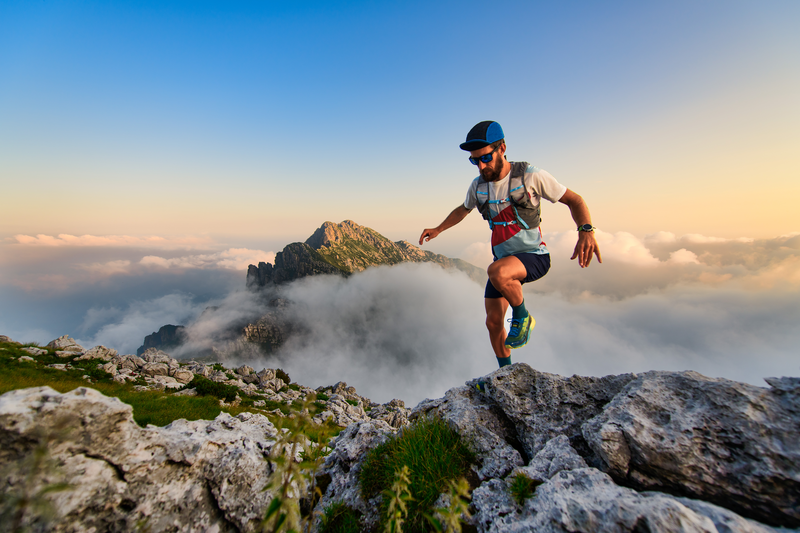
94% of researchers rate our articles as excellent or good
Learn more about the work of our research integrity team to safeguard the quality of each article we publish.
Find out more
ORIGINAL RESEARCH article
Front. Environ. Sci. , 10 January 2023
Sec. Conservation and Restoration Ecology
Volume 10 - 2022 | https://doi.org/10.3389/fenvs.2022.1097030
This article is part of the Research Topic Structure and Function of Vegetation During Ecological Restoration of Degraded Land View all 6 articles
Most plant‒soil feedback studies have been conducted on the mechanism by which soil directly influences plant growth performance and mostly in indoor pot experiments; however, it is unclear how plant‒soil feedback is influenced by plant, soil and microbial diversity in grassland ecosystems in alpine meadows with high plant diversity. In this study, plant‒soil feedback patterns were investigated by analyzing plant, soil and microbial characteristics across seven gradients in the time series from light degradation to 10-years of recovery, classified into three categories: ecosystem multifunctionality, biotic and abiotic factors, and comparing the strength and magnitude of plant‒soil feedback in alpine meadows of degradation stages and years of recovery. The results showed that the plant-soil feedback relationships in alpine meadows differed significantly in three aspects: ecosystem multifunctionality, biotic and abiotic factors in the degradation stage and recovery years, and under the degradation gradient, ecosystem multifunctionality decreased from 0.34 to −0.99 with the deepening of degradation, biotic factors increased from −0.17 to 0.09, and abiotic factors increased from −0.17 to 0.15, while in the recovery gradient, ecosystem multifunctionality showed a trend of increasing and then decreasing with increasing recovery years, while biotic and abiotic factors showed fluctuating changes. The plant-soil feedback index indicated that the strength and direction of plant-soil interactions during degradation and recovery were different, and the positive feedback effect was 0.34 and 0.38 in the early stage of degradation and recovery, respectively, which were greater than the negative feedback effect. With the deepening of degradation, the negative feedback effect became more and more obvious, and at the stage of extreme degradation, the negative feedback effect reached −0.99, which was much larger than the positive feedback effect. However, with the increase of the recovery years, the positive feedback effect gradually weakened, and finally all of them were negative feedback effects at 10-years of recovery. This study provides a scientific basis for understanding plant-soil feedback in alpine meadow ecosystems and indicates the direction for the next scientific recovery of alpine meadows.
Plant communities change the soil community structure, and this change in turn influences the growth rate of the plant communities (Bever et al., 1997). Soil organisms can strongly affect the establishment of plant diversity as well as the direction of community succession in grassland wastelands and abandoned arable land (Bever, 2003). Soil organisms can mediate these interactions by directly promoting or inhibiting plant growth or by influencing predation or nutrient availability (Wardle et al., 2004); these interactions are referred to as plant‒soil feedback (Chen et al., 2022). Plant‒soil feedback contributed to the succession of plant communities, the invasiveness of alien species and the coexistence of different species within the community (Van der Putten et al., 1993; Klironomos, 2002; Ehrenfeld et al., 2005; Lee et al., 2012). In addition, changes in intensity and direction can shift plant community dynamics from a state of coexistence between different species to one of successional competition, which plays a positive role in maintaining the stability of ecosystem structure and function (Hector and Bagchi, 2007; Gamfeldt et al., 2008). Thus, interactions between plants and soil biotic and abiotic properties can effectively explain structural differences plant communities on aboveground, as well as the extent to which ecosystems react to environmental change (Ehrenfeld et al., 2005; Bardgett and Wardle, 2010).
As a major ecosystem on the Qinghai-Tibet Plateau, alpine meadows play an important role in water conservation, biodiversity maintenance and carbon sequestration (Breidenbach et al., 2022; Sun et al., 2022). However, in recent years, due to climate warming, unreasonable human activities (e.g., overgrazing, grassland reclamation and construction) and destruction by small rodents (mainly plateau pika and plateau zokor), approximately 4.23 × 105 km2 of grassland on the Qinghai-Tibet Plateau has been degraded to varying degrees, with 16.5% of the degraded grassland severely degraded to “black soil banks”. This is a serious threat to the sustainability of alpine meadow ecosystems (Chapin et al., 2000; Dong et al., 2010; Cardinale et al., 2012; Laughlin, 2014), and artificial grassland revegetation is considered to be one of the main effective ways to restore degraded meadows (Shang et al., 2018).
Most plant‒soil feedback studies have been conducted mainly in grasslands and tropical forests, with the main findings being negative feedback effects of plant species on their conspecifics (Kulmatiski et al., 2008; Petermann et al., 2008; Mangan et al., 2010). However, there are few studies on the differences in plant‒soil feedback during alpine meadow degradation and recovery based on field experiments. Current research on plant‒soil feedback is dominated by controlled field and indoor trials (Bickford et al., 2022; Buchenau et al., 2022; Dan et al., 2022; Dudenhöffer et al., 2022; Huberty et al., 2022), which validate the effects of plant‒soil feedback mechanisms on population dynamics and community composition, and these studies have provided insight into the important role of plant‒soil feedback mechanisms in community development (Kulmatiski et al., 2006). However, most studies on plant‒soil feedback relationships have focused on the individual species level, and fewer study have been conducted at the community level, limiting our understanding of plant‒soil feedback during community-based alpine meadow degradation and recovery (De Deyn et al., 2004; Kardol et al., 2006; Kulmatiski et al., 2006). In addition, controlled indoor experiments do not well reproduce the natural environment in which alpine meadow ecosystems are found, and plant‒soil feedback still need to be studied in the natural environment and at longer time scales (Liu et al., 2014; Wang et al., 2022). Negative plant‒soil feedback plays a major role in maintaining plant species diversity, while positive plant‒soil feedback may lead to community convergence (Bever, 2003; Bever et al., 2010). However, we lack a clear understanding of the mechanism of plant‒soil feedback. Especially in the context of global environmental changes, in the wild natural environment of the widespread alpine meadow ecosystem, plant‒soil feedback interacts with other known effects to influence plant community composition and ecosystem versatility. Solving these problems will contribute to the prediction of plant‒soil feedback in the wild environment.
Therefore, this study took grassland under different degradation gradients and different recovery years in Maqin County as the research object. By analyzing the changes in plant, soil and microbial characteristics in different degradation stages and different recovery years, the characteristics of plant, soil and microbial community diversity in the process of degradation and recovery were quantified, and the occurrence rules in different degradation stages and different recovery years were determined. The feedback relationship between plants and soil was studied, and the interaction mechanism of the plant‒soil relationship was revealed, which provided a theoretical basis and scientific reference for the recovery of alpine meadow ecosystems.
The experimental area is located in Jun Ranch of Sanjiangyuan Alpine Meadow Research Observatory (Jun Ranch, Machin County, Golog Tibetan Autonomous Prefecture, Qinghai Province, 34°22′-34°20′N, 100°30′-100°29′E), at an altitude of 3,783.5 m. It is a continental semihumid climate type on the Qinghai-Tibet Plateau, with an average annual temperature of -5.6–3.8°C, with an average temperature of −12.6°C in January and 9.7°C in July. The average annual precipitation is 500–600 mm, and the annual evaporation is relatively large, generally 730–1700 mm. There is no absolute frost-free period, and the forage grows for approximately 150 days. The soil type is alpine meadow soil, and the grass type is alpine meadow. The vegetation types are Kobresia pygmaea C. B. Clarke and K. humilis Sergievskaya. and K. capillifolia C. B. Clarke as the dominant species and Poa crymophila Keng, Carex atrofusca Schkuhr subsp, and Polygonum viviparum L as companion species in alpine meadows. Due to climate change, human activities and rodent feeding activities, the alpine meadow has been degraded to alpine meadow with Aconitum pendulum busch, Morina chinensis Diels, Pedicularis kansuensis Maxim and Ligularia virgaurea Mattf, and Artemisia hedinii Ostenf. et Pauls, were the dominant severely degraded meadows.
In August 2020, an experimental plot was selected in the Military Pasture of Sanjiangyuan Alpine Meadow Research and Observation Station. According to the ‘Grading Standard of Natural Grassland Degradation, Desertification and Salinization’ (GB19377-2003) and the classification standard of Ma et al. (2002) (Table 1), the method and principle of temporal succession were replaced by spatial sequence. The meadows with basically the same climate and soil conditions in the study area were divided into slightly degraded alpine meadow (D1), moderately degraded alpine meadow (D2), highly degraded alpine meadow (D3) and severely degraded alpine meadow (D4) (She et al., 2021) (Table 2). The plots with different recovery years were compared with the severely degraded meadow, and the native vegetation was sparse in the severely degraded stage. The vegetation was low or dead, the soil surface was exposed in patches, poisonous weeds were overgrown and spread, and the grazing value basically disappeared. Before the establishment of artificial grassland, it was a typical severely degraded grassland. The dominant species of Cyperaceae were replaced by poisonous weeds, and the coverage of native vegetation was less than 10%. The vegetation is composed of A. pendulum, Potentilla chinensis, Ligularia virgaurea, P. resupinata, and Ajania tenuifolia. In 2019, 2015 and 2010, perennial artificial grasslands dominated by Elymus nutans, Festuca sinensis and P. crymophila were replanted on severely degraded grassland. The seeds of Elymus nutans, Festuca chinensis and P. crymophila used in the artificial grassland were produced by the local grass seed breeding farm with a sowing rate of 37.5 kg/hm2 and a sowing rate of 3:1:1, and the amount of fertilizer applied was 45 kg/hm2 (diammonium hydrogen phosphate compound fertilizer). The sowing distance was 20 cm, and the agronomic measures were as follows: deep turning-raking-fertilizing-sowing-covering-soil-compacting-fencing. Each sample plot was 20 hm2, and each treatment had 5 replicates. The management measures after planting were fencing and culturing, without livestock grazing disturbance factors. In August 2020, severely degraded alpine meadow (D4), artificial grassland established in 2019 (1-year-old artificial grassland, 1AG, R1), artificial grassland established in 2015 (5-year-old artificial grassland, 5AG, R5), and artificial grassland established in 2010 (10-year-old artificial grassland, 10AG, R10) were tested (Figure 1; Table 2).
Five randomly set 50 cm × 50 cm samples were set in each sample plot for the vegetation community survey. To avoid edge effects, the interval between each sample square should not be less than 10 m. The vegetation sample survey mainly included the survey of overall community characteristics, including meadow health, dominant species, number of species, total cover, average plant height (multiple replicates to take the average value), and the survey of species characteristics, including the subcoverage of each species, number of plants (multiple degrees), plant height and so on. The average height of the community in the sample square was recorded with a tape measure, and then the total cover of the community in the sample square was measured by visual inspection, followed by measuring the height of a single species in the sample square with a tape measure (5 replicates, less than 5 plants were all measured), measured the cover of a single species by visual estimation, and calculating the multiplicity of species. Afterwards, the plants in the sample plots were cut in flush ground and put into envelopes, brought back to the laboratory and dried at 65°C for constant weight, then weighed and recorded.
After taking the plant samples, the underground parts of the plants were collected in the sample cubes using the soil column method (Φ = 50 mm). Three augers were taken for each sample cube, and each auger was divided into 3 layers of 10 cm soil columns each (0–10, 10–20, 20–30 cm), and the soil samples were sieved with a mesh of 5 mesh. After rinsing the soil with adequate water, the live roots were picked out according to the flexibility of the root system and the color of the cross-section. Finally, washed live root systems were put into large envelopes, dried in an oven at 65°C for constant weight and then weighed. Soil samples from the 0–30 cm soil layers were passed through a 2 mm sieves to remove debris and used for soil property determination. Soil microorganisms were obtained by the X-type five-point method, and soil samples 0–10 cm were taken. All samples were collected in sterilized self-sealing bags, stored frozen and shipped back to the laboratory in time. Ten grams of fresh soil was stored at −80°C in the refrigerator for DNA extraction and high-throughput sequencing. Sample sequencing was performed at Majorbio Cloud platform (http://www.majorbio.com/).
In community analysis, the importance value (IV) can be used as a measure of plant species dominance. The relative coverage (RC), relative height (RH) and relative abundance (RA) of individual species were used to calculate the important values of each species:
RC (%) = The coverage of a species/coverage of all species
RH (%) = the height of the individual species/the sum of the individual heights of all species
RA (%) = Species of number of individuals/number of individuals of all species
The Patrick richness, Shannon‒Wiener diversity, Pielou evenness and Simpson dominance index were used to calculate the species diversity:
Patrick richness index (R)
Shannon‒Wiener diversity index (H)
Pielou evenness index (E)
Simpson dominance index (D)
where s is the sum of all the plant species in the sample box, i is the plant species in the sample box, and Pi is the relative importance of the I species.
The total nitrogen content was determined by the Kjeldahl method, total phosphorus was determined by the sodium hydroxide alkali fusion-mo-sb anti-colorimetric method, total potassium was determined by the sodium hydroxide alkali fusion-flame photometry method, available nitrogen was determined by the sodium hydroxide alkali decomposition-diffusion method, available phosphorus was determined by the sodium bicarbonate leaching-molybdoantimony colorimetric method, available potassium was determined by the ammonium acetate leaching-flame photometric method, and soil organic matter was determined by the potassium dichromate volumetric method-external heating method. The soil organic carbon content was determined by heating with potassium dichromate acid, the soil water content was measured by drying and weighing, the soil pH was determined by the potentiometric method, and the soil bulk density was determined by the ring knife method. Refer to “Soil Agrochemical Analysis” for specific measurement methods (Bao, 2000).
The data of this study involved two sequences, namely, plant and soil characteristics at different stages of degradation (D1, D2, D3 and D4) and at different years of recovery (D4, R1, R5 and R10). The 10 indicators involved in ecosystem multifunctionality (soil nutrients, aboveground biomass, belowground biomass), 12 indicators involved in biotic factors (aboveground biodiversity and belowground biodiversity) and 3 indicators involved in abiotic factors (soil water content, soil pH and soil capacity) were transformed into 3 composite indicators using factor analysis corresponding to different stages of degradation and different stages of recovery years. Three indicators were transformed into three composite indicators (Table 3).
Factor analysis is an approach for studying the internal dependencies of the correlation matrix between multiple variables to identify several random factors that combine key information about all variables and represent the underlying structure of the data. The factors being measured are uncorrelated with each other, and all variables can be represented as linear combinations of common factors. Taking n samples, p indicators and X = (X1, X2..., XP) as random variables, the model expression is reproduced below.
where the matrix A = (aij) is called the factor loading matrix, aij is the factor loading and μ is the special factor representing the variance of the variables that cannot be explained by the common factor and can be ignored in the actual analysis. In the operation process, factor analysis was performed on the variable data set using econometric statistical analysis software, and the combined scores of the factors were finally determined by testing Bartlett’s spherical and Kaiser-Keyer-Olkin statistics of the samples and measuring the main factors using the maximum variance method of rotation.
The feedback effect index (FI) between plants and soil, if FI > 0, is a positive feedback effect; if FI < 0, it shows a negative feedback effect. The specific calculation formula is as follows.
where X is the target treatment group index and X0 is the average of all treatment group indices (Pernilla Brinkman et al., 2010; Jing et al., 2015).
During the degradation of alpine meadows, total aboveground biomass showed a significant decrease (p < 0.05), and belowground biomass showed a decreasing trend. Aboveground biomass of the artificial meadows was significantly higher than that of the severely degraded ones, but the difference between the aboveground biomass of the artificial meadows restored for 5 and 10 years was not significant compared with that of the artificial meadows restored for 10 years. Belowground biomass of artificial grassland showed a trend of increasing and then decreasing with increasing recovery years (p < 0.05), and the difference was not significant when compared with the severely degraded artificial grassland at 1 year of recovery. The establishment of artificial grassland led to an increase in aboveground biomass, which had little effect on belowground biomass, but both aboveground and belowground biomass tended to decrease with increasing years (Figure 2). During the degradation of alpine meadows, the biomass of functional groups evolved from mainly Gramineae and Sedge to mainly Compositae and Forbs, the biomass of Gramineae was the lowest in D2 and D4, and was 0 in D4, the biomass of Sedge showed a gradually decreasing trend, the biomass of Compositae showed a gradually increasing trend up to D3, and decreased in D4, the biomass of Forbs decreased in D2 and D4, and decreased to The biomass of weed species decreased in D2 and D4, and decreased to the lowest in D4. The plant community structure was changed by the establishment of artificial grassland. The dominant species in the D4 was mainly Compositae, while the dominant species in artificial grassland was mainly Gramineae. The dominant species in the 1-year-old artificial grassland was Elymus nutans, the dominant species in the 5-year-old artificial grassland was Elymus nutans and K. humilis, and the dominant species in the 10-year-old artificial grassland decreased, and the species dominated by Compositae started to gain the subdominant species status. The dominance of grass dominated by Elymus nutans gradually decreased with the extension of the establishment period (Figure 3).
FIGURE 2. Changes in plant biomass at different stages of degradation and at different recovery years.
Plant species diversity indices of the plant communities all showed a decreasing trend. Species richness and the Shannon-Weiner diversity index reached the highest at D3, and species richness was significantly different from D2 and D4 (p < 0.05) and not significantly different from D1, unlike the Shannon‒Wiener index D4, which was significantly different from D1, D2, and D3, while there was no significant difference between the first three stages. Neither the uniformity index nor the Simpson index from D1 to D4 was significant (p > 0.05), but both showed the lowest values at D4. Species richness was significantly higher (p < 0.05) in the artificial grassland restored for 1 year and 10 years than in the severely degraded grassland and restored for 5 years. The Shannon-Weiner diversity index was higher in the artificial grassland restored for 1 year than in the severely degraded, restored for 5 years and restored for 10 years (p < 0.05). Differences in evenness index and Simpson’s diversity index between artificial grassland and severely degraded grassland were not significant (Figure 4).
FIGURE 4. Changes in the species diversity of plant communities at different degradation stages and different recovery durations. Note: Different lowercase letters represent significant differences in species richness, Shannon‒Wiener index, species evenness index and Simpson diversity index between different degradation stages and recovery years (p < 0.05).
From D1 to D4, the contents of TN and TP in the 0–10 cm surface soil were higher than those in 10–20 cm and 20–30 cm. From D1 to D4, TN and TP showed a gradually decreasing trend but slightly increased in the D2 stage. There was no significant change in TK content from D1 to D4, and TK showed an increasing tendency in D3 compared with severe degradation. Total nutrients (total N, P and K) in artificial grassland increased with the increase in the recovery years, but there was no significant difference between soil layers (Figure 5).
FIGURE 5. Changes in total soil nutrients under different soil layers at different degradation stages and recovery years.
From D1 to D4, the AN, AP, and AK contents in the 0–10 cm surface soil were higher than those in the 10–20 cm and 20–30 cm soil layers, especially AK in the 0–10 cm soil layer. From D1 to D4, AN, AP, and AK showed a gradually decreasing trend but slightly increased in the D2 stage. From D1 to D4, there was no significant change in AK content, and AK showed an increasing tendency in both D2 and D3. Compared with severe degradation, the available nutrients (available N, P and K) of artificial grassland decreased with increasing recovery years, the available N, P and K of artificial grassland restored for 10 years differed significantly between different soil layers, and the content of available nutrients decreased gradually with the deepening of soil layers (p < 0.05) (Figure 6).
FIGURE 6. Changes in soil available nutrients in different soil layers at different degradation stages and recovery years.
From D1 to D4, the content of SOC in the top layer of 0–10 cm soil was higher than those in 10–20 cm and 20–30 cm, especially in the 0–10 cm layer. Compared with severe degradation, the soil organic carbon of artificial grassland increased (p < 0.05), but with the extension of the recovery period, a “V"-shaped trend was observed in recovery year 1, recovery year 5 and recovery year 10, the lowest in recovery year 5 (Figure 7).
FIGURE 7. Changes in soil organic carbon under different soil layers at different degradation stages and recovery years.
The soil bulk density increased during the process from D1 to D4, while the soil water content gradually decreased. Soil bulk density was highest in the process of recovery in severe degradation and lowest in artificial grassland in 1 year of recovery and gradually increased with the recovery years. The severe degradation of the soil water content increased compared with that of the artificial grassland, but with the increase in recovery years, the soil water content basically did not change (Figure 8).
FIGURE 8. Changes in soil bulk density and soil water content under different soil layers at different degradation stages and recovery years.
The pH increased from D1 to D4. During the recovery process, the soil pH was highest in the same soil layer with severe degradation, lowest in the artificial grass by 1 year of recovery, and gradually increased as the recovery years progressed (Figure 9).
FIGURE 9. Changes in soil pH under different soil layers at different degradation stages and recovery years.
The alpha diversity of bacterial and fungal communities showed opposite trends between degradation and recovery processes (Table 4). The Shannon and Chao indices of bacteria and fungi increased significantly with increasing degradation, the Chao indices of fungi were in the order of D4 (697.1) > D3 (660.5) > D2 (643.3) > D1 (443.7). In contrast, the fungal Shannon and Chao indices of the recovery process were significantly lower (p < 0.05) from R5 to R10. The bacterial Chao index showed a similar trend, while the bacterial Shannon index did not differ significantly between the different recovery years. The Simpson diversity index of bacteria was significantly different (p < 0.05) between the highly and severely degraded stages of degradation, while there was no significant difference between the recovery years, and the Simpson diversity index of fungi was not significantly different between degradation stages and recovery years. The ACE diversity index of bacterial and fungal communities showed no significant patterns during either degradation or recovery.
TABLE 4. Community diversity of bacteria and fungi at different stages of degradation stages and recovery years.
Indicators incorporated in ecosystem multifunctionality are all quantitative indicators of soil nutrient cycling, nutrient pool accumulation and good above- and belowground productivity, where nitrogen and phosphorus are key factors for ecosystem production and biomass are proxies for good grassland productivity. In light and moderate degradation stages, alpine meadow degradation has a positive plant‒soil feedback effect on ecosystem multifunctionality and reaches its maximum at moderate degradation; in the high and severe degradation stages, alpine meadow degradation has a negative plant‒soil feedback effect on ecosystem multifunctionality and reaches its maximum by severe degradation. In different stages of alpine meadow degradation, the plant‒soil feedback on ecosystem multifunctionality changed to negative feedback after reaching the maximum in moderate degradation. Negative plant‒soil feedback of alpine meadow degradation on biological factors was observed in mild degradation, and positive plant‒soil feedback was observed from moderate degradation to severe degradation, which showed a tendency to increase with the degradation degree. In the early stage of the degradation process, alpine meadow degradation has a negative plant‒soil feedback effect on abiotic factors; the heavy and severe degradation stages, alpine meadow degradation has a positive plant‒soil feedback effect on abiotic factors, and it shows a trend of increasing gradually. It can be seen that at the stage of severe degradation, planted artificial meadow has a negative plant‒soil feedback effect on ecosystem multifunctionality, and at 1 and 5 years of recovery, planted artificial meadow has a positive plant‒soil feedback effect on ecosystem multifunctionality, and it reaches the maximum at 1 year of recovery. During the recovery process, plant‒soil feedback on ecosystem multifunctionality changed to negative feedback after reaching a maximum at 1 year of recovery. For severe degradation, the constructed artificial grassland had a positive plant‒soil feedback effect on biotic factors, negative feedback at 1 year of recovery, positive feedback at 5 years of recovery, and negative feedback at 10 years of recovery, indicating that aboveground community diversity and belowground microbial community diversity were richer at 5 years of artificial grassland than at 1 and 10 years of recovery, and the change pattern of the feedback effect of abiotic factors was consistent (Figure 10).
The species diversity index showed an overall decreasing trend from D1 to D4, but a maximum occurred at D3, which is essentially consistent with the findings of this study (Chen et al., 2016; Zhang et al., 2019). The reason for the maximum species diversity in D3 is mainly because the D1 and D2 meadow ecosystems have strong stability, while with the accelerated process of degradation, edible forage grasses decrease, and species such as Stellera chamaejasme, Aconitum pendulum and L. virgaurea begin to occupy the dominant species ecological niche in the community. The increase in the number of species within the community will cause changes in plant community structure and function and will influence the high level of species diversity within the community (Zhou et al., 2012). Disturbances from human activities during the process from D1 to D4 can also have an impact, such as grazing and the survival of rodents in the meadow, cattle, sheep and rodents with foraging preferences, trampling of the meadow during foraging and acts such as seed dispersal as a vector during foraging, which can result in a higher number of species in D3 than in D2 (Wang et al., 2014; Zhang et al., 2021). The species richness and Shannon‒Wiener diversity index during meadow degradation showed a unimodal change, with the maximum value being present at D2 (Hao et al., 2020). This study showed no significant difference in species evenness and Simpson’s diversity index from D1 to D4, suggesting that anthropogenic disturbance inhibited the dominant species in the community, which provided opportunities for the invasion and expansion of toxic weeds and enhanced the competitiveness of these species to dominate the community, resulting in an increase in the species diversity index, thus indicating that moderate disturbance would improve the species diversity within the community (Zhou et al., 2005; Zhou et al., 2012). The community composition is gradually homogenized, and the species richness and Shannon Weiner diversity index decreased significantly (Zhou, 2001; Jianli et al., 2004; Yuan et al., 2004).
The establishment of artificial grasslands at the stage of severe degradation has a positive effect on long-term recovery of artificial grassland ecosystems (Shang et al., 2018). The establishment of artificial grassland not only improves the productivity of the ecosystem but also corresponds to the introduction of new vegetation and the acquisition of organic matter contributed to the soil system by plant residues and dead leaves, thus improving the structure and function of the soil (Zi et al., 2017; Xing et al., 2020). In this study, plant aboveground biomass showed a decreasing trend with the extension of recovery years, and in the early stage of recovery, competition between species was low, and plants of the grass family were larger and soon occupied a higher ecological position and dominated the whole community, so aboveground biomass was higher (Li et al., 2021). After 5 years of recovery, sedge family plants with low resource requirements gradually increased, and a small number of sedge family plants appeared during the gradual succession of artificial grassland, indicating that the artificial grassland entered the natural recovery process with low weed-like biomass (Zhang et al., 2003). After 10 years of recovery, soil fertility declined, and when nutrients in the soil were insufficient, plants tended to transfer resources to the underground root system to improve the uptake and utilization of limited soil resources, the dominant species status of Gramineae declined, and species dominated by Asteraceae began to be in subdominant species status (Zhang et al., 2012). With the increase in recovery years, all four species diversity indices showed a “V” shape change, which was due to the strong competition of planted species of artificial grassland at the early stage of recovery and their rapid dominance, making the species diversity index decrease; however, with the extension of recovery years, the native species gradually invaded and gained dominance, and the species diversity increased significantly (Wu et al., 2019).
Soil available potassium, total nitrogen and total potassium showed a decreasing trend as the degradation level increased. There was no significant change in the distribution of soil total potassium content among soil layers in the meadow samples with different degradation levels (Gao et al., 2006). In the same soil layer of different alpine meadow degradation stages, the soil total potassium content varied significantly, and in different soil layers of the same alpine meadow degradation stages, the total potassium content in the same soil layer did not vary significantly (Chen and Zhang, 2013). Soil available nitrogen, available phosphorus and total phosphorus showed an overall decreasing trend but differed in that a small increase was observed at a specific stage. Soil available nitrogen showed a small increase at the stage of severe degradation, and soil available phosphorus showed a small increase at the stage of mild degradation, while soil total phosphorus showed a small increase at the stage of severe degradation. With the intensification of soil degradation, the total phosphorus content of the soil showed a decreasing trend and then an increasing trend, while the available phosphorus content showed a decreasing trend (Zhao et al., 2009). In alpine meadows of the Tibetan Plateau, mineralization of organic nitrogen is the main source of available nitrogen in the soil (Gao, 2007). Plant uptake and natural factors reduce the content of available nitrogen, and effective nitrogen has a small rebound in the process of severe degradation, which may be because the level of severe degradation plant activity is not high, so the amount of uptake is not high, resulting in a small rebound phenomenon. The soil water content showed a decreasing trend with the deepening of degradation (Zhou et al., 2005; Li et al., 2020). The response of alpine meadows to soil at different stages of degradation focuses on soil bulk, and the specific response is expressed as an increase in bulk weight (Zi et al., 2015). Both of these processes reduce the water holding capacity of the soil, leading to a decrease in soil water content (Zhou et al., 2005). At the same time, enhanced evapotranspiration and infiltration also led to the loss of soil water. The loss of soil water in the surface layer of the meadow may be due to evaporation and infiltration from the ground surface, and in the later stages of degradation, the decrease in soil water content decreased (Fan et al., 2019), probably due to low vegetation cover, low plant activity level and reduced transpiration. With the deepening of degradation, the soil capacitance and soil pH showed an increasing trend. Soil pH was higher in the severely degraded stage than in the other degraded stage and showed a decreasing trend from severely degraded to moderately degraded, which was consistent with the integrated results of this study (Gao et al., 2006). Soil organic carbon showed an overall decreasing trend, but a small increase was observed at the stage of severe degradation, and an overall decreasing trend was observed with increasing soil depth, which was consistent with the integration results of this study (Han et al., 2011).
Soil is the most important carrier of nutrient cycling, water balance and microbial activity in terrestrial ecosystems and plays a key role in plant growth, and its physicochemical properties and nutrient status are the most important indicators of ecosystem stability (Liu and Tan, 1994; Yang et al., 2007). Soil nutrients, water content and pH are key indicators for restoring and maintaining the function of damaged ecosystems and play an important role in plant community succession (Li and Ma, 2002; Cheng et al., 2021). In this study, we found that the soil nutrient content in the 0–10 cm soil layer was higher than that in the 10–20 cm and 20–30 cm soil layers, which is due to the short growing season of alpine plants, which are mostly shallow-rooted plants, resulting in shallow root distribution and decomposition of dead roots into organic matter and other nutrients in the soil, which accumulate in the soil surface layer (Yue et al., 2015). Total nitrogen, phosphorus and potassium in artificial grassland showed an increasing trend with the increase in recovery years, but available N, P and K showed the opposite trend, and some studies also showed that the content of total nitrogen, available nitrogen and available potassium in artificial grassland soil increased with the increase in recovery years (Sun et al., 2019), which was not consistent with the results of this study, which was due to the heterogeneity of artificial grassland in different recovery years. Compared with severe degradation, the soil organic matter and organic carbon of artificial grassland increased (p < 0.05), but with the extension of the recovery period, the trend of the “V" shape was observed in 1-year, 5-year,10-year recovery, the lowest in 5-year recovery, and the overall trend was “high-low-high”. The overall trend of “high-low-high” is consistent with the findings of others (Yang et al., 2015). Compared with severe degradation, the soil pH, water content and bulk density of artificially established grassland will decrease, while high vegetation cover by the time the vegetation community steps into recovery succession will not only reduce evaporation of soil water content but also provide a rich source of organic matter for the soil, further indicating that the improvement of the soil environment is beneficial to the settlement of exotic species, seed germination, root expansion and their nutrient uptake (Wang et al., 2009).
Overall, alpha diversity of bacteria and fungi increased with increasing degradation but decreased with increasing recovery years during recovery. The alpha diversity of bacteria was much higher in artificial grasslands restored for 1–4 years than in those restored by 10–18 years, which are similar to other studies (Gao et al., 2021). Bacterial diversity was higher in the early successional stages of vegetation (4 years) than in the later stages of vegetation succession (8 and 12 years) (Hu et al., 2019). For the degradation process, patch formation significantly increased fungal alpha diversity by approximately 40% (Che et al., 2019). Overall, it is plausible that the significant response of soil bacterial and fungal community alpha diversity to degradation and recovery processes may be influenced in multiple ways. First, soil pH changes frequently during degradation and recovery (Lennon and Houlton, 2017), and an important environmental factor affecting microbial communities is soil pH (Fierer, 2017). Many studies have found that soil pH is negatively correlated with microbial α-diversity during degradation and recovery (Tripathi et al., 2018; Guo et al., 2019). Second, in severely degraded meadows, reduced vegetation cover can accelerate topsoil erosion, increase roughness, and increase light, thus improving the competitiveness of aerobic and photosynthetic autotrophic microorganisms (Lin et al., 2015; Zhang et al., 2017). Bacterial and fungal community structure varies significantly across degradation and recovery processes, with significant differences in microbial communities during the degradation or recovery of alpine grasslands on the Tibetan Plateau (Li et al., 2016; Guo et al., 2019; Zhou et al., 2019). These differences are mainly due to the different vegetation characteristics and soil properties of different degraded and artificial grasslands. Degraded meadows limit plant growth, leading to physical damage to soil structure and nutrient status, which further accelerates soil degradation (Zhou et al., 2019), and alpine meadow degradation will significantly alter bacterial community structure due to reduced availability of microbial substrates as a result of reduced apoplactic inputs (Zhang et al., 2014).
Different soil conditions can have soil-specific effects on plant species (van de Voorde et al., 2011), and changes in soil properties directly affect the direction of plant community succession, providing a theoretical basis for plant‒soil feedback (Bever et al., 1997; Bardgett and Van Der Putten, 2014). When studied at the individual plant level, a positive feedback effect value indicates that the plant grows better in the original or the same plant-influenced soil; a negative feedback effect value indicates that the plant has a negative feedback effect in the soil (Baxendale et al., 2014). Similarly, when the plant‒soil feedback is positive at a certain stage of plant succession. It means that the plant is growing better at that stage and the soil is in a depleted situation, and vice versa. A meta-analysis at the individual species level showed positive plant‒soil feedback effects accounting for approximately 28% of the statistics (Kulmatiski et al., 2008). Plant‒soil positive feedback can increase the asymmetry of plant growth, leading to the dominance of some plants in a given habitat and the formation of communities dominated by a single plant species, influenced by (1) an increase in available soil nutrients due to plant growth, for example, through plant nitrogen fixation (Chapman et al., 2006), and (2) the accumulation of plant symbionts among the root system (Klironomos, 2002). Most studies have shown that plants in late successional stages are more dependent on interroot symbionts, exhibit greater environmental tolerance, and tend to have a positive plant‒soil feedback (Kardol et al., 2006), becomes the dominant species in the community, and gradually become the dominant species in the community. The results of this study showed that in ecosystem multifunctionality, positive feedback was observed in the predegradation period, positive feedback was observed in the moderate to severe degradation for biotic factors, and positive feedback was observed in the moderate degradation for abiotic factors. In the prerecovery stage, plant‒soil feedback in ecosystem multifunctionality showed positive feedback, and in the recovery year of 5 years, all three aspects of ecosystem multifunctionality, biotic factors and abiotic factors were positive feedback effects. In the early stage of succession, the preferentially planted plants promote their own growth by decomposing the dead material produced by aboveground plants and the mineralization of nutrients by soil microorganisms while reducing the competitiveness of other plant species in the community, such as the establishment of Molinia caerulea in the abandoned land where the distribution density of Calluna vulgaris is high. The vegetation type gradually changed to grassland (Van der Putten et al., 1993; Berendse, 1994). The positive plant‒soil feedback effect is a key factor in early plant changes (Reynolds et al., 2003), and contemporaneous changes in soil properties can have potential long-term effects on plant distribution and productivity (Grman and Suding, 2010; Kulmatiski and Beard, 2011). Plant-soil feedback studies are important for the study of plant community succession, ecosystem multifunctionality and productivity formation and maintenance mechanisms, as well as for theoretical guidance in understanding ecosystem responses to global ecological events, such as climate change and biological invasions.
Negative plant‒soil feedback occurs more frequently at the individual species level, accounting for approximately 70% of the included statistics (Kulmatiski et al., 2008). It promotes a balance between the survival states of two plants in a community and, to some extent, species coexistence, with the main influences being (1) nutrient fixation or depletion in the soil, resulting in inadequate supply (Berendse, 1994; de Kroon et al., 2012), (2) accumulation of predators or pathogens under the roots (Van der Putten, 2003) or phytochemical effects of chemicals released by plants (Bais et al., 2003), and (3) some degree of soil carbon aggregation (Ehrenfeld et al., 2005). The results of this study showed that in ecosystem multifunctionality, the late stage of degradation had a negative feedback effect, and biotic factors had a negative feedback effect in mild degradation. The abiotic factors had a negative feedback effect in moderate to severe degradation, and the feedback intensity changed from large to small with the increase in recovery years and the trend from positive to negative feedback. At the recovery year of 10 years in artificial grasslands, the ecosystem multifunctionality, biotic factors and at 10 years of artificial grassland recovery, negative feedback was observed in three aspects: Ecosystem multifunctionality, biotic and abiotic factors. Studies have shown that negative plant‒soil feedback effects are generally present in the middle and late stages of semiarid grassland evolution but have less impact on the dominant species (Reinhart, 2012). Some studies have also found that in early successional stages, when an individual plant exhibits a negative feedback effect on itself but a positive feedback effect on other plants growing together within the community, the dominance of this plant is replaced by the coexist plant if other plants exhibit a negative feedback effect on this plant (van de Voorde et al., 2011). During plant community succession, early successional plants exhibit strong negative plant-soil feedback effects on themselves, and if the co-existing species in the community exhibit negative feedback effects on them, this plant species will be replaced by the co-existing species. Thus, negative plant‒soil feedback effects can affect plant community structure by suppressing dominant species, reducing the number of most species and decreasing the competitiveness of all species within the community.
In this study, plant-soil feedback relationships including ecosystem multifunctionality, biotic and abiotic factors were constructed by analyzing plant, soil and microbial characteristics at seven gradients in the time series of vegetation recovery from mild degradation to 10 years, and the main conclusions were as follows.
1) During the degradation succession of alpine meadows, with the deepening of degradation, above-ground biomass, species diversity index, soil total nitrogen, total phosphorus, available nitrogen, available phosphorus, organic carbon and water content showed a decreasing trend, while soil potassium content showed no significant change trend; soil pH and bulk density showed a significant increase. However, the aboveground biomass showed a decreasing trend with the increase of the recovery years, and the species diversity index showed a “V”-shaped change with the increase of the recovery years, it reached the lowest after 5 years of establishment. Soil total nutrients, organic carbon, pH and bulk density increased, available nutrients decreased, and soil water content was basically unchanged. The alpha diversity of bacterial and fungal communities showed opposite trends between degradation and recovery processes.
2) The positive feedback effect was more than the negative feedback effect in the early stage of degradation, and the feedback effect was stronger with the increase of degradation degree. However, with the increase of the recovery years, the feedback direction changed to negative feedback, and by 10-years of recovery, the feedback was negative. In the process of degradation, ecosystem multifunctionality changed from positive feedback to negative feedback. Biotic factors changed from negative to positive feedback, and abiotic factors had the opposite feedback effect with ecosystem multifunctionality. In the recovery process, there is a trend of positive feedback to negative feedback, and the ecosystem multifunctionality, biotic and abiotic factors all have negative feedback at 10 years of recovery.
The original contributions presented in the study are included in the article/Supplementary Material, further inquiries can be directed to the corresponding author.
ZH conceived the study, supervised the writing, and revised the manuscript. SY and MT led the writing. LH, ZZ, ML, QR, SH, CT, WJ, and HX contributed sections to the manuscript. ZH provided funding support. All authors read and approved the final submission.
This work is supported by the Chinese Academy of Sciences pilot project subproject (XDA26020201), National Natural Science Foundation of China Joint Fund Project (U21A20186), Natural Science Foundation of Qinghai Province Innovation Team Project (2021-ZJ-902).
The authors declare that the research was conducted in the absence of any commercial or financial relationships that could be construed as a potential conflict of interest.
All claims expressed in this article are solely those of the authors and do not necessarily represent those of their affiliated organizations, or those of the publisher, the editors and the reviewers. Any product that may be evaluated in this article, or claim that may be made by its manufacturer, is not guaranteed or endorsed by the publisher.
Bais, H. P., Vepachedu, R., Gilroy, S., Callaway, R. M., and Vivanco, J. M. (2003). Allelopathy and exotic plant invasion: from molecules and genes to species interactions. Science 301, 1377–1380. doi:10.1126/science.1083245
Bardgett, R. D., and Van Der Putten, W. H. (2014). Belowground biodiversity and ecosystem functioning. Nature 515, 505–511. doi:10.1038/nature13855
Bardgett, R. D., and Wardle, D. A. (2010). Aboveground-belowground linkages: biotic interactions, ecosystem processes, and global change. Oxford: Oxford University Press.
Baxendale, C., Orwin, K. H., Poly, F., Pommier, T., and Bardgett, R. D. (2014). Are plant–soil feedback responses explained by plant traits? New Phytol. 204, 408–423. doi:10.1111/nph.12915
Berendse, F. (1994). Litter decomposability--a neglected component of plant fitness. J. Ecol. 82, 187–190. doi:10.2307/2261398
Bever, J. D., Westover, K. M., and Antonovics, J. (1997). Incorporating the soil community into plant population dynamics: the utility of the feedback approach. J. Ecol. 85, 561–573. doi:10.2307/2960528
Bever, J. D., Dickie, I. A., Facelli, E., Facelli, J. M., Klironomos, J., Moora, M., et al. (2010). Rooting theories of plant community ecology in microbial interactions. Trends Ecol. Evol. 25, 468–478. doi:10.1016/j.tree.2010.05.004
Bever, J. D. (2003). Soil community feedback and the coexistence of competitors: conceptual frameworks and empirical tests. New Phytol. 157, 465–473. doi:10.1046/j.1469-8137.2003.00714.x
Bickford, W. A., Goldberg, D. E., Zak, D. R., Snow, D. S., and Kowalski, K. P. (2022). Plant effects on and response to soil microbes in native and non-native Phragmites australis. Ecol. Appl. 32, e2565. doi:10.1002/eap.2565
Breidenbach, A., Schleuss, P.-M., Liu, S., Schneider, D., Dippold, M. A., De La Haye, T., et al. (2022). Microbial functional changes mark irreversible course of Tibetan grassland degradation. Nat. Commun. 13, 2681–2710. doi:10.1038/s41467-022-30047-7
Buchenau, N., Van Kleunen, M., and Wilschut, R. A. (2022). Direct and legacy-mediated drought effects on plant performance are species-specific and depend on soil community composition. Oikos 2022, e08959. doi:10.1111/oik.08959
Cardinale, B. J., Duffy, J. E., Gonzalez, A., Hooper, D. U., Perrings, C., Venail, P., et al. (2012). Biodiversity loss and its impact on humanity. Nature 486, 59–67. doi:10.1038/nature11148
Chapin, F. S., Zavaleta, E. S., Eviner, V. T., Naylor, R. L., Vitousek, P. M., Reynolds, H. L., et al. (2000). Consequences of changing biodiversity. Nature 405, 234–242. doi:10.1038/35012241
Chapman, S. K., Langley, J. A., Hart, S. C., and Koch, G. W. (2006). Plants actively control nitrogen cycling: uncorking the microbial bottleneck. New Phytol. 169, 27–34. doi:10.1111/j.1469-8137.2005.01571.x
Che, R., Wang, Y., Li, K., Xu, Z., Hu, J., Wang, F., et al. (2019). Degraded patch formation significantly changed microbial community composition in alpine meadow soils. Soil Tillage Res. 195, 104426. doi:10.1016/j.still.2019.104426
Chen, S., and Zhang, D. (2013). Potassium variation in alpine meadow soil at different degeneration stages. Grassl. Turf 33, 74–77. doi:10.13817/j.cnki.cyycp.2013.03.018
Chen, L., Shi, J., Wang, Y., Ma, Y., Dong, Q., and Hou, X. (2016). Study on different degraded degrees grassland community structure characteristics of the alpine area. Acta Agrectir Sin. 24, 210–213. doi:10.11733/j.issn.1007-0435.2016.01.029
Chen, J., Zhang, Y., Kuzyakov, Y., Wang, D., and Olesen, J. E. (2022). Challenges in upscaling laboratory studies to ecosystems in soil microbiology research. Glob. Change Biol 00, 1–6. doi:10.1111/gcb.16537
Cheng, Z., Cui, Z., Shi, J., Liu, Y., La Pierre, K. J., and Wu, G.-L. (2021). Plant functional types drive differential responses of grassland ecosystem functions along a precipitation gradient. Ecol. Indic. 133, 108433. doi:10.1016/j.ecolind.2021.108433
Dan, X., Meng, L., He, M., He, X., Zhao, C., Chen, S., et al. (2022). Regulation of nitrogen acquisition in vegetables by different impacts on autotrophic and heterotrophic nitrification. Plant Soil 474, 581–594. doi:10.1007/s11104-022-05362-z
De Deyn, G., Raaijmakers, C., and Van Der Putten, W. (2004). Plant community development is affected by nutrients and soil biota. J. Ecol. 92, 824–834. doi:10.1111/j.0022-0477.2004.00924.x
De Kroon, H., Hendriks, M., Van Ruijven, J., Ravenek, J., Padilla, F. M., Jongejans, E., et al. (2012). Root responses to nutrients and soil biota: drivers of species coexistence and ecosystem productivity. J. Ecol. 100, 6–15. doi:10.1111/j.1365-2745.2011.01906.x
Dong, S., Li, J., Li, X., Wen, L., Zhu, L., Li, Y., et al. (2010). Application of design theory for restoring the “black beach” degraded rangeland at the headwater areas of the Qinghai-Tibetan Plateau. Afr. J. Agric. Res. 5, 3542–3552. doi:10.5897/AJAR10.005
Dudenhöffer, J.-H., Luecke, N. C., and Crawford, K. M. (2022). Changes in precipitation patterns can destabilize plant species coexistence via changes in plant–soil feedback. Nat. Ecol. Evol. 6, 546–554. doi:10.1038/s41559-022-01700-7
Ehrenfeld, J. G., Ravit, B., and Elgersma, K. (2005). Feedback in the plant-soil system. Annu. Rev. Environ. Resour. 30, 75–115. doi:10.1146/annurev.energy.30.050504.144212
Fan, Y., Hou, X., Zhao, D., Shi, H., Zhou, H., and Li, X. (2019). Preliminary study on soil water-holding capacity along degradation gradients of alpine meadow. J. Southwest Minzu Univ. Natural Sci. Ed. 45, 10–15. doi:10.11920/xnmdzk.2019.01.002
Fierer, N. (2017). Embracing the unknown: disentangling the complexities of the soil microbiome. Nat. Rev. Microbiol. 15, 579–590. doi:10.1038/nrmicro.2017.87
Gamfeldt, L., Hillebrand, H., and Jonsson, P. R. (2008). Multiple functions increase the importance of biodiversity for overall ecosystem functioning. Ecology 89, 1223–1231. doi:10.1890/06-2091.1
Gao, X., Tian, Z., Hao, X., and Jiang, G. (2006). The changes of alpine grassland soil nutrition at different deteriorate degree on high mountain meadow of three river source. J. Qinghai Univ. 24, 37–40. doi:10.13901/j.cnki.qhwxxbzk.2006.05.012
Gao, X., Dong, S., Xu, Y., Li, Y., Li, S., Wu, S., et al. (2021). Revegetation significantly increased the bacterial-fungal interactions in different successional stages of alpine grasslands on the Qinghai-Tibetan Plateau. Catena 205, 105385. doi:10.1016/j.catena.2021.105385
Gao, Y. (2007). Study on carbon and nitrogen distribution pattern and cycling process in an alpine meadow ecosystem under different grazing intensity. Chengdu: Chengdu Institute of Biology, Chinese Academy of Sciences.
Grman, E., and Suding, K. N. (2010). Within-year soil legacies contribute to strong priority effects of exotics on native California grassland communities. Restor. Ecol. 18, 664–670. doi:10.1111/j.1526-100x.2008.00497.x
Guo, Y., Hou, L., Zhang, Z., Zhang, J., Cheng, J., Wei, G., et al. (2019). Soil microbial diversity during 30 years of grassland restoration on the Loess Plateau, China: Tight linkages with plant diversity. Land Degrad. Dev. 30, 1172–1182. doi:10.1002/ldr.3300
Han, D., Cao, G., Guo, X., Zhang, F., Li, Y., Lin, L., et al. (2011). The potential of carbon sink in alpine meadow ecosystem on the Qinghai-Tibetan Plateau. Shengtai Xuebao/Acta Ecol. Sin. 31, 7408–7417.
Hao, A., Xue, X., Peng, F., You, Q., Liao, J., Duan, H., et al. (2020). Different vegetation and soil degradation characteristics of a typical grassland in the Qinghai-Tibetan Plateau. Acta Ecol. Sin. 40, 964–975. doi:10.5846/stxb201809162019
Hector, A., and Bagchi, R. (2007). Biodiversity and ecosystem multifunctionality. Nature 448, 188–190. doi:10.1038/nature05947
Hu, L., Zi, H., Wu, P., Wang, Y., Lerdau, M., Wu, X., et al. (2019). Soil bacterial communities in grasslands revegetated using Elymus nutans are largely influenced by soil pH and total phosphorus across restoration time. Land Degrad. Dev. 30, 2243–2256. doi:10.1002/ldr.3414
Huberty, M., Steinauer, K., Heinen, R., Jongen, R., Hannula, S. E., Choi, Y. H., et al. (2022). Temporal changes in plant–soil feedback effects on microbial networks, leaf metabolomics and plant–insect interactions. J. Ecol. 110, 1328–1343. doi:10.1111/1365-2745.13872
Jianli, Y., Xiaolei, J., Wenbing, H., and Gang, W. (2004). Effects of grazing intensity and grazing season on plant species diversity in alpine meadow. Acta Prataculturae Sin. 13, 16–21.
Jing, J., Bezemer, T. M., and Van Der Putten, W. H. (2015). Interspecific competition of early successional plant species in ex-arable fields as influenced by plant–soil feedback. Basic Appl. Ecol. 16, 112–119. doi:10.1016/j.baae.2015.01.001
Kardol, P., Martijn Bezemer, T., and Van Der Putten, W. H. (2006). Temporal variation in plant–soil feedback controls succession. Ecol. Lett. 9, 1080–1088. doi:10.1111/j.1461-0248.2006.00953.x
Klironomos, J. N. (2002). Feedback with soil biota contributes to plant rarity and invasiveness in communities. Nature 417, 67–70. doi:10.1038/417067a
Kulmatiski, A., and Beard, K. H. (2011). Long-term plant growth legacies overwhelm short-term plant growth effects on soil microbial community structure. Soil Biol. Biochem. 43, 823–830. doi:10.1016/j.soilbio.2010.12.018
Kulmatiski, A., Beard, K. H., and Stark, J. M. (2006). Soil history as a primary control on plant invasion in abandoned agricultural fields. J. Appl. Ecol. 43, 868–876. doi:10.1111/j.1365-2664.2006.01192.x
Kulmatiski, A., Beard, K. H., Stevens, J. R., and Cobbold, S. M. (2008). Plant–soil feedbacks: a meta-analytical review. Ecol. Lett. 11, 980–992. doi:10.1111/j.1461-0248.2008.01209.x
Laughlin, D. C. (2014). Applying trait-based models to achieve functional targets for theory-driven ecological restoration. Ecol. Lett. 17, 771–784. doi:10.1111/ele.12288
Lee, M. R., Flory, S. L., and Phillips, R. P. (2012). Positive feedbacks to growth of an invasive grass through alteration of nitrogen cycling. Oecologia 170, 457–465. doi:10.1007/s00442-012-2309-9
Lennon, E. F., and Houlton, B. Z. (2017). Coupled molecular and isotopic evidence for denitrifier controls over terrestrial nitrogen availability. ISME J. 11, 727–740. doi:10.1038/ismej.2016.147
Li, Q., and Ma, K. (2002). Advances in plant succession ecophysiology. Chin. J. Plant Ecol. 26, 9. doi:10.3321/j.issn:1005-264X.2002.z1.002
Li, Y., Wang, S., Jiang, L., Zhang, L., Cui, S., Meng, F., et al. (2016). Changes of soil microbial community under different degraded gradients of alpine meadow. Agric. Ecosyst. Environ. 222, 213–222. doi:10.1016/j.agee.2016.02.020
Li, J.-H., Yang, G.-J., and Wang, S.-P. (2020). Vegetation and soil characteristics of degraded alpine meadows on the qinghai-tibet pla-teau, China: A review. Ying Yong Sheng tai xue bao= J. Appl. Ecol. 31, 2109–2118. doi:10.13287/j.1001-9332.202006.002
Li, J., Pan, P., Wang, C.-T., Hu, L., Chen, K.-Y., and Yang, W.-G. (2021). Root dynamics of artificial grassland for swards of differing ages in the ‘Three-River Source’ region. Acta Prataculturae Sin. 30, 28. doi:10.11686/cyxb2020161
Lin, L., Li, Y., Xu, X., Zhang, F., Du, Y., Liu, S., et al. (2015). Predicting parameters of degradation succession processes of Tibetan Kobresia grasslands. Solid earth. 6, 1237–1246. doi:10.5194/se-6-1237-2015
Liu, H., Xu, B., Fan, X., Zhang, H., and He, Y. (2014). Review on the mechanism of plant‒soil feedbacks. J. Qingdao Agric. Univ. Nat. Sci. 31, 142–147. doi:10.3969/J.ISSN.1674-148X.2014.02.013
Ma, Y., Lang, B., Li, Q., Shi, J., and Dong, Q. (2002). Study on rehabilitating and rebuilding technologies for degenerated alpine meadow in the Changjiang and Yellow river source region. Cao ye ke xue= Pratacultural Science= Caoye Kexue 19, 1–5. doi:10.3969/j.issn.1001-0629.2002.09.001
Mangan, S. A., Schnitzer, S. A., Herre, E. A., Mack, K. M., Valencia, M. C., Sanchez, E. I., et al. (2010). Negative plant–soil feedback predicts tree-species relative abundance in a tropical forest. Nature 466, 752–755. doi:10.1038/nature09273
Pernilla Brinkman, E., Van Der Putten, W. H., Bakker, E. J., and Verhoeven, K. J. (2010). Plant–soil feedback: Experimental approaches, statistical analyses and ecological interpretations. J. Ecol. 98, 1063–1073. doi:10.1111/j.1365-2745.2010.01695.x
Petermann, J. S., Fergus, A. J., Turnbull, L. A., and Schmid, B. (2008). Janzen-Connell effects are widespread and strong enough to maintain diversity in grasslands. Ecology 89, 2399–2406. doi:10.1890/07-2056.1
Reinhart, K. O. (2012). The organization of plant communities: negative plant–soil feedbacks and semiarid grasslands. Ecology 93, 2377–2385. doi:10.1890/12-0486.1
Reynolds, H. L., Packer, A., Bever, J. D., and Clay, K. (2003). Grassroots ecology: Plant–microbe–soil interactions as drivers of plant community structure and dynamics. Ecology 84, 2281–2291.
Shang, Z., Dong, Q., Shi, J., Zhou, H., Dong, S., Shao, X., et al. (2018). Research progress in recent ten years of ecological restoration for “black soil land” degraded grassland on Tibetan plateau—concurrently discuss of ecological restoration in sanjiangyuan region. Acta Agrestia Sin. 26, 1–21. doi:10.11733/j.issn.1007-0435.2018.01.001
She, Y., Yang, X., Ma, L., Zhang, Z., Wang, D., Huang, X., et al. (2021). Study on the characteristics and interrelationship of plant community and soil in degraded alpine meadow. Acta Agrestia Sin. 29, 62–71. doi:10.11733/j.issn.1007-0435.2021.Z1.008
Sun, H., Li, X., Jin, L., Zhang, J., and Li, Q. (2019). Variation of vegetation community and soil nutrients of artificial grassland in the source zone of the Yellow River. Bull. Soil Water Conserv. 39, 25–30. doi:10.13961/j.cnki.stbctb.2019.03.005
Sun, J., Wang, Y., Piao, S., Liu, M., Han, G., Li, J., et al. (2022). Toward a sustainable grassland ecosystem worldwide. Innovation 3, 100265. doi:10.1016/j.xinn.2022.100265
Tripathi, B. M., Stegen, J. C., Kim, M., Dong, K., Adams, J. M., and Lee, Y. K. (2018). Soil pH mediates the balance between stochastic and deterministic assembly of bacteria. ISME J. 12, 1072–1083. doi:10.1038/s41396-018-0082-4
Van De Voorde, T. F., Van Der Putten, W. H., and Martijn Bezemer, T. (2011). Intra-and interspecific plant–soil interactions, soil legacies and priority effects during old-field succession. J. Ecol. 99, 945–953. doi:10.1111/j.1365-2745.2011.01815.x
Van Der Putten, W., Van Dijk, C., and Peters, B. (1993). Plant-specific soil-borne diseases contribute to succession in foredune vegetation. Nature 362, 53–56. doi:10.1038/362053a0
Van Der Putten, W. H. (2003). Plant defense belowground and spatiotemporal processes in natural vegetation. Ecology 84, 2269–2280. doi:10.1890/02-0284
Wang, Q.-L., Wang, C.-T., Liu, W., Cao, G.-M., and Long, R.-J. (2009). Changes in plant diversity, biomass and soil C, in alpine meadows at different degradation stages in the headwater region of three rivers, China. Yingyong Shengtai Xuebao 20, 187–198. doi:10.1002/ldr.879
Wang, X., Dong, S., Sherman, R., Liu, Q., Liu, S., Li, Y., et al. (2014). A comparison of biodiversity–ecosystem function relationships in alpine grasslands across a degradation gradient on the Qinghai–Tibetan Plateau. Rangel. J. 37, 45–55. doi:10.1071/rj14081
Wang, Z., Jin, K., Ding, Y., Paul, C., Zhang, Y., and Li, Y. (2022). The mechanism of pants-soil microbial feedback in grassland succession. Chin. J. Grassl. 44, 95–103.
Wardle, D. A., Bardgett, R. D., Klironomos, J. N., Setala, H., Van Der Putten, W. H., and Wall, D. H. (2004). Ecological linkages between aboveground and belowground biota. Science 304, 1629–1633. doi:10.1126/science.1094875
Wu, S., Zhang, X., Gao, X., Xu, Y., Wu, X., Shan, X., et al. (2019). Succession dynamics of a plant community of degraded alpine meadow during the human-induced restoration process in the Three Rivers Source region. Acta Ecol. Sin. 39, 2444–2453. doi:10.5846/stxb201804070783
Xing, Y., Wang, X., Liu, Y., Hua, R., Wang, C., Wu, J., et al. (2020). Characteristics of plant community and soil organic carbon and nitrogen in artificial grassland with different planting years. Acta Agrestia Sin. 28, 521–528. doi:10.11733/j.issn.1007-0435.2020.02.028
Yang, X., Zhou, Q., and Wang, T. (2007). Connotation and ecologicalindicators of soil health and its research prospect. Ecol. Sci. 26, 374–380. doi:10.3969/j.issn.1008-8873.2007.04.014
Yang, X., Wang, C., Zi, H., and Liu, M. (2015). Soil microbial community structure characteristics in artificial grassland with different cultivation years in the headwater region of three rivers, China. Chin. J. Appl. Environ. Biol. 21, 341–349. doi:10.3724/SP.J.1145.2014.10033
Yuan, J., Jiang, X., Huang, W., and Wang, G. (2004). Effects of grazing intensity and grazing season on plant species diversity in alpine meadow. Acta Prataculturae Sin. 13, 16–21. doi:10.3321/j.issn:1004-5759.2004.03.003
Yue, G., Zhao, L., Wang, Z., Zou, D., Zhang, L., Qiao, Y., et al. (2015). Relationship between alpine meadow root distribution and active layer temperature variation in permafrost areas. J. Glaciol. Geocryol. 37, 1381–1387. doi:10.7522/j.isnn.1000-0240.2015.0152
Zhang, Y.-S., Zhao, X.-Q., and Huang, D.-Q. (2003). The study on sustainable using of perennial sowing grassland in the Qinghai-Tibet Plateau pasture. Acta Prataculturae Sin. 12, 22–27. doi:10.3321/j.issn:1004-5759.2003.03.004
Zhang, L., Wang, C.-T., Liu, W., and Wang, Q.-L. (2012). Relationships of dominant species root activity, plant community characteristics and soil micro-environment in artificial grassland over different cultivation periods. Acta Prataculturae Sin. 21, 185. doi:10.11686/cyxb20120523
Zhang, Y., Liu, Z., Li, Y., and Chi, R. (2014). Optimization of ionic liquid-based microwave-assisted extraction of isoflavones from Radix puerariae by response surface methodology. Sep. Purif. Technol. 129, 71–79. doi:10.1016/j.seppur.2014.03.022
Zhang, L., Unteregelsbacher, S., Hafner, S., Xu, X., Schleuss, P. M., Miehe, G., et al. (2017). Fate of organic and inorganic nitrogen in crusted and non-crusted Kobresia grasslands. Land Degrad. Dev. 28, 166–174. doi:10.1002/ldr.2582
Zhang, J., Wang, L., Yao, T., Li, H., Gao, Y., Yang, X., et al. (2019). Plant community structure and species diversity differences in alpine grassland in the Qilian Mountains with different levels of degradation. Acta Prataculturae Sin. 28, 15–25. doi:10.11686/cyxb2018302
Zhang, Z., Liu, Y., Sun, J., and Wu, G.-L. (2021). Suitable duration of grazing exclusion for restoration of a degraded alpine meadow on the eastern Qinghai-Tibetan Plateau. Catena 207, 105582. doi:10.1016/j.catena.2021.105582
Zhao, Y., Chen, W., Li, C., Chen, J., and Zhang, D. (2009). Content of soil organic matter and its relationships with main nutrients on degraded alpine meadow in Eastern Qilian Mountains. Pratacultural Sci. 26, 20–25. doi:10.3969/j.issn.1001-0629.2009.05.004
Zhou, H., Zhao, X., Zhou, L., Liu, W., Li, Y., and Tang, Y. (2005). A study on correlations between vegetation degradation and soil degradation in the 'Alpine Meadow' of the Qinghai-Tibetan Plateau. Acta Prataculturae Sin. 14, 31–40.
Zhou, H., Zhao, X., Wen, J., Chen, Z., Yao, B., Yang, Y., et al. (2012). The characteristics of soil and vegetation of degenerated alpine steppe in the Yellow River Source Region. Acta Prataculturae Sin. 21, 1. doi:10.11686/cyxb20120501
Zhou, H., Zhang, D., Jiang, Z., Sun, P., Xiao, H., Yuxin, W., et al. (2019). Changes in the soil microbial communities of alpine steppe at Qinghai-Tibetan Plateau under different degradation levels. Sci. Total Environ. 651, 2281–2291. doi:10.1016/j.scitotenv.2018.09.336
Zi, H., Hu, L., Ade, L., and Wang, C. (2015). Distribution patterns of ratio of root to soil and soil physical chemical characteristics at the different degraded successional stages in an alpine meadow. Acta Agrestia Sin. 23, 1151–1160. doi:10.11733/j.issn.1007-0435.2015.06.004
Keywords: plant-soil feedback, ecosystem multifunctionality, biotic factors, abiotic factors, degradation, recovery
Citation: Yandi S, Tao M, Huakun Z, Honglin L, Zhonghua Z, Li M, Ruimin Q, Hongye S, Tao C, Jingjing W and Xue H (2023) Characterization of the Plant‒Soil feedback index in alpine meadow degradation and recovery: A field experiment. Front. Environ. Sci. 10:1097030. doi: 10.3389/fenvs.2022.1097030
Received: 13 November 2022; Accepted: 12 December 2022;
Published: 10 January 2023.
Edited by:
Xiaoyu Li, Northeast Institute of Geography and Agroecology (CAS), ChinaCopyright © 2023 Yandi, Tao, Huakun, Honglin, Zhonghua, Li, Ruimin, Hongye, Tao, Jingjing and Xue. This is an open-access article distributed under the terms of the Creative Commons Attribution License (CC BY). The use, distribution or reproduction in other forums is permitted, provided the original author(s) and the copyright owner(s) are credited and that the original publication in this journal is cited, in accordance with accepted academic practice. No use, distribution or reproduction is permitted which does not comply with these terms.
*Correspondence: Zhou Huakun, aGt6aG91QG53aXBiLmNhcy5jbg==
Disclaimer: All claims expressed in this article are solely those of the authors and do not necessarily represent those of their affiliated organizations, or those of the publisher, the editors and the reviewers. Any product that may be evaluated in this article or claim that may be made by its manufacturer is not guaranteed or endorsed by the publisher.
Research integrity at Frontiers
Learn more about the work of our research integrity team to safeguard the quality of each article we publish.