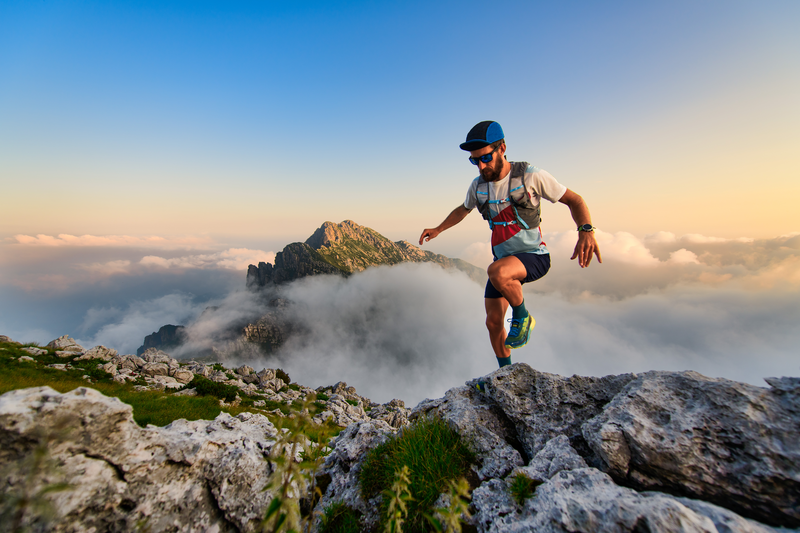
95% of researchers rate our articles as excellent or good
Learn more about the work of our research integrity team to safeguard the quality of each article we publish.
Find out more
REVIEW article
Front. Environ. Sci. , 05 April 2022
Sec. Water and Wastewater Management
Volume 10 - 2022 | https://doi.org/10.3389/fenvs.2022.766743
Water supplies around the world are currently heavily polluted by heavy-metal chemicals, synthetic dyes, and other toxic pollutants released by major factories rather than typical household waste. This pollution necessitates adequate monitoring to protect natural water sources. There are various wastewater treatment methods available, including nanotechnology, i.e., two dimensional (2D) nanomaterials. Rising 2D nanomaterials including graphene, g-C3N4, MoS2, MXene, black phosphorus, and h-BN have exhibited an unparalleled surface-to-volume ratio, promising ultralow usage of material, ultrafast handling time, and ultrahigh treatment performance for cleaning, and monitoring of water. We provide a current overview of tunable 2D nanomaterials and their uses in water management. A brief description of 2D nanomaterials, their types, synthesis strategies and salient features involved in water management is provided. Furthermore, application of 2D nanomaterial in different processes of water treatment such as pollutants adsorption, filtration, disinfection, photocatalysis are discussed in detail. Likewise, the potential of 2D nanomaterials to be used in water quality monitoring gadgets like fluorescent sensors, colorimetric sensors, electrochemical sensors, and field-effect transistors are also explored. The study ends with a look at the current problems, limitations and future prospectus associated with the use of 2D material in water management. The importance of clean and fresh water to upcoming generations will bring new light and innovations to this emerging sector, allowing it to improve the quality and accessibility of water treatment while also ensuring global water supplies in an increasing part of the world.
Water acts as a valuable natural asset and has a significant effect on human continuity circumstances. Water services are essential not only for biodiversity survival but also for improving livelihoods, generating income, and eradicating poverty. Clean and secure drinking water is a vital right of humanity, but only 71% of the world’s population has access to it (World Health & United Nations Children’s Fund, 2017). However, as the national economy and population overgrow, so does the issue of water contamination, which is becoming increasingly severe. Water is essential for all familiar modes of life, and it also plays a critical role in human culture, community creation, and even space investigation (Fu et al., 2016; Bi et al., 2020; Chen et al., 2020). The majority of water on Earth is saline seawater, and fresh and clean water only accounts for round about 3% of the global water resource, mainly in the form of groundwater, icecaps, and glaciers (Van Engelenburg et al., 2019).
Water pollution is now becoming inevitable due to the current need of urbanization and industrialization. Pollutants introduced into water from hazardous waste and incidental spills give rise to significant threats to the atmosphere and human health (Wu et al., 2017; Wang et al., 2019). Pollutants must be disposed efficiently and safely to protect water environments from pollution events and regular waste discharges. Therefore, it is critical to establish precise and effective methods for tracing pollutant origins, determining the sources of contaminants, being emitted into waterways, and determining the amount of pollution emitted (Jiabiao et al., 2018).
Different factories release different water contaminants during different hydrological cycles; thus, it is critical to implement effective sample construction techniques for the better refining of polluted water. Most sample construction techniques focus on adsorbent materials and these adsorbents are critical in achieving high clean-up and enrichment performance. As a result, research into advanced adsorbent materials has grown in popularity over the last few decades (Kumar et al., 2017; Premarathna et al., 2019).
Nanotechnology provides a good solution for water treatment. Several nanomaterials, including zero dimensional nanoparticles of metal or metal oxide [e.g., nanoparticles of Au (Wang S et al., 2018; Xu et al., 2019a), Ag (Liu et al., 2018a; Xu et al., 2019b), Fe3O4 (Zhao et al., 2016; Zhao J. et al., 2018), and TiO2 (Zhou and Fang, 2015; MiarAlipour et al., 2018)], 1-dimensional nanowires or nanotubes [e.g., nanowires of titanate (Yin et al., 2017) and carbon nanotubes (Dehghani et al., 2016; Akinpelu et al., 2019)], and 3-dimensional macrostructures (Shehzad et al., 2016) obtained from nanowires, nanoparticles, and nanotubes, are all demonstrated with great merits in water purification, considerably due to their large specific surface areas.
However, a number of constraints limit the number of uses for these low dimensional nanomaterials; for example 1) they prefer to aggregate or re-stabilize in solution, resulting in a significant decrease in surface area (Zeng et al., 2021), 2) complete recovery of depleted nanomaterials are critical, and nanomaterials in water that are not recycled, can significantly harm individuals and ecosystems (Zeng et al., 2021), 3) due to their limited dimension, their application is also limited (Zeng et al., 2021). Two-dimensional (2D) nanomaterials possess atomically thin structures with lateral sizes between tens of nanometers to a few micrometers and possess promising extremely low material consumption, rapid handling, and high treatment reliability (Anichini et al., 2018). The large lateral size and nanometer thickness endow 2D nanomaterials with a high specific surface area, making them desirable in a plethora of environmental remediation applications, such as adsorption, sensing, and catalysis (Chimene et al., 2015). The application of 2D-nanomaterials has been shown in the development of many water-treatment systems with remarkable adsorptive, catalytic, and separation capacity, such as graphene membranes with thin atomic layers (Surwade et al., 2015; Wei et al., 2017), oil adsorbents of high efficiency (Ge et al., 2017), extra-fast photocatalysts that use visible light to speed up the process (Liu et al., 2016; Haque et al., 2017), and other structures that are smart or self-heal (Luo et al., 2016; Oh et al., 2017) due to their ability to regulate the thickness and scale of their nanostructures.
In spite of the advances reported in the literature on 2D nanomaterials based water treatment technologies, relatively less work led it to market up to date, and in the water zone, the social value of 2D material has still to reach its original objective (Zheng et al., 2016a; Wang and Mi, 2017). The main reason behind this review is to lighten the properties of 2D nanomaterials in the range of water treatment and overcome the barriers in their pathway. Because of the increasing interest in 2D nanomaterials for water treatment, this analysis will provide investigators with an overview of the field’s present acquirement. Moreover, the mechanistic basis of how the elementary structures of 2D nanomaterials influence their cumulative nature, types of 2D nanomaterials with their synthesis process and salient features and their applications in water treatment and monitoring have been discussed.
Pollutants introduced into the water from hazardous waste and incidental spills give rise to significant threats to the environment and human health (Fang et al., 2018). Contamination in water is comprised of both natural and artificial components, with each species affecting human health to varying degrees (Sweetman et al., 2017). An overview of the major contaminant classes and example species in each, including their current removal strategies and associated removal difficulties are given in Table 1. Water toxins can take several forms, including dangled solids, chemical pollutants (organic and inorganic), and biological pollution (Wang et al., 2019), all of which are briefly discussed below.
Water contains a variety of microorganisms including Proteobacteria, Cyanobacteria, Bacteroidetes and Firmicutes. Bacteria from the Cyanobacteria, Methylobacteriaceae, Sphingomonadaceae, and Xanthomonadaceae families. Microbial biofilm formation is common in drinking water delivery systems and is exacerbated in older distribution systems (Douterelo et al., 2017).
Along with these microorganisms, concerned pathogens can sometimes make their path into drinking water and increase the consumer’s fear probability (Ashbolt, 2015). Enteric microorganisms and opportunistic pathogens are examples of pathogens that activate the illness in patients with immunological disorders. Waterborne protozoans such as Entamoeba histolytica and Giardia duodenalis become a source of waterborne diseases as well (Efstratiou et al., 2017). Viruses such as Hepatitis (A) and Norovirus are also known to be the causative factor in various diseases including waterborne (Bonadonna et al., 2019). These pathogens are known as the root of a variety of diseases that range in acerbity and can even be lethal. These illnesses cover kidney infections, lung diseases, gastroenteritis, dysentery, cholera, fever, and urinary tract (Khan and Malik, 2019).
Large and visible contaminants in rivers or bodies of water are referred to as macro-pollutants. Trash, whether deliberately spilt into bodies of water or left on the ground and swept into storm drains by runoff, is ultimately drained into waterways. This has resulted in the modeling of the “significant pacific garbage field,” which is now the magnitude of France. Nurdles (small plastic pellets), wood, and metal are other examples of macroscopic contamination. Though it may be simple enough to do, time is of the essence. This larger contaminants must be eliminated to prevent the destruction of marine habitats and damage caused by the chemical breakdown of these objects (Yu et al., 2017).
Petroleum, insecticides and herbicides, detergents, disinfecting cleaners, and pharmaceutical medications are promising examples of organic products. Methyl tert-butyl ether (MTBE) is one of the most often found highly toxic organic chemicals and was previously used as an air-cleaning gas additive (Stupp, 2007), however it is currently considered as a prohibited chemical, nonetheless it will take many years to be fully eliminated from polluted water supplies. Due to this organic chemical, water pollution can cause tumors in the testicles, kidneys, thyroid glands, as well as cause leukemia and lymphoma (Tran et al., 2015; Lipczynska-Kochany, 2018).
Pesticide toxicity is a pervasive problem in aquatic environments, accumulating in the bodies of aquatic organisms and the soil, creating health concerns to humans (Sharma, 2019). Numerous pesticide formulations have been introduced because of the global pesticide market’s rapid growth and their wide use in agricultural and non-agricultural sectors. Pesticides can be transported to surface waters and groundwater through runoff and infiltration, causing contamination to water bodies and thereby reducing the usability of water resources. (Syafrudin et al., 2021). The persistent and ubiquitous nature of various agriculture-based pesticides has posed revengement to the environment due to their bioaccumulation properties (UNEP 2007). Presently, throughout the globe approximately 2 million tons of pesticides are utilized, out of which 47.5% are herbicides, 29.5% are insecticides, 17.5% are fungicides and 5.5% are other pesticides (UNEP 2001).
Pharmaceutical compounds (PCs) are specially manufactured to produce a biological response in a target organism, may also produce the same response in non-target entities following chronic exposure to even trace concentrations of these compounds (Wilkinson et al., 2016). Pharmaceuticals are widely disseminated in the environment but are mainly found in wastewater effluents, however effluents from hospitals considered as the key emission source of PCs in the aquatic environment (Jureczko and Kalka, 2020) Among the wide variety of PCs, nonylphenol and bisphenol-A are the most extensively investigated compounds (Bilal et al., 2018), which compromise the health of different living organisms including humans (Corcoll et al., 2014). Moreover, pharmaceutical pollutants have been documented in groundwater, surface waterways (lakes, rivers, and streams), seawater, wastewater treatment plants (influents and effluents), soils, and sludges. In this scenario, their detection throughout the biosphere has become a supreme environmental concern, and it is of great importance to increase the efficacy of wastewater technologies to overcome environmental pollution. Interestingly, the worldwide detection and monitoring of PCs in surface water, wastewater, groundwater and drinking water (DW) also received the significant consideration of the World Health Organization (WHO), and they published an exclusive report documenting the presence of pharmaceuticals in groundwater and drinking water and the associated potential dangers for human health and ecosystem (World Health Organization, 2011). Given the PCs toxicity, Their removal from aquatic systems has been accomplished using a variety of techniques, including oxidation, photolysis, UV degradation, nanofiltration, reverse osmosis, and adsorption (Patel et al., 2019).
Detergents can contaminate water treatment systems, impairing their performance. Detergents are an integral element of human life and are consumed for a variety of reasons, most notably hygienic ones. Detergent elements can reach soil and water bodies via a variety of routes such as point sources and non-point sources. Point sources include domestic sewage networks, industrial effluents, accidental spills, seepage from waste water treatment plants, and non-point sources include agricultural practices such as irrigation with contaminated water in agricultural soils, washing laundry at the banks of streams at remote and country sides area particularly in developing countries, land run-off, thus imposing negative effects on the sustainability of the different flora and fauna of Earth’s (Holt 2000; Heidari 2012; Olkowska et al., 2014; Mousavi and Khodadoost, 2019). These chemicals can be toxic to both natural plant and wildlife on a chronic and acute basis, thus their removal from water is of prime importance.
Toxic wastes such as ammonia or urea-based nitrogen fertilizer, phosphorous based fertilizer and heavy metals are some examples of inorganic material contamination. Large massive metals like lithium, copper, chromium, nickel, barium, zinc, and mercury though insignificant in minimal quantities, become contaminants when concentrated in water (Verma et al., 2013; Shahzad et al., 2018; Tanveer et al., 2019; Bolan et al., 2021). Inefficient water disposal technique, leaching of polluted water and running off into water streams causes significant water pollution. This type of water contamination, especially at a high absorption level, can result in dangerous health issues in animals and humans, including death (Nieto-Delgado et al., 2019).
The discharge of heated liquid (such as wastewater from a factory) into natural waters at a temperature harmful to the environment is known as thermal pollution/contamination (Vallero 2019). The single biggest cause of thermal pollution is probably cooling for industrial machinery and power plants (Raptis et al., 2016). Increased water temperatures lower oxygen levels, which can change food chain structure, consume fish, and lessen species biodiversity especially in riverine ecosystems (Miara et al., 2018). Temperatures in surface waters can also rise due to urban runoff (Råman Vinnå et al., 2017).
Nanomaterials are commonly described as structures with dimensions of 1–100 nm (Saleh and Gupta, 2016). Nanomaterials behave differently than bulk form due to their higher ratio of surface area to volume and the possibility of quantum effects (Ding et al., 2017). Because of their unique shapes and properties, 2D nanomaterials have gotten plenty of coverages in recent decades (Bhimanapati et al., 2015; Robertson et al., 2016; Tan and Zhang, 2015). Currently, researchers are interested in using 2D nanomaterials in water treatment and monitoring (Table 2). Particularly, 2D nanomaterials are thought to be ideal replacements for conventional desalination and water purification membrane materials due to their atomically thin shape, wide surface area, and mechanical ability. One well-known example is the strong clumping tendency of 2D materials (i.e., agglomerates), which is frequently associated with unwanted material properties degradation, random structural deformation, and heterogeneous interlayer interference.(Goh and Ismail, 2015; Dervin et al., 2016; Homaeigohar and Elbahri, 2017; Jeong et al., 2020).
In recent years, 2D materials have gained widespread scientific consideration because of their unique structure and appealing applications (Figure 1). As a result, many different 2D nanomaterials have been projected and prepared using various techniques, with excellent implementations of these 2D materials shown. In this section, we will go through some common 2D nanomaterials, like carbon products (Graphene), boron nitride materials (BNM), transition metal oxides (TMOs), transition metal dichalcogenides (TMDs), layered double hydroxides (LDHs), and MXene (Huang et al., 2020).
FIGURE 1. Structures of 2D nanomaterials. Reproduced with permission (T. Hu et al., 2019) (Copyright 2019 ELSEVIER).
Graphene: Graphene is considered as a specific atom thick graphite, a carbon allotrope having a 2D structure. It is made up of a carbon network in a close hexagonal packing. In this structure, the single atom covalently bonded to further three neighbors (carbon atoms). A single sheet of carbon has around 0.142 nm from two adjacent carbon atoms. Individual layers pack collectively to form graphite with the help of the van der Waals power, with a space of around 0.335 nm between neighboring layers (Huang et al., 2020).
Boron nitride materials (BNMs) are isostructural to carbon and being used in various fields of science. BNMs exhibit large surface area, better mechanical strength, exceptional thermal stability and conductivity, excellent corrosion and oxidation resistance, low density, and wide-band gap semiconducting property, making them an ideal candidate for water remediation (Feng et al., 2016; Yu S et al., 2018). BNMs usually exist in four crystalline forms, including graphite like hexagonal BN (h-BN), rhombohedral BN (r-BN), wurtzite BN (w-BN), and diamond-like cubic BN (c-BN). The c-BN and w-BN are low-density phases with sp3 hybridized bonds, while the h-BN and r-BN are a dense phase with sp2 hybridized BeN bonds (Yu S et al., 2018; Sharma and Naushad, 2020). Two of the most widely used BNMs are BN nanosheets (BNNSs) (analogous to graphene) and BN nanotubes (BNNTs) (analogous to carbon nanotubes) (Golberg et al., 2010; Ruiyang Zhang et al., 2017). BNM-based adsorbents showed better performance as adsorbents for water purification compared with others, such as activated carbon (Sharma and Naushad, 2020), chitosan (Anitha, 2016) and carbon nanotubes (Ihsanullah, 2021).
Transition metal dichalcogenides (TMDs) are considered as layered materials with the common chemical formula MX2, here M represents a transition metal and X acts as a chalcogen. TMD monolayers stack collectively with the help of the van der Waals power. Each TMD monolayer is made up of fine three layers of atoms: a layer of transition metal, two layers of chalcogen, and a layer of transition metal interjected between them. TMDs’ ability to shape diverse crystal polytypes is one of their distinguishing characteristics (Manzeli et al., 2017).
Transition metal oxides (TMOs) are used as electrode materials to make electrochemical capacitors (ECs) due to their better redox activity compared with carbon materials. On the other hand, TMO’s lower electrical conductivity restricted their energy storage capacity, especially at higher rates. 2D nanostructured TMOs exhibit a high surface area, better compatibility with electrolytes, and a short way ion transfer pathway, which concomitantly and significantly increases the storage of charge capability of ECs as electrode materials (Tan et al., 2016). MXenes are a kind of two-dimensional layered transition metal carbide or nitride created by specific lithograph of raw MAX phases with the following equations Mn+1Xn (n = 1, 2, or 3), here M represents the transition metal (TM), A is a member of category of IIIA or IVA from periodic table, and X represents may be carbon or nitrogen. M layers are almost hexagonally close packed all together in MAX phases, and X atoms inhabit the octahedral sites. The elements of A and M are metallically bonded with each other and interleaved in the Mn+1Xn layers. Using solid etching solutions such as HF, the A layer could be depicted from the MAX phases, resulting in MXenes with 3 distinct structures, namely M2X, M3X2, and M4X3 (Dhakal et al., 2015). To date, close to 30 MXenes have been successfully synthesized including Ti3C2Tx, Ti2CTx, Nb4C3Tx (Ghidiu et al., 2014), Ti3CNTx, Ta4C3Tx (Naguib et al., 2012), Nb2CTx, V2CTx (Lukatskaya et al., 2013), Nb4C3Tx (Ghidiu et al., 2014); however, many more have been theoretically predicted based on the available MAX phase precursors (Barsoum, 2013; Lukatskaya et al., 2013). Of those, titanium based MXenes, such as Ti2CTx, and Ti3C2Tx are most promising for environmental applications due to element abundance and non-toxic decomposition products. Particularly, titanium carbide (Ti3C2Tx) is the most widely studied MXene (Naguib et al., 2014). Furthermore, different MXene compositions have been introduced with two or more transition metals in the M layers in both ordered and disordered structures (Mo2Ti2C3, (Ti0.5,Nb0.5)2C, (V0.5,Cr0.5)3C2) (Anasori et al., 2015; Gogotsi and Anasori, 2019).
Layered double hydroxides (LDH) are positively charged layered materials with loosely bound charge balancing anions or solvation molecules, as well as interlayer molecules of water, with a typical formula of [Mz+1-xM3+x (OH)2]m+ [An−]m/n.yH2O, in interlayer spaces. Much of the time, Mz+ and M3+ reflect divalent and trivalent metal ions, respectively, yielding m = x. An− is a replaceable anion found in the inter—layer region. A distinguishing feature of LDHs is the absence of bonding between the cation layers, which enables the interlayer spacing to expand or contract to accommodate a diverse array of interlayer anions. As a result, An− can be any anion capable of balancing charges (organic or inorganic) (Mohapatra and Parida, 2016; Bukhtiyarova, 2019).
Top-down synthesis method of nanomaterials involves the development of ultrathin like needlelike nano-sheets using either a chemical or physical procedure (Figure 2). The physical top-down synthesis method uses ultrasonic waves or mechanical force to scale off layered van der Waals solids into solo and some layer 2D materials, while the chemical top-down synthesis method depends primarily on chemical reactions induced by ion exchange, heat, and some other means (Chen et al., 2018; Jianghao et al., 2016). The physical top-down approach involves the usage of photons, electrons, and ions while the chemical top-down strategy relies largely on chemical reactions that are brought about by chemical etchants or by application of heat (Yu et al., 2013). The top-down approach is widely used in the semiconductor device industry because it can be used to fabricate a wide range of devices with high reliability and integrity. Many innovative structures, such as nanowires which may be utilized to detect biological samples without labelling, have been produced by adopting this approach. In a top-down technique, the main fabrication stages are 1) lithography, 2) laser ablation, 3) chemical etching, 4) milling, and 5) thermal breakdown (Bellah et al., 2012).
FIGURE 2. Synthesis methods of 2-dimensional Nanomaterials. (A) There are two primary strategies: top-down exfoliation and bottom-up growth. (B) A series of different extensive fabrication techniques to synthesize 2D Nanomaterials (Li, Wang, Yin, Lewis, & Wang, 2020).
The top-down approach is effective for creating ultra-thin, needlelike, high-quality nano-sheets with broad sideways dimensions (Figure 2). Moreover, it should be noted that all of the previously described exfoliation techniques are only relevant to materials with layered crystals in bulk form. The cost of mass production using the methods described previously is typically very low. Materials at nonorange are made from molecular or atomic precursors that may be reactive and expand in size or self-assemble into more structures found in complex form, using a bottom-up method. Thus 2D materials can be easily extended (Karfa et al., 2020). This method offers the ability to construct effective multicomponent devices by self-assembly of atoms and molecules without wasting them or requiring the removal of system components (Biswas et al., 2012). Physical aggregation, chemical reactions, or the use of templates are commonly used to manipulate the assembly of the basic building blocks (Liu and Bashir, 2015). Controlled chemical reactions cause the building blocks to self-assemble and form nanostructures like nanotubes, nanoribbons, and quantum dots (Noah, 2020). Although one of the primary obstacles is ensuring predetermined structures with precise forms and sizes, this strategy has the ability to create nanostructured materials when the top-down approach fails (Bellah et al., 2012).
Two-dimensional nanomaterials are made up of thin films of at least one atomic layer of thickness. In contrast to materials in bulk form, these nanomaterials have a higher ratio of surface area to volume and hence have many atoms present on their surfaces. Since these atoms have a multi-purpose than the inner atoms, enlarging the number of atoms at the surface alters the behavior of 2D nanomaterials. Because of their thickness and dimensions on the macroscale or nanoscale, 2D nanomaterials are thought to be attenuated nanomaterials. These nanomaterials are strongly layered with each other with in-plane bonds and within layers, they are layered through weak van der Waals bonds (Rafiei-Sarmazdeh et al., 2019). A brief description of these features is provided below:
A material’s electrical and optical properties are determined by its electronic band arrangement. It explains how electrons (e−s) pass around the substance and is caused by the periodicity of the crystal structure. When a substance is converted from bulk form to two-dimensional, the consistency in the perpendicular direction to the plane is eliminated, which may significantly alter the structure of bands. The altered band structures are then pledged for graphene’s extraordinarily large conductivity and mono-layer MoS2 fluorescence (Sangwan and Hersam, 2018).
Lessened screening of dielectric between holes and electrons present in semiconductors is another consequence of dimensional confinement. When there is small material available to screen the electric field, Coulomb interaction starts increasing and strongly bounded excitons become more stable than those excitons present in the form of bulk materials. If excitons are trapped in a plane that is narrower than their Bohr radius, as it already happened in many 2D semiconductors, thus quantum confinement starts increasing their energy relative to excitons present in bulk form, increasing the wavelength of light they consume and release (Zheng and Zhang, 2019).
Their energy levels could be regulated by altering the number of layers in the 2D substance (for example, a bi-layer structure can absorb or emit lower-energy light than a mono-layer structure). However, this may have an impact on the band composition, as well as by developing modifications to other properties (for example, bi-layer MoS2 becomes non-emissive analyzed to a mono-layer due to transition in electronic band structure) (Ramalingam et al., 2020).
In comparison to 0D, 1D, and 3D materials, 2D materials have a high surface-to-volume ratio. A high surface area to volume ratio provides a broad surficial area for the contact of 2D materials and analytes, making 2D materials more suited for sensor applications. As a result, low concentration analytes can be detected using such sensors.
The ratio of surface area to volume of a substance determines how much it is available to its surroundings. This is significant for chemical reactions because the larger reactant that comes into connection with the sample, will perform the reaction quicker, so 2D compounds are more reactive than in bulk form equivalents. It also makes 2D materials more receptive to their environment and is used in sensors made of 2D materials (Yu X et al., 2018).
Many covalently bound planes are placed together with the help of weak van der Waals interactions present in a bulk material of layered form. When a force is practiced to an object, these Van der Waals forces are quickly resolved, and the material fractures, giving the impression that it is fragile. The covalent bonds that tie the atoms altogether in the layers, on the other hand, are extremely more powerful. A monolayer has only covalent bonds. After replacing the material’s “weak links,” it tends to become even stronger. For example, graphene has 1,000 times the tensile strength of graphite, and although a graphite pencil is easily broken, graphene is about 100 times stronger than steel (Liu et al., 2019).
Two-dimensional nanomaterials are thought to be ideal replacements for conventional desalination and water purification membrane materials due to their atomically thin shape, wide surface area, and mechanical ability. One well-known example is the strong clumping tendency of 2D materials (i.e., agglomerates), which is frequently associated with unwanted material properties degradation, random structural deformation, and heterogeneous interlayer interference (Goh and Ismail, 2015; Dervin et al., 2016; Homaeigohar and Elbahri, 2017; Jeong et al., 2020). In this section, we have briefly discussed the mechanistic mechanisms of different 2D nanomaterials and their applications in removing and controlling water contaminants.
Adsorption is one of the most efficient techniques that is widely utilized for water purification owing to their ease of operation, regeneration ability, low cost, high removal efficiency, availability of plentiful adsorbents, and less harmful by-products (Zubair et al., 2018; Ihsanullah, 2021). Several nanomaterials such as graphene (Lim et al., 2018), carbon nanotubes (Abbas et al., 2016), metal oxide (Kumar et al., 2013), and MXenes (Rasool et al., 2019; Ihsanullah, 2020) have been extensively exploited as adsorbents for the elimination of numerous toxic impurities from water (Jia et al., 2017; Chang et al., 2017). In this section, we have briefly discussed the mechanism conferring the role of 2D nanomaterials as adsorbents for water treatments.
Graphene is a 2D nanomaterial and exists as sheets of a single layer of graphetically bonded carbon atoms (Geim and Novoselov, 2010). The high available surface area for contaminant species to adsorb to, as well as the potential for exotic chemical modifications and composite fabrication make graphene an attractive material to use for water purification (Dreyer et al., 2010). For water purification applications, graphene is usually converted into graphene oxide (GO) via an acid treatment process, namely the Hummers or improved Hummer’s methods (Han et al., 2013). This conversion to GO introduces a high number of hydrophilic oxygens containing groups across the graphene sheet, including hydroxyls and epoxides (Gao et al., 2011). The most profound effect of the chemical modification is to markedly increase water flow through GO materials due to an increase in hydrophilicity, enabling the formation of membranes with high water permeability and flux (Sweetman et al., 2017). The underlying mechanisms involved in graphene and GO mediate water purification include electrostatic, hydrogen bonding and π–π interactions between contaminant species and the graphene material all contribute to the material’s excellent adsorption capacity (Baig et al., 2019). Furthermore, the high specific surface area (over 1,000 m2/g), the laminar structures and nano-perforated membranes of graphene’s facilitates excellent adsorption capacity (Sen Gupta et al., 2015). Graphene materials for neutralizing microorganisms’ function via disrupting the biological function of these species, comptonization of the bacteria membrane, generation of reactive oxygen species (ROS) and envelopment of microorganisms, are key mechanistic approaches for water purification regulated by graphene (Hu et al., 2010; Chen et al., 2014).
LDHs: adopt a hydrotalcite-like anionic lamellar structure, which is assembled throughnoncovalent bonding interactions between the cationic host layers and the anionic guest interlayers (Zou Y et al., 2016). LDHs exhibit large interlayer spacing, broad chemical composition, and ion-exchange ability, thus making them an excellent 2D materials to use as catalysts or adsorbents in the field of water remediation (Song et al., 2018). Over the last decade, numerous efforts such as surface decoration, calcination, intercalation, and multiple composition methods, have been made to improve LDH materials and graphene-LDH compost (G-LDH) has been widely accepted for water remediation (Pang et al., 2019).
2D BNMs: hexagonal BNM (so-called “white graphene”), a layered material, structurally analogous to graphite has been explored by researchers extensively in water treatment, carbon capture, and catalysis (Fang et al., 2016). Briefly the mechanistic mechanisms of the adsorption process of water pollutants on BNMs can be explained in the context of the electrostatic attractions, pep stacking interactions, physisorption, surface complexation, van der Waals forces, hydrogen bonding, and chemical adsorption, however the adsorption mechanism merely depends on the nature of the pollutants, structure, and surface characteristics of the BN materials (Gonzalez-Ortiz et al., 2020). BNMs can interact with the aromatic rings of organic compounds through hydrophobic interaction and p-p interactions and the hydrophobic interaction between the aromatic rings of organic compounds and the hydrophobic surface of BNMs also plays an important role in the adsorption of pollutants (Ihsanullah, 2021).
MXenes are another potential player for water purification, as due to the high specific surface area, abundant functional –OH and –O groups with tunable surface chemistry, the adsorption performance of MXenes can effectively compete with or outperform with other nanomaterials. Moreover, the presence of functional groups on MXene surface not only provides sites for direct ion exchange, but also reduces some organic molecules and cations (Shahzad et al., 2017; Zhao L et al., 2018). This in-situ reduction ability coupled with adsorption is considered an advantageous over many other nanomaterials and opens a new promising function of MXenes for unique reductive-adsorptive removal of pollutants. Due to a high negative surface charge (zeta-potential between 30 and 80 mV), MXenes have demonstrated an excellent ability to spontaneously intercalate Na+, K+, NH4+, Mg2+ and Al3+ cations and polar organic molecules such as hydrazine, urea, and DMSO between their layers, showing the potential of their use as adsorbents (Lukatskaya et al., 2013; Mashtalir et al., 2013). Moreover, the exterior surface groups of MXenes not only provide direct ion exchange sites, but also reduce some organic molecules and cations like Cu2+and Cr6+ (Zou G et al., 2016; Shahzad et al., 2017). This in-situ reduction ability also reveals a new promising function of MXenes for pollutant removal. For example, DL-Ti3C2Tx nanosheets exhibited efficient reductive removal of Cr6+, where the residual concentration of Cr6+ after treatment was less than 5 parts per billion (ppb) witha maximum removal capacity of 250 mgg 1 (Ying et al., 2015). Some other features of MXenes such as thin interlayer spacing (<2Å) between MXene nanosheets, and the presence of hydrophilic groups and weak bonding forces in hydrated MXene make them very distinctive in adsorbing pollutants more efficiently as compared with other 2D nanomaterials (Ren et al., 2015). Different MXenes have been used as absorbent to treat contaminated water (Table 3).
TABLE 3. Selected examples of the utilization of MXenes for water purification (adapted from Rasool et al. (2019)).
Membranes provide a physical shield to distinguish unwanted materials present in water depending on their size or may be permeability, which is a primary goal of water treatment. Because of the common trade-off between water permeability and selectivity, it is especially difficult to develop practical membranes with both high permeability and high selectivity using conventional membrane materials. The permeability of a membrane is understood to be inversely proportional to its thickness. As a result, 2D nanomaterials with atom-thicknesses ranging from 1 to a few nm have appeared as exciting structural blocks for producing splitting membranes of the future generation. Incorporating 2D nanomaterials through practical membrane architecture, for example, allows for the development of an atomic thin separating structure that allows molecules of water to move along selectively. These isolated membranes can be classified into membrane filtration like liquid state of water and solar membrane distillation like gas state of water depends on the state of water that infused them (such as, gas phase or liquid phase) (Liu et al., 2016; Zhu et al., 2018). A typical example of 2D nanomaterials mediated membranes filtration is desalination process. So far, seawater desalination has been mainly performed via multistage flash distillation and reverse osmosis (RO) (Xu et al., 2016). The desalination plants adopt reverse RO technology, and, in this technology, water is forced through a semi-permeable membrane by an external positive hydrostatic pressure. Accordingly, a larger volume of water passes through the membrane compared with the volume of dissolved salts or organic molecules. RO is indeed the most energy-efficient technology for seawater desalination and is the benchmark for the comparison of any new desalination technology, however this technology still suffers from low desalination capacity, treatment with RO is membrane fouling, which reduces treatment effectiveness and results in difficult-to-manage concentrate output. Separation of ammonium is frequently insufficient during reverse osmosis (Tałałaj et al., 2019) and high capital costs, hampering its broad application in most developing markets (Nicolaï et al., 2014). The core of the RO process is a semipermeable membrane that separates pure water from seawater (Zheng et al., 2016b). The classic semipermeable RO membrane is still based on the same polyamide thin-film composite design that was developed 3 decades ago (Cohen-Tanugi and Grossman, 2015). The most permeable thin-film composite membranes currently offer only 1.5–2 times higher permeability than that 20 years ago and they are still damaged when contacting chlorine; thus, disinfection becomes challenging and the fouling tendency increases (Lee, 2011). To meet the needs of advanced desalination required to address the water challenges of the twenty-first century, a breakthrough in RO membrane technology is a must. In comparison to standard RO membranes, the produced TFC-PE membrane exhibits greater mechanical robustness and resistance to organic solvents, which is mostly due to the PE support’s impressively high mechanical and chemical stability (Park et al., 2018). However, there are certain downsides of polyamide thin film composites membranes, such as chlorine, fouling, and working temperature restrictions (0–45°C) (Ibrahim et al., 2015).
Graphene can be regarded as an ultimate RO membrane, because it is stronger, thinner and more chemically resistant than the polyamide active layers in thin-film composite RO membranes (Cohen-Tanugi and Grossman, 2015; Homaeigohar and Elbahri, 2017). The atomic thinness of graphene (d ≈ 0.34 nm) can lead to larger water permeability than the polyamide active layer in thin-film composite membranes (d ≈ 100 nm) (Goh and Ismail, 2015). In addition, graphene shows better tolerance to chlorine than polyamide, that is, an important advantage in hindering membrane fouling without degradation (Cohen-Tanugi and Grossman, 2015). GOs shows antimicrobial properties, thus lowering membrane biofouling, that is, improving the membrane lifetime and energy consumption of the water purification processes (Mahmoud et al., 2015). Some flaws in graphene sheets, such as grain boundaries, have been proven to be important in assessing their dependability in real desalination plants, since these defects might affect the mechanical and selectivity capabilities ascribed to graphene membranes (Xu and Zhang, 2016). Moreover given the excellent properties of graphene materials for water desalination and purification membranes (Wang and Karnik 2012; You et al., 2016), the difficulty to obtain a large area graphene membranes on appropriate supports, and the lack of suitable strategies to generate pores of proper dimensions to exclude small ions, such as Na+, K+ and Cl−, in a pressurized filtration process, are the two major limitations to be overcome for the wide use of graphene on desalination (Presumido et al., 2021). MXenes on other hand also show very effective results in desalinisation process (Table 3). In a study, it was found that because of excellent electrical conductivities and high specific surface areas, a high salt removal rate of 9.4 mg g−1 min−1 was observed using Mxenes Ti3C2Tx in a capacitive desalination process (Guo et al., 2018).
Photocatalysis is a process which makes use of solar energy to clean water, has been extensively studied and is regarded as one of the most durable approaches to addressing environmental problems with limited energy production. In general, photocatalysis entails 1 or more photo reaction procedures catalyzed by the photocatalyst, in which available unbound electrons (e−s) are excited to the conduction band and holes remain in the same state, i.e., valence band. As a result, these electron-hole pairs are crucial in photocatalytic applications such as splitting water, pollutant oxidation, and reduction of CO2. Though, the excited charge carriers by photo-generated are unstable and simply recombined, lowering photocatalysis conversion efficiency. Because of the distinct structures of 2D nanomaterials and their nanocomposites, 2D material-dependent photocatalysts are promising applicants to give indication characteristics like multiplicity of host-guest species, high specific surface-areas, abundant surface energetic sites, and porous structures. The photo-catalyst black phosphorus (BP) has lately emerged as a promising photo-catalyst for solar H2 generation, but its bulk crystal with a bandgap of 0.3 eV limits the driving force required for optimal photocatalytic activity (Figure 3A). In addition, the massive and intimate 2D nanojunctions created in this smart 2 dimenional-2 dimensional BP/MoS2 can speed up photo-generated charge separation, resulting in strong photocatalytic H2 production activity (Yuan et al., 2019). Graphene, carbon nano-sheets, metal oxide, and BNM and MXene have recently been studied as 2D material-based photocatalysts for a variety of applications.
FIGURE 3. (A) Schematic diagrams of 2D-2D BP-10000/MoS2 photocatalysts. Reproduced with permission from Yuan et al. (2019), (B) Photocatalytic mechanisms of graphene-TiO2 composite under (a) visible light (b) UV light, Reprinted with permission from G. Hu & Tang (2013), (C) The energy diagram of the TiO2 and g-C3N4 composites for photocatalytic H2 evolution under visible light irradiation. Reproduced from (Zhong et al., 2019), (D) Mechanism of photocatalytic generation of RSs on TiO2-rGO under UV and visible irradiation, and in the presence of chloride. Reproduced from Liu et al. (2019).
Graphene-based nanocomposites photocatalysts have showed a significant potential as heterogeneous photocatalysis systems for water purification. The complement of semiconductor with graphene has also emerged as a prominent alternative for improving the photoactivity of the nanocomposites. Three strategies can be mainly considered for the synthesis of graphene nanocomposites.
(1) increasing the contact area between semiconductor and graphene by forming hybrids with a 2D-2D material for efficient charge transfer and, also, formation of trapping channels for electron to lower the rate of recombination. For instance, an electron transport model of graphene/titanate nanotubes (TNTs) photocatalyst under visible-light illumination based on their energy band topologies. A metal–semiconductor junction is formed by graphene and TNTs, and graphene can be used as a sensitizer to provide the photocatalyst with an outstanding visible-light response (Figure 3B). Xiang et al. (2012) prepared TiO2/graphene and graphene/g-C3N4 composites as 2D-2D materials which, under the visible light, exhibited enhanced photo-efficiency. Moreover, three different synthetic protocols (co-calcination, solvothermal treatment, and charge-induced aggregation) were used to make TiO2 and g-C3N4 2D/2D nanocomposites, with different degrees of enhancement (1.4–6.1 fold) in the visible-light-induced photocatalytic hydrogen evolution reaction compared to the simple physical mixture and mechanism (Figure 3C),
(2) improvement in the proximity of bonding between semiconductor and graphene, because it plays a major role in regulating the photocatalytic efficiency,
(3) introducing interfacial mediators between semiconductor and graphene (Han et al., 2014). For instance, using a noble metal as an interfacial mediator to prepare Cu2O/Pd/RGO (reduced graphene oxide) composites (Bai et al., 2014). The role of Pd nanoparticles here is collecting the holes from Cu2O and transferring them onto graphene surface, and thereby suppressing the electron-hole pair recombination to a great extent. Graphene electrical conductivity can be enhanced via solvent-exfoliated graphene (SEG) method, for instance (Yuan et al., 2014), fabricated the SEG/TiO2 which was of higher photo-activity, as compared to RGO/TiO2, for aerobic oxidation (3D).
BNMs are another potential 2D nanomaterials, which facilitate water purification by photocatalysis mechanism (Table 4). BNMs are excellent candidates for the sorption of pollutants, such as oil and organic solvents from heavy industries, and for water purification (Gonzalez-Ortiz et al., 2020). BNMs interact with other metal oxides to increase photocatalytic reaction, for instance, BNMs significantly enhance the photocatalytic performance of TiO2/porous BNMs by facilitating the electron-hole separation on TiO2/P-BNMs. The presence of photoreaction species such as hb and OH, on TiO2 surfaces, and large surface area of BNMs make the fast adsorption of LR2B onto TiO2/porous BNMs (Ihsanullah, 2021). Moreover, BNM doped polyaniline (PANI) hybrid nanocomposite was found effective in photodegradation of pollutants (Shahabuddin et al., 2018) PANI has the conducting polymeric chains that enable them to degrade organic matter; however, doping of PANI with BN amplifies the generation of photoelectrons and holes that enhances the photocatalytic efficiency of the nanocomposite (Shahabuddin et al., 2018). BNM can be used also to enhance TiO2 photocatalytic activity for the treatment of pollutants in water. BNMs/TiO2 nanocomposites have been also tested to remove other contaminants, such as ibuprofen, by photocatalytic oxidation. BNNS addition improved the light absorbance and reduced the electron/hole pair recombination. Moreover, the nanocomposite photocatalytic oxidation rate increased with higher BNMs loads (Lin et al., 2019).
MXenes exhibit metallic behavior with a substantial electron density near the Fermi level (Tang et al., 2012). In addition, the large and anisotropic carrier mobility in MXene facilitates the migration and separation of photogenerated electron-hole pairs, making them promising for photocatalytic applications (Rasool et al., 2019). The higher photocatalytic performance of the TiO2/Ti3C2Tx nanocomposite can be attributed to more effective electron-hole separation and the formation of a Ti3C2Tx and TiO2 heterojunction of the composite over TiO2 or Ti3C2Tx alone under UV light irradiation. TiO2/Ti3C2Tx nanocomposites have also been used for hydrogen production through photocatalytic water splitting (Liu J et al., 2015). In this nanocomposite, Ti3C2Tx acted as an electron sink to promote the separation of photo-generated charge carriers and provide a 2D platform for intimate interactions with TiO2 NPs (Rasool et al., 2019). In a study by Guo et al. (2016), based on ab initio calculations, 48 two-dimensional (2D) transition metal carbides also referred to as MXenes were investigated to understand their photocatalytic properties, and obtained results highlighted 2D Zr2CO2 and Hf2CO2 as the candidate single photocatalysts for possible high efficiency photocatalytic water splitting. A significant property of 2D Zr2CO2 and Hf2CO2 is that they exhibit unexpectedly high and directionally anisotropic carrier mobility, which may effectively facilitate the migration and separation of photogenerated electron–hole pairs (Guo et al., 2016). Some additional examples of the use of MXenes as photocatalytic agent have been given in Table 3.
Disinfection, i.e., the elimination, deactivation, or destruction of pathogenic microorganisms, is the final and most significant phase of water and wastewater treatment to safeguard natural habitats and public health. Disinfectants used in water treatment today include Cl2, NH2Cl, O3, and ClO2. These disinfectants are ineffective at inactivating all contaminants and produce toxic disinfection by-products (DBPs). The need for new disinfectants for water treatment is becoming increasingly urgent. Thus, 2D nanomaterials play an important role in this regard. Recently, application of MXenes (Ti3C2Tx) have been found very effective in treating various bacteria such as against Bacillus subtilis and Escherichia coli (Table 3). The distinctive feature of tremendous resistance to biofouling makes MXenes fit for membrane application. The antibacterial characteristics of MXenes can be attributed to anionic nature of the surface, the direct killing of bacteria due to contact with hydrophilic surface high and hydrogen bonding between the cell membrane and oxygenate groups of MXene that preclude the nutrient intake (Ihsanullah, 2020). The atomic structure of MXenes also plays a role in their antibacterial properties (Jastrzębska et al., 2019).
Likewise, graphene and graphene oxide (GO), even without any functional, also very effective antimicrobial agents (Yang et al., 2011). The antibacterial activity of water-dispersible graphene derivatives; GO and RGO nanosheets can efficiently prohibit the growth of E. coli bacteria while exhibiting minimal cytotoxicity (Table 5) (Hu et al., 2010). GO weakens the metabolic activity of microorganisms (Ahmed and Rodrigues, 2013). This causes a reduction in oxygen consumption and ultimately decreases the value of biological oxygen demand (BOD) which finally leads to cell death. The bactericidal effect of graphene is also induced by the sharp edge of graphene nanosheets which results in physical disruption of the cell membrane and causing the integrity loss in membrane and release of cellular content and eventually cell death (Upadhyay et al., 2014). Graphene-based materials can also cause oxidation of bacterial proteins, lipids, DNA and, also, are capable to oxidize other cellular components and thiols (Upadhyay et al., 2014). The bacterial cytotoxicity of GO and rGO is attributed to both membrane and oxidative stress (Singh et al., 2020).
TABLE 5. Graphene mediated bacterial disinfection in water (adapted from Singh et al. (2020)).
In water treatment systems, precise, sensitive, and rapid identification of contaminants is critical because it quantitatively defines the efficacy of water decontamination. For identifying and tracking toxins in water, a substantial number of nanomaterials have been studied (Farka et al., 2017; Yan et al., 2018; Zhang et al., 2019). Notably, the potential of 2D Nanomaterials to reply to exterior stimuli having extra high surface reactivity explains the vital properties for monitoring water and observing properties for pollution. Recently, 2D nanomaterials with exceptional electrical or optical properties have sparked intense concern in the evolution of great production sensor devices for pollutants. Noncovalent interactions like electro statical force and covalent interconnections, i.e., amide bonding could be used to investigate pollutants depending on the kind of analytes. This section focuses on the phase of the art of 2D material-dependent sensors for monitoring water pollutants via optical (Gu et al., 2017; Li B. L et al., 2017; Li et al., 2015; Liu C et al., 2018; Liu X et al., 2015; Mao K et al., 2015; Mao P et al., 2015; Qian et al., 2015; Wang Y et al., 2016; Zuo et al., 2016) or electrical outputs (Jiang et al., 2015; Seenivasan et al., 2015; Chaiyo et al., 2016; Zhou et al., 2016; Cui et al., 2017; Li P et al., 2017; Mao et al., 2017). Following are the key applications of 2D nanomaterials that can help in controlling and monitoring water contamination.
Fluorescent sensors, also known as photo-luminescent sensors, depending on variations in fluorescence, such as intensity, wavelength, and lifetime caused by pollutants (Xu et al., 2016). Such variations in the intensity of light or wavelength result from pollutant binding and altering the sensor’s structure or composition (Gao and Tang, 2017). The basic principle of fluorescence-based sensors has been built on the fact that the specific interaction between recognition component (nanomaterial) and target analyte (Pb) induce changes in the fluorescence properties of nanomaterial as can be observed in various forms (e.g., fluorescence quenching, fluorescent enhancement/recovery, radiometric fluorescence output, wavelength shifts, and anisotropy) (Luo et al., 2020). Recently, a variety of nanomaterials have been used in the development of fluorescent sensors with improved sensitivity and selectivity along with the formation of nanocomposites and/or functionalization with organic linkers (Singh et al., 2021). As photoluminescence is associated with transfer processes of energy, the electronic band-gap layout of 2D nanomaterials is critical for establishing fluorescent (bright) sensors. With a broad variety of band-gap alternatives, 2-dimensional nanomaterials, such as graphene of zero band-gap, have provided tremendous possibilities in the region of sensing (Zeng and Zhang, 2019). Some 2D nanomaterials with UV-Vis band-gaps could be used directly as fluorescent (bright) sensors or mixed with some other materials for making practical composites (Rong et al., 2015).
Unlike fluorescent sensors which require UV light for laser excitation, colorimetric sensors can alter color when they encounter contaminants (Nirala et al., 2015; Sano et al., 2016). Colorimetric sensor detects pollutants in water via surface plasmon resonance (SPR) approach, enzyme-based catalysis, fluorescent switch, and ligand-receptor binding (Liu et al., 2018b). In SPR mechanism, upon contact with pollutants 2D nanoparticles colorimetric sensors reveal different colors. When the nanoparticles gather, the solution color of the nanoparticles will change, which is convenient for the naked eye to determine. This process of aggregation is caused by the composition of the solution or the properties of the surface of the particles, through the electrostatic interaction or hydrogen bonds between the nanoparticles and their surroundings (Li B. L et al., 2017). For instance, a flow batch-operated AgNP-based automatic colorimetric sensor was used for the detection of trace Cu2+ in water samples (Peng et al., 2017). In 2D colorimetric sensors, detection of pollutants is done by the fluorescence properties of some organic dyes, fluorescent polymers, and fluorescent nanomaterials (such as metal nanoclusters, quantum dots, and carbon dots) (Liu et al., 2019) (For more details see Liu et al. (2018b)).
Enzymatic catalysis based colorimetric sensors utilized two types of enzymes, natural enzymes, and mimic enzymes. Though there is no major difference among these two enzyme types in their catalytic mechanisms, however, the stability and catalytic activity of natural enzymes are excellent (Zeng et al., 2020). Colorimetric sensors mediated by enzyme and mimic enzyme catalysis are usually based on color changes caused by enzyme and biomimetic enzyme catalysis of 3,3,5,5-tetramethylbenzidine (TMB) (Liu et al., 2019).
In ligand-receptor based colorimetric sensors, the interaction between ligands and receptors as indicators (fluorophore and chromophore) and analytes (cation/anion) can change the emission spectrum of the indicator and provide a colorimetric response to different concentrations of analyte (Zhu et al., 2021). The most common ligand or receptor is a Schiff base, which has been widely used in the manufacture of colorimetric chemical sensors to detect metal cations and other anions, for instance multifunctional Schiff bases have been utilized for the colorimetric detection of Fe2+, Zn2+, Cu2+ (Tang X. et al., 2017), Al3+, and cyanide (Lee et al., 2014). Moreover, some selected examples of the colorimetric detection mechanism of various colorimetric sensors and the used materials, as well as their specific detection applications are presented Table 6. The contents are summarized from five aspects: SPR changes, enzyme and mimic enzyme catalysis, fluorescence switches, ligand, and receptor binding.
Detecting electrodes that can generate a detectable electrical indication regarding electrochemical adsorption or reaction with analytes is standard for electrochemical identification of aqueous contaminants. A counter electrode, a working electrode and a reference electrode are also used in electrochemical sensing. Pollution will cause changes in resistance, potential, capacitance, or current both of which can be registered or analyzed. Electrochemical sensors based on 2D nanomaterials can detect other toxins in addition to heavy metals (Tiwari et al., 2016). Gas sensors may also be used to track water quality for hazardous contaminants such as NH3 or toluene. A detailed analysis of gas sensing with 2D nanomaterials could be found in a new review article, and we will briefly discuss a few fresh developments in water for gas sensing of common contaminants (Anichini et al., 2018). The surface chemistry of 2D nanomaterials can be manipulated to create gas sensors.
In field-effect transistors (FETs) for pollutant detection, 2D nanomaterials have gotten a lot of coverage. The adsorption of selected contaminants on 2D nano-sheets affects one or more core variables of the FETs, such as the Ion/Ioff ratio, field-effect mobility and threshold voltage in a standard FETs based sensor. Semiconducting nano-sheets, in particular, have gained a lot of attraction because of their high charge transporter mobility and acceptable band-gaps. Furthermore, the huge sideways scale of 2D nanomaterials allows for conformal interaction with metal electrodes, lowering in contact resistance in field-effect transistors-based sensors. FETs, for example, are quick to react, while fluorescent sensors are known for having a longer detection time. 2D nanomaterials-based FET sensors, in particular, are often characterized by compact dimensions and low energy consumption, making them ideal for use in embedded and wearable sensors (Mao et al., 2017; Tu et al., 2018).
Field-effect transistors dependent sensors for standard control and detection of pollutants in water have been developed using 2D nanomaterials. Due to its larger surface area, high carrier mobility and flexible chemical functionalization, graphene has gained a lot of intention as a symbolic 2D nanomaterial for graphene field-effect transistor (GFET) dependent sensors. A narrow film of safety layers like metal oxide is also required for water-sensitive 2D nanomaterials to avoid irreversible degradation of channel materials. An electrostatic double-layer appears on the surface of adsorbed target analytes during the study of aqueous contaminants, producing harmful effects on FETs sensors. The field formed by charged contaminants or gate potential is reduced by the firm electrostatic double layer (such as smaller Debye length) (Gao et al., 2015).
A host of problems must be overcome to understand the large-scale use of 2D nanomaterials in the purification of water. Since 2D nanomaterials are still in the prompt period of growth, technological challenges and manufacturing problems make their installation in industrial processes costly and limit their use to small-scale structures. The cost of manufacturing many 2D nanomaterials is still relatively high as compared to traditional products, so considerable cost savings is desirable. In addition, the long-term viability of 2D nanomaterial (both in terms of output and application) should be considered. Since water treatment is basically a decontamination procedure, the biocompatibility of 2D nanomaterials must ponder to prevent substance recontamination and environmentally safe 2D materials with lower toxicity (such as LDHs) should be prioritized. It would be essential to improve methods for maintaining specific nanochannels with long-term operating stability. For energetic water transport and high-performance solar desalination, creating larger throughput channels is critical. Thus, up to date self-assembly and guided aggregation schemes that shape sequence structures with high throughput channels could be extremely advantageous. The systematic modulation of material pollutant interaction is required for establishing new procreation of creative nanostructures that are programmable, adaptive, multifunctional, and selective for adsorption, photocatalysis, and sensing processes. Many pristine environments exhibit poor and unspecific encounters with contaminants (Mounet et al., 2018). Until now, the MXenes are mainly synthesized through a top-down route, and very limited literature is available on the manufacturing cost of synthesis of MXene using a bottom-up approach (Ihsanullah, 2021). Considerable attention is needed in this research trend to explore the novel bottom-up methods of MXene synthesis with more control of product characteristics. Storage of MXene requires sub-zero temperatures that is another hurdle for researchers to be overcome. Development of an efficient method is vital for storing MXene solution for a long time without oxidizing. The development of high throughput construction of stable 2D nanomaterials with adequate quality and lower cost is essential for mass commercialization. For large-scale deployment, production expense, processability, scalability stability, and consistency of 2D materials are all critical and often inter-dependent elements to remember (Zhong et al., 2015).
To summarizing all, several extensively utilized water purification techniques such as boiling, sedimentation, distillation, and oxidation, as well as solar and chemical disinfection, are presently incapable of supplying a cost-effective and reliable source of water to the world. Thus, an improved technology must be developed and industrialized to provide clean drinking water and 2D nanomaterials are useful for use in integrated membrane operations and water purification due to their inherent properties. Low-cost 2D material strategies focusing on high scalability and processability can be beneficial. Because of the use of inexpensive and multiple layered crystals, solution-based top-down exfoliation will achieve a scalable output of 2D materials at an affordable cost (Cai et al., 2018). In comparison to one-dimensional nanomaterials, which are often produced with the help of bottom-up techniques from molecular precursors like chemical vapor deposition growth, the top down exfoliation methodology for 2D nanomaterials is almost advanced and easily processed in an industrial setting by exfoliating bulk layered precursors with the help of sonication or shear force (Varrla et al., 2015; Stafford et al., 2018). Given that most of the 2D nanomaterials are produced from Earth plentiful elements such as carbon, nitrogen, boron, Mo, Sulphur, Ti, and so on. Biodiversity and the problem of materials shortages would be achievable if prudent steps like materials recovery are implemented. Continual use of 2D materials does not result in material deterioration, corrosion, injury, or secondary contamination from an application standpoint. As a result, device sustainability is also a critical consideration. Self-assembly of 2D nanomaterials can result in a variety of ordered nano-architectures, including both orientationally and positionally ordered structures (Davidson et al., 2018). According to the hard-soft-acid-base principle, transition metal dichalconides (such as MoS2 and MoSe2) nano-sheets have soft dangling groups such as S and Se, which react firmly with soft acids such as Hg2+ and Pb2+, while MXene surface groups including –F and –OH, which contribute to stronger adsorption on hard acids (Li et al., 2020). With the help of multi-component assemblies and superlattices of nanomaterials, chemical programmability can attain various functions such as the elimination of various pollutants in a single material system. By mixing photocatalysts and membranes, organic foulants can be photo-catalytically degraded, reducing organic fouling in membrane isolation (Runnan Zhang et al., 2016). In addition, the naturally abundant elements that make-up many nanomaterials such as MXenes may be advantageous for cost-sensitive environmental applications. More investigations are also required to improve stability and achieve recyclability of 2D nanomaterials adsorbents, so that consistent performance is achieved even after repeated use. More studies are needed to bridge the gap between the newly predicted and experimentally available 2D nanomaterials with the hope of identifying candidate materials with enhanced thermal, electrical, and catalytic properties that are more suitable for water treatment and environmental remediation. Experimental verification of theoretical predictions is an important task that may facilitate the development of materials with improved photocatalytic, electrocatalytic and other useful properties.
MBT, MT, and AS conceived this review, and JF and AS drafted and finalized the paper. TM helped to improve the draft by providing useful suggestions and information. All authors approved the work for publication.
The authors declare that the research was conducted in the absence of any commercial or financial relationships that could be construed as a potential conflict of interest.
All claims expressed in this article are solely those of the authors and do not necessarily represent those of their affiliated organizations, or those of the publisher, the editors and the reviewers. Any product that may be evaluated in this article, or claim that may be made by its manufacturer, is not guaranteed or endorsed by the publisher.
The authors would like to extend their sincere appreciation to the Researchers Supporting Project Number (RSP-2021/168), King Saud University, Riyadh, Saudi Arabia.
Abbas, A., Al-Amer, A. M., Laoui, T., Al-Marri, M. J., Nasser, M. S., Khraisheh, M., et al. (2016). Heavy Metal Removal from Aqueous Solution by Advanced Carbon Nanotubes: Critical Review of Adsorption Applications. Sep. Purif. Tech. 157, 141–161.
Ahmed, F., and Rodrigues, D. F. (2013). Investigation of Acute Effects of Graphene Oxide on Wastewater Microbial Community: a Case Study. J. Hazard. Mater. 256-257, 33–39. doi:10.1016/j.jhazmat.2013.03.064
Akinpelu, A. A., Ali, M. E., Johan, M. R., Saidur, R., Qurban, M. A., and Saleh, T. A. (2019). Polycyclic Aromatic Hydrocarbons Extraction and Removal from Wastewater by Carbon Nanotubes: A Review of the Current Technologies, Challenges and Prospects. Process Saf. Environ. Prot. 122, 68–82. doi:10.1016/j.psep.2018.11.006
Anasori, B., Xie, Y., Beidaghi, M., Lu, J., Hosler, B. C., Hultman, L., et al. (2015). Two-dimensional, Ordered, Double Transition Metals Carbides (MXenes). ACS nano 9 (10), 9507–9516. doi:10.1021/acsnano.5b03591
Anichini, C., Czepa, W., Pakulski, D., Aliprandi, A., Ciesielski, A., and Samorì, P. (2018). Chemical Sensing with 2D Materials. Chem. Soc. Rev. 47 (13), 4860–4908. doi:10.1039/C8CS00417J
Ashbolt, N. J. (2015). Microbial Contamination of Drinking Water and Human Health from Community Water Systems. Curr. Envir Health Rpt 2 (1), 95–106. doi:10.1007/s40572-014-0037-5
Bai, S., Ge, J., Wang, L., Gong, M., Deng, M., Kong, Q., et al. (2014). A Unique Semiconductor-Metal-Graphene Stack Design to Harness Charge Flow for Photocatalysis. Adv. Mater. 26 (32), 5689–5695. doi:10.1002/adma.201401817
Baig, N., Ihsanullah, M., Sajid, M., and Saleh, T. A. (2019). Graphene-based Adsorbents for the Removal of Toxic Organic Pollutants: A Review. J. Environ. Manag. 244, 370–382. doi:10.1016/j.jenvman.2019.05.047
Barsoum, M. W. (2013). MAX Phases: Properties of Machinable Ternary Carbides and Nitrides. John Wiley & Sons.
Bellah, M., Christensen, S. M., and Iqbal, S. M. (20122012). Nanostructures for Medical Diagnostics. J. Nanomater. doi:10.1155/2012/486301
Bhimanapati, G. R., Lin, Z., Meunier, V., Jung, Y., Cha, J., Das, S., et al. (2015). Recent Advances in Two-Dimensional Materials beyond Graphene. ACS nano 9 (12), 11509–11539. doi:10.1021/acsnano.5b05556
Bi, H., Wang, X., Liu, H., He, Y., Wang, W., Deng, W., et al. (2020). A Universal Approach to Aqueous Energy Storage via Ultralow‐Cost Electrolyte with Super‐Concentrated Sugar as Hydrogen‐Bond‐Regulated Solute. Adv. Mater. 32 (16), 2000074. doi:10.1002/adma.202000074
Bilal, M., Rasheed, T., Iqbal, H. M. N., and Yan, Y. (2018). Peroxidases-assisted Removal of Environmentally-Related Hazardous Pollutants with Reference to the Reaction Mechanisms of Industrial Dyes. Sci. total Environ. 644, 1–13. doi:10.1016/j.scitotenv.2018.06.274
Biswas, A., Bayer, I. S., Biris, A. S., Wang, T., Dervishi, E., and Faupel, F. (2012). Advances in Top-Down and Bottom-Up Surface Nanofabrication: Techniques, Applications & Future Prospects. Adv. Colloid Interf. Sci 170 (1-2), 2–27. doi:10.1016/j.cis.2011.11.001
Bolan, N., Hoang, S. A., Tanveer, M., Wang, L., Bolan, S., Sooriyakumar, P., et al. (2021). From Mine to Mind and Mobiles - Lithium Contamination and its Risk Management. Environ. Pollut. 290, 118067. doi:10.1016/j.envpol.2021.118067
Bonadonna, L., La Rosa, G., and health, p. (2019). A Review and Update on Waterborne Viral Diseases Associated with Swimming Pools. Ijerph 16 (2), 166. doi:10.3390/ijerph16020166
Bukhtiyarova, M. V. (2019). A Review on Effect of Synthesis Conditions on the Formation of Layered Double Hydroxides. J. Solid State. Chem. 269, 494–506. doi:10.1016/j.jssc.2018.10.018
Cai, X., Luo, Y., Liu, B., and Cheng, H.-M. (2018). Preparation of 2D Material Dispersions and Their Applications. Chem. Soc. Rev. 47 (16), 6224–6266. doi:10.1039/C8CS00254A
Cao, B., Cao, S., Dong, P., Gao, J., and Wang, J. (2013). High Antibacterial Activity of Ultrafine TiO2/graphene Sheets Nanocomposites under Visible Light Irradiation. Mater. Lett. 93, 349–352. doi:10.1016/j.matlet.2012.11.136
Chaiyo, S., Mehmeti, E., Žagar, K., Siangproh, W., Chailapakul, O., and Kalcher, K. (2016). Electrochemical Sensors for the Simultaneous Determination of Zinc, Cadmium and lead Using a Nafion/ionic Liquid/graphene Composite Modified Screen-Printed Carbon Electrode. Analytica Chim. Acta 918, 26–34. doi:10.1016/j.aca.2016.03.026
Chen, Z., Chen, X., Di, J., Liu, Y., Yin, S., Xia, J., et al. (2017). Graphene-like boron Nitride Modified Bismuth Phosphate Materials for Boosting Photocatalytic Degradation of Enrofloxacin. J. Colloid Interf. Sci. 492, 51–60. doi:10.1016/j.jcis.2016.12.050
Chen, J., Peng, H., Wang, X., Shao, F., Yuan, Z., and Han, H. (2014). Graphene Oxide Exhibits Broad-Spectrum Antimicrobial Activity against Bacterial Phytopathogens and Fungal Conidia by Intertwining and Membrane Perturbation. Nanoscale 6 (3), 1879–1889. doi:10.1039/c3nr04941h
Chen, L., Cao, L., Ji, X., Hou, S., Li, Q., Chen, J., et al. (2020). Enabling Safe Aqueous Lithium Ion Open Batteries by Suppressing Oxygen Reduction Reaction. Nat. Commun. 11 (1), 2638. doi:10.1038/s41467-020-16460-w
Chen, Y., Fan, Z., Zhang, Z., Niu, W., Li, C., Yang, N., et al. (2018). Two-Dimensional Metal Nanomaterials: Synthesis, Properties, and Applications. Chem. Rev. 118 (13), 6409–6455. doi:10.1021/acs.chemrev.7b00727
Choi, J., Reddy, D. A., and Kim, T. K. (2015). Enhanced Photocatalytic Activity and Anti-photocorrosion of AgI Nanostructures by Coupling with Graphene-Analogue boron Nitride Nanosheets. Ceramics Int. 41 (10), 13793–13803. doi:10.1016/j.ceramint.2015.08.062
Cohen-Tanugi, D., and Grossman, J. C. (2015). Nanoporous Graphene as a Reverse Osmosis Membrane: Recent Insights from Theory and Simulation. Desalination 366, 59–70. doi:10.1016/j.desal.2014.12.046
Corcoll, N., Acuña, V., Barceló, D., Casellas, M., Guasch, H., Huerta, B., ..., , Petrovic, M., Ponsatí, L., Rodríguez-Mozaz, S., and Sabater, S. (2014). Pollution-induced Community Tolerance to Non-steroidal Anti-inflammatory Drugs (NSAIDs) in Fluvial Biofilm Communities Affected by WWTP Effluents. Chemosphere 112, 185–193. doi:10.1016/j.chemosphere.2014.03.128
Cui, J., Xu, S., and Wang, L. (2017). Monolayer MoS2 Decorated Cu7S4-Au Nanocatalysts for Sensitive and Selective Detection of Mercury(II). Sci. China Mater. 60 (4), 352–360. doi:10.1007/s40843-017-9019-4
Davidson, P., Penisson, C., Constantin, D., and Gabriel, J.-C. P. (2018). Isotropic, Nematic, and Lamellar Phases in Colloidal Suspensions of Nanosheets. Proc. Natl. Acad. Sci. USA 115, 6662–6667. doi:10.1073/pnas.1802692115
Dehghani, M. H., Mostofi, M., Alimohammadi, M., McKay, G., Yetilmezsoy, K., Albadarin, A. B., et al. (2016). High-performance Removal of Toxic Phenol by Single-Walled and Multi-Walled Carbon Nanotubes: Kinetics, Adsorption, Mechanism and Optimization Studies. J. Ind. Eng. Chem. 35, 63–74. doi:10.1016/j.jiec.2015.12.010
de Melo, B. A. G., Motta, F. L., and Santana, M. H. A. (2016). Humic Acids: Structural Properties and Multiple Functionalities for Novel Technological Developments. Mater. Sci. Eng. C 62, 967–974. doi:10.1016/j.msec.2015.12.001
Dervin, S., Dionysiou, D. D., and Pillai, S. C. (2016). 2D Nanostructures for Water Purification: Graphene and beyond. Nanoscale 8 (33), 15115–15131. doi:10.1039/c6nr04508a
Dhakal, C., Aryal, S., Sakidja, R., and Ching, W.-Y. (2015). Approximate Lattice thermal Conductivity of MAX Phases at High Temperature. J. Eur. Ceram. Soc. 35 (12), 3203–3212. doi:10.1016/j.jeurceramsoc.2015.04.013
Ding, Y., Kuhlbusch, T. A. J., Van Tongeren, M., Jiménez, A. S., Tuinman, I., Chen, R., et al. (2017). Airborne Engineered Nanomaterials in the Workplace-A Review of Release and Worker Exposure during Nanomaterial Production and Handling Processes. J. Hazard. Mater. 322, 17–28. doi:10.1016/j.jhazmat.2016.04.075
Douterelo, I., Jackson, M., Solomon, C., and Boxall, J. (2017). Spatial and Temporal Analogies in Microbial Communities in Natural Drinking Water Biofilms. Sci. Total Environ. 581-582, 277–288. doi:10.1016/j.scitotenv.2016.12.118
Dreyer, D. R., Park, S., Bielawski, C. W., and Ruoff, R. S. (2010). The Chemistry of Graphene Oxide. Chem. Soc. Rev. 39 (1), 228–240. doi:10.1039/b917103g
Eftekhari, A. (2017). Tungsten Dichalcogenides (WS2, WSe2, and WTe2): Materials Chemistry and Applications. J. Mater. Chem. A. 5 (35), 18299–18325. doi:10.1039/c7ta04268j
Efstratiou, A., Ongerth, J. E., and Karanis, P. (2017). Waterborne Transmission of Protozoan Parasites: Review of Worldwide Outbreaks - an Update 2011-2016. Water Res. 114, 14–22. doi:10.1016/j.watres.2017.01.036
Fang, H., Bai, S.-L., and Wong, C. P. (2016). "White Graphene" - Hexagonal boron Nitride Based Polymeric Composites and Their Application in thermal Management. Composites Commun. 2, 19–24. doi:10.1016/j.coco.2016.10.002
Fang, Q., Wang, G., Xue, B., Liu, T., and Kiem, A. (2018). How and to what Extent Does Precipitation on Multi-Temporal Scales and Soil Moisture at Different Depths Determine Carbon Flux Responses in a Water-Limited Grassland Ecosystem? Sci. Total Environ. 635, 1255–1266. doi:10.1016/j.scitotenv.2018.04.225
Farka, Z., Juřík, T., Kovář, D., Trnková, L., and Skládal, P. (2017). Nanoparticle-Based Immunochemical Biosensors and Assays: Recent Advances and Challenges. Chem. Rev. 117 (15), 9973–10042. doi:10.1021/acs.chemrev.7b00037
Feng, Y., Zhou, Y., Lee, P.-H., and Shih, K. (2016). Mineralization of Perfluorooctanesulfonate (PFOS) and Perfluorodecanoate (PFDA) from Aqueous Solution by Porous Hexagonal boron Nitride: Adsorption Followed by Simultaneous thermal Decomposition and Regeneration. RSC Adv. 6 (114), 113773–113780. doi:10.1039/c6ra15564b
Forgacs, E., Cserháti, T., and Oros, G. (2004). Removal of Synthetic Dyes from Wastewaters: a Review. Environ. Int. 30 (7), 953–971. doi:10.1016/j.envint.2004.02.001
Fu, F., and Wang, Q. (2011). Removal of Heavy Metal Ions from Wastewaters: a Review. J. Environ. Manag. 92 (3), 407–418. doi:10.1016/j.jenvman.2010.11.011
Fu, Y., Li, L., Xie, B., Dong, C., Wang, M., Jia, B., et al. (2016). How to Establish a Bioregenerative Life Support System for Long-Term Crewed Missions to the Moon or Mars. Astrobiology 16 (12), 925–936. doi:10.1089/ast.2016.1477
Gao, X., Li, Z.-K., Xue, J., Qian, Y., Zhang, L.-Z., Caro, J., et al. (2019). Titanium Carbide Ti3C2Tx (MXene) Enhanced PAN Nanofiber Membrane for Air Purification. J. Membr. Sci. 586, 162–169. doi:10.1016/j.memsci.2019.05.058
Gao, N., Zhou, W., Jiang, X., Hong, G., Fu, T.-M., and Lieber, C. M. (2015). General Strategy for Biodetection in High Ionic Strength Solutions Using Transistor-Based Nanoelectronic Sensors. Nano Lett. 15 (3), 2143–2148. doi:10.1021/acs.nanolett.5b00133
Gao, W., Majumder, M., Alemany, L. B., Narayanan, T. N., Ibarra, M. A., Pradhan, B. K., et al. (2011). Engineered Graphite Oxide Materials for Application in Water Purification. ACS Appl. Mater. Inter. 3 (6), 1821–1826. doi:10.1021/am200300u
Gao, J., Zhang, M., Wang, J., Liu, G., Liu, H., and Jiang, Y. (2019). Bioinspired Modification of Layer-Stacked Molybdenum Disulfide (MoS2) Membranes for Enhanced Nanofiltration Performance. ACS omega 4 (2), 4012–4022. doi:10.1021/acsomega.9b00155
Ge, J., Shi, L.-A., Wang, Y.-C., Zhao, H.-Y., Yao, H.-B., Zhu, Y.-B., et al. (2017). Joule-heated Graphene-Wrapped Sponge Enables Fast Clean-Up of Viscous Crude-Oil Spill. Nat. Nanotech 12 (5), 434–440. doi:10.1038/nnano.2017.33
Geim, A. K., and Novoselov, K. S. (2010). “The Rise of Graphene,” in Nanoscience and Technology: A Collection of Reviews from Nature Journals (World Scientific), 11–19.
Ghidiu, M., Naguib, M., Shi, C., Mashtalir, O., Pan, L. M., Zhang, B., et al. (2014). Synthesis and Characterization of Two-Dimensional Nb4C3 (MXene). Chem. Commun. 50 (67), 9517–9520. doi:10.1039/c4cc03366c
Goh, K., Setiawan, L., Wei, L., Si, R., Fane, A. G., Wang, R., et al. (2015). Graphene Oxide as Effective Selective Barriers on a Hollow Fiber Membrane for Water Treatment Process. J. Membr. Sci. 474, 244–253. doi:10.1016/j.memsci.2014.09.057
Goh, P. S., and Ismail, A. F. (2015). Graphene-based Nanomaterial: The State-Of-The-Art Material for Cutting Edge Desalination Technology. Desalination 356, 115–128. doi:10.1016/j.desal.2014.10.001
Golberg, D., Bando, Y., Huang, Y., Terao, T., Mitome, M., Tang, C., et al. (2010). Boron Nitride Nanotubes and Nanosheets. ACS nano 4 (6), 2979–2993. doi:10.1021/nn1006495
Gonzalez-Ortiz, D., Salameh, C., Bechelany, M., and Miele, P. (2020). Nanostructured boron Nitride-Based Materials: Synthesis and Applications. Mater. Today Adv. 8, 100107. doi:10.1016/j.mtadv.2020.100107
Gu, W., Pei, X., Cheng, Y., Zhang, C., Zhang, J., Yan, Y., et al. (2017). Black Phosphorus Quantum Dots as the Ratiometric Fluorescence Probe for Trace Mercury Ion Detection Based on Inner Filter Effect. ACS Sens. 2 (4), 576–582. doi:10.1021/acssensors.7b00102
Guo, L., Wang, X., Leong, Z. Y., Mo, R., Sun, L., and Yang, H. Y. (2018). Ar Plasma Modification of 2D MXene Ti 3 C 2 T X Nanosheets for Efficient Capacitive Desalination. FlatChem 8, 17–24. doi:10.1016/j.flatc.2018.01.001
Guo, Z., Zhou, J., Zhu, L., and Sun, Z. (2016). MXene: a Promising Photocatalyst for Water Splitting. J. Mater. Chem. A. 4 (29), 11446–11452. doi:10.1039/c6ta04414j
Han, R., Ma, X., Xie, Y., Teng, D., and Zhang, S. (2017). Preparation of a New 2D MXene/PES Composite Membrane with Excellent Hydrophilicity and High Flux. RSC Adv. 7 (89), 56204–56210. doi:10.1039/c7ra10318b
Han, C., Yang, M.-Q., Zhang, N., and Xu, Y.-J. (2014). Enhancing the Visible Light Photocatalytic Performance of Ternary CdS-(graphene-Pd) Nanocomposites via a Facile Interfacial Mediator and Co-catalyst Strategy. J. Mater. Chem. A. 2 (45), 19156–19166. doi:10.1039/c4ta04151h
Han, Y., Xu, Z., and Gao, C. (2013). Ultrathin Graphene Nanofiltration Membrane for Water Purification. Adv. Funct. Mater. 23 (29), 3693–3700. doi:10.1002/adfm.201202601
Haque, F., Daeneke, T., Kalantar-zadeh, K., and Ou, J. Z. (2017). Two-Dimensional Transition Metal Oxide and Chalcogenide-Based Photocatalysts. Nano-micro Lett. 10 (2), 23. doi:10.1007/s40820-017-0176-y
Heidari, H. (2012). Effect of Irrigation by Contaminated Water with Cloth Detergent on Plant Growth and Seed Germination Traits of maize (Zea mays). Life Sci. J. 9 (4), 1587–1590.
Holt, M. S. (2000). Sources of Chemical Contaminants and Routes into the Freshwater Environment. Food Chem. Toxicol. 38, S21–S27. doi:10.1016/s0278-6915(99)00136-2
Holinger, E. P., Ross, K. A., Robertson, C. E., Stevens, M. J., Harris, J. K., and Pace, N. R. (2014). Molecular Analysis of point-of-use Municipal Drinking Water Microbiology. Water Res. 49, 225–235. doi:10.1016/j.watres.2013.11.027
Homaeigohar, S., and Elbahri, M. (2017). Graphene Membranes for Water Desalination. NPG Asia Mater. 9 (8), e427. doi:10.1038/am.2017.135
Hu, W., Peng, C., Luo, W., Lv, M., Li, X., Li, D., et al. (2010). Graphene-based Antibacterial Paper. ACS nano 4 (7), 4317–4323. doi:10.1021/nn101097v
Huang, T., Jiang, Y., Shen, G., and Chen, D. (2020). Recent Advances of 2D Nanomaterials for Electrochemical Capacitors. ChemSusChem.
Ibrahim, S. M., Nagasawa, H., Kanezashi, M., and Tsuru, T. (2015). Robust Organosilica Membranes for High Temperature Reverse Osmosis (RO) Application: Membrane Preparation, Separation Characteristics of Solutes and Membrane Regeneration. J. Membr. Sci. 493, 515–523. doi:10.1016/j.memsci.2015.06.060
Ihsanullah, I. (2021). Boron Nitride-Based Materials for Water Purification: Progress and Outlook. Chemosphere 263, 127970. doi:10.1016/j.chemosphere.2020.127970
Ihsanullah, I. (2020). MXenes (Two-dimensional Metal Carbides) as Emerging Nanomaterials for Water Purification: Progress, Challenges and Prospects. Chem. Eng. J. 388, 124340. doi:10.1016/j.cej.2020.124340
Ikram, M., Khan, M. I., Raza, A., Imran, M., Ul-Hamid, A., and Ali, S. (2020). Outstanding Performance of Silver-Decorated MoS2 Nanopetals Used as Nanocatalyst for Synthetic Dye Degradation. Physica E: Low-dimensional Syst. Nanostructures 124, 114246. doi:10.1016/j.physe.2020.114246
Jastrzębska, A. M., Karwowska, E., Wojciechowski, T., Ziemkowska, W., Rozmysłowska, A., Chlubny, L., et al. (2019). The Atomic Structure of Ti 2 C and Ti 3 C 2 MXenes Is Responsible for Their Antibacterial Activity toward E. coli Bacteria. J. Mater. Eng. Perform. 28 (3), 1272–1277.
Jeong, G. H., Sasikala, S. P., Yun, T., Lee, G. Y., Lee, W. J., and Kim, S. O. (2020). Nanoscale Assembly of 2D Materials for Energy and Environmental Applications. Adv. Mater. 32 (35), 1907006. doi:10.1002/adma.201907006
Jia, F., Wang, Q., Wu, J., Li, Y., and Song, S. (2017). Two-Dimensional Molybdenum Disulfide as a Superb Adsorbent for Removing Hg2+ from Water. ACS Sust. Chem. Eng. 5 (8), 7410–7419. doi:10.1021/acssuschemeng.7b01880
Jiang, S., Cheng, R., Ng, R., Huang, Y., and Duan, X. (2015). Highly Sensitive Detection of Mercury(II) Ions with Few-Layer Molybdenum Disulfide. Nano Res. 8 (1), 257–262. doi:10.1007/s12274-014-0658-x
Jureczko, M., and Kalka, J. (2020). Cytostatic Pharmaceuticals as Water Contaminants. Eur. J. Pharmacol. 866, 172816. doi:10.1016/j.ejphar.2019.172816
Karfa, P., Chandra Majhi, K., and Madhuri, R. (2020). “Synthesis of Two-Dimensional Nanomaterials,” in Two-Dimensional Nanostructures for Biomedical Technology. Editors R. Khan, and S. Barua (Elsevier), 35–71. doi:10.1016/b978-0-12-817650-4.00002-4
Kang, Z., Wang, S., Fan, L., Xiao, Z., Wang, R., and Sun, D. (2017). Surface Wettability Switching of Metal-Organic Framework Mesh for Oil-Water Separation. Mater. Lett. 189, 82–85. doi:10.1016/j.matlet.2016.11.088
Khan, S. T., and Malik, A. (2019). Engineered Nanomaterials for Water Decontamination and Purification: From Lab to Products. J. Hazard. Mater. 363, 295–308. doi:10.1016/j.jhazmat.2018.09.091
Kumar, K. Y., Muralidhara, H. B., Nayaka, Y. A., Balasubramanyam, J., and Hanumanthappa, H. (2013). Low-cost Synthesis of Metal Oxide Nanoparticles and Their Application in Adsorption of Commercial Dye and Heavy Metal Ion in Aqueous Solution. Powder Technol. 246, 125–136. doi:10.1016/j.powtec.2013.05.017
Kumar, P., Pournara, A., Kim, K.-H., Bansal, V., Rapti, S., and Manos, M. J. (2017). Metal-organic Frameworks: Challenges and Opportunities for Ion-Exchange/sorption Applications. Prog. Mater. Sci. 86, 25–74. doi:10.1016/j.pmatsci.2017.01.002
Lee, S. A., You, G. R., Choi, Y. W., Jo, H. Y., Kim, A. R., Noh, I., et al. (2014). A New Multifunctional Schiff Base as a Fluorescence Sensor for Al3+ and a Colorimetric Sensor for CN− in Aqueous media: an Application to Bioimaging. Dalton Trans. 43 (18), 6650–6659. doi:10.1039/c4dt00361f
Lee, K. S. (2011). Three Ways of Linking Laboratory Endeavours to the Realm of Policies. The Eur. J. Hist. Econ. Thought 18 (5), 755–776. doi:10.1080/09672567.2011.616593
Li, B. L., Setyawati, M. I., Chen, L., Xie, J., Ariga, K., Lim, C.-T., et al. (2017). Directing Assembly and Disassembly of 2D MoS2 Nanosheets with DNA for Drug Delivery. ACS Appl. Mater. Inter. 9 (18), 15286–15296. doi:10.1021/acsami.7b02529
Li, P., Zhang, D., Jiang, C., Zong, X., and Cao, Y. (2017). Ultra-sensitive Suspended Atomically Thin-Layered Black Phosphorus Mercury Sensors. Biosens. Bioelectron. 98, 68–75. doi:10.1016/j.bios.2017.06.027
Li, P., Zhang, D., Liu, J., Chang, H., Sun, Y. e., and Yin, N. (2015). Air-Stable Black Phosphorus Devices for Ion Sensing. ACS Appl. Mater. Inter. 7 (44), 24396–24402. doi:10.1021/acsami.5b07712
Li, X., Ma, W., Li, H., Zhang, Q., and Liu, H. (2020). Sulfur-functionalized Metal-Organic Frameworks: Synthesis and Applications as Advanced Adsorbents. Coord. Chem. Rev. 408, 213191. doi:10.1016/j.ccr.2020.213191
Lim, J. Y., Mubarak, N. M., Abdullah, E. C., Nizamuddin, S., Khalid, M., and Inamuddin, (2018). Recent Trends in the Synthesis of Graphene and Graphene Oxide Based Nanomaterials for Removal of Heavy Metals - A Review. J. Ind. Eng. Chem. 66, 29–44. doi:10.1016/j.jiec.2018.05.028
Lin, L., Jiang, W., Bechelany, M., Nasr, M., Jarvis, J., Schaub, T., et al. (2019). Adsorption and Photocatalytic Oxidation of Ibuprofen Using Nanocomposites of TiO2 Nanofibers Combined with BN Nanosheets: Degradation Products and Mechanisms. Chemosphere 220, 921–929. doi:10.1016/j.chemosphere.2018.12.184
Lipczynska-Kochany, E. (2018). Humic Substances, Their Microbial Interactions and Effects on Biological Transformations of Organic Pollutants in Water and Soil: A Review. Chemosphere 202, 420–437. doi:10.1016/j.chemosphere.2018.03.104
Liu, X., Wang, X., Han, Q., Qi, C., Wang, C., and Yang, R. (2019). Facile Synthesis of IrO2/rGO Nanocomposites with High Peroxidase-like Activity for Sensitive Colorimetric Detection of Low Weight Biothiols. Talanta 203, 227–234. doi:10.1016/j.talanta.2019.05.070
Liu, A., Wang, C.-Z., Chu, C., Chu, H.-Y., Chen, X., Du, A.-F., et al. (2018). Adsorption Performance toward Organic Pollutants, Odour Control and Anti-microbial Activities of One Ag-Based Coordination Polymer. J. Environ. Chem. Eng. 6 (4), 4961–4969. doi:10.1016/j.jece.2018.07.035
Liu, A., Wang, C.-Z., Chu, C., Chu, H.-Y., Chen, X., Du, A.-F., et al. (2018a). Adsorption Performance toward Organic Pollutants, Odour Control and Anti-microbial Activities of One Ag-Based Coordination Polymer. J. Environ. Chem. Eng. 6 (4), 4961–4969. doi:10.1016/j.jece.2018.07.035
Liu, C., Jia, F., Wang, Q., Yang, B., and Song, S. (2017). Two-dimensional Molybdenum Disulfide as Adsorbent for High-Efficient Pb(II) Removal from Water. Appl. Mater. Today 9, 220–228. doi:10.1016/j.apmt.2017.07.009
Liu, C., Kong, D., Hsu, P.-C., Yuan, H., Lee, H.-W., Liu, Y., et al. (2016). Rapid Water Disinfection Using Vertically Aligned MoS2 Nanofilms and Visible Light. Nat. Nanotech 11 (12), 1098–1104. doi:10.1038/nnano.2016.138
Liu, C., Sun, Z., Zhang, L., Lv, J., Yu, X. F., Zhang, L., et al. (2018). Black Phosphorus Integrated Tilted Fiber Grating for Ultrasensitive Heavy Metal Sensing. Sensors Actuators B: Chem. 257, 1093–1098. doi:10.1016/j.snb.2017.11.022
Liu, J. L., and Bashir, S. (2015). Advanced Nanomaterials and Their Applications in Renewable Energy.
Liu, J., Liu, Y., Liu, N., Han, Y., Zhang, X., Huang, H., et al. (2015). Metal-free Efficient Photocatalyst for Stable Visible Water Splitting via a Two-Electron Pathway. Science 347 (6225), 970–974. doi:10.1126/science.aaa3145
Liu, X., Li, L., Wei, Y., Zheng, Y., Xiao, Q., and Feng, B. (2015). Facile Synthesis of boron- and Nitride-Doped MoS2 Nanosheets as Fluorescent Probes for the Ultrafast, Sensitive, and Label-free Detection of Hg2+. Analyst 140 (13), 4654–4661. doi:10.1039/C5AN00641D
Liu, Y., Huang, Y., and Duan, X. (2019). Van der Waals integration before and beyond two-dimensional materials. Nature 567 (7748), 323–333. doi:10.1038/s41586-019-1013-x
Lukatskaya, M. R., Mashtalir, O., Ren, C. E., Dall’Agnese, Y., Rozier, P., Taberna, P. L., et al. (2013). Cation Intercalation and High Volumetric Capacitance of Two-Dimensional Titanium Carbide. Science 341 (6153), 1502–1505. doi:10.1126/science.1241488
Luo, J., Matios, E., Wang, H., Tao, X., and Li, W. (2020). Interfacial Structure Design of MXene‐based Nanomaterials for Electrochemical Energy Storage and Conversion. InfoMat 2 (6), 1057–1076. doi:10.1002/inf2.12118
Luo, B., Liu, G., and Wang, L. (2016). Recent Advances in 2D Materials for Photocatalysis. Nanoscale 8 (13), 6904–6920. doi:10.1039/C6NR00546B
Low, W.-P., Din, M. F. M., Chang, F.-L., Moideen, S. N. F. B., and Lee, Y. Y. (2018). Empirical Models of Kinetic Rate for River Treatment Analysis of Cellulosic Materials. J. Water Process Eng. 23, 257–264. doi:10.1016/j.jwpe.2018.04.011
Mahmoud, K. A., Mansoor, B., Mansour, A., and Khraisheh, M. (2015). Functional Graphene Nanosheets: The Next Generation Membranes for Water Desalination. Desalination 356, 208–225. doi:10.1016/j.desal.2014.10.022
Mahdavian, A. R., and Mirrahimi, M. A.-S. (2010). Efficient Separation of Heavy Metal Cations by Anchoring Polyacrylic Acid on Superparamagnetic Magnetite Nanoparticles through Surface Modification. Chem. Eng. J. 159 (1), 264–271. doi:10.1016/j.cej.2010.02.041
Manzeli, S., Ovchinnikov, D., Pasquier, D., Yazyev, O. V., and Kis, A. (2017). 2D Transition Metal Dichalcogenides. Nat. Rev. Mater. 2 (8), 17033. doi:10.1038/natrevmats.2017.33
Mao, K., Wu, Z., Chen, Y., Zhou, X., Shen, A., and Hu, J. (2015). A Novel Biosensor Based on Single-Layer MoS2 Nanosheets for Detection of Ag+. Talanta 132, 658–663. doi:10.1016/j.talanta.2014.10.026
Mao, P., Yu, B., Liu, Z., Wang, F., and Ju, Y. (2015). First-principles Investigation on Mechanical, Electronic, and Thermodynamic Properties of Mg2Sr under High Pressure. J. Appl. Phys. 117 (11), 115903. doi:10.1063/1.4915339
Mao, S., Chang, J., Pu, H., Lu, G., He, Q., Zhang, H., et al. (2017). Two-dimensional Nanomaterial-Based Field-Effect Transistors for Chemical and Biological Sensing. Chem. Soc. Rev. 46 (22), 6872–6904. doi:10.1039/C6CS00827E
Mashtalir, O., Cook, K. M., Mochalin, V. N., Crowe, M., Barsoum, M. W., and Gogotsi, Y. (2014). Dye Adsorption and Decomposition on Two-Dimensional Titanium Carbide in Aqueous media. J. Mater. Chem. A. 2 (35), 14334–14338. doi:10.1039/c4ta02638a
Mashtalir, O., Naguib, M., Mochalin, V. N., Dall'Agnese, Y., Heon, M., Barsoum, M. W., et al. (2013). Intercalation and Delamination of Layered Carbides and Carbonitrides. Nat. Commun. 4 (1), 1716–1717. doi:10.1038/ncomms2664
Mayerberger, E. A., Street, R. M., McDaniel, R. M., Barsoum, M. W., and Schauer, C. L. (2018). Antibacterial Properties of Electrospun Ti3C2Tz(MXene)/chitosan Nanofibers. RSC Adv. 8 (62), 35386–35394. doi:10.1039/c8ra06274a
Miara, A., Vörösmarty, C. J., Macknick, J. E., Tidwell, V. C., Fekete, B., Corsi, F., et al. (2018). Thermal Pollution Impacts on Rivers and Power Supply in the Mississippi River Watershed. Environ. Res. Lett. 13 (3), 034033. doi:10.1088/1748-9326/aaac85
MiarAlipour, S., Friedmann, D., Scott, J., and Amal, R. (2018). TiO2/porous Adsorbents: Recent Advances and Novel Applications. J. Hazard. Mater. 341, 404–423. doi:10.1016/j.jhazmat.2017.07.070
Mohapatra, L., and Parida, K. (2016). A Review on the Recent Progress, Challenges and Perspective of Layered Double Hydroxides as Promising Photocatalysts. J. Mater. Chem. A. 4 (28), 10744–10766. doi:10.1039/C6TA01668E
Mousavi, S. A., and Khodadoost, F. (2019). Effects of Detergents on Natural Ecosystems and Wastewater Treatment Processes: a Review. Environ. Sci. Pollut. Res. 26 (26), 26439–26448. doi:10.1007/s11356-019-05802-x
Mounet, N., Gibertini, M., Schwaller, P., Campi, D., Merkys, A., Marrazzo, A., et al. (2018). Two-dimensional Materials from High-Throughput Computational Exfoliation of Experimentally Known Compounds. Nat. Nanotech 13 (3), 246–252. doi:10.1038/s41565-017-0035-5
Naguib, M., Mashtalir, O., Carle, J., Presser, V., Lu, J., Hultman, L., et al. (2012). Two-Dimensional Transition Metal Carbides. ACS Nano. 6 (2), 1322–1331.
Naguib, M., Mashtalir, O., Lukatskaya, M. R., Dyatkin, B., Zhang, C., Presser, V., et al. (2014). One-step Synthesis of Nanocrystalline Transition Metal Oxides on Thin Sheets of Disordered Graphitic Carbon by Oxidation of MXenes. Chem. Commun. 50 (56), 7420–7423. doi:10.1039/c4cc01646g
Nicolaï, A., Sumpter, B. G., and Meunier, V. (2014). Tunable Water Desalination across Graphene Oxide Framework Membranes. Phys. Chem. Chem. Phys. 16 (18), 8646–8654.
Nieto-Delgado, C., Partida-Gutierrez, D., and Rangel-Mendez, J. R. (2019). Preparation of Activated Carbon Cloths from Renewable Natural Fabrics and Their Performance during the Adsorption of Model Organic and Inorganic Pollutants in Water. J. Clean. Prod. 213, 650–658. doi:10.1016/j.jclepro.2018.12.184
Nirala, N. R., Pandey, S., Bansal, A., Singh, V. K., Mukherjee, B., Saxena, P. S., et al. (2015). Different Shades of Cholesterol: Gold Nanoparticles Supported on MoS2 Nanoribbons for Enhanced Colorimetric Sensing of Free Cholesterol. Biosens. Bioelectron. 74, 207–213. doi:10.1016/j.bios.2015.06.043
NHMRC (2011). Australian Drinking Water Guidelines Paper 6 National Water Quality Management Strategy. Canberra: National Health and Medical Research Council, National Resource Management Ministerial Council, Commonwealth of Australia, 5–7.
Noah, N. M. (2020). Design and Synthesis of Nanostructured Materials for Sensor Applications. J. Nanomater. doi:10.1155/2020/8855321
Oh, Y., Armstrong, D. L., Finnerty, C., Zheng, S., Hu, M., Torrents, A., et al. (2017). Understanding the pH-Responsive Behavior of Graphene Oxide Membrane in Removing Ions and Organic Micropollulants. J. Membr. Sci. 541, 235–243. doi:10.1016/j.memsci.2017.07.005
Olkowska, E., Ruman, M., and Polkowska, Ż. (2014). Occurrence of Surface Active Agents in the Environment. J. Anal. Methods Chem. doi:10.1155/2014/769708
Pang, H., Wu, Y., Wang, X., Hu, B., and Wang, X. (2019). Recent Advances in Composites of Graphene and Layered Double Hydroxides for Water Remediation: a Review. Chem. Asian J. 14 (15), 2542–2552. doi:10.1002/asia.201900493
Pandey, R. P., Rasool, K., Madhavan, V. E., Aïssa, B., Gogotsi, Y., and Mahmoud, K. A. (2018). Ultrahigh-flux and Fouling-Resistant Membranes Based on Layered silver/MXene (Ti3C2Tx) Nanosheets. J. Mater. Chem. A. 6 (8), 3522–3533. doi:10.1039/c7ta10888e
Park, S.-H., Kwon, S. J., Shin, M. G., Park, M. S., Lee, J. S., Park, C. H., et al. (2018). Polyethylene-supported High Performance Reverse Osmosis Membranes with Enhanced Mechanical and Chemical Durability. Desalination 436, 28–38. doi:10.1016/j.desal.2018.02.007
Patel, M., Kumar, R., Kishor, K., Mlsna, T., Pittman, C. U., and Mohan, D. (2019). Pharmaceuticals of Emerging Concern in Aquatic Systems: Chemistry, Occurrence, Effects, and Removal Methods. Chem. Rev. 119 (6), 3510–3673. doi:10.1021/acs.chemrev.8b00299
Peng, Q., Guo, J., Zhang, Q., Xiang, J., Liu, B., Zhou, A., et al. (2014). Unique lead Adsorption Behavior of Activated Hydroxyl Group in Two-Dimensional Titanium Carbide. J. Am. Chem. Soc. 136 (11), 4113–4116. doi:10.1021/ja500506k
Peng, C., Yang, X., Li, Y., Yu, H., Wang, H., and Peng, F. (2016). Hybrids of Two-Dimensional Ti3C2 and TiO2 Exposing {001} Facets toward Enhanced Photocatalytic Activity. ACS Appl. Mater. Inter. 8 (9), 6051–6060. doi:10.1021/acsami.5b11973
Peng, C., Wang, H., Yu, H., and Peng, F. (2017). (111) TiO 2-x /Ti 3 C 2 : Synergy of Active Facets, Interfacial Charge Transfer and Ti3+ Doping for Enhance Photocatalytic Activity. Mater. Res. Bull. 89, 16–25. doi:10.1016/j.materresbull.2016.12.049
Peng, J., Liu, G., Yuan, D., Feng, S., and Zhou, T. (2017). A Flow-Batch Manipulated Ag NPs Based SPR Sensor for Colorimetric Detection of Copper Ions (Cu2+) in Water Samples. Talanta 167, 310–316. doi:10.1016/j.talanta.2017.02.015
Perreault, F., Fonseca de Faria, A., and Elimelech, M. (2015). Environmental Applications of Graphene-Based Nanomaterials. Chem. Soc. Rev. 44 (16), 5861–5896. doi:10.1039/c5cs00021a
Premarathna, K. S. D., Rajapaksha, A. U., Sarkar, B., Kwon, E. E., Bhatnagar, A., Ok, Y. S., et al. (2019). Biochar-based Engineered Composites for Sorptive Decontamination of Water: A Review. Chem. Eng. J. 372, 536–550. doi:10.1016/j.cej.2019.04.097
Presumido, P. H., Primo, A., Vilar, V. J. P., and Garcia, H. (2021). Large Area Continuous Multilayer Graphene Membrane for Water Desalination. Chem. Eng. J. 413, 127510. doi:10.1016/j.cej.2020.127510
Qian, Z. S., Shan, X. Y., Chai, L. J., Chen, J. R., and Feng, H. (2015). A Fluorescent Nanosensor Based on Graphene Quantum Dots-Aptamer Probe and Graphene Oxide Platform for Detection of lead (II) Ion. Biosens. Bioelectron. 68, 225–231. doi:10.1016/j.bios.2014.12.057
Rafiei-Sarmazdeh, Z., Zahedi-Dizaji, S. M., and Kang, A. K. (2019). “Two-Dimensional Nanomaterials,” in Nanostructures: IntechOpen.
Rahmanian, E., Mayorga-Martinez, C. C., Malekfar, R., Luxa, J., Sofer, Z., and Pumera, M. (2018). 1T-phase Tungsten Chalcogenides (WS2, WSe2, WTe2) Decorated with TiO2 Nanoplatelets with Enhanced Electron Transfer Activity for Biosensing Applications. ACS Appl. Nano Mater. 1 (12), 7006–7015. doi:10.1021/acsanm.8b01796
Ramalingam, G., Kathirgamanathan, P., Manivannan, N., and Kasinathan, K. (2020). “Quantum Confinement Effect of 2D Nanomaterials,” in Quantum Dots-Fundamental And Applications: IntechOpen. doi:10.5772/intechopen.90140
Råman Vinnå, L., Wüest, A., and Bouffard, D. (2017). Physical Effects of thermal Pollution in Lakes. Water Resour. Res. 53 (5), 3968–3987. doi:10.1002/2016WR019686
Ran, J., Zhu, B., and Qiao, S. Z. (2017). Phosphorene Co‐catalyst Advancing Highly Efficient Visible‐Light Photocatalytic Hydrogen Production. Angew. Chem. Int. Ed. 56 (35), 10373–10377. doi:10.1002/anie.201703827
Raptis, C. E., van Vliet, M. T. H., and Pfister, S. (2016). Global thermal Pollution of Rivers from Thermoelectric Power Plants. Environ. Res. Lett. 11 (10), 104011. doi:10.1088/1748-9326/11/10/104011
Rasool, K., Pandey, R. P., Rasheed, P. A., Buczek, S., Gogotsi, Y., and Mahmoud, K. A. (2019). Water Treatment and Environmental Remediation Applications of Two-Dimensional Metal Carbides (MXenes). Mater. Today 30, 80–102. doi:10.1016/j.mattod.2019.05.017
Rasool, K., Helal, M., Ali, A., Ren, C. E., Gogotsi, Y., and Mahmoud, K. A. (2016). Antibacterial Activity of Ti3C2Tx MXene. ACS nano 10 (3), 3674–3684. doi:10.1021/acsnano.6b00181
Rasool, K., Mahmoud, K. A., Johnson, D. J., Helal, M., Berdiyorov, G. R., and Gogotsi, Y. (2017). Efficient Antibacterial Membrane Based on Two-Dimensional Ti3C2Tx (MXene) Nanosheets. Sci. Rep. 7 (1), 1598–1611. doi:10.1038/s41598-017-01714-3
Rashed, M. N. (2013). Adsorption Technique for the Removal of Organic Pollutants from Water and Wastewater. Org. pollutants-monitoring, Risk Treat. 7, 167–194.
Rasheed, S., Campos, L. C., Kim, J. K., Zhou, Q., and Hashmi, I. (2016). Optimization of Total Trihalomethanes' (TTHMs) and Their Precursors' Removal by Granulated Activated Carbon (GAC) and Sand Dual media by Response Surface Methodology (RSM). Water Sci. Technol. Water Supply 16 (3), 783–793. doi:10.2166/ws.2015.175
Ren, C. E., Hatzell, K. B., Alhabeb, M., Ling, Z., Mahmoud, K. A., and Gogotsi, Y. (2015). Charge- and Size-Selective Ion Sieving through Ti3C2Tx MXene Membranes. J. Phys. Chem. Lett. 6 (20), 4026–4031. doi:10.1021/acs.jpclett.5b01895
Richardson, S. (2012). “Mass Spectrometry Identification and Quantification of Toxicologically Important Drinking Water Disinfection By-Products,” in Comprehensive Environmental Mass Spectrometry. Editor A. T. Lebedev (ILMPublications), 263–285.
Robertson, E. J., Battigelli, A., Proulx, C., Mannige, R. V., Haxton, T. K., Yun, L., et al. (2016). Design, Synthesis, Assembly, and Engineering of Peptoid Nanosheets. Acc. Chem. Res. 49 (3), 379–389. doi:10.1021/acs.accounts.5b00439
Rong, M., Lin, L., Song, X., Wang, Y., Zhong, Y., Yan, J., et al. (2015). Fluorescence Sensing of Chromium (VI) and Ascorbic Acid Using Graphitic Carbon Nitride Nanosheets as a Fluorescent “Switch”. Biosens. Bioelectron. 68, 210–217. doi:10.1016/j.bios.2014.12.024
Saleh, T. A., and Gupta, V. K. (2016). Nanomaterial and Polymer Membranes: Synthesis, Characterization, and Applications. Elsevier.
Sangwan, V. K., and Hersam, M. C. (2018). Electronic Transport in Two-Dimensional Materials. Annu. Rev. Phys. Chem. 69, 299–325. doi:10.1146/annurev-physchem-050317-021353
Sano, K., Kim, Y. S., Ishida, Y., Ebina, Y., Sasaki, T., Hikima, T., et al. (2016). Photonic Water Dynamically Responsive to External Stimuli. Nat. Commun. 7 (1), 1–9. doi:10.1038/ncomms12559
Schreiber, B., Brinkmann, T., Schmalz, V., and Worch, E. (2005). Adsorption of Dissolved Organic Matter onto Activated Carbon-The Influence of Temperature, Absorption Wavelength, and Molecular Size. Water Res. 39 (15), 3449–3456. doi:10.1016/j.watres.2005.05.050
Seenivasan, R., Chang, W.-J., and Gunasekaran, S. (2015). Highly Sensitive Detection and Removal of Lead Ions in Water Using Cysteine-Functionalized Graphene Oxide/Polypyrrole Nanocomposite Film Electrode. ACS Appl. Mater. Inter. 7 (29), 15935–15943. doi:10.1021/acsami.5b03904
Sen Gupta, S., Chakraborty, I., Maliyekkal, S. M., Adit Mark, T., Pandey, D. K., Das, S. K., et al. (2015). Simultaneous Dehalogenation and Removal of Persistent Halocarbon Pesticides from Water Using Graphene Nanocomposites: a Case Study of Lindane. ACS Sust. Chem. Eng. 3 (6), 1155–1163. doi:10.1021/acssuschemeng.5b00080
Shahabuddin, S., Khanam, R., Khalid, M., Sarih, N. M., Ching, J. J., Mohamad, S., et al. (2018). Synthesis of 2D boron Nitride Doped Polyaniline Hybrid Nanocomposites for Photocatalytic Degradation of Carcinogenic Dyes from Aqueous Solution. Arabian J. Chem. 11 (6), 1000–1016. doi:10.1016/j.arabjc.2018.05.004
Shahzad, A., Rasool, K., Miran, W., Nawaz, M., Jang, J., Mahmoud, K. A., et al. (2017). Two-Dimensional Ti3C2Tx MXene Nanosheets for Efficient Copper Removal from Water. ACS Sust. Chem. Eng. 5 (12), 11481–11488. doi:10.1021/acssuschemeng.7b02695
Shahzad, B., Tanveer, M., Rehman, A., Cheema, S. A., Fahad, S., Rehman, S., et al. (2018). Nickel; whether Toxic or Essential for Plants and Environment - A Review. Plant Physiol. Biochem. 132, 641–651. doi:10.1016/j.plaphy.2018.10.014
Sharp, E. L., Parsons, S. A., and Jefferson, B. (2006). Seasonal Variations in Natural Organic Matter and its Impact on Coagulation in Water Treatment. Sci. Total Environ. 363 (1), 183–194. doi:10.1016/j.scitotenv.2005.05.032
Sharma, A. (2019). Worldwide Pesticide Usage and its Impacts on Ecosystem. SN Appl. Sci. 1 (11), 1–16. doi:10.1007/s42452-019-1485-1
Sharma, G., and Naushad, M. (2020). Adsorptive Removal of Noxious Cadmium Ions from Aqueous Medium Using Activated Carbon/zirconium Oxide Composite: Isotherm and Kinetic Modelling. J. Mol. Liquids 310, 113025. doi:10.1016/j.molliq.2020.113025
Shehzad, K., Xu, Y., Gao, C., and Duan, X. (2016). Three-dimensional Macro-Structures of Two-Dimensional Nanomaterials. Chem. Soc. Rev. 45 (20), 5541–5588. doi:10.1039/C6CS00218H
Singh, H., Bamrah, A., Bhardwaj, S. K., Deep, A., Khatri, M., Kim, K.-H., et al. (2021). Nanomaterial-based Fluorescent Sensors for the Detection of lead Ions. J. Hazard. Mater. 407, 124379. doi:10.1016/j.jhazmat.2020.124379
Singh, P., Shandilya, P., Raizada, P., Sudhaik, A., Rahmani-Sani, A., and Hosseini-Bandegharaei, A. (2020). Review on Various Strategies for Enhancing Photocatalytic Activity of Graphene Based Nanocomposites for Water Purification. Arabian J. Chem. 13 (1), 3498–3520. doi:10.1016/j.arabjc.2018.12.001
Song, S., Yin, L., Wang, X., Liu, L., Huang, S., Zhang, R., et al. (2018). Interaction of U(VI) with Ternary Layered Double Hydroxides by Combined Batch Experiments and Spectroscopy Study. Chem. Eng. J. 338, 579–590. doi:10.1016/j.cej.2018.01.055
Srinivasan, S., Morgan, M. T., Liu, C., Matsen, F. A., Hoffman, N. G., Fiedler, T. L., et al. (2013). More Than Meets the Eye: Associations of Vaginal Bacteria with Gram Stain Morphotypes Using Molecular Phylogenetic Analysis. PloS one 8 (10), e78633. doi:10.1371/journal.pone.0078633
Stafford, J., Patapas, A., Uzo, N., Matar, O. K., and Petit, C. (2018). Towards Scale-Up of Graphene Production via Nonoxidizing Liquid Exfoliation Methods. Aiche J. 64 (9), 3246–3276. doi:10.1002/aic.16174
Stupp, H. D. (2007). “Remediation Technologies and Costs for Cleaning MTBE Contaminated Groundwater,” in Fuel Oxygenates (Springer), 249–273. doi:10.1007/978-3-540-72641-8_11
Surwade, S. P., Smirnov, S. N., Vlassiouk, I. V., Unocic, R. R., Veith, G. M., Dai, S., et al. (2015). Water Desalination Using Nanoporous Single-Layer Graphene. Nat. Nanotech 10 (5), 459–464. doi:10.1038/nnano.2015.37
Sweetman, M. J., May, S., Mebberson, N., Pendleton, P., Vasilev, K., Plush, S. E., et al. (2017). Activated Carbon, Carbon Nanotubes and Graphene: Materials and Composites for Advanced Water Purification. C 3 (2), 18. doi:10.3390/c3020018
Syafrudin, M., Kristanti, R. A., Yuniarto, A., Hadibarata, T., Rhee, J., Al-onazi, W. A., et al. (2021). Pesticides in Drinking Water-A Review. Int. J. Environ. Res. Public Health. 18 (2), 468. doi:10.3390/ijerph18020468
Tałałaj, I. A., Biedka, P., and Bartkowska, I. (2019). Treatment of Landfill Leachates with Biological Pretreatments and Reverse Osmosis. Environ. Chem. Lett. 17 (3), 1177–1193.
Tan, C., and Zhang, H. (2015). Two-dimensional Transition Metal Dichalcogenide Nanosheet-Based Composites. Chem. Soc. Rev. 44 (9), 2713–2731. doi:10.1039/C4CS00182F
Tan, H. T., Sun, W., Wang, L., and Yan, Q. (2016). 2D Transition Metal Oxides/Hydroxides for Energy-Storage Applications. ChemNanoMat 2 (7), 562–577. doi:10.1002/cnma.201500177
Tang, X., Han, J., Wang, Y., Ni, L., Bao, X., Wang, L., et al. (2017). A Multifunctional Schiff Base as a Fluorescence Sensor for Fe3+ and Zn2+ Ions, and a Colorimetric Sensor for Cu2+ and Applications. Spectrochimica Acta A: Mol. Biomol. Spectrosc. 173, 721–726. doi:10.1016/j.saa.2016.10.028
Tang, X., Han, J., Wang, Y., Ni, L., Bao, X., Wang, L., et al. (2017). A Multifunctional Schiff Base as a Fluorescence Sensor for Fe3+ and Zn2+ Ions, and a Colorimetric Sensor for Cu2+ and Applications. Spectrochimica Acta Part A: Molecular and Biomolecular Spectroscopy 173, 721–726.
Tang, Q., Zhou, Z., and Shen, P. (2012). Are MXenes Promising Anode Materials for Li Ion Batteries? Computational Studies on Electronic Properties and Li Storage Capability of Ti3C2 and Ti3C2X2 (X = F, OH) Monolayer. J. Am. Chem. Soc. 134 (40), 16909–16916. doi:10.1021/ja308463r
Tanveer, M., Hasanuzzaman, M., and Wang, L. (2019). Lithium in Environment and Potential Targets to Reduce Lithium Toxicity in Plants. J. Plant Growth Regul. 38 (4), 1574–1586. doi:10.1007/s00344-019-09957-2
T., A., P., S. K., and K., S. K. (2016). Synthesis of Nano-Sized Chitosan Blended Polyvinyl Alcohol for the Removal of Eosin Yellow Dye from Aqueous Solution. J. Water Process Eng. 13, 127–136. doi:10.1016/j.jwpe.2016.08.003
Tiwari, J. N., Vij, V., Kemp, K. C., and Kim, K. S. (2016). Engineered Carbon-Nanomaterial-Based Electrochemical Sensors for Biomolecules. ACS nano 10 (1), 46–80. doi:10.1021/acsnano.5b05690
Tran, V. S., Ngo, H. H., Guo, W., Zhang, J., Liang, S., Ton-That, C., et al. (2015). Typical Low Cost Biosorbents for Adsorptive Removal of Specific Organic Pollutants from Water. Bioresour. Tech. 182, 353–363. doi:10.1016/j.biortech.2015.02.003
Tu, J., Gan, Y., Liang, T., Hu, Q., Wang, Q., Ren, T., et al. (2018). Graphene FET Array Biosensor Based on ssDNA Aptamer for Ultrasensitive Hg2+ Detection in Environmental Pollutants. Front. Chem. 6 (333). doi:10.3389/fchem.2018.00333
UNEP (2001). Stockholm Convention on Persistent Organic Pollutants (POPs). United Nations Environment Programme. http://www.pops.int/.
UNEP (2007). Stockholm Convention on Persistent Organic Pollutants (POPs). United Nations Environment Programme. http://www.pops.int.
Upadhyay, R. K., Soin, N., and Roy, S. S. (2014). Role of Graphene/metal Oxide Composites as Photocatalysts, Adsorbents and Disinfectants in Water Treatment: a Review. RSC Adv. 4 (8), 3823–3851. doi:10.1039/c3ra45013a
Vallero, D. A. (2019). “Thermal Pollution,” in Waste (Academic Press), 381–404. doi:10.1016/b978-0-12-815060-3.00020-7
Van Engelenburg, J., Van Slobbe, E., and Hellegers, P. (2019). Towards Sustainable Drinking Water Abstraction: an Integrated Sustainability Assessment Framework to Support Local Adaptation Planning. J. Integr. Environ. Sci. 16 (1), 89–122. doi:10.1080/1943815X.2019.1636284
Varrla, E., Backes, C., Paton, K. R., Harvey, A., Gholamvand, Z., McCauley, J., et al. (2015). Large-Scale Production of Size-Controlled MoS2 Nanosheets by Shear Exfoliation. Chem. Mater. 27 (3), 1129–1139. doi:10.1021/cm5044864
Verma, R., Dwivedi, P. J. R. R. i. S., and Technology, . (2013). Heavy Metal Water Pollution-A Case Study. 5(5).
Wang, F., Pan, Y., Cai, P., Guo, T., and Xiao, H. (2017). Single and Binary Adsorption of Heavy Metal Ions from Aqueous Solutions Using Sugarcane Cellulose-Based Adsorbent. Bioresour. Technol. 241, 482–490. doi:10.1016/j.biortech.2017.05.162
Wang, E. N., and Karnik, R. (2012). Graphene Cleans up Water. Nat. Nanotech 7 (9), 552–554. doi:10.1038/nnano.2012.153
Wang, G., Li, J., Sun, W., and Xue, B. (2019). Non-point Source Pollution Risks in a Drinking Water protection Zone Based on Remote Sensing Data Embedded within a Nutrient Budget Model. Water Res. 157, 238–246. doi:10.1016/j.watres.2019.03.070
Wang, J., Li, G., and Li, L. (2016). “Characterization And Potential Applications,” in Synthesis Strategies about 2D Materials. Two-Dimensional Materials—Synthesis. Editor P. K. Nayak, 1–20.
Wang, J., Zhao, J., Lei, X., and Wang, H. (2018). New Approach for point Pollution Source Identification in Rivers Based on the Backward Probability Method. Environ. Pollut. 241, 759–774. doi:10.1016/j.envpol.2018.05.093
Wang, S., Niu, H., Cai, Y., and Cao, D. (2018). Multifunctional Au NPs-Polydopamine-Polyvinylidene Fluoride Membrane Chips as Probe for Enrichment and Rapid Detection of Organic Contaminants. Talanta 181, 340–345. doi:10.1016/j.talanta.2018.01.038
Wang, Y., Hu, J., Zhuang, Q., and Ni, Y. (2016). Label-Free Fluorescence Sensing of Lead(II) Ions and Sulfide Ions Based on Luminescent Molybdenum Disulfide Nanosheets. ACS Sust. Chem. Eng. 4 (5), 2535–2541. doi:10.1021/acssuschemeng.5b01639
Wang, Z., and Mi, B. (2017). Environmental Applications of 2D Molybdenum Disulfide (MoS2) Nanosheets. Environ. Sci. Technol. 51 (15), 8229–8244. doi:10.1021/acs.est.7b01466
Wang, H., Edwards, M. A., Falkinham, J. O., and Pruden, A. (2013). Probiotic Approach to Pathogen Control in Premise Plumbing Systems? A Review. Environ. Sci. Technol. 47 (18), 10117–10128. doi:10.1021/es402455r
Wei, M.-p., Chai, H., Cao, Y.-l., and Jia, D.-z. (2018). Sulfonated Graphene Oxide as an Adsorbent for Removal of Pb2+ and Methylene Blue. J. Colloid Interf. Sci. 524, 297–305. doi:10.1016/j.jcis.2018.03.094
Wei, G., Quan, X., Chen, S., and Yu, H. (2017). Superpermeable Atomic-Thin Graphene Membranes with High Selectivity. ACS nano 11 (2), 1920–1926. doi:10.1021/acsnano.6b08000
Wilkinson, J. L., Hooda, P. S., Barker, J., Barton, S., and Swinden, J. (2016). Ecotoxic Pharmaceuticals, Personal Care Products, and Other Emerging Contaminants: A Review of Environmental, Receptor-Mediated, Developmental, and Epigenetic Toxicity with Discussion of Proposed Toxicity to Humans. Crit. Rev. Environ. Sci. Tech. 46 (4), 336–381. doi:10.1080/10643389.2015.1096876
World Health Organization (2017). & United Nations Children's Fund.Progress on Drinking Water, Sanitation and hygiene: 2017 Update and SDG Baselines. Geneva: World Health Organization.
World Health Organization (WHO) (2011). Pharmaceuticals in Drinking Water: Public Health and Environment Water. Geneva: Sanitation, Hygiene and Health World Health Organization.
Wu, M.-h., Li, L., Liu, N., Wang, D.-j., Xue, Y.-c., and Tang, L. (2018). Molybdenum Disulfide (MoS2) as a Co-catalyst for Photocatalytic Degradation of Organic Contaminants: A Review. Process Saf. Environ. Prot. 118, 40–58. doi:10.1016/j.psep.2018.06.025
Wu, B., Wang, G., Wang, Z., Liu, C., and Ma, J. (2017). Integrated Hydrologic and Hydrodynamic Modeling to Assess Water Exchange in a Data-Scarce Reservoir. J. Hydrol. 555, 15–30. doi:10.1016/j.jhydrol.2017.09.057
Xiang, Q., Yu, J., and Jaroniec, M. (2012). Graphene-based Semiconductor Photocatalysts. Chem. Soc. Rev. 41 (2), 782–796. doi:10.1039/c1cs15172j
Xu, K., Feng, B., Zhou, C., and Huang, A. (2016). Synthesis of Highly Stable Graphene Oxide Membranes on Polydopamine Functionalized Supports for Seawater Desalination. Chem. Eng. Sci. 146, 159–165. doi:10.1016/j.ces.2016.03.003
Xu, L., Suo, H., Liu, R., Liu, H., and Qiu, H. (2019a). Design of GO-Ag-Functionalized Fe3O4@CS Composite for Magnetic Adsorption of Rhodamine B. RSC Adv. 9 (52), 30125–30133. doi:10.1039/C9RA04897A
Xu, L., Suo, H., Wang, J., Cheng, F., Liu, H., and Qiu, H. (2019b). Magnetic Graphene Oxide Decorated with Chitosan and Au Nanoparticles: Synthesis, Characterization and Application for Detection of Trace Rhodamine B. Anal. Methods 11 (30), 3837–3843. doi:10.1039/C9AY01287G
Xu, Q., and Zhang, W. J. A. i. C. N. (2016). Next-generation Graphene-Based Membranes for Gas Separation and Water Purifications, 39–61.
Yan, X., Li, H., and Su, X. (2018). Review of Optical Sensors for Pesticides. Trac Trends Anal. Chem. 103, 1–20. doi:10.1016/j.trac.2018.03.004
Yang, Y., Ren, L., Zhang, C., Huang, S., and Liu, T. (2011). Facile Fabrication of Functionalized Graphene Sheets (FGS)/ZnO Nanocomposites with Photocatalytic Property. ACS Appl. Mater. Inter. 3 (7), 2779–2785. doi:10.1021/am200561k
Yin, L., Wang, P., Wen, T., Yu, S., Wang, X., Hayat, T., et al. (2017). Synthesis of Layered Titanate Nanowires at Low Temperature and Their Application in Efficient Removal of U(VI). Environ. Pollut. 226, 125–134. doi:10.1016/j.envpol.2017.03.078
Ying, Y., Liu, Y., Wang, X., Mao, Y., Cao, W., Hu, P., et al. (2015). Two-Dimensional Titanium Carbide for Efficiently Reductive Removal of Highly Toxic Chromium(VI) from Water. ACS Appl. Mater. Inter. 7 (3), 1795–1803. doi:10.1021/am5074722
You, Y., Sahajwalla, V., Yoshimura, M., and Joshi, R. K. (2016). Graphene and Graphene Oxide for Desalination. Nanoscale 8 (1), 117–119. doi:10.1039/c5nr06154g
Yu, H.-D., Regulacio, M. D., Ye, E., and Han, M.-Y. (2013). Chemical Routes to Top-Down Nanofabrication. Chem. Soc. Rev. 42 (14), 6006–6018. doi:10.1039/c3cs60113g
Yu, L., Ruan, S., Xu, X., Zou, R., and Hu, J. (2017). One-dimensional Nanomaterial-Assembled Macroscopic Membranes for Water Treatment. Nano Today 17, 79–95. doi:10.1016/j.nantod.2017.10.012
Yu, S., Wang, X., Pang, H., Zhang, R., Song, W., Fu, D., et al. (2018). Boron Nitride-Based Materials for the Removal of Pollutants from Aqueous Solutions: a Review. Chem. Eng. J. 333, 343–360. doi:10.1016/j.cej.2017.09.163
Yu, X., Yun, S., Yeon, J. S., Bhattacharya, P., Wang, L., Lee, S. W., et al. (2018). Emergent Pseudocapacitance of 2D Nanomaterials. Adv. Energ. Mater. 8 (13), 1702930. doi:10.1002/aenm.201702930
Yuan, L., Yu, Q., Zhang, Y., and Xu, Y.-J. (2014). Graphene-TiO2 Nanocomposite Photocatalysts for Selective Organic Synthesis in Water under Simulated Solar Light Irradiation. RSC Adv. 4 (29), 15264–15270. doi:10.1039/c4ra01190b
Yuan, Y.-J., Shen, Z., Wu, S., Su, Y., Pei, L., Ji, Z., et al. (2019). Liquid Exfoliation of G-C3n4 Nanosheets to Construct 2D-2D MoS2/g-C3n4 Photocatalyst for Enhanced Photocatalytic H2 Production Activity. Appl. Catal. B: Environ. 246, 120–128. doi:10.1016/j.apcatb.2019.01.043
Zhang, J., Xing, C., and Shi, F. (2020). MoS2/Ti3C2 Heterostructure for Efficient Visible-Light Photocatalytic Hydrogen Generation. Int. J. Hydrogen Energ. 45 (11), 6291–6301. doi:10.1016/j.ijhydene.2019.12.109
Zhang, R., Liu, Y., He, M., Su, Y., Zhao, X., Elimelech, M., et al. (2016). Antifouling Membranes for Sustainable Water Purification: Strategies and Mechanisms. Chem. Soc. Rev. 45 (21), 5888–5924. doi:10.1039/c5cs00579e
Zeng, M., Chen, M., Huang, D., Lei, S., Zhang, X., Wang, L., et al. (2021). Engineered Two-Dimensional Nanomaterials: an Emerging Paradigm for Water Purification and Monitoring. Mater. Horiz. 8 (3), 758–802. doi:10.1039/D0MH01358G
Zeng, J., Zhang, Y., Zeng, T., Aleisa, R., Qiu, Z., Chen, Y., et al. (2020). Anisotropic Plasmonic Nanostructures for Colorimetric Sensing. Nano Today 32, 100855. doi:10.1016/j.nantod.2020.100855
Zeng, M., and Zhang, Y. (2019). Colloidal Nanoparticle Inks for Printing Functional Devices: Emerging Trends and Future Prospects. J. Mater. Chem. A. 7 (41), 23301–23336. doi:10.1039/C9TA07552F
Zhang, R., Liu, Y., He, M., Su, Y., Zhao, X., Elimelech, M., et al. (2016). Antifouling Membranes for Sustainable Water Purification: Strategies and Mechanisms. Chem. Soc. Rev. 45 (21), 5888–5924. doi:10.1039/C5CS00579E
Zhang, R., Wan, W., Qiu, L., Wang, Y., and Zhou, Y. (2017). Preparation of Hydrophobic Polyvinyl Alcohol Aerogel via the Surface Modification of boron Nitride for Environmental Remediation. Appl. Surf. Sci. 419, 342–347. doi:10.1016/j.apsusc.2017.05.044
Zhang, Y.-n., Niu, Q., Gu, X., Yang, N., and Zhao, G. (2019). Recent Progress on Carbon Nanomaterials for the Electrochemical Detection and Removal of Environmental Pollutants. Nanoscale 11 (25), 11992–12014. doi:10.1039/C9NR02935D
Zhao, J., Liu, J., Li, N., Wang, W., Nan, J., Zhao, Z., et al. (2016). Highly Efficient Removal of Bivalent Heavy Metals from Aqueous Systems by Magnetic Porous Fe3O4-MnO2: Adsorption Behavior and Process Study. Chem. Eng. J. 304, 737–746. doi:10.1016/j.cej.2016.07.003
Zhao, J., Niu, Y., Ren, B., Chen, H., Zhang, S., Jin, J., et al. (2018). Synthesis of Schiff Base Functionalized Superparamagnetic Fe3O4 Composites for Effective Removal of Pb(II) and Cd(II) from Aqueous Solution. Chem. Eng. J. 347, 574–584. doi:10.1016/j.cej.2018.04.151
Zhao, L., Deng, J., Sun, P., Liu, J., Ji, Y., Nakada, N., et al. (2018). Nanomaterials for Treating Emerging Contaminants in Water by Adsorption and Photocatalysis: Systematic Review and Bibliometric Analysis. Sci. Total Environ. 627, 1253–1263. doi:10.1016/j.scitotenv.2018.02.006
Zheng, X., and Zhang, X. (2019). “Excitons in Two-Dimensional Materials,” in Advances in Condensed-Matter and Materials Physics-Rudimentary Research to Topical Technology.
Zheng, Z., Grünker, R., and Feng, X. (2016a). Synthetic Two-Dimensional Materials: A New Paradigm of Membranes for Ultimate Separation. Adv. Mater. 28 (31), 6529–6545. doi:10.1002/adma.201506237
Zheng, Z., Grünker, R., and Feng, X. (2016b). Synthetic Two-Dimensional Materials: A New Paradigm of Membranes for Ultimate Separation. Adv. Mater. 28 (31), 6529–6545. doi:10.1002/adma.201506237
Zhong, Y. L., Tian, Z., Simon, G. P., and Li, D. (2015). Scalable Production of Graphene via Wet Chemistry: Progress and Challenges. Mater. Today 18 (2), 73–78. doi:10.1016/j.mattod.2014.08.019
Zhou, G., Chang, J., Pu, H., Shi, K., Mao, S., Sui, X., et al. (2016). Ultrasensitive Mercury Ion Detection Using DNA-Functionalized Molybdenum Disulfide Nanosheet/Gold Nanoparticle Hybrid Field-Effect Transistor Device. ACS Sens. 1 (3), 295–302. doi:10.1021/acssensors.5b00241
Zhu, J., Hou, J., Uliana, A., Zhang, Y., Tian, M., Bruggen, B. V., et al. (2018). The Rapid Emergence of Two-Dimensional Nanomaterials for High-Performance Separation Membranes. J. Mater. Chem. A. 6 (9), 3773–3792. doi:10.1039/c7ta10814a
Zhu, D., Liu, B., and Wei, G. (2021). Two-dimensional Material-Based Colorimetric Biosensors: a Review. Biosensors 11 (8), 259. doi:10.3390/bios11080259
Zhou, Q., and Fang, Z. (2015). Graphene-modified TiO2 Nanotube Arrays as an Adsorbent in Micro-solid Phase Extraction for Determination of Carbamate Pesticides in Water Samples. Analytica Chim. Acta 869, 43–49. doi:10.1016/j.aca.2015.02.019
Zou, G., Guo, J., Peng, Q., Zhou, A., Zhang, Q., and Liu, B. (2016). Synthesis of Urchin-like Rutile Titania Carbon Nanocomposites by Iron-Facilitated Phase Transformation of MXene for Environmental Remediation. J. Mater. Chem. A. 4 (2), 489–499. doi:10.1039/c5ta07343j
Zou, Y., Wang, X., Ai, Y., Liu, Y., Li, J., Ji, Y., et al. (2016). Coagulation Behavior of Graphene Oxide on Nanocrystallined Mg/Al Layered Double Hydroxides: Batch Experimental and Theoretical Calculation Study. Environ. Sci. Technol. 50 (7), 3658–3667. doi:10.1021/acs.est.6b00255
Zubair, M., Jarrah, N., Ihsanullah, A., Khalid, A., Manzar, M. S., Kazeem, T. S., et al. (2018). Starch-NiFe-layered Double Hydroxide Composites: Efficient Removal of Methyl orange from Aqueous Phase. J. Mol. Liquids 249, 254–264. doi:10.1016/j.molliq.2017.11.022
Zuo, J. Y., Gisolf, A., Wang, K., Dubost, F., Pfeiffer, T., Dumont, H., et al. (2016). Quantitative Mixing Rules for Downhole Oil-Based Mud Contamination Monitoring in Real Time Using Multiple Sensors. J. Pet. Sci. Eng. 137, 214–226. doi:10.1016/j.petrol.2015.11.025
Keywords: 2D nanomaterials, water quality monitoring sensors, water pollution, nanomaterials synthesis, water treatment
Citation: Fatima J, Shah AN, Tahir MB, Mehmood T, Shah AA, Tanveer M, Nazir R, Jan BL and Alansi S (2022) Tunable 2D Nanomaterials; Their Key Roles and Mechanisms in Water Purification and Monitoring. Front. Environ. Sci. 10:766743. doi: 10.3389/fenvs.2022.766743
Received: 30 August 2021; Accepted: 21 February 2022;
Published: 05 April 2022.
Edited by:
Alberto Tiraferri, Politecnico di Torino, ItalyReviewed by:
Mostafa Dadashi Firouzjaei, University of Alabama, United StatesCopyright © 2022 Fatima, Shah, Tahir, Mehmood, Shah, Tanveer, Nazir, Jan and Alansi. This is an open-access article distributed under the terms of the Creative Commons Attribution License (CC BY). The use, distribution or reproduction in other forums is permitted, provided the original author(s) and the copyright owner(s) are credited and that the original publication in this journal is cited, in accordance with accepted academic practice. No use, distribution or reproduction is permitted which does not comply with these terms.
*Correspondence: Mohsin Tanveer, bW9oc2luLnRhbnZlZXJAdXRhcy5lZHUuYXU=
Disclaimer: All claims expressed in this article are solely those of the authors and do not necessarily represent those of their affiliated organizations, or those of the publisher, the editors and the reviewers. Any product that may be evaluated in this article or claim that may be made by its manufacturer is not guaranteed or endorsed by the publisher.
Research integrity at Frontiers
Learn more about the work of our research integrity team to safeguard the quality of each article we publish.