- Department of Children’s Medical Center, Affiliated Hospital of Guangdong Medical University, Zhanjiang, China
Objective: This study aims to explore the toxic effect of PM2.5 on the hematopoietic microenvironment of the bone marrow, and investigate the effect of PM2.5 on oxidative stress injury, the secretion of inflammatory cytokines and the expression of NF-κB p65 and C x 43 in the bone marrow of mice.
Methods: A total of 615 mice were treated with normal saline, low dose (0.1 mg/ml) PM2.5 suspension, and high dose (0.2 mg/ml) PM2.5 suspension by intratracheal instillation, respectively. The ROS content, activity of total SOD (T-SOD), DNA injury, and the protein expression levels of IL-1 β, IL-6, TNF-α, NF-κB p65 and Cx43 in bone marrow cells (BMCs) of mice were detected in these three groups.
Results: Compared with the normal saline control group, the expression level of reactive oxygen species (ROS) significantly increased in BMCs, while the activity of SOD enzymes significantly decreased in PM2.5 exposed mice. Furthermore, DNA injury significantly increased in PM2.5 exposed mice, and the expression of IL-1 β, IL-6, TNF-α and NF-κB p65 significantly increased, while the protein expression of Cx43 significantly decreased in the PM2.5 exposed mice. The differences were statistically significant (p < 0.05). There were significant differences in ROS content, T-SOD activity, DNA injury (TL, TD and TM), protein expression of IL-1β, IL-6, TNF-α and Cx43 between the high-dose PM2.5 exposure group and low-dose PM2.5 exposure group (p < 0.05), but there was no significant difference in the expression of NF-κB p65 between the high-dose and low-dose PM2.5 exposure groups (p > 0.05).
Conclusion: PM2.5 has toxic effects on the bone marrow of mice. Oxidative stress injury, inflammatory reaction and abnormal intercellular communication may be the underlying mechanism.
1 Introduction
In recent years, air pollution has become more and more serious, and smog occurs in more and more places. At present, it has been considered that the particulate matter (PM) floating in the air is the main cause of smog. In particular, PM2.5, which has a particle size of <2.5 μm, cause the most serious injury to the human body. PM2.5 refers to particles with an aerodynamic diameter of ≤2.5 μm in size. The main components of PM2.5 include inorganic components (water-soluble components such as sulfate, nitrate, chloride, etc., water-insoluble components such as K, Fe, AI, etc.) and organic components (organic carbon, aliphatic hydrocarbons, aromatic hydrocarbons, etc.) Polycyclic aromatic hydrocarbons and alcohols, ketones, acids, fats, etc.). Most of it comes from man-made fossil fuel combustion source emissions (mainly coal combustion and motor vehicle exhaust). PM2.5 has a small particle size and large surface area, which is easy to accumulate toxic and harmful substances in the air, and it is easier to accumulate heavy metal elements and volatilize Sex organic compounds (VOC) and polycyclic aromatic hydrocarbons (PAH) can enter the trachea, bronchus, and even cross the blood barrier with human breathing, enter the blood circulation, and are widely distributed in organs and tissues in the body, aggravating or causing various diseases, The components contained in PM2.5 and the proportions of specific components are affected by various factors such as meteorological conditions, specific sources, particle characteristics, and so on. Therefore, the composition of components in different regions is very different. At present, most cities across the country have begun to adopt the gravimetric method and the micro-oscillation balance method to implement PM2.5 detection and use it as an important detection indicator of the local air pollution index. Due to its small particle size, large area and strong activity, it can easily attach to toxic and harmful substances (such as heavy metals, microorganisms, etc.), and has a long stay time and long transportation distance in the atmosphere, making it belong to the category with the greatest harm to the environment and human health.
In 2013, the World Health Organization defined PM2.5 as a new complex form of air carcinogen. It was suggested that PM2.5 can enter the blood system, leading to respiratory, cardiovascular, immune and endocrine diseases (Pfeffer and Mudway, 2018). A large number of epidemiological studies have shown that there is a great relationship between PM2.5 and the occurrence of leukemia (Tamayo- Uria et al., 2018; Puett et al., 2020; Ribeiro et al., 2021). Recent toxicological studies have shown that PM2.5 and its main components, such as polycyclic aromatic hydrocarbons, benzene and leukemia There is a close relationship with the increasing of PM2.5, suggesting that PM2.5 is one of the causes of leukemia. A recent cell experimental study showed that PM2.5 may promote the occurrence and progression of acute myeloid leukemia by affecting the expression of cytokines (Chen et al., 2018). However, the impact of PM2.5 on the hematopoietic system mainly comes from epidemiological investigations of large sample data in different countries and regions. Whether exposure to PM2.5 can affect other hematopoietic tissues and organs such as bone marrow and their physiological There is a lack of sufficient and direct experimental evidence for the negative impact on function and the specific impact pathway.
At present, related research on bone marrow injury caused by direct exposure to PM2.5 remains limited and uncomprehensive, and the specific mechanism and method of causing injury and toxicity remains unclear at present. In the present study, 615 mice were used as animal models to observe the effects of long-term exposure to different doses of PM2.5 on the bone marrow of mice. The characteristic indexes were selected and detected from the aspects of oxidative stress, the level of inflammatory factors, and the intercellular communication between gap junctions (Bekki et al., 2016; Grevendonk et al., 2016). The present study aimed at explore the injury caused by PM2.5 exposure on the bone marrow and the potential mechanism of injury, as well as the experimental basis and biological evidence for the occurrence and development of PM2.5 hematopoiesis toxicity and hematopoiesis-related diseases (Raaschou -Nielsen et al., 2016).
2 Materials and Methods
2.1 Materials
2.1.1 Experimental Animals and Main Materials
A total of 36 female, 8-week-old 615 mice of specific pathogen-free (SPF) grade, with a body weight of 20 ± 2 g, were purchased from the Experimental Animal Center of the Institute of Hematology, Chinese Academy of Medical Sciences [Certification number: SCXK (Jin)2017.0001]. In addition, the following were also purchased: RBC lysate (Beijing Solarbio Biotechnology Co., Ltd.), total SOD (T-SOD) activity assay kit (WST-8 method; Biyuntian Biotechnology Co., Ltd.), Comet assay kit (Cell Biolabs), ROS fluorescence Probe-DHE (Beijing Vigras Biotechnology Co., Ltd.), BCA protein assay kit (Solarbio Biotechnology, Beijing, China), nuclear factor kappa B (NF-κb) p65 rabbit mAb (Sigma), ELISA Kit (China Yanhui Biology), and C x 43 rabbit mAb (Abcam, United States).
2.1.2 Preparation of Animal Models
2.1.2.1 Animal Grouping
A total of 36 female, 8-week-old 615 mice of SPF grade were randomly divided into three groups, with 12 mice in each group: normal saline control group, low-dose PM2.5 exposure group (0.1 mg/ml), and high-dose PM2.5exposure group (0.2 mg/ml).
2.1.2.2 Preparation of the Models
The section of East Wenming Road (urban center) in Xiashan District, Zhanjiang City, Guangdong Province was selected as the PM2.5 collection site and choose to collect it in winter. PM2.5 was collected using a QJS-100D multi-stage particulate collector for 3 months continuously. The PM2.5 dry powder was prepared by collecting fine particles, soaking, ultrasonic vibration, elution, filtration, and freeze-drying. Then, the PM2.5 suspension was prepared with normal saline at doses of 0.1 mg/ml and 0.2 mg/ml, respectively.
According to the grouping, 36 mice were treated with 50 ul of normal saline, 0.1 mg/ml of PM2.5 suspension, and 0.2 mg/ml of PM2.5 suspension by intratracheal instillation toxication, respectively. These mice were exposed to PM2.5 once a week for 1 month. Detailed steps of animal handling: Tracheal drip method of poisoning: ① Anesthesia: 1% pentobarbital, 10 ml/kg, was injected intraperitoneally to anesthetize the mice. ② Exposure of trachea: After the mice were fully anesthetized, that is, after the mice did not struggle, scratching and other performance, the mice were placed in a supine position, with a thin rope over the upper teeth to fix the head, torso and limbs fixed with tape, fully exposed the neck, so that the head, neck and torso into a straight line. The mouse was disinfected with alcohol, and a 0.5-cm-long incision was made at the cricoid cartilage in the midline to expose the trachea by blunt separation. ③ Tracheal drip dyeing: Use a 4.5-gauge scalp needle to perform subcricoid cartilage tracheal puncture, about 3 mm into the needle, and take 50 ul each of saline, 0.1 mg/ml PM2.5, and 0.2 mg/ml PM2.5 solution according to the experimental group, and inject 0.1 ml of gas from the scalp needle into the trachea, and then continue to inject 0.1 ml of gas to ensure that the liquid fully enters the trachea. ④ Suture: Immediately after gas injection, the scalp needle was withdrawn and the skin was sterilized and sutured.
2.1.2.3 Materials Drawing and Storage
At 1 month after toxication, mice were killed by neck execution, and the marrow cell suspension was collected and stored in the refrigerator at -80°C for future use. Detailed steps of bone marrow cell collection: After the end of staining, the mice were decollated and executed. The mouse was placed in 75% ethanol for 3 min ophthalmic forceps were used to lift the mouse skin along the groin, and ophthalmic scissors were used to cut the groin skin to expose the leg muscles and cut the mouse thigh from the joint. The muscles and soft tissues attached to the bone surface were carefully removed, and the left femur of the mouse was taken and fixed in 10% formalin for pathological examination. The right femur was placed in a sterile Petri dish filled with PBS buffer, and the bone was flushed out of the bone marrow cavity by using forceps to clamp up one end of the bone and aspirating PBS buffer with a syringe, and the bone marrow tissue was flushed out from one end of the bone marrow cavity, repeated 3 times, and then flushed out of the bone marrow in the opposite direction, and repeated 3 times until the femur was clear and the bone marrow cavity flushing solution became clear and bright, and then stopped. Blow the cell suspension in order to disperse the cells, collect the bone marrow cell suspension in a 1.5 ml centrifuge tube and store in a -80°C refrigerator.
3 Methods
3.1 Detection of the Content of Active Oxygen and SOD Enzyme Activity
The bone marrow cell suspensions collected from the three groups were diluted to approximately 1 × 105/ ml, added with red cell lysate at a ratio of 1:3, and centrifuged at 4°C for 10 min at 450 rpm, and the sediment was taken for re-suspension. Next, 1 ml of the re-suspension liquid taken, 5 μl of ROS fluorescence probe-DHE was added, incubation was performed at 37°C in the dark for 30 min, and the average fluorescence intensity was detected by flow cytometry. Then, 1 ml of the re-suspension was taken, and an ultrasonic cell lyser was used to lyse cells to obtain the cell homogenate. Afterwards, centrifugation at 4°C was performed at 1,000 rpm. The supernatant was taken as a sample to detect the SOD activity of bone marrow cells, according to the procedures of the T-SOD activity assay kit. The SOD activity was calculated according to the following functions: 1) inhibiting percentage = (OD of blank control hole 1—OD of sample)/(OD of blank control hole 1—OD of blank control hole 2) × 100%; 2) SOD enzyme activity unit in the sample = SOD enzyme activity unit in the detecting system = [inhibiting percentage/(1—inhibiting percentage)] units/protein dosage (mg/ml).
3.2 Bone Marrow Detection by Comet Test
After combining with the DNA staining agent, green fluorescence from the DNA in cells was produced under blue excitation (460–485 nm). The DNA of normal cells was not injured, and after cracking the nuclear membrane, the DNA mainly existed in the form of a super helix. Under the action of a current, the large DNA molecules moved for a short distance, and the fluorescence was concentrated in the nucleus (including the large molecular weight DNA). With the aggravation of DNA injury in cells, the DNA broke. Under the action of electric current, fragments (forming the tail of the comet) migrated out of the nucleus (forming the head of the comet). The degree of DNA injury can be indicated by the migration distance and DNA content in the tail of the comet. Procedures of DNA injury detection: 1) slice preparation, 2) glue spreading, 3) lysis, 4) helix unwinding, 5) electrophoresis, 6) washing and staining, and 7) shooting and image capturing.
3.3 Pathological Detection of the Bone Marrow of Mice
The unilateral femurs were randomly selected from two mice in each group. Then, the pathological sections were prepared and stained with hematoxylin and eosin (H&E). Afterwards, the histopathological changes were observed using an optical microscope.
3.4 Assay for IL-1β, IL-6 and TNF-α Secretion
Enzyme-linked immunosorbent assay (ELISA) and the double antibody one step sandwich method (the reagent was provided by China Yanhui biological Co., Ltd.) were used to detect the secretion level of IL-1β, IL-6 and TNF-α.
3.5 Protein Expression of NF-κB p65 and C x 43 in Bone Marrow of Mice by Western Blotting Analysis
Bone marrow cells were collected, and total protein was extracted for protein content determination. For each group, 60 ug of protein samples were taken and ddH2O was added to bring the volume to 8 ul. Then, 2 ul of 5 × SDS sample buffer was added and mixed uniformly. Denatured samples were subjected to electrophoresis (SDS-PAGE) on a 10% sodium dodecyl sulfate (SDS) polyacrylamide gel at a constant current of 250 mA across the membrane, and the corresponding bands of the target and internal reference genes were cut according to the position of the markers. These bands were blocked for 1.5 h at room temperature with a blocking solution prepared from 5% skim milk. Primary antibodies at a 1:1,000 dilution of blocking solution are added to the PVDF membrane and incubated overnight in the refrigerator. The next day the primary antibody is discarded and the corresponding 1:1,000 dilution of secondary antibody is added and incubated for 1.5 h. Then, wash three times with TBS-T. After draining the PVDF membrane, chemiluminescent detection reagents are added and exposed in a Bio-Rad versadoctm 50,000mp system. After exposure, the grayscale values were analyzed using the Gel Pro Analyzer image analysis software and recorded for statistical analysis.
4 Statistical Analysis
SPSS 23.0 software was used for the statistical analysis of data. Measurement data were expressed as mean ± standard deviation (x ± S), and one-way ANOVA was used for comparisons between groups. The LSD-t test was used to compare the mean multiple comparison for homogeneity of variance. Otherwise, Dunnett’s T3 test was used to determine whether the difference was statistically significant, and the test level was α = 0.05.
5 Results
5.1 Effects of PM2.5 on the Content of Bone Marrow Cells Reactive Oxygen Species and Activity of Total SOD Enzyme Activity
After infecting mice with different concentrations of PM2.5, the content of reactive oxygen species in BMCs of mice increased, which was different from that in the control group. The ROS content in the high-concentration group was higher than that in the low-concentration exposure group, and the difference was statistically significant (p < 0.05).
After infecting mice with different concentrations of PM2.5, the SOD enzyme activity of BMCs in mice decreased, and the difference was statistically significant (p < 0.05). The SOD enzyme activity in the high-concentration group was lower than that in the low-concentration exposure group, but the difference was not statistically significant (p > 0.05, Table 1).
5.2 Effects of PM2.5 on Bone Marrow Cells DNA Injury
As shown in Figure 1, Figure 1A demonstrates that the whole morphology of BMCs in mice in the normal saline control group was basically round, and only a small amount of tails could be seen after analysis with the CASP software. Figure 1B shows that in mice in the low-dose PM2.5 exposure group, some of the BMCs were not round, and the tail was visible by the naked eye. Figure 1C illustrates the morphology of BMCs in the high-dose PM2.5 exposure group. It could be observed that most BMCs had long tails, indicating that many DNA fragments migrated to the extracellular under a current. As shown in Figure 1D, the tail length (TL), tail DNA content (TD) and olive tail distance (TM) of cells in each group were analyzed using the CASP software. Through statistical analysis of variance, the difference between the experimental groups and normal saline control group was statistically significant (p < 0.05), and the difference between the high-dose PM2.5 exposure group and low-dose PM2.5 exposure group also had statistical significance (p < 0.05).
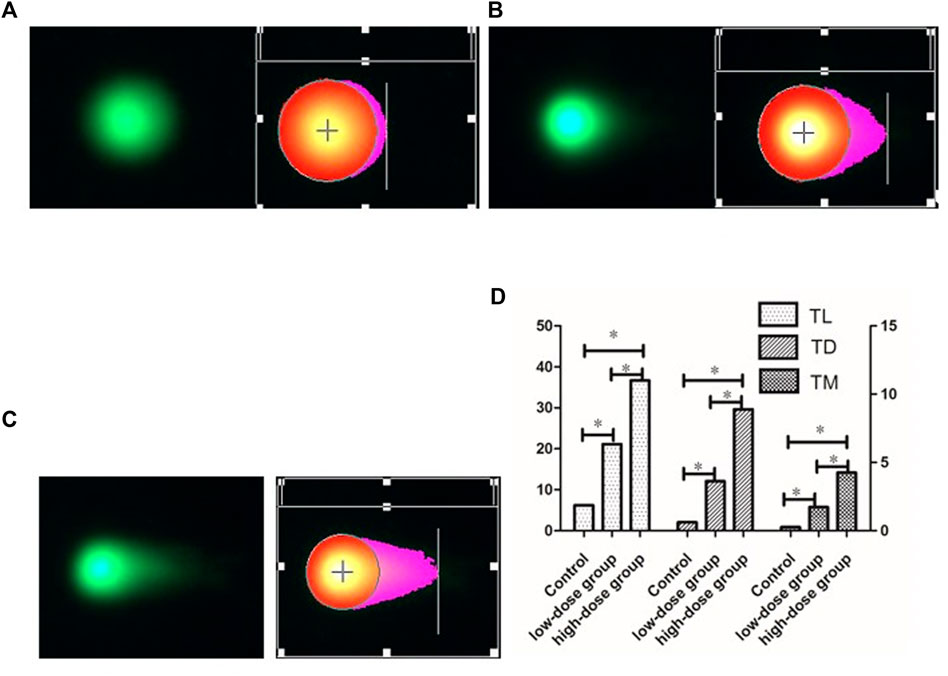
FIGURE 1. comet images of BMCs DNA damage at different dose of PM2.5. (A):Control (0 mg/ml) (20 × ). (B):low-dose group (0.1 mg/ml) (20 × ). (C):high-dose group (0.2 mg/ml) (20 × ). (D): Comet assessment of BMCs DNA damage by PM2.5, including TL, TD, TM. ∗p < 0.05.
5.3 Pathological Changes of Bone Marrow Tissues After H&E Staining in Each Group
As shown in Figure 2, the bone marrow sections in the normal saline control group revealed that the cells were in normal shape, with a small number of scattered megakaryocytes. Furthermore, the number of megakaryocytes in the bone marrow section of mice exposed to low-dose PM2.5 and high-dose PM2.5 significantly changed.
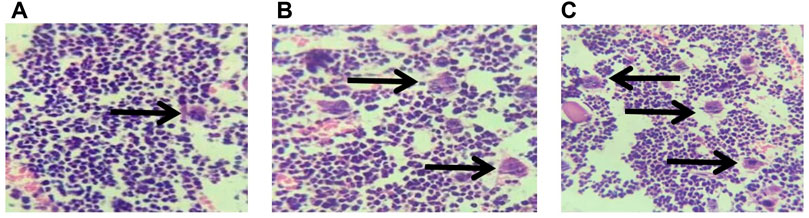
FIGURE 2. pathological sections of mouse bone marrow tissues (HE staining, 40 x ). (A):Control (0 mg/ml) (40 × ). (B):Low-dose group (0.1 mg/ml) (40 × ). (C):High-dose group (0.2 mg/ml) (40 × ).
5.4 Protein Expression Levels of IL-1β, IL-6, TNF-α, NF-κB p65, and C x 43 in Mice Bone Marrow
Compared with those in the normal saline control group, the expression of IL-1 β, IL-6, TNF-α and NF-κB p65 in the low-dose and high-dose PM2.5 exposure groups significantly increased, while the relative expression of C x 43 significantly decreased, and the difference was statistically significant (p < 0.05). The difference in the protein expression of IL-1β, IL-6, TNF-α, and C x 43 between the high-dose PM2.5 exposure group and low-dose PM2.5 group was statistically significant (p < 0.05), while the difference in the expression of NF-κB p65 between the high-dose and low-dose exposure group was not statistically significant (p > 0.05, Table 2).
5.5 The Protein Expression Level of NF-κB p65 in the Bone Marrow of Mice
Western blotting was performed to analyze the protein expression of NF-κB p65 in mice bone marrow. After the gray analysis and internal reference correction, the relative protein expression of NF-κB p65 was obtained. The results are presented in Figure 3 and Table 2. Compared with the normal saline control group, the protein expression of NF-κB p65 in these two PM2.5 exposure groups was significantly upregulated, and the difference was statistically significant (p < 0.01). However there was no significant difference in protein expression between the high-dose PM2.5 exposure group and low-dose PM2.5 exposure group (p > 0.05).
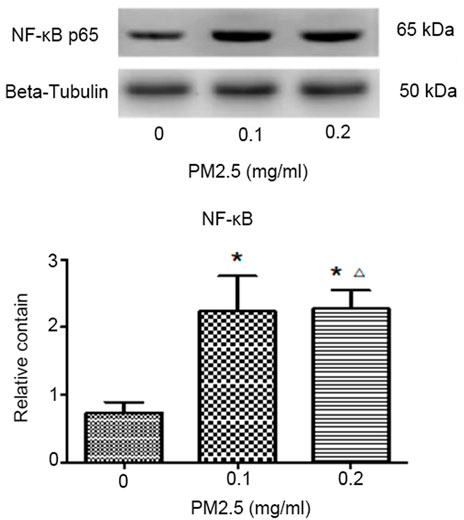
FIGURE 3. Western blotting to analyze the protein expression of NF-κB p65 and the relative protein expression of NF-κB p65. Note: *p < 0.01, vs. control; △p > 0.05, vs. low-dose group.
5.6 C x 43 Protein Expression in the Bone Marrow of Mice by Western Blotting Analysis
Western blotting was adopted to analyze the protein expression of C x 43 in mice bone marrow. After the gray analysis and internal reference correction, the relative protein expression of C x 43 was obtained. The results are presented in Figure 4 and Table 2. Compared with the normal saline control group, the protein expression of C x 43 in these two PM2.5 exposure groups was significantly upregulated, and the difference was statistically significant (p < 0.01). There was no significant difference in protein expression between the high-dose PM2.5 exposure group and low-dose PM2.5 exposure group (p > 0.05; Table 2).
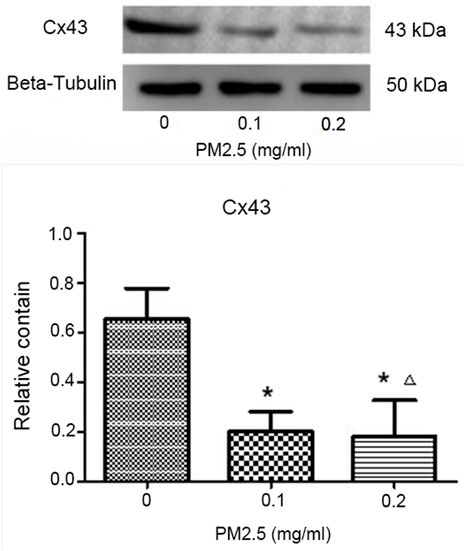
FIGURE 4. Western blotting to analyze the protein expression of C x 43 and the relative protein expression of C x 43.
6 Discussion
Under normal circumstances, the bone marrow has a hypoxic microenvironment (Snyder and Chandel, 2009), and plays an important role in protecting the normal hematopoietic function of hematopoietic stem cells in the bone marrow (Simon and Keith, 2008). However, the increased production of ROS may cause the abnormal proliferation and differentiation of hematopoietic stem cells, which may lead to the occurrence and progression of malignant tumors (Snyder and Chandel, 2009). In the present study, PM2.5 was infused into mice by tracheal instillation. After a chronic process of exposure, the ROS of bone marrow cells significantly increased, suggesting that PM2.5 could directly or indirectly produce long-distance toxic effects and change the oxidative level of the microenvironment in the bone marrow. The difference between the high-dose PM2.5 exposure group and low-dose PM2.5 exposure group was statistically significant, which suggests that PM2.5 toxicity is dose-dependent. Yuqi Cui et al. (Cui et al., 2015) has proven that fine PM inhibited the proliferation of bone marrow mesenchymal stem cells (BMSCs) in vivo by tracheal toxicity in mice, and detected a significant increase in ROS level in BMSCs, demonstrating that PM inhibited the proliferation of BMSCs by activating the Akt signaling pathway through the ROS regulation mechanism. In addition, it was suggested that the PM2.5-induced autophagy of pulmonary macrophages might be mediated through the P13K/ATK/mTOR pathway caused by oxidative stress (Su et al., 2017). Therefore, it was speculated that the long-term exposure of PM2.5 increased the oxidation level of BMCs and changed the hypoxic microenvironment in the bone marrow, which may be a promoting factor for some hematological diseases.
After 1 month of exposure to PM2.5, the activity of T-SOD in the BMCs of mice was significantly lower than that in the normal saline control group, while the ROS level significantly increased, indicating that PM2.5 inhalation might cause oxidative stress in the bone marrow. There was no difference in T-SOD activity between the low-dose PM2.5 exposure group and high-dose PM2.5 exposure group. This may be attributable to the fact that the high dose of PM2.5 stimulated the whole activation of the anti-oxygen free radical injury system of BMCs. Under the mechanism of free radical induction and body compensation, the antioxidant capacity of BMCs was enhanced. SOD can specifically scavenge O− to obtain the catalytic product H2O2, while the clearance of H2O2 must depend on other enzymes. The inconsistency between the increase in ROS and the decrease in T-SOD enzyme activity suggests that other antioxidants might participate in this process. However, eventually, the balance of oxidative stress might be destroyed, placing BMCs in a state of high oxidation and low anti-oxidation level.
It was suggested by the present study that PM2.5 might arouse DNA injury in BMCs. There were also a small number of comet cells in the normal saline control group, which may have been caused by the injury of cells in the process of cell extraction. The TL, TD and TM of comet cells in the high-dose PM2.5 exposure group were significantly higher than those in the low-dose PM2.5 exposure group, indicating that a high dose of PM2.5 is more toxic, and that DNA injury caused by PM might be dose-dependent. In some studies, it was reported that the tail length of comet cells might not be directly proportional to the dose of toxic substances after exposure. The toxic injury may lead to DNA protein cross-linking, there increasing the quality of broken DNA and shortening the migration distance under the current (Ventura et al., 2013). In the present study, the tail shortening of comet cells was not observed in the high-dose PM2.5 exposure group. It might be inferred that PM2.5 could cause DNA single or double strand breakage, but this is not enough to cause DNA protein crosslinking injury. Thus, it might be speculated that after long-term exposure to PM2.5, the level of ROS in mice BMCs significantly increased, and that the BMC DNA injury was aggravated. PM2.5 may cause DNA injury by inducing oxidative stress injury in BMCs in mice (Vattanasit et al., 2014; Grevendonk et al., 2016).
The occurrence of hematopoietic system diseases usually accompanies the histological changes of the bone marrow. Some abnormal changes in the bone marrow can be used to indicate some diseases. The histopathological assay of the bone marrow can provide direct evidence for the toxic effect of PM2.5 exposure on the bone marrow of mice. It was found that the number of megakaryocytes in the exposed group significantly changed in the present study. However, the abnormal changed in megakaryocytes might lead to the onset of diseases, such as megakaryocytic leukemia, and the main pathological mechanism of which is the abnormal proliferation of megakaryocytes and the fibrosis of the bone marrow (Meira et al., 2008), together with immune thrombocytopenic purpura and other blood system diseases. PM2.5 exposure may increase the incidence of these above diseases. Megakaryocytes are the precursor cells of platelets, and their main function is to produce platelets. Recent studies have shown that they, as important regulatory cells of the hematopoietic microenvironment, can interact with hematopoietic stem cells and leukemia stem cells directly or indirectly, and can change the hematopoietic microenvironment It plays an important role in regulating normal and abnormal hematopoiesis in the body. The imbalance of the hematopoietic microenvironment is closely related to the occurrence of various malignant hematological diseases. After exposure to PM2.5, the levels of TNF-α, IL-6 and IL-1β in mice in the low-dose PM2.5 exposure group and high-dose PM2.5 exposure group significantly increased, which indicates that PM2.5 could cause inflammatory reaction in bone marrow cells, and the difference in the above three levels between the high-dose and low-dose PM2.5 exposure groups was also statistically significant, indicating that the production of inflammatory factors was PM2.5 dose-dependent.
NF-κb is a protein with multidirectional nuclear transcriptional regulation function. Its main structure includes five subunits. The main structure that exerts physiological function in vivo is p50-p65, which plays an important regulatory role in the process of inflammation and oxidative stress. It has been suggested that PM2.5 could increase the level of oxidative stress in cells, activate NF-κB, and further promote the secretion of TNF-α, IL-1β, IL-6 and other inflammatory cytokines (Li et al., 2015; Bekki et al., 2016), thereby eventually leading to the occurrence of inflammatory injury. In the present study, the expression of NF-κB significantly increased after PM2.5 exposure, and the levels of inflammatory cytokines TNF-α, IL-6, and IL-1β also significantly increased, which was consistent with the above results. NF-κB activation is also common in cancer, which is mainly driven by inflammatory cytokines in the tumor microenvironment. In malignant tumors, NF-κB acts on cells in a specific manner, that is, activating the survival genes in cancer cells and promoting inflammation genes in the tumor microenvironment (Didonato et al., 2012). A large number of studies have confirmed that PM2.5 can significantly increase the secretion of IL-1β, IL-2, IL-6, IL-10, IL-17A, TNF-α, and NF-κB by inducing the proliferation of leukemic cells, thereby increasing the tumor volume of human myeloid leukemia in a time- and dose-dependent manner (Jin et al., 2016; Chen et al., 2018). Combined with the results of the present study, it could be speculated that long-term exposure of PM2.5 could lead to inflammation of the bone marrow microenvironment which indirectly leads to various blood system diseases such as anemia, thrombocytopenia, abnormal increase in white blood cells or changes in platelet or white blood cell function, etc.
C x 43 is an important cellular gap junction protein, which is expressed in a variety of normal tissue cells. The C x 43 mediated gap junctional inter-communication (GJIC) is an essential condition for the bone marrow microenvironment to regulate hematopoiesis (Morrison and Scadden, 2014). It has been confirmed by a large number of studies that GJIC mediated by C x 43 plays an important role in the pathogenesis and progression of leukemia. The level of GJIC expression is negatively correlated to the malignancy of the tumor (Liu et al., 2015; Yang et al., 2015). The results reported by Solan et al. (Solan and Lampe, 2009) also indicated that there was a general downregulation or defect of C x expression after the malignant transformation of cells. In the present study, the expression of C x 43 in the bone marrow in the PM2.5 exposure groups was significantly downregulated, when compared to those in the normal saline control group. Hence, the long-term exposure to PM2.5 may lead to the occurrence of blood system diseases.
7 Conclusion
The results of this study demonstrate that long-term exposure to PM2.5 produced toxic effects on bone marrow tissues and cells in mice and affected the stability of the hematopoietic microenvironment. The inflammatory response, oxidative stress, and abnormalities in intercellular junctional communication may be the underlying mechanisms. Although the reports related to the toxic effects of PM2.5 exposure on bone marrow tissues and the induction of hematologic disorders are not comprehensive, inflammatory factors in bone marrow tissues and abnormalities in intercellular junctional communication function have been considered as important features in the development and progression of hematologic disorders.
Data Availability Statement
The raw data supporting the conclusions of this article will be made available by the authors, without undue reservation.
Ethics Statement
The animal study was reviewed and approved by This study was conducted with approval from the Ethics Committee of Affiliated Hospital of Guangdong Medical University.
Author Contributions
L-LL, JY, Z-LY, and X-HM conceived the idea and conceptualised the study. CT, X-LH, and H-QZ collected the data. Z-LY and H-QZ analysed the data. Z-LY and L-LL drafted the manuscript, then L-LL and JY reviewed the manuscript. All authors read and approved the final draft.
Funding
This study was funded by the Doctoral Research Project of The Affiliated Hospital of Guangdong Medical University (No.40102B20170007), the Special competitive distribution project of Zhanjiang financial funds for science and technology (No. 20130A304) and Guangdong science and technology project (No. 2010B031500033). The funding body had no role in the design of the study and collection, analysis, and interpretation of data and in writing the manuscript.
Conflict of Interest
The authors declare that the research was conducted in the absence of any commercial or financial relationships that could be construed as a potential conflict of interest.
Publisher’s Note
All claims expressed in this article are solely those of the authors and do not necessarily represent those of their affiliated organizations, or those of the publisher, the editors and the reviewers. Any product that may be evaluated in this article, or claim that may be made by its manufacturer, is not guaranteed or endorsed by the publisher.
Acknowledgments
We would like to acknowledge the hard and dedicated work of all the staff that implemented the intervention and evaluation components of the study.
References
Bekki, K., Ito, T., Yoshida, Y., He, C., Arashidani, K., He, M., et al. (2016). PM 2.5 Collected in China Causes Inflammatory and Oxidative Stress Responses in Macrophages through the Multiple Pathways. Environ. Toxicol. Pharmacol. 45, 362–369. doi:10.1016/j.etap.2016.06.022
Chen, T., Zhang, J., Zeng, H., Zhang, Y., Zhang, Y., Zhou, X., et al. (2018). The Impact of Inflammation and Cytokine Expression of PM2.5 in AML. Oncol. Lett. 16, 2732–2740. doi:10.3892/ol.2018.8965
Cui, Y., Jia, F., He, J., Xie, X., Li, Z., Fu, M., et al. (2015). Ambient Fine Particulate Matter Suppresses In Vivo Proliferation of Bone Marrow Stem Cells through Reactive Oxygen Species Formation. PLOS ONE 10, e0127309. doi:10.1371/journal.pone.0127309
Didonato, J. A., Mercurio, F., and Karin, M. (2012). NF-κB and the Link between Inflammation and Cancer. Immunol. Rev. 246, 379–400. doi:10.1111/j.1600-065x.2012.01099.x
Grevendonk, L., Janssen, B. G., Vanpoucke, C., Lefebvre, W., Hoxha, M., Bollati, V., et al. (2016). Mitochondrial Oxidative DNA Damage and Exposure to Particulate Air Pollution in Mother-Newborn Pairs. Environ. Health 15, 10. doi:10.1186/s12940-016-0095-2
Jin, X.-T., Chen, M.-L., Li, R.-J., An, Q., Song, L., Zhao, Y., et al. (2016). Progression and Inflammation of Human Myeloid Leukemia Induced by Ambient PM2.5 Exposure. Arch. Toxicol. 90, 1929–1938. doi:10.1007/s00204-015-1610-x
Li, R., Kou, X., Xie, L., Cheng, F., and Geng, H. (2015). Effects of Ambient PM2.5 on Pathological Injury, Inflammation, Oxidative Stress, Metabolic Enzyme Activity, and Expression of C-Fos and C-Jun in Lungs of Rats. Environ. Sci. Pollut. Res. 22, 20167–20176. doi:10.1007/s11356-015-5222-z
Liu, Y., Wen, Q., Chen, X.-l., Yang, S.-j., Gao, L., Gao, L., et al. (2015). All-trans Retinoic Acid Arrests Cell Cycle in Leukemic Bone Marrow Stromal Cells by Increasing Intercellular Communication through Connexin 43-mediated Gap Junction. J. Hematol. Oncol. 8, 110. doi:10.1186/s13045-015-0212-7
Meira, L. B., Bugni, J. M., Green, S. L., Lee, C. W., Pang, B., Borenshtein, D., et al. (2008). DNA Damage Induced by Chronic Inflammation Contributes to Colon Carcinogenesis in Mice. J. Clin. Invest. 118, 2516–2525. doi:10.1172/JCI35073
Morrison, S. J., and Scadden, D. T. (2014). The Bone Marrow Niche for Haematopoietic Stem Cells. Nature 505, 327–334. doi:10.1038/nature12984
Pfeffer, P. E., and Mudway, I. S. (2018). The Impact of Real-World Particulate Matter Air Pollution on the Airways of Susceptible Individuals. Am. J. Respir. Crit. Care Med. 198, 1362–1363. doi:10.1164/rccm.201807-1206ed
Puett, R. C., Poulsen, A. H., Taj, T., Ketzel, M., Geels, C., Brandt, J., et al. (2020). Relationship of Leukaemias with Long-Term Ambient Air Pollution Exposures in the Adult Danish Population[J]. Br. J. Cancer 123, 1818–1824. doi:10.1038/s41416-020-01058-2
Raaschou-Nielsen, O., Ketzel, M., Harbo Poulsen, A., and Sørensen, M. (2016). Traffic-related Air Pollution and Risk for Leukaemia of an Adult Population. Int. J. Cancer 138, 1111–1117. doi:10.1002/ijc.29867
Ribeiro, A. G., Vermeulen, R., Cardoso, M. R. A., Latorre, M., Hystad, P., Downward, G. S., et al. (2021). Residential Traffic Exposure and Lymphohematopoietic Malignancies Among Children in the City of São Paulo, Brazil: An Ecological Study. Cancer Epidemiol. 70, 101859. doi:10.1016/j.canep.2020.101859
Simon, M. C., and Keith, B. (2008). The Role of Oxygen Availability in Embryonic Development and Stem Cell Function. Nat. Rev. Mol. Cell. Biol. 9, 285–296. doi:10.1038/nrm2354
Snyder, C. M., and Chandel, N. S. (2009). Mitochondrial Regulation of Cell Survival and Death during Low-Oxygen Conditions. Antioxidants Redox Signal. 11, 2673–2683. doi:10.1089/ars.2009.2730
Solan, J. L., and Lampe, P. D. (2009). Connexin43 Phosphorylation: Structural Changes and Biological Effects. Biochem. J. 419, 261–272. doi:10.1042/bj20082319
Su, R., Jin, X., Zhang, W., Li, Z., Liu, X., and Ren, J. (2017). Particulate Matter Exposure Induces the Autophagy of Macrophages via Oxidative Stress-Mediated PI3K/AKT/mTOR Pathway. Chemosphere 167, 444–453. doi:10.1016/j.chemosphere.2016.10.024
Tamayo-Uria, I., Boldo, E., García-Pérez, J., Gómez-Barroso, D., Romaguera, E. P., Cirach, M., et al. (2018). Childhood Leukaemia Risk and Residential Proximity to Busy Roads. Environ. Int. 121 (Pt 1), 332–339. doi:10.1016/j.envint.2018.08.056
Vattanasit, U., Navasumrit, P., Khadka, M. B., Kanitwithayanun, J., Promvijit, J., Autrup, H., et al. (2014). Oxidative DNA Damage and Inflammatory Responses in Cultured Human Cells and in Humans Exposed to Traffic-Related Particles. Int. J. Hyg. Environ. Health 217, 23–33. doi:10.1016/j.ijheh.2013.03.002
Ventura, L., Giovannini, A., Savio, M., Donà, M., Macovei, A., Buttafava, A., et al. (2013). Single Cell Gel Electrophoresis (Comet) Assay with Plants: Research on DNA Repair and Ecogenotoxicity Testing. Chemosphere 92, 1–9. doi:10.1016/j.chemosphere.2013.03.006
Keywords: PM2.5, Oxidative Stress, DNA Damage, Inflammatory reaction, Cx43protein, NF-κB
Citation: Liu L-L, Yang J, Ye Z-L, Tian C, Huang X-L, Zhang H-Q and Mo X-H (2022) Effects of Long-Term Exposure to PM2.5 on Oxidative Stress Injury and Expression of Inflammatory Factors, NF-κB p65 and Cx43 in Bone Marrow of Mice. Front. Environ. Sci. 10:747286. doi: 10.3389/fenvs.2022.747286
Received: 07 September 2021; Accepted: 06 May 2022;
Published: 08 June 2022.
Edited by:
Hideko Sone, Yokohama College of Pharmacy, JapanReviewed by:
Zhong-Ji Qian, Guangdong Ocean University, ChinaAnnamaria Colacci, Environment and Energy of Emilia-Romagna (ARPAE), Italy
Copyright © 2022 Liu, Yang, Ye, Tian, Huang, Zhang and Mo. This is an open-access article distributed under the terms of the Creative Commons Attribution License (CC BY). The use, distribution or reproduction in other forums is permitted, provided the original author(s) and the copyright owner(s) are credited and that the original publication in this journal is cited, in accordance with accepted academic practice. No use, distribution or reproduction is permitted which does not comply with these terms.
*Correspondence: Zhong-Lv Ye, zhonglv996@163.com
†These authors have contributed equally to this work