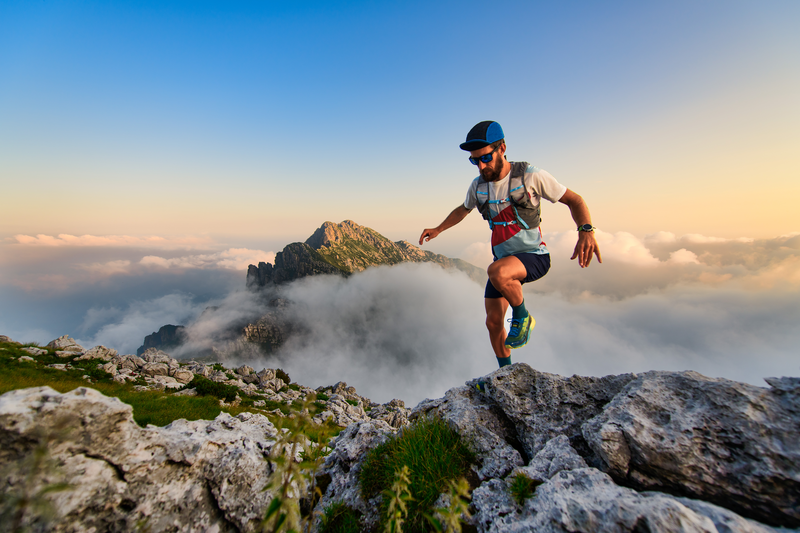
94% of researchers rate our articles as excellent or good
Learn more about the work of our research integrity team to safeguard the quality of each article we publish.
Find out more
ORIGINAL RESEARCH article
Front. Environ. Sci. , 05 January 2023
Sec. Toxicology, Pollution and the Environment
Volume 10 - 2022 | https://doi.org/10.3389/fenvs.2022.1095731
This article is part of the Research Topic Hazardous Pollutants in the Environment: Analysis, Assessment and Remediation View all 10 articles
The water quality of the Golmud River is essential for environmental preservation and economic growth of Golmud city and Qarhan Salt Lake in China. Thirty-four samples of surface water and sediment from seventeen places in the Golmud River and thirty-two dustfall samples in the Qaidam Basin were collected. The concentrations of heavy metals (HMs) were measured; water quality, risk assessment, and multiple source analysis were applied. Concentrations of HMs in water were Zn > Cu > Ni > As > Pb > Cd > Hg, and in sediment were Ni > Zn > Pb > As > Cu > Cd > Hg. In water, the Nemerow pollution index (NP) values indicated that most of the sampling points seemly were seriously polluted; other water quality assessment results suggested no pollution. In sediment, the concentrations of 27% HMs exceeded the background values of soil in Qinghai; 48% exceeded the Earth crust background values, which were As, Hg, and Cd. The single factor index method (Pi), geological accumulation index (Igeo), and contamination factor (CF) revealed that As pollution is serious, followed by Hg and Cd; the pollution load index (PLI) and modified pollution index (mCd) values indicated that 64% and 57% of samples were polluted. NP values are shown serious pollution. The ecological risk results demonstrated a low risk in water and a medium risk in sediment. The average total hazard quotient values in sediment and water for adults and children revealed low non-carcinogenic risks. Carcinogenic risk indicated Ni in water and sediment, and As in sediment may be involved in cancer risk. Multivariate statistics showed that the HMs mainly came from nature, and human activities will also impact them. The upper continental crust values indicated that As and Hg have high background values. The saline dust storm was one of the essential sources of HMs, especially Hg. Various provenances constituted the material cycling of HMs in the surface environment.
In recent years, HM pollution has become an important environmental hazard and has significantly increased (Ahmed et al., 2015; Vu et al., 2017; Li et al., 2020a). Moreover, it has drawn much attention due to its heavy toxicity and endurance (Zahra et al., 2014; Wei et al., 2016; Lanzerstorfer, 2018; Liu et al., 2018a; Chen et al., 2019). Under natural environmental conditions, the content of HMs in rivers is usually very low, but through unreasonable human actions, such as the emission of agricultural, industrial, domestic wastewater, waste residue, and exhaust gas, excessive HMs are supplied to rivers, lakes, and other surface waters (Tchounwou et al., 2012; Deng et al., 2016; Goher et al., 2019; Bashir et al., 2020; Gopinathan et al., 2022a; Gopinathan et al., 2022b); surface and underground runoff, polluted river sediment, and atmospheric sedimentation can also release HMs, thus resulting in a grave threat to the aquatic environment (Saleem et al., 2015; Harikrishnan et al., 2017; Benson et al., 2018; Sun et al., 2018). In addition, it has been demonstrated that exposure to HM can result in a variety of health problems (Sharma and Agrawal, 2005; Tchounwou et al., 2012; Jaishankar et al., 2014), including harm to the kidneys (Tchounwou et al., 2012) and the development of neuroscience (Jaishankar et al., 2014). Cd, Hg, As, and Pb are toxic heavy metals (Clemens and Ma, 2016); these HMs tend to accumulate in different tissues of the human body and are highly toxic even at low concentrations (Sharafi et al., 2019). Among them, Cd and As are carcinogenic heavy metals, which are widely distributed in the crust (Mehdi et al., 2019). Cu and Zn are essential elements for human metabolism and various biological molecules (Meghdad et al., 2016). However, if their concentrations exceed certain limits, they may endanger human health (Ali et al., 2013).
For the transport and transformation of HMs, water and sediment are essential transporters and storage mediums (Chen et al., 2022). Sediments serve as a supply and a sink for HMs (Liu et al., 2012). Adsorption, hydrolysis, and coprecipitation reactions can all cause the deposition of HMs in sediment (Singh et al., 2005). As it continues to increase, the presence of HMs in the surface sediment is becoming an important issue of global concern. HMs can harm ecological systems and human health since they are durable in river silt and are not easily broken down by bacteria (João et al., 2019). At present, there are many assessment methods for HM pollution in water bodies and sediment, but they all have certain limitations. To compensate for the shortcomings of different methods, a variety of HM pollution assessment methods should be jointly used for comprehensive analysis and evaluation. In recent years, a number of indicators, such as Igeo, CF, and PLI, have been established to determine the level of HM pollution in sediments (Wang et al., 2018; Withanachchi et al., 2018; Zhang et al., 2018), and numerous techniques, including multivariate statistical methods (e.g., principal component analysis, factor analysis, and cluster analysis) (Bodrud-Doza et al., 2016; Gulgundi and Shetty, 2018), have been created to assess water quality.
The Qinghai-Tibet Plateau is frequently referred to as the Third Pole and the Roof of the World (Li D. et al., 2022). It is distinguished by having the most high-altitude rivers in the world, a small number of residents, and few anthropogenic activities (Liu et al., 2021). The main water supply for industrial production, agricultural irrigation, and routine activities in Golmud city is the Golmud River, the second-largest inland river in the Qaidam Basin. At the same time, it is an important source of salt-forming substances for Qarhan Salt Lake. Therefore, the water quality of the Golmud River is vital for regional socioeconomic development and environmental protection. Unfortunately, there is still a shortage of comprehensive analyses and studies on water and sediment quality assessments, ecological-health risk assessments, and source identification of HMs in the Golmud River. Therefore, carrying out this research is crucial. Thirty-four surface water and sediment samples from 17 sites in the Golmud River were collected to thoroughly understand the current distribution of HMs in the surface water and sediment of the Golmud River; seven HM (As, Hg, Pb, Cd, Cu, Ni, and Zn) and eight constant ion (CO32−, HCO3−, SO42−, Cl−, K+, Ca2+, Na+, and Mg2+) concentrations were measured. Meanwhile, to determine the secondary source of HMs, thirty-two dustfall samples from the Qaidam Basin were collected, and HMs were also tested. The evaluation of water and sediment quality, the risk to public health, and the recognition of HM sources in the Golmud River can all be provided in this research.
With a length of 468 km, the Golmud River is located at the southern edge of the Qaidam Basin in the north of the Qinghai Tibet Plateau, with a drainage area of 35°49′46″–37°5′19″N and 94°21′13″–95°12′46″E, its main stream length is 325 km and has a basin area of 18,648 km2 (Wang, 2014a). The terrain in the area is generally high in the South and low in the North, with the Kunlun Mountains in the South and Qaidam Basin in the North. The upstream of the Golmud River is divided into two branches: the Kunlun River and the Xueshui River. Both branches are mainly supplied by the melting water of ice and snow and atmospheric precipitation in the Kunlun Mountains. After converging in the mountains, the runoff flows from South to North and flows into the Qaidam Basin through the southern mountain pass. The Golmud River Basin has a dry climate with little precipitation. The annual evaporation is high, reaching 3,066 mm/a. According to earlier research, except for the eastern reach of the alluvial fan sector (a groundwater recharge river), the entire area is dominated by river recharge to groundwater. The arid climatic conditions of the inland basin formed a typical desertification landscape. Considering Golmud city as the boundary, the southern part is dominated by desertification, and the northern part is dominated by salinization. The landforms of the Golmud River Basin can be divided into five units from South to North: the Gobi, alluvial fans, flood alluvial plain, lacustrine plain, and salt lake.
Thirty-four samples of surface water (W1–W17) and sediment (S1–S17) were collected from seventeen sampling stations in December 2021 (Figure 1). The studied sites were divided into freshwater (1–14) and saline (15–17) groups. Sampling was conducted every 20 km from the Golmud River to Dabuxun Salt Lake. Surface water samples were obtained from the top layer (0–50 cm) of the river at every sampling location used a plastic spoon. The samples were then put into 500 ml polypropylene bottles after being conditioned with nitric acid (HNO3) to a pH of under two. With the aid of a shovel, surface sediment samples were obtained and kept in disposable polypropylene bags. The obtained water and sediment samples were immediately sent to the laboratory for additional analysis after being stored in a cooler at 4°C. In addition, thirty-two dustfall samples in the Qaidam Basin were collected to analyze the source of HMs, and the collection method of dustfall samples refers to Gen Jun’s paper (Gen, 2021).
According to previous analysis data (Xiang, 2020), an ICAP QC inductively coupled plasma mass spectrometer (ICP-MS) was utilized to identify HM concentrations, and its detection thresholds were as follows: .015 [Pb] and [As], .001 [Hg], .0160 [Ni], .133 [Cu], .470 [Zn], and .035 μg/L [Cd]. While measuring HMs in saline using ICP-MS, the mineralization should be below 2 g/L. Excessively high mineralization will cause the salt to block the sampling cone (Song et al., 2014). Using a NexION 2000 inductively coupled plasma optical emission spectrometer (ICP-OES), Na+, K+, Mg2+, Ca2+, and SO42− were detected, and HCO3− and Cl− were titrated by chemical titration (allowable error 3‰). To avoid contamination during testing, all glassware was soaked in HNO3 at a concentration of 5% for 24 h before use and then washed and dried. The testing and analysis employed only the purest reagents. For this quality control analysis, spiked blanks, duplicate method blanks, and spiked samples were utilized to test the reliability of the results.
Various environmental and statistical methods have been used to analyze the impact of human disturbance and contamination (Yao et al., 2006; Suresh et al., 2012; Tang et al., 2014; Wang et al., 2014b; Xiao et al., 2014; Gopal et al., 2016; Iqbal et al., 2016). In this paper, to quantitatively evaluate HM pollution in the Golmud River, Pi, CF (Hakanson, 1980), NP (Li et al., 2018), HPI (Qiao et al., 2020; Karunanidhi et al., 2021a), mCd (Abrahim and Parker, 2008), and HEI (Edet and Offiong, 2002) were chosen to assess water quality. PLI (Tomlinson et al., 1980), Igeo (Taylor and McLennan, 1985; Nethaji et al., 2017), Pi, NP, mCd, and CF were applied to assess the pollution of HMs in sediment.
A useful instrument for obtaining a complete picture of lake or river water quality is the water quality index (WQI) (Tiwari and Mishra, 1985; Carvalho et al., 2021). The index condenses many water quality parameters from a significant amount of data to a solo value (Ravindra et al., 2019; Ustaoglu et al., 2020). Supplementary Table S1 displayed the relative weights (
where relative weight
The potential risk of one or more HMs on the ecological impact value is assessed by the potential ecological risk index (PERI), which was first presented by the Swedish researcher Hakanson and later widely used to assess the ecological risk of pollutants in sediment and soil (Liu et al., 2017). It has been used recently to assess the ecological risk value of water (Liu et al., 2018b). The PERI was utilized to assess HM pollution in water and sediment.
Hazard quotients (HQs) are frequently used to measure the toxicity produced by HMs (Özgür et al., 2020; Githaiga et al., 2021; Lu et al., 2021), and the hazard index (HI) is used to estimate the total possible non-carcinogenic risks deriving from various approaches (USEPA, 2004). Only HMs with carcinogenic slope factors (CSFs) are evaluated for carcinogenic risks (CRs) (Özgür et al., 2020). Surface water and sediment health risks were calculated independently in this study. The formula is provided in Supplementary Material S1–S14 for surface water and Supplementary Material S15–S18 for sediment.
The HI evaluates the total potential non-carcinogenic risks of each HM. The total hazard index (THI) was computed using Supplementary Material S19, S20 for water and Supplementary Material S21 for sediment. Carcinogenic risks (CRs) were used to compute the total carcinogenic risk (TCR) and were calculated using Supplementary Material S22, S23. Supplementary Table S2 displayed all specific definitions, units, and relevant equation values; Supplementary Table S1 provided the figures for the oral reference dosage (RfDo), oral slope factor (CSFo), gastrointestinal absorption (GIABS), and dermal permeability constant (Kp).
Surface water contains a variety of HMs, each of which can have several sources. This study used principal component analysis (PCA) and pearson correlation analysis (Singh et al., 2014; Lu, et al., 2022a; Lu, et al., 2022b) to explore the correlations among the seven HMs in surface water and sediment.
Supplementary Table S3 shows the content of HMs in water and sediment samples from all locations. Figures 2A, B depict the concentrations of seven HMs in the surface water and sediment of the different sampling sites. In surface water, the average TDS content was 1,757 mg/L, with a range of 473–15,042 mg/L. The cation was Na+ (329.87 mg/L), followed by Mg2+ (122.20 mg/L), Ca2+ (59.61 mg/L), and K+ (17.24 mg/L). The anion was Cl− (801.68 mg/L), followed by HCO3− (230.60 mg/L), SO42− (161.00 mg/L), and CO32− (71.48 mg/L). In freshwater samples, the average of As was 1.37 μg/L, while concentration values varied from .40 to 4.67 μg/L, Hg had a maximum value of .10 and an average of .04 μg/L, Pb varied from .44 to 2.37 and had an average of 1.47 μg/L, Cd varied from .01 to .16 and had an average of .05 μg/L, Cu varied from 1.24 to 13.48 and had an average of 4.52 μg/L, Ni varied from .88 to 24.64 and had an average of 7.90 μg/L, Zn varied from 3.72 to 23.66 with a mean value of 9.38 μg/L. HMs can be distinguished into two types depending on concentration, Hg and Cd were <1 μg/L and were categorized as low abundance elements; As, Pb, Cu, Ni, and Zn ranged from 1 to 100 μg/L and were deemed moderately abundant elements. Cu, Ni, and Zn were the main HMs, accounting for 18%, 32%, and 38% of the total HM elements, respectively. Hg and Cd had the lowest contents at all sampling locations. The concentration of HMs at site W14 was higher than that at other locations due to its proximity to Qarhan Salt Lake, which has a high Cl− concentration, and Cl− can form soluble complexes with HMs, and these HM complexes have a strong migration ability (Wang et al., 2013). The concentration of soluble HM complexes on the shore was high, resulting in a significant concentration of HMs at the mouth of the Golmud River. In addition, the hydrodynamic effect was significantly weakened near the salt lake, resulting in the accumulation of HMs. Combined with the Surface Water Environmental Quality (GB3838-2002), all the HMs in freshwater were suitable for Class I Standard. The water was of great quality and fit for drinking.
FIGURE 2. Heavy metal spatial distributions in the surface water (A) and sediment (B) of the Golmud River (μg/L).
In sediments, the HM concentration ranges were as follows: 9.10–16.08 for As, .05–.15 for Hg, 12.84–31.34 for Pb, .09–.21 for Cd, 7.39–10.72 for Cu, 17.39–24.52 for Ni, and 16.26–23.46 mg/kg for Zn. Pb, Ni and Zn were generally more abundant than the other elements. 27% HM concentrations were above the background levels of the soil in Qinghai, especially Hg and Cd. 48% of HMs exceeded the Earth’s crustal background values, which were As, Hg, and Cd. Therefore, Hg and Cd are the primary contaminants, and As has a high background value in the Qinghai-Tibet Plateau (Yang et al., 2020). Compared to the results of Xiang, 2020, the current investigation of Cu and Zn concentrations was much lower, and other elements were quite similar. Overall, the sediment contained the highest concentration of HMs, which had higher quantities than the water. In contrast, the lowest mean values occurred in freshwater. The concentrations of HMs are often higher in sediment than in water due to their limited solubility; they can bond to particles and accumulate in the sediment (Wang et al., 2015). Comparing saline samples to freshwater samples revealed greater HM content. Generally, the HM content at each sampling point was basically consistent. The As, Pb, Cu, Ni, and Zn concentrations in saline ranged from 1 to 100 μg/L; these were moderately abundant elements. Low abundance elements Hg and Cd were present at <1 μg/L. This is consistent with the classification of freshwater. The concentration of HMs in the freshwater changed little, and in saline, they were relatively high, which was consistent with Li D. et al. (2022) conclusion. Due to the enrichment of HMs in the salt lake, the average levels of HMs were greater.
Brine is a resource for element obtaining rather than drinking. Thus, it was impossible to determine the water quality of brine (Li L. M. et al., 2022). Figure 3 shows the Pi values of surface water and the mCd, CF and NP values. The average Pi for all HMs was less than .7, indicating that the studied region was clean (Figure 3A). All sites had clean levels of mCd, which ranged from .02 to .23; the WQI values had a mean of .11 (Figure 3B). The CF mean values of the sampling were all less than 1 (Figure 3C), indicating low pollution. Accordingly, 100% of the sampling sites demonstrated that the surface water of the Golmud River was not contaminated using the ranking criteria of Pi, mCd, and CF. The max Pi and CF have the same value of 1.23. However, Pi and CF evaluated the total pollution degree. These two HM pollution indices in surface water indicated that most test locations were not polluted, except for one site where Ni made the most dominant contribution. The range of NP values in water was 2.72–18.23, with an average of 7.96 (Figure 3D); there was heavy pollution. NP values consider the average and maximum values of each HM, and severe contaminants have a significant influence on them (Li et al., 2018; Mahmudul et al., 2022). Using NP amplifies the effects of higher concentrations of HMs such as Ni and Zn.
The HEI values had a mean of .11 and a range of .00–.40 (Supplementary Table S3). The results indicated that the HEI for each sample was less than 1. All locations had low pollution levels. The mean HPI value was 10.65, ranged from .45 to 39.52 (Supplementary Table S3); all sites were at a low pollution level and showed similar results with HEI. There was moderate pollution (30–100) of HPI with Ni, which showed similar trends with Pi and CF.
A useful method for keeping track of surface water is the WQI. It condenses several water quality characteristics from a considerable amount of data into one number (Tiwari and Mishra, 1985). In the research area, the WQI value of freshwater samples changed from 13.95 to 46.11, with a mean value of 28.25 (Figure 4). 100% of the water samples fell into the “good” category.
The mean Pi value of As was 8.69, belonged to heavy pollution; the mean Pi values of Hg and Cd were 1.59 and 1.49, belonged to low pollution; and the other HMs had clean levels (Figure 5A). PLI was calculated depending on the measured contamination factors (Anbazhagan et al., 2022). Figure 5B shows the PLI results. The PLI values of 36% of the sampling points were below 1, indicated no contamination, while the others were polluted. The Igeo was employed to quantitatively measure an individual HM in the sediment samples (Hong et al., 2020). Figure 5C shows the Igeo values of the HMs. The averaged Igeo results showed the following order of contamination: As > Hg > Cd > Ni > Pb > Cu > Zn. Depending on the criteria of pollution degree, the mean Igeo values of HMs showed no contamination in surface sediment other than As and Hg. The Igeo values of As at all sampling locations showed moderate contamination, and Hg was at different contamination levels. 50% of the sampling sites indicated no pollution, and the other half indicated low pollution. The most notable elements were Ni and Zn because their considerably higher concentrations resulted in the NP values of all sites reached heavy pollution (Figure 5D). In Figure 5E, the CF values for Pb, Cu, Ni, and Zn were .81, .35, 1.00, and .28, respectively, showed low pollution. In contrast, the CF value of As was 8.69, showed high pollution, and the CF values of Hg and Cd were 1.59 and 1.49, indicated moderate pollution. The order of As > Hg > Cd > Ni > Pb > Cu > Zn was the mean CF for all HMs. The mCd values varied from 1.71 to 2.33, with a mean value of 2.03; 43% of sampling sites were low pollution, and 57% showed moderate pollution (Figure 5F). This indicated that the accumulation of HM in the sediment was more severe than in the surface water. As, Hg, and Cd were the major HMs threatened the sediment.
FIGURE 5. Pi (A), PLI (B), Igeo (C), NP (D), CF (E), and mCd (F) in the sediment of the Golmud River.
Seven HMs are divided by their respective elemental concentrations to determine their PERI values in surface water and sediment (Figure 6). In surface water, the PERI value was 4.18, indicated a low risk (Figure 6A). The PERI values of As varied from .04 to .47 with a mean value of .14; the PERI values of Hg varied from .00 to 4.00 with an average of 1.57; the PERI values of Pb varied from .04 to .24 with an average of .15; the PERI values of Cd ranged from .08 to .98 with an average of .31; the PERI values of Cu varied from .01 to .07 with a mean value .02; Ni varied from .22 to 6.16 with an average of 1.98, and Zn varied from .00 to .01 with an average of .00. In sediment, the PERI value was 206.01, indicated medium risk (Figure 6B); the result was the same as that of Yang et al. (2020). As ranged from 60.64 to 107.19 with an average value of 86.93; Hg ranged from 32.28 to 98.71 with an average value of 63.43. Pb ranged from 3.21 to 5.20 with an average value of 4.06; Cd varied from 28.83 to 62.99 with a mean value of 44.55; Cu varied from 1.48 to 2.13 with a mean value of 1.77; Ni had PERI values between 4.35 and 6.05, with a mean value of 5.00; and Zn had PERI values between .23 and .32, with a mean value of .28. The ecological risk in the water was below that in sediment, especially As, Hg, and Cd; more attention should be given.
FIGURE 6. Heatmaps of the potential ecological risk index for heavy metals in water (A) and sediment (B) samples.
The USEPA developed the total hazard quotient (THQ) approach, which is frequently used in risk assessment (USEPA, 2004). The THQs of HMs for residential adults and children were between .01–.48 and .04–.93, with an average of .17 and .34, respectively (Figure 7A). The average THQs for adults and children were below 1.0, indicated that neither group faced high non-carcinogenic risks. With average values of .007 and .021, the THQs of HMs for recreational adults and children were between .002–.021 and .006–.062, respectively (Figure 7B). Humans are most commonly exposed by ingestion, inhalation, and dermal absorption (Karunanidhi et al., 2021b; Karunanidhi et al., 2022). The non-carcinogenic risks for water ingestion were .167 and .317 for residential adults and children, respectively, and .002 and .013 for dermal contact in the water of the Golmud River. At the same time, for recreational adults and children, the non-carcinogenic risks for water ingestion were .004 and .019, respectively, and for dermal contact, they were .002 and .003, respectively (Supplementary Table S4). For residential adults and children, the non-carcinogenic risks in saline were .0123 and .0689 by dermal contact, respectively. For dermal contact, the non-carcinogenic risks for recreational adults and children were .0128 and .017, respectively (Supplementary Table S4). These findings demonstrated that inhabitants were more susceptible than recreational receptors to water ingestion. In addition, the water ingestion way had a more detrimental impact on residents’ health than dermal contact. Notably, the non-carcinogenic risks for dermal contact and ingestion were lower in residential and recreational adults than in children. This suggested that adults were less susceptible than children when subjected to HMs in water, which was in line with the conclusions of earlier investigations (Xiao et al., 2019; Li et al., 2020b; Aravinthasamy et al., 2021; Karunanidhi et al., 2021c; Karunanidhi et al., 2021d; Li D. et al., 2022). Saline, however, was unfit for drinking, so the health risk from ingestion was not calculated.
FIGURE 7. Total hazard index (THI) and total carcinogenic risk (TCR) for residential (A,C) and recreational (B,D) receptors from heavy metals in surface water.
Figures 7C, D depict the total carcinogenic risk (TCR) results for all sample locations in the research area. As, Pb, Cd, and Ni were used for CR assessment in this paper because they have carcinogenic slope factors. For residential and recreational receptors, the TCRs of As, Pb, Cd, and Ni were 6.61 × 10−6–6.42 × 10–4 (Figure 7C) and 2.03 × 10−6–5.06 × 10–5 (Figure 7D), respectively. Correspondingly, all locations pose acceptable hazards to both residential and recreational people. The CR values of residents for Ni by the ingestion contact exceeded the threshold risk of 1 × 10–4 in freshwater. However, the CR values of residents for dermal contact were lower than that. For residents, the levels of Cd by dermal and ingestion contact were lower than 1 × 10–4, and the values of Pb were lower than 1 × 10–6 (Supplementary Table S4). As values of residents by dermal contact ways were less than 1 × 10–6, and those by ingestion contact were less than 1 × 10–4. For recreators, the CR values of As and Pb by ingestion and dermal contact were lower than 1 × 10–6, and the CR values of Cd by ingestion contact were lower than those in freshwater. The CR values for Ni by ingestion and dermal contact for recreators were lower than 1 × 10–4, and the dermal contact value of Cd for recreators was lower than that in freshwater (Supplementary Table S4). Residents had lower CR values of As, Pb, Cd, and Ni in saline for dermal contact than recreationists. As, Pb, Cd, and Ni in saline had CR values that were less than the target risk. By ingestion and dermal contact, Ni was the main contributor to the total CR for residents and recreators. Additionally, all the CR values for Ni in saline fell between 1 × 10–4 and 1 × 10–6. The findings showed that As, Pb, and Cd would not present a carcinogenic risk for freshwater inhabitants and recreators, whereas people may be at risk for cancer from Ni. All HMs would not put inhabitants and recreators in saline at carcinogenic risk.
The sediment in the Golmud River has revealed elevated concentrations of HMs, especially As, Hg, and Cd. Supplementary Table S5 displayed the THI and TCR values of HMs for sediment samples taken from the study area. The THI of HMs ranged from .06–.38 for adults and .07–.87 for children, with averages of .28 and .62, respectively. The THI mean values for adults and children were less than 1.0, indicated that neither group faced high non-carcinogenic hazards. In general, the THI for children and adults followed the order As > Pb > Ni > Cd > Hg > Cu > Zn. Adults and children had non-carcinogenic risks of .311 and .727 for sediment ingestion and .0124 and .0145 for dermal contact, respectively. Adults’ and children’s non-carcinogenic risks in salt lake sediment were .0668 and .0779 dermal contacts, respectively. This finding was consistent with the surface water results, THI values were higher for children than adults at all sampling locations. Meanwhile, skin contact had less of a negative impact on health than oral ingestion.
The TCR values of As and Ni for adults and children were above 1 × 10–4 in sediment, whereas Cd was higher than 1 × 10–6. Meanwhile, Pb values for children exceeded 1 × 10–6, whereas those for adults fell below 1 × 10–6. The CR values of As and Ni in saline for adults and children were between 1 × 10–6 and 1 × 10–4. The CR values of Pb and Cd were below 1 × 10–6). The main contributors to the TCR were As and Ni. The findings suggested that As and Ni might be carcinogenic risks in sediment, while Pb and Cd would not. In saline, none of the HMs would present a carcinogenic risk.
The correlation analysis results indicated that Pb-As (.78), Cd-Pb (.88), Cu-As (.99), Cu-Pb (.82), Cu-Cd (.63), Ni-Pb (.64), and Ni-Cd (.73) were significantly positively correlated; Cd-As (.58), Zn-As (.60) and Zn-Cu (.54) were positively correlated; and Hg and other HMs revealed insignificantly positive correlations (Figure 8A). The data were shown to be fit for PCA by the KMO = .653 and Bartlett’s sphericity tests (<.001). Three major components were identified by removing eigenvalues >1, accounting for 90.579% of the overall variability. As, Pb, Cd, Cu, and Ni were the elements that were grouped into the first principal component (PC1) and contributed 56.7% of the variation, this was further confirmed by the significant correlation between these elements, which suggested that their sources were similar; the contribution rate of the second principal component (PC2) was 18.9%, and the positive load on Zn was relatively high. As and Cu were weakly correlated with Zn; the contribution rate of the third principal component (PC3) was 15.1% (Figure 8C). The positive load on Hg was relatively high, indicated that Hg has an independent source and was consistent with the results in Figure 8A.
FIGURE 8. Pearson correlation (A,B) and principal component analysis (PCA) (C,D) of HMs in surface water and sediment samples.
Human activities are important factors that affect natural processes and the migration and accumulation of HMs: 1) Point-source pollution of HMs from human activities: Golmud is the major industrial city in the Qaidam Basin. The typical components of industry include Ni, As, Pb, Cd, and Cu (Cheng and Hu, 2010; Pourkhabbaz and Pourkhabbaz, 2012; Yuen et al., 2012; McComb et al., 2015; Chen et al., 2016). Previous studies revealed that untreated industrial wastewater and sewage discharge might be the source of Ni, Cd, Pb, and Cu (Shamuyarira and Gumbo, 2014; Rzetala, 2016; Gopinathan et al., 2022c). With social and economic development, wastewater and industrial fuel combustion containing HMs discharged from human activities in Golmud city and Qarhan Salt Lake directly impact the Golmud River. Moreover, brine has many HMs, it is a source of element extraction. 2) Line source pollution of HMs from human activities: Golmud city is the hub of essential transportation lines such as the Qinghai-Tibet Highway and Qinghai-Tibet Railway. These transportation lines parallel the Golmud River (Xiang, 2020). Pb, Zn, Cu, and As are the main elements in traffic exhaust and dust (Lough et al., 2005; Cheng and Hu, 2010; Li et al., 2012; Pourkhabbaz and Pourkhabbaz, 2012; Yuen et al., 2012; McComb et al., 2015; Zhao et al., 2019). Therefore, transportation emissions directly caused linear pollution in the Golmud River. 3) Non-point source pollution of HMs from human activities: Oasis agriculture has developed in the surrounding areas of Golmud city. The misuse of phosphate fertilizer in agriculture may cause Zn contamination (Luo et al., 2007; Ke et al., 2017); essential parts of insecticides, herbicides, and fertilizers include As (Chen et al., 2020). The primary source of Cu is typically agriculture, which is frequently obtained via pesticides and fertilizers. As a result, the Cu concentration of farmland has significantly increased (Qu et al., 2018).
Except for the anthropogenic sources mentioned above, the river water chemistry characteristics are closely related to the regional bedrock geochemical background and weathering (Karim and Veizer, 2000). Thus, understanding the geochemical background of the bedrock distribution areas is vital to determine the sources of HMs. We used UCC standardized diagrams (Taylor and Mclennan, 1985) for comparison. That is, the mean value of HMs in the Kunlun River bedrock was compared with the average UCC value. The analysis revealed that Hg and As were significantly enriched after UCC standardization (Supplementary Table S6). This conclusion was in accordance with the result that the As background of the Qinghai-Tibet Plateau is high (Yang et al., 2020). This showed that human activities had a minimal impact on the presence of As and Hg in this basin and was mainly influenced by the weathering of the local parent rock. In contrast, the other five elements did not change significantly; in the bedrock area rocks, the influence of the geochemical background of HMs, such as As, Hg, and other HMs with high contents on the river, was unquestionable.
A significant source of HM pollution is sediment, and it has more stable HM concentrations than water (Ustaoglu et al., 2020). When the river environment changes, HMs may be liberated from sediment, resulting in secondary contamination. The sediment of the Golmud River was also polluted with Hg and Cd. The conclusions of correlation analysis indicated that Cu-As (.70), Ni-Cd (.69), Zn-Pb (.66), Zn-Cd (.66), Zn-Cu (.67), and Zn-Ni (.64) were significantly positively correlated; Ni-Pb (.50), Ni-Cu (.54) and Zn-As (.59) were positively correlated (Figure 8B). The data were shown to be fit for PCA by the KMO = .686 and Bartlett’s sphericity tests (<.001). Three principal components were found to account for 83.325% of the overall variability by extracting eigenvalues >1, as shown in Figure 8D. A total of 52.0% of the variation was contributed by PC1. The dominant elements changed to Zn (.92), Ni (.80), Cu (.78), Pb (.76), As (.67), and Cd (.65), implied a more complicated source. PC2 demonstrated a positive loading for Cu (.47) and As (.62), which explained 17.6% of the overall variation. PC3 had a significant positive loading for Hg (.95) and 13.6% of the total variance. There were parallels between the sources of HMs in sediment and those in water. Hg was also the single dominant metal for PC3, while As and Cu represent PC2.
The Qaidam Basin is one of the vital places of dust storm disasters in China (Gen, 2021), so saline dust storms are a source of HMs that cannot be ignored. Sheng (2015) studied the chemical composition of the Golmud dust storm and revealed that Pb, Zn, Ni, and Cu were gathered in dust aerosols, which anthropogenic emissions may have caused. This paper tested the HM components in atmospheric dustfall (thirty-two samples in total were gathered) in the Qaidam Basin to understand the enrichment and impact of salt-laden dustfall on HMs. The results indicated that the average HMs in dustfall were 15.94 mg/kg for As, .30 mg/kg for Hg, 43.71 mg/kg for Pb, .92 mg/kg for Cd, 16.35 mg/kg for Cu, 19.94 mg/kg for Ni, and 46.32 mg/kg for Zn (Supplementary Table S6). The content of the element was in the order of Zn > Pb > Ni > Cu > As > Cd > Hg. Compared with the background values of soil in Qinghai, China, and the World (Wei et al., 1990). The average As, Hg, Pb, and Cd contents were higher, and the Cu, Ni, and Zn contents were lower. These findings indicated that the content of these four HMs (As, Hg, Pb, and Cd) in the dustfall was relatively high, especially the Hg content in the dustfall, which was fifteen times as much as the background values of the soil in Qinghai. Therefore, dustfall was one of the essential sources of HMs, especially Hg. HMs in dustfall are neither different from those due to natural sources, such as rock weathering, nor human activities, such as pollutant emissions. It has both natural and artificial sources and is a secondary mixture source in a unique climatic environment. There is still a lack of comprehensive research on the impact of heavy-metal-containing dust on the river environment.
Based on the sources of HMs discussed above, we proposed a new model: the cycling model of HMs in the surface environment (Figure 9). The process from source to sink is the primary line of migration and enrichment of HMs. Undoubtedly, the bedrock area of the Kunlun Mountains has a high HM geochemical background and is the important source of HMs in the Golmud River. After the rock is weathered and leached, many ions, including HMs, are released into the river, which flows downstream to the basin. From the mountainous watershed of the river to the terminal salt lake, the arid climate causes the continuous salinization of the river and constant enrichment of HMs, which are highly enriched in the salt lake and salt deposits (including playa). In summary, the analysis above considers common natural processes and human actions; however, the source of HMs in the Golmud River is comprehensive and complex. HMs continue to undergo complex migration, enrichment, and transformation with various carriers in atmospheric, soil, and water environments. Under the natural geological and geographical conditions of the Qaidam Basin, various provenances in different environments constitute the material cycle of HM elements in the regional surface environment.
In this research, the decreasing trends of HM concentrations in both water and sediment were noted as Zn > Cu > Ni > As > Pb > Cd > Hg and Ni > Zn > Pb > As > Cu > Cd > Hg. The HM contents in water were low but relatively high in sediment. Brine is a particular water type, and existing water quality standards are unsuitable for measuring whether HMs pollute it. Pi, mCd, CF, HPI, HEI, and WQI revealed no risk to the water of the Golmud River, and it was clean. The NP values of HMs in water and sediment were primarily influenced by high concentrations of Ni and Zn. In sediment, the concentrations of 27% HMs exceeded the background values of soil in Qinghai; 48% exceeded the Earth’s crust background values, and six water quality evaluation methods showed that As, Hg, and Cd were the major pollutants. The ecological risk values of HMs showed a low risk in water and a medium risk of Hg in sediment. The average THQ values for adults and children suggested that the non-carcinogenic risks were low. According to the carcinogenic risk, Ni may be carcinogenic in freshwater; As and Ni may be carcinogenic in sediment. Multivariate statistical analysis showed that the HMs mainly came from nature, and human activities will also have a certain impact on them. As and Hg had high background values, saline dust storms were one of the critical sources of HMs, especially Hg. The influence of the natural background, human activities, and various provenances in different environments constitutes the material cycling of HM elements in the regional surface environment.
The original contributions presented in the study are included in the article/Supplementary Material, further inquiries can be directed to the corresponding author.
NC: Sample collection, data analysis, writing of the original draft. LL: Reviewing and editing. HZ: Conducted fieldwork and revised the initial draft. LC: Drew figures. SL: Sample collection, investigation. FM: Article language modification. XZ: Supervision, modify the article, funding acquisition. All authors agree to be accountable for the content of the work.
This work was financially supported by the Second Tibetan Plateau Scientific Expedition and Research (STEP) (2019QZKK0805), the Science and Technology Plan of Qinghai Province of China (2021-ZJ-T07), the National Natural Science Foundation of China (grant no. 41561144009) and the Basic Frontier Scientific Research Program of the Chinese Academy of Sciences (grant no. ZDBS-LY-DQC021).
We thank Donglin Gao for his help in drawing. Xiaolong Yuan, Rongchang Hong, LC, and Zheng Chang for their assistance in the fieldwork and Bo Wang, Haijun Li, Yuan Xue, Yuan Li, Guangqin Zhu, and Xiuzhen Ma for their help with sample pretreatment and analysis.
The authors declare that the research was conducted in the absence of any commercial or financial relationships that could be construed as a potential conflict of interest.
All claims expressed in this article are solely those of the authors and do not necessarily represent those of their affiliated organizations, or those of the publisher, the editors and the reviewers. Any product that may be evaluated in this article, or claim that may be made by its manufacturer, is not guaranteed or endorsed by the publisher.
The Supplementary Material for this article can be found online at: https://www.frontiersin.org/articles/10.3389/fenvs.2022.1095731/full#supplementary-material
Abrahim, G., and Parker, R. (2008). Assessment of heavy metal enrichment factors and the degree of contamination in marine sediments from Tamaki Estuary, Auckland, New Zealand. Environ. Monit. Assess. 136, 227–238. doi:10.1007/s10661-007-9678-2
Ahmed, M. K., Baki, M. A., Islam, M. S., Kundu, G. K., Habibullah-Al-Mamun, M., Sarkar, S. K., et al. (2015). Human health risk assessment of heavy metals in tropical fish and shellfish collected from the river Buriganga, Bangladesh. Environ. Sci. Pollut. Res. Int. 22 (20), 15880–15890. doi:10.1007/s11356-015-4813-z
Ali, H., Khan, E., and Sajad, M. A. (2013). Phytoremediation of heavy metals-concepts and applications. Chemosphere 91, 869–881. doi:10.1016/j.chemosphere.2013.01.075
Anbazhagan, V., Partheeban, E. C., and Rajendran, R. (2022). Ecological risk assessment and seasonal variation of heavy metals in water and sediment collected from industrially polluted Cuddalore coast, Southeastern India. Regional Stud. Mar. Sci. 49, 102134. doi:10.1016/j.rsma.2021.102134
Aravinthasamy, P., Karunanidhi, D., Shankar, K., Subramani, T., Setia, R., Bhattacharya, P., et al. (2021). COVID-19 lockdown impacts on heavy metals and microbes in shallow groundwater and expected health risks in an industrial city of South India. Environ. Nanotechnol. Monit. Manag. 16, 100472. doi:10.1016/j.enmm.2021.100472
Azhari, A. E., Rhoujjati, A., Hachimi, M. L. E., and Ambrosi, J. P. (2017). Pollution and ecological risk assessment of heavy metals in the soil-plant system and the sediment-water column around a former Pb/Zn-mining area in NE Morocco. Ecotoxicol. Environ. Saf. 144, 464–474. doi:10.1016/j.ecoenv.2017.06.051
Bashir, I., Lone, F. A., Bhat, R. A., Mir, S. A., Dar, Z. A., and Dar, S. A. (2020). Concerns and threats of contamination on aquatic ecosystems. Bioremediation Biotechnol. 27, 1–26. doi:10.1007/978-3-030-35691-0_1
Benson, N. U., Adedapo, A. E., Fred-Ahmadu, O. H., Williams, A. B., Udosen, E. D., Ayejuyo, O. O., et al. (2018). New ecological risk indices for evaluating heavy metals contamination in aquatic sediment: A case study of the gulf of Guinea. Reg. Stud. Mar. Sci. 18, 44–56. doi:10.1016/j.Prisma.2018.01.004
Bodrud-Doza, M., Islam, A. R. M. T., Ahmed, F., Das, S., Saha, N., and Rahman, M. S. (2016). Characterization of groundwater quality using water evaluation indices, multivariate statistics and geostatistics in central Bangladesh. Water Sci. 30, 19–40. doi:10.1016/j.wsj.2016.05.001
Carvalho, V. S. D., Santos, I. F. D., Almeida, L. C., Souza, C. T. D., Júnior, J. B. D. S., Souza, L. A., et al. (2021). Spatio-temporal assessment, sources and health risks of water pollutants at trace levels in public supply river using multivariate statistical techniques. Chemosphere 282, 130942. doi:10.1016/j.chemosphere.2021.130942
Chen, J., Huang, Q., Lin, Y., Fang, Y., Qian, H., Liu, R., et al. (2019). Hydrogeochemical characteristics and quality assessment of groundwater in an irrigated region, Northwest China. Water 11, 96. doi:10.3390/w11010096
Chen, J. W., Zhang, H., Xue, J. Z., Yuan, L., Yao, W., and Wu, H. X. (2022). Study on spatial distribution, potential sources and ecological risk of heavy metals in the surface water and sediments at Shanghai Port, China. Mar. Pollut. Bull. 181, 113923. doi:10.1016/j.marpolbul.2022.113923
Chen, T., Chang, Q. R., Liu, J., Clevers, J. G. P. W., and Kooistra, L. (2016). Identification of soil heavy metal sources and improvement in spatial mapping based on soil spectral information: A case study in northwest China. Sci. Total Environ. 565, 155–164. doi:10.1016/j.scitotenv.2016.04.163
Chen, Y., Liu, Q., Xu, M., and Wang, Z. (2020). Inter-annual variability of heavy metals pollution in surface sediments of jiangsu coastal region, China: Case study of the dafeng port. Mar. Pollut. Bull. 150, 110720. doi:10.1016/j.marpolbul.2019.110720
Cheng, H., and Hu, Y. (2010). Lead (Pb) isotopic fingerprinting and its applications in lead pollution studies in China: A review. Environ. Pollut. 158 (5), 1134–1146. doi:10.1016/j.envpol.2009.12.028
Clemens, S., and Ma, J. F. (2016). Toxic heavy metal and metalloid accumulation in crop plants and foods. Annu. Rev. Plant Biol. 67, 489–512. doi:10.1146/annurev-arplant-043015-112301
Deng, J. C., Wang, Y. S., Liu, X., Hu, W. P., Zhu, J. G., and Zhu, L. (2016). Spatial distribution and risk assessment of heavy metals and as pollution in the sediments of a shallow lake. Environ. Monit. Assess. 188, 296. doi:10.1007/s10661-016-5301-8
Edet, A. E., and Offiong, O. E. (2002). Evaluation of water quality pollution indices for heavy metal contamination monitoring. A study case from the Akpabuyo-Odukpani area, Lower Cross River Basin (southeastern Nigeria). GeoJournal. 57, 295–304.
Gen, J. (2021). Dissertation. China: University of Chinese Academy of Sciences.Temporal and spatial distribution characteristics and discussion on provenance of soluble salt in atmospheric dustfall in the south of Qaidam Basin.
Githaiga, K. B., Njuguna, S. M., Gituru, R. W., and Yan, X. (2021). Water quality assessment, multivariate analysis and human health risks of heavy metals in eight major lakes in Kenya. J. Environ. Manag. 297, 113410. doi:10.1016/j.jenvman.2021.113410
Goher, M. E., Ali, M. H. H., and El-Sayed, S. M. (2019). Heavy metals contents in nasser lake and the nile river, Egypt: An overview. Egypt. J. Aquatic Res. 45, 301–312. doi:10.1016/j.ejar.2019.12.002
Gopal, V., Krishnakumar, S., Peter, T. S., Nethaji, S., Kumar, K. S., Jayaprakash, M., et al. (2016). Assessment of trace element accumulation in surface sediments off Chennai coast after a major flood event. Mar. Poll. Bull. 114 (02), 1063–1071. doi:10.1016/j.marpolbul.2016.10.019
Gopinathan, P., Ashok, K. S., Pradeep, K. S., and Madhu, J. (2022a). Sulphur in Jharia and Raniganj coalfields: Chemical fractionation and its environmental implications. Environ. Res. 204, 112382. doi:10.1016/j.envres.2021.112382
Gopinathan, P., Madhu, J., Ashok, K. S., Mahato, A., Subramani, T., Pradeep, K. S., et al. (2022b). Geochemical characteristics, origin and forms of sulphur distribution in the Talcher coalfield, India. Fuel 316, 123376. doi:10.1016/j.fuel.2022.123376
Gopinathan, P., Santosh, M. S., Dileepkumar, V. G., Subramani, T., Roopa, R., Masto, R. E., et al. (2022c). Geochemical, mineralogical and toxicological characteristics of coal fly ash and its environmental impacts. Chemosphere 307, 135710. doi:10.1016/j.chemosphere.2022.135710
Gulgundi, M. S., and Shetty, A. (2018). Groundwater quality assessment of urban Bengaluru using multivariate statistical techniques. Appl. Water Sci. 8, 43. doi:10.1007/s13201-018-0684-z
Hakanson, L. (1980). An ecological risk index for aquatic pollution control. A sedimentological approach. Water Res. 14 (8), 975–1001. doi:10.1016/0043-1354(80)90143-8
Harikrishnan, N., Ravisankar, R., Chandrasekaran, A., Gandhi, M. S., Kanagasabapathy, K. V., Prasad, M. V. R., et al. (2017). Assessment of heavy metal contamination in marine sediments of east coast of Tamil nadu affected by different pollution sources. Mar. Pollut. Bull. 121, 418–424. doi:10.1016/j.marpolbul.2017.05.047
Hong, G. H., Chitsan, L., Huu, T. T., Chow, F. C., Xuan, T. B., Nicholas, K. C., et al. (2020). Heavy metal contamination trends in surface water and sediments of a river in a highly industrialized region. Environ. Technol. Innovation 20, 101043. doi:10.1016/j.eti.2020.101043
Iqbal, J., Saleem, M., and Shah, M. H. (2016). Spatial distribution, environmental assessment and source identification of metals content in surface sediments of freshwater reservoir, Pakistan. Geochemistry 76 (01), 171–177. doi:10.1016/j.chemer.2016.02.002
Jaishankar, M., Tseten, T., Anbalagan, N., Mathew, B. B., and Beeregowda, K. N. (2014). Toxicity, mechanism and health effects of some heavy metals. Interdiscip. Toxicol. 7 (2), 60–72. doi:10.2478/intox-2014-0009
João, P. V., Artur, J. M. V., and Luisa, D. (2019). Assessment of heavy metal pollution from anthropogenic activities and remediation strategies: A review. J. Environ. Manag. 246, 101–118. doi:10.1016/j.jenvman.2019.05.126
Karim, A., and Veizer, J. (2000). Weathering processes in the Indus River Basin: Implications from riverine carbon, sulfur, oxygen, and strontium isotopes. Chem. Geol. 170 (1-4), 153–177. doi:10.1016/S0009-2541(99)00246-6
Karunanidhi, D., Aravinthasamy, P., Subramani, T., and Kumar, M. (2021b). Human health risks associated with multipath exposure of groundwater nitrate and environmental friendly actions for quality improvement and sustainable management: A case study from texvalley (tiruppur region) of India. Chemosphere 265, 129083. doi:10.1016/j.chemosphere.2020.129083
Karunanidhi, D., Aravinthasamy, P., Deepali, M., Subramani, T., and Shankar, K. (2021c). Groundwater pollution and human health risks in an industrialized region of southern India: Impacts of the COVID-19 lockdown and the monsoon seasonal cycles. Arch. Environ. Contam. 80, 259–276. doi:10.1007/s00244-020-00797-w
Karunanidhi, D., Aravinthasamy, P., Subramani, T., Chandrajith, R., Janardhana Raju, N., and Antunes, I. M. H. R. (2022). Provincial and seasonal influences on heavy metals in the Noyyal River of South India and their human health hazards. Environ. Res. 204, 111998. doi:10.1016/j.envres.2021.111998
Karunanidhi, D., Aravinthasamy, P., Subramani, T., Kumar, D., and Venkatesan, G. (2021d). Chromium contamination in groundwater and Sobol sensitivity model based human health risk evaluation from leather tanning industrial region of South India. Environ. Res. 199, 111238. doi:10.1016/j.envres.2021.111238
Karunanidhi, D., Aravinthasamy, P., Subramani, T., and Setia, R. (2021a). Effects of COVID-19 pandemic lockdown on microbial and metals contaminations in a part of thirumanimuthar river, south India: A comparative health hazard perspective. Hazard. Mater. 416, 125909. doi:10.1016/j.jhazmat.2021.125909
Ke, X., Gui, S., Huang, H., Zhang, H., Wang, C., and Guo, W. (2017). Ecological risk assessment and source identification for heavy metals in surface sediment from the Liaohe River protected area, China. Chemosphere 175, 473–481. doi:10.1016/j.chemosphere.2017.02.029
Lanzerstorfer, C. (2018). Heavy metals in the finest size fractions of road deposited sediments. Environ. Pollut. 239, 522–531. doi:10.1016/j.envpol.2018.04.063
Li, D., Yu, R., Chen, J., Leng, X., Zhao, D., Jia, H., et al. (2022). Ecological risk of heavy metals in lake sediments of China: A national-scale integrated analysis. J. Clean. Prod. 334, 130206. doi:10.1016/j.jclepro.2021.130206
Li, F. L., Liu, C. Q., Yang, Y. G., Bi, X. Y., Liu, T. Z., and Zhao, Z. Q. (2012). Natural and anthropogenic lead in soils and vegetables around guiyang city, southwest China: A Pb isotopic approach. Sci. Total Environ. 431, 339–347. doi:10.1016/j.scitotenv.2012.05.040
Li, L. M., Wu, J., Lu, J., Li, K. X., Zhang, X. Y., Min, X. Y., et al. (2022). Water quality evaluation and ecological-health risk assessment on trace elements in surface water of the northeastern Qinghai-Tibet Plateau. Ecotoxicol. Environ. Saf. 241, 113775. doi:10.1016/j.ecoenv.2022.113775
Li, L. M., Wu, J., Lu, J., Min, X. Y., Xu, J., and Yang, L. (2018). Distribution, pollution, bioaccumulation, and ecological risks of trace elements in soils of the northeastern Qinghai-Tibet Plateau. Ecotoxicol. Environ. Saf. 166, 345–353. doi:10.1016/j.ecoenv.2018.09.110
Li, L., Wu, J., Lu, J., and Xu, J. (2020a). Speciation, risks and isotope-based source apportionment of trace elements in soils of the northeastern Qinghai-Tibet Plateau. Geochem Explor, Environ. Anal. 20, 315–322. doi:10.1144/geochem2019-042
Li, L., Wu, J., Lu, J., and Xu, J. (2020b). Trace elements in Gobi soils of the northeastern Qinghai-Tibet Plateau. Chem. Ecol. 36 (10), 967–981. doi:10.1080/02757540.2020.1817403
Liu, Q. Q., Wang, F. F., Meng, F. P., Jiang, L., Li, G. J., and Zhou, R. G. (2018a). Assessment of metal contamination in estuarine surface sediments from Dongying City, China: Use of a modified ecological risk index. Mar. Pollut. Bull. 126, 293–303. doi:10.1016/j.marpolbul.2017.11.017
Liu, W., Xie, C., Li, R., Liu, G., Wang, W., Liu, H., et al. (2021). Rapid expansion of lakes in the endorheic basin on the Qinghai-Tibet Plateau since 2000 and its potential drivers. Catena 197, 104942. doi:10.1016/j.catena.2020.104942
Liu, Y., Cheng, Y., Li, F. X., Zhang, C. P., Liu, J. W., Zhang, L., et al. (2012). Pollution evaluation of vertical sediments in the Yalu River Estuary over the past century. Res. Environ. Sci. 25 (5), 489–494.
Liu, Y. F., Huang, H. H., Sun, T., Yuan, Y., Pan, Y., Xie, Y. J., et al. (2018b). Comprehensive risk assessment and source apportionment of heavy metal contamination in the surface sediment of the Yangtze River Anqing section, China. Environ. Earth Sci. 77, 493. doi:10.1007/s12665-018-7621-1
Liu, Y. X., Wang, Q., Zhuang, W., Yuan, Y. L., Yuan, Y. N., Jiao, K. Q., et al. (2017). Calculation of thallium’s toxicity coefficient in the evaluation of potential ecological risk index: A case study. Chemosphere 194, 562–569. doi:10.1016/j.chemosphere.2017.12.002
Lough, G. C., Schauer, J. J., Park, J. S., Shafer, M. M., Deminter, J. T., and Weinstein, J. P. (2005). Emissions of metals associated with motor vehicle roadways. Environ. Sci. Technol. 39 (3), 826–836. doi:10.1021/es048715f
Lu, J., Lin, Y., Wu, J., and Zhang, C. (2021). Continental-scale spatial distribution, sources, and health risks of heavy metals in seafood: Challenge for the water-food-energy nexus sustainability in coastal regions. Environ. Sci. Pollut. Res. 28, 63815–63828. doi:10.1007/s11356-020-11904-8
Lu, J., Wu, J., and Wang, J. H. (2022a). Metagenomic analysis on resistance genes in water and microplastics from a mariculture system. Front. Environ. Sci. Eng. 16, 4. doi:10.1007/s11783-021-1438-y
Lu, J., Zhang, Y. X., Wu, J., and Wang, J. H. (2022b). Intervention of antimicrobial peptide usage on antimicrobial resistance in aquaculture. J. Hazard Mater 427, 128154. doi:10.1016/j.jhazmat.2021.128154
Luo, W., Lu, Y., Giesy, J. P., Wang, T., Shi, Y., Wang, G., et al. (2007). Effects of land use on concentrations of metals in surface soils and ecological risk around Guanting Reservoir, China. Environ. Geochem Health 29, 459–471. doi:10.1007/s10653-007-9115-z
Mahmudul, H., Mahfujur, R., Alif, A., Md, Atikul, I., and Mahfuzur, R. (2022). Heavy metal pollution and ecological risk assessment in the surface water from a marine protected area, Swatch of No Ground, north-western part of the Bay of Bengal. Reg. Stud. Mar. Sci. 52, 102278. doi:10.1016/j.rsma.2022.102278
McComb, J. Q., Han, F. X., Rogers, C., Thomas, C., Arslan, Z., Ardeshir, A., et al. (2015). Trace elements and heavy metals in the grand bay national estuarine reserve in the northern gulf of Mexico. Mar. Pollut. Bull. 99, 61–69. doi:10.1016/j.marpolbul.2015.07.062
Meghdad, P., Nazir, F., Kiomars, S., Razieh, K., and Zahra, A. (2016). Essential and toxic heavy metals in cereals and agricultural products marketed in Kermanshah, Iran, and human health risk assessment. Food Addit. Contam. Part B 9 (1), 15–20. doi:10.1080/19393210.2015.1099570
Mehdi, Q., Mahmoud, S., Seyed, A. S., Mansoureh, F., Saeed, E., Mahmood, Y., et al. (2019). Cadmium in groundwater consumed in the rural areas of Gonabad and Bajestan, Iran: occurrence and health risk assessment. Biol. Trace Elem. Res. 192 (2), 106–115. doi:10.1007/s12011-019-1660-7
Ministry of Health (2006). Standards for drinking water quality. GB57492006. Beijing: Ministry of Health of the People’s Republic of China.
Nethaji, S., Kalaivanan, R., Arya, V., and Jayaprakash, M. (2017). Geochemical assessment of heavy metals pollution in surface sediments of vellar and coleroon estuaries, southeast coast of India. Mar. Pollut. Bull. 115 (1-2), 469–479. doi:10.1016/j.marpolbul.2016.11.045
Özgür, C., Memet, V., Özlem, Ö. O., Kürsad, K. E., and Metin, Ç. (2020). A comparison of trace element concentrations in surface and deep water of the Keban Dam Lake (Turkey) and associated health risk assessment. Environ. Res. 190, 110012. doi:10.1016/j.envres.2020.110012
Pourkhabbaz, A., and Pourkhabbaz, H. (2012). Investigation of toxic metals in the tobacco of different Iranian cigarette brands and related health issues. Iran. J. Basic Med. Sci. 15, 636–644.
Qiao, J., Zhu, Y., Jia, X., Shao, M., Niu, X., and Liu, J. (2020). Distributions of arsenic and other heavy metals, and health risk assessments for groundwater in the Guanzhong Plain region of China. Environ. Res. 181, 108957. doi:10.1016/j.envres.2019.108957
Qu, M., Wang, Y., Huang, B., and Zhao, Y. (2018). Source apportionment of soil heavy metals using robust absolute principal component scores-robust geographically weighted regression (RAPCS-RGWR) receptor model. Sci. Total Environ. 626, 203–210. doi:10.1016/j.scitotenv.2018.01.070
Ravindra, K., Thind, P. S., Mor, S., Singh, T., and Mor, S. (2019). Evaluation of groundwater contamination in chandigarh: Source identification and health risk assessment. Environ. Pollut. 255, 113062. doi:10.1016/j.envpol.2019.113062
Rzetala, M. A. (2016). Cadmium contamination of sediments in the water reservoirs in Silesian Upland (southern Poland). J. Soils Sediments 16, 2458–2470. doi:10.1007/s11368-016-1477-3
Saleem, M., Iqbal, J., and Shah, M. H. (2015). Geochemical speciation, anthropogenic contamination, risk assessment and source identification of selected metals in freshwater sediments a case study from Mangla Lake, Pakistan. Environ. Nanotechnol. Monit. Manag. 4, 27–36. doi:10.1016/j.enmm.2015.02.002
Sener, S., Sener, E., and Davraz, A. (2017). Evaluation of water quality using water quality index (WQI) method and GIS in Aksu River (SW-Turkey). Sci. Total Environ. 584-585, 131–144. doi:10.1016/j.scitotenv.2017.01.102
Shamuyarira, K. K., and Gumbo, J. R. (2014). Assessment of heavy metals in municipal sewage sludge: A case study of limpopo Province, south Africa. Int. J. Environ. Res. Public Health 11, 2569–2579. doi:10.3390/ijerph110302569
Sharafi, K., Nodehi, R. N., Yunesian, M., Mahvi, A. H., Pirsaheb, M., and Nazmara, S. (2019). Human health risk assessment for some toxic metals in widely consumed rice brands (domestic and imported) in tehran, Iran: Uncertainty and sensitivity analysis. Food Chem. 277, 145–155. doi:10.1016/j.foodchem.2018.10.090
Sharma, R. K., and Agrawal, M. (2005). Biological effects of heavy metals: An overview. J. Environ. Biol. 26, 301–313.
Sheng, Y. (2015). Physical and chemical characteristics of dust aerosol from Golmud and its source analysis. Master. China: Nanjing Normal University.
Singh, K. P., Mohan, D., Singh, V. K., and Malik, A. (2005). Studies on distribution and fractionation of heavy metals in Gomti river sediments-A tributary of the Ganges, India. J. Hydrol. 312, 14–27. doi:10.1016/j.jhydrol.2005.01.021
Singh, V. B., Ramanathan, A., Pottakkal, J. G., and Kumar, M. (2014). Seasonal variation of the solute and suspended sediment load in Gangotri glacier meltwater, central Himalaya, India. J. Asian Earth Sci. 79, 224–234. doi:10.1016/j.jseaes.2013.09.010
Song, W. J., Yang, S. Z., and Mu, B. Z. (2014). Determination methods for rubidium and cesium in salt lake brine. Inorg. Chem. Ind. 46 (11), 55–58.
Sun, X., Fan, D., Liu, M., Tian, Y., Pang, Y., and Liao, H. J. (2018). Source identification, geochemical normalization and influence factors of heavy metals in Yangtze River Estuary sediment. Environ. Pollut. 241, 938–949. doi:10.1016/j.envpol.2018.05.050
Suresh, G., Sutharsan, P., Ramasamy, V., and Venkatachalapathy, R. (2012). Assessment of spatial distribution and potential ecological risk of the heavy metals in relation to granulometric contents of Veeranam Lake sediments, India. Ecotoxicol. Environ. Saf. 84, 117–124. doi:10.1016/j.ecoenv.2012.06.027
Tang, W. Z., Shan, B. Q., Zhang, H., Zhang, W. Q., Zhao, Y., Ding, Y. K., et al. (2014). Heavy metal contamination in the surface sediments of representative limnetic ecosystems in eastern China. Sci. Rep. 4 (7152), 7152–7157. doi:10.1038/srep07152
Taylor, S. R., and Mclennan, S. M. (1985). The continental crust: Its composition and evolution. London: Blackwell.
Tchounwou, P. B., Yedjou, C. G., Patlolla, A. K., and Sutton, D. J. (2012). Heavy metal toxicity and the environment. Mol. Clin. Environ. Toxicol. 101, 133–164. doi:10.1007/978-3-7643-8340-4_6
Tiwari, T. N., and Mishra, M. (1985). A preliminary assignment of water quality index of major Indian rivers. Indian J. Environ. Prot. 5 (4), 276–279.
Tomlinson, D. L., Wilson, J. G., Harris, C. R., and Jeffrey, D. W. (1980). Problems in the assessment of heavy-metal levels in estuaries and the formation of a pollution index. Helgol Meeresunters 33 (1-4), 566–575. doi:10.1007/bf02414780
USEPA,1991. Human health evaluation manual, supplemental guidance:“Standard default exposure factors”. 6–03. OSWER Directive 9285.
USEPA, 2004. Risk assessment guidance for superfund volume I: Human health evaluation manual (Part E, supplemental guidance for dermal risk assessment) final. OSWER 9285.7-02EP, July 2004.
Ustaoglu, F., Tepe, Y., and Tas, B. (2020). Assessment of stream quality and health risk in a subtropical Turkey river system: A combined approach using statistical analysis and water quality index. Ecol. Indic. 113, 105815. doi:10.1016/j.ecolind.2019.105815
Vu, C. T., Lin, C., Yeh, G., and Villanueva, M. C. (2017). Bioaccumulation and potential sources of heavy metal contamination in fish species in taiwan: Assessment and possible human health implications. Environ. Sci. Pollut. Res. 24 (23), 19422–19434. doi:10.1007/s11356-017-9590-4
Wang, H., Nie, L., Xu, Y., Du, C., Zhang, T., and Wang, Y. (2018). Effects of highway related pollutant on the groundwater quality of turfy swamps in the Changbai Mountain Area. Int. J. Environ. Res. Public Health. 15, 1652. doi:10.3390/ijerph15081652
Wang, L., Wang, Y., Zhang, W. Z., Xu, C. X., and An, Z. Y. (2014b). Multivariate statistical techniques for evaluating and identifying the environmental significance of heavy metal contamination in sediments of the Yangtze River, China. Environ. Earth Sci. 71 (3), 1183–1193. doi:10.1007/s12665-013-2522-9
Wang, Y. H. (2014a). Geochemistry evolution and water cycle patterns of groundwater in Golmud River basin. Dissertation. China: Chang’an University.
Wang, Y. P., Bai, H. J., Xiao, R., Gao, H. F., Huang, L. B., and Huang, C. (2013). Assessment of heavy metal contamination in the soil-plant system of the Suaeda salsa wetland in the Yellow River Estuary. Acta Ecol. Sin. 33 (10), 3083–3091. doi:10.5846/stxb201202230246
Wang, Z. H., Feng, J., and Nie, X. P. (2015). Recent environmental changes reflected by metals and biogenic elements in sediments from the Guishan Island, the Pearl River Estuary, China. Estuar. Coast Shelf Sci. 164, 493–505. doi:10.1016/j.ecss.2015.08.002
Wei, F. S., Chen, J. S., Wu, Y. Y., Zhen, C. J., Jiang, D. Z., Liu, Z. H., et al. (1990). Chinese soil element background values. China: China Environmental Press.
Wei, X., Han, L. F., Gao, B., Zhou, H. D., Lu, J., and Wan, X. H. (2016). Distribution, bioavailability, and potential risk assessment of the metals in tributary sediments of Three Gorges Reservoir: The impact of water impoundment. Ecol. Indic. 61, 667–675. doi:10.1016/j.ecolind.2015.10.018
Withanachchi, S., Ghambashidze, G., Kunchulia, I., Urushadze, T., and Ploeger, A. (2018). Water quality in surface water: A preliminary assessment of heavy metal contamination of the mashavera river, Georgia. Int. J. Environ. Res. Public Health 15, 621. doi:10.3390/ijerph15040621
Xiang, J. Y. (2020). Source identification, pollution and potential ecological risk assessment of the potentially toxic elements (PTEs) in the area of the Golmud city-golmud river-dabuxun lake. Dissertation. China: University of Chinese Academy of Sciences.
Xiao, H. F., Zhang, S. Y., Guan, Y., Liu, S. J., Gao, Y., Sun, Q. Z., et al. (2014). Assessment of potential risks associated with heavy metal contamination in sediment in Aobaopao Lake, China, determined from sediment cores. Ecotoxicology 23, 527–537. doi:10.1007/s10646-014-1220-z
Xiao, J., Wang, L., Deng, L., and Jin, Z. (2019). Characteristics, sources, water quality and health risk assessment of trace elements in river water and well water in the Chinese Loess Plateau. Sci. Total Environ. 650 (2), 2004–2012. doi:10.1016/j.scitotenv.2018.09.322
Yang, A., Wang, X. X., Xing, W. C., Hu, J., Liu, X. L., and Li, J. (2020). Source and risk evaluation of heavy metals in surface sediments of rivers, lakes and their surrounding soils in Qinghai Province. J. Tianjin Normal Univ. Nat. Sci. Ed. 40 (06), 44–53. doi:10.19638/j.issn1671-1114.20200608
Yao, Z. G., Bao, Z. Y., and Gao, P. (2006). Environmental assessments of trace metals in sediments from dong ting lake, central China. Jour China Univ. Geo Sci. 17, 310–319. doi:10.1016/S1002-0705(07)60004-1
Yuen, J. Q., Olin, P. H., Lim, H. S., Benner, S. G., Sutherland, R. A., and Ziegler, A. D. (2012). Accumulation of potentially toxic elements in road deposited sediments in residential and light industrial neighborhoods of Singapore. J. Environ. Manag. 101, 151–163. doi:10.1016/j.jenvman.2011.11.017
Zahra, A., Hashmi, M. Z., Malik, R. N., and Ahmed, Z. (2014). Enrichment and geo-accumulation of heavy metals and risk assessment of sediments of the kurang nallah—feeding tributary of the rawal lake reservoir, Pakistan. Sci. Total Environ. 470-471, 925–933. doi:10.1016/j.scitotenv.2013.10.017
Zhang, L. L., and Liu, J. L. (2014). In situ relationships between spatial-temporal variations in potential ecological risk indexes for metals and the short-term effects on periphyton in a macrophyte-dominated lake: A comparison of structural and functional metrics. Ecotoxicology 23 (4), 553–566. doi:10.1007/s10646-014-1175-0
Zhang, X., Hu, B., Wang, P., Chen, J., Yang, L., Xiao, K., et al. (2018). Hydrogeochemical evolution and heavy metal contamination in groundwater of a reclaimed land on Zhoushan Island. Water 10, 316. doi:10.3390/w10030316
Zhao, R., Guan, Q. Y., Luo, H. P., Lin, J. K., Yang, L. Q., Wang, F. F., et al. (2019). Fuzzy synthetic evaluation and health risk assessment quantification of heavy metals in Zhangye agricultural soil from the perspective of sources. Sci. Total Environ. 697 (20), 134126. doi:10.1016/j.scitotenv.2019.134126
Keywords: Golmud River, water quality, surface water and sediment, heavy metals, risk assessment, source
Citation: Cai N, Li L, Zhu H, Chen L, Li S, Meng F and Zhang X (2023) Multiple evaluations, risk assessment, and source identification of heavy metals in surface water and sediment of the Golmud River, northeastern Qinghai-Tibet Plateau, China. Front. Environ. Sci. 10:1095731. doi: 10.3389/fenvs.2022.1095731
Received: 11 November 2022; Accepted: 19 December 2022;
Published: 05 January 2023.
Edited by:
Xiaohu Wen, Northwest Institute of Eco-Environment and Resources (CAS), ChinaReviewed by:
Chengkai Qu, China University of Geosciences Wuhan, ChinaCopyright © 2023 Cai, Li, Zhu, Chen, Li, Meng and Zhang. This is an open-access article distributed under the terms of the Creative Commons Attribution License (CC BY). The use, distribution or reproduction in other forums is permitted, provided the original author(s) and the copyright owner(s) are credited and that the original publication in this journal is cited, in accordance with accepted academic practice. No use, distribution or reproduction is permitted which does not comply with these terms.
*Correspondence: Xiying Zhang, eHl6aGNoaW5hQGlzbC5hYy5jbg==
Disclaimer: All claims expressed in this article are solely those of the authors and do not necessarily represent those of their affiliated organizations, or those of the publisher, the editors and the reviewers. Any product that may be evaluated in this article or claim that may be made by its manufacturer is not guaranteed or endorsed by the publisher.
Research integrity at Frontiers
Learn more about the work of our research integrity team to safeguard the quality of each article we publish.