- 1Département Productions Forestières, Institut de l’Environnement et de Recherches Agricoles, Ougadougou, Burkina Faso
- 2IAMGOLD Essakane SA, Département Environnement, Ouagadougou, Burkina Faso
- 3Laboratoire Commun de Microbiologie (LCM) IRD/ISRA/UCAD, Département de Biologie végétale, Université Cheikh Anta Diop, Dakar-Fann, Sénégal
- 4Centre d’Étude de la Forêt (CEF) and the Institut de Biologie Intégrative et des Systèmes (IBIS), Université Laval, Québec, QC, Canada
Senegalia senegal (L.) Britton (Fabaceae) is a widespread, multipurpose tree capable of colonizing disturbed Sub-Saharan mining sites given its adaptations to arid lands. The purpose of this study was to determine the potential of the association of microbial and/or manure amendments with S. senegal seedlings for the effective rehabilitation of the environment post mining. A multi-year factorial experiment involving this species was conducted in a nursery and on waste rock dumps (Essakane gold mine, NE Burkina Faso). Inoculation with arbuscular mycorrhizal (AM) fungi (native isolate Rhizophagus aggregatus DAOM2277128; commercial isolate Rhizophagus irregularis DAOM197198) and Mesorhizobium plurifarium strain ORS3588 was conducted on S. senegal seedlings. These were planted in black polythene bags filled with three substrate types: 100% sandy soil (substrate A); 75% sandy soil, 25% manure (substrate B); and 50% sandy soil, 50% manure (substrate C). Seedlings were then out-planted on waste rock to determine whether microbial inoculation improves their growth and survival rates. Under nursery conditions, manure-enriched substrates harboured less nodulation and AM colonization, but substantially increased plant height, and dry mass compared to un-amended substrate. Inoculation on manure-enrich substrates did not consistently increase root AM colonization and plant growth parameters among treatments. Plants that were inoculated with R. aggregatus alone or with R. irregularis or Mesorhizobium plurifarium ORS3588 showed the greatest increase in growth variables. On un-amended substrates, inoculation with R. aggregatus in combination with M. plurifarium or R. irregularis significantly enhanced root colonization rates, without altering plant growth parameters. Yet, inoculation with R. irregularis or M. plurifarium alone did not elicit increases in these parameters, although nodulation was greatly improved by the latter treatment. In field conditions, plant growth and survival were reduced under high rate manure amendments, likely due to less AM colonization and root nodulation that was observed for these treatments in nursery before out-planting. Strongly colonized plants on the un-amended substrate and moderately amended substrate showed greater survival after out-planting. Our results support the general conclusion that microbial inoculation and manure-enriched substrates are a viable option for mining site rehabilitation using S. senegal. They also suggest that manure amendments in forest nurseries should be cautiously applied given that high rates may impede establishment of plant symbioses, thereby affecting their performance on waste rock dumps following out-planting.
Introduction
Mining activities produce large quantities of waste, which often creates extremely stressful conditions for restoration (Straker et al., 2007; Li et al., 2014; Wang, 2017). In gold mining, the two main solid wastes are the tailings that result from the metal extraction process of gold and the overburden that can be used for pad and tailings dam construction. Accumulations of these two types of wastes are alkaline, biologically impoverished substrates (Buta et al., 2019) that contain excess inorganic contaminants, which leads to the alteration of landforms and reductions in the fauna, flora and microorganisms (Rosenvald et al., 2011; Novo et al., 2018). At Essakane (Burkina Faso), the area that was affected by mining activities reached 309.4 ha. Such extensive land disturbance needs to be ameliorated to restore it to original ecosystem conditions, or at least as closest to those conditions as it was prior to mining activities. The mining company is responsible for reclaiming disturbed sites following mining activities. To accelerate the process, land reclamation needs to be conducted using ecological technologies, including the role of native plant species as well as soil microorganisms (Bacchetta et al., 2015; Thavamani et al., 2017; Kumar et al., 2018; Novo et al., 2018). The use of phytotechnologies in remediation is recognized as a potentially cost-effective and ecologically sound alternative to current norms of degraded land rehabilitation (Grant et al., 2002; Gastauer et al., 2018; Kumar et al., 2018). One of the key factors in the success of phytoremediation technology is the use of native species and local provenances (Grant et al., 2002; Bacchetta et al., 2015; Borišev et al., 2018), which has the advantage of maintaining the integrity of natural biodiversity by minimizing changes in the existing ecosystem (Borišev et al., 2018; Gastauer et al., 2018). Indigenous species are well adapted to edapho-climatic conditions of the host site; hence, their reintroduction would ensure the greatest chance for remediation success. When used appropriately, local species can facilitate natural soil formation processes, and can bring about pollutant degradation or removal (Bacchetta et al., 2015; Borišev et al., 2018).
In Burkina Faso, as is the case across the entire sub-Saharan region, one important native species is Senegalia senegal (L.) Britton (syn. Acacia senegal (L.) Willd.) (Kyalangalilwa et al., 2013; Odee et al., 2015). This species, the gum acacia or gum Arabic tree, is a thorny, N2-fixing deciduous species in the Fabaceae that is native to arid and semi-desert regions of sub-Saharan Africa and the Middle East (Fagg and Allison, 2004). Senegalia Senegal can attain a height of 5–12 m, and grows on sandy, skeletal and slightly loamy soils, with a soil pH of 5–8. The gum acacia also grows in areas that receive 100–950 mm annual rainfall (Lyam et al., 2018). It can tolerate five to 11 months of drought and can survive temperatures high as 43°C, dry wind, and sandstorms.
The species, together with Vachellia seyal (Delile) P.J.H. Hurter (red acacia), is renowned for the copious sap that is produced when the bark is cut. The hardened exudates are collectively referred to as “gum Arabic”. The astringent, emulsifying, film-forming and encapsulating properties of gum arabic represents an important economic resource, which is used extensively in the food-processing, pharmaceutical, cosmetic, and lithographic ink industries (Raddad et al., 2005). In addition, S. senegal has considerable potential for fuelwood production and as forage for animals (Midgley and Bond, 2001) and, therefore, represents an important source of income for the local populace.
One of the factors that favours improved growth and survival rates of seedlings after transplantation is the role that is played by symbiotic soil microorganisms (Thavamani et al., 2017; Kumar et al., 2018; Singh and Gupta, 2018). Studies of symbiotic interactions between plant species and their associated microorganisms have provided evidence that nitrogen-fixing bacteria and arbuscular mycorrhizal fungi (AMF), which are adapted to local conditions, can play an important role in nutrient absorption and stimulate plant growth (Smith and Read, 2008; Ravellini et al., 2016; Kumar et al., 2018). They also exert many “non-nutritional” effects on plant physiology, frequently alleviating plant stress that is caused by biotic and abiotic factors (Kahindi et al., 1997; Cardoso and Kuyper, 2006), acting as bio-control agents (Cardoso and Kuyper, 2006), stabilizing soil aggregates (Rillig and Mummey, 2006), preventing erosion, and influencing plant fitness, and sustainability of the whole plant-soil system (Van der Heijden et al., 2006).
Restoration of mine wastelands has been the subject of much research worldwide over the past four decades. Yet, the pace of post-mining landscape restoration research and practice in Africa is sluggish compared to other parts of the world (Festin et al., 2019; Berrahmouni and Mansourian, 2021). Our goal was to evaluate the growth, survival and symbiotic responses of S. senegal seedlings to inoculation with native and exotic AM fungal and rhizobial strains using different levels of manure amendment in nursery and field conditions. We had high expectations that microbial inoculation and organic amendments would improve nutrient uptake and biomass of S. senegal that was grown under the aforementioned conditions. Findings of this study will be used to promote the successful reclamation of degraded land in the Essakane mining area.
Material and Methods
Site Description
The study was conducted at the Essakane gold mine (14°22′22.7″ N, 000°05′21″ E; 279 m asl), northeastern Burkina Faso. The mine is owned by IAMGOLD (90%) and the Government of Burkina Faso (10%). The study site straddles the boundary between Oudalan and Seno Provinces in the Sahel region of Burkina Faso and is about 330 km northeast of the capital city, Ouagadougou. The climate at this site is typical of semi-arid lands of West Africa that are characterized by a short rainy season from June to September and a dry season from October to May. Average annual rainfall fluctuates between 400 and 600 mm (Figure 1), with temperatures ranging from 23.7 to 37.7°C. Potential evapotranspration (PET) is relatively constant (1856.4 mm) from year to year and relative humidity ranges from 23.8 to 59.2% (Direction Générale de la Météorologie, Burkina Faso).
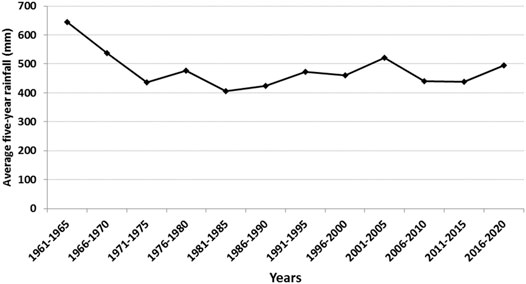
FIGURE 1. Rainfall evolution from 1961 to 2020 in Seno Province (historical data; from 1961 to 2010 were provided by Direction Générale de la Météorologie; those from 2011 to 2020 were from Essakane Pulsonic meteorological station, Burkina Faso).
Vegetation in the study area forms a patchy steppe, with large and small shrubs and trees. The dominant species in this environment are umbrella thorn (Vachellia tortilis [Forssk.] Galasso & Banfi), red acacia or shittah tree (Vachellia seyal), gum Arabic tree (Senegalia senegal (L.) Britton), and Egyptian balsam (Balanites aegyptiaca [L.] Delile) (Abdelaziz et al., 2020). The Essakane deposit has been an active artisanal mining site since 1985. IAMGOLD Gold industrial mining started in July 2010. Mining activities in this area have led to production of large quantities of wastes. This work focused upon waste rock dumps. The rock dump forms an artificial hill that has a slope of 18° (3/1), with intermediate benches, which will eventually rise to a height of 55 m and store 383 megatonnes of waste rock.
Seedling Preparation
Seeds of S. senegal were obtained from the Centre National des Semences Forestières (CNSF, Ouagadougou, Burkina Faso). Surface-scarified and surface-sterilized seeds were germinated in the dark at 28°C for 48 h in Petri dishes containing soft agar (1%). The duration of seed scarification in concentrated sulfuric acid (H2SO4) and rinsing with tap water was 10 and 15 min, respectively (Khasa, 1993).
Arbuscular Mycorrhizal Fungal and Rhizobial Inoculants and Inoculation
Two strains of arbuscular mycorrhizal fungi (AMF) and one strain of Mesorhizobium plurifarium (ORS 3588, Gene Bank number JQ039735) were used as inoculants. The AMF included the native isolate Rhizophagus aggregatus (Schenke and Smith emend. Koske, DAOM2277128, Dindéresso-Burkina Faso) and the commercial isolate Rhizophagus irregularis (DAOM197198), which was provided by Premier Tech Biotechnologies (Rivière-du-Loup, QC) under the commercial name Myke Pro WP). The Mesorhizobium strain was provided by the Common Laboratory of Microbiology (LCM) IRD/ISRA/UCAD in Dakar, Senegal. This strain was isolated from rhizospheric soils of S. senegal (Bakhoum et al., 2012). It was grown in YEM medium (Vincent, 1970) for 48 h at 28°C using a rotary shaker at 150 rpm.
The first endomycorrhizal inoculant (R. aggregatus) was also provided by the LCM. It was multiplied on Zea mays for 12 weeks under nursery conditions on sterilized substrate (soil and sand 1:1 v/v), and was comprised of spores, mycorrhizal root fragments, and soil. Each Gram of this inoculum contained an average of 40 spores and root fragments with 85% colonized root lengths. The second endomycorrhizal fungus (R. irregularis) was a commercial product of Myke Pro WP (Premier Tech Biotechnologies). Each Gram of inoculant contained 500 viable propagules.
Rhizobial inoculum was applied alone or in combination with AMF as a 10 ml suspension containing 108 cells ml−1. For AM fungal inoculation, both strains were first mixed in equal amounts (v/v) and 20 g of the inoculant were placed beneath the seedlings. Treatments without rhizobial or AM fungal inocula received 10 ml or 20 g of autoclaved inocula to avoid differences in soil nutrient content that was linked to rhizobial or AM fungal inoculum additions.
Greenhouse Experimental Design and Growth Conditions
The experiment was conducted in a nursery (daylight: 10 h; average daily temperature: 25°C day, 20°C night) belonging to the mining company IAMGOLD at Essakane. The factorial experiment consisted of three substrate types: sandy soil 100% (substrate A), sandy soil (75%) mixed with manure at 25% (substrate B); and sandy soil (50%) mixed with manure at 50% (substrate C). The three substrates were crossed with eight mycorrhizal or rhizobial inoculations levels: non-inoculated (CTRL-plants); inoculated with Mesorhizobium plurifarium ORS3588 (R-plants); inoculated with R. aggregatus (RA); inoculated with R. irregularis (RI-plants); inoculated with R. irregularis and R. aggregatus (RI + GA-plants); inoculated with Mesorhizobium ORS3588, R. irregularis and R. aggregatus (R + RI + GA-plants); inoculated with Mesorhizobium ORS3588 and R. aggregatus (R + GA-plants); and inoculated with Mesorhizobium ORS3588 and R. irregularis (R + RI-plants). Manure that was used for preparing substrates was collected from different villages in Essakane. Sandy soil samples were collected in an area where S. senegal tree plants are naturally growing with other plant species (V. tortilis, V. seyal, B. aegyptiaca). Soil samples were collected in mid-January at a depth of 0–20 cm. At each site, five core samples were taken every 10 m along three parallel transects using a soil auger, and homogeneously bulked to obtain a composite sample. After bulking, soil samples were air-dried, sieved to pass a 2-mm mesh sieve, and subsampled for physical and chemical analyses. In the field experiment, waste rock samples were sampled and compared to soils of the surrounding non-contaminated area for physico-chemical analyses. Physico-chemical characteristics of the different soils and those of substrates A, B, and C were analyzed at the Bureau National des Sols (BUNASOL), Burkina Faso, and are presented in Table 1.
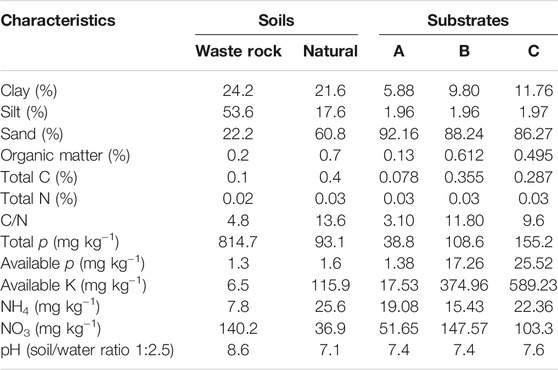
TABLE 1. Physical and chemical characteristics of the waste rock (WR) soils compared to those of an undisturbed (Natural) site located outside the mining perimeter and the different cultivation substrates.
In the nursery, germinated seedlings were aseptically and singly transplanted into black polythene bags (22 × 7 cm, with 400 ml capacity) that were filled with substrates (A, B, and C). The pots were arranged in a factorial randomized complete block design on the bench 1 m above the ground in a nursery. The experimental unit consisted of 3 plants giving rise to an experimental plot of 72 plants repeated eight (8) times. Duplicate trials were set up and supplied daily with tap water. After 75 days of culture, the first set of plants was sacrificed for analyses, while the second set was transplanted to the waste rock dumps.
Measurements and Harvest
Plants that were removed from the pots and roots were carefully washed free of soil particles under a stream of tap water to separate them from the substrate. Growth parameters (height, collar diameter, shoot, and root dry mass), root mycorrhizal colonization and rhizobial nodulation (number of nodules, nodule dry mass) were estimated. Root, shoot, and nodule dry masses were estimated after oven-drying the samples at 70°C for 72 h. Percentage mycorrhizal root colonization was estimated following microscope examination of arbuscular fungal structures after first clearing the fine roots in 10% KOH and staining them with 0.05% trypan blue in lactophenol (v/v), according to Phillips and Hayman (1970). Mycorrhizal colonization was calculated according to gridline intersect method (Giovannetti and Mosse, 1980). Mycorrhizal inoculation dependency (MID) was calculated using the following formula (Plenchette et al., 1983): MID (%) = 100 x [(dry mass of mycorrhizal plants—dry mass of non-mycorrhizal plants)/dry mass of mycorrhizal plants].
Transplanting in the Mining Site
We transplanted the seedlings onto the waste rock dumps using the same experimental design that was set up in the nursery. The plants were transplanted into holes (20 cm in diameter, 30 cm deep) with 2 m distance between holes in the direction of the slope, and 3 m along the slope perpendicular. Blocks were arranged perpendicular to the slope gradient to contain experimental units that were as homogenous as possible. To avoid destructive harvesting of the plants, the plant characteristics related to height were measured at 3 months, then at 12 and 72 months after transplantation. This monitoring also made it possible to evaluate the survival rate for each treatment.
Statistical Analysis
All data were subjected to analysis of variance (ANOVA) with XLSTAT version 2016 on Windows. A multi-factorial model of ANOVA that takes into account the factors block, substrate and inoculation, together with their interactions was used. A negative binomial regression model was applied to the nodule counts, and for post-hoc comparisons of averages, the Student-Newman-Keuls (SNK) test was implemented (p < 0.05).
Results
Soil Properties
Soil characteristics of the different substrates are presented in Table 1 and compared to those of waste rock samples and soil samples from the undisturbed natural zone. Mine tailings showed low levels of C, N and p compared to soil taken from the undisturbed natural environment. The waste rock contained higher silt content with alkaline pH. Yet, substrates A, B, and C and the soil samples from the natural environment exhibited a sandy texture and pH values were close to neutrality. As expected, manure-enriched substrates B and C had higher levels of organic matter and other nutrients compared to the 100% sandy substrate A (Table 1).
Plant Nodulation in the Nursery
Data on nodule dry masses are presented in Table 2. Nodule dry masses varied significantly (p < 0.01) not only among substrates, but also among inocula. Negative binomial regression revealed significant differences among the substrates A, B, and C. The highest nodule dry masses per plant were recorded on substrate A (Table 2). Nodule dry masses also varied according to the inoculum that was used (p < 0.01). Our results showed greater nodule dry masses with plants that were inoculated with either Mesorhizobium plurifarium (R) alone or in combination with R. aggregatus (R + GA). Lowest nodule dry masses were observed on plants that were inoculated with only R. irregularis (RI) and on the uninoculated control plants (CTRL). Yet, the interaction between the substrate and inoculation treatments did not significantly affect this variable. Neither the substrates nor the inocula significantly affected nodule dry masses, but their interaction showed significant variation (p < 0.05) for this variable (Table 2).
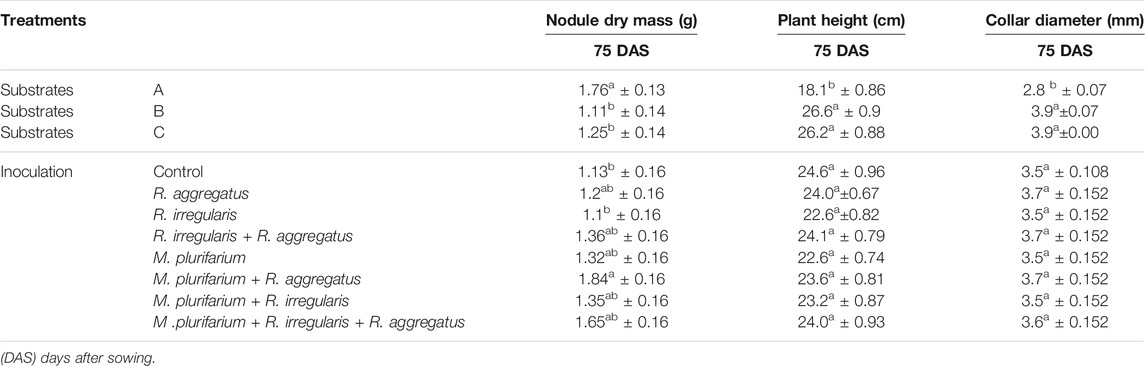
TABLE 2. Nodulation (nodule dry mass) and Growth (height) of S. senegal seedlings that were grown on different inoculated or uninoculated substrates in nursery conditions (75 DAS). For each factor, means of variables followed by the same letter in the same column do not significantly differ according to the Bonferroni step-down test at p < 0.05. Means ± standard errors are shown (n = 8).
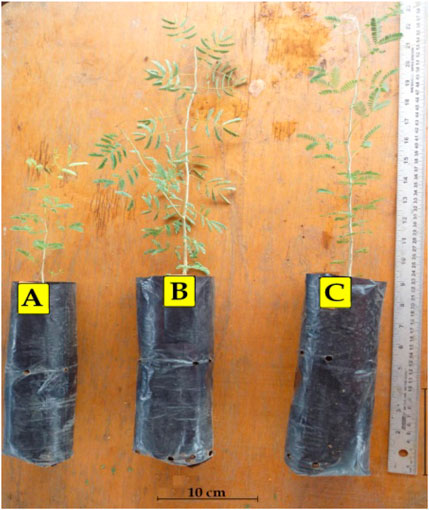
FIGURE. PHOTO 1: View of S. senegal plants 75 days after sowing in nursery conditions. Note the difference in height between substrates A, B and C.
Mycorrhizal Colonization in the Nursery
Mycorrhizal root colonization rates are presented in Figure 2. AM fungi colonized seedlings in all treatments at 75 days after sowing, but at various rates. The intensity of mycorrhizal colonization was significantly different (p < 0.001) among the substrates, the inoculation treatments and their interactions (Table 3). The colonization rate that was expressed here as the intensity of mycorrhizal colonization was greater on substrate A than on substrates B and C, regardless of inoculum. For the same substrate type, plants that were inoculated with R. aggregatus strain alone or in combination with other microbial strains showed the greatest mycorrhizal colonization compared to other treatments (Figure 2). There was no difference among inoculated treatments for substrate B. For substrate C, triple inoculation (Mesorhizobium + R. irregularis + R. aggregatus) exhibited the best mycorrhizal colonization compared to the others (Figure 2).
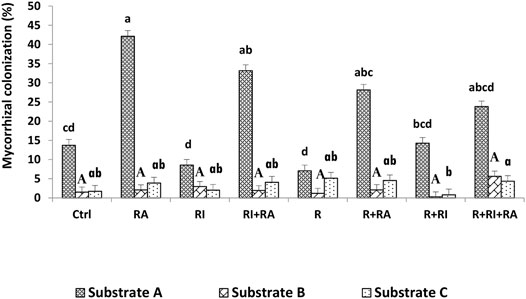
FIGURE 2. Root mycorrhizal colonization of S. senegal seedlings that were grown on different inoculated or uninoculated substrates in nursery conditions. For each substrate, bars with the same letters do not significantly differ according to the Bonferroni step-down test at p < 0.05. (Ctrl) control; (R), Mesorhizobium plurifarium; (RI), Rhizophagus irregularis; (RA), Rhizophagus aggregatus.
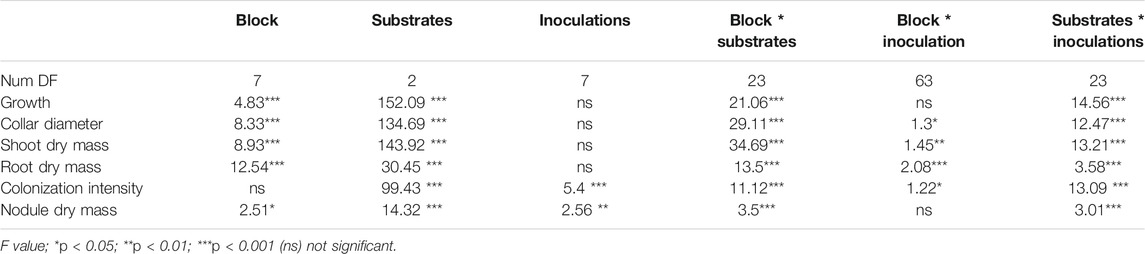
TABLE 3. Significance level obtained from two-way ANOVA testing the effects of inoculation and substrates on different parameters measured in the nursery and mine field conditions.
Our results showed that regardless of the substrate used, the mycorrhizal inoculation dependency (MID) of the plant species was higher with R. aggregatus for substrate A (Table 4) and with R. aggregatus + R. irregularis for substrate B. When taking into account the culture substrates, the plants exhibited greatest mycorrhizal dependency on substrate A, and followed by the substrate B. Substrate C had the lowest MID, with negative values for all mycorrhizal treatments (Table 4). No AM fungal structure was found in the root systems of plant controls or those inoculated with M. plurifarium alone.
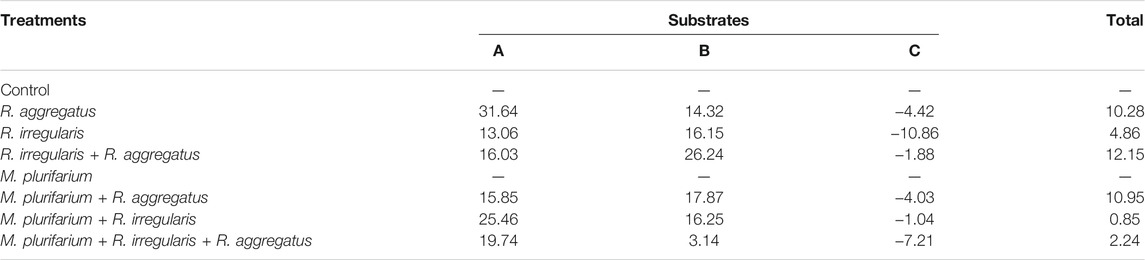
TABLE 4. Mycorrhizal dependency of S. senegal seedlings as a function of substrate types (A, B, and C).
Plant Growth in the Nursery
The block factor, together with the block by substrate interaction, influenced almost all growth parameters (shoot and root biomasses, plant height, and collar diameter). Data on the effects of substrate types and inoculation treatments on plant growth are reported in Tables 2 and 3; Figures 3, 4. Blocks and the type of substrate influenced plant growth, with plant height and collar diameter varying significantly (p < 0.001) among substrates (Table 3, Photograph 1). Substrate B and substrate C recorded the greatest plant heights at 26.6 and 26.2 cm, respectively. Plant heights for these treatments differed from those of substrate A, which averaged 18.1 cm (Table 2). Collar diameters followed the same trend (4.0, 3.9, and 2.8 mm for the substrates B, C, and A, respectively). Inoculation treatments and the substrate by inoculation interaction did not reveal significant differences in either plant height or collar diameter (Table 2).
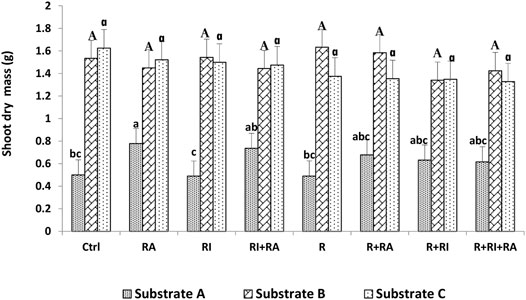
FIGURE 3. Shoot dry mass of S. senegal seedlings that were grown on different inoculated or uninoculated substrates in nursery conditions. For each substrate, bars with the same letters do not significantly differ according to the Bonferroni step-down test at p < 0.05. (Ctrl) control; (R) Mesorhizobium plurifarium; (RI), Rhizophagus irregularis; (RA), Rhizophagus aggregatus.
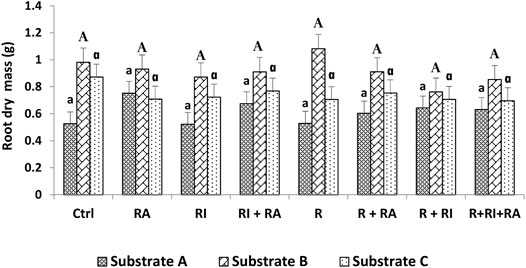
FIGURE 4. Root dry mass of S. senegal seedlings that were grown on different inoculated or uninoculated substrates in nursery conditions. For each substrate, bars with the same letters do not significantly differ according to the Bonferroni step-down test at p < 0.05. (Ctrl) control; (R), Mesorhizobium plurifarium; (RI), Rhizophagus irregularis; (RA), Rhizophagus aggregatus.
Similarly, for the plant height, both shoot, and root biomasses that were recorded on substrate B and substrate C were significantly higher than those of substrate A (Figures 3, 4). For inoculation treatments, a significant difference was only noted for inoculated plants on substrate A. For this substrate, plants that were inoculated with R. aggregatus exhibited the greatest shoot biomass. Control plants (CTRL), and those that were inoculated with R. irregularis and Mesorhizobium plurifarium alone showed the lowest shoot biomass (Figure 3).
Regardless of the substrate types that were used, there was no difference in the inoculation factor. The interaction between substrates and inoculation treatments had a significant (p < 0.05) effect on both root and shoot biomass production (Table 3).
Plant Growth and Survival Rate in the Field
Results for plant growth and survival rates are presented in Tables 3, 5 and 6. The variation in most parameters among blocks underscores the inherent heterogeneity of the study site and would be justified by the choice of experimental set-up that minimizes the effect of uncontrolled factors on the plant responses during the study (Table 3). Three months after transplantation onto the mining site, mean plant growth on the waste rock varied significantly among the substrates (p < 0.001). We observed a greater increase for the seedlings that were grown on substrate B in the nursery. Yet, there was no significant difference for the comparisons between inocula that were applied to the different substrates (Table 5).
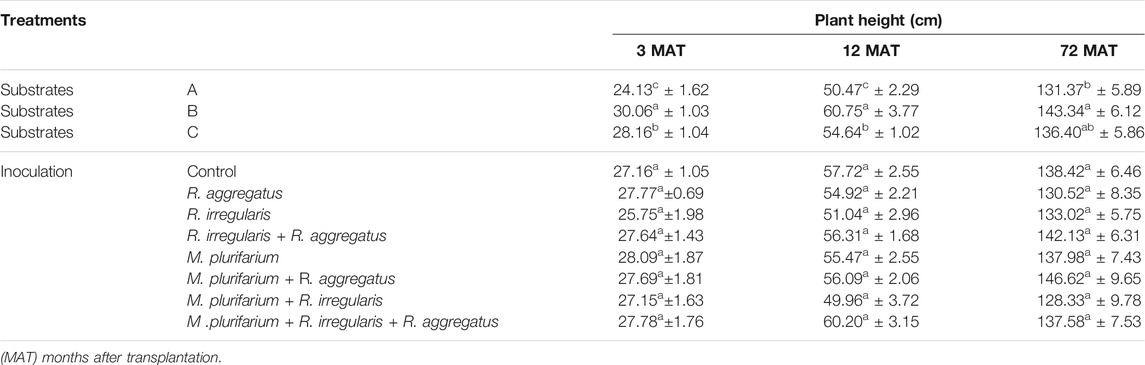
TABLE 5. Growth (height) of S. senegal seedlings that were grown on different inoculated or uninoculated substrates in field mine from 3 to 72 MAT. For each factor, means of values followed by the same letter in the same column do not significantly differ according to the Bonferroni step-down test at p < 0.05. Means ± standard errors are shown (n = 8).
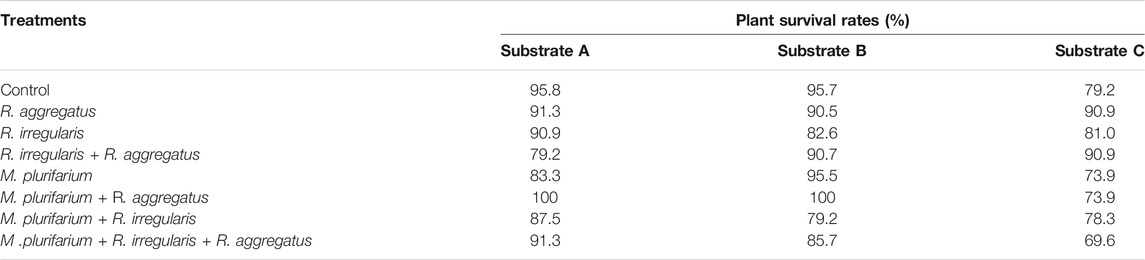
TABLE 6. Survival rates (%) at 72 months after transplantation of S. senegal seedlings that were grown on different inoculated or uninoculated substrates in field mine.
Plant growth was remarkably high on substrate B, particularly during the first 72 months. Substrate B showed a significant difference compared to substrate A. Inoculation did not affect plant height, although each treatment varied in terms of plant growth. Nevertheless, the trend was that dual inoculations of R. irregularis + R. aggregatus and Mesorhizobium + R. aggregatus, together with the triple inoculation of Mesorhizobium + R. irregularis + R. aggregatus, showed the best growth, and even though the differences that were obtained are not significant (Table 5).
Neither substrates nor inocula exerted an effect on seedling survival rate 72 months after transplantation (MAT). We recorded an overall survival of 95.14% at 3 months after transplantation. Overall survival rates of seedlings were 94.1% at 12 MAT and 89.06% at 72 MAT (Table 6). The seedlings that were grown on the substrate A survived at rates (mean ± standard deviations) of 97.39 ± 2.9, 96.83 ± 2.75, and 89.58 ± 5.89% at 3, 12, and 72 MAT, respectively. For seedlings that were grown on substrate B, plant survival was 93.23 ± 4.63, 93.75 ± 4.65, and 85.42 ± 5.89% at 3, 12, and 72 MAT, respectively. Plants of substrate C showed rates of 94.79 ± 2.75, 94.20 ± 2.95, and 79.58 ± 9.53% at 3, 12, and 72 MAT, respectively. Therefore, the survival rates tended to decrease from substrate A, followed by substrate B, although differences were not significant.
Discussion
The “Arabic gum” species that was used in this study is a multipurpose tree commonly grown in agroforestry systems under arid and semi-arid climatic conditions of West Africa (Atangana et al., 2014). It usually tolerates drought and can survive under high temperature, making it an ideal candidate for testing the potential of microbial, and manure amendments for the rehabilitation of mining sites in this area.
Plant Responses to Microbial Inoculation
A potentially major limitation to vegetation establishment on mine soils is likely the absence of the microflora (Rapai et al., 2016; Thavamani et al., 2017; Wang 2017; Novo et al., 2018) that are intimately involved in plant nutrition (Smith and Read, 2008; Ravellini et al., 2016; Kumar et al., 2018) and nutrient cycling processes in natural soils (Kahindi et al., 1997). Among these soil-dwelling microorganisms, AM fungi and nitrogen-fixing bacteria play a crucial role in nutrient acquisition by plants in most disturbed ecosystems (Kahindi et al., 1997; Smith and Read, 1997; Smith and Read, 2008). Examination of waste rock samples from the Essakane mining site showed that they are physically, chemically, and biologically poor materials (Table 1) that could hinder vegetation development. To ensure rapid and effective establishment of S. senegal seedlings and nutrient cycling processes, we tested the introduction of selected soil microorganisms to overcome plant nutritional limitations in waste rock dumps and further form soils that would support and sustain native vegetation. The results from nursery experiments showed that inoculation did not consistently increase plant height or shoot and root dry mass between treatments of the manure-enriched substrates. The overall trend was that plants inoculated with the indigenous AM fungus R. aggregatus alone or concurrently with R. irregularis or Mesorhizobium plurifarium ORS3588 demonstrated the best increase for plant height or for shoot and root dry mass. Nevertheless, the nodulation and AM fungal colonization rates of plants inoculated with R. aggregatus for the un-amended substrate were significantly increased, together with plant growth, and biomass production. These responses agree with past studies that have shown that AM fungal applications generate positive plant responses in mine restoration (Rapai et al., 2016; Wulandari and Tawaraya, 2016; Ohsowski et al., 2018) and on phosphorus-poor substrates (Gemma et al., 2002; Ndoye et al., 2015; Guissou et al., 2016). The increase that was observed with R. aggregatus was more significant when M. plurifarium ORS3588 was inoculated concurrently. This result suggests a potential synergistic effect between these AM fungal and rhizobial strains and confirmed results of several reports on increased plant growth attributable to dual or triple microbial inoculation (Sene et al., 2010; Bakhoum et al., 2012; Ndoye et al., 2015).
In contrast, seedlings that were inoculated with the commercial AM fungus R. irregularis alone did not show any increase in root plant colonization rate and growth with neither for the manure-enriched nor un-amended substrates. These results suggest that R. aggregatus isolated from the rhizosphere of Australasian black wattle or mangium (Acacia mangium Willd.) growing at Dinderesso, Burkina Faso, is more efficient than the commercial introduced AM fungus in this harsh landscape. Similar negative or neutral effects after seedling inoculation with the commercial fungus R. irregularis had been observed by Sarasin et al. (2017) for the native Mimosa latispinosa Lam. in Madagascar, and likewise by Ohsowski et al. (2018) in a sandpit restoration to grassland in Southern Ontario, Canada. In Senegal, previous tracing of inoculants with three cultivars of peanut (Arachis hypogaea L.) showed that the exotic commercial strain did not form symbioses with plants (Sene et al., 2010). These data and our results from the Essakane mining site raise questions about the efficacy of commercial microbial isolates in harsh tropical ecosystems. Indeed, the AM fungal strain R. irregularis was isolated from a boreal environment in the Province of Quebec, Canada (Stockinger et al., 2009). Despite its wide ecological valence, this AM fungal strain is less efficient compared to the indigenous strain R. aggregatus in the present study. A gap in the ecological adaptation of this AM fungal strain should be considered, as has been suggested in the study by Sarasin et al. (2017).
Biological nitrogen-fixation by Acacia species has been previously reported to reach optimal rates on extremely contrasting soils (Vincent et al., 2018). Bakhoum et al. (2016) reported beneficial effects of M. plurifarium ORS3588 inoculation on S. senegal growth that could be attributed to the rhizobial inoculant that was used and its highest performance in comparison to indigenous microorganisms. From this study, rhizobial inoculation improved root nodulation, yet we found no evidence that inoculation by M. plurifarium ORS3588 increased plant growth and biomass. At first sight, the physico-chemical analyses of the unamended substrate showed limited nutrient contents (Table 1). Thus, the lack of an inoculation effect on plant growth and biomass production may be due to the fact that efficient N2-fixation requires additional substances, which must be available in the soil (Lekberg and Koide, 2005), such as phosphorus and specific trace metals (Bellenger et al., 2011; González-Guerrero et al., 2014). On the amended substrates, macro-nutrient elements were available, but we did not measure micro-nutrients, particularly available Fe, Mo, and V concentrations. Moreover, we had failed to detect a positive effect of rhizobial inoculation. Thus, the application of a solitary M. plurifarium ORS3588 isolate may not benefit S. senegal plants when restoring disturbed areas such as mining sites. Therefore, we suggest dual inoculation using compatible rhizobial and mycorrhizal fungal strains for enhancing restoration of gold waste dumps using legume species.
Plant Response to Manure-enriched Soils
Manure-enriched soils are traditionally used and recommended to favour nursery plant growth. Since analyses of the waste rock samples showed low nutrient contents, we tested manure amendments at the rates of 25 and 50% to increase soil nutrient levels. Our data showed that seedlings grown on manure-enriched soils harboured fewer nodules and were less colonized by AM fungi than seedlings that were grown in soil without the amendment. This result suggests that levels of manure used do not ensure the efficient establishment of plant-microbe symbioses. Inhibitory effects of compost and biochar amendments on plant symbioses have been reported by Rapai et al. (2016) and may affect competitive relationships between plants and AM fungi. In the case of our study, this might have occurred because the seedlings that were not grown on manure-enriched substrate had more to gain than those grown on manure-enriched substrates. Seedlings on the un-enriched substrate were prone to establishing symbioses with microbial inoculants in this nutrient-impoverished state. Another probable explanation is the fact that manure-enriched substrates may contain bacteria that could negatively influence seedling symbioses. Notwithstanding these negative effects on seedling symbioses, manure-enriched substrates that were applied at the rate of 25% increased plant growth (height and collar diameter) and biomass production under both nursery and field conditions at the mine. An inhibitory effect on plant performance was observed for manure amendments at the rate of 50%. There is a strong likelihood that the latter imposes stress upon plant relationships with the soil biota, thereby reducing their performance.
The survival rate of seedlings in the field is critical to phytoremediation success (Grant et al., 2002; Gastauer et al., 2018; Kumar et al., 2018). From this work, plants exhibiting the best growth in the nursery (given that nutrients were present in the manure treatments) did not survive better on the ground because of insufficient mycorrhizal infection to take-up the few nutrients that were available in the mining soil. The highest plant survival rates coincided with the most AM-dependent ones. High nutrient availability from manure amendments was beneficial to nutrient acquisition for seedlings, but may gain less for the establishment of their symbioses, which could have dramatic long-term effects on planting survival in stressful field conditions. This result, together with data from Wulandari and Tawaraya, (2016) and Rapai et al. (2016), suggests that healthy pre-inoculated seedlings under nursery conditions may lead to great post-planting success on mining sites. In fact, plants in mine sites are dependent, to varying degrees, on associations between their roots, and soil microorganisms for efficient nutrient acquisition (Wulandari and Tawaraya, 2016; Ohsowski et al., 2018). Therefore, a better interaction among manure and microbial amendments that could facilitates a high degree of root colonization might be more beneficial for plant establishment. Based upon the results of this study, the rate of 25% of manure could provide sufficient organic matter, increasing substrate aggregation, and plant root colonization. Therefore, we suggest production of S. senegal pre-inoculated seedlings with Rhizophagus aggregatus DAOM2277128 and Mesorhizobium plurifarium strain ORS3588 in 25% of manure-enriched sandy soil in nursery.
Conclusion and Further Directions
This study demonstrated the adaptive capacity of the legume tree S. senegal on the Essakane mining site after being exposed to inoculants and manure amendments. This is an important step in the process of disturbed site rehabilitation. On relatively nutrient-poor substrates, AM fungal and rhizobial colonization was greater, but plants were less developed in the nursery. In the field, however, the measurements indicated that the plants, which were small in the nursery, but better colonized by the fungi, and rhizobia, survived well compared to those of the manure-enriched treatments which were vigorous in the nursery but with fewer mycorrhiza and rhizobia. The results support the general conclusion that manure-enriched substrates and microbial inoculation could be an option for mining site rehabilitation. Yet, manure amendments should be applied with caution, given that high rates may induce further stress in plant symbioses and, therefore, their performance in waste rock dumps. Nevertheless, when these soil additives are used optimally, plants may gain more from manure, and microbial co-amendments. To maximize rehabilitation efforts on mining sites, pioneer herbaceous plants should be further taken into account since they are highly mycotrophic and decompose quickly, thereby augmenting the soil organic matter pool. In this sense, the agroforestry concept combining herbaceous and wood plants, and soil co-amendments with bio-inputs is the way forward that can enhance and accelerate mine restoration for full recovery of ecosystem services.
Data Availability Statement
The raw data supporting the conclusion of this article will be made available by the authors, without undue reservation.
Author Contributions
All authors participated to the Conceptualization, Methodology, Data analysis, Writing—original draft, review, and editing manuscript.
Funding
The work received funding from IAMGOLD Essakane SA, MITACS and support from NSERC (Natural Sciences and Engineering Research Council of Canada) Discovery Grant.
Conflict of Interest
The authors declare that the research was conducted in the absence of any commercial or financial relationships that could be construed as a potential conflict of interest.
Publisher’s Note
All claims expressed in this article are solely those of the authors and do not necessarily represent those of their affiliated organizations, or those of the publisher, the editors and the reviewers. Any product that may be evaluated in this article, or claim that may be made by its manufacturer, is not guaranteed or endorsed by the publisher.
Acknowledgments
This paper is published in memory of our esteemed colleague, KS. We are thankful to our collaborators at INERA/DEF (Burkina Faso) and the Common Laboratory of Microbiology (LCM) IRD/ISRA/UCAD (Senegal), together with the Centre for Forest Research (CEF) at Laval University, Quebec, Canada. Discovery Grant to DPK is also acknowledged. W.F.J. Parsons corrected the English.
References
Abdelaziz, S. M., Medraoui, L., Alami, M., Pakhrou, O., Makkaoui, M., Boukhary, A.O.M.S., et al. (2020). Inter simple sequence repeat markers to assess genetic diversity of the desert date (Balanites aegyptiaca Del.) for Sahelian ecosystem restoration. Sci. Rep. 10, 1–8. doi:10.1038/s41598-020-71835-9
Atangana, A. R., Khasa, D. P., Chang, S. X., and Degrande, A. (2014). Tropical Agroforestry. Springer Science+Business Media, Dordrecht. ISBN 978-94-007-7722-4. doi:10.1007/978-94-007-7723-1
Bacchetta, G., Cappai, G., Carucci, A., and Tamburini, E. (2015). Use of Native Plants for the Remediation of Abandoned Mine Sites in Mediterranean Semiarid Environments. Bull. Environ. Contam. Toxicol. 94 (3), 326–333. doi:10.1007/s00128-015-1467-y
Bakhoum, N., Ndoye, F., Kane, A., Assigbetse, K., Fall, D., Sylla, S. N., et al. (2012). Impact of Rhizobial Inoculation on Acacia senegal (L.) Willd. Growth in Greenhouse and Soil Functioning in Relation to Seed Provenance and Soil Origin. World J. Microbiol. Biotechnol. 28, 2567–2579. doi:10.1007/s11274-012-1066-6
Bakhoum, N., Odee, D. W., Fall, D., Ndoye, F., Kane, A., Kimiti, J. M., et al. (2016). Senegalia senegal Response to Inoculation with Rhizobial Strains Vary in Relation to Seed Provenance and Soil Type. Plant Soil. 398 (1–2), 181–193. doi:10.1007/s11104-015-2655-6
Bellenger, J.-P., Wichard, T., Xu, Y., Kraepiel, A. M. L., and Kraepiel, A. M. L. (2011). Essential Metals for Nitrogen Fixation in a Free-Living N2-Fixing Bacterium: Chelation, Homeostasis and High Use Efficiency. Environ. Microbiol. 13, 1395–1411. doi:10.1111/j.1462-2920.2011.02440.x
Berrahmouni, N., and Mansourian, S. (2021). Review of forest and Landscape Restoration in Africa. Accra: FAO and AUDA-NEPAD. doi:10.4060/cb6111en
Borišev, M., Pajević, S., Nikolić, N., Pilipović, A., Arsenov, D., and Župunski, M. (2018). “Mine Site Restoration Using Silvicultural Approach,” in Bio-Geotechnologies for Mine Site Rehabilitation. Editors M. N. V. Prasad, P. J. C. Favas, and S. K. Maiti. 1st ed (Elsevier), 115–130. doi:10.1016/B978-0-12-812986-9.00007-5
Buta, M., Blaga, G., Paulette, L., Păcurar, I., Roca, S., and Borsai, O. (2019). Soil Reclamation of Abandoned Mine Lands by Revegetation in Northwestern Part of Transylvania: A 40-Year Retrospective Study. Sustainability 11, 3393.
Cardoso, I., and Kuyper, T. (2006). Mycorrhizas and Tropical Soil Fertility. Agric. Ecosyst. Environ. 116, 72–84. doi:10.1016/j.agee.2006.03.011
Fagg, C., and Allison, G. E. (2004). Acacia senegal and the Gum Arabic Trade. Monograph and Annotated Bibliography. Trop. For. 42, 261.
Festin, E. S., Tigabu, M., Chileshe, M. N., Syampungani, S., and Odén, P. C. (2019). Progresses in Restoration of post-mining Landscape in Africa. J. For. Res. 30 (2), 381–396. doi:10.1007/w11676-018-0621-x10.1007/s11676-018-0621-x
Gastauer, M., Silva, J. R., Caldeira Junior, C. F., Ramos, S. J., Souza Filho, P. W. M., Furtini Neto, A. E., et al. (2018). Mine Land Rehabilitation: Modern Ecological Approaches for More Sustainable Mining. J. Clean. Prod. Prod. 172, 1409–1422. doi:10.1016/j.jclepro.2017.10.223
Gemma, J. N., Koske, R. E., and Habte, M. (2002). Mycorrhizal Dependency of Some Endemic and Endangered Hawaiian Plant Species. Am. J. Bot. 89, 337–345. doi:10.3732/ajb.89.2.337
Giovannetti, M., and Mosse, B. (1980). An Evaluation of Techniques for Measuring Vesicular Arbuscular Mycorrhizal Infection in Roots. New Phytol. 84, 489–500. doi:10.1111/j.1469-8137.1980.tb04556.x
González-Guerrero, M., Matthiadis, A., Sáez, Á. n., and Long, T. A. (2014). Fixating on Metals: New Insights into the Role of Metals in Nodulation and Symbiotic Nitrogen Fixation. Front. Plant Sci. 5, 45. doi:10.3389/fpls.2014.00045
Grant, C. D., Campbell, C. J., and Charnock, N. R. (2002). Selection of Species Suitable for Derelict Mine Site Rehabilitation in New South Wales, Australia. Water Air Soil Pollut. 139, 215–235. doi:10.1023/A:1015860025136
Guissou, T., Babana, A. H., Sanon, K. B., and Bâ, A. M. (2016). Effects of Arbuscular Mycorrhizae on Growth and mineral Nutrition of Greenhouse Propagated Fruit Trees from Diverse Geographic Provenances. Biotechnol. Agron. Soc. Environ. 20 (3), 417–426. doi:10.25518/1780-4507.13149
Kahindi, J. H. P., Woomer, P., George, T., de Souza Moreira, F. M., Karanja, N. K., and Giller, K. E. (1997). Agricultural Intensification, Soil Biodiversity and Ecosystem Function in the Tropics: the Role of Nitrogen-Fixing Bacteria. Appl. Soil Ecol. 6, 55–76. doi:10.1016/S0929-1393(96)00151-5
Khasa, P. D. (1993). Acid Scarification and Hot Water Soaking of Racosperma Auriculiforme Seeds. For. Chronicle. 69, 331–334. doi:10.5558/tfc69331-3
Kumar, A., Tripti, M. N. V., Prasad, M. N. V., Maiti, S. K., and Favas, P. J. C. (2018). “Mycoremediation for Mine Site Rehabilitation,” in Bio-Geotechnologies for Mine Site Rehabilitation. Editors M. N. V. Prasad, P. J. C. Favas, and S. K. Maiti. 1st ed (Elsevier), 233–260. doi:10.1016/B978-0-12-812986-9.00014-2
Kyalangalilwa, B., Boatwright, J. S., Daru, B. H., Maurin, O., and van der Bank, M. (2013). Phylogenetic Position and Revised Classification ofAcacia s.l.(Fabaceae: Mimosoideae) in Africa, Including New Combinations inVachelliaandSenegalia. Bot. J. Linn. Soc. 172 (4), 500–523. doi:10.1111/boj.12047
Lekberg, Y., and Koide, R. T. (2005). Arbuscular Mycorrhizal Fungi, Rhizobia, Available Soil P and Nodulation of Groundnut (Arachis hypogaea) in Zimbabwe. Agric. Ecosyst. Environ. 110, 143–148. doi:10.1016/j.agee.2005.03.011
Li, Z., Ma, Z., van der Kuijp, T. J., Yuan, Z., and Huang, L. (2014). A Review of Soil Heavy Metal Pollution from Mines in China: Pollution and Health Risk Assessment. Sci. Total Environ. 468-469, 843–853. doi:10.1016/j.scitotenv.2013.08.090
Lyam, P. T., Duque-Lazo, J., Durka, W., Hauenschild, F., Schnitzler, J., Michalak, I., et al. (2018). Genetic Diversity and Distribution of Senegalia senegal (L.) Britton under Climate Change Scenarios in West Africa. PLoS ONE 13, e0194726. doi:10.1371/journal.pone.0194726
Midgley, J. J., and Bond, W. J. (2001). A Synthesis of the Demography of African Acacias. J. Trop. Ecol. 17, 871–886. doi:10.1017/S026646740100164x
Ndoye, F., Kane, A., Bakhoum, N., Sanon, A., Fall, D., Diouf, D., et al. (2013). Response of Acacia senegal (L.) Willd. To Inoculation with Arbuscular Mycorrhizal Fungi Isolates in Sterilized and Unsterilized Soils in Senegal. Agroforest Syst. 87, 941–952. doi:10.1007/s10457-013-9610-4
Ndoye, F., Fall, D., Sadio, O., Sy, M. O., Noba, K., Diouf, D., et al. (2015). Effects of Dual Inoculation with Arbuscular Mycorrhizal Fungi and Rhizobia on Acacia senegal (L.) Willd. Seedling Growth and Soil Enzyme Activities in Senegal. Int. J. Biosci. 6 (2), 36–48. doi:10.12692/ijb/6.2.36-48
Novo, L. A. B., Castro, P. M. L., Alvarenga, P., and da Silva, E. F. (2018). “Plant Growth-Promoting Rhizobacteria-Assisted Phytoremediation of Mine Soils,” in Bio-Geotechnologies for Mine Site Rehabilitation. Editors M. N. V. Prasad, P. J. C. Favas, and S. K. Maiti. 1st ed (Elsevier), 281–295. doi:10.1016/B978-0-12-812986-9.00016-6
Odee, D. W., Wilson, J., Omondi, S., Perry, A., and Cavers, S. (2015). Rangewide Ploidy Variation and Evolution in Acacia senegal: a north-south divide? AoB Plants 7, plv011. doi:10.1093/aobpla/plv011
Ohsowski, B. M., Dunfield, K., Klironomos, J. N., and Hart, M. M. (2018). Plant Response to Biochar, Compost, and Mycorrhizal Fungal Amendments in post-mine Sandpits. Restor. Ecol. 26 (1), 63–72. doi:10.1111/rec.12528
Phillips, J. M., and Hayman, D. S. (1970). Improved Procedures for Clearing Roots and Staining Parasitic and Vesicular-Arbuscular Mycorrhizal Fungi for Rapid Assessment of Infection. Trans. Br. Mycol. Soc. 55, 158–IN18. doi:10.1016/S0007-1536(70)80110-3
Plenchette, C., Fortin, J. A., and Furlan, V. (1983). Growth Responses of Several Plant Species to Mycorrhizae in a Soil of Moderate P-Fertility. Plant Soil 70, 199–209. doi:10.1007/BF02374780
Raddad, E. A. Y., Salih, A. A., Fadl, M. A. E., Kaarakka, V., and Luukkanen, O. (2005). Symbiotic Nitrogen Fixation in Eight Acacia senegal Provenances in Dryland Clays of the Blue Nile Sudan Estimated by the 15N Natural Abundance Method. Plant Soil 275 (1), 261–269. doi:10.1007/s11104-005-2152-4
Rapai, S. B., Hunt, S., Bainard, L. D., Turgeon, M.-H., and Newmaster, S. G. (2016). Soil Inoculation with Arbuscular Mycorrhizal Fungi Promotes the Growth of Boreal Plant Communities in Gold Mine Overburden. Ecol. Rest. 34 (3), 216–224. doi:10.3368/er.34.3.216
Revillini, D., Gehring, C. A., and Johnson, N. C. (2016). The Role of Locally Adapted Mycorrhizas and Rhizobacteria in Plant-Soil Feedback Systems. Funct. Ecol. 30, 1086–1098. doi:10.1111/1365-2435.12668
Rillig, M. C., and Mummey, D. L. (2006). Mycorrhizas and Soil Structure. New Phytol. 171, 41–53. doi:10.1111/j.1469-8137.2006.01750.x
Rosenvald, K., Kuznetsova, T., Ostonen, I., Truu, M., Truu, J., Uri, V., et al. (2011). Rhizosphere Effect and fine-root Morphological Adaptations in a Chronosequence of Silver Birch Stands on Reclaimed Oil Shale post-mining Areas. Ecol. Eng. 37, 1027–1034. doi:10.1016/j.ecoleng.2010.05.011
Sarasin, G., Behavana, I. M., Rakotoarimanga, N., Randriatafika, F., Ramanankierana, H., Rabenantoandro, J., et al. (2017). Inoculation of Mimosa Latispinosa Lam with the Commercial Arbuscular Mycorrhizal Fungus Rhizophagus Irregularis DAOM197198, and Bradyrhizobium Spp. Under nursery Production Conditions in South-East Madagascar. Tropicultura 1, 3–11. doi:10.25518/2295-8010.1119
Sene, G., Thiao, M., Samba-Mbaye, R., Ndoye, F., Kane, A., Diouf, D., et al. (2010). Response of Three Peanut Cultivars toward Inoculation with Two Bradyrhizobium Strains and an Arbuscular Mycorrhizal Fungus in Senegal. Afr. J. Microbiol. Res. 4 (23), 2520–2527. doi:10.5897/AJMR.9000631
Singh, J. S., and Gupta, V. K. (2018). Soil Microbial Biomass: A Key Soil Driver in Management of Ecosystem Functioning. Sci. Total Environ. 634, 497–500. doi:10.1016/j.scitotenv.2018.03.373
Stockinger, H., Walker, C., and Schüßler, A. (2009). ' Glomus Intraradices DAOM197198', a Model Fungus in Arbuscular Mycorrhiza Research, Is Not Glomus Intraradices. New Phytol. 183, 1176–1187. doi:10.1111/j.1469-8137.2009.02874.x
Straker, C. J., Weiersbye, I. M., and Witkowski, E. T. F. (2007). Arbuscular Mycorrhiza Status of Gold and Uranium Tailings and Surrounding Soils of South Africa's Deep Level Gold Mines: I. Root Colonization and Spore Levels. South Afr. J. Bot. 73, 218–225. doi:10.1016/j.sajb.2006.12.006
Thavamani, P., Samkumar, R. A., Satheesh, V., Subashchandrabose, S. R., Ramadass, K., Naidu, R., et al. (2017). Microbes from Mined Sites: Harnessing Their Potential for Reclamation of Derelict Mine Sites. Environ. Pollut. 230, 495–505. doi:10.1016/j.envpol.2017.06.056
Van der Heijden, M. G. A., Bakker, R., Verwaal, J., Scheublin, T. R., Rutten, M., Van Logtestijn, R., et al. (2006). Symbiotic Bacteria as a Determinant of Plant Community Structure and Plant Productivity in Dune Grassland. FEMS Microbiol. Ecol. 56, 178–187. doi:10.1111/j.1574-6941.2006.00086.x
Vincent, B., Jourand, P., Juillot, F., Ducousso, M., and Galiana, A. (2018). Biological In Situ Nitrogen Fixation by an Acacia Species Reaches Optimal Rates on Extremely Contrasted Soils. Eur. J. Soil Biol. 86, 52–62. doi:10.1016/j.ejsobi.2018.03.003
Vincent, J. M. (1970). International Biological Program. Oxford: Blackwell Scientific Publications, 164.A Manual for the Practical Study of Root-Nodule Bacteria. IBP Handbook 15.
Wang, F. (2017). Occurrence of Arbuscular Mycorrhizal Fungi in Mining-Impacted Sites and Their Contribution to Ecological Restoration: Mechanisms and Applications. Crit. Rev. Environ. Sci. Tech. 47 (20), 1901–1957. doi:10.1080/10643389.2017.1400853
Keywords: disturbed soils, waste rock mining sites, arbuscular mycorrhizal fungi, legume nodulating rhizobia, manure amendments, Senegalia senegal (L.) Britton, restoration, Sahel
Citation: Yonli HH, Sene G, Sanon KB, Dianda M and Khasa DP (2022) Senegalia senegal (L.) Britton Response to Microbial and Manure Amendments for the Rehabilitation of Waste Rock Dumps in the Essakane Gold Mining Site, Burkina Faso. Front. Environ. Sci. 10:803009. doi: 10.3389/fenvs.2022.803009
Received: 27 October 2021; Accepted: 04 February 2022;
Published: 25 February 2022.
Edited by:
Ali Mahbub Quoreshi, Kuwait Institute for Scientific Research, KuwaitReviewed by:
Hayssam M. Ali, King Saud University, Saudi ArabiaRichard Cheruiyot, Kenyatta University, Kenya
Copyright © 2022 Yonli, Sene, Sanon, Dianda and Khasa. This is an open-access article distributed under the terms of the Creative Commons Attribution License (CC BY). The use, distribution or reproduction in other forums is permitted, provided the original author(s) and the copyright owner(s) are credited and that the original publication in this journal is cited, in accordance with accepted academic practice. No use, distribution or reproduction is permitted which does not comply with these terms.
*Correspondence: Godar Sene, senegodar@yahoo.fr">godar.sene@ucad.edu.sn,senegodar@yahoo.fr
§Deceased in 2017