- 1National Ecological Observatory Network, Battelle Memorial Institute, Boulder, CO, United States
- 2Institute of Arctic and Alpine Research University of Colorado, Boulder, CO, United States
Quantifying the resilience of ecological communities to increasingly frequent and severe environmental disturbance, such as natural disasters, requires long-term and continuous observations and a research community that is itself resilient. Investigators must have reliable access to data, a variety of resources to facilitate response to perturbation, and mechanisms for rapid and efficient return to function and/or adaptation to post-disaster conditions. There are always challenges to meeting these requirements, which may be compounded by multiple, co-occurring incidents. For example, travel restrictions resulting from the COVID-19 pandemic hindered preparations for, and responses to, environmental disasters that are the hallmarks of resilient research communities. During its initial years of data collection, a diversity of disturbances—earthquakes, wildfires, droughts, hurricanes and floods—have impacted sites at which the National Ecological Observatory Network (NEON) intends to measure organisms and environment for at least 30 years. These events strain both the natural and human communities associated with the Observatory, and additional stressors like public health crises only add to the burden. Here, we provide a case-study of how NEON has demonstrated not only internal resilience in the face of the public health crisis of COVID-19, but has also enhanced the resilience of ecological research communities associated with the network and provided crucial information for quantifying the impacts of and responses to disturbance events on natural systems—their ecological resilience. The key components discussed are: 1) NEON’s infrastructure and resources to support its core internal community, to adapt to rapidly changing situations, and to quickly resume operations following disruption, thus enabling the recovery of information flow crucial for data continuity; 2) how NEON data, tools, and materials are foundational in supporting the continuation of research programs in the face of challenges like those of COVID-19, thus enhancing the resilience of the greater ecological research community; and 3) the importance of diverse and consistent data for defining baseline and post-disaster conditions that are required to quantify the effects of natural disasters on ecosystem patterns and processes.
Introduction
Resilience in the face of environmental disaster is particularly critical at a time when continually rising global mean surface temperature is causing substantial increases in the frequency and/or severity of critical events like floods, wildfires, hurricanes, and droughts (Romero-Lankao et al., 2014). The concept of resilience, however, is complex and multifaceted. For natural ecosystems, resilience is a measure of how well a system withstands or adapts to stress or disturbance, and/or how long it takes for stable states to be reached following the events (Gunderson 2000; Falk et al., 2019). Continuous ecological data are critical for informing these measurements. For research communities studying natural ecosystems the definition is similar, but oriented toward the capacity of investigators and educators to withstand or adapt to stress or disturbance, and the time required to return to a stable state of being. Additional sociological factors are important for measuring research community resilience. For example, the forms of capital available during recovery from natural disasters, and capacity of individuals to learn following these events, impact overall community resilience (Gunderson, 2010).
Evaluating resilience capacity requires uninterrupted data collection before, during, and after disturbance events, with years of planning, numerous and repeated measurements, and the flexibility to adapt to changing conditions. This is exceptionally challenging, particularly as most disturbance events are not known in advance and are extremely disruptive. For example, the 2020 COVID-19 outbreak was followed by abrupt travel and social-distancing restrictions that impacted data collection, instrument maintenance, and information flow. Almost 2 years into the pandemic, the effects of COVID-19 on environmental research programs are ongoing, and they vary from the continuation of the programs, albeit with data gaps (Bourzac 2020; Viglione 2020), to altered design or objectives (i.e., Bourzac 2020; Stokstad 2020), to temporary or possibly permanent cessation of entire studies (Perinchery 2020). The programs most resilient to public health crises like COVID-19 are those with stable infrastructures and large resource pools, that can remain engaged in the absence of data collection and then quickly resume activities when restrictions lift.
The National Ecological Observatory Network (NEON) is a National Science Foundation (NSF) funded large-scale scientific research infrastructure program, managed by Battelle, that is designed to provide a wide array of consistently collected, standardized, and easily integrated datasets for a variety of environmental measurements in terrestrial and aquatic ecosystems for at least 30 years. Data are collected across the continental United States, Hawaii, Alaska, and Puerto Rico, and they are freely available to the public. Given the large temporal and geographic coverage, the Observatory is positioned to provide critical information to researchers investigating ecological resilience in the face of environmental disaster. Additionally, several components of NEON’s stable infrastructure can remain operable in periods of restricted site visitation, and its large staffing and equipment resources support continued community engagement and a rapid return to operations following disruptions.
Context
This case study explores several aspects of resilience in the face of environmental disaster during COVID-19. First, we discuss the NEON program’s internal resilience during the public health crisis. Next, we demonstrate how NEON provides support to the broader ecological research community during various disruptions, enhancing the resilience of this community. Finally, we explore how NEON data reveal immediate impacts of critical events and may be used in the future to assess longer-term resilience of ecosystems to environmental disasters.
Organismal and meteorological data used here are from the 2021 Data Release1 and provisional data from the NEON data portal. Released data are available through June 2020 for Instrumented Systems (IS) data products, and through December 2019 for Observational Systems (OS) data products. Users will see two data references when both provisional and released data are utilized in this work.
Detail
Resilience During a Pandemic
Two overarching characteristics of the NEON program have contributed to resiliency during the COVID-19 pandemic: a stable data collection infrastructure and robust staffing resources. The first is a result of almost two decades of careful design and planning (Collinge 2018), culminating in an Observatory engineered with many automated data streams that have continued to provide measurements even as human site access has been limited during COVID-19. For observational data, which must be collected by people, Battelle’s internal leadership, resources, and design infrastructure have minimized data loss. Of primary importance, the Observatory has a nimble staffing pool with personnel that are often cross trained on multiple protocols for instrument maintenance, sampling, lab analysis, and data management. When the NSF supported seasonal staff retention despite uncertain field access due to the pandemic, this workforce was able to redirect efforts toward other Observatory functions and scientific community engagement. For example, NEON’s Domain 17 (California) staff used this time to prepare for and host a virtual “Explore NEON” workshop2. As a result of this level of flexibility, the Observatory could hire its full pool of approximately 250 seasonal staff in early 2020 and maintain the productivity of this workforce during the subsequent months. Such a commitment to staff retention is especially important for the early career scientists composing much of NEON’s seasonal staff pool, who need temporary field and lab jobs to gain experience and bolster resumes (Corlett et al., 2020). Staff retention also allowed the Observatory to rapidly redeploy field collections when pandemic-related restrictions lifted. NEON’s staffing structure is a key element behind the Observatory’s resilience and ability to support its internal community during disruptions like COVID-19.
Supporting the Ecological Research Community
Staff retention during the COVID-19 public health crisis demonstrates one way in which NEON meets researcher’s basic needs and supports community resilience internally, but there is also evidence that the Observatory lends support to the broader ecological research community. First and foremost, studies already relying on NEON data have been able to continue largely uninterrupted due to the Observatory’s data collection capabilities, as discussed above. Additionally, evidence suggests that NEON data are in fact helping sustain growing numbers of research programs, particularly in the time of COVID-19. Since April 2019, the Observatory has seen marked increases in: unique IP addresses using NEON’s Application Programming Interface (API) for information and data downloads; CRAN downloads of the neonUtilities code package (Laney and Lunch, 2019), which provides programmatic tools for data download, discovery, and analysis; and requests for assignable assets, a program through which researchers may utilize some components of NEON’s infrastructure [i.e., sensors, staff time, the Airborne Observation Platform (AOP), etc.] for their own work. For each of these services, however, the US declaration of a public health emergency due to the COVID-19 outbreak marked the beginning of elevated overall level of usage (Figure 1), with awareness of their availability and value potentially raised by the onset of the pandemic.
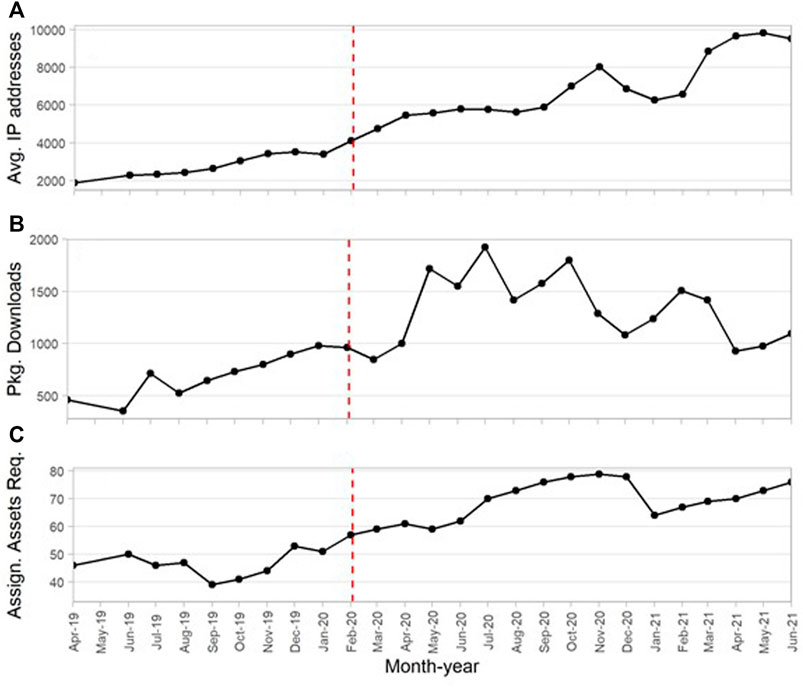
FIGURE 1. Increases in the mean number of unique IP addresses using NEON’s API, averaged over a 3-months rolling window (A), downloads of the neonUtilities code package (B), and NEON assignable asset requests (C). The red dashed line indicates the date the US declared a public health emergency due to the COVID-19 outbreak (03 February 2020—Staff, 2020). Note that in panel (A) there is a missing data point for May 2019 due to an internal system administration error, which deleted log files.
It is important to acknowledge that while staff retention and continued data provision are essential components of meeting the ecological research communities’ needs in the face of pandemics and natural disasters, there are additional stakeholders to consider in assessing overall community resilience. Such overarching community resilience is not explored in this case study because we do not feel that NEON data are well suited to assessing social conditions pre- and post-disaster, and thus providing insight into community resilience at this scale.
Assessing Environmental Resilience in the Face of Natural Disaster
We have thus far focused on the resilience of the NEON Observatory and its support of the ecological research community in the context of the COVID-19 pandemic. However, NEON data are also important for quantifying the resilience of ecological communities to natural disasters. As previously highlighted, the spatial and temporal breadth of NEON data collection uniquely position the Observatory to provide information for studies of this nature. As follows, although full operations were achieved in May 2019, data have been collected at several NEON sites in years prior to this time and this sometimes coincided with natural disasters and anthropogenic site disturbance—events which can be further explored in NEON’s Site management and event reporting data product (NEON data product catalgue). Measurements taken before and after these events facilitate investigations into their direct and indirect impacts on ecological communities, and short-term effects. And the 30-years duration of the NEON project means there will continuous data available for future evaluations of the long-term effects of COVID-19 and natural disturbances on ecosystem composition and function. Here, we provide a few preliminary explorations of how some of NEON’s 181 data products demonstrate trends and patterns that are important for research into the impacts of natural disturbance on ecological patterns and processes.
Hurricanes have already repeatedly impacted sites at which NEON collects data. In October of 2018, during the highly destructive North Atlantic hurricane season (Saunders et al., 2020), hurricane Michael swept through the Jones Ecological Research Center (JERC) site in Georgia as a category five storm. Direct impacts of this event are captured in several NEON data products, with sensor measurements recording until power was no longer available and data collection resuming immediately upon its restoration 2 weeks later. These include meteorological data products like mean barometric pressure (NEON, 2022a), which dropped precipitously before the hurricane (Figure 2A), and automated Phenology images (Seyednasrollah et al., 2019b). Imagery from before and after the hurricane not only confirm the immediate effects of significant winds on the plant community, with notable canopy defoliation following the event (Figure 2B), but also enable the study of longer-term impacts and resilience. For example, vegetative regrowth and/or change can be quantified with derived metrics like Green Chromatic Coordinate (GCC). This measure of canopy greenness (Brown et al., 2016) is freely available from the PhenoCam Network3 for NEON images [methods and information in Seyednasrollah et al. (2019]). When NEON Phenology images became available again following hurricane Michael, mean daily GCC of deciduous broadleaf trees was well below the 3-years average (0.3586) for the date (Figure 2C). Furthermore, data from spring to fall of the year following the hurricane indicate that mean daily GCC did not immediately rebound (with a maximum of 0.3995 and 0.3622 in 2017 and 2019, respectively), due to persistent reductions in canopy cover. The impacts of the hurricane were evident in other vegetative growth forms as well. Evergreen conifers within view of the PhenoCam, for example, were reduced to a degree that the region of interest (ROI) mask used to calculate GCC for this vegetation type was re-drawn following the storm (see Supplementary Figure S1.1).
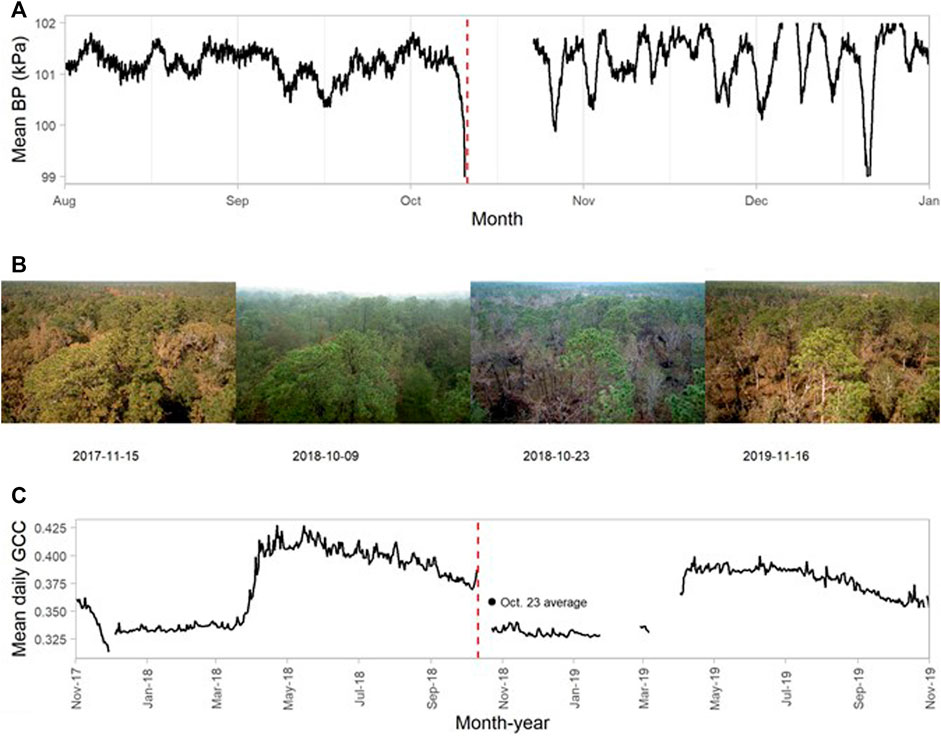
FIGURE 2. NEON data capturing the impacts of hurricane Michael on mean daily barometric pressure in 2018 (A) and canopy cover across growing seasons (B), with daily mean GCC measurements from the PhenoCam Network to quantify canopy greenness for deciduous broadleaf trees across months and years (C). The red dashed line indicates power loss due to the hurricane, which took sensors and cameras offline and resulted in the subsequent data gap. Data collection resumed on 23 October 2018, and the 3-years mean daily GCC average for this data is shown for comparison.
The intense rains that accompany hurricanes also have serious consequences for natural ecosystems, particularly in relatively dry regions. The Guánica Dry Forest Reserve (GUAN) site lies in Puerto Rico’s coastal subtropical dry zone, where average annual rainfall (∼84 cm4) is considerably lower than it is anywhere else on the island (U.S. Geological Survey, 2021). In September 2017, back-to-back hurricanes Irma and Maria deposited upward of 23 cm of rain (∼5–7.6 and ∼18 cm, respectively; National Weather Service 2017a; National Weather Service 2017b) at this site in just 2 weeks. This has important implications for soil microbial community structure and function, which responds to alterations in soil water and nutrient availability in varying ways (Zeglin et al., 2013; Zhao et al., 2016; Zhao et al., 2018). Cursory exploration of NEON’s soil microbe community composition data product (NEON, 2022b) from GUAN supports this varied response and demonstrates the value of NEON data for ecologists working to better understand disparities in the effects of natural disasters on microbial communities. Figure 3 shows fungal diversity, as measured by the average number of classes identified by Internal Transcribed Spacer (ITS) sequencing, across various pre- and post-hurricane sampling bouts for plots within GUAN. Some plots demonstrate a significant reduction in fungal diversity between pre- and post-hurricane sampling periods (e.g., GUAN_006 and GUAN_043), while fungal diversity is largely stable over time, to varying degrees, in others. Additional analyses would allow researchers to better quantify the relative abundances of various community members, ratios of bacteria to fungi, and other metrics important for understanding the nature of ecosystem change. These metrics could then be combined with additional NEON soil biogeochemical and organismal data products to fully examine ecosystem response and resilience to these natural disasters.
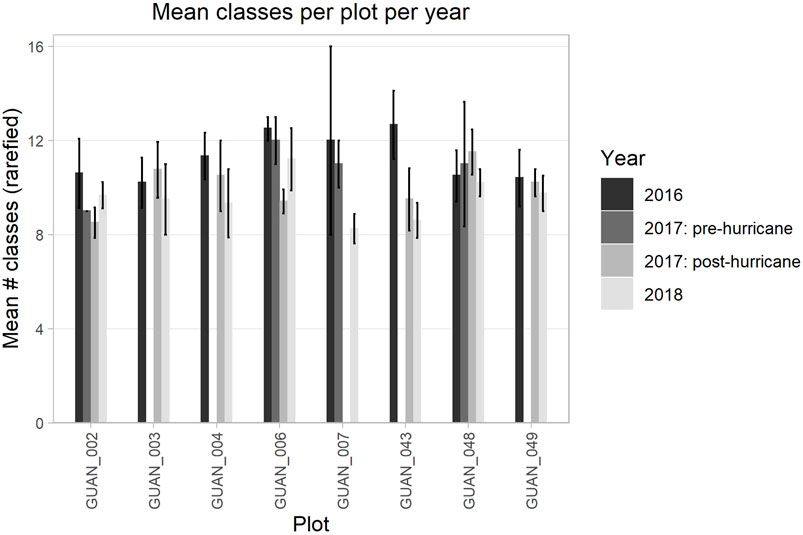
FIGURE 3. Mean number of classes of soil fungi, with standard errors, across plots and years at GUAN - with 2017 data split into pre- and post-hurricane data collection events. To calculate the mean number of classes, NEON’s raw abundance estimates were first grouped by sample and then rarefied using the vegan package (Oksanen et al., 2020; RRID:SCR_011950) in R (R Core Team 2020). Plots represented are only those with a full complement (at least two samples per plot per sampling period) of data. Finally, only fungal data are shown due to ongoing data corrections in provisional microbial abundance records at the time of this writing.
The Puerto Rican sites of Río Cupeyes (CUPE) and Río Guilarte (GUIL) were also impacted by hurricanes Irma and Maria, which caused severe flooding. Photos from these inland, freshwater systems show flood-induced changes to stream morphology, with downed trees, bank erosion, and significant scouring (Supplementary Figure S1.2). Such alterations to aquatic systems are known to impact macroinvertebrate communities, causing changes in patterns of richness and abundance (Covich et al., 2006; Engle et al., 2009). Data from NEON’s Macroinvertebrate collection data product (NEON, 2022d) can help researchers better understand these phenomena and assess variation in response among disparate communities. CUPE, for example, is a relatively pristine aquatic environment where early explorations of NEON data indicate the flooding may have introduced instability to macroinvertebrate community dynamics. Figure 4A suggests the dominant macroinvertebrate classes remained dominant following the floods, but with larger inter-bout variability in relative mean abundance among classes than was previously seen. Additional factors not shown here, such as periphyton and detritus nutrient sources, may also be expected to exhibit varied responses to the floods (Covich et al., 2006). Conversely, the impact of flooding on GUIL, which is surrounded by managed agricultural landscape, may have been less pronounced despite arguably greater changes to stream morphology at this site (Supplementary Figures S1.2C,D). In this system, overall community composition and mean abundance patterns appear to be relatively seasonal, and the catastrophic floods of 2017 seemed to have little effect on these patterns (Figure 4B). This is similar to findings by Covich et al. (2006), where palaemonid shrimp at the Luquillo Mountains Long-Term Ecological Research (LTER) site were relatively unaffected by hurricanes and storm flows. NEON data are ideal for further investigation of these, and other related patterns, to elucidate causes and long-term consequences and better quantify variability in community resilience in the face of natural disaster.
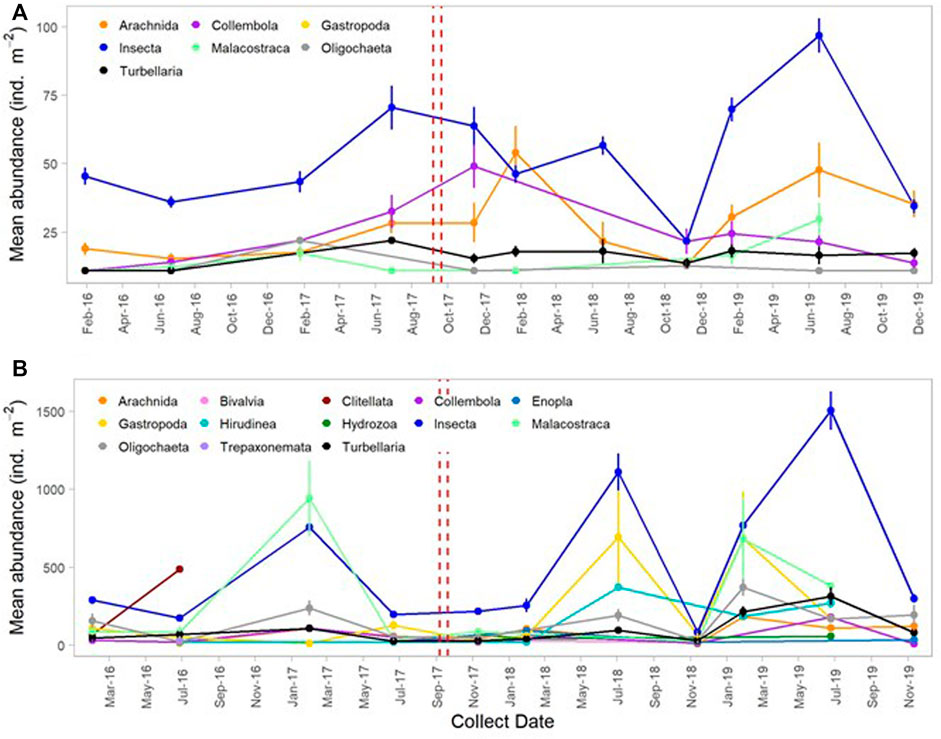
FIGURE 4. Mean abundance (per meter squared) of each taxonomic class of macroinvertebrate present at CUPE (A) and GUIL (B) across collection dates (a.k.a. collection “bouts”) spanning the occurrences of hurricanes Irma and Maria (dashed lines). Raw abundance estimates are divided by total sampling area to normalize data collected via differing sampling methods, and error bars are standard errors.
In addition to hurricanes, non-hurricane-related floods, earthquakes, droughts, and wildfires have all occurred at NEON sites during early data collection (pre-2020). Wildfires generate particular interest among environmental researchers, and several studies have been conducted or are currently under way to quantify the effects of this disturbance on: soil organic matter (SOM) dynamics (Matosziuk et al., 2020); Biomass Burning Fluxes (BB-Flux) of trace gases and aerosols5; post-fire water quality (Egan 2020), sediment storage, and soil erosion (Brogan et al., 2019a; Brogan et al., 2019b); flood dynamics (Brogan et al., 2017); and more. In 2020, during the COVID-19 pandemic, California experienced a particularly severe wildfire season, with over 9,500 fires that burned more than 4 million acres (CalFire 2021). Several of these incidents occurred in or near the US Forest Service site of Soaproot Saddle (SOAP), and approximately half of the area where NEON sampling occurs burned. As seen in NEON’s phenology imagery, the sky above SOAP was filled with thick smoke on 07 September 2020, just before power to the area was cut (Figures 5A,B). Also evident from NEON data streams were the drought conditions leading up to these events. Single aspirated air temperature (NEON, 2022c; NEON, 2022e) demonstrated higher than normal mean temperatures in the months preceding the fires compared with the same period in previous years (Figure 5C). Likewise, precipitation (NEON, 2022f; NEON, 2022g) showed a general absence of the typical monsoonal rains in the winter and spring before the events (Figure 5D). As more NEON data become available, they will further enable researchers to investigate the direct and indirect, and short- and long-term impacts of these natural disasters. For example, the drought conditions before the fires and poor air quality during may impact additional ecosystems dynamics like canopy foliar chemistry and water quality.
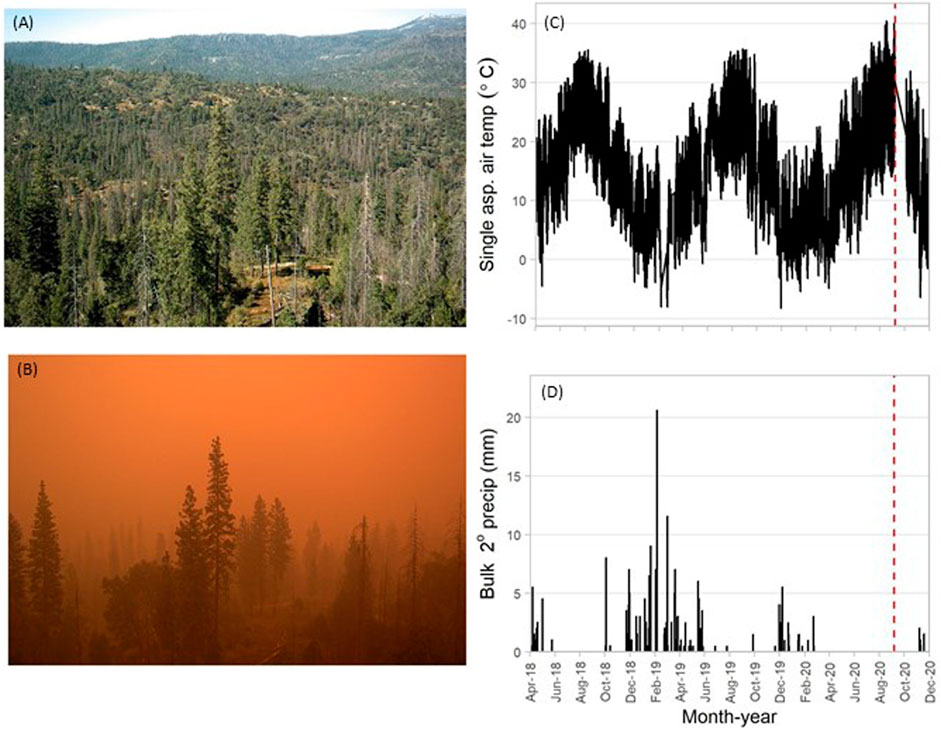
FIGURE 5. NEON data relating to the fires in the area of the SOAP site. Phenology images show clean air on 06 August 2020 (A) replaced by smoke-filled skies on 07 September 2020 (B), mean, averaged over 30 min, single aspirated air temperature (C), and bulk secondary precipitation (total accumulated precipitation measured with a tipping bucket located at the top of the NEON Meteorological Flux Tower), also averaged over 30 min (D). The red dashed line indicates the date power was cut to the site. Note: data preceding June 2020 are released NEON data, while those following this date are provisional.
Discussion
Altered climate regimes and increasing anthropogenic pressure will continue to bring about disturbances that drive environmental change. The NEON infrastructure represents a commitment to documenting this change by providing consistent measurements across large geographic extents and over many years. These measurements chronicle ecological states before and after disturbance and are crucial for understanding shifting ecological patterns and community resilience. The types of “big science” initiatives NEON represents have seen growing support as researchers recognize that the greatest needs in ecology (see Grand Challenges in Environmental Sciences, 2001; National Research Council, 2004) may not be easily addressed by the intensive study of a single site (Heffernan et al., 2014; Levy et al., 2014). Spatially and temporally robust “big data” may thus be important for elucidating emergent ecological properties associated with major environmental challenges (Hampton et al., 2013; LaDeau et al., 2017; Peters and Okin 2017). This may be particularly true for ecological forecasting studies, which rely on data-model-loops that iteratively test continually updating predictions against novel data (Dietze et al., 2018).
The recent shift toward adopting network data is demonstrated in Figure 1, with NEON data and resource usage having steadily increased for several years. In 2020, however, COVID-19 caused a perturbation to the ecological research community that has arguably accelerated this paradigm shift. Data collection capabilities of big science initiatives like NEON may never have been so important as during the unusually large reduction in human activities, dubbed the “anthropause” (Rutz et al., 2020; Stokstad 2020), of the pandemic. Beyond providing data when many researchers were unable to generate their own, however, disruptions caused by the COVID-19 pandemic demonstrated additional roles of large-scale scientific research infrastructure programs in broader community resilience. With considerable resource pools and organizational infrastructure, programs like NEON may provide critical resources to both internal and external communities during crises. For example, the flooding resulting from hurricanes in Puerto Rico devastated infrastructure and livelihood on the island. Battelle not only continued to provide stable jobs to its employees, but also delivered aid and assistance in the aftermath of the storms, including food, water, communication systems, emergency medical supplies, and financial resources to facilitate recovery (Inside Battelle 2017). By investing in people and contributing to the resilience of the broader community, Battelle was able to both foster confidence in the NEON Observatory’s presence on the island and also ensure the integrity of the Observatory and minimize gaps in the data that facilitate an understanding of how ecological communities respond to these same events.
Even with their capabilities, large networks do not provide perfect solutions to quantifying and assessing resilience in the face of environmental disaster in the age of COVID-19. Travel restrictions and flooding still delay observational data collection at sites, and automated instrument streams are still disrupted by maintenance issues, hurricanes, and fires. But our explorations demonstrate that the scope and breadth of NEON’s measurements provide important information for investigations of ecological resilience during natural and public health disturbance, and these features may overcome short-term data disruptions. This is particularly true when NEON data are combined with those from other networks. New innovations, driven by a resilient ecological research community, also continually augment the existing strengths of large networks. For example, NEON is assessing how effectively drones might collect some of its measurements and prevent future data disruption (NEON, 2020). Existing ecological data also could be combined with satellite data, and the National Aeronautical Space Association (NASA) has recently funded several projects exploring connections between the environment and COVID-19 using satellites6, because these instruments remain operational despite disruptions like the pandemic. These and other projects will bring about fresh perspectives and new insights into ecosystem and research community resilience. A more robust understanding of community resilience requires additional data that address sociological components of interactions between the research community and biophysical conditions.
Acknowledgment of Conceptual or Methodological Constraints
It is important to note that this community case study uses some provisional NEON data7. These data are dynamic and can be updated at any time, without guarantee of reproducibility. They are provided on the NEON data portal in order to allow for near-immediate availability but may be corrected or added to as needed.
Figures presented here include data for which quality flags were found to be acceptable during processing. Quality flagging is applied individually per data product, and more information can be found in data product downloads on the NEON data portal.
Data Availability Statement
All data used in this case study are publicly available. The data can be found in the supplementary materials (Supplementary Data 2.1) or obtained from the NEON data portal or PhenoCam Network websites. API calls using the neonUtilities and phenocamapi (Seyednasrollah 2019) packages are provided in the Supplementary Data 2.2 and 2.3.
Author Contributions
NR conceived of and wrote this manuscript. DB, KJ, LS, and SP contributed content, analysis, and editing support.
Funding
The National Ecological Observatory Network is a program sponsored by the National Science Foundation and operated under cooperative agreement by Battelle Memorial Institute. This material is based in part upon work supported by the National Science Foundation through the NEON Program (NSF #1724433).
Conflict of Interest
The authors declare that the research was conducted in the absence of any commercial or financial relationships that could be construed as a potential conflict of interest.
Publisher’s Note
All claims expressed in this article are solely those of the authors and do not necessarily represent those of their affiliated organizations, or those of the publisher, the editors and the reviewers. Any product that may be evaluated in this article, or claim that may be made by its manufacturer, is not guaranteed or endorsed by the publisher.
Acknowledgments
We appreciate comments and editing support from Z. Gentes, K. Thibault, P. Mabee, and two reviewers.
Supplementary Material
The Supplementary Material for this article can be found online at: https://www.frontiersin.org/articles/10.3389/fenvs.2022.653666/full#supplementary-material
Footnotes
1https://www.neonscience.org/data-samples/data-management/data-revisions-releases/release-2021
2https://www.neonscience.org/get-involved/events/explore-neon-workshop-domain-17
3https://phenocam.sr.unh.edu/webcam/about/
4https://www.neonscience.org/field-sites/guan
5https://volkamergroup.colorado.edu/timeline/field/bb-flux
6https://www.nasa.gov/feature/esd/2020/new-projects-explore-connections-between-environment-and-covid-19
7https://www.neonscience.org/data-samples/data-management/data-revisions-releases
References
Bourzac, K. (2020). COVID-19 Has Disrupted Fieldwork. Here’s How Environmental Chemists Are Coping. Chem. Eng. News 98, 25. Available at: https://cen.acs.org/environment/climate-change/COVID-19-disrupted-fieldwork-how-environmental-chemists-are-coping/98/i25 (Accessed January 7, 2021). doi:10.1021/cen-09825-feature1
Brogan, D. J., MacDonald, L. H., Nelson, P. A., and Morgan, J. A. (2019b). Geomorphic Complexity and Sensitivity in Channels to Fire and Floods in Mountain Catchments. Geomorphology 337, 53–68. doi:10.1016/j.geomorph.2019.03.031
Brogan, D. J., Nelson, P. A., and MacDonald, L. H. (2017). Reconstructing Extreme post‐wildfire Floods: a Comparison of Convective and Mesoscale Events. Earth Surf. Process. Landforms 42, 2505–2522. doi:10.1002/esp.4194
Brogan, D. J., Nelson, P. A., and MacDonald, L. H. (2019a). Spatial and Temporal Patterns of Sediment Storage and Erosion Following a Wildfire and Extreme Flood. Earth Surf. Dynam. 7, 563–590. doi:10.5194/esurf-7-563-2019
Brown, T. B., Hultine, K. R., Steltzer, H., Denny, E. G., Denslow, M. W., Granados, J., et al. (2016). Using Phenocams to Monitor Our Changing Earth: Toward a Global Phenocam Network. Front. Ecol. Environ. 14, 84–93. doi:10.1002/fee.1222
CalFire (2021). 2020 Incident Archive. Available at: https://www.fire.ca.gov/incidents/2020/ (Accessed January 7, 2021).
Collinge, S. K. (2018). NEON Is Your Observatory. Front. Ecol. Environ. 16, 371. doi:10.1002/fee.1939
Committee on Grand Challenges in Environmental Sciences (2001). Grand Challenges in Environmental Sciences. Washington, D.C: National Academy Press. doi:10.17226/9975
Corlett, R. T., Primack, R. B., Devictor, V., Maas, B., Goswami, V. R., Bates, A. E., et al. (2020). Impacts of the Coronavirus Pandemic on Biodiversity Conservation. Biol. Conservation 246, 108571. doi:10.1016/j.biocon.2020.108571
Covich, A. P., Crowl, T. A., and Heartsill-Scalley, T. (2006). Effects of Drought and hurricane Disturbances on Headwater Distributions of Palaemonid River Shrimp (Macrobrachium spp.) in the Luquillo Mountains, Puerto Rico. J. North. Am. Benthol. Soc. 25. doi:10.1899/0887-3593(2006)25[99:eodahd]2.0.co;2
Dietze, M. C., Fox, A., Beck-Johnson, L. M., Betancourt, J. L., Hooten, M. B., Jarnevich, C. S., et al. (2018). Iterative Near-Term Ecological Forecasting: Needs, Opportunities, and Challenges. Proc. Natl. Acad. Sci. USA 115, 1424–1432. doi:10.1073/pnas.1710231115
Egan, J. K. (2020). Exploring post-wildfire Water Quality: The Photodegradation of Dissolved Pyrogenic Carbon. Master’s thesis. Boulder (CO): University of Colorado.
Engle, V. D., Hyland, J. L., and Cooksey, C. (2009). Effects of Hurricane Katrina on Benthic Macroinvertebrate Communities along the Northern Gulf of Mexico Coast. Environ. Monit. Assess. 150, 193–209. doi:10.1007/s10661-008-0677-8
Falk, D. A., Watts, A. C., and Thode, A. E. (2019). Scaling Ecological Resilience. Front. Ecol. Evol. 7. doi:10.3389/fevo.2019.00275
Gunderson, L. (2010). Ecological and Human Community Resilience in Response to Natural Disasters. E&S 15. doi:10.5751/ES-03381-150218
Gunderson, L. H. (2000). Ecological Resilience-In Theory and Application. Annu. Rev. Ecol. Syst. 31, 425–439. doi:10.1146/annurev.ecolsys.31.1.425
Hampton, S. E., Strasser, C. A., Tewksbury, J. J., Gram, W. K., Budden, A. E., Batcheller, A. L., et al. (2013). Big Data and the Future of Ecology. Front. Ecol. Environ. 11, 156–162. doi:10.1890/120103
Heffernan, J. B., Soranno, P. A., Angilletta, M. J., Buckley, L. B., Gruner, D. S., Keitt, T. H., et al. (2014). Macrosystems Ecology: Understanding Ecological Patterns and Processes at continental Scales. Front. Ecol. Environ. 12, 5–14. doi:10.1890/130017
Inside Battelle (2017). Employees Helping Employees after Hurricane Maria. Available at: https://inside.battelle.org/blog-details/employees-helping-employees-after-hurricane-maria (Accessed January 11, 2021).
LaDeau, S. L., Han, B. A., Rosi-Marshall, E. J., and Weathers, K. C. (2017). The Next Decade of Big Data in Ecosystem Science. Ecosystems 20, 274–283. doi:10.1007/s10021-016-0075-y
Laney, C., and Lunch, C. (2019). Package ‘neonUtilities’’ - Utilities for Working with NEON Data. CRAN Repos. Available at https://cran.r-project.org/web/packages/neonUtilities
Levy, O., Ball, B. A., Bond-Lamberty, B., Cheruvelil, K. S., Finley, A. O., Lottig, N. R., et al. (2014). Approaches to advance Scientific Understanding of Macrosystems Ecology. Front. Ecol. Environ. 12, 15–23. doi:10.1890/130019
Matosziuk, L. M., Gallo, A., Hatten, J., Bladon, K. D., Ruud, D., Bowman, M., et al. (2020). Short-Term Effects of Recent Fire on the Production and Translocation of Pyrogenic Carbon in Great Smoky Mountains National Park. Front. For. Glob. Change 3. doi:10.3389/ffgc.2020.00006
National Ecological Observatory Network (2022a). Barometric Pressure. RELEASE-2021 (DP1.00004.001)Dataset accessed from Available at: https://data.neonscience.org (on January 10, 2022). doi:10.48443/rxr7-pp32
National Ecological Observatory Network (2020). Blog. Ecology and COVID #3: Can Technology Fill the Coronavirus Data Gap? Ecotone | News and Views on Ecological Science. Available at: https://www.esa.org/esablog/guest-posts/ecology-and-covid-3-can-technology-fill-the-coronavirus-data-gap/ (Accessed January 6, 2021).
National Ecological Observatory Network (2022d). Macroinvertebrate Collection. RELEASE-2021 (DP1.20120.001)Dataset accessed from https://data.neonscience.org (on January 10, 2022). doi:10.48443/855x-0n27
National Ecological Observatory Network (2022f). Precipitation. RELEASE-2021 (DP1.00006.001)Dataset accessed from https://data.neonscience.org (on January 10, 2022).doi:10.48443/rpms-dx03
National Ecological Observatory Network (2022g). Precipitation. (DP1.00006.001). Available at: https://data.neonscience.org (accessed January 10, 2022).
National Ecological Observatory Network (2022c). Single Aspirated Air Temperature. RELEASE-2021 (DP1.00002.001)Dataset accessed from https://data.neonscience.org (on January 10, 2022).doi:10.48443/2nt3-wj42
National Ecological Observatory Network (2022e). Single Aspirated Air Temperature. (DP1.00002.001). Available at: https://data.neonscience.org (accessed January 10, 2022).
National Ecological Observatory Network (2022b). Soil Microbe Community Composition. RELEASE-2021 (DP1.10081.001)Dataset accessed from https://data.neonscience.org (on January 10, 2022).doi:10.48443/3czr-g515
National Research Council (2004). Neon: Addressing the Nation's Environmental Challenges. Washington, DC: The National Academies Press. doi:10.17226/10807
National Weather Service (2017b). Major Hurricane. September 20, 2017. Available at: https://www.weather.gov/sju/maria2017 (Accessed January 5, 2021).
National Weather Service (2017a). Major Hurricane Irma. September 20, 2017. Available at: https://www.weather.gov/sju/irma2017 (Accessed January 5, 2021).
Oksanen, J., Blanchet, F. G., Friendly, M., Kindt, R., Legendre, P., Mcglinn, D., et al. (2020). Package “Vegan” Title Community Ecology Package. R 5 (7), 2.
Perinchery, A. (2020). How COVID-19 Has Hurt Long-Term Ecological Monitoring Research in India. Menlo Park, CA: Mongabay. Available at: https://india.mongabay.com/2020/11/covid-19-cripples-long-term-ecological-monitoring-research-in-india/ (Accessed January 6, 2021).
Peters, D. P. C., and Okin, G. S. (2017). A Toolkit for Ecosystem Ecologists in the Time of Big Science. Ecosystems 20, 259–266. doi:10.1007/s10021-016-0072-1
R Core Team (2020). R: A Language and Environment for Statistical Computing. Vienna, Austria: R Foundation for Statistical Computing. URL Available at: https://www.R-project.org/.
Romero-Lankao, P., Smith, J. B., Davidson, D. J., Diffenbaugh, N. S., Kinney, P. L., Kirshen, P., et al. (2014). “North America,” in Climate Change 2014: Impacts, Adaptation, and Vulnerability. Part B: Regional Aspects. Contribution of Working Group II to the Fifth Assessment Report of the Intergovernmental Panel on Climate Change. Editors V. R. Barros, C. B. Field, D. J. Dokken, M. D. Mastrandrea, K. J. Mach, D. E. Biliret al. (Cambridge, New York: Cambridge University Press), 1439–1498.
Rutz, C., Loretto, M.-C., Bates, A. E., Davidson, S. C., Duarte, C. M., Jetz, W., et al. (2020). COVID-19 Lockdown Allows Researchers to Quantify the Effects of Human Activity on Wildlife. Nat. Ecol. Evol. 4, 1156–1159. doi:10.1038/s41559-020-1237-z
Saunders, M. A., Klotzbach, P. J., Lea, A. S. R., Schreck, C. J., and Bell, M. M. (2020). Quantifying the Probability and Causes of the Surprisingly Active 2018 North Atlantic Hurricane Season. Earth Space Sci. 7. doi:10.1029/2019EA000852
Seyednasrollah, B. (2019). Package “Phenocamapi”: Interacting with the PhenoCam Data and API. CRAN Repos. Available at https://github.com/bnasr/phenocamapi
Seyednasrollah, B., Young, A. M., Hufkens, K., Milliman, T., Friedl, M. A., Frolking, S., et al. (2019b).PhenoCam Dataset v2.0: Vegetation Phenology from Digital Camera Imagery, 2000-2018. Tennessee, USA: ORNL DAAC, Oak Ridge. Dataset accessed from https://phenocam.sr.unh.edu (on January 10, 2022). doi:10.3334/ORNLDAAC/1674
Seyednasrollah, B., Young, A. M., Hufkens, K., Milliman, T., Friedl, M. A., Frolking, S., et al. (2019). Tracking Vegetation Phenology across Diverse Biomes Using Version 2.0 of the PhenoCam Dataset. Sci. Data 6. doi:10.1038/s41597-019-0229-9
Stokstad, E. (2020). Pandemic Lockdown Stirs up Ecological Research. Science 369, 893. doi:10.1126/science.369.6506.893
U.S. Geological Survey (2021). Climate of Puerto Rico. Available at: https://www.usgs.gov/centers/car-fl-water/science/climate-puerto-rico?qt-science_center_objects=0#qt-science_center_objects (Accessed January 8, 2021).
Viglione, G. (2020). How COVID-19 Could Ruin Weather Forecasts and Climate Records. Nature 580, 440–441. doi:10.1038/d41586-020-00924-6
Zeglin, L. H., Bottomley, P. J., Jumpponen, A., Rice, C. W., Arango, M., Lindsley, A., et al. (2013). Altered Precipitation Regime Affects the Function and Composition of Soil Microbial Communities on Multiple Time Scales. Ecology 94, 2334–2345. doi:10.1890/12-2018.1
Zhao, C., Miao, Y., Yu, C., Zhu, L., Wang, F., Jiang, L., et al. (2016). Soil Microbial Community Composition and Respiration along an Experimental Precipitation Gradient in a Semiarid Steppe. Sci. Rep. 6. doi:10.1038/srep24317
Keywords: natural disaster, resilience, NEON, COVID-19, ecosystem, disturbance
Citation: Robinson N, Barnett DT, Jones KD, Stanish LF and Parker SM (2022) Multiple Dimensions of Resilience: How NEON Supports Ecology and the Research Community in the Face of Compounding Disasters. Front. Environ. Sci. 10:653666. doi: 10.3389/fenvs.2022.653666
Received: 14 January 2021; Accepted: 08 February 2022;
Published: 16 March 2022.
Edited by:
Sumana Chattopadhyay, Marquette University, United StatesReviewed by:
Arren Mendezona Allegretti, Santa Clara University, United StatesMin Xu, Oak Ridge National Laboratory (DOE), United States
Copyright © 2022 Robinson, Barnett, Jones, Stanish and Parker. This is an open-access article distributed under the terms of the Creative Commons Attribution License (CC BY). The use, distribution or reproduction in other forums is permitted, provided the original author(s) and the copyright owner(s) are credited and that the original publication in this journal is cited, in accordance with accepted academic practice. No use, distribution or reproduction is permitted which does not comply with these terms.
*Correspondence: Natalie Robinson, nrobinson@battelleecology.org