- 1State Key Laboratory of Freshwater Ecology and Biotechnology, Institute of Hydrobiology, Chinese Academy of Sciences, Wuhan, China
- 2Institute of Hydrobiology, University of Chinese Academy of Sciences, Beijing, China
- 3Institute for Ecological Research and Pollution Control of Plateau Lakes, School of Ecology and Environmental Science, Yunnan University, Kunming, China
- 4College of Fisheries, Huazhong Agricultural University, Wuhan, China
- 5College of Fisheries and Life Science, Dalian Ocean University, Dalian, China
- 6School of Marine Sciences, Ningbo University, Ningbo, China
Nutrient loading reduction is an essential approach for controlling eutrophication. In addition to external nutrient loading, internal phosphorus (P) loading is usually considered one of the most important factors determining nutrient levels in water. The underwater light climate plays a pivotal role. However, few studies have been reported on the effect of light intensity on P release, and the existing studies have not drawn a definitive conclusion on whether underwater light has a positive or negative effect on P release. To confirm the effect of underwater light on internal P release, a 1-month microcosm experiment was carried out (18 November–15 December 2020) under three light intensities. The P release flux (FP) was significantly higher in the control (no light) group than in the low light group during the first 2 weeks (p = 0.03). No difference among treatments occurred for accumulative FP in the past 4 weeks, although it tended to be higher in the control than in both the low and high light groups (p > 0.05). Spearman rank correlations showed that photosynthetic photon flux density (PPFD) was positively correlated with DO, pH, phytoplankton chlorophyll a (Chl aPhyt) and benthic algae chlorophyll a (Chl aBent). DO was positively correlated with Chl aPhyt and Chl aBent. The results indicate that light may promote the growth of phytoplankton and benthic algae, both of which may increase DO and pH. DO inhibits P release from sediment and pH promotes P release from sediment. Lake-scale studies are needed to fully evaluate its effect under natural conditions, as many other factors (e.g., wind or fish disturbance) in natural lakes could regulate P release from sediment.
Introduction
Eutrophication of lakes is becoming a worldwide environmental problem caused by excess nutrient loading, e.g., phosphorus (P) and nitrogen (N) (Schindler, 1977; Schindler et al., 2008). The problems arising from eutrophication are the appearance of toxic algal blooms, death of submersed aquatic vegetation, fish kills, water quality deterioration and other serious environmental issues, which ultimately lead to hazards to public health (Smith, 2003; Gubelit and Berezina, 2010; Liu et al., 2016; Jeppesen et al., 2020; Wang et al., 2021). Controlling nutrient loading is essential for inhibiting eutrophication, especially P loading. However, in addition to external P loading, internal P release from sediment contributes substantial amounts of bioavailable P to overlying waters and is a considerable nutrient resource for the water column in eutrophic lakes (Søndergaard et al., 2001).
Internal P release is regulated by several environmental factors, such as temperature, pH, dissolved oxygen concentration (DO), and frequent hydrodynamic disturbances (Fan et al., 2001; Søndergaard et al., 2001; Li et al., 2013). Various measures have been applied to reduce the internal loading of P, including physical (dredging, in situ capping, etc.), chemical (chemical flocculants and synthetic materials) and biological (submerged macrophyte restoration) means (Zhang et al., 2021). Among physical methods, dredging can remove the nutrient-rich surface layer of sediment, which has been widely applied to control the internal nutrient loading of shallow lakes (Gustavson et al., 2008). The caps can form physical barriers on the surface of sediment mainly by spraying natural materials (sand, clay, gravel and rock), which effectively inhibit internal nutrient release. Chemical methods are often combined with physical barriers. Geoengineering materials act as solid phase P sorbents and have been widely used in many restoration practices, such as flocculants and phosphorus-locking materials (Wang et al., 2017; Yin et al., 2018; Jin et al., 2019; Yang et al., 2021).
Reestablishment of submerged macrophytes has become one of the most widely used methods in lake restoration considering their positive effect in maintaining a clear-water state. Submerged macrophytes have, but are not limited to, the capability to absorb nutrients and inhibit phytoplankton growth and sediment resuspension which may lead to decrease of internal P release (Hilt, 2006; Hilt and Gross, 2008; Zhang et al., 2016). In eutrophic lakes, impaired underwater light climate is one of the main factors hindering the growth of submerged macrophytes (Schelske et al., 2010; Zhang et al., 2016). Recently, artificial light-emitting diodes (LED) have been proposed as a potentially useful method for macrophyte reestablishment in eutrophic lakes. It has been confirmed that LED light supplementation can promote the growth rate of submerged macrophytes (Xu et al., 2019). Consequently, supplementation with artificial light can be a potentially useful approach for the restoration of eutrophic lakes. Some studies have found that artificial light supplementation could promote not only macrophyte growth (Grubisic et al., 2018) but also phytoplankton growth and then lead to internal P release by increasing alkaline phosphatase activity (APA) (Ma et al., 2018). However, few works have studied about the effects of light on internal P loading.
To study whether LED light can ultimately influence internal P loading under eutrophic conditions, a 1-month experiment with three light intensities was carried out in microcosms. The objectives of this study were to explore 1) the effect of LED light on P release from sediment and 2) the possible mechanism underlying the changes in P release under different light conditions. The results could provide a scientific basis for the control of internal P release in eutrophic lakes and may contribute to the development of the technology in lake restoration.
Material and Methods
Study Area and Experimental System Set-Up
The experiment was conducted in nine transparent Perspex tubes (inner diameter: 0.08 m; height: 1 m; volume: 5.0 L) at our field station near Lake Bao’an, which is located in the middle and lower Yangtze River Basin. Water (TN = 16.4 mg L−1, TP = 0.8 mg L−1) and sediment (TNSed, 4.96 g kg−1 dw; TPSed, 2.74 g kg−1 dw; OMSed, 43.87 g kg−1 dw) were collected in a hypertrophic urban lake—Lake Nanhu (Wuhan, China) (area: 7.67 km2, N 30°29ʹ10ʺ, E 114°22ʹ39ʺ). According to a survey conducted in Lake Nanhu from August 2019 to April 2020, the annual mean Secchi depth (ZSD) was 0.27 m, pH was 7.3, dissolved oxygen concentration (DO) was 8.4 mg L−1, total phosphorus (TP) was 0.46 mg L−1, and phytoplankton chlorophyll a (Chl aPhyt) was 112.2 μg L−1 at half water depth. Sediment (0.2 m in height) were collected using transparent Perspex tubes. To avoid disturbing the sediment, well-mixed lake water was then slowly added to the tubes along the wall and adjusted to a height of approximately 0.8 m (Supplementary Figure SA1). The introduction of sediment and water was completed on 12 November 2020.
Experimental Treatments
A gradient of three light intensities (at the sediment-water interface of the tubes) with three replicates was set: control (0 μmol m−2 s−1), low light (100 μmol m−2 s−1), and high light (200 μmol m−2 s−1). The light intensity in control group was near to 0 μmol m−2 s−1, the lowest value can be seen in the field and also the real situation in many hypertrophic urban lakes, such as Lake Nanhu in Wuhan (China). The low light of 100 μmol m−2 s−1 corresponds to the light intensity at one half of the water depth in Lake Nanhu. The high light of 200 μmol m−2 s−1 corresponds to light saturation point of benthic algae (Hill et al., 1995). Lights (white, 380–780 nm) were provided by the Institute of Semiconductors, Chinese Academy of Sciences. The dark/light cycle was 12:12 h. The experiment lasted 28 days, from 18 November 2020 to 15 December 2020.
Sampling and Sample Analyses
The first survey was conducted on 18 November, 7 days after the introduction of sediment and water. Environmental parameters pH, dissolved oxygen concentration (DO) and water temperature (WT) were measured in situ at the bottom of the water with a YSI Pro Plus (Yellow Spring Inc., Yellow Springs, OH, United States) once every week. Light intensity was measured twice (before and after treatment) at the sediment-water interface using an illuminometer (LI-192SA, LI-COR, Lincoln, NE, United States).
Water samples were taken at three depths (0.2, 0.4, 0.6 m, the distance between the sediment surface and the water surface). Well-mixed water samples were brought to the laboratory for chemical analysis once a week. In order to reduce the interference of sampling on water and sediment, the process of sampling was not repeated. Total nitrogen (TN) was determined following an alkaline potassium persulfate digestion-UV spectrophotometric method (PERSEE, TU-1810, Beijing) (Huang et al., 1999). Total phosphorus (TP) in the water column was determined using an ammonium molybdate-ultraviolet spectrophotometric method after digestion with K2S2O8 solution (PERSEE, TU-1810, Beijing) (Huang et al., 1999). Soluble reactive phosphorus (SRP) in the water column was measured using the molybdenum blue method (Murphy and Riley, 1962) with water filtered through 0.45 μm Millipore filter paper (Whatman, GE Health care UK Limited, Buckinghamshire, United Kingdom). Phytoplankton chlorophyll a (Chl aPhyt) was extracted using 90% acetone (at 4°C for 24 h) after filtration through GF/C filters (Whatman, GE Health care UK Limited, Buckinghamshire, United Kingdom), and absorbance was then measured at 665 and 750 nm, both before and after acidification with 10% HCl using a spectrophotometer (PERSEE, TU-1810, Beijing) (Huang et al., 1999).
Pore-water samples of the surficial 10 cm sediment in each tube were collected once a week using a soil moisture sampler (SMS rhizons, Eijkelkamp, Giesbeek, Netherlands) and were subsequently filtered with a 0.45 μm cellulose acetate membrane to measure SRP (Murphy and Riley, 1962).
Surface sediment samples (0–0.1 m) were taken by a plexiglass corer (inner diameter: 0.08 m). The top layer (1 cm) of the samples was placed in 10 ml plastic tubes to measure the benthic algae chlorophyll a (Chl aBent). The samples were extracted with 7 ml of 90% acetone at 4°C for 24 h and then centrifuged for 10 min at approximately 4000 rpm. The absorbance at 665 and 750 nm, both before and after acidification with 10% HCl, was used to calculate Chl aBent using a spectrophotometer (PERSEE, TU-1810, Beijing) (Huang et al., 1999; Boer et al., 2009).
Phosphorus release flux was calculated by comparing TP concentrations in overlying water (Boström and Pettersson, 1982; Nürnberg and Gertrud, 1987).
where FP is the phosphorus release flux (mg (m2 d)−1), M is the variation in phosphorus with time t (mg), T is the days to calculate FP (d), Vt is the volume of overlying water with time t (L), A is the area of the sediment-water interface (m2), and Ct and Ct−1 are the TP concentrations in water at t and t−1, respectively (mg L−1). Equation 1 represents the cumulative effect through calculating Fp.
Statistical Analyses
R 4.0.3 and OriginPro 2021 were used to process and analyze the data. Spearman rank correlations were applied to test the relationships between environmental parameters using R 4.0.3. Regression analysis were used to test the effect of photosynthetic photon flux density (PPFD) on FP. These two analyses are commonly used to study the relationship between variables in statistics. They can determine that two sets of variables have statistical correlation. The difference is that regression analysis can quantitatively obtain the relationship between the two variables, one of which can be regarded as a cause and the other as a result. To determine the difference between treatments, a Friedman test (F-test) was conducted using R. Wilcoxon-Nemenyi-McDonald-Thompson post-hoc test was used when the Friedman test gave a significant p value (<0.05).
Results
Variation in Environmental Factors
The initial water quality parameters differed very little among treatments (Figure 1). PPFD among the treatments showed a clear gradient, which was highest in the high light (207.75 ± 22.09 μmol m−2 s−1), followed by low light (121.17 ± 3.6 μmol m−2 s−1) and control (0.00 ± 0.00 μmol m−2 s−1) (p = 0.05), as expected (Table 1).
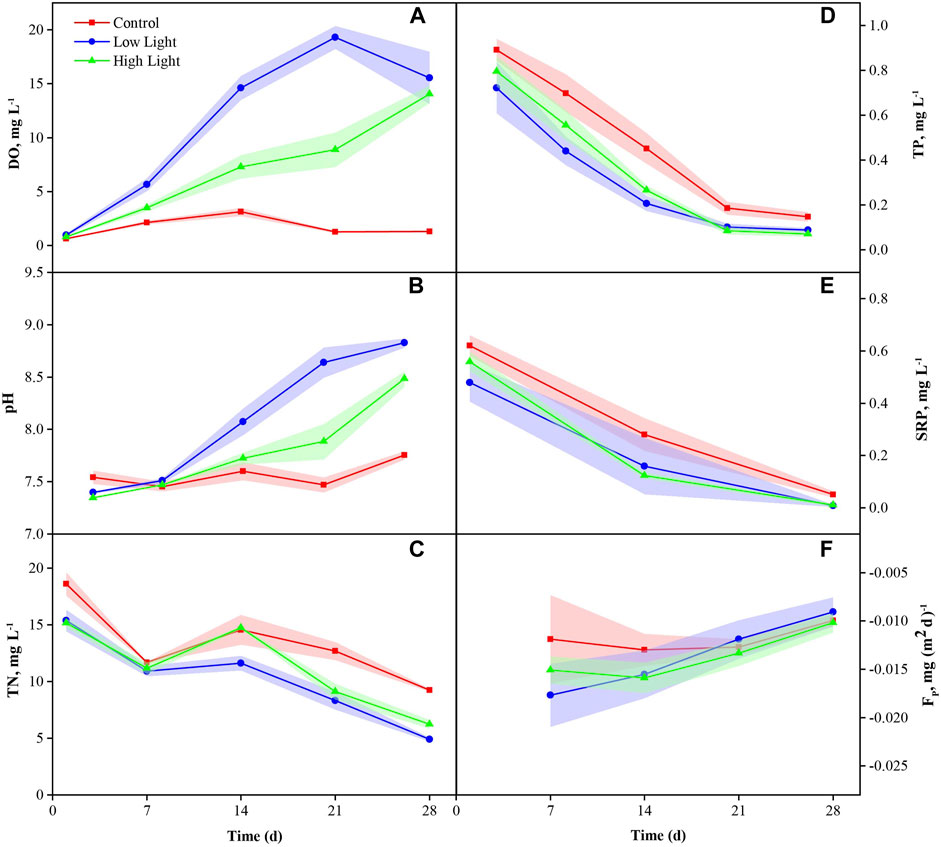
FIGURE 1. Dissolved oxygen (DO) (mean ± SE) (A), pH (B), total nitrogen (TN) (C), total phosphorus (TP) (D), soluble reactive phosphorus (SRP) (E) and P release flux (FP) (F) in various treatments during the experiment.
DO showed a significant difference among the treatments (p < 0.01) on the 14th day of the experiment, being significantly higher in the low light and high light groups than in the control (p = 0.03). On the 28th day, DO showed a pattern like the previous 2 weeks, being significantly higher in the low light and high light groups than in the control (p = 0.03) (Supplementary Table SA1; Figure 1A).
The pH showed no significant differences among the treatments in the first 2 weeks (p > 0.05), while a significant difference was discerned between the low light and high light groups, being significantly higher in the low light group than in the control (p = 0.05). On the 28th day, the pH was significantly higher in the low light and high light groups than in the control (p < 0.01) and was significantly higher in the low light group than in the high light group (p < 0.01) (Supplementary Table SA1; Figure 1B).
The low light and high light groups had similar Chl aPhyt (p = 0.75), both were significantly higher than that in the control group (p = 0.03) (Supplementary Table SA1; Figure 2B). No difference was found in Chl aBent in any treatment (p = 0.37) (Supplementary Table SA1; Figure 2A).
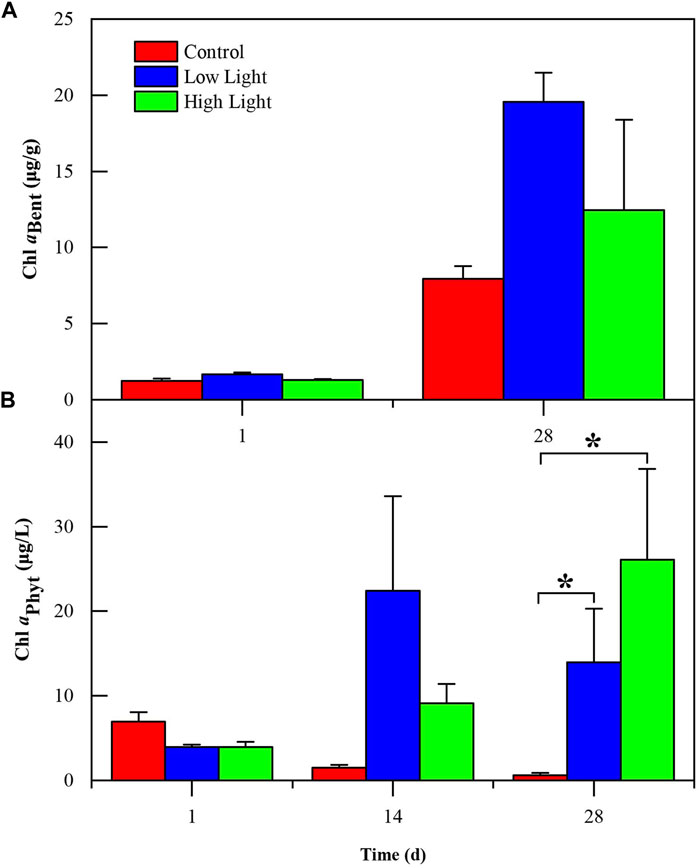
FIGURE 2. Phytoplankton chlorophyll a (Chl aPhyt) (mean ± SE) (A) and benthic algae chlorophyll a (Chl aBent) (B) in various treatments during the experiment. The label (*) indicates significant differences (p < 0.05) among treatments.
Variation in Phosphorus Variables
For TP in the water column, both the low light and high light groups showed no significant difference, and both were significantly lower than the control (p < 0.05) (Supplementary Table SA1; Figure 1D). TN in the water column showed a similar pattern (p < 0.05) (Supplementary Table SA1; Figure 1C). The SRP in the water column showed no significant differences among the treatments in the first 2 weeks (p > 0.05). In the last week, the SRP was significantly higher in the control group than in the high light group (p < 0.03) (Supplementary Table SA1; Figure 1E).
FP showed no significant differences among the treatments in the first 2 weeks (p > 0.05), while a significant difference was discerned between the control and low light groups, being significantly higher in the control than in the low light group (p = 0.03). No difference of accumulative FP among treatments occurred in the past 4 weeks, although it tended to be higher in the control group than in the low light and high light groups (p > 0.05) (Supplementary Table SA1; Figure 1F).
Relationships of PPFD With Phosphorus Variables and Influencing Factors
Spearman rank correlation showed that DO, pH, Chl aPhyt and Chl aBent were positively correlated with PPFD. PPFD was significantly positively correlated with Chl aPhyt (p = 0.03) (Supplementary Table SA2; Figure 3). TP, SRP and TN were negatively correlated with PPFD. PPFD was significantly negatively correlated with TP and SRP (p < 0.05) (Supplementary Table SA2; Figure 3). In the scatterplots, PPFD has a significantly positive regression with DO, pH and Chl aPhyt and a significantly negative regression with TP, SRP and TN (p < 0.05) (Figure 4).
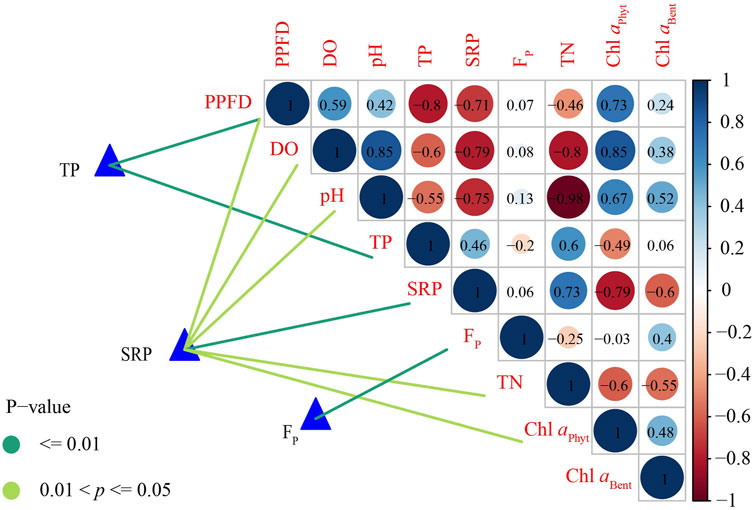
FIGURE 3. Spearman rank correlation of phosphorus variables with environmental factors (blue: positive correlation; red: negative correlation; color shade and circle size: proportion to correlation coefficient; number: correlation coefficient). Total phosphorus (TP), soluble reactive phosphorus (SRP) and P release flux (FP) were related to each environmental factor by Mantel tests (edge color denotes statistical significance) (PPFD, photosynthetic photon flux density; DO, dissolved oxygen; TN, total nitrogen; Chl aPhyt, phytoplankton chlorophyll a; Chl aBent, benthic algae chlorophyll a).
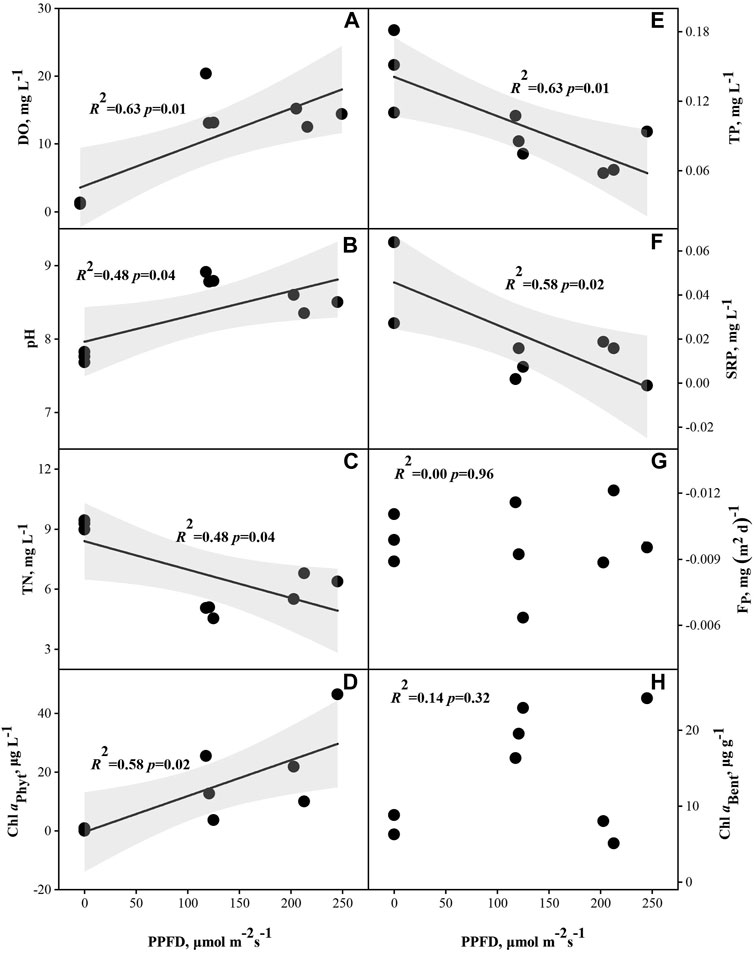
FIGURE 4. Relationships between photosynthetic photon flux density (PPFD) and dissolved oxygen (DO) (n = 9) (A), pH (B), total nitrogen (TN) (C), total phosphorus (TP) (E), soluble reactive phosphorus (SRP) (F), P release flux (FP) (G), phytoplankton chlorophyll a (Chl aPhyt) (D) and benthic algae chlorophyll a (Chl aBent) (H).
Relationships of Influencing Factors With Phosphorus Variable
Spearman rank correlation showed that SRP were significantly positive correlated with TN and a significantly negative correlation with DO, pH and Chl aPhyt (p < 0.05) (Supplementary Table SA2; Figure 5). TP and SRP were negatively correlated with Chl aBent. There were no significant relationships between FP and PPFD, DO, Chl aBent, Chl aPhyt and TN (p > 0.05) (Supplementary Table SA2; Figure 3). In the scatterplots, TP has a significantly negative regression with DO and pH and a significantly positive regression with TN, which was the same as SRP (p < 0.05) (Figure 5). In addition, there was a significantly negative regression between SRP and Chl aPhyt (p < 0.05) (Figure 5).
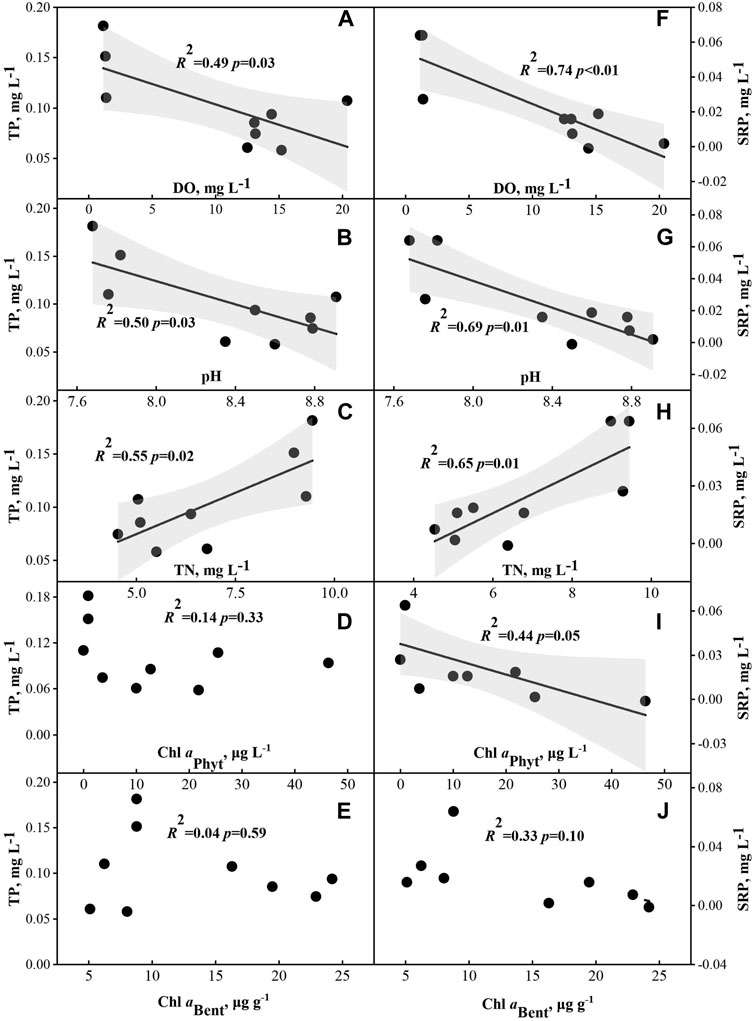
FIGURE 5. Relationships between phosphorus variables (total phosphorus (TP) (A–E); soluble reactive phosphorus (SRP) (F–J)) and dissolved oxygen (DO) (n = 9), pH, total nitrogen (TN), phytoplankton chlorophyll a (Chl aPhyt) and benthic algae chlorophyll a (Chl aBent).
Discussion
In our 1-month experiment with gradient artificial light supplementation, artificial light inhibited internal P release in at least the first 14 days. FP was lower in the treatments with light from LEDs, even though only a significant difference was discerned between the control and low light groups (p = 0.03) in the first 14 days. TP and SRP declined significantly with increasing PPFD, although they decreased in all treatments with time, which might indicate that our experimental systems favor the settlement of suspended particles (Schindler, 1998) (Figure 4E; Figure 4F). The results demonstrated that the addition of light from LEDs can inhibit sediment P release. Wang and Pei (2013) reported that the absence of light can significantly increase the SRP concentrations, which increased from 0.013 mg L−1 (10 days) to 0.021 mg L−1 (30 days). Similar patterns were found in dissolved total phosphorus (DTP), and the concentration of DTP under dark conditions was approximately eight times that under light conditions (Jiang et al., 2008). However, it was also reported in previous studies that illumination can increase PO43- because of photocatalysis (Zhang et al., 2019; Li X. et al., 2017), which increased from 0.31 mg L−1 to 0.41 mg L−1 after illumination (Li X. et al., 2017). These findings suggested that photocatalysis can change organic phosphorus into available phosphorus. However, there was less organic phosphorus in our experimental system, so the photocatalytic effect was weak.
In aquatic ecosystems, algae can generate O2 via photosynthesis under light conditions, which would reduce P release. O2 oxidizes Fe2+ into Fe3+, which easily combines with phosphorus to form a stable state, thereby reducing P release (Carlton and Wetzel, 1988; Hemond and Lin, 2010). In our experiment, Chl aPhyt was 14 times greater in the low light and 26 times greater in the high light than that in the control at the end of the experiment (p = 0.75) (Supplementary Table SA1; Figure 2A). Chl aBent followed the same pattern as Chl aPhyt, which was 2.5 times greater in the low light and 1.6 times greater in the high light than in the control at the end of the experiment (Supplementary Table SA1; Figure 2B). DO in the low light was 12 times greater and in the high light was 11 times greater than in the control and was significantly higher in the low light than in the high light on the 14th day of the experiment (p < 0.01) (Supplementary Table SA1; Figure 1A). TP and SRP showed a significant negative correlation with DO (p < 0.05) (Supplementary Table SA2; Figure 3). According to correlation analysis, the increase in DO caused by the growth of benthic algae and phytoplankton under light conditions might be one of the reasons for inhibiting sediment P release in our study.
Photosynthesis of algae produce O2 and OH− and elevate pH, which might regulate sediment P release (Zhang and Sun, 2004). P speciation according to binding forms includes the NaOH-P (P bound to metal oxides) and the HCl-P (consist mainly of apatite P) (Jin et al., 2006). pH influences chemical processes, such as NaOH-P release under alkaline conditions (pH > 8), HCl-P release under acidic conditions (pH < 6) while no sediment P release under neutral conditions (pH ≈ 7) (Jin et al., 2006; Li et al., 2013). In this study, pH was significantly higher in the low light group (pH = 8.8) than in the high light group (pH = 8.5) (p < 0.01) (Supplementary Table SA1; Figure 1B). The pH was positively correlated with Chl aPhyt and Chl aBent even though not significantly (p > 0.05) (Supplementary Table SA2; Figure 3). FP showed a significant positive correlation with pH (p < 0.05). The results suggested that the increased pH in the light treatment might promote internal P release.
The concentrations of SRP declined significantly with increasing Chl aPhyt (p = 0.05) (Figure 5I), which may promote sediment P release. The above results showed that increasing light intensity may increase the uptake of phosphorus by algae in the surrounding environment, resulting in a decrease in the concentrations of SRP in water and an increase in the phosphorus concentration gradient at the water-sediment interface, thereby increasing the upward diffusion of sediment P to overlying water (Xie et al., 2003).
In aquatic ecosystems, microbial activity significantly affects sediment P release, in which bacteria can directly promote sediment P release through decomposition (Petterson, 1998). Algae and bacteria may promote P release by increasing APA which could decompose organic P into PO43−, which provides the required P for the growth of algae and then promote internal P release (Ma et al., 2018). The increased Chl aPhyt and Chl aBent in light treatments indicated the possible positive effect of APA on P release in this study. Photodegradation is also one of the possible factors that promotes sediment P release, which converts organic P into available P with direct and indirect conversion (Li X. et al., 2017). The direct decomposition of organic P under light is determined by its structure and morphology and the indirect decomposition of organic P into PO43− by reactions with reactive oxygen species (ROS) (Nowack, 2003). In this study, APA and photodegradation were not measured, while light supplement finally decreased sediment P release through several combination approaches.
Our 1-month microcosm results suggest that light might inhibit sediment P release by promoting the growth of phytoplankton and benthic algae and improving the oxidation conditions in the sediment, and phosphorus inhibition effect of 100 μmol m−2 s−1 (low light treatment) was better than 200 μmol m−2 s−1 (high light treatment). Therefore, it is feasible to control sediment P release by artificial light supplementation in the ecological restoration of eutrophic lakes. However, our study has some limitations: 1) such small experimental system was unable to simulate the sediment resuspension caused by biotic (fish, crab, etc.) and abiotic (wind, hydrodynamic, etc.) factors in a natural lake; 2) this study set up a limited light intensity gradient. There were three light gradients in our experiment (0, 100, and 200 μmol m−2 s−1), and low light was the best among the three light intensities. However, the threshold of light intensity for inhibiting sediment P release remains to be verified; 3) the mechanism underlying the changes in P release under different light conditions remains to be futher studied. In sum, the feasibility of LED light supplementation needs further exploration in larger-scale experimental systems or field studies.
Data Availability Statement
The original contributions presented in the study are included in the article/Supplementary Material, further inquiries can be directed to the corresponding authors.
Author Contributions
MZ, YL, H-JW, and H-ZW designed the research. MZ, Y-XY, J-HL, R-TQ, and ML carried out the research. MZ, YL, H-JW, and H-ZW performed the data analyses. MZ and YL prepared the original draft of the paper. H-JW, H-ZW, and S-NM commented on the various drafts.
Funding
The authors declare that this study received funding from the State Key Laboratory of Freshwater Ecology and Biotechnology (2019FBZ01). H-JW was supported by the Youth Innovation Association of Chinese Academy of Sciences (Y201859) as an excellent member and by the Yunnan Provincial Department of Science and Technology (202001BB050078, 202103AC100001). YL was supported by the Wuhan Science and Technology Plan Project 2020020602012152). These funders were not involved in the study design, collection, analysis, interpretation of data, the writing of this article, or the decision to submit it for publication.
Conflict of Interest
The authors declare that this study received funding from The Research Project of Wuhan Municipal Construction Group Co., Ltd. (wszky202014). The funder had the following involvement with the study: involvement in the design of the article.
Publisher’s Note
All claims expressed in this article are solely those of the authors and do not necessarily represent those of their affiliated organizations, or those of the publisher, the editors and the reviewers. Any product that may be evaluated in this article, or claim that may be made by its manufacturer, is not guaranteed or endorsed by the publisher.
Acknowledgments
The authors thank Prof. Hua Yang of the Institute of Semiconductors, Chinese Academy of Sciences, and Prof. Xue-Gong Hu of the Institute of Engineering Thermophysics, Chinese Academy of Sciences for providing the LED lights.
Supplementary Material
The Supplementary Material for this article can be found online at: https://www.frontiersin.org/articles/10.3389/fenvs.2022.801469/full#supplementary-material
References
Böer, S. I., Arnosti, C., van Beusekom, J. E. E., and Boetius, A. (2009). Temporal Variations in Microbial Activities and Carbon Turnover in Subtidal Sandy Sediments. Biogeosciences 6, 1149–1165. doi:10.5194/bg-6-1149-2009
Boström, B., and Pettersson, K. (1982). Different Patterns of Phosphorus Release from Lake Sediments in Laboratory Experiments. Hydrobiologia 91-92, 415–429. doi:10.1007/bf02391957
Carlton, R. G., and Wetzel, R. G. (1988). Phosphorus Flux from Lake Sediments: Effect of Epipelic Algal Oxygen Production. Limnol. Oceanogr. 33, 562–570. doi:10.4319/lo.1988.33.4.0562
Fan, C. X., Zhang, L., and Qu, W. C. (2001). Lake Sediment Resuspension and Caused Phosphate Release- A Simulation Study. J. Environ. Sci. 13 (4), 406–410. CNKI:SUN:HJKB.0.2001-04-004. doi:10.3321/j.issn:1001-0742.2001.04.005
Grubisic, M., Van Grunsven, R. H. A., Manfrin, A., Monaghan, M. T., and Hölker, F. (2018). A Transition to White LED Increases Ecological Impacts of Nocturnal Illumination on Aquatic Primary Producers in a Lowland Agricultural Drainage Ditch. Environ. Pollut. 240, 630–638. doi:10.1016/j.envpol.2018.04.146
Gubelit, Y. I., and Berezina, N. A. (2010). The Causes and Consequences of Algal Blooms: The Cladophora Glomerata Bloom and the Neva Estuary (Eastern Baltic Sea). Mar. Pollut. Bull. 61 (4), 183–188. doi:10.1016/j.marpolbul.2010.02.013
Gustavson, K. E., Burton, G. A., Francingues, N. R., Reible, D. D., Vorhees, D. J., and Wolfe, J. R. (2008). Evaluating the Effectiveness of Contaminated-Sediment Dredging. Environ. Sci. Technol. 42 (14), 5042–5047. doi:10.1021/es087185a
Hemond, H. F., and Lin, K. (2010). Nitrate Suppresses Internal Phosphorus Loading in an Eutrophic Lake. Water Res. 44, 3645–3650. doi:10.1016/j.watres.2010.04.018
Hill, W. R., Ryon, M. G., and Schilling, E. M. (1995). Light Limitation in a Stream Ecosystem: Responses by Primary Producers and Consumers. Ecology 76 (4), 1297–1309. doi:10.2307/1940936
Hilt, S. (2006). Allelopathic Inhibition of Epiphytes by Submerged Macrophytes. Aquat. Bot. 85, 252–256. doi:10.1016/j.aquabot.2006.05.004
Hilt, S., and Gross, E. M. (2008). Can Allelopathically Active Submerged Macrophytes Stabilise Clear-water States in Shallow Lakes? Basic Appl. Ecol. 9, 422–432. doi:10.1016/j.baae.2007.04.003
Huang, X. F., Chen, W. M., and Cai, Q. M. (1999). Standard Methods for Observation and Analysis in Chinese Technology of Lake Ecology. Beijing, China: Standards Press of China. (in Chinese).
Jeppesen, E., Beklioğlu, M., Özkan, K., and Akyürek, Z. (2020). Salinization Increase Due to Climate Change Will Have Substantial Negative Effects on Inland Waters: A Call for Multifaceted Research at the Local and Global Scale. Innovation (N Y) 1 (2), 100030. doi:10.1016/j.xinn.2020.100030
Jiang, X., Jin, X., Yao, Y., Li, L., and Wu, F. (2008). Effects of Biological Activity, Light, Temperature and Oxygen on Phosphorus Release Processes at the Sediment and Water Interface of Taihu Lake, China. Water Res. 42, 2251–2259. doi:10.1016/j.watres.2007.12.003
Jin, X., Bi, L., Lyu, T., Chen, J., Zhang, H., and Pan, G. (2019). Amphoteric Starch-Based Bicomponent Modified Soil for Mitigation of Harmful Algal Blooms (HABs) with Broad Salinity Tolerance: Flocculation, Algal Regrowth, and Ecological Safety. Water Res. 165, 115005. doi:10.1016/j.watres.2019.115005
Jin, X., Wang, S., Pang, Y., and Chang Wu, F. (2006). Phosphorus Fractions and the Effect of pH on the Phosphorus Release of the Sediments from Different Trophic Areas in Taihu Lake, China. Environ. Pollut. 139, 288–295. doi:10.1016/j.envpol.2005.05.010
Li, H., Liu, L., Li, M., and Zhang, X. (2013). Effects of pH, Temperature, Dissolved Oxygen, and Flow Rate on Phosphorus Release Processes at the Sediment and Water Interface in Storm Sewer. J. Anal. Methods Chem. 2013, 1–7. doi:10.1155/2013/104316
Li, X., Zhou, Y., Liu, G., Lei, H., and Zhu, D. (2017). Mechanisms of the Photochemical Release of Phosphate from Resuspended Sediments under Solar Irradiation. Sci. Total Environ. 595, 779–786. doi:10.1016/j.scitotenv.2017.04.039
Li, Y., Wang, H. Z., Liang, X. M., Yu, Q., Xiao, X. C., Shao, J. C., et al. (2017). Total Phytoplankton Abundance Is Determined by Phosphorus Input: Evidence from an 18-month Fertilization experiment in Four Subtropical Ponds. Can. J. Fish. Aquat. Sci. 74 (9), 1454–1461. doi:10.1139/cjfas-2016-0057
Liu, Y., Xu, Y., Wang, Z., Xiao, P., Yu, G., Wang, G., et al. (2016). Dominance and Succession of Microcystis Genotypes and Morphotypes in Lake Taihu, A Large and Shallow Freshwater Lake in China. Environ. Pollut. 219, 399–408. doi:10.1016/j.envpol.2016.05.021
Ma, S. N., Wang, H. J., Wang, H. Z., Li, Y., Liu, M., Liang, X. M., et al. (2018). High Ammonium Loading Can Increase Alkaline Phosphatase Activity and Promote Sediment Phosphorus Release: A Two-Month Mesocosm Experiment. Water Res. 145, 388–397. doi:10.1016/j.watres.2018.08.043
Murphy, J., and Riley, J. P. (1962). A Modified Single Solution Method for the Determination of Phosphate in Natural Waters. Analytica Chim. Acta 27, 31–36. doi:10.1016/s0003-2670(00)88444-5
Nowack, B. (2003). Environmental Chemistry of Phosphonates. Water Res. 37 (11), 2533–2546. doi:10.1016/S0043-1354(03)00079-4
Nürnberg, G. K., and Gertrud, K. (1987). A Comparison of Internal Phosphorus Loads in Lakes with Anoxic Hypolimnia: Laboratory Incubation versus In Situ Hypolimnetic Phosphorus Accumulation. Limnol. Oceanogr. 32 (5), 1160–1164. doi:10.4319/lo.1987.32.5.1160
Pettersson, K. (1998). Mechanisms for Internal Loading of Phosphorus in Lakes. Hydrobiologia 373/374, 21–25. doi:10.1023/A:1017011420035
Schelske, C. L., Lowe, E. F., Kenney, W. F., Battoe, L. E., Brenner, M., and Coveney, M. F. (2010). How Anthropogenic Darkening of Lake Apopka Induced Benthic Light Limitation and Forced the Shift from Macrophyte to Phytoplankton Dominance. Limnol. Oceanogr. 55 (3), 1201–1212. doi:10.4319/lo.2010.55.3.1201
Schindler, D. W. (1977). Evolution of Phosphorus Limitation in Lakes. Science 195 (4275), 260–262. doi:10.1126/science.195.4275.260
Schindler, D. W., Hecky, R. E., Findlay, D. L., Stainton, M. P., Parker, B. R., Paterson, M. J., et al. (2008). Eutrophication of Lakes Cannot Be Controlled by Reducing Nitrogen Input: Results of A 37-year Whole-Ecosystem Experiment. Proc. Natl. Acad. Sci. 105 (32), 11254–11258. doi:10.1073/pnas.0805108105
Schindler, D. W. (1998). Replication versus Realism: the Need for Ecosystem-Scale Experiments. Ecosystems 1 (4), 323e334. Available at: http://www.jstor.org/stable/3658915. doi:10.1007/s100219900026
Smith, V. H. (2003). Eutrophication of Freshwater and Coastal marine Ecosystems a Global Problem. Environ. Sci. Pollut. Res. 10 (2), 126–139. doi:10.1065/espr2002.12.142
Sondergaard, M., Jensen, P. J., and Jeppesen, E. (2001). Retention and Internal Loading of Phosphorus in Shallow, Eutrophic Lakes. Scientific World J. 1, 427–442. doi:10.1100/tsw.2001.72
Wang, C., and Pei, Y. (2013). Effects of Light, Microbial Activity, and Sediment Resuspension on the Phosphorus Immobilization Capability of Drinking Water Treatment Residuals in Lake Sediment. Environ. Sci. Pollut. Res. 20, 8900–8908. doi:10.1007/s11356-013-1865-9
Wang, G., Wang, Y., Guo, Y., and Peng, D. (2017). Effects of Four Different Phosphorus-Locking Materials on Sediment and Water Quality in Xi'an Moat. Environ. Sci. Pollut. Res. 24, 264–274. doi:10.1007/s11356-016-7796-5
Wang, H., Xu, C., Liu, Y., Jeppesen, E., Svenning, J.-C., Wu, J., et al. (2021). From Unusual Suspect to Serial Killer: Cyanotoxins Boosted by Climate Change May Jeopardize Megafauna. The Innovation 2 (2), 100092. doi:10.1016/j.xinn.2021.100092
Xie, L., Xie, P., Li, S., Tang, H., and Liu, H. (2003). The Low TN:TP Ratio, a Cause or a Result of Microcystis Blooms? Water Res. 37, 2073–2080. doi:10.1016/S0043-1354(02)00532-8
Xu, C., Wang, H.-J., Yu, Q., Wang, H.-Z., Liang, X.-M., Liu, M., et al. (2019). Effects of Artificial LED Light on the Growth of Three Submerged Macrophyte Species during the Low-Growth Winter Season: Implications for Macrophyte Restoration in Small Eutrophic Lakes. Water 11, 1512. doi:10.3390/w11071512
Yang, C., Yang, P., and Yin, H. (2021). In Situ Control of Internal Nutrient Loading and Fluxes in the Confluence Area of an Eutrophic Lake with Combined P Inactivation Agents and Modified Zeolite. Sci. Total Environ. 775, 145745. doi:10.1016/j.scitotenv.2021.145745
Yin, H., Douglas, G. B., Cai, Y., Liu, C., and Copetti, D. (2018). Remediation of Internal Phosphorus Loads with Modified Clays, Influence of Fluvial Suspended Particulate Matter and Response of the Benthic Macroinvertebrate Community. Sci. Total Environ. 610-611, 101–110. doi:10.1016/j.scitotenv.2017.07.243
Zhang, H., Lyu, T., Liu, L., Hu, Z., Chen, J., Su, B., et al. (2021). Exploring a Multifunctional Geoengineering Material for Eutrophication Remediation: Simultaneously Control Internal Nutrient Load and Tackle Hypoxia. Chem. Eng. J. 406, 127206. doi:10.1016/j.cej.2020.127206
Zhang, P. L., and Sun, C. J. (2004). The Influence of Algae Growing on pH and Do in Surface Water. Environ. Monit. China 20 (4), 49–50. 1002-6002(2004)20:4<49:DBSTZZ>2.0.TX;2-B. doi:10.3969/j.issn.1002-6002.2004.04.016
Zhang, X., Li, J., Fan, W.-Y., and Sheng, G.-P. (2019). Photomineralization of Effluent Organic Phosphorus to Orthophosphate under Simulated Light Illumination. Environ. Sci. Technol. 53 (9), 4997–5004. doi:10.1021/acs.est.9b00348
Keywords: artificial light supplement system, Eutrophication, internal P loading, Phytoplankton, Periphyton
Citation: Zhang M, Li Y, Wang H-, Yu Y-, Liu J-, Qiao R-, Liu M, Ma S- and Wang H- (2022) Decreased Internal Phosphorus Loading From Eutrophic Sediment After Artificial Light Supplement: Preliminary Evidence From a Microcosm Experiment. Front. Environ. Sci. 10:801469. doi: 10.3389/fenvs.2022.801469
Received: 25 October 2021; Accepted: 09 February 2022;
Published: 24 March 2022.
Edited by:
Zhengwen Liu, Jinan University, ChinaReviewed by:
Changhui Wang, Nanjing Institute of Geography and Limnology (CAS), ChinaJing Lu, Griffith University, Australia
Copyright © 2022 Zhang, Li, Wang, Yu, Liu, Qiao, Liu, Ma and Wang. This is an open-access article distributed under the terms of the Creative Commons Attribution License (CC BY). The use, distribution or reproduction in other forums is permitted, provided the original author(s) and the copyright owner(s) are credited and that the original publication in this journal is cited, in accordance with accepted academic practice. No use, distribution or reproduction is permitted which does not comply with these terms.
*Correspondence: Hai-Jun Wang, d2FuZ2hqQGloYi5hYy5jbg==; Hong-Zhu Wang, d2FuZ2h6QGloYi5hYy5jbg==
†These authors have contributed equally to this work and share first authorship