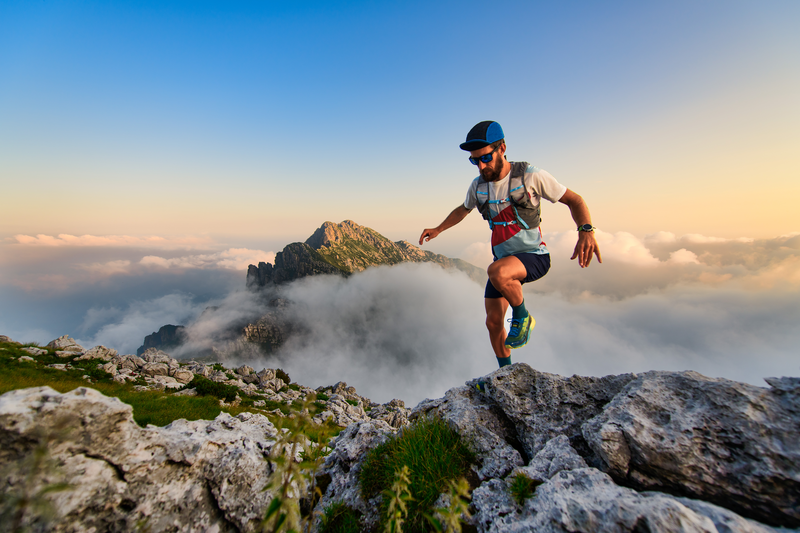
94% of researchers rate our articles as excellent or good
Learn more about the work of our research integrity team to safeguard the quality of each article we publish.
Find out more
ORIGINAL RESEARCH article
Front. Environ. Sci. , 11 August 2022
Sec. Toxicology, Pollution and the Environment
Volume 10 - 2022 | https://doi.org/10.3389/fenvs.2022.778544
This article is part of the Research Topic Hydrobiogeochemistry of Major Asian Rivers View all 11 articles
The study area was selected in the Rupsha river basin and the sediment samples were collected to determine trace metal concentrations of As, Pb, Cd, and Cr along with biological effects, and potential ecological and human health hazards for adults and children. The concentrations of trace metals were detected from sixty composite sediment samples using an Atomic Absorption Spectrophotometer (AAS) following some sequential analytical procedures. The mean concentrations of trace metals were organized in the descending order of chromium (Cr) (43.2 mg/kg) > lead (Pb) (29.21 mg/kg) > arsenic (As) (5.18 mg/kg) > cadmium (Cd) (1.8 mg/kg). The current study highlighted that metals were attributed to the riverine sediments from natural sources and other anthropogenic sources, particularly from various industries. Based on the effect-range classifications of threshold effect concentration (TEC) and probable effect concentration (PEC), the trace metal concentrations can impact on the sediment-dwelling organisms occasionally especially for Cr, Cd, and Pb. That is, the concentrations had negative biological consequences on aquatic creatures. The assessed potential ecological risk of Cd offers a significant risk to the aquatic ecosystem, whereas As, Cr, and Cd were in low-risk. Most of the sites of the study area were within the range of moderate risk, indicated by the risk index (RI) values. Furthermore, the applied sediment quality indices, geo-accumulation index (Igeo) indicated that sediment was contaminated by Cd whereas contamination factor (CF) denoted that the sediment of the study area was moderately polluted by Pb. However, pollution load index (PLI) revealed that the study area was polluted for cumulative sence especially in winter season. The age-group risk index (HI) was much lower than the threshold limit of 1, showing that the pollution had no non-carcinogenic risk effect. Total carcinogenic risk (TCR) was less than one-tenth of a percentile. For the sake of human and environmental health, proper monitoring of metal element attribution and strict regulation are required to lessen trace metal pollution.
Because of the toxicity and persistence in the environment, heavy metal pollution has become a global, regional, and local concern (Ahmed et al., 2020; Islam A et al., 2020; Khan et al., 2020; Babu et al., 2021; Kumar et al., 2021; Ali et al., 2022). The aquatic ecology is effected by the various toxic trace metals as most of them are long-persistent, bio-accumulative character and non-biodegradable in nature (Bhuyan et al., 2017; Ali et al., 2018; Bhuyan et al., 2019). Many anthropogenic actions released heavy metals into the aquatic habitats directly or indirectly and these metals are carried via the water column, deposited in sediment, and biomagnified through the food chain (Kahal et al., 2020; Ali et al., 2022), resulting from a considerable risk to benthic invertebrates, fish and humans (Ali et al., 2016; Islam et al., 2016; Ali et al., 2018, Shen et al., 2019; Shaheen et al., 2019; Ali et al., 2020a, b).
In a riverine ecosystem, the concentration of HM in sediment is thought to be an indicator of monitoring the environmental conditions and pollution status (Han et al., 2018; Rahman et al., 2019; Hossain et al., 2021; Ali et al., 2022). As metals tend to accumulate in the bottom layers of riverine water bodies, heavy metals are much higher in sediment than in the water column (Bhuyan et al., 2017; Islam et al., 2021; Jolly et al., 2021). Heavy metals are not permanently attached to sediments, and they can be released into the water column due to the change in environmental circumstances such as temperature and pH, or other physical or biological disturbances (Agarwal et al., 2005). A lower concentration of HMs is necessary for organism survival, whereas higher concentrations are poisonous and adversely impact organisms (Fu and Wang 2011; Ahmed et al., 2020). Thus, the monitoring of sediment provides valuable information on a variety of pollution indicators.
Different sediment quality indicators can be used to determine the extent of contamination and the biological characteristics of sediment in any aquatic ecosystem (Ganugapenta et al., 2018). For pollution control, it is important to understand the origins of pollutants in sediments of the aquatic environments. Multivariate statistical approaches, such as Pearson correlation analysis, principal components analysis (PCA), and cluster analysis, are considered to be practical tools for identifying pollution sources and have been successfully used in several studies on heavy metal pollution in sediments (Larrose et al., 2010; Bai et al., 2011; Yi et al., 2011; Chabukdhara and Nema 2012). Analysis of toxic elements in riverine sediment is essential for environmental contamination assessment (Ali et al., 2018). Heavy metals in sediments pose distinct ecological risks to different receptors. The possible harmful impacts of heavy metals on sediment-dwelling organisms in freshwater systems have been assessed using the criteria in sediment quality guidelines (SQGs) (Persaud et al., 1993; Ingersoll et al., 1996; Smith et al., 1996; MacDonald et al., 2000). Few SQGs, on the other hand, have been conducted to assess the negative impacts of heavy metals in sediment on higher trophic levels (fish or other species) (Bhavsar et al., 2010). Hakanson (1980) proposed a prospective ecological risk index based on heavy metal concentrations in sediment, the simplest and most widely used method for ecological risk assessment of the sediment.
Metal contamination has become a major problem in many rapidly developing countries (Ali et al., 2016; Bhuyan et al., 2019; Jolly et al., 2021; Rahman et al., 2021). Especially, in developing country people like Bangladesh, human healths are suffering from various diseases due to the contact of the toxic metals (Islam et al., 2016; Rehman et al., 2018). Many industries dump their untreated agricultural and chemical effluents into rivers directly. The water from these rivers and their tributaries and distributaries is used to irrigate agricultural lands for crop production (Ali et al., 2016; Islam et al., 2016; Ali et al., 2020a). The Rupsha River is an ecologically and economically significant urban river. Since, Rupsha River is being considered as the most important river for fish production. Moreover, various native crops were cultivated near the river bank employing different agricultural chemicals and fertilizers. Still, the bio-magnifications of HMs in fish species from water and sediments of this river may cause harmful effects to humans. Moreover, some studies like Jiménez-Oyola et al. (2021), Islam et al. (2014), Ali et al. (2022), Duodu et al. (2016), Jiang et al. (2013) and Abdelhafez & Li. (2015) investigated metal concentration level along with the ecological, biological and provable human health risk near different river basins. Unfortunately, there is no critical information about the research area’s including all the issues above due to the trace metal pollution has been determined. As a result, the current study focused on several crucial objectives, including determining the trace metals (As, Pb, Cr, and Cd) contamination in the sediment using several geochemical pollution indices and identifying the major sources of the specified metals. Finally, the potential ecological and biological consequences of these metals were assessed to determine the risks to adult’s and children’s health. The novel aspect of this work is that it is the inclusive investigation on riverine sediments pollution by heavy metals causing ecological and human health risks in the south-western area of Bangladesh. Also, the study will provide the relevant information which will help to introduce some actions plans regarding the surrounding environment of the Rupsha River.
The present study was conducted in ten (10) sampling sites of the Rupsha River (Figure 1). Rupsha River is one of the important rivers in the South-west region of Bangladesh. It is formed as a result of the union of the Bhairab and Atrai rivers. Natural disasters like the destruction of spawning ground by ox-bow cutting (U-shaped lake that forms when a wide meander of a river is cut off, creating a free-standing body of water), massive quarry of sands from river bed illegally removed by a section of unscrupulous traders, pollution by industrial waste, unplanned construction sluice gates for irrigation purpose and indiscriminate catching and killing of the brood fishes have posed a serious threat to the biodiversity of Rupsha River.
FIGURE 1. Map of the study area indicates the Rupsha River located south-western part of Bangladesh.
The Rupsha River is a Ganges distributary located in southwestern Bangladesh. It is formed when the Bhairab and Madhumati rivers meet and flow into the Pashur River. The tides have an impact on the entire length of it. It flows beside Khulna and links to the Bay of Bengal at Mongla channel via the Pashur River. It changes its name to Pashur River at Chalna and drains into the Bay of Bengal (Saran et al., 2018). This river has an average width of 486 m, with maximum and minimum widths of 650 and 322 m, respectively (Saran et al., 2018). This river’s bank is home to many fisheries, dockyards, shipyards, and manufacturers. Many companies have sprouted near the Rupsha River. The Bangladeshi Department of Environment has designated this area as the country’s most polluted hotspot (Department of Environment, 2001). Glass and plastic industries, chemical complexes, fish processing plants, steel mills, paper mill complexes, rayon mill complexes, cement factories, paint and dye manufacturing plants, several soaps and detergent factories, and many light industrial units, as well as wastes from brickyards and agricultural runoff, discharge untreated toxic effluent directly. Sewage and municipal wastes and undesirable residues mixed with Fe2O3.2H2O as a result of shipbreaking yard activities are released immediately. These enterprises dump around 4,500 m3/ha of wastewater into the Rupsha River, which flows into the Sundarbans via the Bhairab-Rupsha River system (Department of Environment, 2001; Samad et al., 2015).
Sampling was carried out from August 2018 to February 2019, considering the winter and summer seasons by maintaining standard protocol (U.S Environmental Protection Agency [USEPA], 2001). The present study selected ten stations of the Rupsha River (Figure 1) based upon human activities such as fish farming, the geographical proximity of industrial and urban discharge of effluents, and dredging of the river for sand mining. Composite surface sediment samples were collected from different points of Rupsha River at a depth of 0–5 cm by Ekman dredge and kept in fresh plastic packets. Each sediment sample was taken at each sampling point by mixing randomly collected sediments (3 times) and sixty composite sediment samples were collected. After collection, sediment was placed in a separate cleaned with distilled water airtight polythene bag, sealed, labeled, packed, and transported to the Govt.-MoFL’s Quality Control (QC) Laboratory in Khulna, Bangladesh laboratory testing. The samples were dried in an oven at 45°C for 48 h to gain constant weight (Islam et al., 2015b). The dried mass of each sample was then pulverized to fine powder using a mortar and pestle, sieved through 106 µm apertures and preserved in a plastic vial with the identification mark inside a desiccator (Islam et al., 2015b). Finally, a pellet maker used the homogeneous powder to prepare the pellet using 10-ton pressure (or elemental analysis (Hossain M. B. et al., 2020) using a tool, Specac, United Kingdom.
In a 100 ml beaker, a 2 g sediment sample was placed, and 15 ml of ultrapure HNO3 was added. The contents were cooked for 5 h at 130°C, or only 2–3 ml remained. Materials were passed through Whatman 41 filter paper after digestion (colorless or weak color of content), rinsed with 0.1 M HNO3 solution, and produced up to 100 ml volume for metal analysis (Ali et al., 2021).
An atomic absorption spectrophotometer (Model ZEEnit700P# 150Z7P0110, Analytikjena, Germany) was used to test all samples for As, Pb, Cr, and Cd. All of the procedures were validated in-house following EC567/2002 (Ali et al., 2022). ST 1 summarizes the analytical conditions for measuring heavy metals in samples using AAS. The instrument calibration standards were created using Sigma Aldrich’s (Switzerland) diluting standard (1,000 ppm). Dry weight sediment data were recorded as mg/kg. Throughout the experiment, de-ionized ultrapure (0.05 s) water was used. Before usage, all glassware and containers were carefully cleaned with 20% nitric acid, then rinsed multiple times with De-ionized ultrapure water and oven-dried. DORM-4, a verified reference material, was used to check the analytical method. The National Research Council of Canada prepared and supplied these sediment samples. The certified and observed values were found to be in good agreement. As shown in ST 2, the standard deviations of the averages for the reported certified materials were 0.65–8%, and the percentage recovery was 89–99%.
The selection of background values is critical in the interpretation of geochemical data. As reference baselines, Olivares-Rieumont et al. (2005), Pekey (2006), and Singh et al. (2005) employed the average shale values or average crustal abundance data. The best alternative is to compare quantities between contaminated and uncontaminated sediments that are mineralogically and texturally comparable (Rubio et al., 2000; Sakan et al., 2009). Because no background concentrations for the analyzed Rupsha River sediment from nearby locations were available, the background values in this paper were estimated using the mean heavy metal concentrations in uncontaminated sediments from the study area. The enrichment factor and Geo-accumulation index were employed to determine the degree of heavy metal pollution in Rupsha River sediments.
This study employed consensus-based sediment-quality guidelines (SQGs) to assess the potential danger of heavy metal contamination in the study area’s sediments (Ahmed et al., 2019a). The consensus-based SQGs were created using published sediment-quality criteria that were derived using many methods (Feng et al., 2011). A threshold effect concentration (TEC) below which harmful effects are unlikely to occur and a probable effect concentration (PEC) above which adverse effects are likely to occur more frequently than not are included in these synthesis guidelines (Long et al., 1995; MacDonald et al., 2000).
Geoaccumulation index (Igeo) is a quantitave measurement of the degree of contamination of sediment was introduced by Muller (1969) (Hossain et al., 2019). It is actually based on the background concentration of a particular metal element (Milićević et al., 2017) The following equation defines the geoaccumulation index (Igeo):
Where, Cn denotes the metal concentration in sediment samples and BAn denotes the metal’s geochemical background concentration (n). Due to lithospheric influences, the background matrix correction factor is 1.5. Müller (1981) defined seven classifications in the geoaccumulation index (Table 1).
To assess contamination in the study region, the contamination factor (CF) is used (Wang et al., 2007; Kadhum et al., 2016). PLI was used as a quick and simple means to determine the sediment quality in the research region (Jolly et al., 2021).
Divide the concentration of each metal in sediments by the background level to get the CF value (Hakanson 1980; Ali et al., 2016). For PLI, ‘n’ is the total number of element. CF < 1: Low contamination; 1 ≤ CF < 3: Moderate contamination; 3 ≤ CF < 6: Considerable contamination, CF ≥ 6: Very high pollution (Turekian and Wedepohl 1961; Hakanson 1980; Loska et al., 1997) And PLI = 0: perfect quality, PLI < 1: No pollution; PLI > 1: Polluted.
Hakanson (1980) developed a system for assessing ecological risks in the context of heavy metals pollution. However, the assumption of an aquatic environment’s sensitivity is dependent on its productivity, which may also be assessed using the approach. In addition, the possible ecological risk index (RI) was created to quantify the severity of sediment pollution (Liu et al., 2018). By combining ecological and environmental consequences with toxicological, the index allows for a more accurate assessment of heavy metal contamination’s possible ecological risk factor (Bi et al., 2018). The employed formula is as follows:
Where, RI is the sum of all heavy metal risk aspects in sediment,
Chronic daily intake (CDI) was used to assess the risk of human health from exposure to the trace metals found in the sediment as well as soil (Hu et al., 2017). CDIs can be evaluated for the following routes since humans can uptake metal contents through three methods (inhalation, cutaneous contact, and ingestion) (USEPA 2011; Kusin et al., 2018; Ali et al., 2020a, 2022):
where
Due to varying exposures to an individual’s metal contents, HQ was determined to determine HI as the non-carcinogenic risk for a given element. The ratio of CDI (mg/kg/d) to reference dose (mg/kg/day) was used to compute HQ (USEPA 1989). The HQ and HI assessment equations are as follows (USEPA 2000):
RfD values (mg/kg/day) for various elements, as well as their exposure pathways (USEPA 2011): As (3.00E-04), Pb (3.5E-03), Cr (3.00E-03), Cd (1.00E-03), and Hg (3.00E-04) (JECFA 1993) (3.00E-04). There is no way to mitigate the non-carcinogenic effect, the result of HI > 1 indicates that humans may be exposed to it (Saha et al., 2016; Zhao et al., 2018).
The lifetime carcinogenic risk (CR) exposure for each pathway was calculated using the cancer risk factor (CSF) of the individual metal content (USEPA 2000; Fantke et al., 2012). CSFs of As, Cr, and Cd are 1.5 mg/kg/day (USEPA 2002), 0.5 mg/kg/day (Cancer IAfRo 2011), and 15 mg/kg/day (Cancer IAfRo 2011) respectively. The following equation can be used to calculate CR:
The acceptable lifetime CR limit is between 10−6 and 10−4 (USEPA 2002; FAO 2014; Yin et al., 2015). A value greater than 10−5 indicates that an individual’s cancer progression would be greater than 1 in 100,000 (Ahmed et al., 2019b; Traina et al., 2019).
To determine the data distribution, the Kolmogorov-Smirnov and Shapiro-Wilk tests were used to avoid difficulties like normal/non-normal data dispersion. Correlation analysis was used to evaluate relationships among the variables under consideration, with statistical significance set at p ≤ 0.05. Cluster analysis (CA), an unsupervised pattern recognition technique, reveals the intrinsic structure of a data set without making a prior assumption about the data, allowing the system’s objects to be classified or clusters based on their proximity or similarity (Varol and Sen 2009). Clusters are produced progressively by starting with the most similar pair of items and constructing higher clusters in a step-by-step method in hierarchical clustering, which is the most frequent approach. A “distance” can be expressed by the “difference” between analytical values from both samples, and the Euclidean distance usually gives similarities between two samples (Otto 1998). Using Ward’s technique and Euclidean distances as a measure of similarity, hierarchical agglomerative CA was done on the normalized data set in this work (Ward, 1963). This method evaluates cluster distances using analysis of variance while seeking to minimize the sum of squares of any two clusters that can be constructed at each step. As a way to standardize the linkage distance represented on the y-axis, the linkage distance is reported as Dlink/Dmax, which means the quotient between the linkage distances for a particular case divided by the maximal distance, multiplied by 100 (Simeonov et al., 2000; Shrestha and Kazama 2007; Varol and Sen 2009).
In the summer and winter, heavy metals (As, Cr, Cd, and Pb) were measured in the Rupsha River’s surface sediments and data are shown in Figures 2A,B. Metal concentrations in sediment were higher in the winter than in the summer due to lower water flow, presumably helping accumulate heavy metals in sediment (Bhuyan M. and Bakar M., 2017; Ali et al., 2020a; 2021; Islam M et al., 2020). In Bangladesh, there is no rainfall during the winter season is lower water flow in the river basin and the industrial operation is increased during the winter season. Meanwhile, higher industrial/agricultural activities cause excess levels of metals in river sediment. The average concentration of heavy metals in sediments was in the decreasing order of Cr > Pb > As > Cd for both the summer and winter seasons (Table 2).
TABLE 2. Metal concentrations (mg/kg) in the estuary and coastal sediment from throughout the world are compared.
Heavy metal concentrations and sources are being determined. As is a naturally occurring element in Bangladesh causes poisoning over a short period of exposure. Total arsenic concentrations in uncontaminated nearshore marine and estuarine sediments ranged from 5.0 to 15 mg/kg dry weight. The average concentration of arsenic in deep-sea sediments was found 40 mg/kg (Moore and Ramamoorthy 2012). Arsenic concentrations in river sediments in England and Wales ranged from 7 to 950 mg/kg (Langston 1980). Metal concentrations in sediments from estuaries receiving drainage from metal-mining areas could be much higher. It also has long-term effects on the human body, such as congenital disabilities, reproductive problems, and skin and vascular illnesses, all of which can lead to cancer (Bhuyan M. S. and Bakar M. A., 2017; Bhuyan et al., 2019; Ali et al., 2020a, 2022). The highest concentration of As was found 7.58 mg/kg and the lowest level was observed 3.12 mg/kg, respectively. The maximum concentration (7.58 mg/kg) was identified at site 7 during the winter, while the lowest concentration (3.12 mg/kg) was recorded at site 4 during the summer (Figure 3). At the Feni River Estuary, found 0.85 mg/kg (Table 2). This result is lower than that of the current study. Magesh et al. (2013) observed As levels 7.30, 5.06 and 5.69 mg/kg at Korampallam Creek, Punnakayal Estuary, and Kallar Estuary. The findings were likewise somewhat similar to those of the current study. Ali et al. (2018) found almost identical results in the Pasur River, Bangladesh (Table 2).
FIGURE 3. Contamination factor (CF) and pollution load index (PLI) in the sediment of the Rupsha River.
Chromium (Cr) compounds are potent oxidizers that are irritating and corrosive, making them appear more dangerous (Rahman et al., 2012). In our study, Cr concentrations ranged from 18.67–67.12 mg/kg, with a mean value of 43.2 mg/kg. During the winter, the maximum level of 67.12 mg/kg was reported at site 5 (Figure 2A). During the summer, a minimum of 18.67 mg/kg was recorded at site 2 (Figure 2B). The metal concentration levels at all of the sites were higher than the WHO (2004), USEPA (1999, 2004), and Ayers and Westcot. (1985) recommended levels (Table 2). At the Kutubdia Channel, Hossain M. B. et al. (2020) found a much lower level (11.0 mg/kg) (Table 2). In the Feni River Estuary, Karnaphuli River and Bhairab River, Islam et al. (2018), Ali et al. (2016, 2022), Islam et al. (2013), and Mamun et al. (2013) found lower levels of As. At the Yangtze River Estuary in China, Fan et al. (2020) found 34.4 mg/kg. At the Kallar Estuary, Korampallam Creek, and Punnakayal Estuary, Magesh et al. (2013) found 10.12 mg/kg, 26.85 mg/kg, and 9.34 mg/kg, respectively. The lower level of Cr in the Vellar estuary, India, was found by Ramanathan et al. (1999) (Table 2).
The average Cd concentration in the summer was 1.45 mg/kg, whereas 2.18 mg/kg in the winter (Figures 2A,B). The average concentration of Cd was 1.8 mg/kg on. The current findings are noted as exceeding the USEPA’s (1999) recommended limit. Cd concentrations were high throughout the winter, which could be linked to variations in water capacity in the study area, where restricted water flow caused Cd precipitation in sediment, elevating its concentration (Ali et al., 2016). In the Pasur River and Bhairab River, Ali et al. (2018) and Ali et al. (2022) found 0.39–3.17 and 1.41–1.92 mg/kg Cd respectively (Table 2). In the Karnaphuli River, Islam et al. (2013) and Siddique and Aktar (2012) found 0.24 mg/kg and 0.43 mg/kg Cd, respectively. Cd levels in the Passur river varied from 0.80 to 2.70 mg/kg, according to Rahman et al. (2011). In the Yangtze River Estuary, China, Fan et al. (2020) and Wang et al. (2020) found decreased levels of Cd. In Tokyo Bay, Japan, Fukushima et al. (1992) found 0.996 mg/kg Cd. Magesh et al. (2013) found greater levels of Cd than the current findings in the Kallar Estuary, Korampallam Creek, and Punnakayal Estuary. In India, Ramanathan et al. (1999) found high levels of Cd in the Vellar estuary, the Pichavaram mangrove, and the Coleroon Estuary (Table 2).
Lead (Pb) is a non-essential metal that causes neurotoxicity and nephrotoxicity in humans (Garca-Lestón et al., 2010). The mean Pb concentration was 29.21 mg/kg during the winter, with the maximum value being 48.52 mg/kg at site 3 (Figure 2A). The lowest concentration of 12.69 mg/kg was recorded during the summer season at site 7 (Figure 2B). In the Kutubdia Channel, Bangladesh, Hossain M. S. et al. (2020) found 22.0 mg/kg Pb. About 23.82 mg/kg, 6.47 mg/kg, 4.96 mg/kg, 15.49 mg/kg, 26.7 mg/kg, 26.42 mg/kg, and 3.12 mg/kg were found in the Bhairab River, Feni River Estuary, Karnaphuli River, Matamuhuri River, and Bakkhali River, respectively, by Ali et al. (2021), Islam et al. (2018), Islam et al. (2013), Mamun et al. (2013), Siddique and Aktar (2012), Ashraful et al. (2009). These results were lower than those found in the current study. In the Yangtze River Estuary, China, Fan et al. (2020) and Wang et al. (2020) reported decreased levels of Cd (Table 2). Magesh et al. (2013) found reduced Pb levels in the Kallar and Punnakayal Estuaries in India. The value of Cd observed in this study was higher than that found by Ramanathan et al. (1999) in India’s Vellar estuary, Pichavaram mangrove, and Coleroon Estuary. Bothner et al. (1998) and Ali et al. (2016) found a greater concentration of Cd in Boston Harbor and Karnaphuli River than in the current research (Table 2).
The ecotoxicological sensibility of the contaminants and the identification of metal components in an aquatic environment were assessed using sediment quality guidelines (SQGs) (Kumwimba et al., 2016). The SQGs were consensus-based assessments expressed in TEC, PEC, and ASV (Table 2). Cr metal had the highest TEC (43.40) while Pb had the highest level of PEC (128). The evaluation method, protection level connected to sediment characteristics such as TOC, pH, acid-volatile sulfide (AVS), and screening of important environmental elements are all aspects that contribute to the difference of findings in different sites (Chen et al., 2005; Ahmed et al., 2019a). These characteristics can make the usage of SQGs and their development more difficult (Gao et al., 2015). In our study area, 100% of the samples were below TEC limit for As in both summer and winter seasons. However, it was 60, 80, and 10% in summer and 60% 100%, and 30% in winter season for Cr, Cd, and Pb respectively, remained in the TEC-PEC range. Hence, the findings indicated tha the research area could be occasionally and directly influenced by anthropogenic activity. Furthermore, SQG evaluations revealed that pollutants in river sediment constitute major ecological harm to sediment-dwelling organisms. In comparison, our findings were similar to those of Wu et al. (2014). They observed that the sediment-dwelling organisms in the Baihua and Hongfeng reservoirs posed a moderate toxicological risk due to heavy metals exposure. However, to correctly assess the current condition of trace metal contamination, numerous developed geochemical indices must be evaluated (Siddique et al., 2020).
Igeo is a critical ecological index for distinguishing between natural and human-caused metal sources and determining the level of contamination in sediment samples. The contamination of the HMs in the research region was delineated in the following order: Cd > Pb > Cr > As, based on average Igeo values. Cd (1.89), had the highest Igeo value, while Cr had the lowest (-1.96). Cd was found to be “moderately contaminated” at the sample site, while Pb, As, and Cr was found to be “least contaminated” (Table 3). Furthermore, a minor difference was observed among the metals in sampling sites due to the change of metal concentrations. All of the Igeo values of As, Pb, Cr, and Cd in the sampling area were found to be less contaminated, according to the findings. However, Ke et al. (2017) found that the Igeo values in the Liaohe River protected area were classified as highly contaminated. In addition, Mohiuddin et al. (2011) conducted a study on the Turag river, finding that Igeo values for Pb and Cu remained in the unpolluted category. The main explanation for this was that the attribution of metals in the Liaohe and Turag rivers was much higher than in our study sites.
The CF values of the metals are shown in Figure 3, and they can be sorted in the following order: As varies from 0.33 to 0.45 (mean 0.39); Pb ranges from 1.34 to 1.59 (mean 1.47); Cr varies from 0.44 to 0.52 (mean 0.48), and Cd ranges from 4.75 to 7.25 (mean 6.0). The CF value of Cd indicated that there was significant contamination. According to the CF value, sediments of the study river were moderately polluted with Pb. CF values of As and Cr were found to be less than 1, indicating low contamination. The CF value was higher in the winter than in the summer. Islam et al. (2015a) and Ali et al. (2016, 2022) found similar results in a Bangladeshi urban river. According to the research, domestic wastewater drainage, industrial effluents, municipal runoffs, and atmospheric deposition are the main sources of the observed higher levels of metals in the surface sediment (Ali et al., 2020a). Hassan et al. (2015) conducted a study in the Meghna river, and their findings were identical to those of the current study.
The PLI can provide information about sediment quality to the general public. It also provides decision-makers with critical information on the state of pollution in the research area (Suresh et al., 2012). The pollution load index (PLI) values for metals in sediments are reported in Figure 3 (inside). If the PLI score is larger than one, the tested region is entirely polluted, according to. PLI values ranged from 1.01 to 1.42 at all sampling sites in the winter, indicating that the sediment in the study river was polluted (PLI > 1). Except at sites 8, 9, and 10, the PLI fell below 1 in the summer. In all sampling locations, PLI values are more than unity, owing to the impact of municipal and other industrial activity in these areas.
The PERI was assessed using a monomial ecological risk model and specific features that highlighted the cumulative ecological-toxicological effects of numerous contaminants in the aquatic environment (Karydas et al., 2015). In both the summer and winter seasons, Cd’s monomial potential ecological risk assessment was determined at a higher level. At all of the sites, the PERI value for Cd exceeded the allowed limit. The PERI score ranged from 160 to 320, indicating a significant risk posed by Cd in the study area (Figure 4). Pb and Cr were determined to be in a low-risk status. Sites were organized in descending order based on the RI values for all cumulative metal concentrations: S4 > S2> S5 >S6 > S3 > S1 > S9 > S8 > S7 > S10 for the summer season, and S5 > S4 > S1 > S2 > S6 > S9 > S3 > S8 > S7 > S10 for the winter season. During the winter season, the highest value of RI (333.92) was recorded at site 10. During the summer season, the lowest reading of 102.45 was observed at site 4 (Figure 4). The majority of the research region was found to be at a moderate risk to the aquatic ecology. Because industry and urbanization are quickly developing in our study area, more environmental elements should be carefully monitored to assess sediment quality and investigated new sources of metal content (Ali et al., 2022).
The local people surrounding the river basin were directly involved in various seasonal growing crops, therefore the human health risk was explored. The majority of the residents exploited the riverbank sediment for their agricultural plots. Sometimes river bed sediments are brought to the house for preparing household stuff. The local farmers also applied the river bed sediment to the agriculture fields to supplement organic matter. As a result, they have to be in direct touch with the sediment to grow seasonal vegetables. One of the significant characterizations and critical tools for understanding unfavorable human health impacts and environmental hazard exposures is risk assessment (Chonokhuu et al., 2019; El-Alfy et al., 2020). The following processes were followed to assess the danger to human health for three significant pathways:
The metals’ CDIs from the investigated sites were analyzed for adults and children, as shown in Table 4. CDIs were higher in children than in adults, according to the data, due to pathways that were grouped in decreasing order: ingestion > dermal > inhalation. For the targeted groups of people exposed through the exposure pathways, As had a higher CDI value than the other metals (Table 4). Through the dermal method, a child’s concentration was observed to be 5.56E-06, while an adult’s concentration was determined to be 8.49E-07. As consumption in children (3.31E-05) is higher than in adults (7.09E-06). Through all of the processes, Pb and Cr levels were shown to be higher in children than in adults. The intake of Cd was lower than the other metals in both children and adults (Table 4). Ali et al. (2022) found similar results where the CDIs were higher in children than adults.
The average CDI values were used to assess the non-carcinogenic risk. In both age groups, Table 4 showed the maximum HQ value for the As metal content via the ingestion method (adult: 2.36E-02, children: 1.10E-01). In addition, metal As had a greater HQ attribution via the inhalation method, whereas As demonstrated a leadership position for all types of persons (Table 4). The overall results of HQ for all the pathways of the nearby local community were observed in the following order: As > Cr > Pb > Cd. The measurement of HQ was used to assess HI. Children were found to be more sensitive than adults as a result of the total HI of five components (Table 4). The overall results of 1 suggested that there was no substantial non-carcinogenic risk effect in the research area. A similar conclusion was made in the Yangtze River (Hu et al., 2017), where residents were safe from exceeding the worrying level (HI < 1). Ahmed et al. (2020) and Ali et al. (2022) found similar results in Bangladesh’s Gomti river and Bhairab River respectively.
Because the USEPA did not give a carcinogen slope factor for Pb, the carcinogenic risk (CR) for the other elements, As, Pb, and Cr was approximated. Table 1 showed the results of the CR. For both adults and children, ingestion exposure was the most common among the three exposure routes. The CRs of different metals for different age groups, for example, may differ dramatically (Table 4). Children were shown to have higher CR values than adults (Table 2). Furthermore, children were exposed to higher CR in terms of As (5.80E-05) and Cd (1.74E-04) with a stronger effect than any other element through the ingestion channel. All As, Cr, and Cd risk values were found to be above 1 × 10–5, indicating that the explored research region was not devoid of the detrimental effects of CR in both children and adults (Table 4). In contrast, El-Alfy et al. (2020) observed that consuming metals from the Burullus Lake silt exposed children rather than adults to the carcinogenic risk.
Results from Kolmogorov-Smirnov and Shapiro-Wilk revealed that elements were generally distributed in the sediments of the study river. Other statistical analyses were concerned to provide some prospects which delivered some correlated possibilities using CM, PCA, and cluster analysis (CA).
The interrelationship between the metals was depicted in the correlation matrix. Pb and Cr (r = 0.148) and Cd and Cr (r = 0.135) had a weak positive connection in the summer. Cr Vs. was identified to have a moderately negative relationship (−0.513). Weak negative associations between Pb and Cd (r = −0.298) and Pb and As (r = −0.158) were investigated (Table 2). Pb Vs. Cd (r = 0.357), Cd Vs. Cr (r = 0.024), and Cd Vs. As (r = 0.230) all showed a positive weak linear relationship in the winter. Between Cr Vs. As (r = −0.274) and Pb Vs. As (r = −0.265), there was a negative linear connection (Table 5).
PCA was used to analyze the clustering behavior of several features qualitatively. Figure 5 shows the PCA findings for each factor with an eigenvalue greater than 1, a variance of 100%, and the cumulative variance. With a total variance of 89.90%, the PCA investigated the three grouping components. Due to the significant loadings of As, PC1 contributed 38.72% of the total variation (0.718). PC1 was shown to come from both geogenic and anthropogenic sources, such as manufacturing companies and refineries, according to the findings (Shikazono et al., 2012). PC2 accounted for 27.37% of the entire variance in the high Cd loading (0.816), which was attributed to battery manufacturing and other industrial concerns.
A comparable set of sites was displayed in one group of the cluster, while a different group of sites was displayed in another group to pinpoint specific contamination locations (Yang et al., 2009; Sundaray et al., 2011). The cluster was explored using Ward Linkage and Euclidean distance. The dendrogram produced by the cluster analysis revealed a major cluster at (Dlink/Dmax)×100 < 30 and four prominent clusters: cluster 1, cluster 2, cluster 3, and cluster 4. Cluster 1 was possessed by S1, S7, and S2; cluster 2 was possessed by S4, S6, S9, and S8; while cluster 3 was possessed by S10. Finally, S5 was found in cluster 3. (Figure 6A). A similar strategy was used in the cluster analysis at (Dlink/Dmax)× 100 < 1 to determine the relationship between the studied metals and possible sources (Chung et al., 2011). Cluster 1 included As and Cd, while Cluster 2 comprised Cr and Pb (Figure 6B).
FIGURE 6. (A) Hierarchical cluster (dendrogram) of heavy metals in terms of sites. (B) Hierarchical cluster (dendrogram) of heavy metals in terms of metals.
Heavy metals such as As, Cr, Cd, and Pb were examined in the Rupsha River sediment to estimate the contamination level as these contents are now available in the sediment throughout the world. We observed that Cr exhibited the highest concentration followed by Pb, As, and Cd. In the current study, Cd exceeded various standard limits among the metals examined. SQGs revealed that metals like Cd, Cr, and Pb had negative biological as well as ecological effects on the sediment-dwelling organisms. After measuring the river’s sediment quality, we can conclude that according to Igeo, the river’s sediment was moderately contaminated for Cd. CFs revealed that the study area was polluted especially for Cd whilst PLI indicated that investigated area was polluted in the winter season as it was > 1. The study region was located within the moderate-risk line, which can adversely influence the river’s aquatic creatures, according to the possible ecological risk index and the risk index along with the sites. Multivariate analysis revealed that the metals were attributed to the river by diverse anthropogenic activities, largely from industries and municipal trash. Nonetheless, constant monitoring should be conducted, and appropriate rules should be put in place to limit any invasive anthropogenic activity across the river. This study suggested that the chemical fractionation of sediments should be considered to provide a more accurate appraisal of trace metals’ risk and their distribution pathways in the riverine ecosystems.
The original contributions presented in the study are included in the article/Supplementary Material, further inquiries can be directed to the corresponding authors.
All authors listed have made a substantial, direct, and intellectual contribution to the work and approved it for publication.
This manuscript is part of the master’s thesis of KK from the Dept. of Aquaculture, Faculty of Fisheries, Patuakhali Science and Technology University, Bangladesh.
We are thankful to Muhammad Abdur Razzak, Dept. of Aquaculture, and other faculty members. The authors would like to express their gratefulness and sincerest thanks to the authority and staff members of the Quality Control (QC) Laboratory, Khulna, Bangladesh, for providing laboratory facilities.
The authors declare that the research was conducted in the absence of any commercial or financial relationships that could be construed as a potential conflict of interest.
All claims expressed in this article are solely those of the authors and do not necessarily represent those of their affiliated organizations, or those of the publisher, the editors and the reviewers. Any product that may be evaluated in this article, or claim that may be made by its manufacturer, is not guaranteed or endorsed by the publisher.
The Supplementary Material for this article can be found online at: https://www.frontiersin.org/articles/10.3389/fenvs.2022.778544/full#supplementary-material
Abdelhafez, A. A., and Li, J. (2015). Environmental monitoring of heavy metal status and human health risk assessment in the agricultural soils of the Jinxi River area, China. Hum. Ecol. Risk Assess. An Int. J. 21 (4), 952–971. doi:10.1080/10807039.2014.947851
Agarwal, A., Singh, R. D., Mishra, S. K., and Bhunya, P. K. (2005). ANN-based sediment yield river basin models for Vamsadhara (India). Water sa. 31 (1), 95–100. doi:10.4314/wsa.v31i1.5125
Ahmed, A. S., Rahman, M., Sultana, S., Babu, S. O. F., and Sarker, M. S. I. (2019a). Bioaccumulation and heavy metal concentration in tissues of some commercial fishes from the Meghna River Estuary in Bangladesh and human health implications. Mar. Pollut. Bull. 145, 436–447. doi:10.1016/j.marpolbul.2019.06.035
Ahmed, A. S. S., Hossain, M. B., Semme, S. A., Babu, S. M. O. F., Hossain, K., Moniruzzaman, M., et al. (2020). Accumulation of trace elements in selected fish and shellfish species from the largest natural carp fish breeding basin in asia: A probabilistic human health risk implication. Environ. Sci. Pollut. Res. 27 (30), 37852–37865. doi:10.1007/s11356-020-09766-1
Ahmed, A. S., Sultana, S., Habib, A., Ullah, H., Musa, N., Hossain, M. B., et al. (2019b). Bioaccumulation of heavy metals in some commercially important fishes from a tropical river estuary suggests higher potential health risk in children than adults. Plos one 14 (10), e0219336. doi:10.1371/journal.pone.0219336
Ali, M. M., Ali, M. L., Islam, M. S., and Rahman, M. Z. (2018). Assessment of toxic metals in water and sediment of Pasur River in Bangladesh. Water Sci. Technol. 77 (5), 1418–1430. doi:10.2166/wst.2018.016
Ali, M. M., Ali, M. L., Islam, M. S., and Rahman, M. Z. (2016). Preliminary assessment of heavy metals in water and sediment of Karnaphuli River, Bangladesh. Environ. Nanotechnol. Monit. Manag. 5, 27–35. doi:10.1016/j.enmm.2016.01.002
Ali, M. M., Ali, M. L., Proshad, R., Islam, S., Rahman, Z., Kormoker, T., et al. (2020b). Assessment of trace elements in the demersal fishes of a coastal river in Bangladesh: A public health concern. Thalassas. 36 (2), 641–655. doi:10.1007/s41208-020-00227-7
Ali, M. M., Ali, M. L., Proshad, R., Islam, S., Rahman, Z., Tusher, T. R., et al. (2020a). Heavy metal concentrations in commercially valuable fishes with health hazard inference from Karnaphuli river, Bangladesh. Hum. Ecol. risk Assess. Int. J. 26 (10), 2646–2662. doi:10.1080/10807039.2019.1676635
Ali, M. M., Rahman, S., Islam, M. S., Rakib, M. R. J., Hossen, S., Rahman, M. Z., et al. (2022). Distribution of heavy metals in water and sediment of an urban river in a developing country: A probabilistic risk assessment. Int. J. Sediment Res. 37 (2), 173–187. doi:10.1016/j.ijsrc.2021.09.002
Ashraful, M. A. K., Assim, Z. B., and Ismail, N. (2009). Monitoring and assessment of heavy metals levels in littoral sediments from the north eastern part of the Bay of Bengal coast, Bangladesh. J. Industrial Pollut. Control 25 (2), 105–111.
Ayers, R. S., and Westcot, D. W. (1985). Water quality for agriculture. Rome: FAO. Irrigation and Drainage Paper No. 29: Rev.1.
Babu, S. M., Hossain, M. B., Rahman, M. S., Rahman, M., Ahmed, A. S., Hasan, M., et al. (2021). Phytoremediation of toxic metals: A sustainable green solution for clean environment. Appl. Sci. 11 (21), 10348. doi:10.3390/app112110348
Bai, J., Cui, B., Chen, B., Zhang, K., Deng, W., Gao, H., et al. (2011). Spatial distribution and ecological risk assessment of heavy metals in surface sediments from a typical plateau lake wetland, China. Ecol. Model. 222 (2), 301–306. doi:10.1016/j.ecolmodel.2009.12.002
Bhavsar, S. P., Gewurtz, S. B., Helm, P. A., Labencki, T. L., Marvin, C. H., Fletcher, R., et al. (2010). Estimating sediment quality thresholds to prevent restrictions on fish consumption: Application to polychlorinated biphenyls and dioxins–furans in the Canadian Great Lakes. Integr. Environ. Assess. Manag. 6 (4), 641–652. doi:10.1002/ieam.102
Bhuyan, M., and Bakar, M. (2017b). Assessment of water quality in Halda River (the Major carp breeding ground) of Bangladesh. Pollution 3 (3), 429–441. doi:10.7508/pj.2017.03
Bhuyan, M. S., Bakar, M. A., Akhtar, A., Hossain, M. B., Ali, M. M., Islam, M. S., et al. (2017). Heavy metal contamination in surface water and sediment of the Meghna River, Bangladesh. Environ. Nanotechnol. Monit. Manag. 8, 273–279. doi:10.1016/j.enmm.2017.10.003
Bhuyan, M. S., Bakar, M. A., Rashed-Un-Nabi, M., Senapathi, V., Chung, S. Y., Islam, M. S., et al. (2019). Monitoring and assessment of heavy metal contamination in surface water and sediment of the Old Brahmaputra River, Bangladesh. Appl. Water Sci. 9 (5), 125. doi:10.1007/s13201-019-1004-y
Bhuyan, M. S., and Bakar, M. A. (2017a). Seasonal variation of heavy metals in water and sediments in the Halda River, Chittagong, Bangladesh. Environ. Sci. Pollut. Res. 24 (35), 27587–27600. doi:10.1007/s11356-017-0204-y
Bi, B., Liu, X., Guo, X., and Lu, S. (2018). Occurrence and risk assessment of heavy metals in water, sediment, and fish from Dongting Lake, China. Environ. Sci. Pollut. Res. 25 (34), 34076–34090. doi:10.1007/s11356-018-3329-8
Boström, K., and Valdes, S. (1969). Arsenic in ocean floors. Lithos 2 (4), 351–360. doi:10.1016/s0024-4937(69)80041-1
Bothner, M. H., Ten Brink, M. B., and Manheim, F. T. (1998). Metal concentrations in surface sediments of Boston Harbor-changes with time. Mar. Environ. Res. 45 (2), 127–155. doi:10.1016/s0141-1136(97)00027-5
Cancer IAfRo (2011). Agents classified by the IARC monographs. IARC monographs on the evaluation of carcinogenic risks to humans. Lyon, France: IARC).
Chabukdhara, M., and Nema, A. K. (2012). Assessment of heavy metal contamination in hindon river Sediments: A chemometric and geochemical approach. Chemosphere 87 (8), 945–953. doi:10.1016/j.chemosphere.2012.01.055
Chen, Y. Z., Yang, H., Zhang, Z. K., and Qin, M. (2005). The difference and cause analyses of freshwater sediment quality guidelines. J. Lake Sci. 3.
Chonokhuu, S., Batbold, C., Chuluunpurev, B., Battsengel, E., Dorjsuren, B., Byambaa, B., et al. (2019). Contamination and health risk assessment of heavy metals in the soil of major cities in Mongolia. Int. J. Environ. Res. Public Health 16 (14), 2552. doi:10.3390/ijerph16142552
Chung, C. Y., Chen, J. J., Lee, C. G., Chiu, C. Y., Lai, W. L., Liao, S. W., et al. (2011). Integrated estuary management for diffused sediment pollution in Dapeng Bay and neighboring rivers (Taiwan). Environ. Monit. Assess. 173 (1-4), 499–517. doi:10.1007/s10661-010-1401-z
Covelli, S., and Fontolan, G. (1997). Application of a normalization procedure in determining regional geochemical baselines. Environ. Geol. 30 (1-2), 34–45. doi:10.1007/s002540050130
Department of Environment (DoE) (2001). Water quality data of rivers buriganga, Meghna, balu, shitalakhya and jamuna. Dhaka, Bangladesh: Department of Environment.
Duodu, G. O., Goonetilleke, A., and Ayoko, G. A. (2016). Comparison of pollution indices for the assessment of heavy metal in Brisbane River sediment. Environ. Pollut. 219, 1077–1091. doi:10.1016/j.envpol.2016.09.008
El-Alfy, M. A., Darwish, D. H., and El-Amier, Y. A. (2020). Land use Land cover of the Burullus Lake shoreline (Egypt) and health risk assessment of metal-contaminated sediments. Hum. Ecol. Risk Assess. An Int. J. 27, 898–920. doi:10.1080/10807039.2020.1786667
Fan, H., Chen, S., Li, Z., Liu, P., Xu, C., Yang, X., et al. (2020). Assessment of heavy metals in water, sediment and shellfish organisms in typical areas of the Yangtze River Estuary, China. Mar. Pollut. Bull. 151, 110864. doi:10.1016/j.marpolbul.2019.110864
Fantke, P., Friedrich, R., and Jolliet, O. (2012). Health impact and damage cost assessment of pesticides in Europe. Environ. Int. 49, 9–17. doi:10.1016/j.envint.2012.08.001
FAO (2014). The state of the world fisheries and aquaculture. Rome, Italy: FAO Fisheries and Aquaculture Department.
Feng, H., Jiang, H., Gao, W., Weinstein, M. P., Zhang, Q., Zhang, W., et al. (2011). Metal contamination in sediments of the Western Bohai Bay and adjacent estuaries, China. J. Environ. Manag. 92 (4), 1185–1197. doi:10.1016/j.jenvman.2010.11.020
Fu, F., and Wang, Q. (2011). Removal of heavy metal ions from wastewaters: A review. J. Environ. Manag. 92 (3), 407–418. doi:10.1016/j.jenvman.2010.11.011
Fukushima, K., Saino, T., and Kodama, Y. (1992). Trace metal contamination in Tokyo Bay, Japan. Sci. total Environ. 125, 373–389. doi:10.1016/0048-9697(92)90402-e
Ganugapenta, S., Nadimikeri, J., Chinnapolla, S. R. R. B., Ballari, L., Madiga, R., Nirmala, K., et al. (2018). Assessment of heavy metal pollution from the sediment of Tupilipalem Coast, southeast coast of India. Int. J. Sediment Res. 33 (3), 294–302. doi:10.1016/j.ijsrc.2018.02.004
Gao, L., Gao, B., Wei, X., Zhou, H., Xu, D., Wang, Y., et al. (2015). Assessment of metal toxicity and development of sediment quality guidelines using the equilibrium partitioning model for the Three Gorges Reservoir, China. Environ. Sci. Pollut. Res. 22 (22), 17577–17585. doi:10.1007/s11356-015-4959-8
García-Lestón, J., Méndez, J., Pásaro, E., and Laffon, B. (2010). Genotoxic effects of lead: An updated review. Environ. Int. 36 (6), 623–636. doi:10.1016/j.envint.2010.04.011
Hakanson, L. (1980). An ecological risk index for aquatic pollution control. A sedimentological approach. Water Res. 14 (8), 975–1001. doi:10.1016/0043-1354(80)90143-8
Han, L., Gao, B., Hao, H., Zhou, H., Lu, J., Sun, K., et al. (2018). Lead contamination in sediments in the past 20 years: A challenge for China. Sci. Total Environ. 640, 746–756. doi:10.1016/j.scitotenv.2018.05.330
Hassan, M., Rahman, M. A. T., Saha, B., and Kamal, A. K. I. (2015). Status of heavy metals in water and sediment of the Meghna River, Bangladesh. Am. J. Environ. Sci. 11 (6), 427–439. doi:10.3844/ajessp.2015.427.439
Hossain, M. B., Habib, S. B., Hossain, M. S., Jolly, Y. N., Kamal, A. H. M., Idris, M. H., et al. (2020a). Data set on trace metals in surface sediment and water from a sub-tropical estuarine system, Bay of Bengal, Bangladesh. Data brief 31, 105911. doi:10.1016/j.dib.2020.105911
Hossain, M. B., Semme, S. A., Ahmed, A. S. S., Hossain, M. K., Porag, G. S., Parvin, A., et al. (2021). Contamination levels and ecological risk of heavy metals in sediments from the tidal river Halda, Bangladesh. Arab. J. Geosci. 14 (3), 158. doi:10.1007/s12517-021-06477-w
Hossain, M. B., Shanta, T. B., Ahmed, A. S., Hossain, M. K., and Semme, S. A. (2019). Baseline study of heavy metal contamination in the Sangu River estuary, Chattogram, Bangladesh. Mar. Pollut. Bull. 140, 255–261. doi:10.1016/j.marpolbul.2019.01.058
Hossain, M. S., Ahmed, M. K., Sarker, S., and Rahman, M. S. (2020b). Seasonal variations of trace metals from water and sediment samples in the northern Bay of Bengal. Ecotoxicol. Environ. Saf. 193, 110347. doi:10.1016/j.ecoenv.2020.110347
Hu, B., Jia, X., Hu, J., Xu, D., Xia, F., Li, Y., et al. (2017). Assessment of heavy metal pollution and health risks in the soil-plant-human system in the Yangtze River Delta, China. Int. J. Environ. Res. Public Health 14 (9), 1042. doi:10.3390/ijerph14091042
Ingersoll, C. G., Haverland, P. S., Brunson, E. L., Canfield, T. J., Dwyer, F. J., Henke, C. E., et al. (1996). Calculation and evaluation of sediment effect concentrations for the amphipod Hyalella azteca and the midge Chironomus riparius. J. Gt. Lakes. Res. 22 (3), 602–623. doi:10.1016/s0380-1330(96)70984-x
Islam, A. R. M. T., Hasanuzzaman, M., Islam, H. M. T., Mia, M. U., Khan, R., Habib, M. A., et al. (2020). Quantifying source apportionment, Co‐occurrence, and ecotoxicological risk of metals from upstream, lower midstream, and downstream river segments, Bangladesh. Environ. Toxicol. Chem. 39 (10), 2041–2054. doi:10.1002/etc.4814
Islam, F., Rahman, M., Khan, S. S. A., Ahmed, B., Bakar, A., and Halder, M. (2013). Heavy metals in water, sediment and some fishes of Karnofuly River, Bangladesh. Pollut. Res. 32 (4), 715–721.
Islam, M. A., Das, B., Quraishi, S. B., Khan, R., Naher, K., Hossain, S. M., et al. (2020). Heavy metal contamination and ecological risk assessment in water and sediments of the halda river, Bangladesh: A natural fish breeding ground. Mar. Pollut. Bull. 160, 111649. doi:10.1016/j.marpolbul.2020.111649
Islam, M. M., Rahman, S. L., Ahmed, S. U., and Haque, M. K. I. (2014). Biochemical characteristics and accumulation of heavy metals in fishes, water and sediments of the river Buriganga and Shitalakhya of Bangladesh. J. Asian Sci. Res. 4 (6), 270.
Islam, M. S., Ahmed, M. K., Habibullah-Al-Mamun, M., and Hoque, M. F. (2015a). Preliminary assessment of heavy metal contamination in surface sediments from a river in Bangladesh. Environ. Earth Sci. 73 (4), 1837–1848. doi:10.1007/s12665-014-3538-5
Islam, M. S., Ahmed, M. K., Habibullah-Al-Mamun, M., Raknuzzaman, M., Ali, M. M., Eaton, D. W., et al. (2016). Health risk assessment due to heavy metal exposure from commonly consumed fish and vegetables. Environ. Syst. Decis. 36 (3), 253–265. doi:10.1007/s10669-016-9592-7
Islam, M. S., Ahmed, M. K., Raknuzzaman, M., Habibullah-Al-Mamun, M., and Islam, M. K. (2015b). Heavy metal pollution in surface water and sediment: A preliminary assessment of an urban river in a developing country. Ecol. Indic. 48, 282–291. doi:10.1016/j.ecolind.2014.08.016
Islam, M. S., Hossain, M. B., Matin, A., and Sarker, M. S. I. (2018). Assessment of heavy metal pollution, distribution and source apportionment in the sediment from Feni River estuary, Bangladesh. Chemosphere 202, 25–32. doi:10.1016/j.chemosphere.2018.03.077
Islam, M. S., Idris, A. M., Islam, A. R. M. T., Ali, M. M., and Rakib, M. R. J. (2021). Hydrological distribution of physicochemical parameters and heavy metals in surface water and their ecotoxicological implications in the Bay of Bengal coast of Bangladesh. Environ. Sci. Polluttion 28 (48), 68585–68599. doi:10.1007/s11356-021-15353-9
Jiang, M., Zeng, G., Zhang, C., Ma, X., Chen, M., Zhang, J., et al. (2013). Assessment of heavy metal contamination in the surrounding soils and surface sediments in Xiawangang River, Qingshuitang District. PloS one 8 (8), e71176. doi:10.1371/journal.pone.0071176
Jiménez-Oyola, S., García-Martínez, M. J., Ortega, M. F., Chavez, E., Romero, P., García-Garizabal, I., et al. (2021). Ecological and probabilistic human health risk assessment of heavy metal (loid) s in river sediments affected by mining activities in Ecuador. Environ. Geochem. Health 43, 4459–4474. doi:10.1007/s10653-021-00935-w
Jolly, Y. N., Rakib, M. R. J., Islam, M. S., Akter, S., Idris, A. M., Phoungthong, K., et al. (2021). Potential toxic elements in sediment and fishes of an important fish breeding river in Bangladesh: A preliminary study for ecological and health risks assessment. Toxin Rev., 1–14. doi:10.1080/15569543.2021.1965624
Kadhum, S. A., Ishak, M. Y., and Zulkifli, S. Z. (2016). Evaluation and assessment of baseline metal contamination in surface sediments from the Bernam River, Malaysia. Environ. Sci. Pollut. Res. 23 (7), 6312–6321. doi:10.1007/s11356-015-5853-0
Kahal, A., El-Sorogy, A. S., Qaysi, S., Almadani, S., Kassem, O. M., Al-Dossari, A., et al. (2020). Contamination and ecological risk assessment of the Red Sea coastal sediments, southwest Saudi Arabia. Mar. Pollut. Bull. 154, 111125. doi:10.1016/j.marpolbul.2020.111125
Karydas, C. G., Tzoraki, O., and Panagos, P. (2015). A new spatiotemporal risk index for heavy metals: Application in Cyprus. Water 7 (8), 4323–4342. doi:10.3390/w7084323
Ke, X., Gui, S., Huang, H., Zhang, H., Wang, C., Guo, W., et al. (2017). Ecological risk assessment and source identification for heavy metals in surface sediment from the Liaohe River protected area, China. Chemosphere 175, 473–481. doi:10.1016/j.chemosphere.2017.02.029
Khan, R., Islam, M. S., Tareq, A. R. M., Naher, K., Islam, A. R. M. T., Habib, M. A., et al. (2020). Distribution, sources and ecological risk of trace elements and polycyclic aromatic hydrocarbons in sediments from a polluted urban river in central Bangladesh. Environ. Nanotechnol. Monit. Manag. 14, 100318. doi:10.1016/j.enmm.2020.100318
Kumar, S., Islam, A. R. M. T., Hasanuzzaman, M., Roquia, S., Khan, R., Islam, M. S., et al. (2021). Preliminary assessment of heavy metals in surface water and sediment in Nakuvadra-Rakiraki River, Fiji using indexical and chemometric approaches. J. Environ. Manag. 298, 113517. doi:10.1016/j.jenvman.2021.113517
Kumwimba, M. N., Zhu, B., Wang, T., and Muyembe, D. K. (2016). Distribution and risk assessment of metals and arsenic contamination in man-made ditch sediments with different land use types. Environ. Sci. Pollut. Res. 23 (24), 24808–24823. doi:10.1007/s11356-016-7690-1
Kusin, F. M., Azani, N. N. M., Hasan, S. N. M., and Sulong, N. A. (2018). Distribution of heavy metals and metalloid in surface sediments of heavily-mined area for bauxite ore in Pengerang, Malaysia and associated risk assessment. Catena 165, 454–464. doi:10.1016/j.catena.2018.02.029
Langston, W. J. (1980). Arsenic in UK estuarine sediments and its availability to benthic organisms. J. Mar. Biol. Assoc. U. K. 60 (4), 869–881. doi:10.1017/s0025315400041953
Larrose, A., Coynel, A., Schäfer, J., Blanc, G., Massé, L., Maneux, E., et al. (2010). Assessing the current state of the Gironde Estuary by mapping priority contaminant distribution and risk potential in surface sediment. Appl. Geochem. 25 (12), 1912–1923. doi:10.1016/j.apgeochem.2010.10.007
Liu, X., Jiang, J., Yan, Y., Dai, Y., Deng, B., Ding, S., et al. (2018). Distribution and risk assessment of metals in water, sediments, and wild fish from Jinjiang River in Chengdu, China. Chemosphere 196, 45–52. doi:10.1016/j.chemosphere.2017.12.135
Long, E. R., MacDonald, D. D., Smith, S. L., and Calder, F. D. (1995). Incidence of adverse biological effects within ranges of chemical concentrations in marine and estuarine sediments. Environ. Manag. 19 (1), 81–97. doi:10.1007/bf02472006
Loska, K., Cebula, J., Pelczar, J., Wiechuła, D., and Kwapuliński, J. (1997). Use of enrichment, and contamination factors together with geoaccumulation indexes to evaluate the content of Cd, Cu, and Ni in the Rybnik water reservoir in Poland. Water Air Soil Pollut. 93 (1-4), 347–365. doi:10.1007/bf02404766
MacDonald, D. D., Ingersoll, C. G., and Berger, T. A. (2000). Development and evaluation of consensus-based sediment quality guidelines for freshwater ecosystems. Archives Environ. Contam. Toxicol. 39 (1), 20–31. doi:10.1007/s002440010075
Magesh, N. S., Chandrasekar, N., Kumar, S. K., and Glory, M. (2013). Trace element contamination in the estuarine sediments along Tuticorin coast–Gulf of Mannar, southeast coast of India. Mar. Pollut. Bull. 73 (1), 355–361. doi:10.1016/j.marpolbul.2013.05.041
Mamun, A., Sumon, K. A., Sukhan, Z. P., Hoq, E., Alam, M. W., Haq, M. S., et al. (2013). “Heavy metal contamination in water and sediments of the river Karnafuli from south-east coast of Bangladesh,” in Conference paper, 4th the international conference on environmental aspects of Bangladesh (Japan: Fukuoka), 113–116.
Milićević, T., Relić, D., Škrivanj, S., Tešić, Ž., and Popović, A. (2017). Assessment of major and trace element bioavailability in vineyard soil applying different single extraction procedures and pseudo-total digestion. Chemosphere 171, 284–293. doi:10.1016/j.chemosphere.2016.12.090
Mohiuddin, K. M., Ogawa, Y., Zakir, H. M., Otomo, K., and Shikazono, N. (2011). Heavy metals contamination in water and sediments of an urban river in a developing country. Int. J. Environ. Sci. Technol. (Tehran). 8 (4), 723–736. doi:10.1007/bf03326257
Moore, J. W., and Ramamoorthy, S. (2012). Heavy metals in natural waters: Applied monitoring and impact assessment. Berlin, Germany: Springer Science & Business Media.
Müller, G. (1981). Die schwermetallbelstung der sedimente des neckars und seiner nebenflusse: Eine bestandsaufnahme. Chemiker-Zeitung 105, 157–164.
Olivares-Rieumont, S., De la Rosa, D., Lima, L., Graham, D. W., Katia, D., Borroto, J., et al. (2005). Assessment of heavy metal levels in Almendares River sediments-Havana City, Cuba. Water Res. 39 (16), 3945–3953. doi:10.1016/j.watres.2005.07.011
Otto, M. (1998). “Multivariate methods,” in WIDMER, HM. Analytical chemistry. Editors R. KELLNER, J. M. MERMET, and M. OTTO (Weinheim: Wiley VCH).
Pandey, J., and Singh, R. (2017). Heavy metals in sediments of ganga river: Up-and downstream urban influences. Appl. Water Sci. 7 (4), 1669–1678. doi:10.1007/s13201-015-0334-7
Pekey, H. (2006). The distribution and sources of heavy metals in Izmit Bay surface sediments affected by a polluted stream. Mar. Pollut. Bull. 52 (10), 1197–1208. doi:10.1016/j.marpolbul.2006.02.012
Persaud, D., Jaagumagi, R., and Hayton, A. (1993). Guidelines for the protection and management of aquatic sediment quality in Ontario. Ontario: Ministry of Environment and Energy.
Rahman, M. S., Ahmed, A. S., Rahman, M. M., Babu, S. O. F., Sultana, S., Sarker, S. I., et al. (2021). Temporal assessment of heavy metal concentration and surface water quality representing the public health evaluation from the Meghna River estuary, Bangladesh. Appl. Water Sci. 11 (7), 121. doi:10.1007/s13201-021-01455-9
Rahman, M. S., Hossain, M. B., Babu, S. O. F., Rahman, M., Ahmed, A. S., Jolly, Y. N., et al. (2019). Source of metal contamination in sediment, their ecological risk, and phytoremediation ability of the studied mangrove plants in ship breaking area, Bangladesh. Mar. Pollut. Bull. 141, 137–146. doi:10.1016/j.marpolbul.2019.02.032
Rahman, M. S., Molla, A. H., Saha, N., and Rahman, A. (2012). Study on heavy metals levels and its risk assessment in some edible fishes from Bangshi River, Savar, Dhaka, Bangladesh. Food Chem. 134 (4), 1847–1854. doi:10.1016/j.foodchem.2012.03.099
Rahman, M. T., Rahman, M. S., Quraishi, S. B., Ahmad, J. U., Choudhury, T. R., and Mottaleb, M. A. (2011). Distribution of heavy metals in water and sediments in Passur river, sundarban mangrove forest. Bangladesh. J. Int. Environ. Appl. Sci. 6 (4), 537.
Raj, S. M., and Jayaprakash, M. (2008). Distribution and enrichment of trace metals in marine sediments of Bay of Bengal, off Ennore, south-east coast of India. Environ. Geol. 56 (1), 207–217. doi:10.1007/s00254-007-1156-1
Ramanathan, A. L., Subramanian, V., Ramesh, R., Chidambaram, S., and James, A. (1999). Environmental geochemistry of the Pichavaram mangrove ecosystem (tropical), southeast coast of India. Environ. Geol. 37 (3), 223–233. doi:10.1007/s002540050380
Rehman, K., Fatima, F., Waheed, I., and Akash, M. S. H. (2018). Prevalence of exposure of heavy metals and their impact on health consequences. J. Cell. Biochem. 119 (1), 157–184. doi:10.1002/jcb.26234
Rubio, B., Nombela, M. A., and Vilas, F. (2000). Geochemistry of major and trace elements in sediments of the ria de Vigo (NW Spain): An assessment of metal pollution. Mar. Pollut. Bull. 40 (11), 968–980. doi:10.1016/s0025-326x(00)00039-4
Saha, N., Mollah, M. Z. I., Alam, M. F., and Rahman, M. S. (2016). Seasonal investigation of heavy metals in marine fishes captured from the Bay of Bengal and the implications for human health risk assessment. Food control. 70, 110–118. doi:10.1016/j.foodcont.2016.05.040
Saha, P. K., and Hossain, M. D. (2011). “Assessment of heavy metal contamination and sediment quality in the Buriganga River, Bangladesh,” in Proceeding: 2nd international conference on environmental science and Technology (Singapore: IPCBEE), 6, 384–388.
Sakan, S. M., Đorđević, D. S., Manojlović, D. D., and Predrag, P. S. (2009). Assessment of heavy metal pollutants accumulation in the Tisza River sediments. J. Environ. Manag. 90 (11), 3382–3390. doi:10.1016/j.jenvman.2009.05.013
Samad, M. A., Mahmud, Y., Adhikary, R. K., Rahman, S. B. M., Haq, M. S., and Rashid, H. (2015). Chemical profile and heavy metal concentration in water and freshwater species of Rupsha River, Bangladesh. Am. J. Environ. Prot. 3 (6), 180–186.
Saran, S. H., Rahman, A., and Yunus, A. (2018). Comparative analysis on flow and salinity of Rupsha-Passur river system of Bangladesh. J. Mod. Sci. Technol. 6 (2), 19–31.
Shaheen, S. M., Abdelrazek, M. A., Elthoth, M., Moghanm, F. S., Mohamed, R., Hamza, A., et al. (2019). Potentially toxic elements in saltmarsh sediments and common reed (phragmites australis) of Burullus coastal lagoon at north nile delta, Egypt: A survey and risk assessment. Sci. Total Environ. 649, 1237–1249. doi:10.1016/j.scitotenv.2018.08.359
Shen, F., Mao, L., Sun, R., Du, J., Tan, Z., Ding, M., et al. (2019). Contamination evaluation and source identification of heavy metals in the sediments from the Lishui River Watershed, Southern China. Int. J. Environ. Res. Public Health 16 (3), 336. doi:10.3390/ijerph16030336
Shikazono, N., Tatewaki, K., Mohiuddin, K. M., Nakano, T., and Zakir, H. M. (2012). Sources, spatial variation, and speciation of heavy metals in sediments of the Tamagawa River in Central Japan. Environ. Geochem. Health 34 (1), 13–26. doi:10.1007/s10653-011-9409-z
Shrestha, S., and Kazama, F. (2007). Assessment of surface water quality using multivariate statistical techniques: A case study of the fuji river basin, Japan. Environ. Model. Softw. 22 (4), 464–475. doi:10.1016/j.envsoft.2006.02.001
Siddique, M. A. M., and Aktar, M. (2012). Heavy metals in salt marsh sediments of porteresia bed along the Karnafully River coast, Chittagong. Soil &. Water Res. 7 (3), 117–123. doi:10.17221/7/2012-swr
Siddique, M. A. M., Rahman, M., Rahman, S. M. A., Hassan, M. R., Fardous, Z., Chowdhury, M. A. Z., et al. (2020). Assessment of heavy metal contamination in the surficial sediments from the lower Meghna River estuary, Noakhali coast, Bangladesh. Int. J. Sediment Res. 36 (3), 384–391. doi:10.1016/j.ijsrc.2020.10.010
Simeonov, V., Massart, D. L., Andreev, G., and Tsakovski, S. (2000). Assessment of metal pollution based on multivariate statistical modeling of ‘hot spot’sediments from the Black Sea. Chemosphere 41 (9), 1411–1417. doi:10.1016/s0045-6535(99)00540-8
Singh, K. P., Mohan, D., Singh, V. K., and Malik, A. (2005). Studies on distribution and fractionation of heavy metals in Gomti river sediments-a tributary of the Ganges, India. J. hydrology 312 (1-4), 14–27. doi:10.1016/j.jhydrol.2005.01.021
Smith, S. L., MacDonald, D. D., Keenleyside, K. A., Ingersoll, C. G., and Field, L. J. (1996). A preliminary evaluation of sediment quality assessment values for freshwater ecosystems. J. Gt. Lakes. Res. 22 (3), 624–638. doi:10.1016/s0380-1330(96)70985-1
Sundaray, S. K., Nayak, B. B., Lin, S., and Bhatta, D. (2011). Geochemical speciation and risk assessment of heavy metals in the river estuarine sediments-a case study: Mahanadi basin, India. J. Hazard. Mater. 186 (2-3), 1837–1846. doi:10.1016/j.jhazmat.2010.12.081
Suresh, G., Sutharsan, P., Ramasamy, V., and Venkatachalapathy, R. (2012). Assessment of spatial distribution and potential ecological risk of the heavy metals in relation to granulometric contents of Veeranam lake sediments, India. Ecotoxicol. Environ. Saf. 84, 117–124. doi:10.1016/j.ecoenv.2012.06.027
Traina, A., Bono, G., Bonsignore, M., Falco, F., Giuga, M., Quinci, E. M., et al. (2019). Heavy metals concentrations in some commercially key species from Sicilian coasts (Mediterranean Sea): Potential human health risk estimation. Ecotoxicol. Environ. Saf. 168, 466–478. doi:10.1016/j.ecoenv.2018.10.056
Turekian, K. K., and Wedepohl, K. H. (1961). Distribution of the elements in some major units of the earth's crust. Geol. Soc. Am. Bull. 72 (2), 175. doi:10.1130/0016-7606(1961)72[175:doteis]2.0.co;2
USEPA (2011). Exposure factors handbook 2011 edition (Final). Retrieved from: http://cfpub.epa.gov/.
USEPA (2001). Methods for collection, storage and manipulation of sediments for chemical and toxicological analyses. Washington, DC: Office of Water. Technical Manual, EPA-823-B-01-002.
USEPA (2006). National recommended water quality criteria. Washington, D.C.: United States Environmental Protection Agency. Office of Water, Office of Science and Technology.
USEPA (1989). Risk assessment guidance for Superfund. Human health evaluation manual. Washington, DC: Office of emergency and remedial response.
USEPA (2002). Supplemental guidance for developing soil screening levels for superfund sites OSWER 9355. Washington, DC, USA, 4–24.
USEPA (1999). US environmental protection agency: Screening level ecological risk assessment protocol for hazardous waste combustion facilities. Append. E Toxic. Ref. Values 3.
Varol, M., and Şen, B. (2009). Assessment of surface water quality using multivariate statistical techniques: A case study of behrimaz stream, Turkey. Environ. Monit. Assess. 159 (1), 543–553. doi:10.1007/s10661-008-0650-6
Venkatramanan, S., Chung, S. Y., Ramkumar, T., and Selvam, S. (2015). Environmental monitoring and assessment of heavy metals in surface sediments at Coleroon River Estuary in Tamil Nadu, India. Environ. Monit. Assess. 187 (8), 505. doi:10.1007/s10661-015-4709-x
Wang, X. C., Feng, H., and Ma, H. Q. (2007). Assessment of metal contamination in surface sediments of Jiaozhou Bay, Qingdao, China. Clean. Soil Air Water 35 (1), 62–70. doi:10.1002/clen.200600022
Wang, X., Liu, B., and Zhang, W. (2020). Distribution and risk analysis of heavy metals in sediments from the Yangtze River Estuary, China. Environ. Sci. Pollut. Res. 27 (10), 10802–10810. doi:10.1007/s11356-019-07581-x
Ward, J. H. (1963). Hierarchical grouping to optimize an objective function. J. Am. Stat. Assoc. 58 (301), 236–244. doi:10.1080/01621459.1963.10500845
Who, (2004). Guidelines for drinking water. Quality. 3rd Edition. Geneva, Switzerland: World Health Organization, 515.
Word, J. Q., Albrecht, B. B., Anghera, M. L., Baudo, R., Bay, S. M., Di Toro, D. M., et al. (2005). Predictive ability of sediment quality guidelines. Use of sediment quality guidelines and related tools for the assessment of contaminated sediments. Pensacola (Florida): Society of Environmental Toxicology and Chemistry, 121–161.
Wu, B., Wang, G., Wu, J., Fu, Q., and Liu, C. (2014). Sources of heavy metals in surface sediments and an ecological risk assessment from two adjacent plateau reservoirs. PLoS One 9 (7), e102101. doi:10.1371/journal.pone.0102101
Yang, Z., Wang, Y., Shen, Z., Niu, J., and Tang, Z. (2009). Distribution and speciation of heavy metals in sediments from the mainstream, tributaries, and lakes of the Yangtze River catchment of Wuhan, China. J. Hazard. Mater. 166 (2-3), 1186–1194. doi:10.1016/j.jhazmat.2008.12.034
Yi, Y., Yang, Z., and Zhang, S. (2011). Ecological risk assessment of heavy metals in sediment and human health risk assessment of heavy metals in fishes in the middle and lower reaches of the Yangtze River basin. Environ. Pollut. 159 (10), 2575–2585. doi:10.1016/j.envpol.2011.06.011
Yin, S., Feng, C., Li, Y., Yin, L., and Shen, Z. (2015). Heavy metal pollution in the surface water of the Yangtze estuary: A 5-year follow-up study. Chemosphere 138, 718–725. doi:10.1016/j.chemosphere.2015.07.060
Yu, X., Yan, Y., and Wang, W. X. (2010). The distribution and speciation of trace metals in surface sediments from the Pearl River Estuary and the Daya Bay, Southern China. Mar. Pollut. Bull. 60 (8), 1364–1371. doi:10.1016/j.marpolbul.2010.05.012
Zhao, G., Lu, Q., Ye, S., Yuan, H., Ding, X., Wang, J., et al. (2016). Assessment of heavy metal contamination in surface sediments of the west Guangdong coastal region, China. Mar. Pollut. Bull. 108 (1-2), 268–274. doi:10.1016/j.marpolbul.2016.04.057
Zhao, X. M., Yao, L. A., Ma, Q. L., Zhou, G. J., Wang, L., Fang, Q. L., et al. (2018). Distribution and ecological risk assessment of cadmium in water and sediment in Longjiang River, China: Implication on water quality management after pollution accident. Chemosphere 194, 107–116. doi:10.1016/j.chemosphere.2017.11.127
Keywords: trace metals, biological effects, ecological risk, human health risk, rupsha river
Citation: Kubra K, Mondol AH, Ali MM, Palash MAU, Islam MS, Ahmed ASS, Masuda MA, Islam ARMT, Bhuyan MS, Rahman MZ and Rahman MM (2022) Pollution level of trace metals (As, Pb, Cr and Cd) in the sediment of Rupsha River, Bangladesh: Assessment of ecological and human health risks. Front. Environ. Sci. 10:778544. doi: 10.3389/fenvs.2022.778544
Received: 17 September 2021; Accepted: 12 July 2022;
Published: 11 August 2022.
Edited by:
Oladele Ogunseitan, University of California, Irvine, United StatesReviewed by:
Bodrud-Doza, International Centre for Climate Change and Development (ICCCAD), BangladeshCopyright © 2022 Kubra, Mondol, Ali, Palash, Islam, Ahmed, Masuda, Islam, Bhuyan, Rahman and Rahman. This is an open-access article distributed under the terms of the Creative Commons Attribution License (CC BY). The use, distribution or reproduction in other forums is permitted, provided the original author(s) and the copyright owner(s) are credited and that the original publication in this journal is cited, in accordance with accepted academic practice. No use, distribution or reproduction is permitted which does not comply with these terms.
*Correspondence: Mir Mohammad Ali, bWlyLmFsaUBzYXUuZWR1LmJk; Md. Mostafizur Rahman, cmFobWFubW1AanVuaXYuZWR1
Disclaimer: All claims expressed in this article are solely those of the authors and do not necessarily represent those of their affiliated organizations, or those of the publisher, the editors and the reviewers. Any product that may be evaluated in this article or claim that may be made by its manufacturer is not guaranteed or endorsed by the publisher.
Research integrity at Frontiers
Learn more about the work of our research integrity team to safeguard the quality of each article we publish.