater Treatment Systems. J. Clean. Prod. 219, 350–358. doi:10.1016/j.jclepro.2019.02.024
Malm, A., Svensson, G., Bäckman, H., and Morrison, G. M. (2013). Prediction of Water and Wastewater Networks Rehabilitation Based Current Age and Material Distribution. Water Sci. Technol. Water Supply 13, 227–237. doi:10.2166/ws.2013.011
Marttinen, S., Venelampi, O., Iho, A., Koikkalainen, K., Lehtonen, E., Luostarinen, S., et al. (2017). Kohti Ravinteiden Kierrätyksen Läpimurtoa. Nykytila Ja Suositukset Ohjauskeinojen Kehittämiseksi Suomessa. (Towards the Breakthrough in Nutrient recyclingCurrent Status and Recommendations for Developing Guidelines in Finland) Luonnonvarakeskus. Helsinki. Luonnonvara- ja biotalouden tutkimus 45/2017. Available at: http://ju-kuri.luke.fi/bitstream/handle/10024/540214/luke-luobio_45_2017.pdf?sequence=12 [in Finnish].
Maurer, M., Pronk, W., and Larsen, T. A. (2006). Treatment Processes for Source-Separated Urine. Water Res. 40, 3151–3166. doi:10.1016/j.watres.2006.07.012
McConville, J. R., Kvarnström, E., Jönsson, H., Kärrman, E., and Johansson, M. (2017). Source Separation: Challenges & Opportunities for Transition in the Swedish Wastewater Sector. Resour. Conservation Recycling 120, 144–156. doi:10.1016/J.RESCONREC.2016.12.004
Ministry of the Environment, Finland (2019). Ravinteiden Kierrätyksen Toimenpideohjelma 2019-2030. (Operational Program for Nutrient Recycling 2019-2030). Available at: Ravinteiden-kierratyksen-toimenpideohjelma-2019-2030-allekirjoitettu-D7F9043A_0090_4785_B029_9C119B566BDD-146284.pdf (ym.fi) [In Finnish].
Moerland, M. J., Borneman, A., Chatzopoulos, P., Fraile, A. G., van Eekert, M. H. A., Zeeman, G., et al. (2020). Increased (Antibiotic-Resistant) Pathogen Indicator Organism Removal during (Hyper-)Thermophilic Anaerobic Digestion of Concentrated Black Water for Safe Nutrient Recovery. Sustainability 12, 9336–9413. doi:10.3390/su12229336
Møller, H. B., Sommer, S. G., and Ahring, B. K. (2002). Separation Efficiency Andparticle Size Composition in Relation to Manure Type and Storageconditions. Bioresour. Technol 85, 189–196.
Morelli, B., Hawkins, T. R., Niblick, B., Henderson, A. D., Golden, H. E., Compton, J. E., et al. (2018). Critical Review of Eutrophication Models for Life Cycle Assessment. Environ. Sci. Technol. 52 (17), 9562–9578. doi:10.1021/acs.est.8b00967
Motiva, (2020). Vedenkulutus (Water Consumption). Available: https://www.motiva.fi/koti_ja_asuminen/hyva_arki_kotona/vedenkulutus [In Finnish].
Myllymaa, T., Moliis, K., Tohka, A., Rantanen, P., Ollikainen, M., and Dahlbo, H. (2008). “Jätteiden Kierrätyksen Ja Polton Käsittelyketjujen Ympäristökuormitus Ja Kustannukset – Inventaarioraportti,” in Suomen ympäristökeskuksen raportteja (Helsinki: Finnish Environment Institute), Vol. 28 [In Finnish].
Nagy, J., and Zseni, A. (2017). Human Urine as a Efficient Fertilizer Product in Agriculture. Agron. Res. 15 (2), 490–500.
Natural Resources Institute Finland (2021). Utilized agricultural area by ELY Centre. Finland: Statisics database, Natural Resources Institute Finland. Available at: http://statdb.luke.fi/PXWeb/pxweb/en/LUKE/LUKE__02%20Maatalous__04%20Tuotanto__22%20Kaytossa%20oleva%20maatalousmaa/01_Kaytossa_oleva_maatalousmaa_ELY.px/?rxid=001bc7da-70f4-47c4-a6c2-c9100d8b50db.
Naturvårdsverket, (2018). Advanced Wastewater Treatment for Separation and Removal of Pharmaceutical Residues and Other Hazardous Substances. Needs, Technologies and Impacts. Bromma, Sweden: The Swedish Environmental Protection Agency.
Nordin, A., Ottoson, J. R., and Vinnerås, B. (2009). Sanitation of Faeces from Source-Separating Dry Toilets Using Urea. J. Appl. Microbiol. 107, 1579–1587. doi:10.1111/j.1365-2672.2009.04339.x
Öberg, G., Metson, G. S., Kuwayama, Y., and A. Conrad, S. (2020). Conventional Sewer Systems Are Too Time-Consuming, Costly and Inflexible to Meet the Challenges of the 21st century. Sustainability 12, 6518. doi:10.3390/su12166518
Olshammar, M., RosenquistEk, M. L., Ejhed, H., Sidvall, A., and Svanström, S. (2015). På Uppdrag Av Havs-Och Vattenmyndigheten Uppdatering Av Kunskapsläget Och Statistik För Små Avloppsanläggningar The State of Knowledge and Statistics for Small Sewage Plants. Sweden: Swedish Agency for Marine and Water Management. Report 166, Norrköping. [In Swedish].
Petersen, B. M., Knudsen, M. T., Hermansen, J. E., and Halberg, N. (2013). An Approach to Include Soil Carbon Changes in Life Cycle Assessments. J. Clean. Prod. 52, 217–224. doi:10.1016/j.jclepro.2013.03.007
Puig, S., van Loosdrecht, M. C. M., Colprim, J., and Meijer, S. C. F. (2008). Data Evaluation of Full-Scale Wastewater Treatment Plants by Mass Balance. Water Res. 42 (18), 4645–4655. doi:10.1016/j.watres.2008.08.009
Räike, A., Taskinen, A., and Knuuttila, S. (2020). Nutrient export from Finnish Rivers into the Baltic Sea Has Not Decreased Despite Water protection Measures. Ambio 49, 460–474. doi:10.1007/s13280-019-01217-7
Rossi, L., Reuna, S., Fred, T., and Heinonen, M. (2018). RAVITA Technology - New Innovation for Combined Phosphorus and Nitrogen Recovery. Water Sci. Tech. 78 (12), 2511–2517. doi:10.2166/wst.2019.011
ROTI (2021). Rakennetun Omaisuuden Tila 2021. (Value of the Built Environment in 2021. Available at https://www.ril.fi/media/2021/vaikuttaminen/roti2021_low.pdf [In Finnish].
Ruuhela, S. 2017. Puhdistamolietteen Käsittelyn Hankinnan Laatukriteerien Kehittäminen – RANKU-Hanke. Varsinais-Suomen Elinkeino-, Liikenne- Ja Ympäristökeskus, Turku. Raportteja 18 | 2017. Available at: https://www.doria.fi/bitstream/han-dle/10024/136226/Raportteja_18_2017.pdf?sequence=10&isAllowed=y [In Finnish].
Saliu, T. D., and Oladoja, N. A. (2021). Nutrient Recovery from Wastewater and Reuse in Agriculture: a Review. Environ. Chem. Lett. 19, 2299–2316. doi:10.1007/s10311-020-01159-7
Schoen, M. E., Xue, X., Wood, A., Hawkins, T. R., Garland, J., and Ashbolt, N. J. (2017). Cost, Energy, Global Warming, Eutrophication and Local Human Health Impacts of Community Water and Sanitation Service Options. Water Res. 109, 186–195. doi:10.1016/j.watres.2016.11.044
SEI (2020). Swedish Sludge Management at the Crossroads. Sweden: Stockholm Environment Institute. policy brief January 2020. Available at: https://www.sei.org/wp-content/uploads/2020/01/sei-2020-pb-sludge-crossroads.pdf.
Seleiman, M. F., Santanen, A., and Mäkelä, P. S. A. (2020). Recycling Sludge on Cropland as Fertilizer - Advantages and Risks. Resour. Conservation Recycling 155 (2020), 104647. doi:10.1016/j.resconrec.2019.104647
Simha, P. (2021). Alkaline Urine Dehydration. How to Dry Source Separated Human Urine and Recover Nutrients. Doctoral Thesis No. 2021:28. Uppsala: Faculty of Natural Resources and Agricultural Sciences. Swedish University of Agricultural Sciences. Available at: https://pub.epsilon.slu.se/23473/1/simha_p_210511.pdf.
Simha, P., Friedrich, C., Randall, D. G., and Vinnerås, B. (2021). Alkaline Dehydration of Human Urine Collected in Source-Separated Sanitation Systems Using Magnesium Oxide. Front. Environ. Sci. 8, 286. doi:10.3389/fenvs.2020.619901
Simha, P., and Ganesapillai, M. (2017). Ecological Sanitation and Nutrient Recovery from Human Urine: How Far Have We Come? A Review. Sust. Environ. Res. 27, 107–116. doi:10.1016/j.serj.2016.12.001
Skambraks, A.-K., Kjerstadius, H., Meier, M., Davidsson, Å, Wuttke, M., and Giese, T. (2017). Source Separation Sewage Systems as a Trend in Urban Wastewater Management: Drivers for the Implementation of Pilot Areas in Northern Europe. Sust. Cities Soc. 28, 287–296. doi:10.1016/j.scs.2016.09.013
Soimakallio, S., Cowie, A., Brandão, M., Finnveden, G., Ekvall, T., ErlandssonKoponen, M. K., et al. (2015). Attributional Life Cycle Assessment: Is a Land-Use Baseline Necessary? Int. J. Life Cycle Assess. 20, 1364–1375. doi:10.1007/s11367-015-0947-y
Spångberg, J., Tidåker, P., and Jönsson, H. (2014). Environmental Impact of Recycling Nutrients in Human Excreta to Agriculture Compared with Enhanced Wastewater Treatment. Sci. Total Environ. 493, 209–219. doi:10.1016/j.scitotenv.2014.05.123
Statistics Finland (2019). Key Figures on Population by Region, 1990-2020. Available at: https://pxnet2.stat.fi/PXWeb/pxweb/en/StatFin/StatFin__vrm__vaerak/statfin_vaerak_pxt_11ra.px/.
Statistics Sweden (2020c). Production and Use of Sewage Sludge Form Municipal Waste Water Treatment Plants by County and Use Category.
Statistics Sweden (2020d). Sales of mineral Fertilisers for Agriculture and Horticulture after Region and Plant Nutrient, Years 2018/2019.
Statistics Sweden (2020a). The Land in Sweden (Quick Facts). Available at: https://www.scb.se/hitta-statistik/sverige-i-siffror/miljo/marken-i-sverige/.
Stowa (2014). Evaluatie Nieuwe Sanitatie Noorderhoek Sneek [Evaluation of the New Sanitation in Noorderhoek Sneek]. Available at: https://www.stowa.nl/sites/default/files/assets/PUBLICATIES/Publicaties%202014/STOWA%202014-38.pdf (Vierailtu 1 18, 2021).
Swedish EPA (2018). Wastewater Treatment in Sweden- Swedish EPA Publications. Stockholm. Available at: https://www.naturvardsverket.se/Documents/publikationer6400/978-91-620-8809-5.pdf?pid=22471.
Swedish Meteorological and Hydrological Institute (2021). Data Series with normal Values of Precipitation for the Period 1981-2010. Accessible at https://www.smhi.se/data/meteorologi/dataserier-med-normalvarden-for-perioden-1981-2010-1.167776 (Accessed May 24th, 2021).
Swedish Meteorological and Hydrological Institute (2017). Thermal Growing Season. Accessible at https://www.smhi.se/data/meteorologi/temperatur/vegetationsperiodens-langd-1.4076 (Accessed May 24th, 2021).
SYKE (2019). Yhdyskuntien Jätevesien Kuormitus Vesiin. Available at: https://www.ymparisto.fi/fi-FI/Kartat_ja_tilastot/Vesihuoltorapor-tit/Yhdyskuntien_jatevesien_kuormitus_vesiin.
Talvitie, J., Mikola, A., Koistinen, A., and Setälä, O. (2017). Solutions to Microplastic Pollution - Removal of Microplastics from Wastewater Effluent with Advanced Wastewater Treatment Technologies. Water Res. 123, 401–407. doi:10.1016/j.watres.2017.07.005
Tarkka, P., and Leppänen, E. (2019). Hajajätevesilietteet Hyötykäyttöön Itä-Lapissa. Hankesuunnitelma. Accessible at https://www.savukoski.fi/wp-content/uploads/2020/02/hankesuunnitelma-02042019.pdf (Accessed January 13, 2022).
Tattari, S., Puustinen, M., Koskiaho, J., Röman, E., and Riihimäki, J. (2015). “Vesistöjen Ravinnekuormituksen Lähteet Ja Vähentämismahdollisuudet,” in Suomen ympäristökeskuksen raportteja (Helsinki: Finnish Environment Instutute), Vol. 35 [In Finnish].
Teisman, G., van Buuren, A., Edelenbos, J., and Warner, J. (2013). Water Governance: Facing the Limits of Managerialism, Water-Centricity, and Technocratic Problem-Solving. Int. J. Water Governance 1 (01-02), 1–11. doi:10.7564/12-ijwg4
Tervahauta, T., Hoang, T., Hernández, L., Zeeman, G., and Buisman, C. (2013). Prospects of Source-Separation-Based Sanitation Concepts: A Model-Based Study. Water 5 (3), 1006–1035. doi:10.3390/w5031006
Tervahauta, T., Rani, S., Hernández Leal, L., Buisman, C. J. N., and Zeeman, G. (2014). Black Water Sludge Reuse in Agriculture: Are Heavy Metals a Problem? J. Hazard. Mater. 274, 229–236. doi:10.1016/J.JHAZMAT.2014.04.018
Thibodeau, C., Monette, F., Bulle, C., and Glaus, M. (2014). Comparison of Black Water Source-Separation and Conventional Sanitation Systems Using Life Cycle Assessment. J. Clean. Prod. 67, 45–57. doi:10.1016/j.jclepro.2013.12.012
Tidåker, P., Kärrman, E., Baky, A., and Jönsson, H. (2006b). Wastewater Management Integrated with Farming -an Environmental Systems Analysis of a Swedish Country Town. Resour. Conservation Recycling 47, 295–315. doi:10.1016/j.resconrec.2005.12.003
Tidåker, P., Sjöberg, C., and Jönsson, H. (2007a). Local Recycling of Plant Nutrients from Small-Scale Wastewater Systems to farmland-A Swedish Scenario Study. Resour. Conservation Recycling 49, 388–405. doi:10.1016/j.resconrec.2006.05.004
Turlan, L. (2019). Nutrient Recovery from Urine to Agriculture in Northern Sweden. Sweden: Luleå University of Technology. Master’s thesis.
Udert, K. M., Larsen, T. A., and Gujer, W. (2006). Fate of Major Compounds in Source-Separated Urine. Water Sci. Tech. 54 (11–12), 413–420. doi:10.2166/wst.2006.921
Uzkurt Kaljunen, J., Al-Juboori, R. A., Mikola, A., Righetto, I., and Konola, I. (2021). Newly Developed Membrane Contactor-Based N and P Recovery Process: Pilot-Scale Field Experiments and Cost Analysis. J. Clean. Prod. 281, 125288. doi:10.1016/j.jclepro.2020.125288
Valtanen, M., Sillanpää, N., and Setälä, H. (2015). Key Factors Affecting Urban Runoff Pollution under Cold Climatic Conditions. J. Hydrol. 529, 1578–1589. doi:10.1016/j.jhydrol.2015.08.026
Vatten and Miljöbyrån i Sverige AB (2017). Avloppshantering I Torne Internationella Vattendistrikt-Inventering, Bedömning Och Åtgärdsförslag [Wastewater Management in the Torne Internacional Water District - Inventory, Assessment and Action Proposal]. Luleå. Report nr 16154 [In Swedish].
Venkatesan, A. K., Hamdan, A.-H. M., Chavez, V. M., Brown, J. D., and Halden, R. U. (2016). Mass Balance Model for Sustainable Phosphorus Recovery in a US Wastewater Treatment Plant. J. Environ. Qual. 45 (1), 84–89. doi:10.2134/jeq2014.11.0504
Vidal, B., Hedström, A., Barraud, S., Kärrman, E., and Herrmann, I. (2019). Assessing the Sustainability of On-Site Sanitation Systems Using Multi-Criteria Analysis. Environ. Sci. Water Res. Technol. 5, 1599–1615. doi:10.1039/c9ew00425d
Vidal, B., Kinnunen, J., Hedström, A., Heiderscheidt, E., Rossi, P. M., and Herrmann, I. (2021). Treatment Efficiency of Small-Scale Package Plants in Northern Sweden and Finland. Nordic Wastewater Conference. NORDIWA 2021 (ONLINE), 28/09-01/10/2021.
Vieno, N. (2014). Haitalliset Aineet Jätevedenpuhdistamoilla –hankkeen Loppuraportti. Harmful substances at wastewater treatment plant – final report. Helsinki: Finnish Water Utilities Association. Vesilaitosyhdistyksen monistesarja nro 34. Available at: https://www.vvy.fi/haitta-aineselvitys [In Finnish].
Vieno, N., Sarvi, M., Salo, T., Rämö, S., Ylivainio, K., Pitkänen, T., et al. (2018). Puhdistamolietteiden Sisältämien Haitta-Aineiden Aiheuttamat Riskit Lannoitekäytössä. (Risks Caused by Harmful Substances in Wastewater Sludge in Fertilizer Use). Helsinki: Natural Resources Institute Finland. Luonnonvara- ja biotalouden tutkimus 58/2018. Available at: http://urn.fi/URN:ISBN:978-952-326-661-2 ([In Finnish].
Vienonen, S. (2007). Haja-asutuksen Vedenhankinnan Ja Jätevedenkäsittelyn Tilanne Vuonna 2007. Helsinki: Finnish Environment Institute. Suomen ympäristökeskuksen raportteja 24 | 2007. Available at: http://hdl.handle.net/10138/39801 [In Finnish].
Viskari, E.-L., Grobler, G., KarimäkiGorbatova, K. A., Vilpas, R., and Lehtoranta, S. (2018). Nitrogen Recovery with Source Separation of Human Urine – Preliminary Results of its Fertilizer Potential and Use in Agriculture. Front. Sust. Food Syst. 2 (32), 1–14. doi:10.3389/fsufs.2018.00032
Viskari, E-L., Lehtoranta, S., and Malila, R. (2021). Urine: The Potential, Value Chain and its Sustainable Management. Sanitation Value Chain 5/2, 27–43.
Viskari, E-L., Vilpas, R., Lehtoranta, S., Pakula, S., and Tuukkanen, K. (2017). BIOUREA. Erilliskerätyn Virtsan Lannoitepotentiaali, Kokeelliset Tutkimukset Ja Elinkaaritarkastelu. Loppuraportti. (BIOUREA. Fertilizer Potential of Source Separated Urine, Experimental Research and Life Cycle Assessment). Available at: http://www.huussi.net/wp-content/uploads/2017/01/Huussi_loppuraportti_net_VALMIS.pdf [In Finnish].
von Bahr, B., and Kärrman, E. (2019). Tekniska Processer För Fosforåtervinning Ur Avloppsslam. RISE Rapport 2019:59. Available at: http://www.diva-portal.org/smash/get/diva2:1386080/FULLTEXT01.pdf.
Wäger-Baumann, F. (2011). Physical and Biological Methods for the Treatment of the Liquid Fraction of Anaerobic Digester Effluent. Vienna: University of Natural Resources and Applied Life Sciences. Available at: https://zidapps.boku.ac.at/abst-racts/oe_list.php?paID=3&paSID=8522&paSF=-1&paCF=0&paLIST=0&language_id=DE.
Warman, P. R., and Termeer, W. C. (2005). Evaluation of Sewage Sludge, Septic Waste and Sludge Compost Applications to Corn and Forage: Yields and N, P and K Content of Crops and Soils. Bioresour. Tech. 96, 955–961. doi:10.1016/j.biortech.2004.08.003
Webb, J., Menzi, H., Pain, B. F., Misselbrook, T. H., Dämmgen, U., Hendriks, H., et al. (2005). Managing Ammonia Emissions from Livestock Production in Europe. Environ. Pollut. 135 (3), 399–406. doi:10.1016/j.envpol.2004.11.013
Wielemaker, R. C., Weijma, J., and Zeeman, G. (2018). Harvest to Harvest: Recovering Nutrients with New Sanitation Systems for Reuse in Urban Agriculture. Resour. Conservation Recycling 128, 426–437. doi:10.1016/j.resconrec.2016.09.015
Wiesmeier, M., Urbanski, L., Hobley, E., Lang, B., von Lützow, M., Marin-Spiotta, E., et al. (2019). Soil Organic Carbon Storage as a Key Function of Soils - A Review of Drivers and Indicators at Various Scales. Geoderma 333, 149–162. doi:10.1016/j.geoderma.2018.07.026
Wood, A., Blackhurst, M., Hawkins, T., Xue, X., Ashbolt, N., and Garland, J. (2015). Cost-effectiveness of Nitrogen Mitigation by Alternative Household Wastewater Management Technologies. J. Environ. Manage. 150, 344–354. doi:10.1016/j.jenvman.2014.10.002
World Bank (2020). Population Density (People Per Sq. Km of Land Area). DNST. Accessible at https://data.worldbank.org/indicator/EN.POP (Accessed January 11, 2021).
Xue, X., Hawkins, T., Schoen, M., Garland, J., and Ashbolt, N. (2016). Comparing the Life Cycle Energy Consumption, Global Warming and Eutrophication Potentials of Several Water and Waste Service Options. Water 8 (154), 154. doi:10.3390/w8040154
Ylivainio, K., Äystö, L., Fjäder, P., Suominen, K., Lehti, A., Perkola, M., et al. (2020). Jätevesilietteen Pitkäkestoinen Fosforilannoitusvaikutus Ja Yhteys Ympäristö- Ja Ruokaturvallisuuteen: Jätevesilietteen Potentiaali Kasvintuotannossa Ja Vaikutukset Ympäristöön Ja Elintarviketurvallisuuteen (PProduct)-Hankkeen Loppuraportti. (Potential of Sewage Sludge Phosphorus in Plant Production and Impacts on Environ‐ Ment and Food Chain (PProduct)- Final Report. Natural Resources Institute Finland [In Finnish].
Keywords: wastewater, source separation, nutrient recovery, nutrient balance, environmental impacts, regional assessment
Citation: Lehtoranta S, Laukka V, Vidal B, Heiderscheidt E, Postila H, Nilivaara R and Herrmann I (2022) Circular Economy in Wastewater Management—The Potential of Source-Separating Sanitation in Rural and Peri-Urban Areas of Northern Finland and Sweden. Front. Environ. Sci. 10:804718. doi: 10.3389/fenvs.2022.804718
Received: 29 October 2021; Accepted: 27 January 2022;
Published: 23 February 2022.
Edited by:
Zifu Li, University of Science and Technology Beijing, ChinaReviewed by:
Prithvi Simha, Swedish University of Agricultural Sciences, SwedenSahar Dalahmeh, Swedish University of Agricultural Sciences, Sweden
Copyright © 2022 Lehtoranta, Laukka, Vidal, Heiderscheidt, Postila, Nilivaara and Herrmann. This is an open-access article distributed under the terms of the Creative Commons Attribution License (CC BY). The use, distribution or reproduction in other forums is permitted, provided the original author(s) and the copyright owner(s) are credited and that the original publication in this journal is cited, in accordance with accepted academic practice. No use, distribution or reproduction is permitted which does not comply with these terms.
*Correspondence: Suvi Lehtoranta, c3V2aS5sZWh0b3JhbnRhQHN5a2UuZmk=